- 1Division of Cariology and Endodontology, Faculty of Medicine, CUMD, University Clinics of Dental Medicine, University of Geneva, Geneva, Switzerland
- 2Department of Economics and Quantitative Methods, University of Genoa, Genoa, Italy
This study aimed to compare marginal adaptation in enamel and dentin before and after aging of laser vs. bur-prepared mixed class V cavities restored by different restorative systems. Seventy two caries-free human molars were distributed to nine experimental groups; cavities were prepared using two different lasers: a handpiece -integrated 2.94 μm Er:YAG laser at 4.5 W, 300 mJ, and 0.75 W, 50 mJ with 15 Hz (LiteTouch, Light Instruments, Israel) and a novel CO2 laser at 12.95 W, 19.3 mJ, and 4.1 W, 6.11 mJ with 671 Hz (Solea 9.3 μm, Convergent Dental, USA). Cavities prepared with conventional diamond burs (Intensiv, Switzerland) in a red contra angle at high speed under maximal water cooling served as control. Cavities were prepared under simulation of dentinal fluid and restored using three different self-etching universal adhesives in combination with three nanohybrid composites, applied in two layers: Scotchbond Universal with Filtek Supreme XTE (3M, USA), G-Premio BOND with Essentia Universal (GC, Japan), and OptiBond Universal with Harmonize Universal (Kerr, USA). After restorations' polishing and simultaneous thermal (5–50°C, 2 min each) and mechanical loading (max. 49 N; 200,000 cycles), replicas of restoration margins were examined under SEM at × 200 magnification. Percentages of continuous margins (CMs) were quantified before and after the fatigue test and statistically compared (two-way ANOVA with Fisher's least significant difference [LSD] post hoc test). Significant differences were found in almost all groups between the results before and after the fatigue test, as well as between the different preparation tools and restorative materials (p < 0.05). Traditional bur preparations are confirmed as gold standard in enamel and dentin, as all three tested restorative systems provide results of marginal adaptation of more than 80% CM after loading. Er:YAG laser preparations can be equally effective in combination with SBU/Filtek Supreme XTE. CO2 laser ablation could not provide convincing results with the tested self-etching restorative systems. Marginal adaptation has been highly dependent on the substrate and showed impaired adhesion, especially in enamel. Scotchbond Universal/Filtek Supreme XTE showed the highest and most stable values of CM. The other two restorative systems were highly dependent on the preparation device of the substrate.
Introduction
Minimally invasive dentistry strongly relies on both adhesion due to micromechanical retention owing to mineral replacement by resin monomers and a potential chemical bonding of reactive monomers to hydroxyapatite (1). Likewise, maximum preservation of sound tooth substance is possible thanks to selective ablation methods for caries removal, contributing to patients' quality of life by preserving their own dental tissue up to an advanced age.
Since the introduction of adhesion by Buonocore (2) seven generations of adhesive systems have been developed. Currently available bonding systems can be hierarchically classified into two major categories: etch-and-rinse and self-etch (3, 4). High diversity on the market and differences in the composition of materials and the manner in which they are applied resulted in an increased demand for simpler, more user-friendly, and less technique-sensitive adhesives (3). This is why the seventh generation of one-step universal adhesives has been developed by diverse manufacturers by applying their own concepts and their proprietary ingredients, resulting in products with different characteristics that lead to diverse in vivo and in vitro results (5, 6).
Preparation of dental hard tissue is an important factor with regard to tissues' surface morphology and thus to the general interaction of adhesive materials to enamel and dentin, resulting in differences in bond strength, in microleakage, in quality of marginal adaptation, and finally in restorations' clinical success (7–9). As shown in previous studies, (7–11) lasers with emission wavelengths which are strongly absorbed in water and hydroxyapatite offer new possibilities in selective and minimally invasive ablation of caries-infected dental tissues. Herein, 2.94 μm Er:YAG lasers and the recently introduced 9.3 μm CO2 lasers can be used. The Er:YAG laser, in particular, can promote equal adhesion results to conventional bur treatments when using a self-etching universal adhesive, when enamel and dentin cavities are prepared with optimized laser settings with regard to power, frequency, water amount, and air pressure (7, 8). In this context, it has been shown that finishing or rather smoothening of the cavity surface with less powerful settings seems to be essential for optimal marginal adaptation and adhesion (8).
With respect to enamel adhesion, 9.3 μm CO2 laser preparations seem to adversely affect adhesion of adhesives (10). This might be due to chemical changes in the substrate caused by the occurrence of high temperatures (~1,000°C) next to melting of the surface, which may explain the smooth and glazed surface micromorphology after laser use. Contrarily, Er:YAG laser preparations do not exceed temperatures of 250–300°C and produce honeycomb patterns, which can be—due to its micro retentive properties—considered as favorable to bonding procedures in enamel without the necessity of previous acid etching, under the condition that subsurface damage is not induced by the procedure.
Regarding dentin, both laser types delivered good results in terms of micromechanical adhesion in dentin without previous phosphoric acid etching (11–13).
Conflicting outcomes have been reported in the literature with regard to different one-component universal adhesives, as well as new laser technologies. Success of laser preparation can be directly related to its wavelength as well as to the applied power, water spray settings, and its morphological effects on dental hard tissues (8, 10, 14, 15).
The performances of adhesive systems are strongly dependent on differences in the composition of hydrophobic and hydrophilic monomers, photoinitiators and co-initiators, organic solvents as well as additives such as nanofillers and bioactive components, and also the manufacturers' instructions for application and not only by the bonding approach (additional step for etching or self-etching) (16–20).
There is a need for further investigations and analysis of the interaction between simplified universal adhesives, preparation methods, and their interaction with enamel and dentin substrates (21). Therefore, this study aimed to assess the quality of marginal adaptation delivered by three different commercially available restorative systems: one-component universal adhesive systems and resin composites on restorations with enamel and dentin margins prepared by two lasers (Er:YAG and a recently developed 9.3 μm CO2 laser) where conventional bur preparation served as the positive control.
The null hypothesis tested was that there were no differences in terms of marginal adaptation in enamel and dentin, between the three ways of cavity preparation, or between the three restorative systems tested.
Materials and Methods
Seventy-two caries-free human molars were randomly assigned to nine experimental groups of equal size (Tables 1, 2). They were stored immediately after extraction in 0.1% thymol solution and ultrasonically cleaned and brushed with a rotative brush embedded in toothpaste (Signal RDA 50, Colgate-Palmolive, New York, NY, USA). The teeth were prepared for the simulation of dentinal fluid as earlier detailed by Krejci and co-workers (22). To this purpose, the apices were sealed with an adhesive system (OptiBond FL, KaVo Kerr, Orange, CA, USA), and the roots were fixed in the center of custom-made specimen holders using a cold polymerizing resin (Technovit 4071 resin cold curing, Kulzer, Hanau, Germany). Throughout the study, during cavity preparation, adhesive procedures related to cavity filling, and thermomechanical loading, teeth were flooded for simulation of dentin fluid with 1:3 diluted horse serum (donor horse serum, Bioswisstec, Schaffhausen, Switzerland) and phosphate-buffered saline (PBS, Bioswisstec, Schaffhausen, Switzerland), using a dentinal fluid simulation device (PAA Laboratories, Linz, Austria). Therefore, a cylindrical hole was drilled at the side surface of the tooth at the cementoenamel junction, and a metal tube (needle TERUMO with a diameter of 1.2 mm, Somerset, NJ, USA) was inserted, luted with an adhesive system (OptiBond FL), and then connected to a flexible silicone hose. On each tooth, one saucer-shaped class V cavity was prepared at the dentinoenamel junction under × 20 stereomicroscope magnification with a 2.94 μm Er:YAG laser (LiteTouch III, Light Instruments, Israel) in focus with a tip–tissue distance of 1–2 mm, a 9.3 μm CO2 laser (Solea 9.3 μm, Convergent Dental, USA) with a focus distance of about 10 mm—both measured once per sample with a periodontal probe and a 25 μm diamond bur in the shape of a football (see Table 1). The groups prepared with burs served as the positive control.
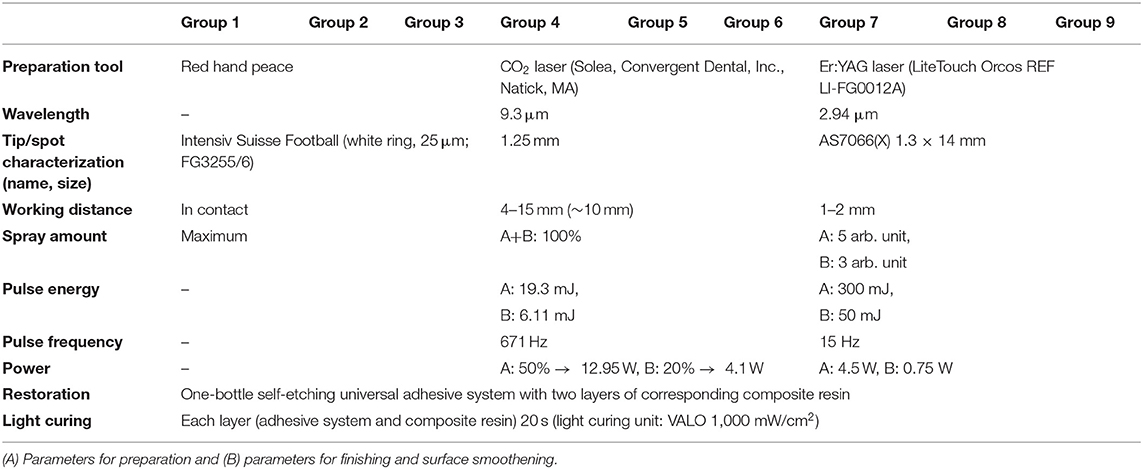
Table 1. Parameters used for the cavity preparation by the two lasers (Grades 4 to 9) and for the bur drilling (Grades 1 to 3).
Cavities were prepared at the dentinoenamel junction with standardized dimensions of 3.0–3.5 mm length by 2.5–3.0 mm height and a depth of 1.5 mm, which have been verified with a periodontal probe. The enamel half of the margin was beveled to a crescent shape with a maximal width of 1.2 mm (23).
Prepared teeth were air-dried for 5 s before bonding to three different adhesive systems following the manufacturer's instructions for the corresponding self-etch protocol and light-cured for 20 s with a distance of 1 mm (VALO Cordless standard power 1,000 mW/cm2, Ultradent, Salt Lake City, UT, USA) (Table 2). After bonding, cavities were filled with a corresponding universal composite in two oblique layers, and each was light-cured for 20 s. The first layer was placed in the cervical half of the cavity and the second one occlusally, completing obturation. Finishing and polishing were then performed with flexible disks (Sof-Lex, 3M, St. Paul, MN, USA), and for concave areas, rubber polishing tips (Brownie and Greenie, Shofu, Kyoto, Japan) were used under × 20 stereomicroscope magnification.
To obtain resin replicas of each restoration, impressions with a polyvinylsiloxane impression material (PRESIDENT light body, Coltène Whaledent, Altstätten, Switzerland) were taken after brush-cleaning the surface with toothpaste (Signal).
The restored teeth were then subjected to repeated thermal and mechanical stresses in a chewing machine (MW-Basis CH [230 V/50 Hz], JULABO Labortechnik GmbH, Seelbach, Germany), under constant simulation of dentin fluid flow (mechanical stress 200,000 × with max. 49 N and thermal stress between 5°C and 50°C) (7, 8).
After loading, replicas were taken again following the above-described procedures.
For the evaluation of marginal adaptation, replicas before and after aging were poured out with an epoxy resin (EpoFix, Struers, Willich, Germany), gold sputtered, and subjected to a quantitative marginal analysis in a scanning electron microscope under × 200 magnification (Zeiss Gemini, Sigma 300 VP, Karl Zeiss Microscopy, Cambridge, UK) and a custom-made module programmed within an image processing software (Scion Image, Scion Corp, Frederik, MA, USA) (7, 8, 24).
For statistical analysis of the normally distributed data, Kolmogorov–Smirnov and Shapiro–Wilk tests were run to assess normality assumption, and two repeated-measures analysis of variance (ANOVA) on the differences between data before and after loading were performed, the first one on enamel and dentin measurements of continuous margins (CMs) and the second on measurements of the entire margin length. Pairwise differences in group means were evaluated using Fisher's post hoc test. The level of confidence was set to 95%.
Results
Percentages of CMs (%CM) before and after aging (thermomechanical loading) on enamel, on dentin, and at the entire margin (total margin length [TML]) are presented in Table 3. Figures 1A–C demonstrate an example with a restoration after CO2 preparation with non-continuous (Figure 1B) and continuous (Figure 1C) margins. Significant differences before and after aging were observed between several experimental groups (p-values 1, 2, 3 in Table 3, statistically significant differences at p < 0.05).
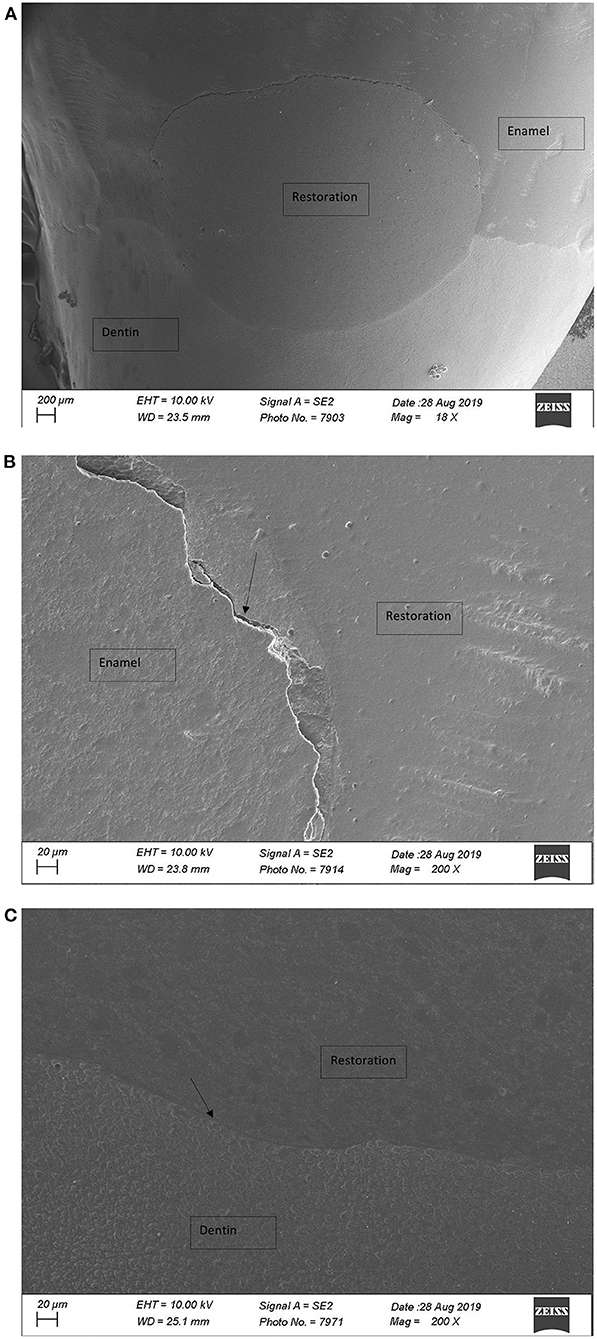
Figure 1. (A) Overview over restoration after CO2 laser preparation (×18; FE-SEM); non-CM in enamel and CM in dentin. (B) Restoration margin after CO2 laser preparation (×200; FE-SEM), non-CM. (C) Restoration margin after CO2 laser preparation (×200; FE-SEM), CM.
At the TML, marginal adaptation in the three bur-prepared groups (Groups 1, 2, and 3) and in one Er:YAG-prepared group (Group 7) was still above 80% after loading (Table 3).
Aging due to thermomechanical loading led to significant marginal degradation in all groups (Table 3 in Groups 5, 6, 8, and 9 p-values1 < 0.001; Group 1 p = 0.048, Group 3 p = 0.025, and Group 4 p = 0.004) except Group 2 (G-Premio BOND [GPB] in bur-prepared cavities) and Group 7 (Scotchbond Universal [SBU] in Er:YAG laser-prepared cavities); in these two groups, no significant differences could be detected between the results before and after aging.
With respect to the performance of the adhesive systems, SBU provided the most stable results with all three preparation methods (Figure 2A). Said differently, SBU was the only adhesive to perform well on bur-, CO2-, and Er: YAG-prepared cavities.
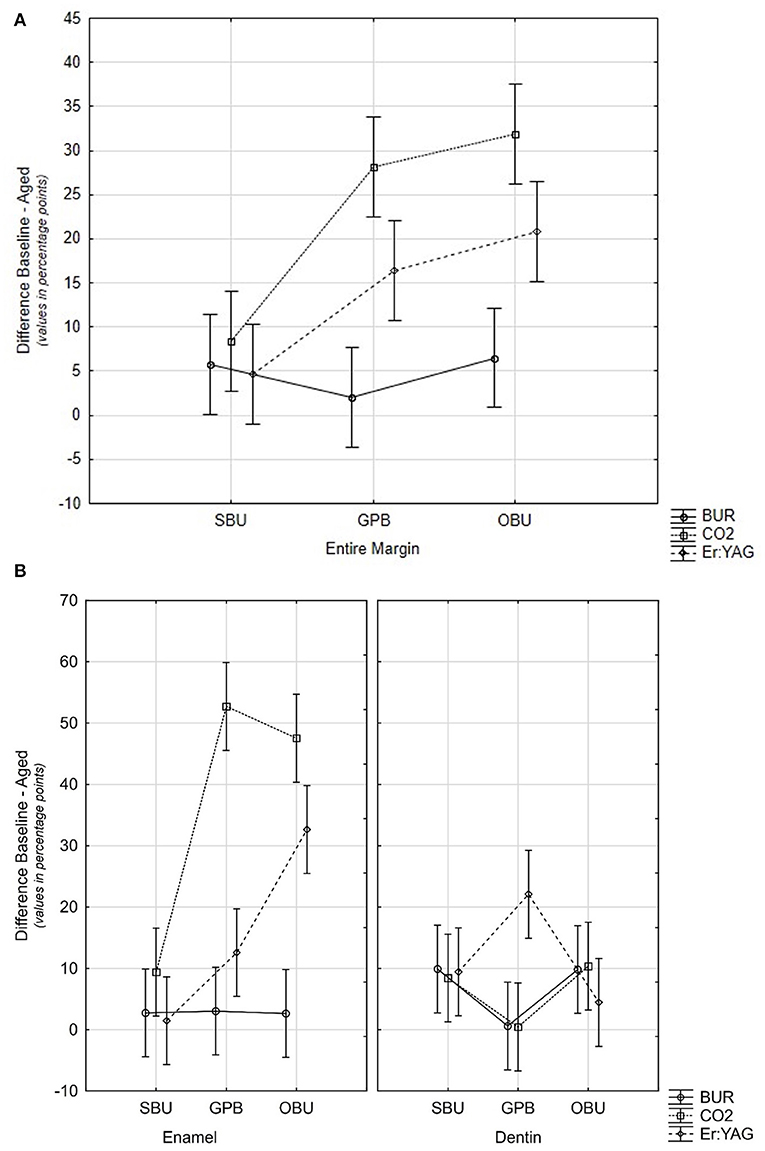
Figure 2. (A) Graph showing differences between baseline-loaded %CM at the entire margin (TML) for the different materials and for the different methods of cavity preparation. Confidence intervals including zero values of the y-axis are not statistically different. (B) Plot showing differences of baseline-loaded values in percentage points for materials and devices on enamel and dentin. Confidence intervals including zero values of the y-axis are not statistically different. SBU, Scotchbond Universal; GPB, G-Premio BOND; OBU, OptiBond Universal.
On enamel, both material and ablation devices as well as their interaction significantly influenced the %CMs (p < 0.001). Fatigue loading led to a significant decrease of marginal adaptation for all groups prepared with both lasers (Table 3 p-values2: Group 4 p = 0.009 and Groups 5, 6, 8, and 9 p < 0.001) with the exception of one group prepared by Er:YAG laser (Group 7) and bur-prepared ones. In these four groups, %CMs were still above 80% after aging. On enamel, SBU provided the most stable results for all three preparation devices (Figure 2B).
On dentin, aging and interaction of materials and ablation devices significantly influenced the performance of restorations in contrast to the preparation device alone (p < 0.0059). Fatigue loading in all three devices led to a significant reduction of the values in combination with specific materials. GPB in combination with bur and CO2 laser ablation as well as OptiBond Universal (OBU) with Er:YAG laser did not degrade significantly due to fatigue. However, median values of all bur and laser groups (except G4 SBU with CO2 laser) ranged between 80% and 100% of CM. Significant differences between materials could be seen with all preparation devices (see Figure 2B). The highest values of aged CMs have been attained with GPB and bur or CO2 laser ablation without significant reduction of CM values due to loading.
In Table 3, p-values4 show differences between aged enamel and dentin %CMs. Only Group 2 (GPB with bur) and Group 4 (SBU with CO2 laser) attained similar %CM in enamel and dentin. Groups 5, 6, and 9 with p-values <0.001, Group 7 with p = 0.08, Group 8 with p = 0.032, Group 1 with p = 0.023, and Group 3 with p = 0.057 are also close to statistically significant. Only bur groups (Groups 1–3) presented %CM above 80%, on both enamel and dentin margins.
Discussion
To attain well-tolerated restorations and ensure their long-term clinical success, challenges such as biocompatibility of materials and technique sensitivity must be faced, in addition to the presence of moisture in the working area. This is further influenced by chemical and micromorphology changes in dental surfaces induced by distinct ablation mechanisms of traditional bur, Er:YAG laser, and CO2 laser, as well as diversity in compositions and application recommendations of adhesive systems (5). Since one-component self-etch universal adhesive systems and lasers offer new possibilities in minimally invasive adhesive dentistry, this study aimed to analyze the influence of cavity preparation with an Er:YAG laser and a novel 9.3 μm CO2 laser combined with three one-component self-etch universal adhesives and to compare the quality of marginal adaptation reached with these lasers to conventional bur preparation. In view of the results of the present study, adhesive systems performed significantly differently, depending on the device used for tissue ablation. While all three one-component self-etching universal adhesives delivered high results of marginal adaptation on bur-prepared cavities, the Er:YAG laser performed distinctly depending on the adhesive system, and the 9.3 μm CO2 laser showed impaired adhesion for all tested materials especially on enamel. In this sense, the null hypotheses stating that all three ablation devices and adhesive systems would perform equally on both enamel and dentin before and after fatigue loading had to be rejected.
The testing protocol for this study was designed based on a previous publication, (7, 8) as well as on the parameters for Er:YAG and CO2 lasers provided by the manufacturers. The selection of the three one-component self-etch universal adhesives was based on differences in their principal attributes such as pH, functional monomers, solvents, initiator systems, and application methods. These characteristics may affect the bonding effectiveness due to their influence on hydrophily, wettability, viscosity, and conversion rate of resin monomers, which may consequently modify the stability and thickness of the hybrid layer (25–27).
Tooth sample preparation with dentinal fluid simulation was performed according to an established protocol (28) to ensure proximity to in vivo conditions and to avoid impaired interaction of laser and adhesive systems due to excessive tooth dehydration. With respect to fatigue test, mild forces of 49 N were applied to simulate chewing forces occurring in oral conditions for approximately 1 year (22, 29). The analysis of quality of marginal adaptation under SEM based on percentages of continuous restoration margins before and after thermal and mechanical loading/fatigue may indicate results for clinical long-term performances of the adhesive systems and may therefore be considered as closer and relevant to clinical reality (8, 30).
The discussion of the underlying results focuses on the interaction of different universal adhesive systems to dentin and enamel without specifically considering potential influences in terms of shrinkage stress due to differences between the resin composites between the experimental groups. Universal composites are in all groups accompanied by a resin composite from the same manufacturer's product line to avoid potential adverse interferences in combining different product lines. However, it should be pointed out that differences in the composite resin's composition might also influence results, especially in view of shrinking stress (31, 32).
In restorations where cavities were prepared with conventional burs, all three adhesive systems delivered %CMs higher than 80%. However, even in the bur-prepared cavities, especially in dentin-surrounded parts of the margins, statistically significant differences in %CMs were observed between the tested adhesives (see Figure 2B): GPB presented highly load-stable results, in contrast to SBU and OBU, which showed significantly lower but still high %CM values of over 80% after loading. These results might be explained by differences in composition and chemical behavior of the adhesive systems, as well as by their way of application as described below. Significantly different results in bur-prepared cavities after loading in dentin may be explained by the fact that dentin has a complex micromorphology, composition and heterogeneity of water, and organic and inorganic components, resulting in a more challenging substrate for adhesion than enamel, where results did not differ significantly.
Restorations where cavities were prepared by the 9.3 μm CO2 laser presented a poor marginal adaptation on enamel (Table 3 and Figures 1A–C). This might be explained by the laser ablation process itself, which may induce chemical and morphological alterations of the enamel. The wavelength of 9.3 μm has a strong absorption in hydroxyapatite, leading to important heating and water vaporization, decompensation of proteins, reduction of organic components, and melting as well as rapid recrystallization of the hard tissue with a loss of the carbonated phase and a change in the calcium/phosphate ratio, which makes enamel more acid resistant (33, 34). Enamel surfaces appeared glazed, probably due to high temperatures and melting of dental substrates. In a previous study, we examined the micromorphological changes of dental tissues under SEM (7). Enamel surfaces appeared homogenous and totally even without microroughness. We further found that the acidic monomers in the applied universal self-etching adhesive system (One-Coat 7 Universal from Coltène Whaledent) has not been able, in contrast to 35% phosphoric acid, to ablate this superficial laser-altered enamel layer (7). The underlying study with different universal adhesive systems that have diverse pH values confirms these findings. %CMs dropped significantly after the fatigue test, which may indicate an insufficient etching pattern by one-component self-etching universal adhesives (GPB and OBU) on the chemically modified and more acid-resistant surface (Figure 1B). These findings support the need of enamel etching with phosphoric acid before the application of a self-etching adhesive, at least after the preparation with the 9.3 μm CO2 laser, which is coherent with the available literature (7, 10). On CO2 laser-ablated enamel surfaces, SBU values decreased significantly due to loading but did not drop as much as in the GPB and the OBU groups (Table 3, Groups 5 and 6). The explanation for these findings might be related to a more stable chemical interaction of enamel with SBU than that with GPB and OBU. SBU, in contrast to the other two adhesives tested, contains polycarboxylic acids. Since the 1970s, polycarboxylate-based adhesive materials, such as glass ionomer cements (GICs), have been shown to bond chemically to dentin (35). Studies have found, for glass ionomer restoration of non-carious cervical lesions, higher retention rates compared to composite resins/adhesive combinations (16). The liquid of conventional GIC contains a copolymer of polyacrylic acid and other polyalkenoic acids, as is the case with SBU. The phosphate ions in the hydroxyapatite of enamel and dentin may thus be replaced by carboxyl groups of the polyalkenoic acids, which may lead to an ionic bond with the contained calcium. Polyalkenoic acids interact in the same way regardless of their concentration and pH (36, 37). This might explain the results obtained after loading with this adhesive. CO2 laser-prepared dentin presents a smear-layer-free surface with mainly open tubules, only partly occluded by drops of melted material. The surface does not appear glazed and completely even. As seen in a previous study, the micromorphological differences between bur-, ER:YAG laser-, and CO2 laser -ablated dentin is clearly less obvious than those in enamel (7). This might be an explanation for the findings of this study that values for CM in dentin showed comparable results to bur and Er:YAG laser preparations (Table 3 and Figure 1C). Within the initial dentin values, SBU showed significantly lower values with both lasers than with bur preparations. This leads to the assumption that the establishment of mechanical interlock with resin tags might have been impaired in these groups (Groups 4 and 7). The differences between laser and bur preparations in dentin may be explained by the laser-promoted dentin fluid exudation due to opening of dentinal tubules caused by smear layer removal and the chemical interaction of the consequently higher amount of liquid to the adhesive systems. Bur preparation produces a smear layer that blocks dentinal tubules and might therefore reduce dentinal fluid exudation (7).
Marginal adaptation of Er:YAG laser-prepared restorations attained high %CMs. High initial values of CMs in enamel and dentin obtained with all three adhesive systems might have been due to the advantageous rough micromorphology of laser-ablated surfaces (7). Values after fatigue did not drop significantly in enamel with SBU, in contrast to GPB and OBU, which may be explained by impaired interaction of adhesive systems to chemically changed substrates due to laser ablation as described above. Results were stable in dentin for OBU but showed a significant decrease after loading for SBU and GPB. Initial dentin values of the SBU group showed already lower %CMs in comparison to the other two products, as already seen within the CO2 laser groups. Reduction of dentin values due to loading may also be explained with the laser-specific dentin micromorphology with open dentinal tubules and therefore pronounced dentin fluid exudation that may interact with the adhesive system. According to previous investigations, this effect might be more important for Er:YAG than for CO2 laser, as Er:YAG does not melt the substrate (7). The CO2 laser leads to sealing and formation of recrystallized droplets which partly occlude dentinal tubules. Thus, the liquid exudation might be even more important in the Er:YAG laser groups than in the CO2 groups. However, this does not explain the significantly different behavior of GBP and OBU between both laser groups (7). Nevertheless, one possible explanation for the lower values after loading of SBU and especially GPB might be, next to the laser-specific dentin properties, the adhesive system's water content. Literature reports the double amount of water in GPB in comparison to SBU, which might be more difficult to evaporate in the microporosities of Er:YAG laser dentin (19). It may be even further speculated that the more acidic GPB compared to SBU and OBU demineralizes deeper on the already laser-etched surface with its microporosities where the adhesive system itself does not penetrate entirely, thus producing an incomplete hybrid layer, potentially explaining its lower resistance to loading (38).
The pH value of the adhesives may be considered as one major factor influencing adhesion, due to its influence on the extent of demineralization and dissolution of the smear layer. Nevertheless, in view of the different performance of the three adhesive systems, our results did not seem to depend on the pH (39, 40). There is no supporting evidence that a lower pH of the adhesive systems will improve the material's adhesion to enamel (and to dentin), as both mild adhesive systems (41), OBU (pH 2.1) and SBU (pH 2.7), performed equally well in bur groups before and after thermomechanical loading in enamel and performed better than the intermediate one, GPB (pH 1.5). The more load-stable performance of SBU might further be explained by its solvents ethanol and butanone, compared to OBU and GPB whose solvents are based on water and acetone (see Table 2). Solvents such as acetone or ethanol are added to enhance monomer miscibility into one solution and to accelerate water elimination from the adhesive surface. Evaporation of these solvents may lead to phase separation of the water (adding to the dissociation of the acidic monomers and thus for etching) from the other adhesive ingredients due to changes in the solvent–monomer balance, leading to water droplet formation at the bottom of the adhesive layer, adjacent to the hybrid layer, and potentially enhancing low fatigue resistance (42, 43). Literature reports that due to the low vapor pressure, water droplets moved upwards and evaporated completely after 4 to 10 min of application time. Since the vapor pressure (at 25°C) for acetone is four times higher than that for ethanol, acetone is more volatile, potentially leading to more water-remnant droplets and thus instabilities and interferences with the polymerization reaction, causing blister formation or intensifying permeability of the adhesive layer, triggering hydrolytic degradation of polymers and collagen, which is potentiated by the acidic pH of the monomer (44–49). It seems therefore to be crucial, next to a coordinated timing of solvent evaporation, to accelerate and improve water droplet removal and evaporation with strong air-drying of the adhesive system prior to polymerization, as acetone is evaporated much faster than water (50). These facts may support the hypothesis that differences in recommendations for air-drying may be at least partly responsible for the distinct performance of OBU and GPB in dentin. Literature reports a significant improvement of self-etching universal adhesives by increasing the time for air-drying from 5 to 15 s but also by varying the air pressure (51, 52). This may lead to the suspicion that OBU performs better with prolonged air-drying, as already recommended for GPB by its manufacturer. Furthermore, excessive air-drying (as recommended for GPB) helps to thin the adhesive layer, which is reported to have a positive impact on water and solvent evaporation and polymerization shrinkage as well as on stress concentration, potentially improving bond strength (53).
Besides the differences in solvent agents and application instructions, monomer type may influence the interaction between the adhesive and the substrate. SBU contains Bis-GMA, in contrast to the other two adhesive systems tested. Ethanol and Bis-GMA tend to make the material more viscous than acetone and TEGDMA. The lower volatility and diffusivity of Bis-GMA may hinder infiltration into dentin, but on the other side, it may improve the photopolymerization and conversion rate due to autoacceleration. Moreover, Bis-GMA is known for its low volumetric shrinkage, high reactivity, and good mechanical properties, which might explain the relatively high stability or resistance to loading of SBU to all dental surfaces independent from the preparation device (54). Another aspect is that SBU and GPB are based on an MDP monomer, which is reported to provide stable adhesion due to ionic bonds formed with the calcium ions of the hydroxyapatite crystals and covalent bonds of the phosphate groups in MDP with the corresponding phosphate groups of hydroxyapatite crystals resulting in insoluble salts. The continuous deposition of successive coats of these salts on the outer surface of the hydroxyapatite crystals is a process known as “nanolayering” and might explain the superior performance of SBU and GPB over OBU, which contains a GPDM-P monomer (glycerophosphate dimethacrylate) instead of MDP (55–58).
Another factor influencing adhesives' performance might be related to photoinitiators. The main photoinitiator used in methacrylate-based materials currently marketed is camphorquinone, a hydrophobic substance that may contribute to phase separation when polymerization occurs in an aqueous medium, leading to decreased longevity and biocompatibility of adhesion (59, 60). SBU and OBU do both contain camphorquinone, but in contrast to OBU, SBU is not based on water and includes additional photoinitiations, which may contribute to more stable adhesion results. The water-based GPB uses diphenylphosphinoxid as an initiator system, probably preventing phase-separation phenomena due to its distinct characteristics in comparison to the hydrophobic character of camphorquinone.
Finally, in view of the results of this study, it appears still necessary either to improve adhesive formulations or to modify their application protocol on laser-prepared substrates. Air-drying time and pressure, which seem to be material dependent, deserve special attention. Performance of adhesive systems might be improved on Er:YAG laser-prepared enamel and dentin as well as on CO2 laser-prepared dentin by longer and stronger air-drying. Improvements on CO2 laser-ablated enamel might be reached by a precedent etching step with highly concentrated phosphoric acid. Future research should also focus on the interaction of dentinal fluid and the specific composition of adhesive materials.
Conclusions
The results of this study lead to the conclusion that among the three universal adhesives, SBU showed the highest values of continuous marginal adaptation when applied on bur-, 9.3 μm CO2 laser-, and Er:YAG laser-prepared cavities. This evidenced the capacity of this universal adhesive to deal with bur- and laser-prepared enamel and dentin substrates.
The two universal adhesives GPB and OBU did not provide consistent results on enamel and dentin. Results were highly dependent on the preparation method of enamel and dentin.
In bur-prepared cavities, the three self-etching universal adhesive systems delivered results of marginal adaptation between 80 and 100% CM after loading, on both enamel and dentin margins.
Er:YAG laser may represent a valuable alternative to conventional bur preparation, but only if used in combination with SBU.
The quality of marginal adaptation delivered by the 9.3 μm CO2 laser was inferior on enamel in comparison to dentin. The specific interaction of this laser with the enamel surface may have adversely affected adhesion to this substrate.
Data Availability Statement
The raw data supporting the conclusions of this article will be made available by the authors, without undue reservation.
Author Contributions
CA and IK contributed to conception and design of the study. CA performed the laboratory work and organized the data as well as wrote the first draft of the manuscript. ED performed the statistical analysis. TB and ED wrote sections of the manuscript. All authors contributed to manuscript revision, read, and approved the submitted version.
Conflict of Interest
The authors declare that the research was conducted in the absence of any commercial or financial relationships that could be construed as a potential conflict of interest.
Acknowledgments
We would like to thank Mr. Antonio Baccio, Ms. Isaline Rossier, and Ms. Luciana Caseiro for their assistance during the practical part of this protocol, as well to the dental companies 3M, GC, and Kerr for providing the materials and to Convergent Dental for providing the laser device.
References
1. Van Meerbeek B, De Munck J, Yoshida Y, Inoue S, Vargas M, Vijay P, et al. Buonocore memorial lecture. Adhesion to enamel and dentin: current status and future challenges. Oper Dent. (2003) 28:215–35.
2. Buonocore MG. A simple method of increasing the adhesion of acrylic filling materials to enamel surfaces. J Dent Res. (1955) 34:849–53. doi: 10.1177/00220345550340060801
3. Van Meerbeek B, Yoshihara K, Yoshida Y, Mine A, De Munck J, and Van Landuyt KL. State of the art of self-etch adhesives. Dent Mater. (2011) 27:17–28. doi: 10.1016/j.dental.2010.10.023
4. Pashley DH, Tay FR, Breschi L, Tjaderhane L, Carvalho RM, Carrilho M, et al. State of the art etch-and-rinse adhesives. Dent Mater. (2011) 27:1–16. doi: 10.1016/j.dental.2010.10.016
5. McLean DE, Meyers EJ, Guillory VL, and Vandewalle KS. Enamel bond strength of new universal adhesive bonding agents. Oper Dent. (2015) 40:410–7. doi: 10.2341/13-287-L
6. Nagarkar S, Theis-Mahon N, and Perdigão J. Universal dental adhesives: current status, laboratory testing, and clinical performance. J Biomed Mater Res Part B. (2019) 107:2121–31. doi: 10.1002/jbm.b.34305
7. Antony Otero C, Di Bella E, Bortolotto T, and Krejci I. Influence of 9.3 μm CO2 and Er:YAG laser preparations on marginal adaptation of adhesive mixed class V composite restorations with one component universal adhesive. Am J Dent. (2021) 34:31–38.
8. Bader C, and Krejci I. Marginal quality in enamel and dentin after preparation and finishing with an Er:YAG laser. Am J Dent. (2006) 19:337–42.
9. Zhang Y, and Jiang A. The influence of Er:YAG laser treatment on the shear bond strength of enamel and dentin: a systematic review and meta-analysis. Quintessence Int. (2020) 51:8–16. doi: 10.3290/j.qi.a43648
10. Rechmann P, Bartolome N, Kinsel R, Vaderhobli R, and Rechmann BMT. Bond strength of etch-and-rinse and self-etch adhesive systems to enamel and dentin irradiated with a novel CO2 9.3 mum short-pulsed laser for dental restorative procedures. Lasers Med Sci. (2017) 32:1981–93. doi: 10.1007/s10103-017-2302-y
11. Esteves-Oliveira M, Zezell DM, Apel C, Turbino ML, Aranha AC, Eduardo Cde P, et al. Bond strength of self-etching primer to bur cut, Er,Cr:YSGG, and Er:YAG lased dental surfaces. Photomed Laser Surg. (2007) 25:373–80. doi: 10.1089/pho.2007.2044
12. Malkoc MA, Tasdemir ST, Ozturk AN, Ozturk B, and Berk G. Effects of laser and acid etching and air abrasion on mineral content of dentin. Lasers Med Sci. (2011) 26:21–7. doi: 10.1007/s10103-009-0751-7
13. Ramos TM, Ramos-Oliveira TM, Moretto SG, de Freitas PM, Esteves-Oliveira M, and de Paula Eduardo C. Microtensile bond strength analysis of adhesive systems to Er:YAG and Er,Cr:YSGG laser-treated dentin. Lasers Med Sci. (2014) 29:565–73. doi: 10.1007/s10103-012-1261-6
14. Bader C, and Krejci I. Indications and limitations of Er:YAG laser applications in dentistry. Am J Dent. (2006) 19, 178–86
15. Rizcalla N, Bader C, Bortolotto T, and Krejci I. Improving the efficiency of an Er:YAG laser on enamel and dentin. Quintessence Int. (2012)43:153–60.
16. Peumans M, Kanumilli P, De Munck J, Van Landuyt K, Lambrechts P, and Van Meerbeek B. Clinical effectiveness of contemporary adhesives: a systematic review of current clinical trials. Dent Mater. (2005) 21:864–81. doi: 10.1016/j.dental.2005.02.003
17. da Silva EM, de Sa Rodrigues CU, de Oliveira Matos MP, de Carvalho TR, dos Santos GB, and Amaral CM. Experimental etch-and-rinse adhesive systems containing MMP-inhibitors: physicochemical characterization and resin-dentin bonding stability. J Dent. (2015) 43:1491–7. doi: 10.1016/j.jdent.2015.10.004
18. Rosa WL, Piva E, and Silva AF. Bond strength of universal adhesives: a systematic review and meta-analysis. J Dent. (2015) 43:765–76. doi: 10.1016/j.jdent.2015.04.003
19. Yamauchi K, Tsujimoto A, Jurado CA, Shimatani Y, Nagura Y, Takamizawa T, et al. Etch-and-rinse vs self-etch mode for dentin bonding effectiveness of universal adhesives. J Oral Sci. (2019) 61:549–53. doi: 10.2334/josnusd.18-0433
20. Papadogiannis D, Dimitriadi M, Zafiropoulou M, Gaintantzopoulou MD, and Eliades G. Universal Adhesives: setting characteristics and reactivity with dentin. Materials (Basel). 12 (2019) 12:1720. doi: 10.3390/ma12101720
21. Foscaldo T, Dos Santos GB, Miragaya LM, Garcia M, Hass, and da Silva VEM. Effect of HEMA phosphate as an alternative to phosphoric acid for dentin treatment prior to hybridization with etchand-rinse adhesive systems. J Adhes Dent. (2016) 18:425–434. doi: 10.3290/j.jad.a36891
22. Krejci I, Kuster M, and Lutz F. Influence of dentinal fluid and stress on marginal adaptation of resin composites. J Dent Res. (1993) 72:490–4. doi: 10.1177/00220345930720020401
23. Bortolotto T, Ferrari M, Onisor I, and Tay Krejci FI. Marginal adaptation of contemporary dentin bonding agents in enamel and dentin under the simulation of dentinal fluid. Int Dent S Afr. (2005) 7:46–56.
24. Bortolotto T, Monaco C, Onisor I, and Krejci I. In vitro assessment of single-retainer tooth-colored adhesively fixed partial dentures for posterior teet. Int J Dent. (2010) 2010:101095. doi: 10.1155/2010/101095
25. Van Meerbeek B, Van Landuyt K, De Munck J, Hashimoto M, Peumans M, Lambrechts P, et al. Technique-sensitivity of contemporary adhesives. Dent Mater J. (2005) 24:1–13. doi: 10.4012/dmj.24.1
26. Marchesi G, Frassetto A, Mazzoni A, Apolonio F, Diolosa M, Cadenaro M, et al. Adhesive performance of a multi-mode adhesive system: 1-year in vitro study. J Dent. (2014) 42:603–12. doi: 10.1016/j.jdent.2013.12.008
27. Tay FR, Pashley DH, King NM, Carvalho RM, Tsai J, Lai SC, and Marquezini L Jr. Aggressiveness of self-etch adhesives on unground enamel. Oper Dent. (2004) 29:309–16.
28. Krejci I, Hàusler T, Sägesser, and Lutz DF. New adhesives in Class V restorations under combined load and simulated dentinal fluid. Dent Mater. (1994) 10:331–5. doi: 10.1016/0109-5641(94)90042-6
29. Bortolotto T, Ferrari M, Tay F, and Krejci I. Degradation of thermo-mechanically loaded adhesive Class V restorations after 18 months of water storage. Am J of Dent. (2007) 20:83–9.
30. Roebuck EM, Whitters CJ, and Saunders WP. The influence of three Erbium:YAG laser energies on the in vitro microleakage of Class V compomer resin restorations. Int J Paediatr Dent. (2001) 11:49–56. doi: 10.1046/j.1365-263x.2001.00230.x
31. Braga RR, Ballester RY, and Ferracane JL. Factors involved in the development of polymerization shrinkage stress in resin-composites: a systematic review. Dent Mater. (2005) 21:962–70. doi: 10.1016/j.dental.2005.04.018
32. Steiner R, Edelhoff D, Stawarczyk B, Dumfahrt H, and Lente I. Effect of dentin bonding agents, various resin composites and curing modes on bond strength to human dentin. Materials (Basel). (2019) 12:3395. doi: 10.3390/ma12203395
33. Featherstone JD. Modeling the modification depth of carbon dioxide laser-treated dental enamel. Laser Surg Med. (1999) 25:335–47. doi: 10.1002/(sici)1096-9101(1999)25:4 <335::aid-lsm8>3.0.co;2-f
34. He Z, Chen L, Hu X, Shimada Y, Otsuki M, and Tagami J. Mechanical properties and molecular structure analysis of subsurface dentin after Er:YAG laser irradiation. J Mech Behav Biomed Mater. (2017) 74:274–82. doi: 10.1016/j.jmbbm.2017.05.036
35. Wilson D, and Kent BE. A new translucent cement for dentistry. The glass ionomer cement. Br Dent J. (1972) 132:133–5. doi: 10.1038/sj.bdj.4802810
36. Yoshida Y, Van Meerbeek B, Nakayama Y, Yoshioka M, Snauwaert J, Abe Y, et al. Adhesion to and decalcification of hydroxyapatite by carboxylic acids. J Dent Res. (2001) 80:1565–9. doi: 10.1177/00220345010800061701
37. Sezinando A, Serrano ML, Pérez VM, Muñoz RA, Ceballos L, and Perdigao J. Chemical adhesion of polyalkenoate-based adhesives to hydroxyapatite. J Adhes Dent. (2016) 18:257–65. doi: 10.3290/j.jad.a36222
38. Isolan P, Münchow EA, Basso GR, Pimentel AH, Schwantz JK, da Silva AV, et al. Bond strength of a universal bonding agent and other contemporary dental adhesives applied on enamel, dentin, composite, and porcelain. App Adhes Sci. (2014) 2:25. doi: 10.1186/s40563-014-0025-x
39. Thanatvarakorn O, Prasansuttiporn T, Takahashi M, Thittaweerat S, Foxton RM, Ichinose S, et al. Effect of scrubbing technique with mild self-etching adhesives on dentin bond strengths and nanoleakage expression. J Adhes Dent. (2016) 18:197–204. doi: 10.3290/j.jad.a36033
40. Tay FR, and Pashley DH. Aggressiveness of contemporary self-etching systems. I: Depth of penetration beyond dentin smear layers. Dent Mater. (2001) 17:296–308. doi: 10.1016/S0109-5641(00)00087-7
41. Miyazaki M, Tsujimoto A, Tsubota K, Takamizawa T, Kurokawa H, and Platt JA. Important compositional characteristics in the clinical use of adhesive systems. J Oral Sci. (2014) 56:1–9. doi: 10.2334/josnusd.56.1
42. Van Landuyt KL, Snauwaert J, De Munck J, Peumans M, Yoshida Y, Poitevin A, et al. Systematic review of the chemical composition of contemporary dental adhesives. Biomaterials. (2007) 28:3757–85. doi: 10.1016/j.biomaterials.2007.04.044
43. Moszner N, Salz U, and Zimmermann J. Chemical aspects of self-etching enamel-dentin adhesives: a systematic review. Dent Mater. (2005) 21:895–910. doi: 10.1016/j.dental.2005.05.001
44. Luque-Martinez V, Perdigao J, Munoz MA, Sezinando A, Reis A, and Loguercio AD. Effects of solvent evaporation time on immediate adhesive properties of universal adhesives to dentin. Dent Mater. (2014) 30:1126–35. doi: 10.1016/j.dental.2014.07.002
45. Chen L, and Suh BI. Effect of hydrophilicity on the compatibility between a dual-curing resin cement and one-bottle simplified adhesives. J Adhes Dent. (2013) 15:325–31. doi: 10.3290/j.jad.a28604
46. Salz U, Zimmermann J, Zeuner F, and Moszner N. Hydrolytic stability of self-etching adhesive systems. J Adhes Dent. (2005) 7:107–16. doi: 10.3290/j.jad.a10282
47. Van Landuyt KL, De Munck J, Snauwaert J, Coutinho E, Poitevin A, Yoshida Y, et al. Monomer-solvent phase separation in one-step self-etch adhesives. J Dent Res. (2005) 84:183–8. doi: 10.1177/154405910508400214
48. Pashley EL, Zhang Y, Lockwood PE, Rueggeberg FA, and Pashley DH. Effects of HEMA on water evaporation from water-HEMA mixtures. Dent Mater. (1998) 14:6–10. doi: 10.1016/S0109-5641(98)00003-7
49. Cho BH, and Dickens SH. Effects of the acetone content of single solution dentin bonding agents on the adhesive layer thickness and the microtensile bond strength. Dent Mater. (2004) 20:107–15. doi: 10.1016/S0109-5641(03)00071-X
50. Golbari N, Kasraei S, Afrasiabi A, Mostajir E, and Mojahedi SM. Effect of diode laser (810 nm) irradiation on marginal microleakage of multi-mode adhesive resins in class V composite restorations. J Lasers Med Sci. (2019) 10:275–82. doi: 10.15171/jlms.2019.45
51. Franz, Lettner S, Watts DC, Graf A, Moritz A, and Schedle A. Analysis of pre-test failures and bond-strengths of seven adhesive systems to bovine dentine: a nine-year novice/beginner operator study. Dent Mater. (2018) 34:1599–609. doi: 10.1016/j.dental.2018.09.009
52. Moosavi H, Forghani M, and Managhebi E. Effect of different air-drying time on the microleakage of single-step self-etch adhesives. Restor Dent Endod. (2013) 38:73–8. doi: 10.5395/rde.2013.38.2.73
53. Jain M, Shetty S, Mogra S, Shetty VS, and Dhakar N. Determination of optimum adhesive thickness using varying degrees of force application with light-cured adhesive and its effect on the shear bond strength of orthodontic brackets: an in vitro study. Orthodontics (Chic.). (2013) 14:e40–9. doi: 10.11607/ortho.919
54. Cornelio RB, Wikant A, Mjosund H, Kopperud HM, Haasum J, Gedde UW, et al. The influence of bis-EMA vs bis GMA on the degree of conversion and water susceptibility of experimental composite materials. Acta Odontol Scand. (2014) 72:440–7. doi: 10.3109/00016357.2013.856467
55. Cardenas AM, Hass V, Malaquias P, Gutierrez MF, Reis A, Perdigão J, et al. Effect of MDP-containing silane and adhesive used alone or in combination on the long-term bond strength and chemical interaction with lithium disilicate ceramics. J Adhes Dent. (2017) 19:203–12. doi: 10.3290/j.jad.a38414
56. Yoshihara K, Yoshida Y, Nagaoka N, Hayakawa S, Okihara T, De Munck J, et al. Adhesive interfacial interaction affected by different carbon-chain monomers. Dent Mater. (2013) 29:888–97. doi: 10.1016/j.dental.2013.05.006
57. Elkaffas HH, Hamama H, and Mahmoud SH. Do universal adhesives promote bonding to dentin? A systematic review and meta-analysis. Restor Dent Endod. (2018) 43:e29. doi: 10.5395/rde.2018.43.e29
58. Cuevas-Suarez E, Ramos TS, Rodrigues SB, Collares FM, Zanchi CH, Lund RG, et al. Impact of shelf-life simulation on bonding performance of universal adhesive systems. Dent Mater. (2019) 35:e204–19. doi: 10.1016/j.dental.2019.05.023
59. Spencer P, and Wang Y. Adhesive phase separation at the dentin interface under wet bonding conditions. J Biomed Mater Res. (2002) 62:447–56. doi: 10.1002/jbm.10364
Keywords: dental hard tissue preparation, marginal adaptation, adhesion, Er:YAG laser, CO2 laser, laser cavity preparation, universal adhesive system
Citation: Anton y Otero CI, Di Bella E, Krejci I and Bortolotto T (2021) Effect of 9.3 μm CO2 and 2.94 μm Er:YAG Laser vs. Bur Preparations on Marginal Adaptation in Enamel and Dentin of Mixed Class V Cavities Restored With Different Restorative Systems. Front. Dent. Med. 2:668056. doi: 10.3389/fdmed.2021.668056
Received: 15 February 2021; Accepted: 01 April 2021;
Published: 17 May 2021.
Edited by:
Vanessa Cavalli, State University of Campinas, BrazilReviewed by:
Bruna Marin Fronza, University of São Paulo, BrazilRodrigo Lins, State University of Paraíba, Brazil
Copyright © 2021 Anton y Otero, Di Bella, Krejci and Bortolotto. This is an open-access article distributed under the terms of the Creative Commons Attribution License (CC BY). The use, distribution or reproduction in other forums is permitted, provided the original author(s) and the copyright owner(s) are credited and that the original publication in this journal is cited, in accordance with accepted academic practice. No use, distribution or reproduction is permitted which does not comply with these terms.
*Correspondence: Clara Isabel Anton y Otero, Y2xhcmEuYW50b255b3Rlcm9AdW5pZ2UuY2g=; Ivo Krejci, SXZvLktyZWpjaUB1bmlnZS5jaA==