- 1Rajaie Cardiovascular Medical and Research Center, Iran University of Medical Sciences, Tehran, Iran
- 2Department of Radiology, School of Medicine, Iran University of Medical Sciences, Tehran, Iran
- 3Department of Radiology, Cardiothoracic Imaging, University of Washington, Seattle, WA, United States
Background: Prompt interventions prevent adverse events (AE) in hypertrophic cardiomyopathy (HCM). We evaluated the pattern and the predictive role of feature tracking (FT)-cardiac magnetic resonance (CMR) imaging parameters in an HCM population with a normal left ventricular ejection fraction (LVEF) and a low fibrosis burden.
Methods: The CMR and clinical data of 170 patients, consisting of 142 HCM (45 ± 15.7 years, 62.7% male) and 28 healthy (42.2 ± 11.26 years, 50% male) subjects, who were enrolled from 2015 to 2020, were evaluated. HCM patients had a normal LVEF with a late gadolinium enhancement (LGE) percentage below 15%. Between-group differences were described, and the potent predictors of AE were determined. A P-value below 0.05 was considered significant.
Results: LV global longitudinal, circumferential, and radial strains (GLS, GCS, and GRS, respectively) and the LV myocardial mass index (MMI) were different between the healthy and HCM cases (all Ps < 0.05). Strains were significantly impaired in the HCM patients with a normal MMI. A progressive decrease in LVGLS and a distinct fall in LVGCS were noted with a rise in MMI. AE were predicted by LVGLS, LVGCS, and the LGE percentage, and LVGCS was the single robust predictor (HR, 1.144; 95% CI, 1.080–1.212; P = 0.001). An LVGCS below 16.2% predicted AE with 77% specificity and 58% sensitivity.
Conclusions: LV strains were impaired in HCM patients with a normal EF and a low fibrosis burden, even in the presence of a normal MMI. CMR parameters, especially FT-CMR values, predicted AE in our HCM patients.
Introduction
Hypertrophic cardiomyopathy (HCM) is an inherited disorder characterized by left ventricular (LV) hypertrophy and is unexplainable by other causes (1–3). HCM is the most common monogenic cardiovascular disorder, with an estimated prevalence of 1:250–500 in the adult population, predominantly affecting adolescents and young adults while rare in children (4–6). The LV ejection fraction (EF), as an index of systolic function, often remains within the normal range in HCM despite disease progression (7).
Myocardial fibers are arranged in 3 different orientations as a continuum of 2 helical geometries, helping amplify myocyte contraction and cardiac function as a single unit. This superstructure deteriorates in HCM, resulting in faulty mechanics despite an apparently preserved EF. HCM diagnosis and characterization are based on echocardiography and magnetic resonance imaging (MRI), although cardiac magnetic resonance imaging (CMR) provides better identification and risk stratification by detecting in vivo fibrosis (8).
Several studies have examined imaging features that could better elucidate myocardial abnormalities and patient outcomes (9–12). One of these methods is the myocardial strain analysis by CMR to assess subclinical function impairment. Strains are reported in 3 directions: longitudinal, circumferential, and radial. The longitudinal strain represents subendocardial fiber deformation, while circumferential and radial strains reveal mid-myocardial and subepicardial fiber changes, respectively (13). Multiple parameters can affect LV myocardial strains in patients with HCM, including LVEF, the myocardial mass, the myocardial fibrosis burden, and the left ventricular outflow tract (LVOT) obstruction (14).
In the present study, utilizing the feasible cardiac magnetic resonance feature-tracking (CMR-FT) method, we aimed to define the myocardial strain pattern in HCM patients. Moreover, we investigated the role of CMR parameters in the prognostication of HCM patients with a normal LVEF and a low fibrosis burden.
Methods
The institutional research committee approved this study and waived the need for informed consent due to the retrospective design of this study.
Study population
The current investigation retrospectively enrolled 241 patients with HCM who underwent CMR between 2015 and 2020 in our institution. Additionally, the CMR findings of 28 healthy volunteers were retrieved from the center's normal CMR examination archives. Healthy subjects had a normal physical examination, no personal or family history of cardiac disease, and no cardiovascular risk factors, composed of hypertension, diabetes mellitus, and dyslipidemia.
Diagnostic criteria
All patients with a definite diagnosis of HCM, according to the American heart association/American college of cardiology guidelines for diagnosing and treating patients with hypertrophic cardiomyopathy, were enrolled (15).
The exclusion criteria were an LVEF below 55%, a late gadolinium enhancement (LGE) percentage of more than 15%, hypertension, aortic valve disease, infiltrative heart diseases (e.g., Fabry disease, Danon disease, and cardiac amyloidosis), athlete's heart, ischemic heart disease, significant cardiac arrhythmias during CMR acquisition, and renal impairment (defined as an estimated glomerular filtration rate <30 ml/min precluding gadolinium injection). Also, other types of HCM, such as apical HCM, are excluded from the study. Furthermore, CMR studies that yielded equivocal findings due to suboptimal quality were excluded from the study (Figure 1).
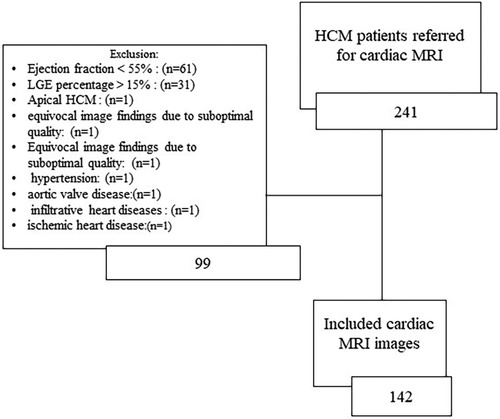
Figure 1. Diagram of the study population. HCM, hypertrophic cardiomyopathy; LGE, late gadolinium enhancement.
Study population classification
The medical records of the patients were reviewed. Then, based on their transthoracic echocardiography (TTE)-measured LVOT gradient, the patients were classified into 2 groups: an LVOT gradient of less than 50 mm Hg and an LVOT gradient of 50 mm Hg or higher. TTE examinations with a maximum interval of 6 months from the CMR examination were selected for analysis. The former group was regarded as a no or mild LVOT obstruction group and the latter as a severe LVOT obstruction group (16, 17).
In another classification, the patients were divided based on their myocardial mass index (MMI) into normal and increased MMI categories. An MMI exceeding 81 g/m2 for females and 85 g/m2 for males was regarded as increased (18).
CMR
CMR images were acquired using a 1.5-T MRI equipment (Siemens Avanto, Erlangen, Germany) with an 8-element phased-array receiver surface coil. A semi-automatic post-processing program (CVi42; Circle Cardiovascular Imaging Inc, Calgary, Canada) was applied for the measurements.
CMR function
Electrocardiography-gated cine steady-state free precession in the 2-, 3-, and 4-chamber views, the right ventricular and LV outflow tracts planes, and a stack of short-axis slices covering LV during breath-hold at end-expiration (slice thickness = 8 mm, the field of view = 300 mm, flip angle = 65°, bandwidth = 925 Hz/Px, imaging matrix = 156 × 192, and repetition time/echo time = 2.7/1.2 ms) were acquired. Parallel imaging was utilized. The endocardial and epicardial borders were manually drawn in short-axis end-diastolic and end-systolic images and propagated throughout all ventricular slices. Functional parameters, composed of the EF, end-diastolic and end-systolic volumes, the LV mass index, the maximal septal thickness, the presence of the systolic anterior motion of the mitral valve, and the ratio of the asymmetric septal hypertrophy, were registered.
CMR-FT
LV end-diastolic and end-systolic frames of 2-, 3- and 4-chamber views and the short-axis plane were selected. Optimal brightness adjustment was done to ensure the best contrast to make accurate discrimination between the endocardium and the blood pool. The endocardial and epicardial contours were defined manually and propagated throughout the slices, and 3D LV strains, consisting of global longitudinal (GLS), global circumferential (GCS), and global radial (GRS) strains, were calculated utilizing the CMR-FT method (Figure 2). The absolute values of strains were utilized for analysis.
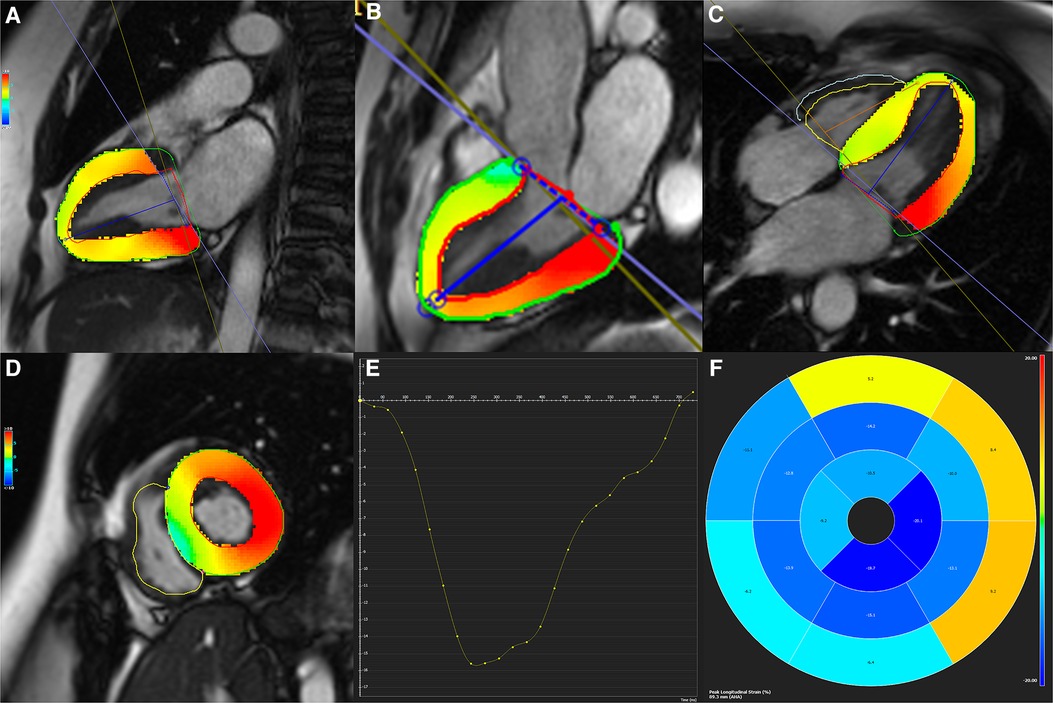
Figure 2. (A–D) Two-, three-, and four-chamber as well as short-axis cine functional sequences with defined endocardial and epicardial contours. (E) Strain curve, and (F) Bull's eye map are depicted for global circumferential and longitudinal strains.
LGE imaging
LGE images were obtained 15 min after the injection of the gadolinium contrast agent, gadotetrate meglumine (Dotarem), applying the phase-sensitive inversion recovery sequence. Breath-held segmented single-shot protocol (slice thickness = 8 mm, the field of view = 320 mm, flip angle = 40°, bandwidth = 1,445 Hz/Px, imaging matrix = 192 × 192, and repetition time/echo time = 2.9/1.1 ms) with selecting the inversion time to null the normal myocardium (typically 200–250 ms) was applied. Considering a 5-standard deviation from the mean myocardial signal intensity, the LGE percentage was measured. The results were assessed visually and modified if needed.
LVOT evaluation
Based on echocardiographic findings obtained from patients' medical records, the patients' LVOT gradients were collected. Gradient measurement was performed based on the modified Bernoulli equation.
On CMR, cine LVOT images in at least 5 consecutive slices were acquired. The presence of the systolic anterior motion of the mitral valve and LVOT turbulence were registered.
Follow-up data
For each patient, we considered at least one follow-up. If a patient had several follow-ups, we considered the last one. A composite of adverse events, consisting of sudden cardiac death, aborted sudden cardiac death (unsuccessful cardiopulmonary resuscitation), implantable cardioverter defibrillator insertion, and deteriorated systolic function (an EF decline to <40%), was considered and registered.
Data collection
CMR measures were registered by two experts with more than five years of expertise (a cardiologist and a radiologist) in cardiovascular imaging. Readers were blind to the study population's data. Interobserver variability was reported, and both examiners' consensus resolved any conflicts.
Echocardiographic, clinical, and follow-up data, including physician visits, lab data, and imaging examinations, were collected by reviewing patients' medical records and/or telephone calls whenever needed.
Statistical analysis
SPSS version 22 (IBM incorporation) was utilized for statistical analysis. Categorical and continuous variables were reported as frequencies (percentages) and mean ± standard deviation (SD), respectively. The Kolmogorov–Smirnov test was utilized to assess the normality of distribution. Between-group comparisons were performed using the t, Mann–Whitney U, analysis of variances (ANOVA), and χ2 tests, whichever was appropriate. The post hoc test of the least significant difference described the pattern of the intergroup changes. Univariate and multivariate Cox regression analyses were applied to evaluate the role of CMR parameters in revealing undesirable outcomes. For the definition of the cutoff point, specificity, and sensitivity of the predictor variables, the receiver operating characteristic (ROC) curve was utilized. Moreover, P-values below 0.1 for the univariate Cox regression analysis and 0.05 for the rest of the tests were considered statistically significant.
Results
Study population characteristics
CMR examinations of 170 patients, consisting of 142 subjects with HCM (mean ± SD age =45 y ± 15.7; 62.7% male) and 28 healthy subjects (mean ± SD age =42.2y ± 11.26; 50% male), were included. Interobserver variability was estimated to be 6.3% and the examiners’ consensus-resolved conflicts.
The mean ± SD of the body surface area was 1.87 m2 ± 0.14, and the mean ± SD of MMI was 59.57 g/m2 ± 8.56 for the healthy subjects. No significant differences were observed in LVEF and end-diastolic and end-systolic volumes between the healthy population and the patients with HCM, whereas significant differences were noted between the 2 groups in LV MMI, LVGLS, LVGCS, and LVGRS (all Ps < 0.05).
HCM patients characteristics in LVOT groups
Table 1 presents the demographic characteristics and the baseline CMR data of the patients with HCM in the two LVOT groups.
The difference in mean strain values was significant between the healthy controls and the HCM group. However, the mean strain values were not different between the 2 LVOT gradient groups (P > 0.05).
CMR-FT parameters in MMI groups
The 1-way ANOVA revealed a significant difference in all 3 strain parameters between the healthy controls and the HCM cases with normal and increased myocardial mass (all Ps < 0.05). Between-group changes were evaluated by applying the post hoc least significant difference test, and the results are depicted in Figure 3.
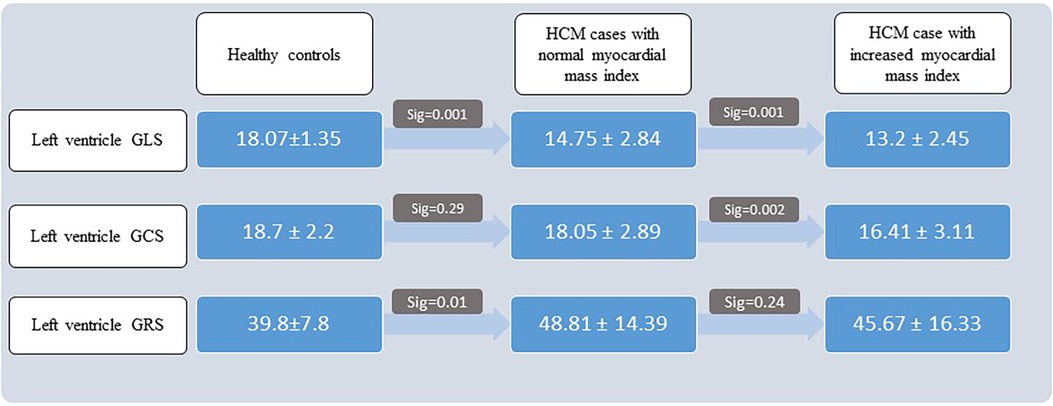
Figure 3. Results of post hoc least significant difference test. HCM, hypertrophic cardiomyopathy; GLS, global longitudinal strain; GCS, global circumferential strain; GRS, global radial strain.
The correlation between strain values and MMI
The Pearson correlation test revealed a moderate inverse linear correlation between MMI and LVGLS and LVGCS (r = −0.4 and r = −0.32, respectively; Ps = 0.001). Scatter plots are demonstrated in Figure 4.
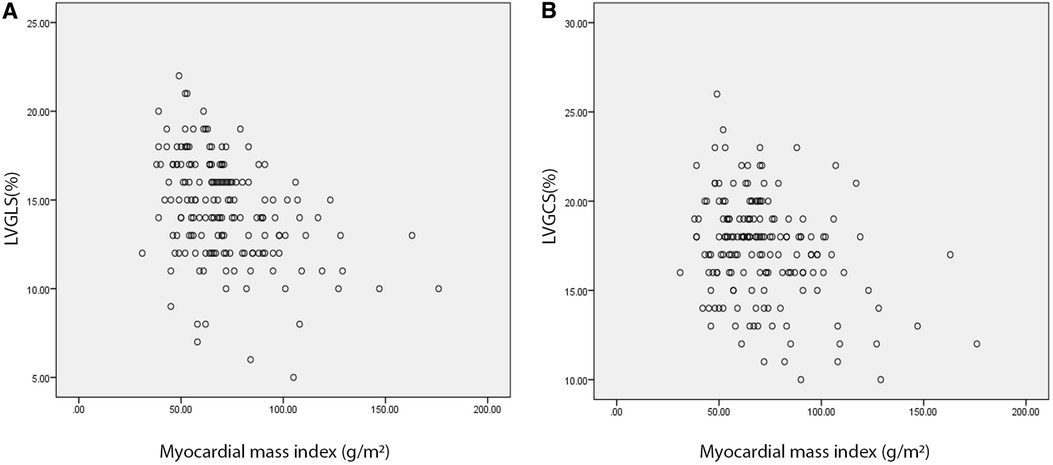
Figure 4. Scatter plots demonstrate a weak inverse correlation of absolute LVGLS (A) and LVGCS (B) values with myocardial mass index. Strain values are revealed on vertical and myocardial mass index on horizontal axes. LVGLS, left ventricle global longitudinal strain; LVGCS, left ventricle global circumferential strain.
Follow-up data
The median (interquartile range) follow-up time was 25 months (23). Twenty-three patients developed adverse events. The results of the independent t-test analysis are depicted in Table 2. It was revealed that absolute values of LVGLS, LVGRS and LVGCS are considerably lesser in patients with adverse events than those without. Also, the myocardial mass index is significantly higher in patients with adverse events compared to others.
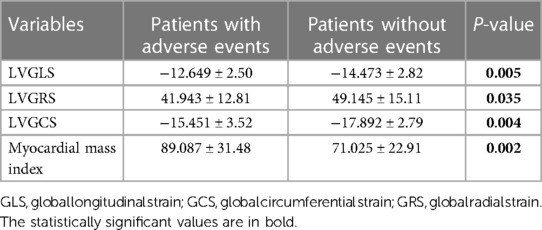
Table 2. Global strains and myocardial mass index comparison among the two groups with and without adverse events.
The results of the Cox regression analyses are depicted in Table 3. Variables with P-values below 0.1, consisting of LVGLS, LVGCS, and the LGE percentage, were entered into the multivariate Cox regression. The results revealed that LVGCS was the single predictor of adverse events (HR, 1.144; 95% CI, 1.080–1.212; P = 0.001).
The ROC analysis determined a cutoff point of 16.2% for LVGCS to predict adverse events with 77% sensitivity and 58% specificity (area under the curve = 0.716; P = 0.001) (Figure 5).
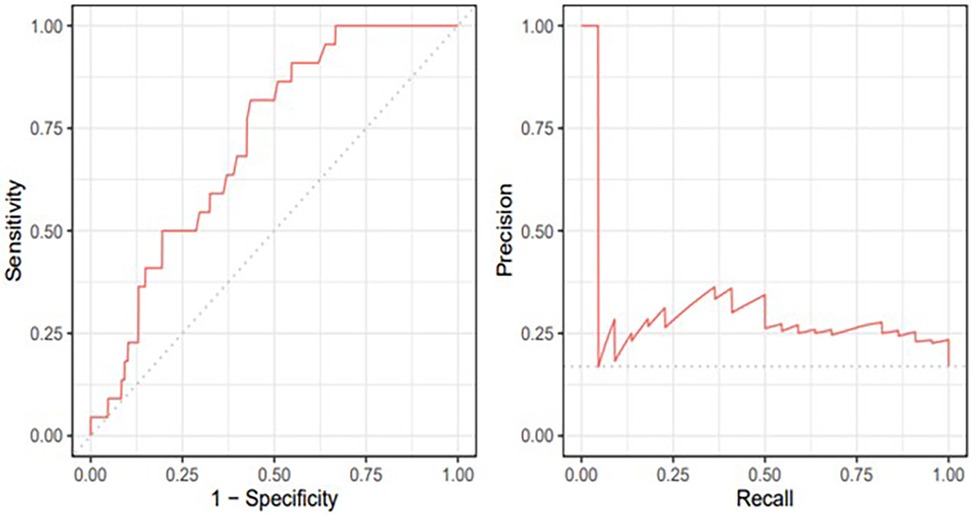
Figure 5. Receiver operating curve and precision-recall plots for left ventricle global circumferential strain.
Discussion
HCM is one of the prevalent causes of sudden cardiac death in young adults, hence the vital significance of its timely diagnosis and appropriate management strategy. In the present study, we investigated the CMR findings of 142 HCM patients with normal LV systolic function and a low myocardial fibrosis burden. Furthermore, we meticulously registered the follow-up data of the study population to determine whether CMR data could predict adverse events. Our principal findings are as follows:
A. The HCM and control groups were not statistically meaningfully different concerning LVEF and end-diastolic and end-systolic volumes, whereas the difference between them in all 3 global strain values and MMI constituted statistical significance.
B. In the HCM group, the severity of LVOT obstruction did not influence strain measurements.
C. LVGLS and LVGRS were impaired in the HCM group even if there was no increase in the myocardial mass. Along with an increase in the myocardial mass, a further decline in the LVGLS and LVGCS was detected.
D. Two of the strain values, LVGLS and LVGCS, and the LV myocardial LGE percentage predicted adverse events, with LVGCS being the single robust predictor.
E. An LVGCS value of 16.2% or less predicted adverse events with 77% sensitivity and 58% specificity.
Our results supported the notion that despite an unimpaired systolic function, abnormal myocardial deformation is present even without significant myocardial fibrosis. A previous study proved that CMR-FT parameters were impaired even in the carriers of HCM without the overt disease (19). Therefore, we suppose that CMR-derived strain values, influenced by myocardial fibers disarray, may reveal subclinical function impairment and assist in prompt cardioprotective treatment.
Multiple factors may affect the measurement of strain values (20–22). According to previous investigations, we assumed that an increased LVOT gradient, indicative of LVOT obstruction, might influence strain parameters (22). Nonetheless, our analysis did not prove this assumption, which may partly be due to our selected HCM population with an unimpaired LVEF and a low fibrosis burden. We believe that further investigations are needed in this regard.
Our study is one of the first investigations to address the mechanical changes secondary to an abnormal MMI. Our selection of HCM patients without systolic dysfunction and with a low fibrosis burden enabled us to evaluate the net effect of the LV myocardial mass to a great extent. We found a decreased LVGLS and an increased LVGRS in our selected HCM patients with normal myocardial mass. On the other hand, when the myocardial mass increases, LVGCS decreases distinctly. We suppose that it could be due to increased myocyte disarrangement in enlarged myocardial mass, which especially results in LVGCS decline. These findings are in agreement with previous studies suggesting that myocardial disarray in patients with HCM is a limiting factor of myocardial compliance and contractile function (23). Nevertheless, the precise myocardial mechanics in different myocardial mass groups have yet to be elucidated.
Remarkably, our results revealed a moderate inverse linear correlation between the net values of LVGLS and LVGCS and MMI. Strain values indicate function, especially in our selected population, who exhibited no decline in EF values. In a previous study, Dohy et al. showed that while there is no linear correlation between LVEF and MMI, LVGLS, as a functional parameter, correlated with MMI (24). Therefore, we suppose that strain values could be sensitive and early indicators of function impairment in HCM patients.
Previous studies have investigated the role of CMR parameters in indicating the outcome of patients with HCM (25–27). Parameters such as the LGE percentage, regional CMR-FT measures, and 3D global strains were reported to be capable of revealing outcomes in HCM subjects. Similarly, we demonstrated that the LGE percentage, LVGLS, and LVGCS were predictors of adverse events in our selected HCM patients. We also found that LVGCS was an independent predictor of adverse events and managed to define a cutoff point of 16.2% with 77% sensitivity and 58% specificity for LVGCS to reveal adverse events. Our findings are in line with previous investigations concluding that an LGE percentage exceeding 15% and an LVGLS decline are valuable in the risk stratification of patients suffering from HCM (28, 29). The discriminative characteristic of our study is that we selected HCM patients with normal systolic function and a low fibrosis burden and demonstrated that even in these benign-appearing clinical phenotypes, we might take advantage of CMR-FT parameters to estimate the probability of future adverse events.Morever, we utilized the CMR-FT method to determine strain values. This method is now established to have excellent reproducibility and agreement with the known MRI tagging and fast strain-encoded CMR imaging techniques. In contrast to other methods, CMR-FT does not require additional sequences and is, thus, more feasible in clinical practice (30).
In the present study, we observed that patients with a positive family history were more likely to show an increased LVOT gradient. It is noteworthy that 60% of HCM patients with a positive family history have at least one of the eleven known sarcomere protein-encoding genes mutations. However, only 30% of HCM patients without positive family history have them (31). These genetic differences lead to significant phenotypic diversity in HCM, affecting various heart structures (32). Our finding confirms the genetic role in different HCM phenotypic subgroups.
Limitations
Despite its remarkable findings, the present investigation has some limitations. Firstly, the retrospective design of this study limited the data to available medical records and CMR images. Prospective studies with precise predefined clinical variables and CMR protocols may provide more reliable findings. For example, although the gold standard for LVOT gradient measurement is cardiac catheterization, we could not find the catheterization data in the medical records. Doppler echocardiography at rest and with provocative maneuvers were employed to estimate the highest LVOT gradient in our cases. However, previous studies' report of a good correlation between Doppler echocardiography estimations and cardiac catheterization results, convinced us to a great extent (33). Additionally, the use of novel CMR methods, including mapping techniques and 4D flow measurements, is warranted in the assessment of patients with HCM. Secondly, our study had a limited number of patients in each subgroup. Further large-scale multicentric investigations may provide more robust results in subgroup analysis. Finally, the number of the healthy population was a limitation in our investigation. We believe that more reliable results could be obtained by increasing age- and sex-matched healthy control subjects in future studies.
Conclusions
Cardiac MRI-derived strain measures are valuable in revealing subclinical functional alterations in HCM patients with unimpaired EF values and low fibrosis burdens. Furthermore, CMR-FT measures, distinctly LVGCS, are powerful predictors of the outcome in this patient population. It is noteworthy that the CMR-FT method can explain alterations in LV mechanics in different myocardial mass measures.
Data availability statement
The raw data supporting the conclusions of this article will be made available by the authors, without undue reservation.
Ethics statement
The studies involving humans were approved by Rajaie Cardiovascular Medical and Research Center, Iran University of Medical Sciences, Tehran, Iran. The studies were conducted in accordance with the local legislation and institutional requirements. Written informed consent for participation was not required from the participants or the participants’ legal guardians/next of kin in accordance with the national legislation and institutional requirements.
Author contributions
SAs: Conceptualization, Methodology, Investigation, Writing. AG: Software, Validation, Formal Analysis, Investigation. AS: Software, Validation, Formal Analysis, Investigation. NS: Data curation. NR: Writing – original draft. HC: Writing. SM: Visualization. SAd: Supervision.
Funding
The author(s) declare that no financial support was received for the research, authorship, and/or publication of this article.
Conflict of interest
The authors declare that the research was conducted in the absence of any commercial or financial relationships that could be construed as a potential conflict of interest.
Publisher's note
All claims expressed in this article are solely those of the authors and do not necessarily represent those of their affiliated organizations, or those of the publisher, the editors and the reviewers. Any product that may be evaluated in this article, or claim that may be made by its manufacturer, is not guaranteed or endorsed by the publisher.
References
1. Elliott PM, Anastasakis A, Borger MA, Borggrefe M, Cecchi F, Charron P, et al. 2014 ESC guidelines on diagnosis and management of hypertrophic cardiomyopathy. Kardiol Pol. (2014) 72(11):1054–126. doi: 10.5603/KP.2014.0212
2. Gersh BJ, Maron BJ, Bonow RO, Dearani JA, Fifer MA, Link MS, et al. 2011 ACCF/AHA guideline for the diagnosis and treatment of hypertrophic cardiomyopathy: executive summary: a report of the American college of cardiology foundation/American heart association task force on practice guidelines. Circulation. (2011) 124(24):2761–96. doi: 10.1161/CIR.0b013e318223e230
3. Marian AJ, Braunwald E. Hypertrophic cardiomyopathy: genetics, pathogenesis, clinical manifestations, diagnosis, and therapy. Circ Res. (2017) 121(7):749–70. doi: 10.1161/CIRCRESAHA.117.311059
4. Maron BJ. Clinical course and management of hypertrophic cardiomyopathy. N Engl J Med. (2018) 379(7):655–68. doi: 10.1056/NEJMra1710575
5. McKenna WJ, Maron BJ, Thiene G. Classification, epidemiology, and global burden of cardiomyopathies. Circ Res. (2017) 121(7):722–30. doi: 10.1161/CIRCRESAHA.117.309711
6. Semsarian C, Ingles J, Maron MS, Maron BJ. New perspectives on the prevalence of hypertrophic cardiomyopathy. J Am Coll Cardiol. (2015) 65(12):1249–54. doi: 10.1016/j.jacc.2015.01.019
7. Marstrand P, Han L, Day SM, Olivotto I, Ashley EA, Michels M, et al. Hypertrophic cardiomyopathy with left ventricular systolic dysfunction: insights from the SHaRe registry. Circulation. (2020) 141(17):1371–83. doi: 10.1161/CIRCULATIONAHA.119.044366
8. He D, Ye M, Zhang L, Jiang B. Prognostic significance of late gadolinium enhancement on cardiac magnetic resonance in patients with hypertrophic cardiomyopathy. Heart Lung. (2018) 47(2):122–6. doi: 10.1016/j.hrtlng.2017.10.008
9. Cavus E, Muellerleile K, Schellert S, Schneider J, Tahir E, Chevalier C, et al. CMR Feature tracking strain patterns and their association with circulating cardiac biomarkers in patients with hypertrophic cardiomyopathy. Clin Res Cardiol. (2021) 110:1–13. doi: 10.1007/s00392-021-01848-5
10. Kinno M, Nagpal P, Horgan S, Waller AH. Comparison of echocardiography, cardiac magnetic resonance, and computed tomographic imaging for the evaluation of left ventricular myocardial function: part 2 (diastolic and regional assessment). Curr Cardiol Rep. (2017) 19:1–13. doi: 10.1007/s11886-017-0817-2
11. Li X, Shi K, Yang Z-g, Guo Y-k, Huang S, Xia C-c, et al. Assessing right ventricular deformation in hypertrophic cardiomyopathy patients with preserved right ventricular ejection fraction: a 3.0-T cardiovascular magnetic resonance study. Sci Rep. (2020) 10(1):1967. doi: 10.1038/s41598-020-58775-0
12. Shimada YJ, Hoeger CW, Latif F, Takayama H, Ginns J, Maurer MS. Myocardial contraction fraction predicts cardiovascular events in patients with hypertrophic cardiomyopathy and normal ejection fraction. J Card Fail. (2019) 25(6):450–6. doi: 10.1016/j.cardfail.2019.03.016
13. Bansal M, Sengupta PP. Longitudinal and circumferential strain in patients with regional LV dysfunction. Curr Cardiol Rep. (2013) 15:1–14. doi: 10.1007/s11886-012-0339-x
14. Kovacheva E, Gerach T, Schuler S, Ochs M, Dössel O, Loewe A. Causes of altered ventricular mechanics in hypertrophic cardiomyopathy: an in-silico study. Biomed Eng Online. (2021) 20(1):1–28. doi: 10.1186/s12938-021-00900-9
15. Cardiology ACo, Cardiology ACo, Association AH. 2020 AHA/ACC guideline for the diagnosis and treatment of patients with hypertrophic cardiomyopathy A report of the American college of cardiology/American heart association joint committee on clinical practice guidelines. Circulation. (2020) 142(25):E558–631. doi: 10.1161/CIR.0000000000000937
16. Maron MS, Olivotto I, Betocchi S, Casey SA, Lesser JR, Losi MA, et al. Effect of left ventricular outflow tract obstruction on clinical outcome in hypertrophic cardiomyopathy. N Engl J Med. (2003) 348(4):295–303. doi: 10.1056/NEJMoa021332
17. Maron MS, Olivotto I, Zenovich AG, Link MS, Pandian NG, Kuvin JT, et al. Hypertrophic cardiomyopathy is predominantly a disease of left ventricular outflow tract obstruction. Circulation. (2006) 114(21):2232–9. doi: 10.1161/CIRCULATIONAHA.106.644682
18. Petersen SE, Khanji MY, Plein S, Lancellotti P, Bucciarelli-Ducci C. European Association of cardiovascular imaging expert consensus paper: a comprehensive review of cardiovascular magnetic resonance normal values of cardiac chamber size and aortic root in adults and recommendations for grading severity. Eur Heart J Cardiovasc Imaging. (2019) 20(12):1321–31. doi: 10.1093/ehjci/jez232
19. Vigneault DM, Yang E, Jensen PJ, Tee MW, Farhad H, Chu L, et al. Left ventricular strain is abnormal in preclinical and overt hypertrophic cardiomyopathy: cardiac MR feature tracking. Radiology. (2019) 290(3):640–8. doi: 10.1148/radiol.2018180339
20. Sharifian M, Rezaeian N, Asadian S, Mohammadzadeh A, Nahardani A, Kasani K, et al. Efficacy of novel noncontrast cardiac magnetic resonance methods in indicating fibrosis in hypertrophic cardiomyopathy. Cardiol Res Pract. (2021) 2021:1–7. doi: 10.1155/2021/9931136
21. Rezaeian N, Hosseini L, Omidi N, Khaki M, Najafi H, Kasani K, et al. Feature-tracking cardiac magnetic resonance method: a valuable marker of replacement fibrosis in hypertrophic cardiomyopathy. Pol J Radiol. (2022) 87(1):263–70. doi: 10.5114/pjr.2022.116548
22. She Jq, Guo Jj, Yu Yf, Zhao Sh, Chen Yy, Ge My, et al. Left ventricular outflow tract obstruction in hypertrophic cardiomyopathy: the utility of myocardial strain based on cardiac MR tissue tracking. J Magn Reson Imaging. (2021) 53(1):51–60. doi: 10.1002/jmri.27307
23. Tseng WYI, Dou J, Reese TG, Wedeen VJ. Imaging myocardial fiber disarray and intramural strain hypokinesis in hypertrophic cardiomyopathy with MRI. J Magn Reson Imaging. (2006) 23(1):1–8. doi: 10.1002/jmri.20473
24. Dohy Z, Szabo L, Toth A, Czimbalmos C, Horvath R, Horvath V, et al. Prognostic significance of cardiac magnetic resonance-based markers in patients with hypertrophic cardiomyopathy. Int J Cardiovasc Imaging. (2021) 37:2027–36. doi: 10.1007/s10554-021-02165-8
25. Chen X, Pan J, Shu J, Zhang X, Ye L, Chen L, et al. Prognostic value of regional strain by cardiovascular magnetic resonance feature tracking in hypertrophic cardiomyopathy. Quant Imaging Med Surg. (2022) 12(1):627. doi: 10.21037/qims-21-42
26. O'Hanlon R, Grasso A, Roughton M, Moon JC, Clark S, Wage R, et al. Prognostic significance of myocardial fibrosis in hypertrophic cardiomyopathy. J Am Coll Cardiol. (2010) 56(11):867–74. doi: 10.1016/j.jacc.2010.05.010
27. Smith BM, Dorfman AL, Yu S, Russell MW, Agarwal PP, Mahani MG, et al. Relation of strain by feature tracking and clinical outcome in children, adolescents, and young adults with hypertrophic cardiomyopathy. Am J Cardiol. (2014) 114(8):1275–80. doi: 10.1016/j.amjcard.2014.07.051
28. Mentias A, Raeisi-Giglou P, Smedira NG, Feng K, Sato K, Wazni O, et al. Late gadolinium enhancement in patients with hypertrophic cardiomyopathy and preserved systolic function. J Am Coll Cardiol. (2018) 72(8):857–70. doi: 10.1016/j.jacc.2018.05.060
29. Makavos G, Kairis C, Tselegkidi ME, Karamitsos T, Rigopoulos AG, Noutsias M, et al. Hypertrophic cardiomyopathy: an updated review on diagnosis, prognosis, and treatment. Heart Fail Rev. (2019) 24:439–59. doi: 10.1007/s10741-019-09775-4
30. Bucius P, Erley J, Tanacli R, Zieschang V, Giusca S, Korosoglou G, et al. Comparison of feature tracking, fast-SENC, and myocardial tagging for global and segmental left ventricular strain. ESC Heart Fail. (2020) 7(2):523–32. doi: 10.1002/ehf2.12576
31. Gersh BJ, Maron BJ, Bonow RO, Dearani JA, Fifer MA, Link MS, et al. 2011 ACCF/AHA guideline for the diagnosis and treatment of hypertrophic cardiomyopathy: a report of the American college of cardiology foundation/American heart association task force on practice guidelines. Circulation. (2011) 124(24):e783–831. doi: 10.1161/CIR.0b013e318223e2bd
32. Baxi AJ, Restrepo CS, Vargas D, Marmol-Velez A, Ocazionez D, Murillo H. Hypertrophic cardiomyopathy from A to Z: genetics, pathophysiology, imaging, and management. Radiographics. (2016) 36(2):335–54. doi: 10.1148/rg.2016150137
Keywords: hypertrophic cardiomyopathy (HCM), cardiac MRI, feature tracking, cardiac function, adverse events
Citation: Salmanipour A, Ghaffari Jolfayi A, Sabet Khadem N, Rezaeian N, Chalian H, Mazloomzadeh S, Adimi S and Asadian S (2023) The predictive value of cardiac MRI strain parameters in hypertrophic cardiomyopathy patients with preserved left ventricular ejection fraction and a low fibrosis burden: a retrospective cohort study. Front. Cardiovasc. Med. 10:1246759. doi: 10.3389/fcvm.2023.1246759
Received: 10 July 2023; Accepted: 28 August 2023;
Published: 13 September 2023.
Edited by:
Arunark Kolipaka, The Ohio State University, United StatesReviewed by:
Juliet Varghese, The Ohio State University, United StatesYu Ding, The Ohio State University, United States
© 2023 Salmanipour, Ghaffari Jolfayi, Sabet Khadem, Rezaeian, Chalian, Mazloomzadeh, Adimi and Asadian. This is an open-access article distributed under the terms of the Creative Commons Attribution License (CC BY). The use, distribution or reproduction in other forums is permitted, provided the original author(s) and the copyright owner(s) are credited and that the original publication in this journal is cited, in accordance with accepted academic practice. No use, distribution or reproduction is permitted which does not comply with these terms.
*Correspondence: Sanaz Asadian YXNhZGlhbl9zQHlhaG9vLmNvbQ==
†These authors have contributed equally to this work and share first authorship