- 1Department of Biology, San Diego State University, San Diego, California, United States
- 2College of Science and Engineering, Flinders University, Bedford Park, SA, Australia
Caribbean reefs have undergone large-scale losses in coral cover in past decades, sparking a search for species that are resilient under stress. Porites astreoides has been considered a “winner” and a key player in sustaining coral cover in the Caribbean as more sensitive species struggle. However, P. astreoides has recently declined in abundance, raising concern about its status as a winner. Here, we reviewed the ecophysiology of P. astreoides in response to environmental stress to elucidate whether this species could thrive in the future of Caribbean reefs. We examined ecophysiological variables of P. astreoides related to photosynthesis, growth, recruitment, tissue condition, and microbiome in response to temperature, pH, macroalgal competition, depth, and sedimentation. Overall, P. astreoides was sensitive to environmental stress and each physiological feature showed varying levels of sensitivity. Coral-algal photosynthesis and coral tissue condition could withstand single events of thermal stress but reflected a metabolic imbalance that hinders recovery from repeated bleaching events, compromising long-term success. Colony growth was particularly vulnerable to low pH and macroalgal competition. Recruitment was unaffected, or even favored, by depth and could tolerate high temperatures, but it was sensitive to exposure to macroalgae, especially in combination with abiotic stressors. The response of the microbiome of P. astreoides to stressors is still poorly understood. In relation to other corals, P. astreoides was frequently reported as the most sensitive species in the reviewed literature. The success of P. astreoides is tightly integrated into the future of Caribbean reefs, which could be losing an old winner.
Introduction
Coral reef community composition has rapidly shifted in the past three decades as coral cover declined worldwide due to local and global stressors (Jones et al., 1998; Randall et al., 2014; Maynard et al., 2015; Hughes et al., 2018; Safaie et al., 2018). These losses are pronounced on shallow water reefs of the Caribbean, where an overall decline in total coral cover of up to 59% has occurred from 1984 to 2014 (Jackson et al., 2014). Thermal stress is one of the greatest threats to coral health causing mass bleaching and disease outbreaks and is especially concerning in conjunction with ocean acidification (OA) in future climate change scenarios (Pandolfi et al., 2011; Hoegh-Guldberg et al., 2017). Macroalgae outcompete corals, either directly (e.g., shading, substrate occupation, abrasion) or indirectly (e.g., allelopathy, microbial-mediated activities) and have caused phase shifts in coral reefs worldwide and especially in the Caribbean, where overfishing and eutrophication are pronounced (Hughes, 1994; Hughes et al., 2007; Dinsdale and Rohwer, 2011; Haas et al., 2016). Factors that affect light availability (e.g., depth, sedimentation), influence the coral-algal photosynthesis and physiology, and therefore, limit coral distribution and success (Kleypas et al., 1999; Anthony and Connolly, 2004; Ziegler et al., 2015). Physiological responses of corals to disturbances vary across different species and populations (Mydlarz et al., 2010; Hoadley et al., 2015) causing the relative abundances of each coral species to fluctuate in a complex dynamic. Across reefs in the Caribbean there was a shift from dominance of superior competitors, (e.g., Acropora), that were dominant since the Pleistocene, to that of stress-tolerant and weedy species, such as Porites and Agaricia in the late 1990’s (Cramer et al., 2020). Coral cover on reefs in Jamaica was ~ 75% in the 1980 at 10 m and declined to less than 5% in 1994. The cover of these weedy coral species started to make up ~ 20% of 4% total coral cover in the 1990 on reefs in St. John, U.S. Virgin Islands, and remained consistent for many years (Edmunds et al., 2021). Loss of the large corals to more encrusting species reduced the rugosity of the reefs creating a loss in structure and habitat for other species, including fish (Dustan et al., 2013). In 2011, the cover of these weedy species started to decline (Edmunds et al., 2021). Ecosystem resistance is modeled to co-vary with increasing degradation, but a threshold may be reached, where changes in species composition and interactions may become irreversible, impairing both resistance and recovery of the ecosystem (Côté and Darling, 2010). Therefore, predicting the “winners” and “losers” (Loya et al., 2001) in the uncertain future of Caribbean reefs is a crucial challenge to be addressed.
Porites astreoides is a strong candidate to persist over time in the Caribbean. This reef-building species colonizes a wide range of habitats across reef zones, from tidal pools and back reef sites (Porter et al., 1982; de Putron and Smith, 2011; Baumann et al., 2016) to mesophotic reefs (Holstein et al., 2016a; Goodbody-Gringley et al., 2018). Pioneer ecophysiological studies showed that this species is resistant to suboptimal conditions, such as high sedimentation rates and elevated temperature (Tomascik and Sander, 1987; Gates, 1990; Gleason, 1998). The high resilience of P. astreoides is supported by “weedy” life-history traits such as short life cycles, high fecundity, and high settlement rates of brooded larvae (Chornesky and Peters, 1987; Soong, 1991; McGuire, 1998). Porites astreoides was considered a winner as the percentage cover relative to total coral cover at six locations spanning a 4100-kilometer arc of the Caribbean increased at a rate of 1.5% per year, ranging from less than 20% in the 1970s to 50% in 2004 (Green et al., 2008). The mortality of Porites spp. juveniles was generally lower than Agaricia spp., and Favia fragum, but higher than S. siderea, and S. radians in late 1990 over 5 sites in the U.S. Virgin Islands, (Edmunds, 2000). Projections suggested that P. astreoides would increase in abundance, even under frequent disturbances (Edmunds, 2010; Soto-Santiago et al., 2017a). However, on the south coast of St. John, the relative contribution of P. astreoides to coral cover peaked around 2008 and has been in decline ever since, raising concern about their winner status in the Caribbean (Edmunds et al., 2021). The inability of P. astreoides to recover from sequential bleaching events (Grottoli et al., 2014) indicates that the species may not be as physiologically resilient as previously thought. Understanding how the species will respond physiologically to increasing frequency and intensity of disturbance may shed light on the future success of P. astreoides.
Will Porites astreoides be a key species in the future of the Caribbean coral community? Here, we reviewed 37 primary literature publications (Table S1), focusing on studies published since P. astreoides was considered a winner in the Caribbean by Green et al. (2008) up to 2021 describing the ecophysiology traits of P. astreoides under stress. We compiled a list of research articles using the Scopus (Elsevier) database, where we applied the following filters of keywords, title, or abstract: “Porites astreoides” ”stress” OR ”physiology” OR ”experiment” OR “tolerance” OR “resistance” OR “resilience” OR “sensitivity”. We selected studies from the list that assessed natural disturbances and/or climate change stressors in experimental settings (laboratory or in situ) or monitoring after extreme events (e.g., thermal anomalies, mass bleaching) in the Caribbean region (including Bermuda). We referred to each research article published as a “study”. “Report” was used to refer to the result of a response variable (e.g., growth rate, respiration, larval recruitment) of P. astreoides to a factor (e.g., temperature, pH, sedimentation). Therefore, a study may include many reports showing different results. For example, sensitivity of growth rates to pH and resistance of growth rates to temperature can be reported in the same research article, therefore, they would be accounted as two reports in one study.
We examined the ecophysiological response variables of P. astreoides across five major categories: 1- Photosynthesis of the coral endosymbionts (Symbiodiniaceae); 2 – Growth; 3 – Recruitment; 4 – Tissue Condition; 5- Microbiome. The environmental factors comprised temperature, pH, macroalgal competition, depth, and sedimentation, including synergistic effects of these variables on the physiology of P. astreoides.
Results
The ecophysiological responses of Porites astreoides were more often reported to be sensitive than resistant and/or resilient to stress. From the total of 84 reports across 37 published studies, 54% concluded that P. astreoides was negatively affected by stressors and 45% reported non-significant responses. However, the tolerance of P. astreoides to stress varied across physiological parameters and types of stressors (Figure 1).
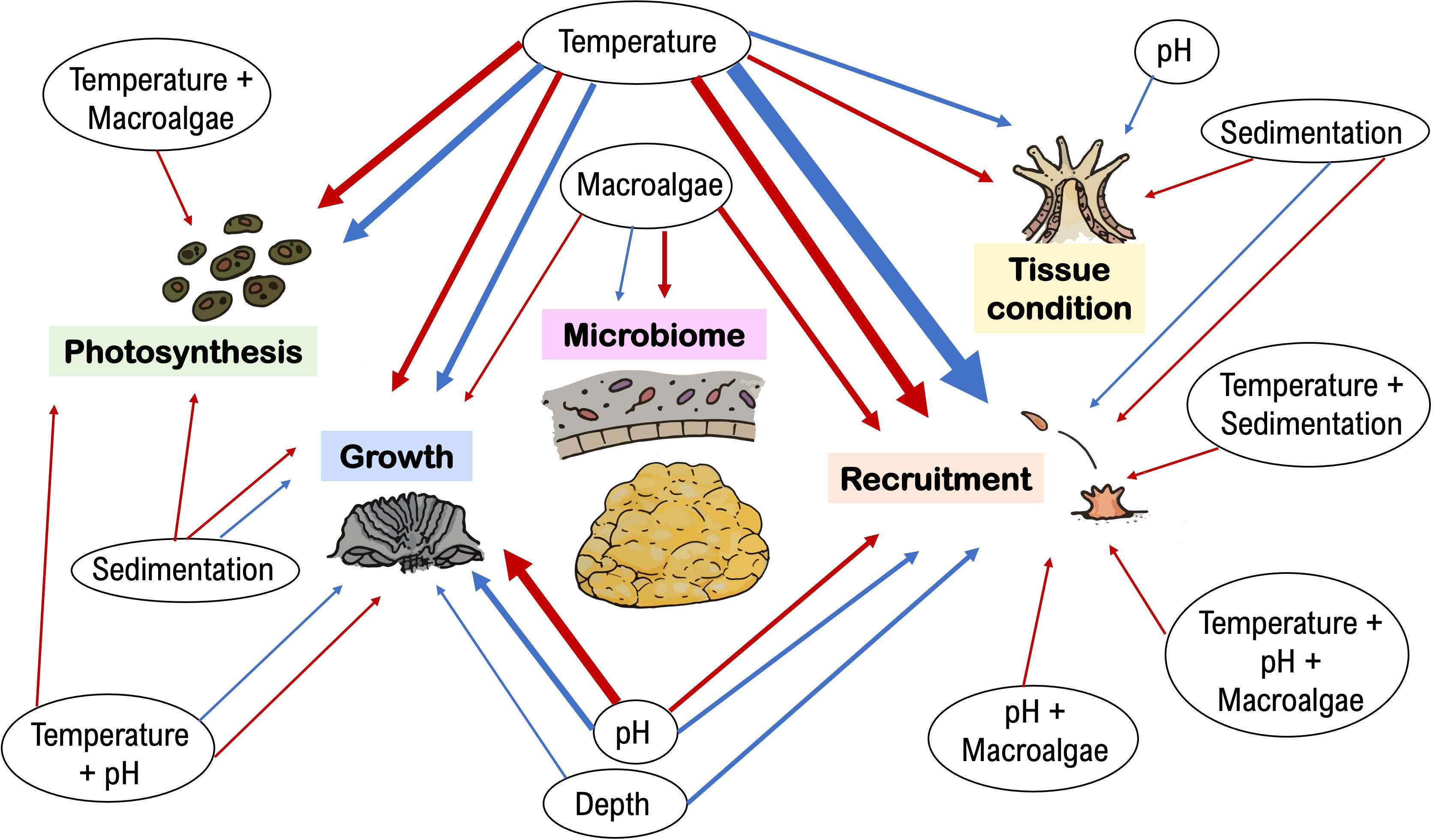
Figure 1 Porites astreoides was more often reported as sensitive than resistant/resilient to environmental stress. Since 2009, 54% of the total of 84 reports (across 37 studies total) showed that P. astreoides ecophysiological parameters related to endosymbiont photosynthesis, coral growth, recruitment, tissue condition, and microbiome, are sensitive (red arrows) to stressors, while 45% showed stress tolerance and/or ability to recover (blue arrows). The weight of the arrow lines corresponds to the frequency that the respective effect was reported in the literature (thinnest lines indicate 1 report; thickest lines represent 10 reports).
Photosynthesis: Endosymbiont density, chlorophyll concentration, productivity, maximum photochemical efficiency of photosystem II (Fv/Fm), and bleaching
Porites astreoides was considered one of the least susceptible coral species to bleaching after exposure to heat waves recorded in the U.S. Virgin Islands in 2005 (Smith et al., 2013) and in the Cayman Islands, in 2009 (van Hooidonk et al., 2012). However, the ability of P. astreoides and their endosymbionts to recover from stress depended on the frequency of the exposure. When exposed to a single bleaching event, P. astreoides recovered endosymbiont abundance after 1.5 months (Grottoli et al., 2014; Levas et al., 2018) or within a year (Schoepf et al., 2015) at reef ambient temperatures. In contrast, colonies that were exposed to a recurrent bleaching treatment lost half of their endosymbionts and were not able to recover after six weeks in ambient temperatures (Grottoli et al., 2014). Porites divaricata was resilient to repeated bleaching via heterotrophic compensation, whereas the mounding corals P. lobata and O. faveolata used a combination of heterotrophy and thermally tolerant Symbiodiniaceae in their response to mild bleaching events (Levas et al., 2018).
Symbiodiniaceae associated with P. astreoides were sensitive to heat (Grottoli et al., 2014; Schoepf et al., 2015; Soto-Santiago et al., 2017b; Levas et al., 2018) and cold (Kemp et al., 2011) stress. Colonies exposed to 32°C lost ~ half of endosymbionts (Grottoli et al., 2014; Soto-Santiago et al., 2017b; Levas et al., 2018) and 40% to 75% of chlorophyll concentrations (Schoepf et al., 2015; Soto-Santiago et al., 2017b). Seawater temperatures lower than 16°C caused a significant reduction in gross photosynthesis and in the maximum photochemical efficiency of photosystem II (Fv/Fm) (Kemp et al., 2011). Also, P. astreoides showed low resilience to sedimentation, as Fv/Fm rates kept decreasing after 5 days of being removed from the treatments (Rushmore et al., 2021).
Synergistic effects of warmer temperatures and other environmental factors were threatening to the P. astreoides coral-algal physiology (Camp et al., 2016; Smith et al., 2019). Elevated temperature combined with OA decreased productivity of colonies collected from both high and low-variance habitats off the Cayman Islands, indicating that acclimatization to natural pH–temperature disturbances did not increase physiological performance of P. astreoides under future climate scenarios (Camp et al., 2016). During a 4-year field experiment in the Florida Keys, the presence of fleshy macroalgae (Dictyota spp. within 10 cm) was the environmental factor that best explained the increase in bleaching of P. asteroides corals in thermal stress (Smith et al., 2019). Bleaching was predominant in P. astreoides, observed in 69.0% of transplants, while only 7.1% of Siderastrea siderea bleached (Smith et al., 2019).
When tested individually, exposure to macroalgae and low pH alone did not negatively affect the algal endosymbionts in P. astreoides. Adult colonies were resistant to allelopathy from macroalgae as Fv/Fm rates were unaffected by crude extracts from Dictyota (Paul et al., 2011). After a two-year transplantation to a low aragonite saturation submarine spring, chlorophyll-a and endosymbiont densities increased, indicating a higher capacity for the photosynthetic activity that could provide additional energy to corals under suboptimum conditions (Martinez et al., 2019).
Growth: Skeleton linear extension, density, and calcification rates
Growth of P. astreoides was reported in the literature to be equally sensitive and resistant/resilient to thermal stress. While P. astreoides showed negative calcification in response to elevated temperature (30.3°C) for two months (Okazaki et al., 2017); projected end-of-century annual mean temperature (31°C) for Caribbean reefs had no significant effect on calcification rates after ~ 3 months at this temperature (Bove et al., 2019). Heat-stress lowered calcification by 69% in experiments where P. astreoides colonies were exposed to 31°C for 7 days in a tank (Schoepf et al., 2015), but P. astreoides was able to recover pre-treatment growth after the heat-treated colonies were transplanted back on the reef for a year (Grottoli et al., 2014; Schoepf et al., 2015; Levas et al., 2018). However, repeated annual bleaching impaired the ability of P. astreoides to recover calcification rates (Grottoli et al., 2014). Calcification of P. astreoides colonies transplanted in situ were negatively correlated with heat stress (Manzello et al., 2015).
The effects of OA on the growth of P. astreoides depended on the pCO2 levels and exposure to heat stress. Calcification was stable at end-of-century pCO2 (701/673 µatm) (Bove et al., 2019), but experienced major declines under higher pCO2 (900, 1300, 3309/3285 µatm) (Okazaki et al., 2017; Bove et al., 2019). Reduced pH had a greater impact on calcification compared to temperature, although these factors had an additive decrease in calcification when combined (Camp et al., 2016). In contrast, the interaction between OA and heat did not significantly lower calcification of P. astreoides after a 30-day acclimation period (Bove et al., 2019).
Acclimatization to low pH did not seem to increase resilience in P. astreoides (Crook et al., 2013; Camp et al., 2016; Wall et al., 2019). The deleterious effects of OA were not significantly different between colonies acclimatized to high and low variance in pH (Camp et al., 2016). Corals collected in proximity to a low-pH groundwater plume showed significant decrease in calcification under OA in experimental conditions; comparable to the lower calcification rates in colonies that had not been previously acclimatized (Crook et al., 2013; Wall et al., 2019). After a two-year transplantation to a low-pH spring, linear extension and calcification were maintained but skeletal density of the coral decreased (Martinez et al., 2019). Similarly, sedimentation did not decrease linear extension rates but affected skeleton density and calcification (Elizalde-Rendón et al., 2010).
In contrast, the linear extension, density, or calcification of the skeleton of P. astreoides were not affected by a wide depth variability from 6 – 47 m, indicating the species growth was unaffected by depth (Groves et al., 2018). In mesophotic reefs (30 – 40 m depth), growth rates were also not significantly impacted by increased temperatures (Groves et al., 2018).
Macroalgal competition was a less understood factor that was very deleterious to P. astreoides. Growth was reduced by 40% on average when colonies were exposed to five different species of benthic macroalgae (Vega Thurber et al., 2012).
Recruitment: Fecundity, larval survival, larval production, larval photosynthesis, larval oxidative damage, settlement rates, juvenile survival, juvenile growth
Recruitment of P. astreoides was unaffected, or even increased, by depth (Holstein et al., 2016b; Goodbody-Gringley et al., 2018). Planulae production increased with depth and was correlated to peak P. astreoides cover at 10 m and at 35 m off the U.S. Virgin Islands (Holstein et al., 2016b). Fecundity was maintained across 2 m to 33 m in Bermuda, and recruits collected from the upper-mesophotic zone showed higher settlement, growth, and survival rates (Goodbody-Gringley et al., 2018). In addition, a reciprocal transplantation experiment between shallow and mesophotic reefs showed that P. astreoides larvae survived and settled independently from the parental origin and that mesophotic light conditions increased survivorship (Goodbody-Gringley et al., 2021). Thus, mesophotic reefs may provide a refuge for P. astreoides enabling recruitment to shallower reefs (Holstein et al., 2016a).
OA decreased P. astreoides larval survivorship (Olsen et al., 2015), metabolism, settlement, post-settlement growth (Albright and Langdon, 2011), and recruit calcification (de Putron et al., 2011), but mild pH reductions (from 8.05 to 7.85) had no effects on settlement and survival of recruits (Campbell et al., 2017).
Larval physiology, survival, settlement, and metamorphosis were resistant to heat stress (Olsen et al., 2013; Ross et al., 2013; Ritson-Williams et al., 2016), although it caused inhibition of larval photochemical efficiency (Olsen et al., 2015), oxidative damage in the larval tissues (Olsen et al., 2013; Ritson-Williams et al., 2016), and post-settlement mortality (Ross et al., 2013). P. astreoides recruitment was reduced by half at sites that were highly exposed to an anomalous cold-water plume in the Florida Keys in 2010, and the effect was apparent three years after the event (Kemp et al., 2016).
Survival of P. astreoides recruits was lower under heat (30°C) when compared to ambient temperatures (26°C) and sedimentation was a key factor in either ameliorating or aggravating the effects of thermal stress (Fourney and Figueiredo, 2017). When the levels of sedimentation were low (30 mg.cm−2, 6.55 NTU), coral recruits survived at rates closer to those under ambient temperatures. In contrast, high amounts of fine, anthropogenic sediment (≥ 60 mg.cm−2, ≥ 14.2 NTU) significantly increased mortality of coral recruits under high temperatures (Fourney and Figueiredo, 2017). In a different study system, the relationship between sediment grain size and P. astreoides recruitment was reversed; fine sediment did not affect recruit survivorship or health, but coarse sediment did (Rushmore et al., 2021).
Exposure to fleshy macroalgae was harmful to P. astreoides recruitment (Paul et al., 2011), particularly when abiotic stressors were added (Olsen et al., 2015; Campbell et al., 2017). Dictyota significantly reduced larval survival and recruitment (Paul et al., 2011; Olsen et al., 2015). In synergy with OA and heat, Dictyota caused a four-fold increase in lipid peroxidation in P. astreoides larvae, which indicated cellular oxidative damage compared to control treatments (Olsen et al., 2015). When in contact with Stypopodium zonale, the survival of larvae was lower after 96 hours, but settlement was not affected (Campbell et al., 2017). In contrast, OA in combination with S. zonale significantly reduced settlement (Campbell et al., 2017).
Tissue Condition: Protein, lipid, and carbohydrate concentration/composition, tissue Carbon and Nitrogen isotopes, oxidative stress biomarkers, innate immune system gene expression
The coral tissue properties of P. astreoides were sensitive to sedimentation (Rushmore et al., 2021), strongly shaped by temperature fluctuations (Kenkel et al., 2015a; Schoepf et al., 2015; Solomon et al., 2019), but sometimes resilient to thermal stress (Haslun et al., 2018; Levas et al., 2018), and OA (Martinez et al., 2019). Porites astreoides colonies showed increased levels of oxidative stress biomarkers in the tissue (carbonyl content, hydroperoxide) after exposure to moderate to high levels of sedimentation and did not show signs of recovery after 5 days of sediment removal (Rushmore et al., 2021). However, innate immune system-related genes maintained low levels of expression under a 32°C treatment, indicating resistance to thermal stress (Haslun et al., 2018). Porites astreoides was able to maintain lipids, proteins, and carbohydrates reserves during and after one year of exposure to a mild bleaching event (Levas et al., 2018), however, the species did not seem to rely on a long-term recovery capacity of their tissue condition following recurrent bleaching events (Schoepf et al., 2015). The most negative effects of thermal stress on P. astreoides tissue condition were a decrease of protein and carbohydrate concentrations and a higher amount of heterotrophic C versus photoautotrophic C (δ13Ch−e) in the coral tissue, suggesting that colonies invested more in heterotrophy to compensate for the lack of energy reserves (Schoepf et al., 2015). Lipid class composition changed dramatically after recurrent bleaching events, with a 50% decline in wax esters (i.e., storage lipids) (Solomon et al., 2019).
Reciprocally transplanted colonies between inner and outer reefs in the Florida Keys experienced a decrease in mass gain and total lipid, protein, and carbohydrate content after one year. Reef-scale specialization to temperature, especially the frequency of thermal fluctuations, was considered the primary driver (Kenkel et al., 2015a). In contrast, high protein concentration was maintained after a two-year transplantation to a low aragonite saturation submarine spring, indicating acclimatization of the tissue properties to the suboptimum conditions (Martinez et al., 2019).
Microbiome: Coral-associated microbial community
The literature search identified only three papers that described the microbiomes associated with P. astreoides in response to stress and the stress in each case was associated with macroalgae. The microbiome associated with P. astreoides was altered in the presence of macroalgae (Vega Thurber et al., 2012; Morrow et al., 2013), but the response depended on the macroalgae species (Morrow et al., 2012). Lobophora variegate aqueous extracts caused dysbiosis, while Dictyota (organic) extracts had a significant effect on bacterial assemblages (Morrow et al., 2012). Microbial taxa composition in the surface mucous layer of P. astreoides was disrupted by exposure to macroalgae, where each macroalgal species induced a different response compared with control treatments (Vega Thurber et al., 2012). The presence of macroalgae increased variability in the coral microbiome among colonies within the same treatment, which could be a sign of the loss of beneficial microbes and microbiome dysbiosis (Vega Thurber et al., 2012). In situ interactions between P. astreoides colonies and macroalgae Dictyota menstrualis and Halimeda opuntia in the U.S. Virgin Islands, the Florida Keys, and Belize, shifted the coral-associated microbial communities (Morrow et al., 2013). In the coral-algal competition zone, the coral P. astreoides maintained a relatively more stable microbiome compared to the coral Montastrea cavernosa and provided a competitive edge for P. astreoides against macroalgae (Morrow et al., 2013).
Discussion
Caribbean reefs have undergone a large-scale degradation in the last four decades (Pandolfi and Jackson, 2006; Eakin et al., 2010; Jackson et al., 2014; Cramer et al., 2021), which has led coral reef ecologists to search for coral species that will bring life and hope to future reefs. Porites astreoides has been considered a winner (Green et al., 2008; Edmunds, 2010; Soto-Santiago et al., 2017a) and expected to play a key role in sustaining coral cover in the Caribbean as more sensitive species (e.g., Acropora spp.) become consummate losers (Baums et al., 2005; Williams et al., 2008; García-Urueña and Garzón-Machado, 2020). However, P. astreoides may not be as resilient as previously assumed (Grottoli et al., 2014; Edmunds et al., 2021). Here we show that Porites astreoides has been more often reported to be sensitive than resistant and/or resilient to stress. However, each physiological feature showed varying levels of sensitivity depending on the stressor, which brings more ambiguity about the ability of the species to thrive under future climate scenarios (Figure 1). The greatest source of resilience of P. astreoides lie in their ability to sustain growth rates and recruitment at higher depths in mesophotic reefs (Holstein et al., 2016b; Goodbody-Gringley et al., 2018; Groves et al., 2018), which may function as a refuge from stressors faced in shallow reefs (Holstein et al., 2016a). In contrast, macroalgal competition and synergistic effects of multiple stressors combined, were highly deleterious to P. astreoides (Olsen et al., 2015; Campbell et al., 2017).
Finally, the least understood relationship was between the microbiome of P. astreoides and environmental stress and was only evaluated in the context of competition with macroalgae (Vega Thurber et al., 2012; Morrow et al., 2013). The microbiome of P. astreoides can be vertically transmitted to brooded larvae (Sharp et al., 2012) and is key to their health and survival (Rodriguez‐Lanetty et al., 2013; Meyer et al., 2014; Welsh et al., 2015; Glasl et al., 2016). Disease susceptibility of P. astreoides ranges from low to intermediate (Williams et al., 2020; Meiling et al., 2021) and could be explained by their microbiome (MacKnight et al., 2021). Future studies should investigate how environmental factors, especially temperature (Lima et al., 2020), affect the microbiome of P. astreoides and which microbial partners and microbial gene functions (Dinsdale and Rohwer, 2011; Haas et al., 2016; Walsh et al., 2017; Santoro et al., 2021) can increase resilience of the species.
In relation to other corals, P. astreoides was the most thermally sensitive species when compared to Porites divaricata (Grottoli et al., 2014; Schoepf et al., 2015), Siderastrea siderea (Kemp et al., 2011; Smith et al., 2019), and Orbicella faveolata (Kemp et al., 2011; Grottoli et al., 2014; Schoepf et al., 2015), and more sensitive than Montastrea cavernosa to sedimentation (Rushmore et al., 2021). Under present-day temperature and pH conditions, P. astreoides was a stronger competitor than M. cavernosa and O. faveolata, but under thermal stress and low pH it lost competitive ability (Johnston et al., 2020). P. astreoides populations already show signs of decline (Edmunds et al., 2021), which could be explained by their relatively low resistance and/or resilience to stress compared to other coral species. In the Pacific Ocean, while some Porites species were short-term winners, none were considered long-term winners in response to bleaching events (van Woesik et al., 2011).
Increasing local and global anthropogenic stressors (Suchley and Alvarez-Filip, 2018; Muñiz-Castillo et al., 2019) may consummate P. astreoides as a loser in Caribbean reefs. However, resilient aspects of P. astreoides such as growth and recruitment at depth, provides competitive advantage to the species. In addition, physiological tolerance of P. astreoides could be manipulated and increased by transgenerational acclimatization mechanisms (Kenkel et al., 2015b) in the coral host (Dimond and Roberts, 2020; Wong et al., 2021), and in the coral microbiome (Webster and Reusch, 2017). The future of P. astreoides and of the Caribbean reefs are tightly integrated and rely on effective management practices supported by more scientific data reporting on their ecophysiology.
Author contributions
LL designed the study, compiled and analyzed the data, wrote and reviewed the manuscript. HB compiled and analyzed the data, and reviewed the manuscript. ED wrote and reviewed the manuscript. All authors contributed to the article and approved the submitted version.
Conflict of interest
The authors declare that the research was conducted in the absence of any commercial or financial relationships that could be construed as a potential conflict of interest.
Publisher’s note
All claims expressed in this article are solely those of the authors and do not necessarily represent those of their affiliated organizations, or those of the publisher, the editors and the reviewers. Any product that may be evaluated in this article, or claim that may be made by its manufacturer, is not guaranteed or endorsed by the publisher.
Supplementary material
The Supplementary Material for this article can be found online at: https://www.frontiersin.org/articles/10.3389/fmars.2022.908734/full#supplementary-material
References
Albright R., Langdon C. (2011). Ocean acidification impacts multiple early life history processes of the Caribbean coral porites astreoides. Glob. Change Biol. 17, 2478–2487. doi: 10.1111/j.1365-2486.2011.02404.x
Anthony K., Connolly S. R. (2004). Environmental limits to growth: physiological niche boundaries of corals along turbidity–light gradients. Oecologia 141, 373–384. doi: 10.1007/s00442-004-1647-7
Baumann J. H., Townsend J. E., Courtney T. A., Aichelman H. E., Davies S. W., Lima F. P., et al. (2016). Temperature regimes impact coral assemblages along environmental gradients on lagoonal reefs in Belize. PloS One 11, e0162098. doi: 10.1371/journal.pone.0162098
Baums I. B., Miller M. W., Hellberg M. E. (2005). Regionally isolated populations of an imperiled Caribbean coral, acropora palmata. Mol. Ecol. 14, 1377–1390. doi: 10.1111/j.1365-294X.2005.02489.x
Bove C. B., Ries J. B., Davies S. W., Westfield I. T., Umbanhowar J., Castillo K. D. (2019). Common Caribbean corals exhibit highly variable responses to future acidification and warming. Proc. R. Soc B. 286, 20182840. doi: 10.1098/rspb.2018.2840
Campbell J. E., Sneed J. M., Johnston L., Paul V. J. (2017). Effects of ocean acidification and contact with the brown alga stypopodium zonale on the settlement and early survival of the coral porites astreoides. Mar. Ecol. Prog. Ser. 577, 67–77. doi: 10.3354/meps12249
Camp E. F., Smith D. J., Evenhuis C., Enochs I., Manzello D., Woodcock S., et al. (2016). Acclimatization to high-variance habitats does not enhance physiological tolerance of two key Caribbean corals to future temperature and pH. Proc. R. Soc B. Biol. Sci. 283, 20160442. doi: 10.1098/rspb.2016.0442
Chornesky E. A., Peters E. C. (1987). Sexual reproduction and colony growth in the scleractinian coral porites astreoides. Biol. Bull. 172, 161–177. doi: 10.2307/1541790
Côté I. M., Darling E. S. (2010). Rethinking ecosystem resilience in the face of climate change. PloS Biol. 8, e1000438. doi: 10.1371/journal.pbio.1000438
Cramer K. L., Jackson J. B., Donovan M. K., Greenstein B. J., Korpanty C. A., Cook G. M., et al. (2020). Widespread loss of Caribbean acroporid corals was underway before coral bleaching and disease outbreaks. Science Adv. 6 (7), eaax9395. doi: 10.1126/sciadv.aax9395
Cramer K. L., Donovan M. K., Jackson J. B. C., Greenstein B. J., Korpanty C. A., Cook G. M., et al. (2021). The transformation of Caribbean coral communities since humans. Ecol. Evol. 11, 10098–10118. doi: 10.1002/ece3.7808
Crook E. D., Cohen A. L., Rebolledo-Vieyra M., Hernandez L., Paytan A. (2013). Reduced calcification and lack of acclimatization by coral colonies growing in areas of persistent natural acidification. Proc. Natl. Acad. Sci. 110, 11044–11049. doi: 10.1073/pnas.1301589110
de Putron S. J., McCorkle D. C., Cohen A. L., Dillon A. B. (2011). The impact of seawater saturation state and bicarbonate ion concentration on calcification by new recruits of two Atlantic corals. Coral. Reefs. 30, 321–328. doi: 10.1007/s00338-010-0697-z
de Putron S. J., Smith S. R. (2011). Planula release and reproductive seasonality of the scleractinian coral porites astreoides in bermuda, a high-latitude reef. Bull. Mar. Sci. 87, 75–90. doi: 10.5343/bms.2009.1027
Dimond J. L., Roberts S. B. (2020). Convergence of DNA methylation profiles of the reef coral porites astreoides in a novel environment. Front. Mar. Sci. 6, 792. doi: 10.3389/fmars.2019.00792
Dinsdale E. A., Rohwer F. (2011). “Fish or germs? Microbial dynamics associated with changing trophic structures on coral reefs,” in Coral reefs: an ecosystem in transition ( Dordrecht: Springer), 231–240.
Dustan P., Doherty O., Pardede S. (2013). Digital reef rugosity estimates coral reef habitat complexity. PloS One 8, e57386. doi: 10.1371/journal.pone.0057386
Eakin C. M., Morgan J. A., Heron S. F., Smith T. B., Liu G., Alvarez-Filip L., et al. (2010). Caribbean Corals in crisis: Record thermal stress, bleaching, and mortality in 2005. PloS One 5 (11), e13969. doi: 10.1371/journal.pone.0013969
Edmunds P. J. (2000). Patterns in the distribution of juvenile corals and coral reef community structure in st. John, US Virgin Islands. Mar. Ecol. Prog. Ser. 202, 113–124. doi: 10.3354/meps202113
Edmunds P. J. (2010). Population biology of porites astreoides and diploria strigosa on a shallow Caribbean reef. Mar. Ecol. Prog. Ser. 418, 87–104. doi: 10.3354/meps08823
Edmunds P. J., Didden C., Frank K. (2021). Over three decades, a classic winner starts to lose in a Caribbean coral community. Ecosphere 12, e03517. doi: 10.1002/ecs2.3517
Elizalde-Rendón E. M., Horta-Puga G., González-Diaz P., Carricart-Ganivet J. P. (2010). Growth characteristics of the reef-building coral porites astreoides under different environmental conditions in the Western Atlantic. Coral. Reefs. 29, 607–614. doi: 10.1007/s00338-010-0604-7
Fourney F., Figueiredo J. (2017). Additive negative effects of anthropogenic sedimentation and warming on the survival of coral recruits. Sci. Rep. 7, 12380. doi: 10.1038/s41598-017-12607-w
García-Urueña R., Garzón-Machado M. A. (2020). Current status of acropora palmata and acropora cervicornis in the Colombian Caribbean: demography, coral cover and condition assessment. Hydrobiologia 847, 2141–2153. doi: 10.1007/s10750-020-04238-6
Gates R. D. (1990). Seawater temperature and sublethal coral bleaching in Jamaica. Coral. Reefs. 8, 193–197. doi: 10.1007/BF00265010
Glasl B., Herndl G. J., Frade P. R. (2016). The microbiome of coral surface mucus has a key role in mediating holobiont health and survival upon disturbance. ISME. J. 10, 2280–2292. doi: 10.1038/ismej.2016.9
Gleason D. F. (1998). Sedimentation and distributions of green and brown morphs of the Caribbean coral porites astreoides lamarck. J. Exp. Mar. Bio. Ecol. 230, 73–89. doi: 10.1016/S0022-0981(98)00084-7
Goodbody-Gringley G., Scucchia F., Ju R., Chequer A., Einbinder S., Martinez S., et al. (2021). Plasticity of porites astreoides early life history stages suggests mesophotic coral ecosystems act as refugia in Bermuda. Front. Mar. Sci. 1215. doi: 10.3389/fmars.2021.702672
Goodbody-Gringley G., Wong K. H., Becker D. M., Glennon K., de Putron S. J. (2018). Reproductive ecology and early life history traits of the brooding coral, porites astreoides, from shallow to mesophotic zones. Coral. Reefs. 37, 483–494. doi: 10.1007/s00338-018-1673-2
Green D. H., Edmunds P. J., Carpenter R. C. (2008). Increasing relative abundance of porites astreoides on Caribbean reefs mediated by an overall decline in coral cover. Mar. Ecol. Prog. Ser. 359, 1–10. doi: 10.3354/meps07454
Grottoli A. G., Warner M. E., Levas S. J., Aschaffenburg M. D., Schoepf V., McGinley M., et al. (2014). The cumulative impact of annual coral bleaching can turn some coral species winners into losers. Glob. Change Biol. 20, 3823–3833. doi: 10.1111/gcb.12658
Groves S. H., Holstein D. M., Enochs I. C., Kolodzeij G., Manzello D. P., Brandt M. E., et al. (2018). Growth rates of porites astreoides and orbicella franksi in mesophotic habitats surrounding st. Thomas, US Virgin Islands. Coral. Reefs. 37, 345–354. doi: 10.1007/s00338-018-1660-7
Haas A. F., Fairoz M. F. M., Kelly L. W., Nelson C. E., Dinsdale E. A., Edwards R. A., et al. (2016). Global microbialization of coral reefs. Nat. Microbiol. 1, 1–7. doi: 10.1038/nmicrobiol.2016.42
Haslun J. A., Hauff-Salas B., Strychar K. B., Ostrom N. E., Cervino J. M. (2018). Biotic stress contributes to seawater temperature induced stress in a site-specific manner for porites astreoides. Mar. Biol. 165, 1–13. doi: 10.1007/s00227-018-3414-z
Hoadley K. D., Pettay D. T., Grottoli A. G., Cai W.-J., Melman T. F., Schoepf V., et al. (2015). Physiological response to elevated temperature and pCO2 varies across four pacific coral species: Understanding the unique host+ symbiont response. Sci. Rep. 5, 1–15. doi: 10.1038/srep18371
Hoegh-Guldberg O., Poloczanska E. S., Skirving W., Dove S. (2017). Coral reef ecosystems under climate change and ocean acidification. Front. Mar. Sci. 4. doi: 10.3389/fmars.2017.00158
Holstein D. M., Paris C. B., Vaz A. C., Smith T. B. (2016a). Modeling vertical coral connectivity and mesophotic refugia. Coral. Reefs. 35, 23–37. doi: 10.1007/s00338-015-1339-2
Holstein D. M., Smith T. B., Paris C. B. (2016b). Depth-independent reproduction in the reef coral porites astreoides from shallow to mesophotic zones. PloS One 11, e0146068. doi: 10.1371/journal.pone.0146068
Hughes T. P. (1994). Catastrophes, phase shifts, and large-scale degradation of a Caribbean coral reef. Sci. (80-. ). 265, 1547–1551. doi: 10.1126/science.265.5178.1547
Hughes T. P., Anderson K. D., Connolly S. R., Heron S. F., Kerry J. T., Lough J. M., et al. (2018). Spatial and temporal patterns of mass bleaching of corals in the anthropocene. Sci. (80-. ). 359, 80–83. doi: 10.1126/science.aan8048
Hughes T. P., Rodrigues M. J., Bellwood D. R., Ceccarelli D., Hoegh-Guldberg O., McCook L., et al. (2007). Phase shifts, herbivory, and the resilience of coral reefs to climate change. Curr. Biol. 17, 360–365. doi: 10.1016/j.cub.2006.12.049
Jackson J. B. C., Donovan M. K., Cramer K. L., Lam V. V. (2014). Status and trends of Caribbean coral reefs. Glob. Coral. Reef. Monit. Network. IUCN. Gland. Switz. 1970–2012.
Johnston N. K., Campbell J. E., Paul V. J., Hay M. E. (2020). Effects of future climate on coral-coral competition. PloS One 15, e0235465. doi: 10.1371/journal.pone.0235465
Jones R. J., Hoegh-Guldberg O., Larkum A. W. D., Schreiber U. (1998). Temperature-induced bleaching of corals begins with impairment of the CO2fixation mechanism in zooxanthellae. Plant. Cell Environ. 21, 1219–1230. doi: 10.1046/j.1365-3040.1998.00345.x
Kemp D. W., Colella M. A., Bartlett L. A., Ruzicka R. R., Porter J. W., Fitt W. K. (2016). Life after cold death: reef coral and coral reef responses to the 2010 cold water anomaly in the Florida keys. Ecosphere 7, e01373. doi: 10.1002/ecs2.1373
Kemp D. W., Oakley C. A., Thornhill D. J., Newcomb L. A., Schmidt G. W., Fitt W. K. (2011). Catastrophic mortality on inshore coral reefs of the Florida keys due to severe low-temperature stress. Glob. Change Biol. 17, 3468–3477. doi: 10.1111/j.1365-2486.2011.02487.x
Kenkel C. D., Almanza A. T., Matz M. V. (2015a). Fine-scale environmental specialization of reef-building corals might be limiting reef recovery in the Florida keys. Ecology 96, 3197–3212. doi: 10.1890/14-2297.1
Kenkel C. D., Setta S. P., Matz M. V. (2015b). Heritable differences in fitness-related traits among populations of the mustard hill coral, porites astreoides. Heredity. (Edinb). 115, 509–516. doi: 10.1038/hdy.2015.52
Kleypas J. A., McManus J. W., Meñez L. A. B. (1999). Environmental limits to coral reef development: Where do we draw the line? Am. Zool. 39, 146–159. doi: 10.1093/icb/39.1.146
Levas S., Schoepf V., Warner M. E., Aschaffenburg M., Baumann J., Grottoli A. G. (2018). Long-term recovery of Caribbean corals from bleaching. J. Exp. Mar. Bio. Ecol. 506, 124–134. doi: 10.1016/j.jembe.2018.06.003
Lima L. F. O., Weissman M., Reed M., Papudeshi B., Alker A. T., Morris M. M., et al. (2020). Modeling of the coral microbiome: The influence of temperature and microbial network. MBio 11 (2), e02691-19. doi: 10.1128/mBio.02691-19
Loya Y., Sakai K., Yamazato K., Nakano Y., Sambali H., Van Woesik R. (2001). Coral bleaching: The winners and the losers. Ecol. Lett. 4, 122–131. doi: 10.1046/j.1461-0248.2001.00203.x
MacKnight N. J., Cobleigh K., Lasseigne D., Chaves-Fonnegra A., Gutting A., Dimos B., et al. (2021). Microbial dysbiosis reflects disease resistance in diverse coral species. Commun. Biol. 4, 679. doi: 10.1038/s42003-021-02163-5
Manzello D. P., Enochs I. C., Kolodziej G., Carlton R. (2015). Coral growth patterns of montastraea cavernosa and porites astreoides in the Florida keys: The importance of thermal stress and inimical waters. J. Exp. Mar. Bio. Ecol. 471, 198–207. doi: 10.1016/j.jembe.2015.06.010
Martinez A., Crook E. D., Barshis D. J., Potts D. C., Rebolledo-Vieyra M., Hernandez L., et al. (2019). Species-specific calcification response of Caribbean corals after 2-year transplantation to a low aragonite saturation submarine spring. Proc. R. Soc B. 286, 20190572. doi: 10.1098/rspb.2019.0572
Maynard J., Van Hooidonk R., Eakin C. M., Puotinen M., Garren M., Williams G., et al. (2015). Projections of climate conditions that increase coral disease susceptibility and pathogen abundance and virulence. Nat. Clim. Change 5, 688. doi: 10.1038/nclimate2625
McGuire M. P. (1998). Timing of larval release by porites astreoides in the Northern Florida keys. Coral. Reefs. 17, 369–375. doi: 10.1007/s003380050141
Meiling S. S., Muller E. M., Lasseigne D., Rossin A., Veglia A. J., MacKnight N., et al. (2021). Variable species responses to experimental stony coral tissue loss disease (SCTLD) exposure. Front. Mar. Sci. 8. doi: 10.3389/fmars.2021.670829
Meyer J. L., Paul V. J., Teplitski M. (2014). Community shifts in the surface microbiomes of the coral porites astreoides with unusual lesions. PloS One 9 (6), e100316. doi: 10.1371/journal.pone.0100316
Morrow K. M., Liles M. R., Paul V. J., Moss A. G., Chadwick N. E. (2013). Bacterial shifts associated with coral–macroalgal competition in the Caribbean Sea. Mar. Ecol. Prog. Ser. 488, 103–117. doi: 10.3354/meps10394
Morrow K. M., Ritson-Williams R., Ross C., Liles M. R., Paul V. J. (2012). Macroalgal extracts induce bacterial assemblage shifts and sublethal tissue stress in Caribbean corals. Appl. Environ.Mmicrobiol. 78 (18), 6438–6449 doi: 10.1371/journal.pone.0044859
Muñiz-Castillo A. I., Rivera-Sosa A., Chollett I., Eakin C. M., Andrade-Gómez L., McField M., et al. (2019). Three decades of heat stress exposure in Caribbean coral reefs: A new regional delineation to enhance conservation. Sci. Rep. 9, 11013. doi: 10.1038/s41598-019-47307-0
Mydlarz L. D., McGinty E. S., Harvell C. D. (2010). What are the physiological and immunological responses of coral to climate warming and disease? J. Exp. Biol. 213, 934–945. doi: 10.1242/jeb.037580
Okazaki R. R., Towle E. K., van Hooidonk R., Mor C., Winter R. N., Piggot A. M., et al. (2017). Species-specific responses to climate change and community composition determine future calcification rates of Florida keys reefs. Glob. Change Biol. 23, 1023–1035. doi: 10.1111/gcb.13481
Olsen K., Paul V. J., Ross C. (2015). Direct effects of elevated temperature, reduced pH, and the presence of macroalgae (Dictyota spp.) on larvae of the Caribbean coral porites astreoides. Bull. Mar. Sci. 91, 255–270. doi: 10.5343/bms.2014.1050
Olsen K., Ritson-Williams R., Ochrietor J. D., Paul V. J., Ross C. (2013). Detecting hyperthermal stress in larvae of the hermatypic coral porites astreoides: The suitability of using biomarkers of oxidative stress versus heat-shock protein transcriptional expression. Mar. Biol. 160, 2609–2618. doi: 10.1007/s00227-013-2255-z
Pandolfi J. M., Connoll S., Marshall D., Cohen A. L. (2011). Projecting coral reef futures under global warming and ocean acidification. Sci. (80-. ). 333, 418–422. doi: 10.1126/science.1204794
Pandolfi J. M., Jackson J. B. C. (2006). Ecological persistence interrupted in Caribbean coral reefs. Ecol. Lett. 9, 818–826. doi: 10.1111/j.1461-0248.2006.00933.x
Paul V. J., Kuffner I. B., Walters L. J., Ritson-Williams R., Beach K. S., Becerro M. A. (2011). Chemically mediated interactions between macroalgae dictyota spp. and multiple life-history stages of the coral porites astreoides. Mar. Ecol. Prog. Ser. 426, 161–170. doi: 10.3354/meps09032
Porter J. W., Battey J. F., Smith G. J. (1982). Perturbation and change in coral reef communities. Proc. Natl. Acad. Sci. 79, 1678–1681. doi: 10.1073/pnas.79.5.1678
Randall C. J., Jordan-Garza A. G., Muller E. M., Van Woesik R. (2014). Relationships between the history of thermal stress and the relative risk of diseases of Caribbean corals. Ecology 95, 1981–1994. doi: 10.1890/13-0774.1
Ritson-Williams R., Ross C., Paul V. J. (2016). Elevated temperature and allelopathy impact coral recruitment. PloS One 11, e0166581. doi: 10.1371/journal.pone.0166581
Rodriguez-Lanetty M., Granados-Cifuentes C., Barberan A., Bellantuono A. J., Bastidas C. (2013). Ecological inferences from a deep screening of the complex b acterial c onsortia associated with the coral, p orites astreoides. Mol. Ecol. 22, 4349–4362. doi: 10.1111/mec.12392
Ross C., Ritson-Williams R., Olsen K., Paul V. J. (2013). Short-term and latent post-settlement effects associated with elevated temperature and oxidative stress on larvae from the coral porites astreoides. Coral. Reefs. 32, 71–79. doi: 10.1007/s00338-012-0956-2
Rushmore M. E., Ross C., Fogarty N. D. (2021) Physiological responses to short-term sediment exposure in adults of the Caribbean coral montastraea cavernosa and adults and recruits of porites astreoides Coral. Reefs. 40 (5), 1579–1591. doi: 10.1007/s00338-021-02156-0
Safaie A., Silbiger N. J., McClanahan T. R., Pawlak G., Barshis D. J., Hench J. L., et al. (2018). High frequency temperature variability reduces the risk of coral bleaching. Nat. Commun. 9 (1), 1–12. doi: 10.1038/s41467-018-04074-2
Santoro E. P., Borges R. M., Espinoza J. L., Freire M., Messias C. S. M. A., Villela H. D. M., et al. (2021). Coral microbiome manipulation elicits metabolic and genetic restructuring to mitigate heat stress and evade mortality. Sci. Adv. 7, eabg3088. doi: 10.1126/sciadv.abg3088
Schoepf V., Grottoli A. G., Levas S. J., Aschaffenburg M. D., Baumann J. H., Matsui Y., et al. (2015). Annual coral bleaching and the long-term recovery capacity of coral. Proc. R. Soc B. Biol. Sci. 282, 20151887. doi: 10.1098/rspb.2015.1887
Sharp K. H., Distel D., Paul V. J. (2012). Diversity and dynamics of bacterial communities in early life stages of the Caribbean coral porites astreoides. ISME. J. 6, 790–801. doi: 10.1038/ismej.2011.144
Smith T. B., Brandt M. E., Calnan J. M., Nemeth R. S., Blondeau J., Kadison E., et al. (2013). Convergent mortality responses of Caribbean coral species to seawater warming. Ecosphere 4, 1–40. doi: 10.1890/ES13-00107.1
Smith K. M., Payton T. G., Sims R. J., Stroud C. S., Jeanes R. C., Hyatt T. B., et al. (2019). Impacts of consecutive bleaching events and local algal abundance on transplanted coral colonies in the Florida keys. Coral. Reefs. 38, 851–861. doi: 10.1007/s00338-019-01823-7
Solomon S. L., Grottoli A. G., Warner M. E., Levas S., Schoepf V., Muñoz-Garcia A. (2019). Lipid class composition of annually bleached Caribbean corals. Mar. Biol. 167, 7. doi: 10.1007/s00227-019-3616-z
Soong K. (1991). Sexual reproductive patterns of shallow-water reef corals in Panama. Bull. Mar. Sci. 49, 832–846.
Soto-Santiago F., Mercado-Molina A., Reyes Maldonado K., Sabat A. (2017b). Physiological response to thermal stress of the Caribbean corals orbicella annularis and porites astreoides. Res. J. Environ. Sci. 11, 48–57. doi: 10.3923/rjes.2017.48.57
Soto-Santiago F. J., Mercado-Molina A., Reyes-Maldonado K., Vélez Y., Ruiz-Díaz C. P., Sabat A. (2017a). Comparative demography of two common scleractinian corals: Orbicella annularis and porites astreoides. PeerJ 5, e3906. doi: 10.7717/peerj.3906
Suchley A., Alvarez-Filip L. (2018). Local human activities limit marine protection efficacy on Caribbean coral reefs. Conserv. Lett. 11, e12571. doi: 10.1111/conl.12571
Tomascik T., Sander F. (1987). Effects of eutrophication on reef-building corals. Mar. Biol. 94, 53–75. doi: 10.1007/BF00392900
van Hooidonk R. J., Manzello D. P., Moye J., Brandt M. E., Hendee J. C., McCoy C., et al. (2012). Coral bleaching at little cayman, Cayman islands 2009. Estuar. Coast. Shelf. Sci. 106, 80–84. doi: 10.1016/j.ecss.2012.04.021
van Woesik R., Sakai K., Ganase A., Loya Y. (2011). Revisiting the winners and the losers a decade after coral bleaching. Mar. Ecol. Prog. Ser. 434, 67–76. doi: 10.3354/meps09203
Vega Thurber R., Burkepile D. E., Correa A. M. S., Thurber A. R., Shantz A. A., Welsh R., et al. (2012). Macroalgae decrease growth and alter microbial community structure of the reef-building coral, porites astreoides. PLoS One 7 (9), e44246. doi: 10.1371/journal.pone.0044246
Wall M., Fietzke J., Crook E. D., Paytan A. (2019). Using b isotopes and B/Ca in corals from low saturation springs to constrain calcification mechanisms. Nat. Commun. 10, 1–9. doi: 10.1038/s41467-019-11519-9
Walsh K., Haggerty J. M., Doane M. P., Hansen J. J., Morris M. M., Moreira A. P. B., et al. (2017). Aura-biomes are present in the water layer above coral reef benthic macro-organisms. PeerJ 5, e3666. doi: 10.7717/peerj.3666
Webster N. S., Reusch T. B. H. (2017). Microbial contributions to the persistence of coral reefs. ISME. J. 11, 2167–2174. doi: 10.1038/ismej.2017.66
Welsh R. M., Zaneveld J. R., Rosales S. M., Payet J. P., Burkepile D. E., Thurber R. V. (2015). Bacterial predation in a marine host-associated microbiome. Isme. J. 10, 1540. doi: 10.1038/ismej.2015.219
Williams D. E., Miller M. W., Kramer K. L. (2008). Recruitment failure in Florida keys acropora palmata, a threatened Caribbean coral. Coral. Reefs. 27, 697–705. doi: 10.1007/s00338-008-0386-3
Williams L., Smith T. B., Burge C. A., Brandt M. E. (2020). Species-specific susceptibility to white plague disease in three common Caribbean corals. Coral. Reefs. 39, 27–31. doi: 10.1007/s00338-019-01867-9
Wong K. H., Goodbody-Gringley G., de Putron S. J., Becker D. M., Chequer A., Putnam H. M. (2021). Brooded coral offspring physiology depends on the combined effects of parental press and pulse thermal history. Glob. Change Biol 27 (13), 3179–3195. doi: 10.1111/gcb.15629
Keywords: climate change, acclimatization, stress response, environmental disturbances, resistance
Citation: Lima LFO, Bursch H and Dinsdale EA (2022) Win some, lose some: The ecophysiology of Porites astreoides as a key coral species to Caribbean reefs. Front. Mar. Sci. 9:908734. doi: 10.3389/fmars.2022.908734
Received: 31 March 2022; Accepted: 19 July 2022;
Published: 16 August 2022.
Edited by:
Cliff Ross, University of North Florida, United StatesReviewed by:
James Dimond, Western Washington University, United StatesBouchon Claude, Université des Antilles, Guadeloupe
Copyright © 2022 Lima, Bursch and Dinsdale. This is an open-access article distributed under the terms of the Creative Commons Attribution License (CC BY). The use, distribution or reproduction in other forums is permitted, provided the original author(s) and the copyright owner(s) are credited and that the original publication in this journal is cited, in accordance with accepted academic practice. No use, distribution or reproduction is permitted which does not comply with these terms.
*Correspondence: Lais F.O. Lima, bGxpbWFAc2RzdS5lZHU=