- 1Department of Geography and Environmental Studies, Wolaita Sodo University, Wolaita Sodo, Ethiopia
- 2College of Developmental Studies, Addis Ababa University, Addis Ababa, Ethiopia
This study investigates the trends of climate change and evaluates the effects of climate-smart agricultural (CSA) practices in Humbo Woreda, Wolaita Zone, Ethiopia. The findings revealed a significant increase in temperature and irregular rainfall patterns, which adversely affected agricultural productivity. This research employed a mixed-method approach, gathering data from household surveys, focus group discussions, and field observations. The key CSA practices adopted by smallholder farmers include agroforestry, soil and water conservation, and integrated soil fertility management. These practices were reported to enhance food security indicators, with 85% of the respondents noting improved income and 80% recognizing increased consumption after adopting CSA practices. Despite these positive outcomes, challenges such as low adoption rates and implementation barriers persist. This study underscores the need for targeted interventions to expand CSA adoption, improve farmers’ capacity, and address environmental vulnerabilities. Policymakers and stakeholders are encouraged to foster environments that support sustainable agricultural practices.
1 Introduction
Agriculture, the cornerstone of livelihoods and economic stability in developing regions, is increasingly threatened by the impacts of climate change. Variations in temperature, erratic rainfall, and extreme weather events disrupt food production systems and disproportionately affect resource-constrained smallholder farmers (Asfaw et al., 2018; Kassie and Alemu, 2021). Sub-Saharan Africa, home to approximately 44 million smallholder farmers who rely on less than five hectares of land, is particularly vulnerable to these challenges (Agarwal et al., 2022). Ethiopia, where agriculture accounts for 34% of the GDP and employs over 70% of the population, epitomizes the struggle of climate-sensitive economies (Shiferaw, 2020; Canton, 2021).
The agricultural sector in Ethiopia faces mounting pressure from rising temperatures, fluctuating precipitation patterns, and frequent extreme events such as droughts and floods (Pathak et al., 2022). Over the past two decades, average temperatures have risen by 1.3°C, with rainfall patterns becoming more unpredictable, leading to prolonged dry spells and reduced crop yields (Deressa et al., 2020). These climate stressors are compounded by socio-economic vulnerabilities, including poverty, limited access to agricultural inputs, and weak institutional support, leaving smallholder farmers particularly exposed to food insecurity and livelihood risks (Dang et al., 2021).
Climate-smart agriculture (CSA) has emerged as a promising framework to address these interconnected challenges. Defined as a set of agricultural practices that enhance productivity, build resilience to climate shocks, and reduce greenhouse gas emissions, CSA offers an integrated solution for the impacts of climate change (Jirata et al., 2016). Core CSA practices include agroforestry, integrated soil fertility management, water conservation techniques, and the use of drought-resistant crop varieties (Kassie and Alemu, 2021). By fostering adaptation and mitigation simultaneously, CSA aligns closely with global sustainable development goals (Shukla et al., 2022). Despite its potential, the adoption of CSA in Ethiopia remains low because of socio-economic, cultural, and institutional barriers (Usmane et al., 2021).
Humbo Woreda, one of Ethiopia’s most climate-vulnerable regions, exemplifies the challenges and opportunities for CSA adoption. This woreda, characterized by semi-arid conditions, erratic rainfall, and frequent droughts, has long struggled with declining agricultural productivity and chronic food insecurity (Asnake and Mammo, 2020). To mitigate these challenges, government and non-governmental organizations have implemented interventions such as household ponds, irrigation systems, and water harvesting structures. However, the effectiveness and sustainability of these initiatives remain limited because of the lack of farmer engagement, resource constraints, and insufficient scaling mechanisms (Shiferaw, 2020; Kassie and Alemu, 2021).
A critical gap in the literature is the lack of empirical evidence on smallholder farmers’ perceptions of CSA practices and the socio-economic and institutional factors that influence their adoption (Dang et al., 2021). Studies have predominantly focused on broader policy frameworks or technological assessments, often overlooking the localized dynamics that determine the feasibility and success of CSA at the community level (Jirata et al., 2016). Understanding farmers’ perceptions, particularly in regions such as Humbo Woreda, is essential for tailoring CSA interventions to specific agro-ecological and socio-economic contexts (Pathak et al., 2022).
This study seeks to address these gaps by providing empirical insights into the adoption of CSA practices in Humbo Woreda. Specifically, it examines the barriers and drivers of CSA adoption, assesses the perceived effectiveness of these practices in enhancing food security and resilience, and identifies strategies to promote sustainable agricultural development. By integrating traditional knowledge with modern CSA approaches, this study aims to inform policy and practice, contributing to the broader goal of climate-resilient agriculture in Ethiopia and similar agro-ecological regions.
2 Materials and methods
2.1 Description of study area
The study was conducted in Humbo Woreda, Wolaita Zone, located 19 km from Wolaita Sodo town and 397 km south of Addis Ababa. Covering 84,646 hectares, the woreda comprises 20 rural and 2 semi-urban kebeles. The landscape is diverse, featuring flat plains, sloping areas, and low-to-moderate hills. The altitude ranges from 1,700 to 2,350 meters above sea level. Humbo falls into two agro-ecological zones: highlands (1,500–2,000 m) and midlands (1,500–2,000 m), which are crucial for agriculture and population distribution. The average temperature ranges between 16°C and 20°C, with annual rainfall varying from 800 mm to 1,200 mm (Tinlot et al., 2012) (see Figure 1).
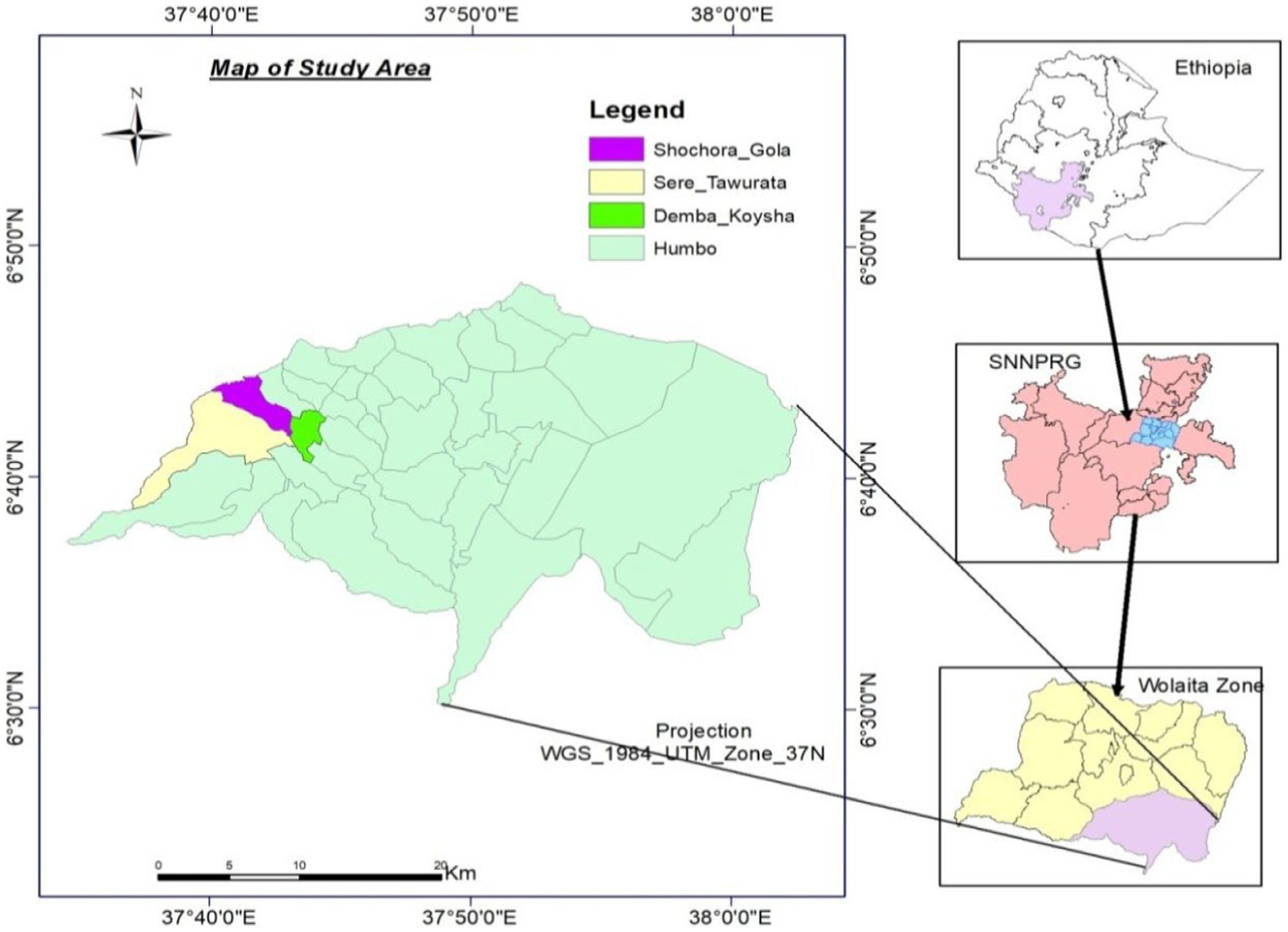
Figure 1. Map of study area. Source: US Geological Survey (USGS) http://glovis.usgs.gov.
2.2 Research design
This study adopted a mixed-methods approach, integrating explanatory and descriptive research designs, to investigate climate-smart agricultural (CSA) practices followed by smallholder farmers and their perceptions of these practices. The descriptive design aimed to characterize, analyze, and interpret the socio-economic, institutional, and demographic factors influencing CSA adoption, while the explanatory component sought to explore the relationships and causations underlying these practices. A cross-sectional study design was used to capture data at a single point in time to provide a snapshot of CSA adoption and associated factors within the study area.
To achieve a comprehensive understanding, both quantitative and qualitative research methods were employed. Quantitative data were collected through structured household surveys, enabling the study to obtain broad and representative information across diverse adoption categories. The qualitative approach complemented this by using key informant interviews, focus group discussions (FGDs), and field observations to gain deeper insights into farmers’ experiences, barriers, and motivations related to CSA practices. This combination of methods ensured a balanced and in-depth analysis of the research objectives.
2.3 Sources of data
The study relied on both primary and secondary data sources to ensure robust and multidimensional analysis. Primary data were collected using structured questionnaires administered to households, focus-group discussions, key informant interviews, and field observations. Respondents included smallholder farmers, development agents, kebele administrators, and agricultural and natural resource experts, providing a broad spectrum of perspectives and information relevant to CSA practices.
Secondary data were gathered from various published and unpublished sources, including institutional reports, research articles, books, and government records. Climate-related data, such as rainfall and temperature trends, from 1983 to 2018 were obtained from the National Meteorological Agency. Additional secondary data covered CSA policies and related interventions, supplementing the primary data and providing historical and policy context for the study. The integration of primary and secondary data allowed for a thorough evaluation of CSA practices, barriers, and outcomes in the study area.
2.4 Sampling techniques and sample size
The study employed the purposive selection of Humbo Woreda, which consists of 20 rural kebeles and 2 semi-urban areas, as the research site. To ensure representativeness, three kebeles, Sere Tawurata, Shochora Gola, and Demba Koysha, were selected using stratified random sampling techniques. These kebeles were chosen to reflect the agro-ecological diversity of the woreda, with Sere Tawurata and Shochora Gola representing the Wienedega (highland) zones and Demba Koysha representing the dega (midland) zone. This stratified approach aimed to capture the variations in agricultural practices and CSA adoption patterns across the study area. To determine the appropriate sample size, the total population of households in the selected kebeles (1,688) was used, and the calculation followed Yamane’s (1967) formula, as seen below in Equation 1:
where e = 1 − precision, e = is the level of precision that assumes e = 0.05, and N = proportion size.
The final sample size was calculated as 323 households, representing the study population at a 95% confidence level with a 5% margin of error. Proportional stratified sampling was then applied to allocate the sample across the three selected kebeles, based on their respective household populations. Sere Tawurata contributed the largest proportion, followed by Shochora Gola, and finally by Demba Koysha, which had the smallest population. This distribution ensured that the sample was proportional to the population sizes of the kebeles, maintaining representativeness and avoiding over- or under-sampling. Households within each kebele were randomly selected from the population list. This method ensured an unbiased selection process while preserving the demographic and agro-ecological diversity of the study area. The resulting sample provided a robust basis for collecting data through household surveys, enabling a comprehensive analysis of CSA adoption patterns and their influencing factors.
2.5 Data types and sources
This study employed both primary and secondary data sources to comprehensively analyze climate-smart agricultural (CSA) practices, their adoption factors, and perceived effectiveness. Primary data were collected through structured questionnaires, key informant interviews (KIIs), focus group discussions (FGDs), and field observations. These tools were designed to gather both quantitative and qualitative insights and capture detailed information on CSA practices, implementation barriers, and livelihood impacts. Secondary data were obtained from a range of published and unpublished sources, including institutional reports, academic journals, books, and climate datasets. Historical climate data, such as rainfall and temperature trends over 36 years (1983–2018), were obtained from the National Meteorological Agency. These datasets provided valuable context for understanding the long-term climatic impacts in the study area.
Data collection followed a mixed-methods approach. Quantitative data were gathered through household surveys that used structured, pre-tested questionnaires administered by trained enumerators. Qualitative data were collected from 12 purposively selected KIIs with development agents, model farmers, and knowledgeable women. These interviews provided in-depth perspectives on CSA adoption challenges and opportunities. FGDs, conducted in three sampled kebeles with seven participants each (50% women), explored CSA awareness, barriers, and livelihood impacts. Field observations complemented these efforts by documenting existing CSA practices and environmental conditions through descriptive notes and photographs, focusing on features such as soil conservation systems and irrigation methods.
This combination of primary and secondary data, along with quantitative and qualitative methodologies, ensured a robust and holistic evaluation of CSA practices in the study area, effectively addressing the study’s objectives.
2.6 Method of data analysis
Data from both primary and secondary sources were analyzed using both quantitative and qualitative approaches. Quantitative data were processed and analyzed using the Statistical Package for the Social Sciences (SPSS, version 2023) and Microsoft Excel (2023), employing descriptive statistics such as means, standard deviations, frequencies, and percentages. The results were visually represented using bar graphs and pie charts to highlight key trends and patterns. Qualitative data from FGDs, KIIs, and field observations were systematically reviewed and organized to identify themes and insights. Descriptive and inferential statistical techniques were used to interpret survey results and explore relationships between variables. This combination of methods ensured rigorous analysis, enabling the study to address its objectives effectively and provide actionable recommendations.
2.6.1 Model specification for climate change trend analysis
Trend analysis was conducted using the Mann–Kendall trend test and Sen’s slope estimator (Mann, 1945; Sen, 1968) as implemented in the R package modifiedmk. All-time series were tested for serial autocorrelation. When autocorrelation was not significant, a standard set of tests was applied (command mkktest). The Mann–Kendall test (Mann, 1945; Sen, 1968) was applied using Equation 2:
where n is the number of data points, xk and xj are data values in time series k and j (j > k), respectively, and sign (xj − xk) is defined in Equation 3:
The variance of S is computed using Equation 4:
where q is the number of tied groups and tp is the number of data points in the pth group. The values of S and VAR(S) are used to compute the test statistic Zs in Equation 5:
positive/negative Zs indicate an upward/downward trend during this period.
The slope of the trend was measured using Sen’s slope estimator (Sen, 1968). Sen’s method can be used in cases where the trend is assumed to be linear and is equal to in Equation 6:
where f (t) is a monotonically increasing or decreasing continuous function of time, Qt is the slope, and B is a constant. To obtain the slope estimate Q in Equation 7, the slopes of all data value pairs were determined as:
where Xj and Xk are data values at times j and k (j > k), respectively. Hence, we have only one datum in each period, and N is computed in Equation 8 as:
2.6.2 Identification of existing climate-smart agricultural practices in the study area
This study shows that smallholder farmers have adopted various climate-smart agricultural practices to overcome several environmental problems such as reducing soil fertility, climate change, and variability. The ultimate goal of adopting such practices is to enhance food security and improve household incomes. The most commonly practiced CSA practices in the study area are shown in Figure. Data were evaluated using descriptive statistics, such as means and percentages, to discover local climate-smart agricultural practices in the study area. The following criteria were used by Saguye (2017) to rank order: rate of adoption based on the percentage of respondents in the overall sample. Adoption rates greater than 70% are very high, adoption rates between 60 and 70% are high, adoption rates between 50 and 59% are moderately high, adoption rates between 40 and 49% are moderately low, and adoption rates below 40% are extremely low.
2.6.3 Measuring perceived effectiveness of CSA on the livelihoods of smallholders
The analytical hierarchy process (AHP) was employed to measure the perceived effectiveness of climate-smart agriculture (CSA) on the livelihoods of smallholders. This method was chosen for its ability to facilitate decision-making among multiple criteria, including both tangible and intangible aspects. AHP is a multi-criteria analysis approach that is effectively used to resolve complex and ambiguous decision-making scenarios. In this study, AHP was applied to evaluate CSA indicators within the pillars of food security and adaptation, aligning these indicators with the observable outcomes reported by smallholder farmers.
Farmers were asked to assess whether specific indicators had improved since the implementation of CSA practices. Using a 5-point Likert scale, respondents scored each indicator, enabling the calculation of mean scores. The Likert scale was characterized as follows: strongly disagree (SDA) = 1, disagree (DA) = 2, neutral (N) = 3, agree (A) = 4, and strongly agree (SA) = 5. A score of 1 indicated strong disagreement, while a score of 5 signified that the indicator had significantly improved since the beginning of the intervention. Indicators under the food security pillar included income, food productivity, livestock productivity, and food consumption, encompassing CSA objectives such as food availability, productivity, and utilization (Sampson et al., 2015).
To ensure consistency in the evaluation, AHP utilized a pairwise comparison matrix by applying Consistency Index (CI) and Consistency Ratio (CR) calculations, as outlined by Wind and Saaty (1980). The CI is calculated using Equation 9:
where represents the largest eigenvalue of the comparison matrix, and n is the number of elements being compared.
The consistency of judgments was validated by calculating the CR, computed using Equation 10:
where RI is the random consistency index, the average CI of a randomly generated reciprocal matrix of the same dimension. According to Saaty (1988), a CR value of 10% or less indicates sufficient consistency in judgment. If the CR value exceeds this threshold, pairwise comparison is deemed unreliable owing to excessive randomness. The application of AHP ensured that the calculated priorities for CSA indicators were both plausible and consistent, providing a robust basis for evaluating the effectiveness of CSA practices in improving food security and adaptation outcomes for smallholder farmers.
3 Results
3.1 Climate change trend analysis in the study area
Analysis of climate data from 1983 to 2018 revealed notable trends in temperature and rainfall within the study area. The average annual maximum temperature was 33.35°C, with the highest recorded in 2005, whereas the average annual minimum temperature was 10.04°C, with the lowest recorded in 2017. Rainfall averages 1273.68 mm annually, with the peak observed in 1996 (Table 1). Despite the observed changes in these parameters, this study highlights varying statistical significance in trends, necessitating further scrutiny to understand the local dynamics of climate variability and change (see Figure 2).
3.1.1 Trends in annual minimum temperature
The analysis of annual minimum temperatures indicated a downward trend with a Sen’s slope of −0.01°C per year, although this was not statistically significant (p = 0.6678). Similar regional studies show both alignments and contradictions with these findings. For instance, Ayalew et al. (2022) reported fluctuating but generally declining trends in the minimum temperatures across Ethiopia’s Rift Valley, mirroring this study’s results. Conversely, Mengistu et al. (2014) identified a significant increase of 0.15°C per decade in the Upper Nile Basin, and Tekleab et al. (2013) observed a marked rise in minimum temperatures in the Abay Basin. These discrepancies suggest that temperature trends in Ethiopia and the wider East African region are highly variable and influenced by geographic, topographic, and climatic factors. On a global scale, the Intergovernmental Panel on Climate Change (IPCC, 2021) emphasized rising minimum temperatures as a defining feature of climate change, driven predominantly by greenhouse gas emissions.
3.1.2 Trends in annual maximum temperature
The analysis revealed a statistically significant upward trend in annual maximum temperature, with a Sen’s slope of 0.03°C per year (p = 0.01983). This finding aligns with broader regional and global studies. For instance, Gebrechorkos et al. (2019) identified consistent warming trends across East Africa, particularly in arid and semi-arid zones. Similarly, Tekleab et al. (2013) and Mengistu et al. (2014) documented rising maximum temperatures in Ethiopia’s Abay and Upper Nile Basins, respectively. These observations reflect broader patterns of global temperature increases, as highlighted by Pathak et al. (2022), who attributed such warming to anthropogenic factors, including fossil fuel consumption and deforestation (see Figure 3).
3.1.3 Trends in annual rainfall
Rainfall trends in the study area showed a non-significant decline, with a Sen’s slope of −2.2373 mm per year (p = 0.713). This aligns with Qu et al. (2019), who reported declining rainfall trends in the Horn of Africa exacerbating water scarcity and agricultural challenges. Conway and Schipper (2011) similarly observed variability and declining rainfall in Ethiopia’s highlands, while Gummadi et al. (2018) documented mixed trends influenced by localized climatic factors. Globally, the IPCC (2021) has noted increasing unpredictability in rainfall patterns, underscoring the challenges posed to agriculture-dependent communities (see Figure 4).
3.1.4 Implications for climate-smart practices
The observed trends in temperature and rainfall underscore the need for targeted climate-adaptation strategies. Smallholder farmers, who are often the most vulnerable to climate variability, require enhanced access to climate information to make informed decisions. Studies have consistently highlighted the importance of climate-smart agricultural (CSA) practices for mitigating the adverse effects of climate change. For example, Ntawuruhunga et al. (2023) documented the effectiveness of CSA interventions, such as agroforestry and water harvesting, in East Africa. Similarly, Matewos (2020) emphasized the role of weather information in enhancing farmers’ adaptive capacity. Globally, Zougmoré et al. (2021) advocated CSA to improve productivity, reduce greenhouse gas emissions, and build resilience against climate shocks.
3.2 Identified respondents’ responses on CSA practices in the study area
The findings of this study indicate that smallholder farmers in the study area have adopted various climate-smart agricultural (CSA) practices to mitigate environmental challenges, such as declining soil fertility, climate change, and variability. These practices aim to enhance food security and improve household incomes. The adoption of CSA practices was evaluated using descriptive statistics, such as means and percentages, to identify locally practiced methods. The adoption rates of these practices were categorized based on the framework proposed by Saguye (2017), which ranks them as follows: very high (>70%), high (60–70%), moderately high (50–59%), moderately low (40–49%), and extremely low (<40%).
The most commonly adopted CSA practices in the study area included agroforestry (76%) and soil and water conservation (70.7%), both of which are highly adopted. Integrated soil fertility management practices, such as compost and manure management, were highly adopted, with an adoption rate of 60.7%. Moderately adopted practices included post-harvest technologies (41.6%), water harvesting technologies (49.2%), and conservation agriculture (47.9%). These practices included reduced tillage, crop residue management, mulching, and crop rotation. However, the adoption of small-scale irrigation (39%) and crop diversification (32%) was categorized as low, suggesting significant gaps in the utilization of CSA practices aimed at year-round cropping and enhanced agricultural resilience.
The relatively low adoption rates of certain CSA practices, such as small-scale irrigation and crop diversification, align with the findings of Teshager Abeje et al. (2019), who highlighted socio-economic, demographic, and institutional barriers as major contributors to low adoption. Challenges such as lack of access to loans, insufficient manpower for compost preparation, shortage of animal feed and fuelwood for mulching, and limited technical knowledge were identified during key informant interviews (KII) and focus group discussions (FGD). These barriers impede the scalability of acceptable and effective CSA practices.
Despite these challenges, existing CSA practices in the study area include well-established measures, such as terracing, integrated crop-livestock systems, soil and stone bunds, agroforestry, and water conservation structures such as check dams and diversion ditches. These practices reflect the farmers’ awareness of the detrimental effects of soil erosion on agricultural productivity and the environment. Schaller et al. (2017) observed similar trends in Ethiopia, noting that CSA practices are frequently integrated into watershed management programs that encompass crop rotation, intercropping, and soil and water conservation measures.
Although farmers in the study area have implemented various CSA practices, adoption rates remain low to moderate for many potentially impactful technologies. This aligns with Schaller et al. (2017) and Abeje et al. (2017), who found that, while CSA practices offer considerable potential for climate change adaptation, productivity enhancement, and mitigation, their adoption is often hindered by systemic barriers. Addressing these challenges requires concerted efforts to improve access to financial resources, enhance knowledge dissemination, and provide necessary tools and inputs to enable widespread adoption of CSA practices (see Figure 5).
This study identified various climate-smart agricultural (CSA) practices adopted by smallholder farmers to mitigate the adverse impacts of climate change. These practices are vital for addressing challenges such as declining soil fertility, erratic rainfall, and increased vulnerability to climate variability. Farmers in the study area have adopted strategies such as improved crop varieties, agroforestry, soil and water conservation, crop rotation, and small-scale irrigation to enhance productivity and resilience.
3.2.1 Mango and coffee seedlings
Smallholder farmers are increasingly planting mangoes and coffee seedlings to adapt to climate change. Mango cultivation contributes to agribusiness resilience, while coffee, Ethiopia’s leading export crop, provides a stable income for farmers. Studies conducted in Ethiopia have highlighted the role of perennial crops in enhancing ecological and economic resilience in the face of climate challenges (Tadesse et al., 2021). Similarly, research in other African nations has shown that perennial crop integration into farming systems increases income stability and reduces vulnerability to climate shocks (Kinyangi et al., 2023) (see Figure 6).
3.2.2 Improved teff variety
Teff, a staple food crop in Ethiopia, is widely adopted because of its adaptability to climate variability, including drought and pests. The adoption of improved teff varieties in the Humbo area has reduced ecological risks and improved household food security and income. According to Berhanu (2021), improved teff value chains have strengthened farmer resilience by ensuring consistent yields and better market opportunities. Comparable findings in Tanzania and Kenya also underline the importance of resilient staple crops for safeguarding food security in developing nations (Mmbando et al., 2020) (see Figure 7).
3.2.3 Soil and water conservation practices
Soil and water conservation (SWC) practices, such as soil bunds and community ponds, play a significant role in reducing soil erosion and improving water availability. These practices are essential for improving the soil fertility and boosting agricultural productivity. Teshome et al. (2021) reported that SWC measures, particularly when combined with biological interventions like planting grasses, enhance crop yields and reduce land degradation in Ethiopian highlands. Similar studies in Malawi and Uganda confirm that SWC structures, combined with agroforestry systems, significantly improve farming system sustainability (Ngwira et al., 2020) (see Figure 8).
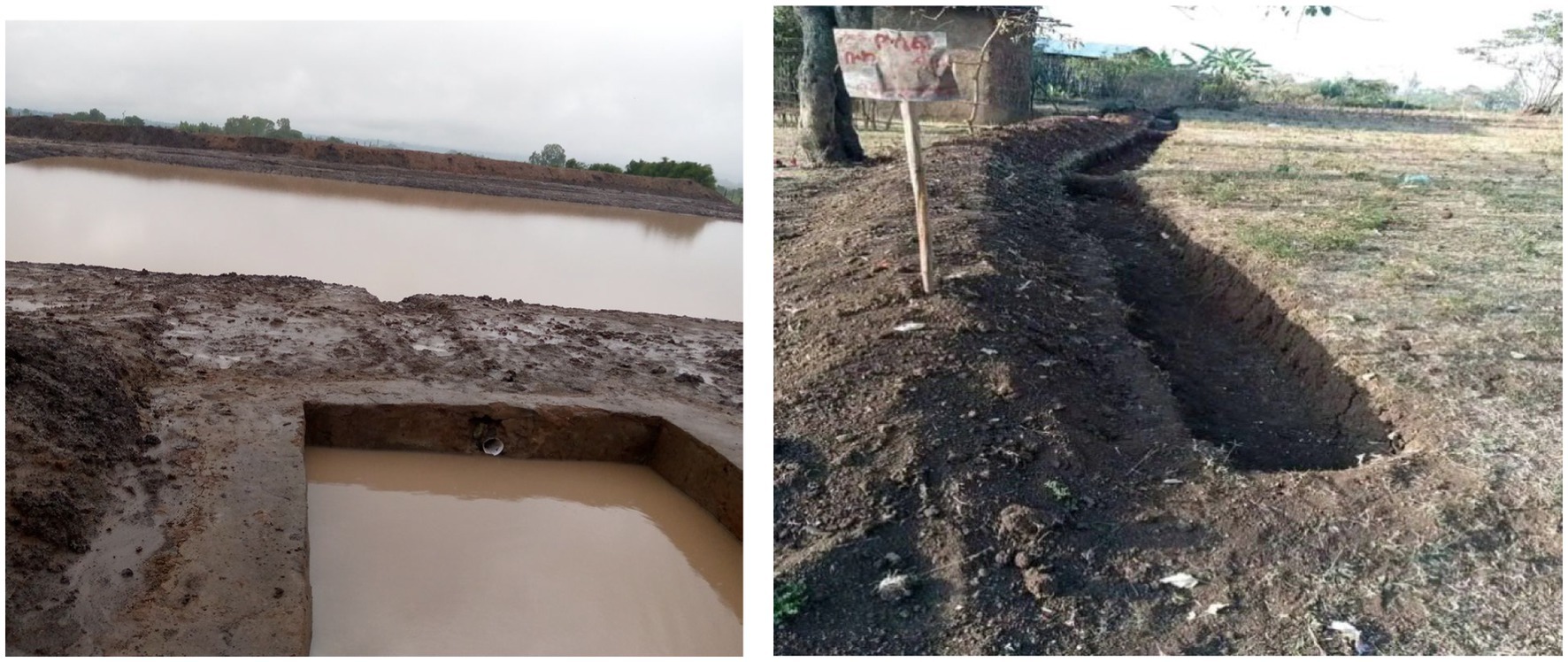
Figure 8. Soil and water conservation practices in the study area (community ponds and soil bound structure).
3.2.4 Crop rotation and diversification
Crop rotation is one of the most widely adopted CSA practices, which allows farmers to maintain soil fertility and manage pests and weeds effectively. This practice also enhances resilience to climate variability by diversifying harvesting periods. Pathak et al. (2022) found that crop rotation improves productivity and reduces climate vulnerability, consistent with evidence from Ethiopia and Kenya (Moges et al., 2021). Crop diversification, another common CSA strategy, is recognized for its economic benefits. Studies in Ethiopia have revealed that diversified cropping systems improve household incomes and reduce exposure to climate-induced risks (Teklewold and Mekonnen, 2020).
3.2.5 Small-scale irrigation practices
The study found that small-scale irrigation systems, such as rainwater harvesting and drip irrigation, were underutilized in the study area, with an adoption rate of only 39%. Nonetheless, irrigation is critical for stabilizing crop production and reducing vulnerability to erratic rainfall. Ayele et al. (2021) emphasized the transformative potential of small-scale irrigation in enhancing water-use efficiency and ensuring food security in Ethiopia. Similar findings from Senegal and Burkina Faso indicate that improved irrigation practices significantly increase crop productivity and farmer resilience (Ouedraogo et al., 2020) (see Figure 9).
3.2.6 Use of improved seeds
The use of improved seed varieties is a key CSA practice that enables farmers to adapt to the changing climatic conditions. These seeds are often drought-tolerant, pest-resistant, and high-yielding, thereby contributing to food security and economic stability. Teklewold and Mekonnen (2020) highlighted the role of improved seeds in enhancing farm productivity in Ethiopia, while studies in Nigeria and Rwanda demonstrate similar outcomes (Mafongoya, 2021).
3.2.7 Integration of CSA practices
Farmers in the study area integrated multiple CSA practices to address environmental and climatic stressors effectively. Techniques such as crop rotation, intercropping, contour planting, agroforestry, and mulching provide holistic solutions for enhancing resilience and productivity. Kassie and Will Tokgoz (2022) demonstrated that the integration of CSA practices in Ethiopia leads to significant improvements in soil health, crop yields, and risk mitigation. Evidence from Ghana and India further supports the benefits of integrated approaches to CSA adoption, particularly in resource-constrained settings (Adjei et al., 2023; Sharma and Gautam, 2020).
3.2.8 Challenges to CSA adoption
Despite its potential benefits, the adoption of CSA practices remains constrained by several factors, including limited access to financial resources, lack of technical knowledge, and insufficient water availability. Studies in Ethiopia and Kenya attribute low adoption rates to socio-economic and institutional barriers, emphasizing the need for targeted interventions (Ayele et al., 2021; Kassie and Will Tokgoz, 2022). Policy measures that enhance farmers’ capacity, improve market access, and provide financial incentives are crucial for scaling up CSA practices across Africa and other developing regions.
3.3 Perceived effectiveness towards climate-smart agricultural practices in the study area
Climate-smart agricultural (CSA) practices implemented in Ethiopia include agronomic measures such as crop rotation, residue incorporation, intercropping, disease-resistant crop varieties, and adjustments in planting and harvesting dates. Integrated soil fertility management (ISFM), irrigation, and the use of improved seeds have been widely adopted. The effectiveness of CSA practices can be assessed through their contributions to food security and adaptation, with two of the three pillars of CSA (the third being mitigation). In this study, the effectiveness of CSA was evaluated in terms of its impact on food security and adaptation measures.
3.3.1 Food security indicators
The results indicated that the majority of the respondents agreed or strongly agreed that CSA practices led to improvements in all four food security indicators. Specifically, 85% of the respondents perceived an increase in income (mean score = 4.61), and 80% reported improved food consumption (mean score = 3.66) after adopting CSA practices. Additionally, 68% noted an improvement in crop yield, although animal productivity received a relatively low score. This aligns with the findings of Meron (2018), who highlighted that farmers associate CSA practices with improved crop and livestock productivity, enhanced incomes, and diversified livelihood options. The application of tools for assessing and monitoring CSA effectiveness at the farm level was noted to enhance these outcomes. This is consistent with studies in Kenya and Uganda, where farmers reported improved food security due to CSA adoption driven by increased crop yields, better management practices, and more reliable income streams (Moges et al., 2021; Ngwira et al., 2020).
3.3.2 Adaptation indicators
The majority of the respondents strongly agreed that CSA practices had enhanced all seven adaptation strategies. Specifically, 79 and 70% agreed or strongly agreed that information and knowledge (mean score = 3.94) and farm productivity (mean score = 3.88) had significantly improved since CSA adoption, respectively. Crop diversity (mean score = 3.65) and animal diversity (mean score = 4.01) were perceived to have increased by 63 and 76% of respondents, respectively. These findings align with those of Kassie and Will Tokgoz (2022), who observed that CSA adoption improves farmers’ capacity to adapt to climate variability by enhancing knowledge transfer, resource use efficiency, and farm productivity. However, relatively lower scores for animal and crop diversity echo challenges that have been observed in other Ethiopian regions, where resource constraints limit the diversification potential of smallholder farms (Teshome et al., 2021).
3.3.3 Perceived benefits and peer learning
Perceived benefits, such as increased income, better access to food, and improved farm productivity, are strong motivators for smallholders to continue engaging in CSA practices. Positive perceptions also foster peer learning and encourage adoption by other farmers. This finding is supported by Meron (2018), who reported that favorable attitudes toward CSA technologies significantly influence community-level adoption rates.
3.3.4 Overall effectiveness of CSA practices
The perceived overall effectiveness of CSA practices in the study area was high, particularly in terms of food security and adaptation indicators. This is evident from the qualitative and quantitative data collected from the farmers, experts, and other stakeholders. CSA practices, such as the use of improved crop varieties, tree planting, crop-livestock integration, and small-scale irrigation, have played a pivotal role in improving resilience to climate variability. Similar findings have been reported in Ethiopia and other African nations where CSA interventions have enhanced smallholders’ adaptive capacities and food security outcomes (Pathak et al., 2022; Ayele et al., 2021) (see Table 2).
3.4 Factors affecting farmers’ adaptation measures to the impact of climate variability
A multinomial logit (MNL) model was applied to evaluate the determinants of farmers’ choices among mutually exclusive adaptation strategies, including early and late planting, crop diversification, and livestock adjustment. The analysis revealed that socio-economic, environmental, and institutional variables significantly influence farmers’ decisions to adapt to climate variability. The key factors analyzed include the sex of the household head, education level, family size, farm size, and farming experience, with regression coefficients, marginal effects, and significance levels provided in Table 3.
3.4.1 Sex of the household head
The gender of the household head was significantly associated with early and late planting (p = 0.006) and crop diversification (p = 0.013). Male-headed households were more likely to adopt these strategies than female-headed households were. This result highlights the existing gender disparities in adaptation capacities, where male farmers have better access to resources, inputs, and agricultural information, enabling them to implement adaptive strategies more effectively.
Female farmers, on the other hand, often face structural barriers, including limited access to credit, extension services, and land tenure, which restrict their ability to adopt CSA practices. These findings are consistent with Temesgen (2009), who reported similar trends in Ethiopia, and Fadina and Barjolle (2018), who highlighted gendered barriers to adaptation in sub-Saharan Africa.
Further evidence from Kristjanson (2020) underscores the need for gender-responsive adaptation policies that address women’s unique challenges, including access to finance and training. Gender-sensitive extension programs, female-led farmer cooperatives, and access to microcredit facilities have been shown to significantly enhance female farmers’ adaptive capacities in regions such as Malawi and Kenya (Ngwira et al., 2020; Ayele et al., 2021).
3.4.2 Educational level of the household head
Education positively influenced all adaptation strategies, with significant associations observed for early and late planting, crop diversification, and livestock adjustment (p < 0.05). A positive coefficient indicates that educated farmers are better equipped to process climate information and implement informed decisions. Education enhances access to agricultural extension services and improves farmers’ abilities to adopt CSA practices.
Taruvinga et al. (2016) demonstrated that higher educational attainment significantly improved the likelihood of adopting crop diversification and irrigation systems in Zimbabwe. Similarly, Deressa et al. (2020) found that Ethiopian farmers with formal education were more likely to implement adaptation measures such as soil and water conservation and livestock diversification.
Educated farmers are also more likely to experiment with innovative practices such as improved seed varieties and precision irrigation. Studies in Ghana and Rwanda further emphasize that education strengthens resilience by enabling farmers to participate in peer-learning networks and farmer-led innovations (Adjei et al., 2023; Mafongoya, 2021).
3.4.3 Family size
Family size had a positive correlation with early and late planting, crop diversification, and livestock adjustment, as larger families provided the labor required for labor-intensive adaptation measures. Larger households can allocate resources more flexibly to manage climate risks such as diversifying crops or constructing soil conservation structures.
These findings align with Deressa et al. (2009), who noted that households with more members were more likely to adopt adaptation strategies in Ethiopia. Similarly, Teshome et al. (2021) found that family labor significantly influenced the adoption of agroforestry and conservation agriculture in Ethiopia. Globally, research in Tanzania and Nigeria supports the idea that larger families are more inclined to adopt CSA practices because of the greater availability of labor (Mmbando et al., 2020; Onyango et al., 2021). However, increasing family size without commensurate resource allocation may also strain household food security, underscoring the need for policies that promote efficient labor utilization and mechanization.
3.4.4 Farm size
Farm size was significantly associated with the livestock adjustment strategies (p < 0.05). Farmers with larger landholdings are more likely to implement adaptive practices such as diversifying livestock or integrating livestock with crops. This is because larger plots offer greater flexibility for experimentation and risk mitigation, including diversification of income sources. Le Dang et al. (2014) highlighted that farmers with larger plots are more willing to invest in adaptation strategies such as irrigation and agroforestry. Conversely, smallholders often lack the financial resources and land security required to adopt long-term measures, leaving them more vulnerable to climatic shocks. Studies in Burkina Faso and Senegal confirmed that farm size is a critical determinant of CSA adoption, particularly for livestock and irrigation systems (Ouedraogo et al., 2020; Ayele et al., 2021). Policies promoting equitable land redistribution and improved access to land use rights could enhance adaptation capacities, especially for marginalized farmers.
3.4.5 Farming experience
Farming experience was positively correlated with all adaptation strategies, particularly with crop diversification and livestock adjustment (p < 0.05). Experienced farmers are more adept at recognizing climate risks and utilizing appropriate adaptation measures as they often rely on accumulated knowledge and traditional practices.
Nhemachena and Hassan (2007) found that experienced farmers in southern Africa were more likely to adopt strategies such as crop rotation and livestock adjustment to mitigate climatic risks. Similar findings in Ethiopia by Kassie and Will Tokgoz (2022) suggest that farming experience enhances adaptive capacity by improving farmers’ abilities to integrate traditional knowledge with modern techniques. Additionally, studies in Kenya and Uganda emphasize the role of experience in shaping farmers’ perceptions of risk and willingness to invest in innovative practices such as drought-tolerant crops and soil fertility management (Pathak et al., 2022; Ngwira et al., 2020).
3.4.6 Policy implications
The findings of this study reveal several key factors influencing farmers’ adaptation strategies, underscoring the need for targeted policy interventions to enhance their farmers’ adaptation to climate variability in Ethiopia. Gender disparities, evident in the lower participation of female-headed households in adaptation measures, call for gender-sensitive policies that address barriers such as limited access to credit, training, and land ownership. Ethiopia’s Climate Resilient Green Economy (CRGE) strategy emphasizes gender equity in climate actions, aligning with global findings that empowering women through tailored interventions significantly improves their adaptive capacity (Kristjanson, 2020; Fadina and Barjolle, 2018).
Education plays a crucial role in enabling farmers to adopt adaptive measures by enhancing their ability to interpret climate information and implement informed strategies. Ethiopia’s National Adaptation Plan (NAP) highlights the importance of education and awareness in building resilience to climate impact. Policies should focus on improving rural education and integrating climate-smart training into agricultural extension programs to empower farmers with the knowledge required for effective adaptation, as suggested by Onyango et al. (2021) and Debesai et al. (2019).
Family size and labor availability were found to influence the adoption of labor-intensive adaptation strategies such as soil conservation and crop diversification. Policies should address labor constraints through mechanization and community labor-sharing initiatives, particularly for smaller households. The Ethiopian Agricultural Transformation Agency (ATA) has advocated for increased mechanization to enhance productivity, which can be further tailored to support adaptation measures (Teshome et al., 2021; Ngwira et al., 2020).
Land access and tenure security significantly affect farmers’ ability to adopt long-term adaptation strategies. Ethiopia’s rural land certification program has shown promise for improving tenure security and encouraging sustainable practices. Expanding such initiatives can help smallholders invest in climate-smart agriculture and enhance their resilience, as observed in the studies by Ouedraogo et al. (2020) and Le Dang et al. (2014).
Finally, access to climate information and financial services is critical to empowering farmers to adapt effectively. Ethiopia’s NAP and CRGE emphasize the dissemination of localized climate forecasts and early warning systems. Strengthening these systems through digital platforms and public-private partnerships can bridge the information gap. Additionally, enhancing access to credit and introducing climate risk insurance can mitigate financial barriers, as evidenced by successful models in Ethiopia and other African countries (Pathak et al., 2022; Ayele et al., 2021).
4 Conclusion and recommendations
The analysis of climate trends and CSA practices in the study area highlights the pressing challenges and opportunities for addressing climate change impacts on smallholder agriculture. The annual rainfall exhibited a declining trend, although it was not statistically significant. Similarly, the annual minimum temperature showed no significant trend, with Sen’s slope results indicating a minor downward trend. In contrast, the Mann–Kendall trend test revealed a significant upward trend in annual maximum temperature. These findings underscore the climatic changes and fluctuations in the research area, contributing to various livelihood risks and necessitating targeted intervention.
In response to these challenges, a range of CSA practices has been adopted by farmers in the study area, including agroforestry, integrated soil fertility management, soil and water conservation, terracing, tree planting, intercropping, crop rotation, and the use of structures such as check dams and diversion ditches. These practices are critical for improving household food security, reducing vulnerability, and lowering greenhouse gas emissions at the farm level. Despite the potential of these technologies, their adoption remains limited, with a significant proportion of farmers not participating or engaging at low levels. This highlights the need for enhanced support and awareness to scale up CSA practice.
The analysis also revealed that CSA practices positively impact household income, food productivity, and animal production, demonstrating their effectiveness in addressing food security and adaptation needs. However, sustainability and widespread adoption of these practices require greater encouragement and support from stakeholders. Farmers who have implemented CSA practices need sustained assistance to improve outcomes, whereas non-adopters require targeted awareness campaigns to motivate adoption. Strengthening CSA practices is essential for achieving sustainable rural livelihoods and ensuring food security amidst the ongoing challenges of climate change.
To address these issues, a comprehensive and collaborative approach is recommended. Farmers should be encouraged to adopt improved varieties, crop rotation, composting, soil and water conservation, and agroforestry, as these practices enhance productivity, resilience, and sustainability. Capacity-building efforts must focus on improving infrastructure, such as roads, markets, education, and extension services, to support farmers in implementing CSA practices effectively. Resolving competing demands for resources, such as crop residues, through innovative solutions, such as planting forage trees, can alleviate barriers to adoption.
Furthermore, policymakers should devise programs and incentives to promote the simultaneous adoption of multiple CSA practices and ensure synergies across CSA pillars. NGOs and civil society organizations must prioritize the adoption of less-utilized practices and strengthen their support for widely adopted ones. The commitment of all stakeholders, government agencies, development partners, and local communities is crucial for fostering the effective use of CSA practices. By supporting adopters and encouraging non-adopters, stakeholders can help achieve sustainable agricultural systems and enhance the resilience of smallholder farmers to climate change impacts.
Data availability statement
The raw data supporting the conclusions of this article will be made available by the authors, without undue reservation.
Author contributions
MT: Conceptualization, Data curation, Formal analysis, Funding acquisition, Investigation, Resources, Methodology, Project administration, Software, Supervision, Validation, Visualization, Writing – original draft, Writing – review & editing. AA: Conceptualization, Data curation, Formal analysis, Funding acquisition, Investigation, Resources, Methodology, Project administration, Software, Supervision, Validation, Visualization, Writing – original draft, Writing – review & editing. ST: Conceptualization, Data curation, Formal analysis, Funding acquisition, Investigation, Resources, Methodology, Project administration, Software, Supervision, Validation, Visualization, Writing – original draft, Writing – review & editing. AB: Conceptualization, Data curation, Formal analysis, Funding acquisition, Investigation, Resources, Methodology, Project administration, Software, Supervision, Validation, Visualization, Writing – original draft, Writing – review & editing. ES: Conceptualization, Data curation, Formal analysis, Funding acquisition, Investigation, Resources, Methodology, Project administration, Software, Supervision, Validation, Visualization, Writing – original draft, Writing – review & editing.
Funding
The author(s) declare that no financial support was received for the research, authorship, and/or publication of this article.
Conflict of interest
The authors declare that the research was conducted in the absence of any commercial or financial relationships that could be construed as a potential conflict of interest.
Publisher’s note
All claims expressed in this article are solely those of the authors and do not necessarily represent those of their affiliated organizations, or those of the publisher, the editors and the reviewers. Any product that may be evaluated in this article, or claim that may be made by its manufacturer, is not guaranteed or endorsed by the publisher.
References
Abeje, A., Ayen, K., Awoke, M., and Abebaw, L. (2017). Adoption and intensity of modern bee hive in Wag Himra and North Wollo Zones, Amhara Region, Ethiopia. Agric. Resour. Econ. Int. Sci. E-J. 3, 5–26. doi: 10.51599/are.2017.03.01.01
Adjei, M. R., Amponsa-Achiano, K., Okine, R., Tweneboah, P. O., Sally, E. T., Dadzie, J. F., et al. (2023). Post introduction evaluation of the malaria vaccine implementation programme in Ghana, 2021. BMC Public Health 23:586. doi: 10.1186/s12889-023-15481-6
Agarwal, T., Goel, P. A., Gartaula, H., Rai, M., Bijarniya, D., Rahut, D. B., et al. (2022). Gendered impacts of climate-smart agriculture on household food security and labor migration: insights from Bihar, India. Int. J. Clim. Change Strateg. Manag. 14, 1–19.
Asfaw, A., Simane, B., Hassen, A., and Bantider, A. (2018). Variability and time series trend analysis of rainfall and temperature in northcentral Ethiopia: a case study in Woleka sub-basin. Weather Clim. Extrem. 19, 29–41. doi: 10.1016/j.wace.2017.12.002
Asnake, G., and Mammo, G. (2020). Farming climate change adaptation mechanisms and factors affecting farmers’ decision to adaptation: a case study in Meket district, North Wollo, Ethiopia. Int. J. Adv. Sci. Tech. Res. 6, 87–114.
Ayalew, A. D., Wagner, P. D., Sahlu, D., and Fohrer, N. (2022). Land use change and climate dynamics in the Rift Valley Lake Basin, Ethiopia. Environ. Monit. Assess. 194:791. doi: 10.1007/s10661-022-10393-1
Ayele, W. T., Ayele, Z. T., Shumey, E. E., and Demessie, S. A. (2021). Performance evaluation of Lower Fetam and Leza small-scale irrigation schemes by using internal and external process indicators: case study in west gojjam zone, Amhara, Ethiopia. Sustain. Water Resour. Manag. 7, 1–10.
Berhanu, E. (2021). “Factors affecting customers’ intention to adopt ATM banking in commercial bank of Ethiopia” in Doctoral dissertation (San Antonio, TX: St. Mary’s University).
Canton, H. (2021). “Food and agriculture organization of the United Nations—FAO” in The Europa directory of international org.
Conway, D., and Schipper, E. L. F. (2011). Adaptation to climate change in Africa: Challenges and opportunities identified from Ethiopia. Glob. Environ. Change 21, 227–237. doi: 10.1016/j.gloenvcha.2010.07.013
Dang, N. M., Anh, D. T., and Dang, T. D. (2021). “Water–food–energy nexus in the context of climate change: developing a water security index for water resource management in Vietnam” in Global Changes and Sustainable Development in Asian Emerging Market Economies (Cham: Springer), 611–629.
Debesai, M. G., Kidane, T. T., Ogbazghi, W., Araia, W., Measho, S., and Amlesom, S. (2019). Understanding drought coping mechanisms in smallholder farm households: evidence from Dry Lands of Eritrea. J. Agric. Econ. Rural Dev. 5, 548–554.
Deressa, T. T., Hassan, R. M., Ringler, C., Alemu, T., and Yesuf, M. (2009). Determinants of farmers’ choice of adaptation methods to climate change in the Nile Basin of Ethiopia. Glob. Environ. Change 19, 248–255. doi: 10.1016/j.gloenvcha.2009.01.002
Deressa, A., Yli-Halla, M., and Mohamed, M. (2020). Soil organic carbon stock and retention rate among land uses along Didessa toposequence in humid Western Ethiopia. Environmental Systems Research, 9, 1-12.anizations 2021 : Routledge, 297–305.
Fadina, A. M. R., and Barjolle, D. (2018). Farmers’ adaptation strategies to climate change and their implications in the Zou Department of South Benin. Environments 5:15. doi: 10.3390/environments5010015
Gebrechorkos, S. H., Bernhofer, C., and Hülsmann, S. (2019). Impacts of projected change in climate on water balance in basins of East Africa. Sci. Total Environ. 682, 160–170. doi: 10.1016/j.scitotenv.2019.05.053
Gummadi, S., Rao, K. P. C., Seid, J., Legesse, G., Kadiyala, M. D. M., Takele, R., et al. (2018). Spatio-temporal variability and trends of precipitation and extreme rainfall events in Ethiopia in 1980–2010. Theor. Appl. Climatol. 134, 1315–1328. doi: 10.1007/s00704-017-2340-1
Kassie, G. T. M., and Will Tokgoz, S. (2022). Analysis of the impacts of agricultural incentives on the performance of agricultural value chains.
Kassie, K. E., and Alemu, B. A. (2021). Does irrigation improve household’s food security? The case of Koga irrigation development project in northern Ethiopia. Food Security 13, 291–307.
Kinyangi, H. N., Musiega, M., and Nelima, M. (2023). Influence of Governance Structure on the Financial Performance of Microfinance Banks in Kenya. Afr. J. Empirical Res. 4, 671–678. doi: 10.51867/ajernet.4.2.67
Kristjanson, P. (2020). Closing gender gaps in forest landscape initiatives. Int. For. Rev. 22, 44–54. doi: 10.1505/146554820829523925
Le Dang, H., Li, E., Nuberg, I., and Bruwer, J. (2014). Understanding farmers’ adaptation intention to climate change: A structural equation modelling study in the Mekong Delta. Viet. Environ. Sci. Policy 41, 11–22.
Mafongoya, M. M. P. L. (2021). Effects of improved pigeonpea fallows on biological and physical soil properties and their relationship with maize yied
Mann, H. B. (1945). Nonparametric tests against trend. Econometrica 13, 245–259. doi: 10.2307/1907187
Matewos, T. (2020). The state of local adaptive capacity to climate change in drought-prone districts of rural Sidama, southern Ethiopia. Clim. Risk Manag. 27:100209. doi: 10.1016/j.crm.2019.100209
Mengistu, D., Bewket, W., and Lal, R. (2014). Recent spatiotemporal temperature and rainfall variability and trends over the Upper Blue Nile River Basin, Ethiopia. Int. J. Climatol. 34, 2278–2292. doi: 10.1002/joc.3837
Meron, E. (2018). From patterns to function in living systems: Dryland ecosystems as a case study. Annu. Rev. Condens. Matter Phys. 9, 79–103. doi: 10.1146/annurev-conmatphys-033117-053959
Mmbando, G. S., Teranishi, M., and Hidema, J. (2020). Very high sensitivity of African rice to artificial ultraviolet-B radiation caused by genotype and quantity of cyclobutane pyrimidine dimer photolyase. Sci. Rep. 10:3158. doi: 10.1038/s41598-020-59720-x
Moges, E., Demissie, Y., Larsen, L., and Yassin, F. (2021). Review: sources of hydrological model uncertainties and advances in their analysis. Water 13:28. doi: 10.3390/w13010028
Ngwira, A. R., Kabambe, V., Simwaka, P., Makoko, K., and Kamoyo, K. (2020). Productivity and profitability of maize-legume cropping systems under conservation agriculture among smallholder farmers in Malawi. Acta Agric. Scand. B 70, 241–251. doi: 10.1080/09064710.2020.1712470
Nhemachena, C., and Hassan, R. (2007). Micro-level analysis of farmers adaption to climate change in Southern Africa. Washington, DC: International Food Policy Research Institute (IFPRI).
Ntawuruhunga, D., Ngowi, E. E., Mangi, H. O., Salanga, R. J., and Shikuku, K. M. (2023). Climate-smart agroforestry systems and practices: a systematic review of what works, what doesn’t work, and why. Forest Policy Econ. 150:102937. doi: 10.1016/j.forpol.2023.102937
Onyango, C. M., Nyaga, J. M., Wetterlind, J., Söderström, M., and Piikki, K. (2021). Precision agriculture for resource use efficiency in smallholder farming systems in sub-saharan africa: A systematic review. Sustainability 13:1158.
Ouedraogo, Y., Taonda, J. B. S., Sermé, I., Tychon, B., and Bielders, C. L. (2020). Factors driving cereal response to fertilizer microdosing in sub‐Saharan Africa: a meta‐analysis. Agron. J. 112, 2418–2431. doi: 10.1002/agj2.20229
Pathak, M., Slade, R., Pichs-Madruga, R., Ürge-Vorsatz, D., Shukla, R., and Skea, J. (2022). Climate change 2022 mitigation of climate change: technical summary. Geneva: IPCC.
Qu, C., Hao, X., and Qu, J. J. (2019). Monitoring extreme agricultural drought over the Horn of Africa (HOA) using remote sensing measurements. Remote Sens. 11:902. doi: 10.3390/rs11080902
Saguye, T. S. (2017). Assessment of farmers’ perception of climate change and variability and it’s implication for implementation of climate-smart agricultural practices: the case of Geze Gofa District, Southern Ethiopia. Assessment 7, 1–15. doi: 10.4172/2167-0587.1000191
Sampson, C. C., Smith, A. M., Bates, P. D., Neal, J. C., Alfieri, L., and Freer, J. E. (2015). A high‐resolution global flood hazard model. Water Resour. Res. 51, 7358–7381. doi: 10.1002/2015WR016954
Saaty, T. L. (1988). “What is the analytic hierarchy process?” in Mathematical models for decision support (Berlin Heidelberg: Springer), 109–121.
Schaller, M., Barth, E. I., Blies, D., Röhrig, F., and Schümmelfeder, M. (2017). Climate smart agriculture (CSA): water harvesting. Berlin: International Center for Tropical Agriculture (CIAT). The Centre for Rural Development (SLE).
Sen, P. K. (1968). Estimates of the regression coefficient based on Kendall’s tau. J. Am. Stat. Assoc. 63, 1379–1389. doi: 10.1080/01621459.1968.10480934
Sharma, M., and Gautam, R. (2020). 2019-nCoV pandemic: a disruptive and stressful atmosphere for Indian academic fraternity. Brain Behav. Immun. 88:948. doi: 10.1016/j.bbi.2020.04.025
Shiferaw, W. (2020). Climate-smart agricultural practices in ethiopia: implications of mitigation of greenhouse gas emissions: a review paper. Ukr. J. Ecol. 11, 23–30.
Shukla, P. R., Skea, J., Slade, R., Al Khourdajie, A., Van Diemen, R., McCollum, D., et al. (2022). “Climate change 2022: mitigation of climate change” in Contribution of Working Group III to the Sixth Assessment Report of the Intergovernmental Panel on Climate Change (Cambridge: Cambridge University Press), 9781009157926.
Tadesse, M., Simane, B., Abera, W., Tamene, L., Ambaw, G., Recha, J. W., et al. (2021). The effect of climate-smart agriculture on soil fertility, crop yield, and soil carbon in Southern Ethiopia. Sustainability 13:4515. doi: 10.3390/su13084515
Taruvinga, A., Visser, M., and Zhou, L. (2016). Barriers and opportunities to climate change adaptation in rural Africa: evidence from the Eastern Cape Province of South Africa. Int. J. Dev. Sustain. 5, 518–535.
Tekleab, S., Mohamed, Y., and Uhlenbrook, S. (2013). Hydro-climatic trends in the Abay/Upper Blue Nile Basin, Ethiopia. Phys. Chem. Earth A/B/C 61–62, 32–42. doi: 10.1016/j.pce.2013.04.017
Teklewold, H., and Mekonnen, A. (2020). Weather at different growth stages, multiple practices and risk exposures: panel data evidence from Ethiopia. Clim. Change Econ. 11:2050009. doi: 10.1142/S2010007820500098
Temesgen, T. (2009). A study on the perception and adaptation to climate change: the case of farmers in the Nile Basin of Ethiopia. Pretoria: Centre for Environmental Economics and Policy for Africa (CEEPA), University of Pretoria, South Africa.
Teshager Abeje, M., Tsunekawa, A., Adgo, E., Haregeweyn, N., Nigussie, Z., Ayalew, Z., et al. (2019). Exploring drivers of livelihood diversification and its effect on adoption of sustainable land management practices in the Upper Blue Nile Basin, Ethiopia. Sustainability 11:2991. doi: 10.3390/su11102991
Teshome, H., Tesfaye, K., Dechassa, N., Tana, T., and Huber, M. (2021). Smallholder farmers’ perceptions of climate change and adaptation practices for maize production in Eastern Ethiopia. Sustainability 13:9622. doi: 10.3390/su13179622
Tinlot, M., Branca, G., and Gorin, P. (2012). The carbon footprint of the agricultural growth project (AGP) in Ethiopia: An application of the EX-Ante Carbon-balance Tool (EX-ACT).
Usmane, I. A., Umer, A. T., Siraj, N., Magersa, O., Urgesa, B., and Urge, T. (2021). Climate smart agriculture interventions in selected agricultural growth program-II districts of Harari region and Dire Dawa Administration: Double Cropping Practices. Int. J. Agric. Sci. Food Technol. 7, 14–19.
Wind, Y., and Saaty, T. L. (1980). Marketing applications of the analytic hierarchy process. Manag. Sci. 26, 641–658.
Yamane, Y. (1967). Mathematical formulae for sample size determination. J. Undergrad. Math. 1, 1–29.
Keywords: adoption, climate change, climate-smart agriculture, Ethiopia, smallholder farmers, rainfall trends
Citation: Tessema MW, Atnafe AD, Tora SE, Bantider A and Shure E (2025) Trend analysis of climate change and effect of climate-smart agricultural practices: the case of Humbo Woreda, Wolaita Zone, South Ethiopia. Front. Clim. 7:1456328. doi: 10.3389/fclim.2025.1456328
Edited by:
Noureddine Benkeblia, University of the West Indies, Mona, JamaicaReviewed by:
John Malcolm Gowdy, Rensselaer Polytechnic Institute, United StatesShubham Pathak, Walailak University, Thailand
Copyright © 2025 Tessema, Atnafe, Tora, Bantider and Shure. This is an open-access article distributed under the terms of the Creative Commons Attribution License (CC BY). The use, distribution or reproduction in other forums is permitted, provided the original author(s) and the copyright owner(s) are credited and that the original publication in this journal is cited, in accordance with accepted academic practice. No use, distribution or reproduction is permitted which does not comply with these terms.
*Correspondence: Mefekir Woldegebriel Tessema, d21lZmVraXJAZ21haWwuY29t