- 1Melbourne Veterinary School, The University of Melbourne, Werribee, VIC, Australia
- 2Australian Rickettsial Reference Laboratory, University Hospital Geelong, Geelong, VIC, Australia
- 3School of Life and Environmental Sciences, Deakin University, Burwood, VIC, Australia
- 4Conservation Department, Phillip Island Nature Parks, Cowes, VIC, Australia
- 5Zoos Victoria, Parkville, VIC, Australia
The Australian fur seal (Arctocephalus pusillus doriferus) has experienced a slower than expected population recovery since the end of the commercial sealing era, with a high third trimester abortion rate. There is currently no known proximate cause. Coxiella burnetii (Cb) is a well-known cause of abortion in domestic and wildlife species and an important zoonotic pathogen. It has been recorded from a small number of northern hemisphere marine mammals and may be a potential contributory factor to decreasing populations of northern fur seals (Callorhinus ursinus) and Steller sealions (Eumetopias jubatus). It has not been recorded from marine mammals in the southern hemisphere but is well documented in ruminants and wildlife in Australia as a cause of reproductive failure. Third trimester aborted fetuses (n = 46) and full-term placentas (n = 66) from Australian fur seals, were collected on Kanowna Island and Seal Rocks in Bass Strait, south-eastern Australia. Utilizing routine hematoxylin and eosin histopathology, Cb immunohistochemistry and two different qPCR targets–htpAB and com1, Cb was identified. Routine histopathology and immunohistochemistry were insensitive for the detection of Cb. The detected Cb prevalence ranged from 10.6% for com1 up to 40.9% with htpAB. Coxiella burnetti was readily detected in full-term placentas but in aborted fetal material only in a single placenta associated with a still birth. The exact significance is currently unclear, but this highlights that Cb is present in Australian fur seals, breeding in Bass Strait. Bass Strait is in one of the world’s fastest warming oceanic regions and marine mammals breeding in the area are likely to be key indicators of marine ecosystem stressors. This first description of Cb in a marine mammal from the southern hemisphere, highlights the need to further investigate the potential risks this pathogen poses to Australian fur seals and sympatric marine mammals. Additionally, it is important to determine the zoonotic risk of this pathogen to persons working with, and in proximity of, Australian fur seal breeding colonies.
Introduction
There are many marine mammal populations that have recovered significantly since the shift toward conservation rather than exploitation. This has been most obvious in the pinnipeds. It appears that their near-shore habitat use and relatively shorter and faster life cycles compared to cetaceans, favor their recovery (Magera et al., 2013). Worldwide, most fur seal populations have displayed an annual recovery rate of 10–15% since bans on commercial harvesting were legislated (Wickens and York, 1997). However, this has not been the case for some species, such as the Australian fur seal (Arctocephalus pusillus doriferus, a benthic forager) (Arnould and Costa, 2006).
Originally, it was postulated that benthic foraging pinnipeds are faced with additional stressors (i.e., energetic expenditure and nutritional composition of benthic prey) that their pelagic foraging counterparts are not exposed to (Trites and Donnelly, 2003), potentially explaining the reduced recovery of certain pinnipeds (Rosen and Trites, 2005). More specifically, this “nutritional stress hypothesis” was considered as potentially the reason for lower fecundity in benthic foragers such as the Steller sea lion (Eumetopias jubatus), Australian sea lion (Neophoca cinerea), and the Australian fur seal (Costa and Gales, 2003; Trites and Donnelly, 2003; Gibbens et al., 2010). Recent studies of the critically endangered Galapagos sea lion (Zalophus wollebaeki) suggest, however, that benthic foraging modes are not necessarily less efficient than pelagic foraging (Blakeway et al., 2021). This then raises the question as to what other factors could be having a significant impact on the ability of the species such as the Australian fur seal to fully recover their population?
The breeding distribution of Australian fur seals is restricted to south-eastern Australia between 32°28′–43°52′ S and 142°00′–152°33′ E, including a limited number of islands within Bass Strait (McIntosh et al., 2018). They were locally extirpated from 17 of their 26 known historical colonies within Australia by the time they got full legislative protection in 1975 (Lancaster et al., 2010). Currently there are only nine regular breeding sites within Bass Strait (McIntosh et al., 2018). It was noted in certain colonies that the number of viable pups being produced each season declined after 2007 (McIntosh et al., 2018). This does not appear to be the case across all colonies but considering that Australian fur seals have shown much slower recovery than other fur seal species (Gibbens et al., 2010), it is possible that low recruitment from an unknown factor may be contributing to the slow recovery of this species (Pemberton and Gales, 2004).
Initial research has identified multiple possible causes for low recruitment, including environmental toxicants such as per-and polyfluoroalkyl substances (PFAS) (Taylor et al., 2021). However, the influence of infectious and toxicological agents, nutritional deficiencies, climate change and other factors has yet to be fully investigated. There has been a single study in which antibodies to Brucella sp. were detected in adult female Australian fur seals (Lynch et al., 2011a). In terrestrial mammals, brucellosis is a serious cause of reproductive failure and abortion but in marine mammals the literature on its pathogenicity is sparse. The organism has not definitively been detected in Australian fur seals and the seroprevalence indicated low levels of this abortifacient pathogen in circulation (Lynch et al., 2011a). One of the only other pathogens associated with reproductive failure that has been identified in Australian fur seals is Mycoplasma but, at present, there is no substantial evidence that it plays an important role in reduced fecundity (Lynch et al., 2011b).
Coxiella burnetii (Cb) was first described in Australia in 1937 as the causative agent of human disease, Query-fever or Q-fever (Eldin et al., 2017). This intracellular gram-negative bacterium has been associated with a broad range of hosts, including domestic animals and wildlife (González-Barrio and Ruiz-Fons, 2019). Although it is an obligate intracellular pathogen, it has two distinct growth forms. The intracellular form is known as the large cell variant (LCV), but once the organism is shed in the reproductive secretions, the organism transforms into a highly resistant condensed form known as the small cell variant (SCV), which is the most infective form of Cb (Abeykoon et al., 2021). In domestic ruminants, Cb has been noted to cause significant reproductive failure including abortion, still-birth, premature birth and weak full-term birth (Agerholm, 2013). It is considered an organism of significant zoonotic risk with the ability to be dispersed as an environmentally resistant aerosol that can remain infective for decades (Bond et al., 2018).
Although infection tends to be localized and with distinct patterns in various geographic regions, occasionally it can occur as large disease outbreaks (Eldin et al., 2017). In humans and domestic animals, the effects of a disease outbreak can be catastrophic, as was the case in Netherlands epidemic (2005–2009) with nearly 4000 human cases reported as a result of aerosols from infected goat herds (Roest et al., 2011). It is often less dramatic in humans, producing mostly influenza-like symptoms with a roughly 1% case fatality rate (Bond et al., 2018). C. burnetii is an important emerging disease in wildlife (González-Barrio and Ruiz-Fons, 2019), but despite Cb being well described in domestic animals and humans, data on its significance in wildlife are sparse in comparison, with very little literature available on the significance of infection in marine mammals. C. burnetii has been detected in the placental tissue of a few species of northern hemisphere marine mammals including harbor seals (Phoca vitulina), harbor porpoises (Phocoena phocoena), Steller sea lions and northern fur seals with the first report in the literature in 1999 (Lapointe et al., 1999; Duncan et al., 2012; Kersh et al., 2012). Whether it poses a significant risk as a pathogen of marine mammals is still uncertain, though it has been associated with declining populations of northern fur seal and Steller sea lion (Minor et al., 2013).
The aims of this study were to investigate whether Cb, a significant cause of reproductive failure in terrestrial mammals, could play a contributory role to low fecundity in Australian fur seals. Aborted fetuses, still births and full-term placentas from two of the largest Victorian breeding colonies were screened histologically and molecularly for the presence of Cb over a period of 3 years.
Materials and Methods
Sample Collection
Spontaneously aborted third trimester Australian fur seal fetuses were purposely collected on Kanowna Island (39°15′48″S; 146°31′04″E) (n = 27) between July and October in 2019 and 2020 and opportunistically on Seal Rocks (38°31′34″S; 145°05′59″E) (n = 19) between August and October in 2018, 2019, and 2020. The Seal Rocks colony consists of approximately 18,400 individuals and is currently the largest for the species (McIntosh et al., 2018). Kanowna Island hosts a colony of approximately 13,000 individuals (Kirkwood et al., 2010) which is currently considered to be the third largest for the species (McIntosh et al., 2018). Fetuses were freshly sampled as per Table 1 on Kanowna Island and those from Seal Rocks were stored frozen at −20°C and thawed at room temperature prior to processing.
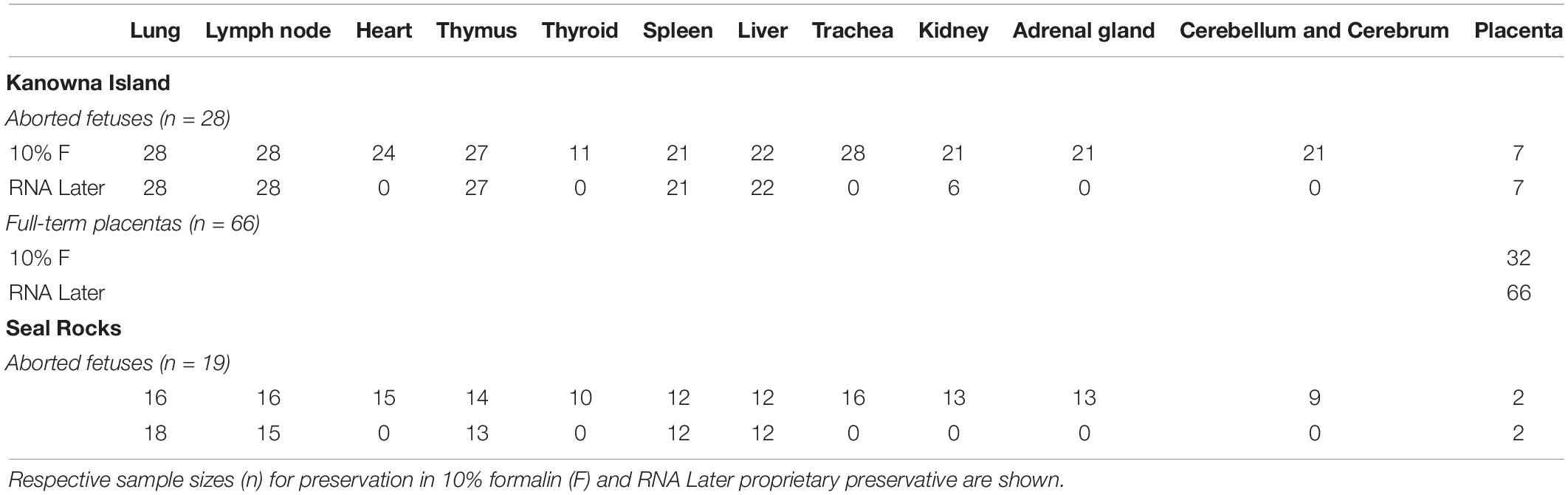
Table 1. Tissue samples collected from aborted fetuses and full-term placentas from Australian fur seals (Arctocephalus pusillus doriferus) from Kanowna Island (KI), Seal Rocks (SR) in Victoria.
Sixty-six fresh placentas from-full term Australian fur seal pups born on Kanowna Island were collected over a 3-week period during November and December 2020 and immediately processed in situ. The placental sections collected into formalin were cut full thickness from the maternal side of the placenta through to the fetal side of the placenta in the cleanest, grossly uncontaminated portions of the placenta. The incision and size of the tissue blocks ensured collection of all placental structures through from the fetal to maternal side of the placenta, including the trophoblast and hematophagous zones. The samples stored in RNAlater for DNA extraction were collected from the internal aspect of the opened placental sac-like unit, cutting on the fetal side of the placenta, to avoid environmental contamination of the samples.
A summary of the samples collected from each location is detailed in Table 1, indicating which were stored in 10% buffered formalin and in RNAlater (Thermo Fisher Scientific, Waltman, MA, United States). Some organs were absent due to scavenging activity of gulls.
Histopathology
Routine hematoxylin and eosin (HE) staining was performed on 5 μm thick sections of formalin fixed fetal tissues (heart, lung, trachea, esophagus, thymus, thyroid, lymph nodes, cerebrum, cerebellum, liver, spleen, kidney, adrenal gland, stomach, and umbilicus) and placenta embedded in paraffin wax. The sections were mounted on microscope slides and examined under light microscopy. Lesions across all representative fetal tissues were described. Table 1 details all the fetal tissues collected and examined histologically. Two sections of representative placenta and placental vasculature were evaluated for each full-term placenta. The placental slides were evaluated for the presence of necrosis, mononuclear and neutrophilic infiltration, fibrin deposition, the accumulation of cellular debris and thrombosis as individual histological traits. Each histological trait was assigned a score between zero and three, to indicate the presence and degree of severity, with 0.5 increments. The total score for each slide was divided by five as five histological traits were examined for each slide to give an average score used to grade the inflammatory changes present.
DNA Extraction and Quantitative Polymerase Chain Reaction
Using a proprietary Invitrogen Purelink genomic DNA extraction kit (Thermo Fisher Scientific, Waltman, Massachusetts, United States), genomic DNA was extracted and purified from a total weight of 250–350 μg of RNAlater preserved tissue for each sample. For fetuses the tissues collected from one individual were combined and extracted together. For full-term placentas the total weight was comprised of solely placental tissue.
Coxiella burnetii was detected and semi-quantified using two real-time TaqMan polymerase chain reaction (qPCR) assays using the proprietary Invitrogen Platinum Quantitative PCR SuperMix-UDG (Thermo Fisher Scientific, Waltman, MA, United States), a two-color tracking dye system to prevent carryover contamination targeting the com1 and htpAB genes. The com1 is a single copy gene whereas the htpAB is a 20-copy gene, that codes for two heat shock proteins (htpA and htpB) and is more sensitive than the single gene com1 (Fournier and Raoult, 2003). The primers and probe concentrations are shown in Table 2. A total volume of 25 μl was used for each reaction, which included 5 μl of extracted DNA. A no template control was run with each reaction using 5 μl nuclease free water (Gibco, Mulgrave, Vic, Australia). A magnetic induction PCR cycler (Mic) was used to perform amplification and fluorescence detection at 50°C for 3 min, thereby activating the uracil DNA glycosylase (UDG) in the SuperMix. Cycling then occurred at 95°C for 5 min to activate the platinum Taq DNA polymerase. For both the com1 and htpAB assays, 40 cycles of denaturation at 95°C for 20 s, with annealing and elongation occurring at 60°C for 40 s were used to amplify the Cb DNA. Data were analyzed using proprietary Mic PCR software version 2.8.13. A positive reaction for Cb DNA on either of the two qPCR was considered a positive result.
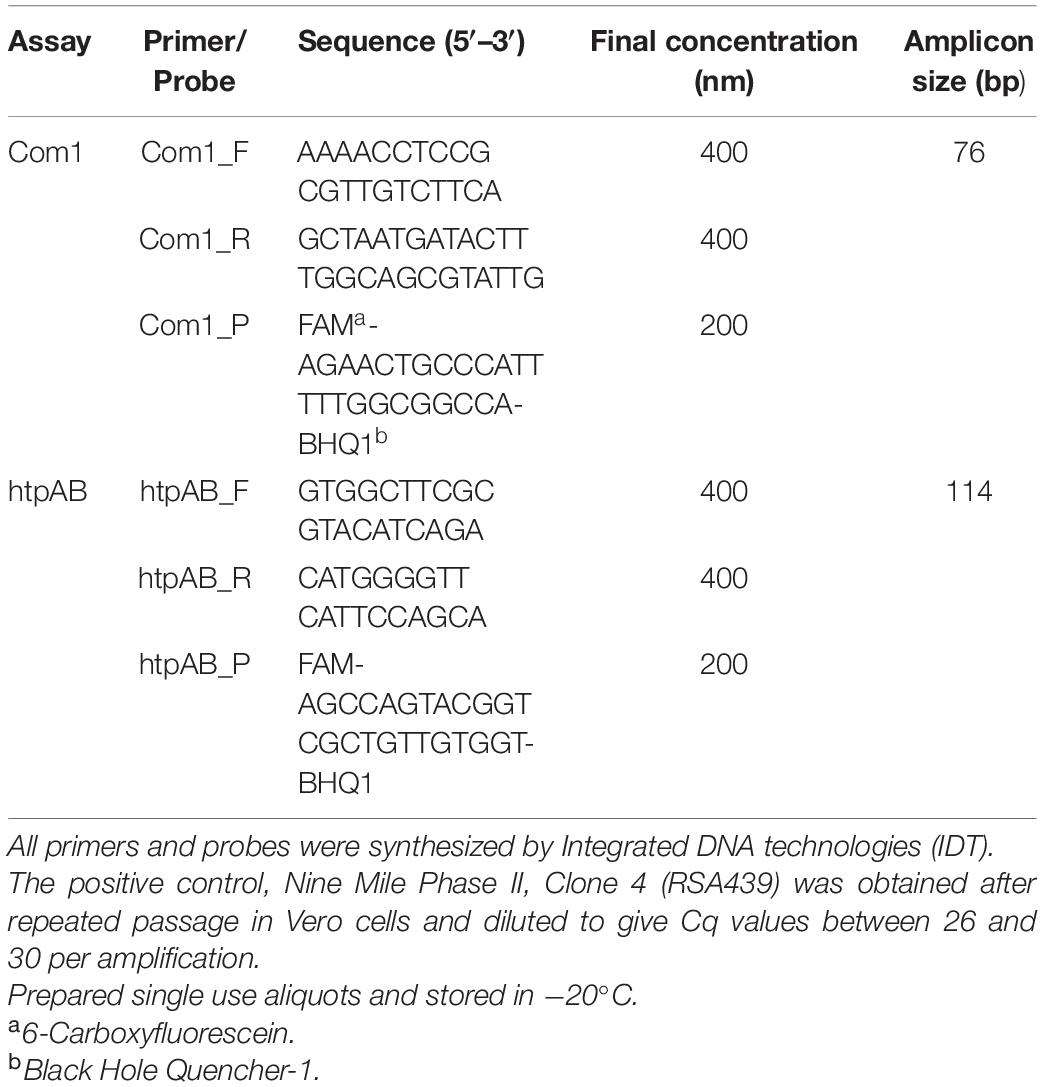
Table 2. Primers and probes used for qPCR assays for the detection of Coxiella burnetii DNA in tissue samples from Australian fur seals (Arctocephalus pusillus doriferus).
Immunohistochemistry
For immunohistochemistry (IHC), paraffin wax embedded placental sections were stained with anti-Coxiella antibodies (dilution 1:2,500) of only the PCR positive samples. PCR negative normal full-term goat placenta was used as the negative control tissue and the positive control was goat placenta with a known positive immunohistochemical staining for intracytoplasmic Cb. The assay was previously developed in-house at the University of Melbourne and internally validated; for details see Muleme et al. (2021).
Immunohistochemistry staining was applied to all placentas that produced a positive result on either of the two qPCRs and for which formalin fixed tissue was available (n = 11). Sections of formalin-fixed tissue of 5 μm thickness were mounted and deparaffinized on slides and then rehydrated with ethanol. Antigen retrieval was performed using a commercial kit (DAKO ARS S1700, Agilent, Santa Clara, CA, United States), the primary antibody was applied in 1:2500 and allowed to incubate for 30 min. Nova Red Chromogen Substrate (Vector Laboratories, Burlingame, CA, United States) was applied as per the manufacturer’s instructions and the slides were then counterstained with hematoxylin.
Results
Routine histopathology did not show any Cb organisms in any of the aborted fetal material including the associated aborted placentas. There were various non-specific lesions present throughout the fetuses, but none were positive for Cb. Amnion aspiration was commonly detectable through the presence of increased numbers of squamous epithelial cells present in the alveoli of the fetal lung and present in 29.8% of aborted fetuses. Nearly a fifth (23.4%) of the fetal lungs had what appeared to be histiocytic pneumonia with occasional neutrophilic infiltrates. There were non-specific cardiac lesions in 14.9% of the aborted fetuses including lymphohistiocytic epicarditis, regional myocarditis and myocardial necrosis. Of all the full-term placentas examined histologically on routine hematoxylin and eosin staining, intracytoplasmic bacteria were only detectable in one sample. The organisms were not uniformly distributed, but when present formed stippled cytoplasmic inclusions with characteristic foamy appearance within the placental trophoblasts (Figure 1). The Cb organisms did not appear to be associated with significant inflammatory reactions.
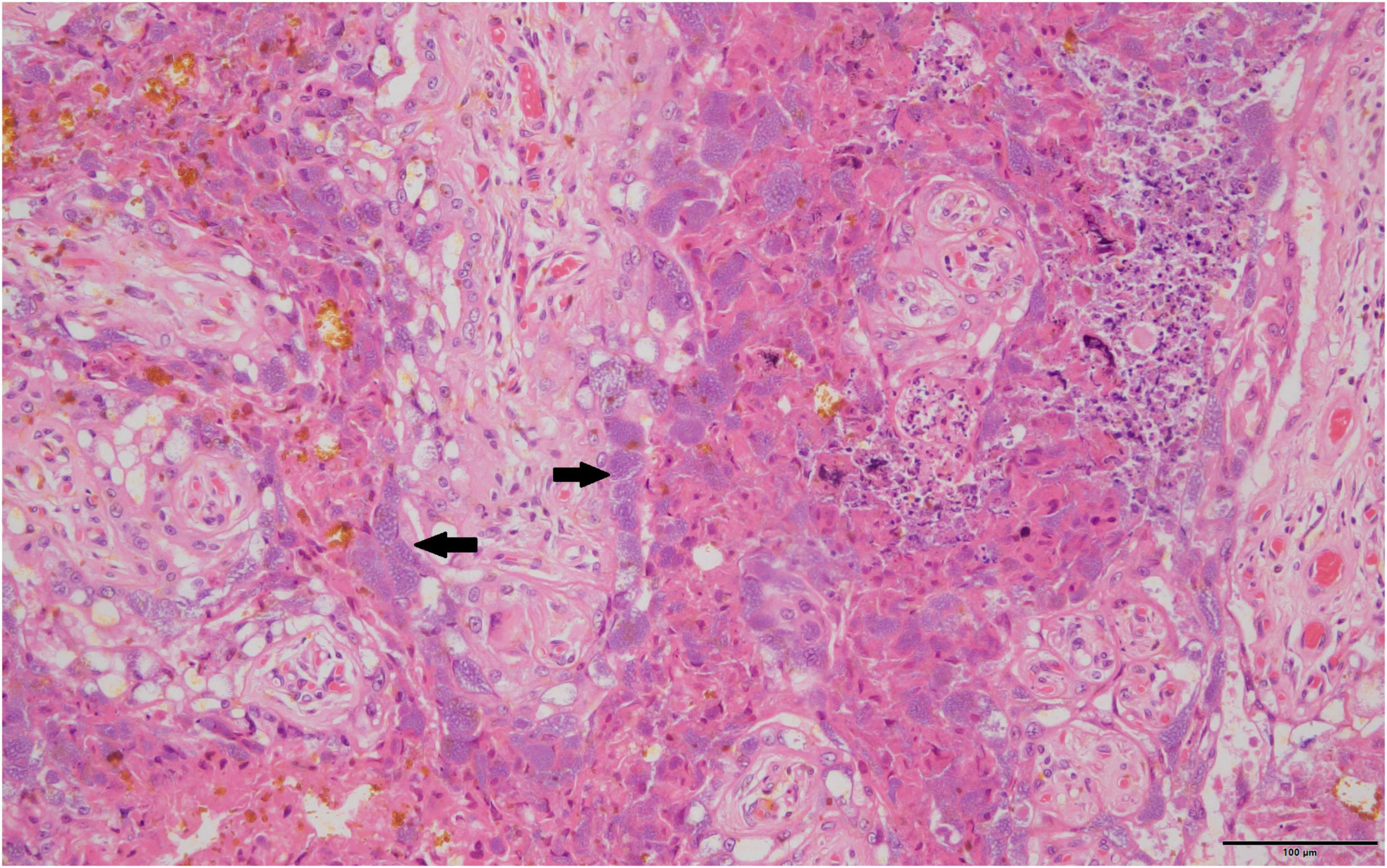
Figure 1. Typical foamy Coxiella burnetii cytoplasmic inclusions within the placental trophoblasts are indicated by solid black arrows, 40×, hematoxylin and eosin stain.
The grading of placental inflammatory lesions on HE, did not correlate with the presence of Cb as determined by qPCR. Placental inflammatory changes were quite focal and, even in more dramatic instances, were often interspersed with large amounts of normal appearing placenta. Many of the inflammatory changes appeared to be associated with the normal birthing process. These included focal areas of hemorrhage and thrombosis, mononuclear and neutrophilic white cell infiltration not associated with organisms or significant necrosis, mild fibrin deposition and the accumulation of surface cellular debris. Trophoblast necrosis was commonly seen as large strips of necrotic tissue surrounded by normal placental architecture. Occasionally the necrotic areas of trophoblast were associated with regions of fibrosis and mild focal calcification in 7.6% of placentas examined microscopically. The latter are not acute changes and not assumed to be associated with normal parturition.
A total of 45 third trimester abortions and a single full-term still birth collected from Kanowna Island and Seal Rocks were tested with both the htpAB and com1 qPCR. Due to scavenging by gulls, placental tissue was rarely available for the abortions and only nine (including the full-term still birth) were recovered and tested with the aborted fetuses. Coxiella burnetti DNA was not detected in any of these samples, except for the placental tissue of the full-term still birth. On the other hand, when the full-term placentas were evaluated using both qPCR, a substantial number of samples produced a positive result for the presence and amplification of Cb DNA. The htpAB produced 27 positive results from 66 samples (40.9%), whereas the com1 produced seven positive results from 66 full-term placental samples (10.6%). All samples that produced a positive com1 amplification were also positive for amplification on the htpAB (Table 3). The quantification cycle (Cq) values ranged from 27.91 to 38.19 for the com1 and 24.58 to 39.64 for the htpAB. Using a Fisher’s exact test, it appears that there is a statistically significant predicted difference using at a 95% confidence interval with a p value of 0.05 of 0.0238 between the presence of Cb in full-term placentas and the placental tissue of third trimester abortions.
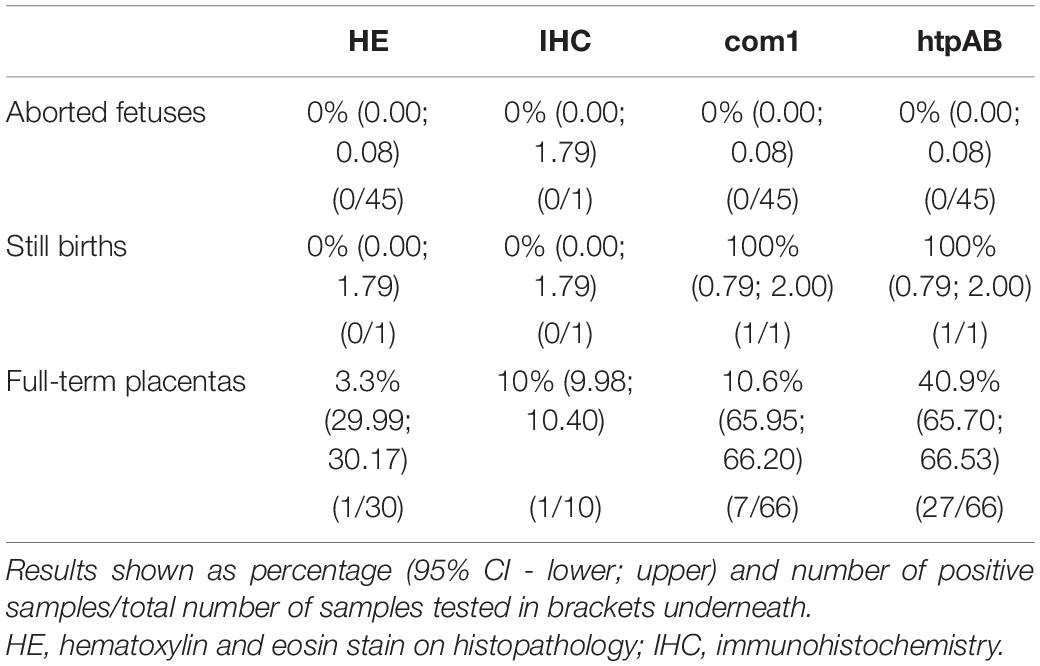
Table 3. Summary of all test results for Coxiella burnetii in Australian fur seals (Arctocephalus pusillus doriferus) from Victoria, across all samples and testing modalities.
Only full-term placentas that produced a positive result on the htpAB qPCR and for which formalin-fixed tissue was available were evaluated using IHC (n = 11). One of these placentas produced a positive result on IHC for immunoreactive coccobacilli (Figure 2), being the sample that was identified on routine histopathology. The IHC produced minimal non-specific staining of degenerate surface non-Cb material and was relatively easy to evaluate.
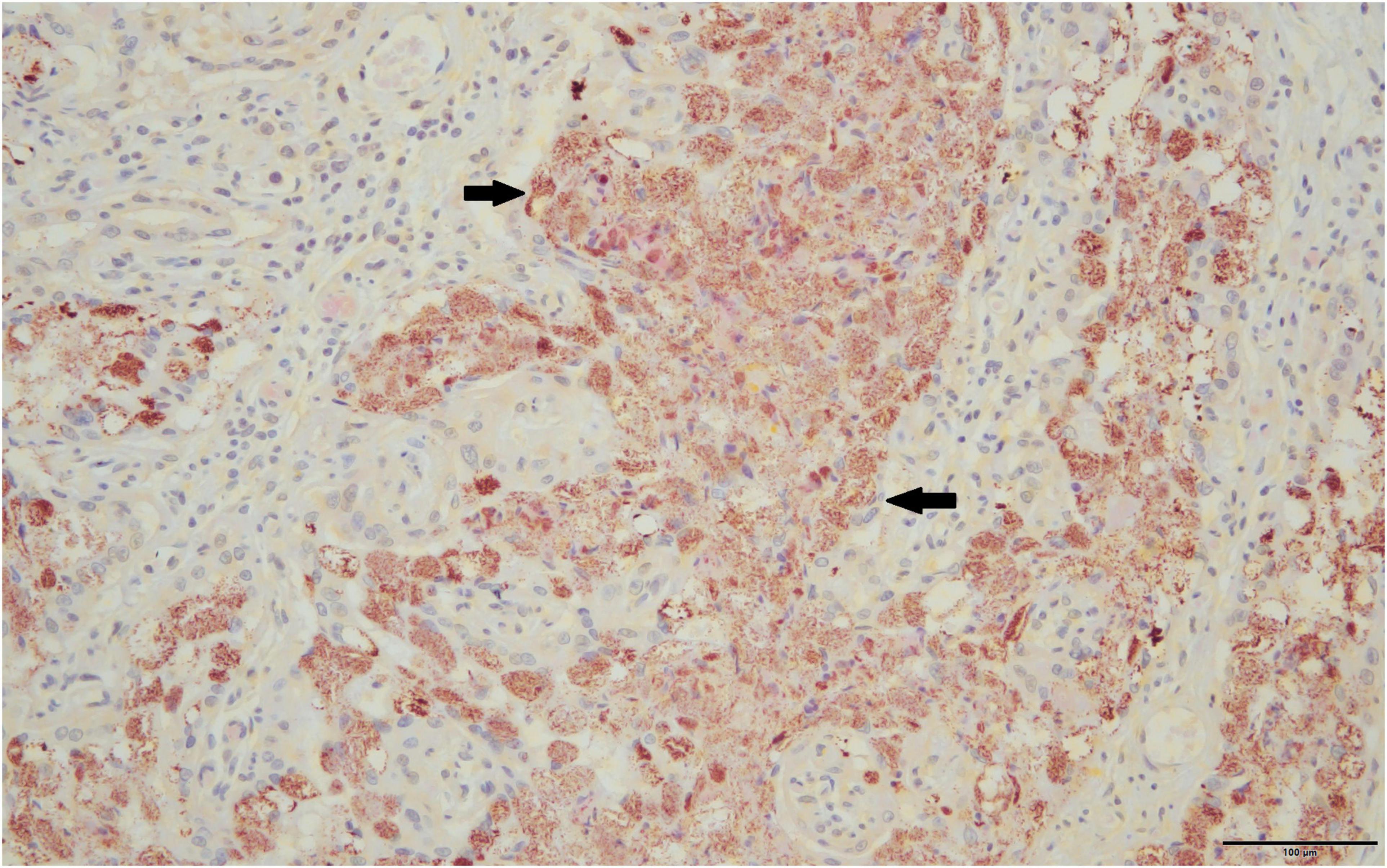
Figure 2. Immunoreactive coccobacilli typical of Coxiella burnetii stain a brick red-orange on immunohistochemistry are indicated by solid black arrows, 40×.
Discussion
With a detected Cb prevalence ranging from 10.6 to 40.9%, this study brings to question what significance Cb holds for the reproductive ecology of Australian fur seals. C. burnetii is known to typically cause abortions, premature birth and other indirect negative impacts on reproduction (Agerholm, 2013). There is a growing body of evidence that Cb is associated with chronic infertility, especially in cattle that do not show the typical overt clinical signs of large-scale abortions as is seen in goats (De Biase et al., 2018). In 2018, it was first described that Cb can produce chronic endometritis in dairy cattle with resultant chronic sub-fertility (De Biase et al., 2018). The results of this study in full-term, previously presumed healthy births might indicate that Australian fur seals experience chronic sub-fertility in part from Cb infection.
Reduced pup abundance has been observed in Australian fur seals at various colonies (McIntosh et al., 2018). Increased pup mortality has also been noted in unpublished observations at Seal Rocks (McIntosh, unpublished data). Some of the colonies exhibit high rates of third trimester abortions (Gibbens et al., 2010). At present the exact cause of the decreased Australian fur seal pup numbers in certain colonies is unknown. C. burnetii is currently an important emerging disease in wildlife (González-Barrio and Ruiz-Fons, 2019) and is widely reported on the Australian mainland (Mathews et al., 2021). Considering the detected prevalence of Cb in full-term placentas, it brings to question if this may potentially have significant impacts on post-partum pup survival.
It has been noted that Australian fur seals tend to have higher rates of abortion in young females despite younger females having high rates of pregnancy (Gibbens et al., 2010). Potentially if younger females are naïve to Cb infection, this could contribute to higher rates of abortion, especially if combined with other environmental stressors. When Cb infection shows endemic circulation in a population, older animals often inadvertently develop more effective immunity (González-Barrio and Ruiz-Fons, 2019). These negative reproductive outcomes could be contributing to a direct lack of recruitment in the Australian fur seals colonies at both study sites through premature and full-term loss of pregnancy. Furthermore, if Cb can produce chronic sub-fertility in Australian fur seals, this would additionally decrease the fecundity of breeding colonies and could contribute to the observed slow population recovery rate.
Routine HE histopathology and IHC had poor sensitivity for the detection of infection with Cb. Full-term placentas regularly had minor histopathological changes that were presumed in most cases to be associated with normal parturition rather than distinct Cb-related pathology. The typical lesions described in this study, including surface debris accumulations associated with placental necrosis, inflammatory white cell infiltrations, thrombosis and occasional mineralization have been identified as non-specific changes associated with parturition and are not necessarily indicators of infectious placentitis in bovine placentas (Botta et al., 2019). In the routine histopathology and the immunohistochemical staining, the distribution of the presence of Cb in the placental tissue was patchy. These results are similar to findings published in a study of several marine mammals of the Pacific Northwest of the United States, which found that IHC is quite insensitive for detecting the presence of Cb in marine mammal placentas (Kersh et al., 2012).
The sensitivity of detection with IHC was very low at 9.1% for qPCR Cb positive samples in the study of marine mammals of the Pacific North-West (Kersh et al., 2012). In a study of northern fur seals, where qPCR was employed in conjunction with IHC, only 3% of placentas evaluated were positive on IHC, compared to qPCR that showed a 74.7% detection rate for Cb using the com1 gene (Duncan et al., 2012). Similarly reported low detection rates of 11% using IHC compared to 30% for the com1 qPCR have been recorded in marine mammals in the Pacific Northwest of the United States (Kersh et al., 2012).
Molecular testing was found to be a more sensitive modality for the determination of the presence of Cb in Australian fur seals. The authors believe the difference in detection of Cb between the abortions and full-term placentas is an inability to detect the organism due to a small sample size for placentas from the abortions. In the few reports of abortions associated with Cb in marine mammals, there were no fetal lesions and Cb organisms could only be detected in placental tissue (Lapointe et al., 1999; Kersh et al., 2010). The results presented here support this being the same in Australian fur seals. It is well documented that the placenta acts as a barrier to pathogens entering the fetus and is a far more common site of infection (Robbins and Bakardjiev, 2012). On the other hand, with abortions being sporadic and not synchronized as is pupping, the risk for cross contamination might also be lower, while the high prevalence in full-term placentas could be at least partially the result of such cross contamination.
Strict care was taken in the field sampling to avoid environmental and cross-contamination of samples. It is not certain if some of the higher Cq (Quantification cycle) values of the qPCR indicate low levels of Cb across most samples or if those with higher Cq values potentially indicate a degree of environmental contamination at the time of maternal expulsion. During the pupping season there are dense congregations of females simultaneously giving birth, potentially increasing the risk of cross-contamination between infected and uninfected placentas.
The association of Cb with Australian fur seal placental tissue does not necessarily prove causality but highlights the need to determine whether this infectious organism is in part responsible for the decreased pup production of this species and its lower-than-expected recovery rate. The results of the present study are geographically restricted, with all samples collected from two colonies and all the positive samples from only one of these, Kanowna Island. The significance of Cb infection to Australian fur seals within this positive colony is not known and is even less so for the other colonies within the species’ range. Indeed, very little is known of the importance of this pathogen in other marine mammals. Its origin in Australian fur seals is unknown and further genetic investigations are required to determine if it is related to spillover of terrestrial Australian genotypes or if it potentially an endemic marine adapted form of Cb. Such information is of importance as these two scenarios have completely different environmental implications.
There are significant zoonotic considerations to the findings presented here. Historically, persons working within the livestock industry, especially those working with small ruminants and in the abattoir industry have been associated with an increased risk of zoonotic exposure to Cb (Mathews et al., 2021). A highly effective national Cb vaccination strategy has been in place in Australia since 2002 for persons at high risk in the abattoir and farming sector (Gidding et al., 2009). It has become apparent, though, that wildlife rescuers and rehabilitators are twice as likely compared to the general Australian public to be exposed to Cb and seroconvert (Mathews et al., 2021). It is also well documented that Australia has one of the highest recorded rates of Q-fever infection in the world (Bond et al., 2018).
Coxiella burnetii can persist in the environment for many years as the SCV, which is extremely resistant to inactivation through environmental degradation (Eldin et al., 2017). This SCV is also highly infectious and even in dilute environmental numbers is still a considerable zoonotic risk (Eldin et al., 2017). Taking into consideration the sheer numbers of animals giving birth in Australian fur seal colonies and the often dry and windy weather conditions during the pupping season (November–December) (O’Grady and McInnes, 2010), there is a clear need to investigate if these conditions pose an occupational health and safety concern for human activities in and around Australian fur seal colonies and if there is a seasonal difference in these risks. In the interim it may well be important to ensure adequate vaccination of persons working in these situations as they should be considered high-risk for potential Cb exposure.
Australian fur seals have overlapping foraging areas, haul-outs and breeding colonies with other pinnipeds including New Zealand fur seals (Arctocephalus forsteri) and Australian sea lions (Shaughnessy et al., 2010; Hoskins et al., 2017). This could serve as a source of cross-species transmission and could have potentially serious risks to the already endangered Australian sea lion population. In South Australia (Kangaroo Island and Casuarina Island), New Zealand fur seals and Australian sea lions have in recent years had increasing contact with Australian fur seals as they expand their breeding range into South Australia (Shaughnessy et al., 2010). The Australian sea lion is Australia’s only endemic pinniped, is classified as endangered and currently shows a decreasing population size facing a variety of population risks (Goldsworthy et al., 2021). If this population is naïve for Cb, there is the potential for introduction through expansion of Australian fur seal colonies that results in additional risk to an already unstable population. Unpublished observations from researchers working on Cape Bridgewater and Lady Julia Percy Islands have noted the presence of male Australian sea lions in Australian fur seal colonies. At present Cb has only been investigated in female animals and abortions. The risk that males pose to transmission of the organism is unknown. Alternatively, if Cb is already present in the population it would need to be explored as a possible contributing factor to the population decline of this endemic marine mammal.
To the authors’ knowledge this is the first description of Cb in a marine mammal in the southern hemisphere despite the organism being first described on the Australian mainland nearly a century ago. It is currently unknown if the terrestrial and marine Cb organisms are of the same genotype. It is critical to understand the relationship between Cb in Australian fur seals and terrestrial genotypes of the organism. There is some preliminary evidence that genotypes might display a degree of host specificity (González-Barrio et al., 2016). Understanding the significance of this well-known zoonotic abortifacient organism is very important both to the ecology of Australian fur seals and other marine mammals. Additionally, also as a potential risk factor to researchers and ecotourism operators conducting work with and in the vicinity of Australian fur seal colonies.
Data Availability Statement
The raw data supporting the conclusions of this article will be made available by the authors, without undue reservation.
Ethics Statement
The animal study was reviewed and approved by Phillip Island Nature Parks Animal Ethics Committee and University of Melbourne Research Ethics and Integrity Committee.
Author Contributions
BG and JH conceived the idea for the project. AS was the primary histologist with assistance by BG. Molecular work was done by AT, MT, and BG. BG led the data analysis with the assistance of JH, JS, JA, RM, and AT, led the writing of the manuscript with assistance from all authors, and collected and processed the samples. All authors contributed critically to the drafts and gave final approval for the published manuscript.
Funding
This research was supported and funded in part by the University of Melbourne and the Merle Ivy Research Scholarship, as part of BRG’s Ph.D. thesis. The costs and logistics of fieldwork were funded by Deakin University and Phillip Island Nature Parks. Molecular work was funded by Phillip Island Nature Parks. A student research award from the Wildlife Disease Association was used to fund the histopathology and immunohistochemistry.
Conflict of Interest
The authors declare that the research was conducted in the absence of any commercial or financial relationships that could be construed as a potential conflict of interest.
Publisher’s Note
All claims expressed in this article are solely those of the authors and do not necessarily represent those of their affiliated organizations, or those of the publisher, the editors and the reviewers. Any product that may be evaluated in this article, or claim that may be made by its manufacturer, is not guaranteed or endorsed by the publisher.
Acknowledgments
This work would not have been possible without the great piloting skills of Sean Best who ensured that the field sites could be accessed despite difficult weather windows and ongoing COVID-19 restrictions. Without the assistance of the Victorian Fisheries Authority mother vessels, access to Seal Rocks would not have been possible. Sincere thanks to Brooke Johnston and Hanna Geeson for their assistance and many hours spent collecting samples in the field. Many thanks to Faye Docherty who spent dedicated hours preparing histopathology and immunohistochemistry microscope slides.
References
Abeykoon, A. H., Clark, N. J., Soares Magalhaes, R. J., Vincent, G. A., Stevenson, M. A., Firestone, S. M., et al. (2021). Coxiella burnetii in the environment: a systematic review and critical appraisal of sampling methods. Zoon. Public Health 68, 165–181. doi: 10.1111/zph.12791
Agerholm, J. S. (2013). Coxiella burnetii associated reproductive disorders in domestic animals-a critical review. Acta Vet. Scand. 55, 1–11. doi: 10.1186/1751-0147-55-13
Arnould, J., and Costa, D. (2006). “Sea lions in drag, fur seals incognito: insights from the otariid deviants,” in Sea lions of the world: proceedings of the symposium sea lions of the world: conservation and research in the 21st Century: Citeseer (Fairbanks: Univ of Alaska Press), 309–323. doi: 10.1093/conphys/coaa111
Blakeway, J.-A., Arnould, J. P., Hoskins, A. J., Martin-Cabrera, P., Sutton, G. J., Huckstadt, L. A., et al. (2021). Influence of hunting strategy on foraging efficiency in Galapagos sea lions. PeerJ 9:e11206. doi: 10.7717/peerj.11206
Bond, K. A., Franklin, L., Sutton, B., Stevenson, M., and Firestone, S. (2018). Review of 20 years of human acute Q fever notifications in Victoria, 1994–2013. Aust. Vet. J. 96, 223–230. doi: 10.1111/avj.12704
Botta, C., Pellegrini, G., Hässig, M., Pesch, T., Prähauser, B., Wunderlin, S., et al. (2019). Bovine fetal placenta during pregnancy and the postpartum period. Vet. Pathol. 56, 248–258. doi: 10.1177/0300985818806453
Costa, D. P., and Gales, N. J. J. E. M. (2003). Energetics of a benthic diver: seasonal foraging ecology of the Australian sea lion, Neophoca cinerea. Ecol. Monogr. 73, 27–43. doi: 10.1890/0012-9615(2003)073[0027:eoabds]2.0.co;2
De Biase, D., Costagliola, A., Del Piero, F., Di Palo, R., Coronati, D., Galiero, G., et al. (2018). Coxiella burnetii in infertile dairy cattle with chronic endometritis. Vet. Pathol. 55, 539–542. doi: 10.1177/0300985818760376
Duncan, C., Kersh, G. J., Spraker, T., Patyk, K. A., Fitzpatrick, K. A., Massung, R. F., et al. (2012). Coxiella burnetii in northern fur seal (Callorhinus ursinus) placentas from St. Paul Island, Alaska. Vector-Borne Zoonotic. Dis. 12, 192–195. doi: 10.1089/vbz.2011.0715
Eldin, C., Mélenotte, C., Mediannikov, O., Ghigo, E., Million, M., Edouard, S., et al. (2017). From Q fever to Coxiella burnetii infection: a paradigm change. Clin. Microbio. Rev. 30, 115–190. doi: 10.1128/CMR.00045-16
Fournier, P.-E., and Raoult, D. (2003). Comparison of PCR and serology assays for early diagnosis of acute Q fever. J. Clin. Microbiol. 41, 5094–5098. doi: 10.1128/JCM.41.11.5094-5098.2003
Gibbens, J., Parry, L. J., and Arnould, J. P. J. J. O. M. (2010). Influences on fecundity in Australian fur seals (Arctocephalus pusillus doriferus). J. Mammal. 91, 510–518. doi: 10.1644/08-MAMM-A-377.1
Gidding, H. F., Wallace, C., Lawrence, G. L., and McIntyre, P. B. (2009). Australia’s national Q fever vaccination program. Vaccine 27, 2037–2041. doi: 10.1016/j.vaccine.2009.02.007
Goldsworthy, S. D., Shaughnessy, P. D., Mackay, A. I., Bailleul, F., Holman, D., Lowther, A. D., et al. (2021). Assessment of the status and trends in abundance of a coastal pinniped, the Australian sea lion Neophoca cinerea. Endan. Spec. Res. 44, 421–437. doi: 10.3354/esr01118
González-Barrio, D., Jado, I., Fernandez de Mera, I. G., delRocio Fernandez-Santos, M., Rodríguez-Vargas, M., Garcia-Amil, C., et al. (2016). Genotypes of Coxiella burnetii in wildlife: disentangling the molecular epidemiology of a multi-host pathogen. Environ. Microbiol. Rep. 8, 708–714. doi: 10.1111/1758-2229.12431
González-Barrio, D., and Ruiz-Fons, F. (2019). Coxiella burnetii in wild mammals: A systematic review. Transbound Emerg. Dis. 66, 662–671. doi: 10.1111/tbed.13085
Hoskins, A. J., Schumann, N., Costa, D. P., and Arnould, J. P. (2017). Foraging niche separation in sympatric temperate-latitude fur seal species. Mar. Ecol. Progr. Ser. 566, 229–241. doi: 10.3354/meps12024
Kersh, G. J., Lambourn, D. M., Raverty, S. A., Fitzpatrick, K. A., Self, J. S., Akmajian, A. M., et al. (2012). Coxiella burnetii infection of marine mammals in the Pacific Northwest, 1997–2010. J. Wildl. Dis. 48, 201–206. doi: 10.7589/0090-3558-48.1.201
Kersh, G. J., Lambourn, D. M., Self, J. S., Akmajian, A. M., Stanton, J. B., Baszler, T. V., et al. (2010). Coxiella burnetii infection of a Steller sea lion (Eumetopias jubatus) found in Washington State. J. Clin. Microbiol. 48, 3428–3431. doi: 10.1128/JCM.00758-10
Kirkwood, R., Pemberton, D., Gales, R., Hoskins, A. J., Mitchell, T., Shaughnessy, P. D., et al. (2010). Continued population recovery by Australian fur seals. J. Mar. Freshwat. Res. 61, 695–701. doi: 10.1071/MF09213
Lancaster, M., Arnould, J., and Kirkwood, R. J. A. C. (2010). Genetic status of an endemic marine mammal, the Australian fur seal, following historical harvesting. Anim. Conserv. 13, 247–255. doi: 10.1111/j.1469-1795.2009.00325.x
Lapointe, J. M., Gulland, F. M., Haines, D. M., Barr, B. C., and Duignan, P. J. J. J. O. V. D. (1999). Placentitis due to Coxiella burnetii in a Pacific harbor seal (Phoca vitulina richardsi). J. Vet. Diagn. Invest. 11, 541–543. doi: 10.1177/104063879901100612
Lynch, M., Duignan, P. J., Taylor, T., Nielsen, O., Kirkwood, R., Gibbens, J., et al. (2011a). Epizootiology of Brucella infection in Australian fur seals. J. Wildl. Dis. 47, 352–363. doi: 10.7589/0090-3558-47.2.352
Lynch, M., Taylor, T. K., Duignan, P. J., Swingler, J., Marenda, M., Arnould, J. P., et al. (2011b). Mycoplasmas in Australian fur seals (Arctocephalus pusillus doriferus): identification and association with abortion. J. Vet. Diagn. Invest. 23, 1123–1130. doi: 10.1177/1040638711425699
Magera, A. M., Mills Flemming, J. E., Kaschner, K., Christensen, L. B., and Lotze, H. K. (2013). Recovery trends in marine mammal populations. PLoS One 8:e77908. doi: 10.1371/journal.pone.0077908
Mathews, K. O., Toribio, J.-A., Norris, J. M., Phalen, D., Wood, N., Graves, S. R., et al. (2021). Coxiella burnetii seroprevalence and Q fever in Australian wildlife rehabilitators. One Health 12:100197. doi: 10.1016/j.onehlt.2020.100197
McIntosh, R. R., Kirkman, S. P., Thalmann, S., Sutherland, D. R., Mitchell, A., Arnould, J. P., et al. (2018). Understanding meta-population trends of the Australian fur seal, with insights for adaptive monitoring. PLoS One 13:e0200253. doi: 10.1371/journal.pone.0200253
Minor, C., Kersh, G. J., Gelatt, T., Kondas, A. V., Pabilonia, K. L., Weller, C. B., et al. (2013). Coxiella burnetii in northern fur seals and steller sea lions of Alaska. J. Wildl. Dis. 49, 441–446. doi: 10.7589/2012-09-226
Muleme, M., Devlin, J. M., Campbell, A., Vincent, G., Benham, P. J., Sprohnle, C., et al. (2021). A randomised controlled trial of the immunogenicity and safety of a formaldehyde-inactivated Coxiella burnetii vaccine in 8-week-old goats. Vet. Immunol. Immunopathol. 236:110253. doi: 10.1016/j.vetimm.2021.110253
O’Grady, J. G., and McInnes, K. L. (2010). Wind waves and their relationship to storm surges in northeastern Bass Strait. Aust. Meteorol. Oceanogr. J. 60, 265–275. doi: 10.22499/2.6004.004
Pemberton, D., and Gales, R. (2004). Australian fur seals (Arctocephalus pusillus doriferus) breeding in Tasmania: population size and status. J. Wildl. Res. 31, 301–309. doi: 10.1071/WR02083
Robbins, J. R., and Bakardjiev, A. I. (2012). Pathogens and the placental fortress. Curr. Opin. Microbiol. 15, 36–43. doi: 10.1016/j.mib.2011.11.006
Roest, H. I., Ruuls, R. C., Tilburg, J. J., Nabuurs-Franssen, M. H., Klaassen, C. H., Vellema, P., et al. (2011). Molecular epidemiology of Coxiella burnetii from ruminants in Q fever outbreak, the Netherlands. Emerg. Infect. Dis. 17:668. doi: 10.3201/eid1704.101562
Rosen, D. A., and Trites, A. W. J. J. O. C. P. B. (2005). Examining the potential for nutritional stress in young Steller sea lions: physiological effects of prey composition. J. Comp. Physio. B 175, 265–273. doi: 10.1007/s00360-005-0481-5
Shaughnessy, P. D., McKenzie, J., Lancaster, M. L., Goldsworthy, S. D., and Dennis, T. E. (2010). Australian fur seals establish haulout sites and a breeding colony in South Australia. Aust. J. Zool. 58, 94–103. doi: 10.1071/zo10017
Taylor, S., Terkildsen, M., Stevenson, G., de Araujo, J., Yu, C., Yates, A., et al. (2021). Per and polyfluoroalkyl substances (PFAS) at high concentrations in neonatal Australian pinnipeds. Sci. Total Environ. 786:147446. doi: 10.1016/j.scitotenv.2021.147446
Trites, A., and Donnelly, C. J. M. R. (2003). The decline of Steller sea lions Eumetopias jubatus in Alaska: a review of the nutritional stress hypothesis. Mamm. Rev. 33, 3–28. doi: 10.1046/j.1365-2907.2003.00009.x
Keywords: Coxiella burnetii, Australian fur seal, abortion, placenta, Arctocephalus pusillus, zoonotic risk
Citation: Gardner BR, Stenos J, Hufschmid J, Arnould JPY, McIntosh RR, Tadepalli M, Tolpinrud A, Marenda M, Lynch M and Stent A (2022) An Old Pathogen in a New Environment–Implications of Coxiella burnetii in Australian Fur Seals (Arctocephalus pusillus doriferus). Front. Mar. Sci. 9:809075. doi: 10.3389/fmars.2022.809075
Received: 04 November 2021; Accepted: 03 January 2022;
Published: 25 January 2022.
Edited by:
Rachel Tinker-Kulberg, Kepley BioSystems, Inc., United StatesReviewed by:
Annalisa Zaccaroni, University of Bologna, ItalyGilbert Kersh, Centers for Disease Control and Prevention (CDC), United States
Copyright © 2022 Gardner, Stenos, Hufschmid, Arnould, McIntosh, Tadepalli, Tolpinrud, Marenda, Lynch and Stent. This is an open-access article distributed under the terms of the Creative Commons Attribution License (CC BY). The use, distribution or reproduction in other forums is permitted, provided the original author(s) and the copyright owner(s) are credited and that the original publication in this journal is cited, in accordance with accepted academic practice. No use, distribution or reproduction is permitted which does not comply with these terms.
*Correspondence: Brett R. Gardner, Z2FyZG5lci5iQHVuaW1lbGIuZWR1LmF1