- 1Department of Health and Human Performance, School of Health Sciences, College of Charleston, Charleston, SC, United States
- 2Department of Environmental Health Sciences, Arnold School of Public Health, University of South Carolina, Columbia, SC, United States
- 3Brookfield Zoo Chicago’s Sarasota Dolphin Research Program, ℅ Mote Marine Laboratory, Sarasota, FL, United States
- 4Conservation Medicine, National Marine Mammal Foundation, San Diego, CA, United States
- 5Executive Operations, National Marine Mammal Foundation, San Diego, CA, United States
- 6Department of Psychology, New College Florida, Sarasota, FL, United States
Introduction: Exposure to phthalate esters has previously been documented in bottlenose dolphins (Tursiops truncatus) inhabiting an urban estuary (Sarasota Bay, FL, USA; 2010-2019). Phthalates are chemicals commonly added to plastic products and consumer goods to enhance qualities such as flexibility, fragrance, and stability. Chemical leaching from products into the marine environment leaves wildlife vulnerable to reproductive, developmental, and metabolic impairment. Environmental phthalate exposure has been shown to vary relative to human activity and urbanization.
Methods: To evaluate potential differences in dolphin exposure risk, urine was collected from free-ranging bottlenose dolphins residing in an urban (Sarasota Bay, FL, USA; 2010-2024; n=71) and rural estuary (Barataria Bay, LA, USA; 2011- 2023; n=45). Urinary phthalate metabolite concentrations were quantified with high-performance liquid chromatography (HPLC; Agilent 1100; WatersXBridge BEH C18, 2.5 μm, 2.1x50 mm analytical column) coupled to a triple quadrupole mass spectrometer (MS; Applied Biosystems Sciex API 4000) with an electrospray ionization (ESI negative) interface.
Results: The magnitude of MEHP detection did not differ significantly between sampling sites (p=0.97); however, MEHP was detected more frequently in Sarasota Bay dolphins (73.24%; n=52; 95% CI: 61.20-82.73) than Barataria Bay dolphins (33.33%; n=15; 95% CI: 20.00-48.95%). Dolphins from Sarasota Bay may be exposed to a greater diversity of phthalates compared to Barataria Bay dolphins, indicated by differences in the detected phthalate metabolite profile.
Discussion: Notably, dolphins from Barataria Bay were impacted by the Deepwater Horizon oil spill, with evidence suggesting long-term negative health outcomes. The endocrine-disrupting effects of phthalates could exacerbate metabolic, reproductive, or immune dysfunction in dolphins, especially those with compromised health. The higher phthalate detection frequency in Sarasota Bay dolphins suggests increased urban exposure risks; however, detection in dolphins from Barataria Bay raises concerns for dolphins in recovering ecosystems. Further research is needed to assess potential synergistic impacts of chemical mixtures, and targeted mitigation strategies in contaminated environments.
1 Introduction
Phthalates are synthetic additives commonly used to increase plastic flexibility (Wormuth et al., 2006; ATSDR, 2019) and added to personal care products as solvents and stabilizers (ATSDR, 1995; Hauser and Calafat, 2005). There are approximately 21 commonly used phthalates that have a basic structure comprising two ester groups attached to consecutive carbons on a benzene ring (Zhang et al., 2015a). The most common phthalates observed in the marine environment are dimethyl phthalate (DMP), diethyl phthalate (DEP), di-n-butyl phthalate (DBP), benzyl butyl phthalate (BBP), and di(2-ethylhexyl) phthalate (DEHP; Gao and Wen, 2016). Phthalates are not chemically bound to the products to which they are added, allowing them to easily separate from the product and leach into the environment (Heudorf et al., 2007). For example, some phthalates (e.g., DEP, DEHP, and DBP) have been reported to leach out of materials composed of polyvinyl chloride (PVC) and polyethylene, as well as car parts, plastic toys, food packaging, paints, glues, and cosmetics (Paluselli et al., 2019; ATSDR, 2022).
Phthalates can enter the marine environment via degradation of plastic pollution (Paluselli et al., 2019), agricultural waste (Zhang et al., 2015a), commercial and industrial runoff and waste (Bergé et al., 2014; Langdon et al., 2019), or wastewater discharge (Clara et al., 2010). For example, Paluselli et al. (2019) observed the release of phthalates from fragments of polyethylene garbage bags, which continue to be a major contributor to marine plastic pollution (Suaria et al., 2020; Thushari and Senevirathna, 2020). In laboratory conditions, phthalates readily leach from plastic material into seawater, reaching peak concentrations within two weeks of introduction; light and bacteria increased aqueous concentrations 5-fold (Paluselli et al., 2019). Agricultural environments are often contaminated with phthalates, particularly during the summer months when plastic mulching films are used to protect crops and improve crop yield (Zhang et al., 2015b; Viljoen et al., 2023). Further, DEHP is a common component of most organic fertilizers (Zorníková et al., 2014), and due to its low solubility in water, this phthalate can accumulate in agricultural soils (Cartwright et al., 2000; Niu et al., 2014). Additionally, environmental contamination from industrial and commercial sources can include leachate or runoff from improper disposal of electronic waste or parts (Liu et al., 2009). This is particularly true for phthalates that are more hydrophilic (e.g., MEP) and easily mobilize from the soil via leachate or runoff into nearby waterways (Langdon et al., 2019). Researchers have also examined environmental pollution relative to manufacturing plants. For example, high concentrations of DEHP have been found in the Houjing River at multiple sampling locations near two different industrial parks in southern Taiwan (20.22 mg/kg and 8.93 mg/kg, respectively; Kaewlaoyoong et al., 2018). Phthalates are also present in influent and effluent wastewater. Across 15 sampled wastewater treatment plants, DEP, DEHP, and BBP were present in 100% of influent wastewater samples, and DEHP and BBP remained present in 100% of treated wastewater samples while DEP remained present in 80% of treated samples (Clara et al., 2010).
Following exposure, mammals rapidly metabolize phthalates to their monoester form. Metabolism occurs through a series of steps, including phase I hydrolysis followed by phase II conjugation (Frederiksen et al., 2007; Choi et al., 2013). Long-branched phthalates, such as DEHP, often require further hydroxylation and oxidation before proceeding to the conjugation phase (Frederiksen et al., 2007). Generally, the more a compound is reduced, the less cytotoxic it becomes, which may be the impetus for metabolic rapidity (Staples et al., 1997). In the case of DEHP, however, metabolism increases toxicity (Gray and Gangolli, 1986; Fan et al., 2010; Gupta et al., 2010). Following metabolism, phthalate metabolites are excreted through urine and feces, where they are able to be detected and quantified (Frederiksen et al., 2007; Choi et al., 2013). Because of the metabolic process, phthalates are not expected to bioaccumulate (Staples et al., 1997; Heudorf et al., 2007); however, ongoing use and release of phthalates into the environment may result in chronic exposure opportunities for wildlife. In humans, urine is the preferred matrix for assessing phthalate exposure due to the reduced potential for contamination from the parent compound (Koch and Calafat, 2009) and the ability to detect metabolites in low concentrations (Blount et al., 2000).
Phthalate exposure has been implicated in many adverse health outcomes, including endocrine disruption (Monneret, 2017; Qian et al., 2020), reproductive impairment (Toft et al., 2012; Ye et al., 2014), and abnormal development (Lyche et al., 2009; Radke et al., 2019). The mechanism of endocrine disruption involves competitive inhibition, in which phthalates bind to endocrine receptors and prevent their target hormones from binding (Lee et al., 2013). The result is a disruption of the normal production, transport, and activity of hormones throughout the body. For example, BBP can bind to estrogen receptors and substantially reduce estrogenic activity (Lee et al., 2013), and DEHP can bind to thyroid signaling hormone receptors and result in hypothyroidism (Zhang et al., 2021). This endocrine disruption can lead to downstream reproductive impacts including infertility (Latini et al., 2003, 2006; Tranfo et al., 2012), poor semen motility (Jurewicz et al., 2013; Axelsson et al., 2015), decreased fecundity (Buck Louis et al., 2014; Seyoum and Pradhan, 2019; Yang et al., 2021), decreased testosterone levels (Kim et al., 2003; Meeker and Ferguson, 2014; Chang et al., 2015), and genital defects in males (Parks et al., 2000; Pant et al., 2014; Radke et al., 2018). For example, among men, exposure to DEHP, DBP, and DEP has been associated with decreased anogenital distance, reduced semen quality, and impacts on sperm motility (Pant et al., 2014; Radke et al., 2018; Yang et al., 2023). Among women, decreased fecundity (Hauser et al., 2016; Jukic et al., 2016), spontaneous abortion (Jukic et al., 2016; Messerlian et al., 2016), and preterm birth (Ferguson et al., 2014, 2019; Shoaff et al., 2016) were associated with exposure to certain phthalates, including DEHP, DBP, and DEP. Impacts on growth and metabolism are also reported, including an increased risk of obesity (Majeed et al., 2017; Stojanoska et al., 2017) and Type II diabetes (Chevalier and Fénichel, 2015; Zhang et al., 2023; Yang et al., 2025). These impacts are likely mediated through the thyroid as it is a target for phthalates, resulting in the abnormal production or secretion of hormones such as triiodothyronine (T3) thyroxine (T4), and insulin-like growth factor 1 (IGF-I;Boas et al., 2010).
Bottlenose dolphins (Tursiops truncatus) inhabiting Sarasota Bay, FL, USA have been the focus of several studies to understand phthalate exposure, given the embayment’s proximity to agricultural, industrial, and residential centers (Hart et al., 2018; Dziobak et al., 2021, 2022a). Nearly 75% of sampled dolphins (n = 51) were exposed to at least one type of phthalate, and exposure was not limited to a particular sex or age class (Dziobak et al., 2021). Among the eight compounds screened (monomethyl phthalate (MMP), monoethyl phthalate (MEP), mono(2-ethylhexyl) phthalate (MEHP), mono(2-ethyl-5-oxohexyl) phthalate (MEOHP), mono(2-ethyl-5-hydroxyhexyl) phthalate (MEHHP), monobenzyl phthalate (MBzP), monobutyl phthalate (MBP), and mono-isobutyl phthalate (MiBP)), the most commonly detected metabolites were MEP and MEHP (Hart et al., 2018; Dziobak et al., 2021), which are metabolites of parent compounds commonly added to personal care products (Hauser and Calafat, 2005) and plastic goods, respectively (Paluselli et al., 2019). When compared to data collected as part of the Centers for Disease Control and Prevention’s (CDC) National Health and Nutrition Examination Survey (NHANES), Sarasota bottlenose dolphin urinary concentrations of MEHP were significantly higher than human samples (Hart et al., 2020). While the exact sources and implications of this exposure are currently unknown for Sarasota Bay dolphins, there is evidence of plastic ingestion (particularly transparent films and white foams; Hart et al., 2022) and thyroid disruption (Dziobak et al., 2022b).
Barataria Bay, LA, USA, is located in the northern Gulf of Mexico, approximately 80 miles south of New Orleans, LA, USA. The bay is bordered by mainland Louisiana to the north and Grand Isle to the south. Compared to the urban environment and coastal development of Sarasota Bay, Barataria is a more remote location with a much smaller human population primarily restricted to the barrier island of Grand Isle. In 2010, an offshore oil rig exploded in the Gulf of Mexico causing the largest domestic marine oil spill in history (Deepwater Horizon; Liu et al., 2011), and bottlenose dolphins in Barataria Bay have since been the focus of long-term monitoring to assess the impacts of oil exposure on health (Schwacke et al., 2017; Smith et al., 2017), movement (Takeshita et al., 2021), and survival (McDonald et al., 2017; Schwacke et al., 2022). This long-term monitoring has included catch-and-release health assessments, providing the opportunity to collect urine samples from free-ranging dolphins.
Phthalate use is extensive, and contamination has been documented in every environmental matrix (e.g., air; Adibi et al., 2008; Lee et al., 2019; sediment; Arfaeinia et al., 2019; Lee et al., 2019; Weizhen et al., 2020; biota; Valton et al., 2014; Fourgous et al., 2016; Baini et al., 2017; Hart et al., 2018; freshwater; (Fatoki et al., 2010; Cheng et al., 2019); seawater; Zhang et al., 2018) across urban and rural locations (Wang et al., 2014); however, the distribution of phthalates has been shown to vary across locations. For example, studies conducted in China have observed increased phthalate pollution along a rural-urban gradient, with the highest concentrations found in the most urbanized areas (Lan et al., 2012; Hongjun et al., 2013). These findings are supported by other studies that detected increased phthalate concentrations in urban and industrialized areas compared to rural (Sun et al., 2013; Wang et al., 2018; Karamianpour et al., 2023). Notably, many studies of phthalates in soils or sediments that documented differences between sites still had detection in the majority of all samples, highlighting the ubiquity of environmental phthalate pollution (Sun et al., 2013; Duong et al., 2014). Similar relationships between phthalate exposure and urbanization have been detected in humans, where urban human populations have higher phthalate metabolite concentrations compared to rural locations (Prasad et al., 2022; Runkel et al., 2022). This is also mirrored in aquatic environments, potentially due to phthalate contamination discharged from industrial effluents and untreated wastewater (Zhang et al., 2015b).
Given widespread phthalate pollution in the environment, contamination is expected for dolphins residing in both urban and rural locations, though the variation between sites is currently unknown. Therefore, the objective of this study is to characterize and compare phthalate exposure among urban Sarasota Bay, Florida dolphins compared to rural Barataria Bay, Louisiana dolphins to better understand the impacts of human activity on environmental pollution and subsequent exposure.
2 Methods
2.1 Site selection
Dolphins sampled for this study were from the Barataria Bay Estuarine System Stock occupying Barataria Basin as well as dolphins from the National Marine Fisheries Service (NMFS) stock that includes dolphins using SSB, and Little SSB (Tyson and Wells, 2016). Although both locations are estuarine systems, the barrier islands of BAR are much less populated than the city of Sarasota (1,005 residents compared to ~55,000, respectively (US Census Bureau, 2024b, a). Further, land bounding the Barataria Basin is primarily privately owned, with 22% of the basin developed (CWPPRA, 2022), while land in Sarasota County is zoned for agricultural, residential, commercial, and industrial use (City of Sarasota, 2024). Therefore, dolphins residing in BAR were identified as “rural” while dolphins residing in SSB were identified as “urban”. However, it should be noted that BAR has a high concentration of petrochemical infrastructure and activity, with most operations based out of Grand Isle and Port Fourchon. Dolphins from both BAR and SSB are known to exhibit year-round site fidelity (Wells, 2009; McDonald et al., 2017), thus contaminant exposure is expected to reflect their local environments.
2.2 Urine collection
Dolphins sampled for this study were individuals considered to be resident to Sarasota Bay, Florida (SB; n~170; Wells, 2009; Lacy et al., 2021) and Barataria Bay, Louisiana (BB; n~2,000; Mullin et al., 2018; Figure 1). Urine was collected opportunistically via catheterization from dolphins sampled during catch-and-release health assessments. For BAR dolphins, urine was collected via urinary catheterization, using an 8.5Fr 60 cm multipurpose drainage catheter (Cook Medical, Bloomington, Indiana) for males and a 10.2Fr 45 cm multipurpose drainage catheter (CookMedical) or a 10Fr 90 cm Foley catheter (Mila International, Erlanger, Kentucky) for females. For SSB dolphins, urine was collected either from free catch using a 50 ml conical vial or via urinary catheterization, using a lubricated (surgilube) 8Fr 22 in (adults) or 5Fr 16 in (juveniles) Kendall Sovereign Feeding Tube and Urethral Catheter (Patterson Veterinary, Loveland, CO). For both locations, samples were frozen with liquid nitrogen for transport in vapor shippers and stored below −20°C until analysis. Health assessments were conducted in BAR during 2011, 2013-2014, 2017-2018, and 2023 as a result of the Deepwater Horizon oil spill, as well as routinely in SSB during 2010-2019, 2022-2024. Some dolphins (n=15) were sampled repeatedly during these health assessments; however, repeated samples were not included in analysis. Health assessment methods have been described elsewhere (Wells et al., 2004, Barratclough et al., 2019). Briefly, dolphins were encircled and temporarily restrained to collect a variety of biological, physiological, and morphological samples/data. Health assessments in BAR were conducted under NMFS Scientific Research Permits Nos. 932-1905, 18786, and 24539. Health assessments in SSB were conducted under NMFS Scientific Research Permits Nos. 522-1785, 15543, 20455, and 26622.
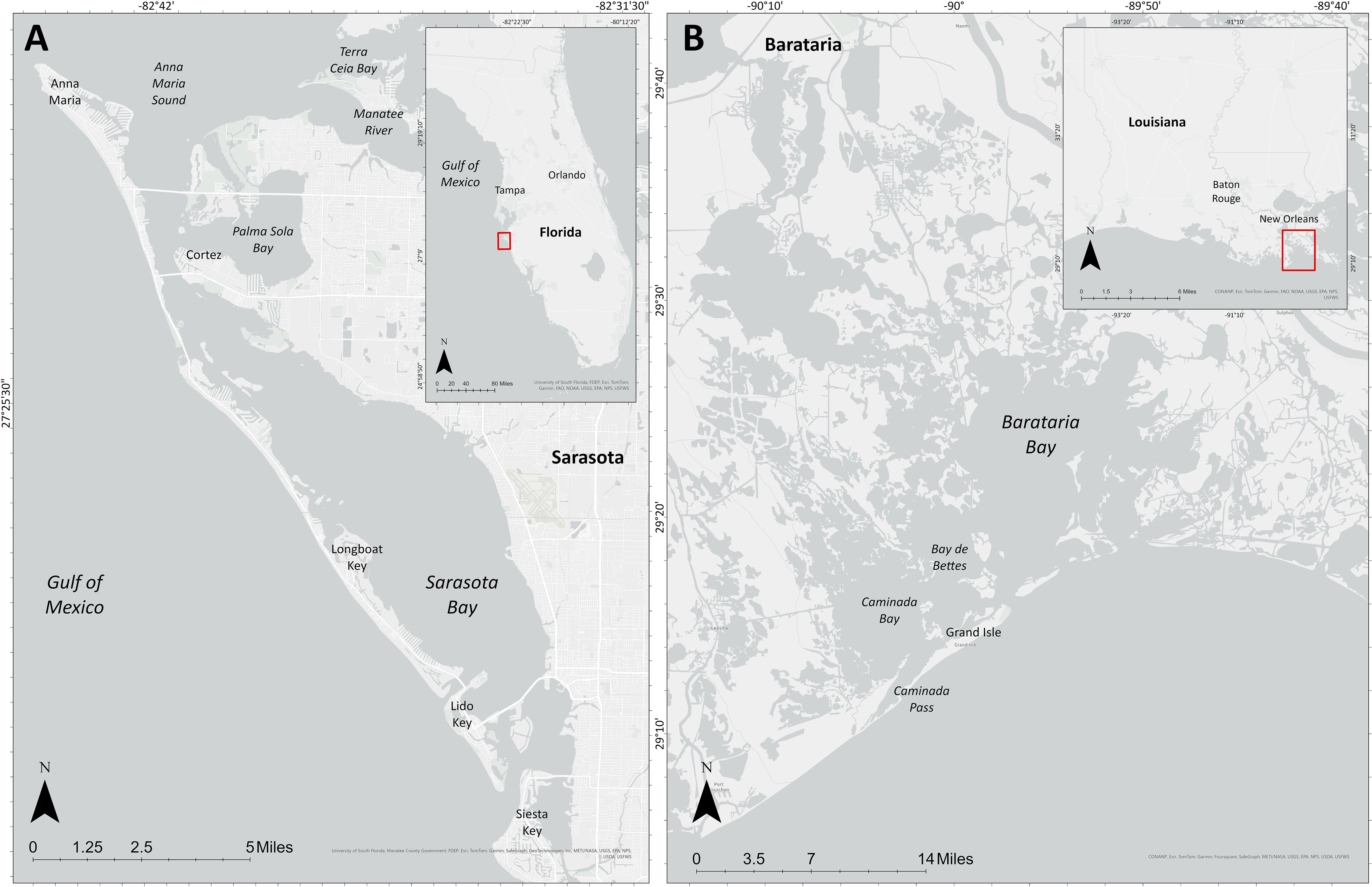
Figure 1. Bottlenose dolphins study sites: (A) Sarasota Bay, Florida and (B) Barataria Bay, Louisiana. Map created using Esri ArcGIS Pro basemap, Esri, TomTom, Garmin, FAO, NOAA, USGS, © OpenStreetMap contributors, and the GIS User Community.
2.3 Sample processing and analysis
Each urine sample was screened for seven phthalate metabolites (MEHP, MEHHP, MEOHP, MBzP, MiBP, and MBP). Male samples containing sperm were centrifuged at 3,000 rpm for 5 minutes prior to solid phase extraction (SPE) to separate the urine from the sperm and prevent clogs in the SPE cartridge. Urine samples (1 mL) were spiked with isotopically labeled internal standards and extracted via SPE (Agilent Bond Elute Nexus) and quantified with high-performance liquid chromatography (HPLC; Agilent 1100; WatersXBridge BEH C18, 2.5 μm, 2.1 x 50 mm analytical column) coupled to a triple quadrupole mass spectrometer (MS; Applied Biosystems Sciex API 4000) with an electrospray ionization (ESI negative) interface. Sample integrations were performed using Analyst software (Sciex, ver 1.5). Prior to the acquisition of sample data, the instrument was calibrated (standard reference material (SRM) 3060: monoester phthalates in acetonitrile); coefficients of determination (r2) for all metabolites were ≥ 0.995.
As reported by Hart et al. (2018), quality assurance/quality control (QA/QC) samples (reagent blanks, reagent spikes, matrix spikes, SRM 3672 Organic Contaminants in Smokers’ Urine, and field blanks) were processed alongside the urine samples. Reagent blank values were subtracted from the determined concentration value to account for any metabolite contamination resulting from laboratory processes. Available field blank metabolite concentrations were not found to be statistically different from each other by year or by metabolite. Field blank concentrations were averaged for each metabolite and subtracted from urine samples for any contamination due to sample collection materials (e.g., catheters). Acceptable QA/QC criteria for spike (reagent and matrix) and SRM recoveries were 70%-130%. The limit of detection (LOD) was determined for each metabolite and is based on the lowest point on the calibration curve that could be detected on the instrument divided by the volume of the sample extracted (Dziobak et al., 2025).
2.4 Statistical analysis
Descriptive statistics were used to summarize phthalate metabolite concentrations and calculate the proportion of dolphins with concentrations above the LOD (“detectable concentrations”; Dziobak et al., 2025. For dolphins sampled more than once, only the sample most recently obtained was used for analysis. All statistical analyses were conducted using R (Version 4.3.2, R Foundation for Statistical Computing, Vienna, Austria) and R Studio (Posit Software, BBC) software packages. For metabolites with greater than 20% of concentrations above LOD, means and standard deviations were calculated using robust regression on order statistics (Helsel, 2006) using the NADA (Lee and Helsel, 2022) and NADA2 (Julian and Helsel, 2023) packages. Previous work demonstrated no demographic differences in phthalate metabolite detection, but used a small sample size (Dziobak et al., 2021). Therefore, summary statistics were calculated overall and by demography (female, male, immature, and mature) within each site to retest relationships with a larger sample size. Immature dolphins were differentiated from mature dolphins on the basis of sexual maturity, which was determined based on several measures, including age, calving history, pregnancy diagnosis via ultrasonography, testis size from ultrasound, and sex hormone concentrations (Wells et al., 1987, 2025; Wells, 2014). Dolphin ages were determined by either life history from photo-identification (id) surveys, analysis of dentinal growth layer groups (Hohn et al., 1989), or analysis of pectoral flipper radiography (Barratclough et al., 2019). The proportion of detectable concentrations was compared between demographic groups and between sites using a Peto-Peto test (NADA and NADA2 R packages; (Helsel, 2006; Dziobak et al., 2021). Among dolphins with detectable concentrations, distribution of concentrations was evaluated using a Shapiro-Wilk test, and means were compared between sites using either an independent t-test or a Mann-Whitney U test, depending on Gaussian distribution. Statistical significance in observed differences was determined using α = 0.05.
2.5 Spatial analysis
Sighting data from BAR were not abundant (compared to SSB, for example) as routine photo-id surveys are not conducted. Instead, location data resulted from a variety of efforts, including photo-id surveys, biopsy locations, radio tracking, stranding locations, health assessment locations, satellite-linked telemetry, and fecundity studies. Because some BAR dolphins had very few observations (some fewer than 10), all available records were used to estimate spatial usage. Sighting data for dolphins residing in SSB was obtained from standardized photo-id surveys (Wells, 2009; Tyson and Wells, 2016) conducted by the Sarasota Dolphin Research Program (SDRP) and included more than 20,000 individual sighting records. In humans, phthalate metabolism is rapid; daily fluctuations have been observed in urine samples (Hauser et al., 2004; Teitelbaum et al., 2008). However, single spot samples can be used as a predictive metric to obtain average metabolite concentrations representative of the previous 3-6 (Hauser et al., 2004; Teitelbaum et al., 2008). Therefore, SSB sighting data were restricted to one calendar year prior to urine sampling to generate the most conservative spatial use estimate for the dolphins.
Spatial analyses were performed to compare spatial usage between dolphins with detectable urinary metabolite concentrations (hereafter referred to as “detects”) and dolphins with urinary metabolite concentrations below LOD (hereafter referred to as “nondetects”) to identify potential areas of phthalate contamination in Barataria and Sarasota Bays. Spatial analyses were based on methods described in Takeshita et al. (2021). Briefly, kernel density estimates (KDEs) were calculated using the adehabitatHR package in R (Calenge, 2006), to determine 50% and 95% percent volume contours (PVCs) which represent the core (50% PVC), and total (95% PVC) area usage for detects and nondetects. The bandwidth parameter was determined using a rule-based ad-hoc approach (Kie, 2013).
3 Results
3.1 Barataria Bay
Urine for phthalate metabolite analysis in BAR was collected from 45 individual dolphins sampled during catch-and-release health assessments conducted in Barataria Bay, LA, USA in 2011, 2013, 2014, 2017, 2018, and 2023 (Table 1; Figure 2). Detectable concentrations of at least one metabolite were observed in 33.33% (n = 15; 95% CI: 20.00 - 48.95%) of samples. Metabolite detection was limited to three compounds including MEHP, MBzP, and MEHHP; none of the dolphins sampled had detectable concentrations of MBP, MEOHP, MEP, or MiBP (Table 1). The most commonly detected metabolite was MEHP (n = 14) with concentrations ranging from 1.40 µg/L to 16.46 µg/L (Tables 1, 2). Given low detection frequencies for the majority of the metabolites, MEHP was the only metabolite that was statistically evaluated for differences in detection relative to demographic characteristics.

Table 1. Frequency of detection of all metabolites in urine samples collected from bottlenose dolphins (Tursiops truncatus) in Barataria Bay, Louisiana (n=45; 2011, 2013, 2014, 2017, 2018, 2023).
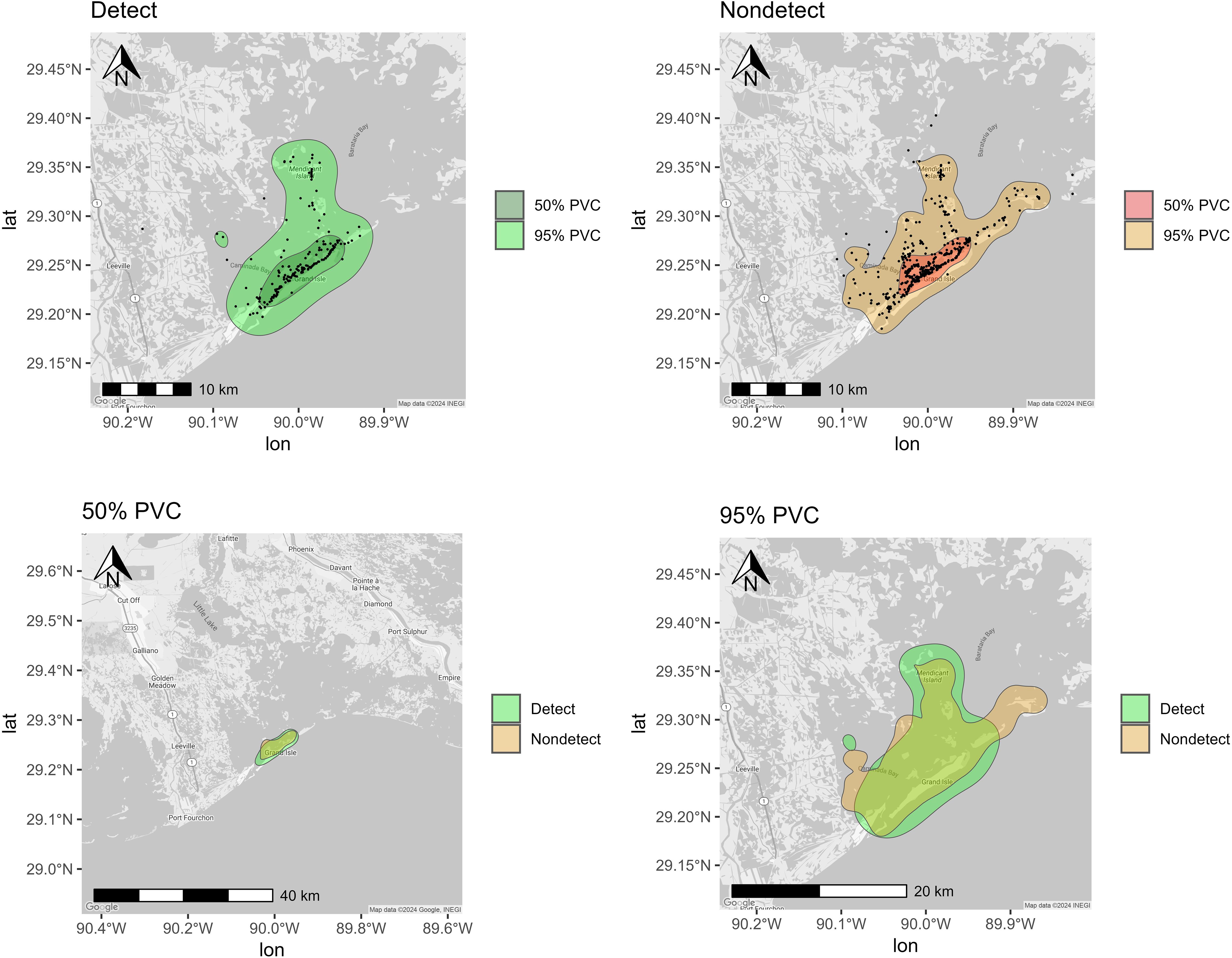
Figure 2. Percent volume contours (PVCs) determined for bottlenose dolphins sampled (n = 45) from Barataria Bay (2011, 2013-2014, 2017-2018, 2023) using all sighting histories. (Detect and Nondetect maps) Core (50% PVC) and total (95% PVC) spatial usage respectively for dolphins with detectable and undetectable urinary mono(2-ethylhexyl) phthalate MEHP concentrations with black points representing individual sighting records. (50% PVC and 95% PVC maps) Core (50% PVC) and Total (95% PVC) spatial usage respectively for dolphins with detectable urinary MEHP concentrations overlayed with spatial usage for dolphins with nondetectable urinary MEHP concentrations.
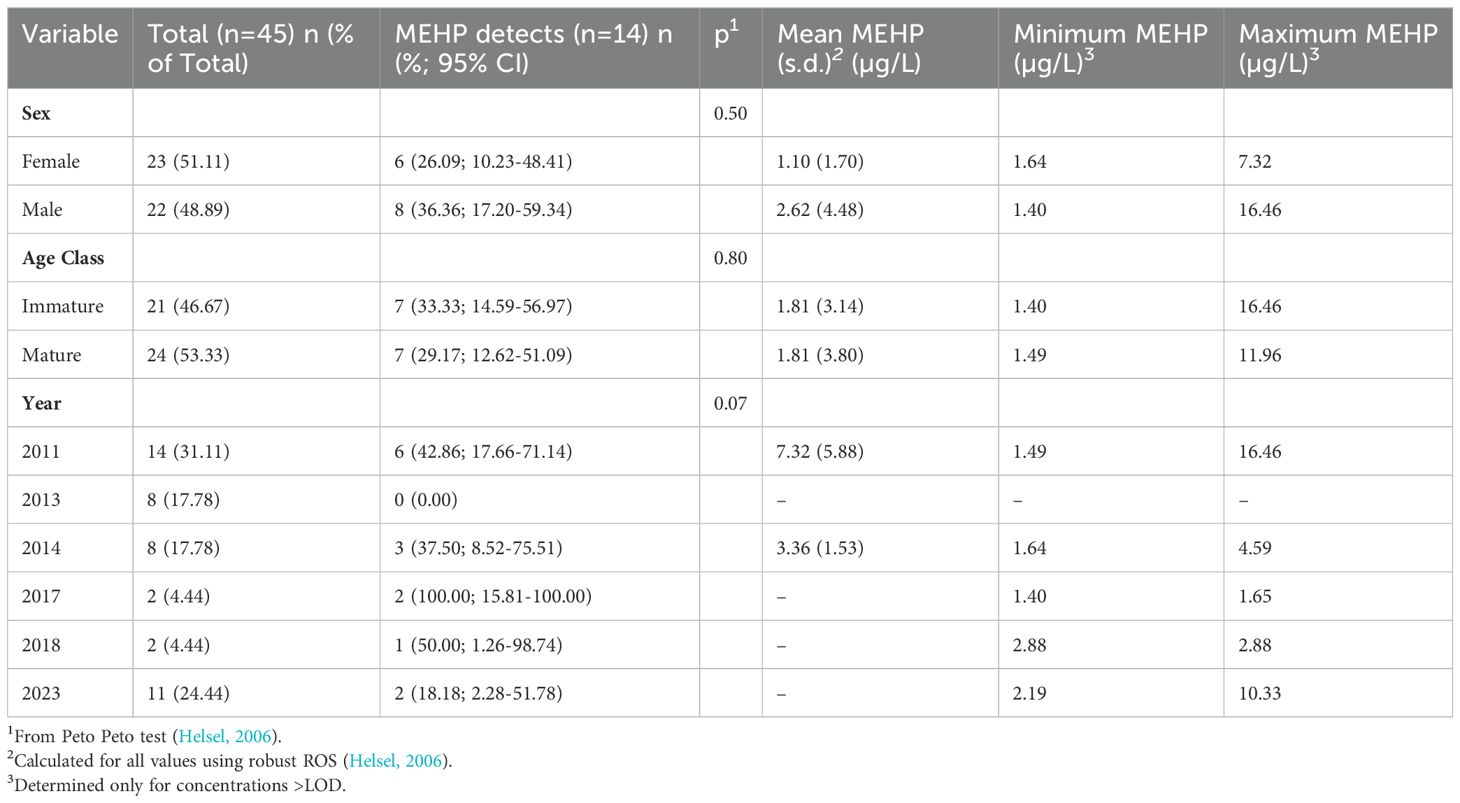
Table 2. Frequency of detection, ROS mean and standard deviation, minimum, and maximum values of mono-2-ethylhexyl phthalate (MEHP) above the limit of detection (LOD; “detects”) by demography in bottlenose dolphins sampled in Barataria Bay during 2011, 2013, 2014, 2017, 2018, 2023.
There was a nearly even distribution of sexes (23 female, 22 male) and age classes (21 immature, 24 mature) sampled (Table 2). The majority of samples were collected in 2011 (n =14), and subsequent years exhibited variability in the number of samples screened (ranging from 2 to 8 samples; Table 2). Regardless, phthalate metabolites were detected in at least one individual from each sampling year except 2013 (Table 2). There were no significant differences in the percentage of females (26.09%; 95% CI: 10.23-48.41%) and males (36.36%; 95% CI: 17.20-59.34%) with detectable concentrations of MEHP (p=0.50; Table 2). Mean concentrations of MEHP for females and males were 1.10 µg/L (s.d. = 1.70 µg/L) and 2.62 µg/L (s.d. = 4.48 µg/L; Table 2), respectively. Similarly, there were no significant differences in the percentage of sampled dolphins with detectable MEHP between mature and immature dolphins (33.33% vs. 29.17%; p=0.80; Table 2). Both age classes had the same mean concentration (immature dolphins: 1.81 µg/L, s.d. = 3.14 µg/L; mature dolphins: 1.81 µg/L, s.d. = 3.80 µg/L; Table 2).
MEHP was the most frequently detected metabolite in BAR dolphin urine samples, so variations in bay usage were compared between dolphins with MEHP concentrations above LOD (“detects”) and equal to or below LOD (“nondetects”). Overall little variation in habitat usage was observed in BAR between detects and nondetects (Figure 2). Both had core (50%) ranging patterns around the barrier islands (e.g., Grand Isle), and total (95%) ranging patterns that extended into BAR (e.g., Caminada Bay; Figure 2). Nondetects appeared to have a range that extended further east past Grand Isle towards Grand Terre than detects, who were not sighted past Barataria Pass (Figure 2).
3.2 Sarasota Bay
Urine for phthalate metabolite analysis in SSB was sampled from 71 individual dolphins during catch-and-release health assessments conducted during 2010-2019, and 2022-2024 (Figure 3), of which 73.24% (n= 52; 95% CI: 61.41-83.06%); had concentrations of at least one metabolite above LOD. Among dolphins with detectable concentrations, one to five metabolites were detected. Six of the seven metabolites screened for were detected, including MBP, MBzP, MEHHP, MEHP, MEOHP, MEP (Table 3). Similarly to previous studies of phthalate exposure in SSB dolphins, the most common metabolites detected were MEHP (n=41, 57.75%; 95%CI: 45.44-69.39%) and MEP (n=13, 18.31%; 95% CI: 10.13-29.27%), and none of the dolphins had detectable concentrations of MiBP (Table 3). Similar to samples from BAR, MEHP was the only metabolite analyzed for demographic differences as the other metabolites had too low detection frequencies.
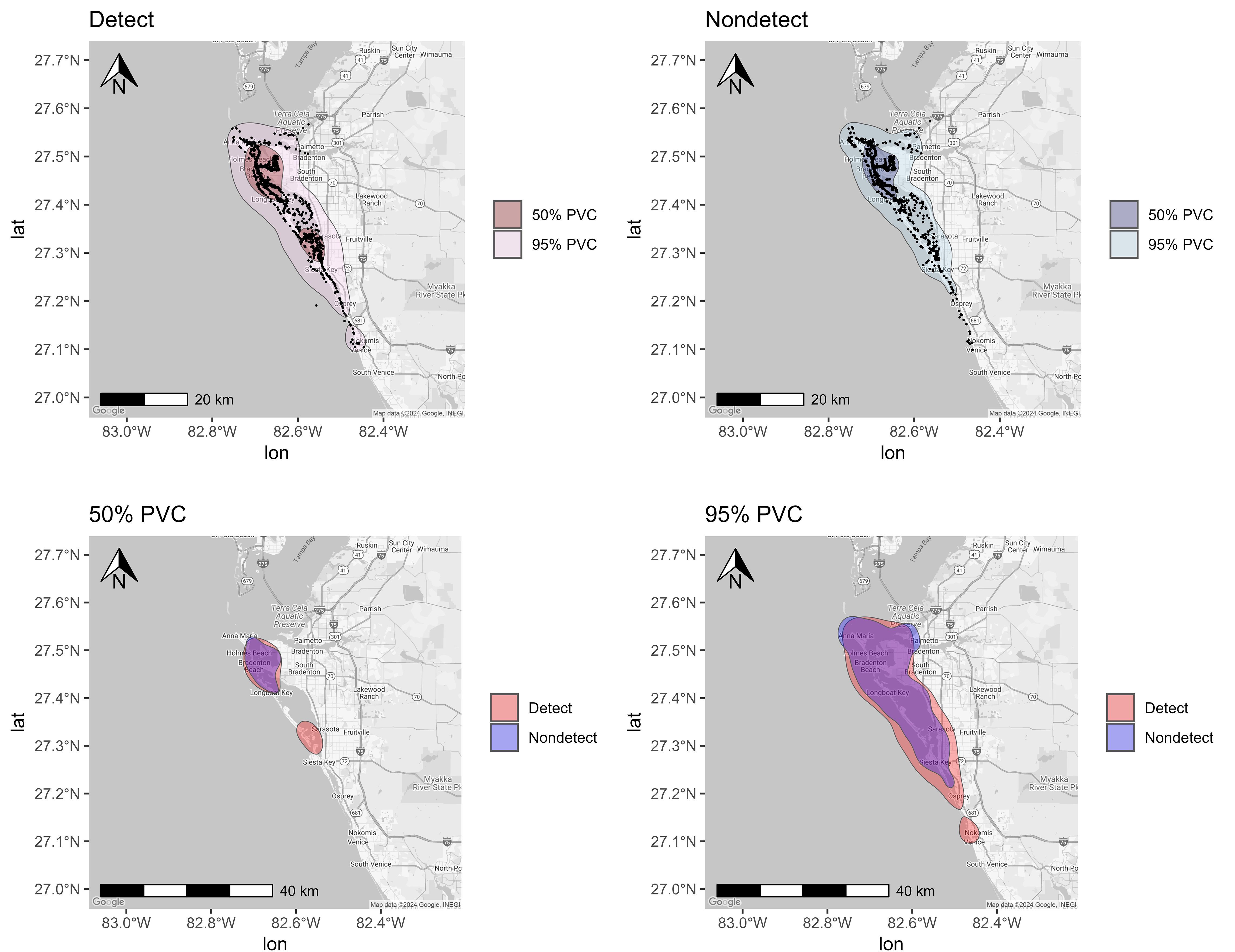
Figure 3. Percent volume contours (PVCs) determined for bottlenose dolphins sampled (n = 71) from Sarasota Bay (2010-2019, 2022-2024) using photo-identification sighting histories. (Detect and Nondetect maps) Core (50% PVC) and total (95% PVC) spatial usage respectively for dolphins with detectable and undetectable urinary mono(2-ethylhexyl) phthalate MEHP concentrations with black points representing individual sighting records. (50% PVC and 95% PVC maps) Core (50% PVC) and Total (95% PVC) spatial usage respectively for dolphins with detectable urinary MEHP concentrations overlayed with spatial usage for dolphins with nondetectable urinary MEHP concentrations.

Table 3. Frequency of detection of all metabolites in urine samples collected from bottlenose dolphins (Tursiops truncatus) in Sarasota Bay, Florida (n=71; 2010-2019; 2022-2024).
The demographic distribution of dolphins was similar by sex (38 female, 33 male); however, there were almost twice as many mature dolphins (n = 44) than immature dolphins (n = 27). Across years, there were variations in the number of dolphins sampled, ranging from 1 (2013) to 15 (2024). There were no significant differences in the proportion of dolphins with detectable MEHP concentrations by sex (63.16% of females; 95% CI: 45.99 - 78.19%; 51.52% of males; 95% CI: 33.54-69.20%; Table 4) or by age class (62.96% of immature dolphins; 95% CI: 42.37-80.60%; 54.55% of mature dolphins; 38.85-69.61%; Table 4). The mean MEHP concentration for females was 9.77 µg/L (s.d. = 16.20 µg/L; Table 4), which was higher than males (3.74 µg/L; s.d., = 9.90 µg/L; Table 4). The mean MEHP concentration was also higher in mature dolphins (9.36 µg/L; s.d. = 16.60 µg/L; Table 4) compared to immature dolphins (3.11 µg/L; s.d. 6.20 µg/L; Table 4).
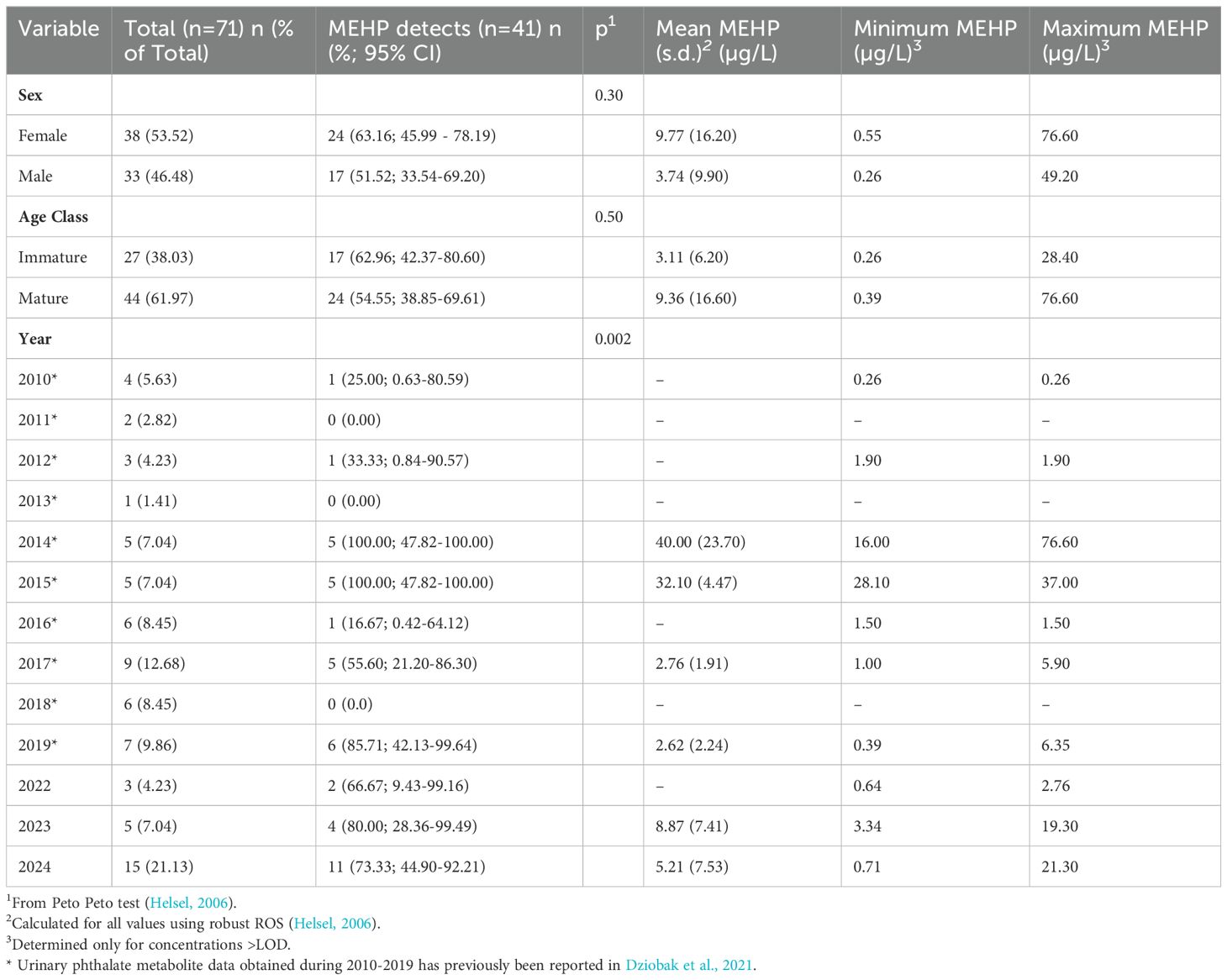
Table 4. Frequency of detection, ROS mean and standard deviation, minimum, and maximum values of mono-2-ethylhexyl phthalate (MEHP) above the limit of detection (LOD; “detects”) by demography in bottlenose dolphins sampled in Sarasota Bay during 2010-2019 and 2022-2024.
Since MEHP was also the most frequently detected metabolite in SSB, variations in bay usage were evaluated between detects and nondetects. Detects appeared to be more likely to use more of SSB than nondetects, as illustrated by a larger total area (95% PVC) used (Figure 3). Areas associated with detects included Anna Maria Sound, Palma Sola Bay, and part of SSB, as well as between New Pass and Venice Inlet (Figure 3). Although nondetect ranges were similar to detects in the northern part of the bay, the southern extension seemed to be limited to Siesta Key and did not go as far as Venice Inlet (Figure 3). Further, detects had core (50%) ranging patterns that were observed in the southern part of the bay near Venice, while nondetects were concentrated around Palma Sola Bay (Figure 3). Overall, the dolphin ranging patterns seemed to be consistent with ranging patterns previously reported (Dziobak et al., 2022a), where detects were more likely to be found in the southern portion of the bay compared to nondetects. Both groups, however, were likely to use the northern portion of the bay near Palma Sola (Figure 3), which was consistent with findings from a previous study (Dziobak et al., 2022a).
3.3 Barataria Bay vs. Sarasota Bay
To explore the potential influence of urbanization on phthalate exposure, urinary MEHP concentrations were compared between BAR and SSB dolphins. MEHP was detected more frequently amongst SSB dolphins (n=41; 57.75%; 95% CI: 45.44-69.39%) than BAR dolphins (n = 14; 31.11%; 95% CI: 18.17-46.65%; p=0.005; Table 5). However, detectable concentrations of urinary MEHP did not differ significantly by site (BAR MEHP mean (s.d.) = 5.18 (4.66) µg/L; SSB MEHP mean (s.d.) = 11.95 (16.67) µg/L; p=0.97; Table 5, Figure 4), indicating similarities in the magnitude of DEHP exposure.
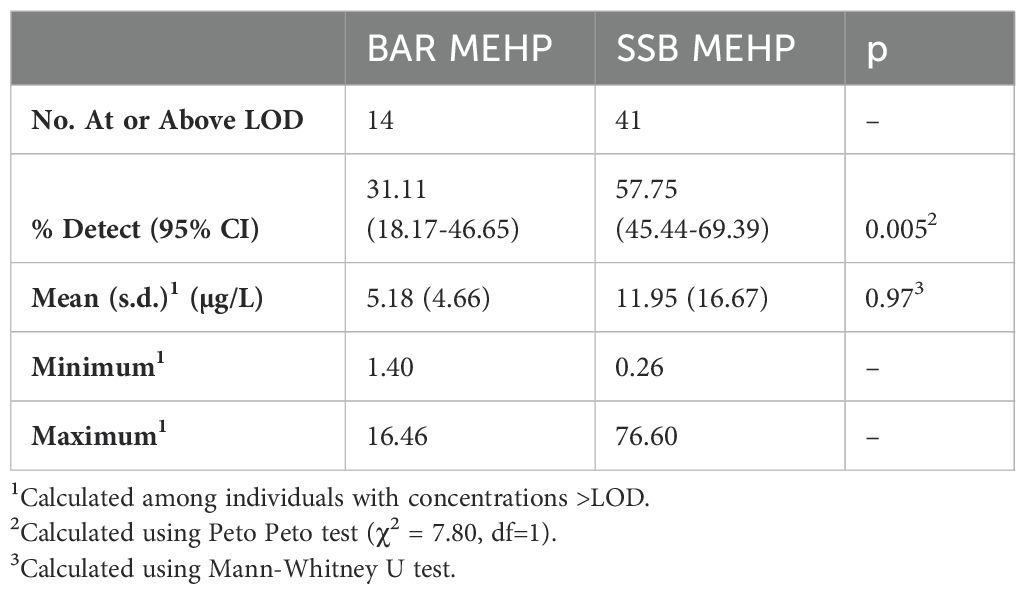
Table 5. Comparison of detectable urinary mono(2-ethylhexyl) phthalate (MEHP) concentrations between dolphins sampled in Barataria Bay (2011, 2013-2014, 2017-2018, 2023) and Sarasota Bay (2010-2019, 2022-2024).
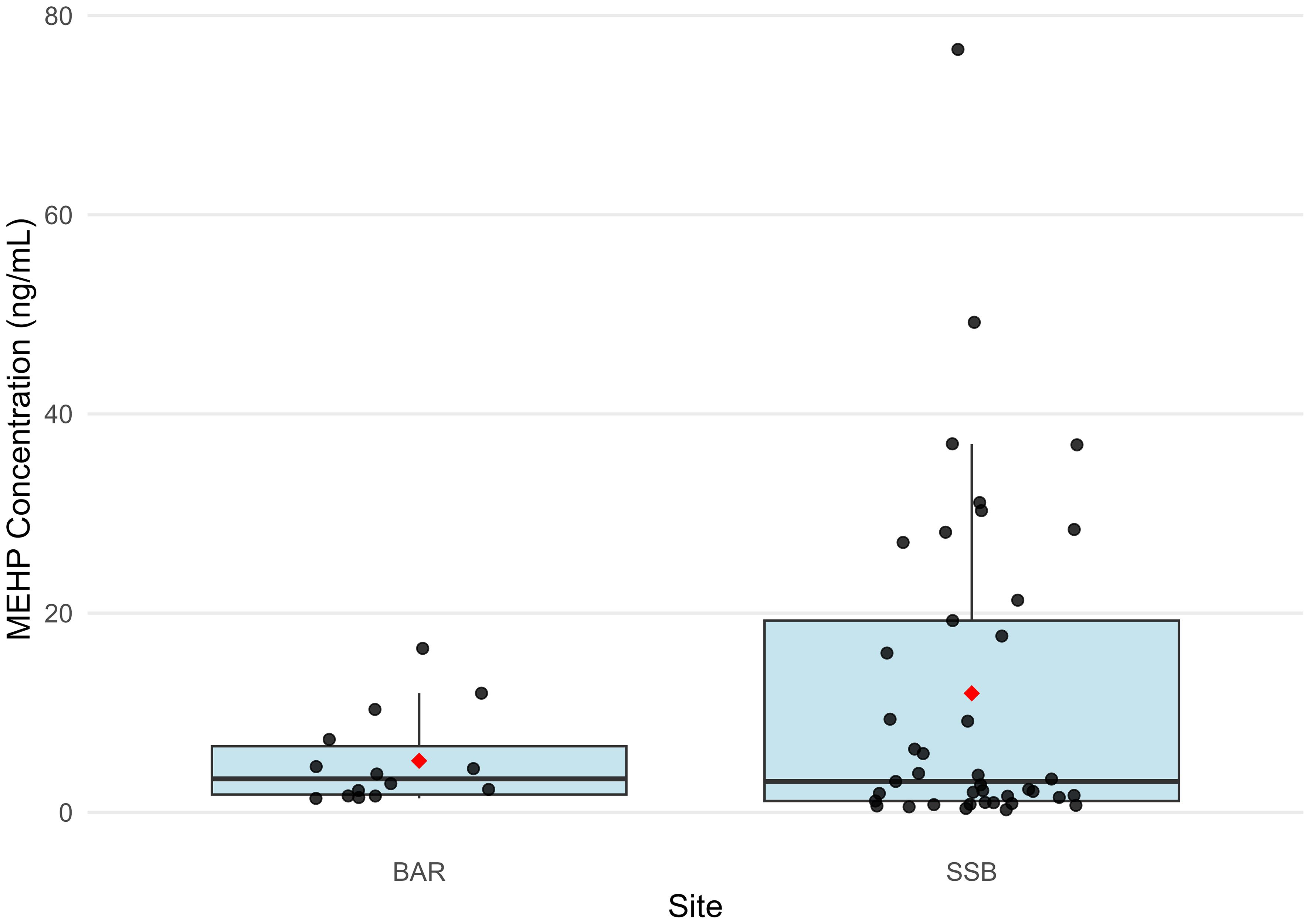
Figure 4. Comparison of urinary MEHP concentrations (>LOD) between bottlenose dolphins sampled in Barataria Bay, LA (BAR) and Sarasota Bay, FL (SSB). Box plot displays median and quartiles, black points represent concentrations above the limit of detection, and red diamond represents mean among concentrations above the limit of detection.
4 Discussion
4.1 Phthalate exposure
Of the 45 individual BAR dolphins screened, one-third had detectable concentrations of at least one phthalate metabolite in their urine. Our findings demonstrate a lower level of phthalate exposure in BAR dolphins compared to a previous study in bottlenose dolphins living near an urban environment with extensive coastal development (Dziobak et al., 2022a). Although there is fishery and petrochemical activity throughout the Barataria estuarine system, the smaller coastal community of residents, workers, and tourists is mostly concentrated around Grand Isle. Most of the dolphins in this study were assessed near Grand Isle and likely spend much of their time near the barrier islands, however some of the animals likely show high site fidelity to the northern, less developed areas of Barataria Basin (Figure 2; Takeshita et al., 2021).
Among bottlenose dolphins sampled in SSB, phthalate metabolites were detected in 73% of urine samples (95% CI: 61.20-82.73). SSB dolphins exhibited exposure to a diverse array of phthalates, with detectable concentrations determined for six of the seven metabolites screened. These metabolites come from parent compounds commonly added to plastic products (e.g., DEHP, DBzP) and personal care products (e.g., DEP). Consistent with previous investigations of SSB dolphins, MEHP was the most frequently detected phthalate metabolite. The majority of land around the SSB watershed is considered to be built up (USF, 2020). Urbanization has been linked with increased environmental phthalate pollution (Teil et al., 2014), so the development and human activity in the SSB area could contribute to marine contamination.
4.2 Comparison of phthalate exposure between Sarasota Bay and Barataria Bay dolphins
Although differing in the frequencies of detection, dolphins from both SSB and BAR exhibited exposure to phthalates, as evidenced by the detection of MEHP, MEHHP, and MBzP. The parent compounds (i.e., DEHP and BzBP) are most commonly added to plastic, which could be the exposure source. When studied in seawater conditions, plastics degraded by at least 85%, leaching large concentrations of these compounds into the aquatic environment (Paluselli et al., 2019). For example, MEHP was detected the most frequently in BAR dolphins in 2011, which coincided with cleanup efforts from the Deepwater Horizon oil spill. Materials deployed during this time period included polypropylene-filled sorbent booms (Kokaly et al., 2013; Blum et al., 2014) and containment booms, which can be composed of polypropylene, polyurethane, nylon, and PE (Schrader, 1991; Dadhich and Lalu, 2018; Pagnucco and Phillips, 2018). Phthalates such as DEHP, DMP, DEP, DiBP, and DnBP are known to leach from these synthetic materials (Paluselli et al., 2019; Zhong et al., 2023) and could have contributed to the increased exposure observed in BAR dolphins.
Beyond the materials used in BAR, plastic contamination in the ocean is extensive, with global abundance estimated at 82–358 trillion plastic particles weighing as much as 4.9 million tonnes (Eriksen et al., 2023). Regardless of worldwide ubiquity, contamination skews higher in urban regions with extensive coastal development (Kwon et al., 2020; Wardlaw et al., 2022). For example, Wardlaw et al. (2022) reported a higher concentration of microplastic fragments and fibers in urban waters compared to rural waters of the Thames River in Ontario, Canada (31–1882 fragments/kg sediment, 15–562 fibers/kg sediment in urban areas, 7–46 fragments/kg sediment, 46–216 fibers/kg sediment in rural areas). Similarly, urban waters of coastal South Korea exhibited higher concentrations of microplastic pollution (2.85 particles/m3) than rural waters (1.86 particles/m3; Kwon et al., 2020). MEHP was detected in BAR dolphins at similar concentrations to dolphins in SSB (Table 5), but the proportion of detects was lower (Table 5, Figure 4), possibly due to differences in land use and development between the two locations. As BAR is relatively remote and has little commercial or residential development use, there are likely fewer sources of plastic pollution compared to SSB. Further, BAR is much larger, covering over 1,600 km2 compared to 114.00 km2 covered by SSB (GulfBase, 2024a, b). Therefore, it is possible that any phthalate inputs to BAR are diluted throughout the bay prior to dolphin exposure.
Phthalate contamination may be more diverse in urban areas compared to areas with less coastal development. MEHP, MBzP, and MEHHP were detected in dolphins from both locations, while MEP and MBP were exclusively detected in SSB dolphins. In particular, MEP was the second most common phthalate metabolite detected in SSB dolphin urine samples (% detect). The parent compound, DEP, is commonly used as a fragrance enhancer for a variety of consumer products, including personal care and cleaning products, laundry detergents, and pet cleansers (Hubinger, 2010; Guo and Kannan, 2013; Guo et al., 2014). As a result, DEP is often found in industrial and domestic effluents (Bergé et al., 2014; Sengupta et al., 2014). Several studies have demonstrated a correlation between levels of DEP in wastewater and proximity to urban development (González-Mariño et al., 2017; Du et al., 2018; Li et al., 2020). Li et al. (2020) observed a strong relationship between DEP and industrial development, where lakes adjacent to industrial activity demonstrated higher median concentrations (14.728 µg/L) than lakes in areas with little industrial development or land use (8.684 µg/L). Similarly, González-Mariño et al. (2017) observed that DEP was the most abundant phthalate in residential wastewater, with a significantly higher average daily exposure load compared to all other measured phthalates. This was further supported by Du et al. (2018), who observed a positive correlation between population density and levels of DEP in samples collected from wastewater treatment plants. Remote areas often lack the commercial and industrial infrastructure that introduces phthalates into the environment. Given the remote location of BAR and the surrounding small population, the lack of MEP detection among dolphins in this rural area may further suggest a relationship between environmental DEP contamination and human activity.
4.3 Significance
The number of dolphins with evidence of overall phthalate and MEHP exposure in BAR was not as great as it was in SSB; however, the magnitude of exposure in phthalate-positive dolphins was consistent across sites (Table 5, Figure 4). Hart et al. (2020) found significantly higher urinary MEHP concentrations in SSB dolphins compared to human reference populations, so these findings suggest that BAR dolphin exposure may also be higher than values reported by the Centers for Disease Control and Prevention. MEHP has been associated with reproductive, metabolic, and developmental health effects in humans (Boas et al., 2010; Toft et al., 2012; Tranfo et al., 2012; Pant et al., 2014; Radke et al., 2019), marine mammals (Routti et al., 2021; Dziobak et al., 2022b), fish (Mathieu-Denoncourt et al., 2015), and laboratory rodent studies (Weaver et al., 2020; Wang et al., 2021; Adam and Mhaouty-Kodja, 2022). Given that the health of BAR dolphins is already compromised due to oil and associated chemical exposure, exposure to MEHP could exacerbate pre-existing conditions such as impaired stress response, suppressed endocrine activity, inflammation, and hypoglycemia (Schwacke et al., 2014; Smith et al., 2017) and further impact reproductive success (Kellar et al., 2017) and population recovery (Schwacke et al., 2024).
The types of phthalate metabolites observed in biological and environmental samples may reveal sources of contamination or environmental stressors. For example, Li et al. (2020) screened for phthalates in lakewater collected from 16 Chinese provinces with varying degrees of industrial and agricultural activity. While there was considerable overlap in the mixture of metabolites detected in water regardless of land use, DIBP concentrations were significantly higher in lakes adjacent to industrial sites, suggesting that detection of this particular phthalate could be indicative of certain human activities (Li et al., 2020). Findings from our study suggest that MEP could similarly characterize coastal or marine ecosystems that differ in the level or types of anthropogenic influence. MEHP has been observed in marine studies globally, including the United States (Brock et al., 2016; Hart et al., 2018; Dziobak et al., 2021), France (Valton et al., 2014; Fourgous et al., 2016), China (Hu et al., 2016), Italy (Fossi et al., 2012), and the Mediterranean Sea (Baini et al., 2017). Given that DEHP is predominately added to plastic products and that our oceans are estimated to contain more than 170 trillion plastic particles (Eriksen et al., 2023), we hypothesize that widespread detection of MEHP reflects the global ubiquity of plastic pollution.
5 Conclusion
Exposure to phthalates was compared between dolphins residing in different geographic regions. Although the magnitude of exposures were similar, dolphins in urban SSB had a higher frequency of phthalate metabolite detection compared to rural BAR (p = 0.005). Specific exposure sources are still widely unknown, but the metabolites detected could provide clues as to origin. For example, MEHP was found in both locations, suggesting evidence of widespread plastic pollution, while MEP detected only in Sarasota Bay dolphins could indicate relationships with human activity and urbanization. Widespread phthalate contamination and subsequent exposure is concerning due to endocrine disrupting properties, as well as the potential for cardiovascular and neurological impacts. Further research is needed to better understand phthalate exposure sources and associations with markers of dolphin health.
Data availability statement
The datasets generated for this study can be found in the Dryad repository: https://doi.org/10.5061/dryad.pc866t20f.
Ethics statement
Health assessments in BAR were conducted under NMFS Scientific Research Permits Nos. 932-1905, 18786, and 24539. Health assessments in SSB were conducted under NMFS Scientific Research Permits Nos. 522-1785, 15543, 20455, and 26622, Mote Marine Laboratory’s Institutional Animal Care and Use Committee (IACUC). The study was conducted in accordance with the local legislation and institutional requirements.
Author contributions
MD: Formal Analysis, Investigation, Validation, Writing – original draft. TC: Formal Analysis, Visualization, Writing – original draft. RW: Investigation, Writing – review & editing. RT: Data curation, Investigation, Resources, Supervision, Writing – review & editing. CS: Investigation, Supervision, Writing – review & editing. EZ: Investigation, Resources, Writing – review & editing. CT: Investigation, Resources, Writing – review & editing. RA: Investigation, Resources, Writing – review & editing. LH: Conceptualization, Data curation, Formal Analysis, Funding acquisition, Investigation, Project administration, Resources, Software, Supervision, Visualization, Writing – original draft.
Funding
The author(s) declare that financial support was received for the research and/or publication of this article. Research reported in this publication was supported by the National Institute Of Environmental Health Sciences of the National Institutes of Health under Award Number R15ES034169. The health assessments leveraged partnerships and collaborations with multiple institutions, including Auburn University, Audubon Nature Institute, Duke University, Florida Institute of Technology, Force Blue, Louisiana Department of Wildlife and Fisheries, Marine Mammal Commission, National Centers for Coastal Ocean Science, National Institute of Standards and Technology, NMFS Health and Stranding Response Program, Omacha, Sarasota Dolphin Research Program, Savannah State, Sea World Australia, SeaWorld and Busch Gardens, University of Connecticut, University of St. Andrews, Valencia Oceanographic, and Woods Hole Oceanographic Institution. Dolphin samples from BAR were obtained through health assessments supported as part of the Deepwater Horizon Natural Resource Damage Assessment and by grants from the Gulf of Mexico Research Initiative and the Strategic Environmental Research and Development Program. Dolphin samples from SRQ were obtained through health assessments supported primarily by Dolphin Quest, Inc. The funders were not involved in the study design, collection, analysis, interpretation of data, the writing of this article or the decision to submit it for publication.
Acknowledgments
We would like to thank Emily Pisarski and Dr. Ed Wirth from the National Oceanic and Atmospheric Administration (NOAA) and Maggie Knight for their assistance with the chemical analyses. Special thanks to Jason Allen, Aaron Barleycorn, Brian Balmer, Larry Hansen, Suzanne Lane, Jeanine Morey, Brian Quigley, Andrew Cheever, Forrest Gomez, Ashley Barratclough, Forrest Townsend, and Amanda Moors for their essential assistance with sample collection and processing, as well as animal handling and examination. Thanks also to Ross Martinson, Andy Garrett, and Larry Fulford for their efforts to ensure the safe capture of the dolphins. We are grateful to the staff and collaborators of the National Marine Mammal Foundation and The Sarasota Dolphin Research Program for ensuring the safe capture, sampling, and release of the dolphins. The specimens used in this study were collected by the contributor and provided by the National Marine Mammal Tissue Bank, which is maintained at the NIST Biorepository at the Hollings Marine Laboratory, Charleston, SC, and which is operated under the direction of NMFS with the collaboration of USGS, USFWS, MMS, and NIST through the Marine Mammal Health and Stranding Response Program.
Conflict of interest
The authors declare that the research was conducted in the absence of any commercial or financial relationships that could be construed as a potential conflict of interest.
Generative AI statement
The author(s) declare that no Generative AI was used in the creation of this manuscript.
Publisher’s note
All claims expressed in this article are solely those of the authors and do not necessarily represent those of their affiliated organizations, or those of the publisher, the editors and the reviewers. Any product that may be evaluated in this article, or claim that may be made by its manufacturer, is not guaranteed or endorsed by the publisher.
Author disclaimer
The content is solely the responsibility of the authors and does not necessarily represent the official views of the National Institutes of Health.
References
Adam N., Mhaouty-Kodja S. (2022). Behavioral effects of exposure to phthalates in female rodents: evidence for endocrine disruption? Int. J. Mol. Sci. 23, 2559. doi: 10.3390/IJMS23052559/S1
Adibi J. J., Whyatt R. M., Williams P. L., Calafat A. M., Camann D., Herrick R., et al. (2008). Characterization of phthalate exposure among pregnant women assessed by repeat air and urine samples. Environ. Health Perspect. 116, 467–473. doi: 10.1289/ehp.10749
Arfaeinia H., Fazlzadeh M., Taghizadeh F., Saeedi R., Spitz J., Dobaradaran S. (2019). Phthalate acid esters (PAEs) accumulation in coastal sediments from regions with different land use configuration along the Persian Gulf. Ecotoxicol. Environ. Saf. 169, 496–506. doi: 10.1016/j.ecoenv.2018.11.033
ATSDR (1995). Toxicological Profile for Diethyl Phthalate (Atlanta, Georgia: Agency for Toxic Substances and Disease Registry Division of Toxicology/Toxicology Information Branch). doi: 10.1201/9781420061888_ch74
ATSDR (2019). Toxicological profile for di(2-ethylhexyl)Phthalate (DEHP) draft for public comment (Atlanta, GA). Available online at: www.regulations.gov (Accessed March 20, 2020).
Atsdr (2022). Toxicological Profile for Di(2-Ethylhexyl)Phthalate (DEHP) (Atlanta, Georgia: Agency for Toxic Substances and Disease Registry Division of Toxicology/Toxicology Information Branch).
Axelsson J., Rylander L., Rignell-Hydbom A., Jönsson B. A. G., Lindh C. H., Giwercman A. (2015). Phthalate exposure and reproductive parameters in young men from the general Swedish population. Environ. Int. 85, 54–60. doi: 10.1016/J.ENVINT.2015.07.005
Baini M., Martellini T., Cincinelli A., Campani T., Minutoli R., Panti C., et al. (2017). First detection of seven phthalate esters (PAEs) as plastic tracers in superficial neustonic/planktonic samples and cetacean blubber. Analytical Methods 9, 1512–1520. doi: 10.1039/c6ay02674e
Barratclough A., Wells R. S., Schwacke L. H., Rowles T. K., Gomez F. M., Fauquier D. A., et al. (2019). Health assessments of common bottlenose dolphins (Tursiops truncatus): past, present, and potential conservation applications. Front. Vet. Sci. 6. doi: 10.3389/FVETS.2019.00444/BIBTEX
Bergé A., Gasperi J., Rocher V., Gras L., Coursimault A., Moilleron R. (2014). Phthalates and alkylphenols in industrial and domestic effluents: Case of Paris conurbation (France). Sci. Total Environ. 488–489, 26–35. doi: 10.1016/J.SCITOTENV.2014.04.081
Blount B. C., Silva M. J., Caudill S. P., Needham L. L., Pirkle J. L., Sampson E. J., et al. (2000). Levels of seven urinary phthalate metabolites in a human reference population. Environ. Health Perspect. 108, 979–982. doi: 10.1289/ehp.00108979
Blum M. J., Bernik B. M., Azwell T., Hoek E. M. V. (2014). Remediation and Restoration of Northern Gulf of Mexico Coastal Ecosystems Following the Deepwater Horizon Event Vol. 6 (Hoboken, New Jersey: Wiley Blackwell), 59–88. doi: 10.1002/9781118825662.CH3
Boas M., Frederiksen H., Feldt-Rasmussen U., Skakkebæk N. E., Hegedüs L., Hilsted L., et al. (2010). Childhood exposure to phthalates: Associations with thyroid function, insulin-like growth factor I, and growth. Environ. Health Perspect. 118, 1458–1464. doi: 10.1289/EHP.0901331/ASSET/0A711348-F49A-4DA4-A315-881AC4F7F6B5/ASSETS/GRAPHIC/EHP-118-1458F2.JPG
Brock J. W., Bell J. M., Guillette L. J. (2016). Urinary Phthalate Metabolites in American alligators (Alligator mississippiensis) from selected Florida wetlands. Arch. Environ. Contam. Toxicol. 71, 1–6. doi: 10.1007/s00244-015-0260-6
Buck Louis G. M., Sundaram R., Sweeney A. M., Schisterman E. F., Maisog J., Kannan K. (2014). Urinary bisphenol A, phthalates, and couple fecundity: the Longitudinal Investigation of Fertility and the Environment (LIFE) Study. Fertil. Steril. 101, 1359–1366. doi: 10.1016/J.FERTNSTERT.2014.01.022
Calenge C. (2006). The package “adehabitat” for the R software: A tool for the analysis of space and habitat use by animals. Ecol. Modell. 197, 516–519. doi: 10.1016/J.ECOLMODEL.2006.03.017
Cartwright C. D., Thompson I. P., Burns R. G. (2000). Degradation and impact of phthalate plasticizers on soil microbial communities. Environ. Toxicol. Chem. 19, 1253–1261. doi: 10.1002/ETC.5620190506
Chang W. H., Li S. S., Wu M. H., Pan H. A., Lee C. C. (2015). Phthalates might interfere with testicular function by reducing testosterone and insulin-like factor 3 levels. Hum. Reprod. 30, 2658–2670. doi: 10.1093/humrep/dev225
Cheng Z., Liu J. B., Gao M., Shi G. Z., Fu X. J., Cai P., et al. (2019). Occurrence and distribution of phthalate esters in freshwater aquaculture fish ponds in Pearl River Delta, China. Environ. Pollution 245, 883–888. doi: 10.1016/j.envpol.2018.11.085
Chevalier N., Fénichel P. (2015). Endocrine disruptors: New players in the pathophysiology of type 2 diabetes? Diabetes Metab. 41, 107–115. doi: 10.1016/J.DIABET.2014.09.005
Choi K., Joo H., Campbell J. L., Andersen M. E., Clewell H. J. (2013). In vitro intestinal and hepatic metabolism of Di(2-ethylhexyl) phthalate (DEHP) in human and rat. Toxicol. Vitro 27, 1451–1457. doi: 10.1016/j.tiv.2013.03.012
City of Sarasota (2024). Zoning (Sarasota, FL: Municode Library). Available online at: https://library.municode.com/fl/sarasota/codes/zoning (Accessed December 30, 2024).
Clara M., Windhofer G., Hartl W., Braun K., Simon M., Gans O., et al. (2010). Occurrence of phthalates in surface runoff, untreated and treated wastewater and fate during wastewater treatment. Chemosphere 78, 1078–1084. doi: 10.1016/j.chemosphere.2009.12.052
CWPPRA (2022). The barataria basin (Coastal Wetlands Planning, Protection and Resoration Act). Available online at: https://lacoast.gov/new/about/basin_data/ba/default.aspx (Accessed February 28, 2022).
Dadhich S., Lalu L. P. (2018). “Oil spill cleaning and recovery by polyurethane sponge coated with silane molecules,” in Society of Petroleum Engineers - SPE International Conference and Exhibition on Health, Safety, Security, Environment, and Social Responsibility 2018, Abu Dhabi, UAE. doi: 10.2118/190484-MS
Du P., Zhou Z., Huang H., Han S., Xu Z., Bai Y., et al. (2018). Estimating population exposure to phthalate esters in major Chinese cities through wastewater-based epidemiology. Sci. Total Environ. 643, 1602–1609. doi: 10.1016/J.SCITOTENV.2018.06.325
Duong H. T., Kadokami K., Pan S., Matsuura N., Nguyen T. Q. (2014). Screening and analysis of 940 organic micro-pollutants in river sediments in Vietnam using an automated identification and quantification database system for GC–MS. Chemosphere 107, 462–472. doi: 10.1016/J.CHEMOSPHERE.2014.01.064
Dziobak M. K., Balmer B. C., Wells R. S., Pisarski E. C., Wirth E. F., Hart L. B. (2022a). Temporal and spatial evaluation of mono(2-ethylhexyl) phthalate (MEHP) detection in common bottlenose dolphins (Tursiops truncatus) from sarasota bay, Florida, USA. Oceans 3, 231–249. doi: 10.3390/OCEANS3030017
Dziobak MK, Curtin T, Wells RS, Takeshita R, Smith CR, Zolman E, Toms CN, Allen RF, Hart LB. (2025). Demographic information and phthalate metabolite concentrations (µg/L) detected in bottlenose dolphins (Tursiops truncatus) urine sampled from Barataria Bay, LA during 2011-2023 and Sarasota Bay, FL during 2010-2019, 2022-2024. DRYAD data repository. doi: 10.5061/dryad.pc866t20f
Dziobak M. K., Wells R. S., Pisarski E. C., Wirth E. F., Hart L. B. (2021). Demographic assessment of mono(2-ethylhexyl) phthalate (MEHP) and monoethyl phthalate (MEP) concentrations in common bottlenose dolphins (Tursiops truncatus) from Sarasota Bay, FL, USA. Geohealth 5, e2020GH000348. doi: 10.1029/2020GH000348
Dziobak M. K., Wells R. S., Pisarski E. C., Wirth E. F., Hart L. B. (2022b). A correlational analysis of phthalate exposure and thyroid hormone levels in common bottlenose dolphins (Tursiops truncatus) from sarasota bay, Florida, (2010-2019). Animals 12, 1–15. doi: 10.3390/ANI12070824
Eriksen M., Cowger W., Erdle L. M., Coffin S., Villarrubia-Gómez P., Moore C. J., et al. (2023). A growing plastic smog, now estimated to be over 170 trillion plastic particles afloat in the world’s oceans—Urgent solutions required. PloS One 18, e0281596. doi: 10.1371/JOURNAL.PONE.0281596
Fan J., Traore K., Li W., Amri H., Huang H., Wu C., et al. (2010). Molecular mechanisms mediating the effect of mono-(2-ethylhexyl) phthalate on hormone-stimulated steroidogenesis in MA-10 mouse tumor leydig cells. Endocrinology 151, 3348–3362. doi: 10.1210/en.2010-0010
Fatoki O. S., Bornman M., Ravandhalala L., Chimuka L., Genthe B., Adeniyi A. (2010). Phthalate ester plasticizers in freshwater systems of Venda, South Africa and potential health effects. Water SA 36, 117–126. doi: 10.4314/wsa.v36i1.50916
Ferguson K. K., McElrath T. F., Meeker J. D. (2014). Environmental phthalate exposure and preterm birth. JAMA Pediatr. 168, 61–67. doi: 10.1001/JAMAPEDIATRICS.2013.3699
Ferguson K. K., Rosen E. M., Rosario Z., Feric Z., Calafat A. M., McElrath T. F., et al. (2019). Environmental phthalate exposure and preterm birth in the PROTECT birth cohort. Environ. Int. 132, 105099. doi: 10.1016/J.ENVINT.2019.105099
Fossi M. C., Panti C., Guerranti C., Coppola D., Giannetti M., Marsili L., et al. (2012). Are baleen whales exposed to the threat of microplastics? A case study of the Mediterranean fin whale (Balaenoptera physalus). Mar. Pollut. Bull. 64, 2374–2379. doi: 10.1016/j.marpolbul.2012.08.013
Fourgous C., Chevreuil M., Alliot F., Amilhat E., Faliex E., Paris-Palacios S., et al. (2016). Phthalate metabolites in the European eel (Anguilla Anguilla) from Mediterranean coastal lagoons. Sci. Total Environ. 569–570, 1053–1059. doi: 10.1016/j.scitotenv.2016.06.159
Frederiksen H., Skakkebæk N. E., Andersson A. M. (2007). Metabolism of phthalates in humans. Mol. Nutr. Food Res. 51, 899–911. doi: 10.1002/mnfr.200600243
Gao D. W., Wen Z. D. (2016). Phthalate esters in the environment: A critical review of their occurrence, biodegradation, and removal during wastewater treatment processes. Sci. Total Environ. 541, 986–1001. doi: 10.1016/j.scitotenv.2015.09.148
González-Mariño I., Rodil R., Barrio I., Cela R., Quintana J. B. (2017). Wastewater-based epidemiology as a new tool for estimating population exposure to phthalate plasticizers. Environ. Sci. Technol. 51, 3902–3910. doi: 10.1021/ACS.EST.6B05612/ASSET/IMAGES/LARGE/ES-2016-05612Z_0002.JPEG
Gray T. J. B., Gangolli S. D. (1986). Aspects of the testicular toxicity of phthalate esters. Environ. Health Perspect. 65, 229–235. doi: 10.1289/ehp.8665229
GulfBase (2024a). Barataria bay. Available online at: https://www.gulfbase.org/geological-feature/barataria-bay (Accessed December 30, 2024).
GulfBase (2024b). Sarasota bay. Available online at: https://www.gulfbase.org/geological-feature/sarasota-bay-0 (Accessed December 30, 2024).
Guo Y., Kannan K. (2013). A survey of phthalates and parabens in personal care products from the United States and its implications for human exposure. Environ. Sci. Technol. 47, 14442–14449. doi: 10.1021/es4042034
Guo Y., Wang L., Kannan K. (2014). Phthalates and parabens in personal care products from China: Concentrations and human exposure. Arch. Environ. Contam. Toxicol. 66, 113–119. doi: 10.1007/s00244-013-9937-x
Gupta R. K., Singh J. M., Leslie T. C., Meachum S., Flaws J. A., Yao H. H. C. (2010). Di-(2-ethylhexyl) phthalate and mono-(2-ethylhexyl) phthalate inhibit growth and reduce estradiol levels of antral follicles in vitro. Toxicol. Appl. Pharmacol. 242, 224–230. doi: 10.1016/j.taap.2009.10.011
Hart L. B., Beckingham B., Wells R. S., Alten Flagg M., Wischusen K., Moors A., et al. (2018). Urinary phthalate metabolites in common bottlenose dolphins (Tursiops truncatus) from sarasota bay, FL, USA. Geohealth 2, 313–326. doi: 10.1029/2018gh000146
Hart L. B., Dziobak M. K., Pisarski E. C., Wirth E. F., Wells R. S. (2020). Sentinels of synthetics – a comparison of phthalate exposure between common bottlenose dolphins (Tursiops truncatus) and human reference populations. PloS One 15, e0240506. doi: 10.1371/journal.pone.0240506
Hart L. B., Dziobak M., Wells R. S., Ertel B., Weinstein J. (2022). Microplastics in gastric samples from common bottlenose dolphins (Tursiops truncatus) residing in Sarasota Bay FL (USA). Front. Mar. Sci. 9. doi: 10.3389/FMARS.2022.947124/BIBTEX
Hauser R., Calafat A. M. (2005). Phthalates and human health. Occup. Environ. Med. 62, 806–818. doi: 10.1136/oem.2004.017590
Hauser R., Gaskins A. J., Souter I., Smith K. W., Dodge L. E., Ehrlich S., et al. (2016). Urinary phthalate metabolite concentrations and reproductive outcomes among women undergoing in vitro fertilization: Results from the EARTH study. Environ. Health Perspect. 124, 831–839. doi: 10.1289/ehp.1509760
Hauser R., Meeker J. D., Park S., Silva M. J., Calafat A. M. (2004). Temporal variability of urinary phthalate metabolite levels in men of reproductive age. Environ. Health Perspect. 112, 1734–1740. doi: 10.1289/ehp.7212
Helsel D. R. (2006). Fabricating data: How substituting values for nondetects can ruin results, and what can be done about it. Chemosphere 65, 2434–2439. doi: 10.1016/j.chemosphere.2006.04.051
Heudorf U., Mersch-Sundermann V., Angerer J. (2007). Phthalates: toxicology and exposure. Int. J. Hyg. Environ. Health 210, 623–634. doi: 10.1016/j.ijheh.2007.07.011
Hohn A. A., Scott M. D., Wells R. S., Sweeney J. C., Irvine A. B. (1989). GROWTH LAYERS IN TEETH FROM KNOWN-AGE, FREE-RANGING BOTTLENOSE DOLPHINS. Mar. Mamm. Sci. 5, 315–342. doi: 10.1111/j.1748-7692.1989.tb00346.x
Hongjun Y., Wenjun X., Qing L., Jingtao L., Hongwen Y., Zhaohua L. (2013). Distribution of phthalate esters in topsoil: A case study in the Yellow River Delta, China. Environ. Monit. Assess. 185, 8489–8500. doi: 10.1007/S10661-013-3190-7/FIGURES/5
Hu X., Gu Y., Huang W., Yin D. (2016). Phthalate monoesters as markers of phthalate contamination in wild marine organisms. Environ. Pollution 218, 410–418. doi: 10.1016/j.envpol.2016.07.020
Hubinger J. C. (2010). A survey of phthalate esters in consumer cosmetic products. J. Cosmet. Sci. 61, 457–465.
Jukic A. M., Calafat A. M., Robert McConnaughey D., Longnecker M. P., Hoppin J. A., Weinberg C. R., et al. (2016). Urinary concentrations of phthalate metabolites and bisphenol a and associations with follicular-phase length, luteal-phase length, fecundability, and early pregnancy loss. Environ. Health Perspect. 124, 321–328. doi: 10.1289/EHP.1408164/SUPPL_FILE/EHP.1408164.S001.ACCO.PDF
Julian P., Helsel D. R. (2023). NADA2: Data Analysis for Censored Environmental Data: R package (R package).
Jurewicz J., Radwan M., Sobala W., Ligocka D., Radwan P., Bochenek M., et al. (2013). Human urinary phthalate metabolites level and main semen parameters, sperm chromatin structure, sperm aneuploidy and reproductive hormones. Reprod. Toxicol. 42, 232–241. doi: 10.1016/J.REPROTOX.2013.10.001
Kaewlaoyoong A., Vu C. T., Lin C., Liao C.S., Chen J. R. (2018). Occurrence of phthalate esters around the major plastic industrial area in southern Taiwan. Environ. Earth Sci. 77, 1–11. doi: 10.1007/S12665-018-7655-4/FIGURES/4
Karamianpour J., Arfaeinia H., Ranjbar Vakilabadi D., Ramavandi B., Dobaradaran S., Fazlzadeh M., et al. (2023). Accumulation, sources, and health risks of phthalic acid esters (PAEs) in road dust from heavily industrialized, urban and rural areas in southern Iran. Heliyon 9, e23129. doi: 10.1016/J.HELIYON.2023.E23129/ATTACHMENT/7C6AF070-2D6B-47DE-9B58-282D153F6413/MMC1.DOC
Kellar N. M., Speakman T. R., Smith C. R., Lane S. M., Balmer B. C., Trego M. L., et al. (2017). Low reproductive success rates of common bottlenose dolphins Tursiops truncatus in the northern Gulf of Mexico following the Deepwater Horizon disaster, (2010-2015). Endanger Species Res. 33, 143–158. doi: 10.3354/ESR00775
Kie J. G. (2013). A rule-based ad hoc method for selecting a bandwidth in kernel home-range analyses. Anim. Biotelemetry 1, 13. doi: 10.1186/2050-3385-1-13
Kim H. S., Saito K., Ishizuka M., Kazusaka A., Fujita S. (2003). Short period exposure to di-(2-ethylhexyl) phthalate regulates testosterone metabolism in testis of prepubertal rats. Arch. Toxicol. 77, 446–451. doi: 10.1007/s00204-003-0466-7
Koch H. M., Calafat A. M. (2009). Human body burdens of chemicals used in plastic manufacture. Philos. Trans. R. Soc. B: Biol. Sci. 364, 2063–2078. doi: 10.1098/RSTB.2008.0208
Kokaly R. F., Couvillion B. R., Holloway J. A. M., Roberts D. A., Ustin S. L., Peterson S. H., et al. (2013). Spectroscopic remote sensing of the distribution and persistence of oil from the Deepwater Horizon spill in Barataria Bay marshes. Remote Sens. Environ. 129, 210–230. doi: 10.1016/J.RSE.2012.10.028
Kwon O. Y., Kang J. H., Hong S. H., Shim W. J. (2020). Spatial distribution of microplastic in the surface waters along the coast of Korea. Mar. Pollut. Bull. 155, 110729. doi: 10.1016/J.MARPOLBUL.2019.110729
Lacy R. C., Wells R. S., Scott M. D., Allen J. B., Barleycorn A. A., Urian K. W., et al. (2021). Assessing the viability of the sarasota bay community of bottlenose dolphins. Front. Mar. Sci. 8. doi: 10.3389/fmars.2021.788086
Lan Q., Cui K., Zeng F., Zhu F., Liu H., Chen H., et al. (2012). Characteristics and assessment of phthalate esters in urban dusts in Guangzhou city, China. Environ. Monit. Assess. 184, 4921–4929. doi: 10.1007/S10661-011-2312-3/TABLES/2
Langdon K. A., Chandra A., Bowles K., Symons A., Pablo F., Osborne K. (2019). A preliminary ecological and human health risk assessment for organic contaminants in composted municipal solid waste generated in New South Wales, Australia. Waste Manage. 100, 199–207. doi: 10.1016/J.WASMAN.2019.09.001
Latini G., De Felice C., Presta G., Del Vecchio A., Paris I., Ruggieri F., et al. (2003). In utero exposure to di-(2-ethylhexyl)phthalate and duration of human pregnancy. Environ. Health Perspect. 111, 1783–1785. doi: 10.1289/ehp.6202
Latini G., Del Vecchio A., Massaro M., Verrotti A., De Felice C. (2006). Phthalate exposure and male infertility. Toxicology 226, 90–98. doi: 10.1016/J.TOX.2006.07.011
Lee R. L., Helsel D. R. (2022). Nondetects and data analysis for environmental data: R-package. NADA version. 1–6.
Lee H. R., Jeung E. B., Cho M. H., Kim T. H., Leung P. C. K., Choi K. C. (2013). Molecular mechanism(s) of endocrine-disrupting chemicals and their potent oestrogenicity in diverse cells and tissues that express oestrogen receptors. J. Cell Mol. Med. 17, 1–11. doi: 10.1111/J.1582-4934.2012.01649.X
Lee Y. M., Lee J. E., Choe W., Kim T., Lee J. Y., Kho Y., et al. (2019). Distribution of phthalate esters in air, water, sediments, and fish in the Asan Lake of Korea. Environ. Int. 126, 635–643. doi: 10.1016/j.envint.2019.02.059
Li Y. Y., He W., Liu W. X., Yang B., He Q. S., Yang C., et al. (2020). Impacts of anthropogenic activities on spatial variations of phthalate esters in water and suspended particulate matter from China’s lakes. Sci. Total Environ. 724, 138281. doi: 10.1016/J.SCITOTENV.2020.138281
Liu W. L., Shen C. F., Zhang Z., Zhang C. B. (2009). Distribution of phthalate esters in soil of E-waste recycling sites from taizhou city in China. Bull. Environ. Contam. Toxicol. 82, 665–667. doi: 10.1007/S00128-009-9699-3/FIGURES/2
Liu Y., Weisberg R. H., Hu C., Zheng L. (2011). Tracking the deepwater horizon oil spill: A modeling perspective. Eos. Trans. Am. Geophysical Union 92, 45–46. doi: 10.1029/2011EO060001
Lyche J. L., Gutleb A. C., Bergman Å., Eriksen G. S., Murk A. J., Ropstad E., et al. (2009). Reproductive and developmental toxicity of phthalates. J. Toxicol. Environ. Health B Crit. Rev. 12, 225–249. doi: 10.1080/10937400903094091
Majeed K. A., ur Rehman H., Yousaf M. S., Zaneb H., Rabbani I., Tahir S. K., et al. (2017). Sub-chronic exposure to low concentration of dibutyl phthalate affects anthropometric parameters and markers of obesity in rats. Environ. Sci. Pollution Res. 24, 25462–25467. doi: 10.1007/S11356-017-9952-Y/TABLES/5
Mathieu-Denoncourt J., Wallace S. J., de Solla S. R., Langlois V. S. (2015). Plasticizer endocrine disruption: Highlighting developmental and reproductive effects in mammals and non-mammalian aquatic species. Gen. Comp. Endocrinol. 219, 74–88. doi: 10.1016/j.ygcen.2014.11.003
McDonald T. L., Hornsby F. E., Speakman T. R., Zolman E. S., Mullin K. D., Sinclair C., et al. (2017). Survival, density, and abundance of common bottlenose dolphins in Barataria Bay (USA) following the Deepwater Horizon oil spill. Endanger Species Res. 33, 193–209. doi: 10.3354/ESR00806
Meeker J. D., Ferguson K. K. (2014). Urinary phthalate metabolites are associated with decreased serum testosterone in men, women, and children from NHANES 2011-2012. J. Clin. Endocrinol. Metab. 99, 4346–4352. doi: 10.1210/jc.2014-2555
Messerlian C., Wylie B. J., Mínguez-Alarcón L., Williams P. L., Ford J. B., Souter I. C., et al. (2016). Urinary concentrations of phthalate metabolites and pregnancy loss among women conceiving with medically assisted reproduction. Epidemiology 27, 879–888. doi: 10.1097/EDE.0000000000000525
Monneret C. (2017). What is an endocrine disruptor? C R Biol. 340, 403–405. doi: 10.1016/J.CRVI.2017.07.004/
Mullin K. D., Barry K., Mcdonald T., Morey J., Quigley B., Ronje E., et al. (2018). Assessment of the overlap of Terrebone - Timbalier Bay and Barataria Bay common bottlenose dolphins (Tursiops truncatus) stock based on photo-identification of individual dolphins. NOAA Technical Memorandum. (Pascagoula, Mississippi: U.S. DEPARTMENT OF COMMERCE National Oceanic and Atmospheric Administration National Marine Fisheries Service Southeast Fisheries Science Center). doi: 10.25923/8g4y-dg29
Niu L., Xu Y., Xu C., Yun L., Liu W. (2014). Status of phthalate esters contamination in agricultural soils across China and associated health risks. Environ. Pollution 195, 16–23. doi: 10.1016/J.ENVPOL.2014.08.014
Pagnucco R., Phillips M. L. (2018). Comparative effectiveness of natural by-products and synthetic sorbents in oil spill booms. J. Environ. Manage 225, 10–16. doi: 10.1016/J.JENVMAN.2018.07.094
Paluselli A., Fauvelle V., Galgani F., Sempéré R. (2019). Phthalate release from plastic fragments and degradation in seawater. Environ. Sci. Technol. 53, 166–175. doi: 10.1021/acs.est.8b05083
Pant N., Kumar G., Upadhyay A. D., Patel D. K., Gupta Y. K., Chaturvedi P. K. (2014). Reproductive toxicity of lead, cadmium, and phthalate exposure in men. Environ. Sci. Pollution Res. 21, 11066–11074. doi: 10.1007/s11356-014-2986-5
Parks L. G. P., Ostby J. S., Lambright C. R., Abbott B. D., Klinefelter G. R., Barlow N. J., et al. (2000). The plasticizer diethylhexyl phthalate induces malformations by decreasing fetal testosterone synthesis during sexual differentiation in the male rat. Toxicological Sci. 58, 339–349. doi: 10.1093/toxsci/58.2.339
Prasad B., Prasad K. S., Dave H., Das A., Asodariya G., Talati N., et al. (2022). Cumulative human exposure and environmental occurrence of phthalate esters: A global perspective. Environ. Res. 210, 112987. doi: 10.1016/J.ENVRES.2022.112987
Qian Y., Shao H., Ying X., Huang W., Hua Y. (2020). The endocrine disruption of prenatal phthalate exposure in mother and offspring. Front. Public Health 8. doi: 10.3389/FPUBH.2020.00366/BIBTEX
Radke E. G., Braun J. M., Meeker J. D., Cooper G. S. (2018). Phthalate exposure and male reproductive outcomes: A systematic review of the human epidemiological evidence. Environ. Int. 121, 764–793. doi: 10.1016/J.ENVINT.2018.07.029
Radke E. G., Galizia A., Thayer K. A., Cooper G. S. (2019). Phthalate exposure and metabolic effects: a systematic review of the human epidemiological evidence. Environ. Int. 132, 104768. doi: 10.1016/j.envint.2019.04.040
Routti H., Harju M., Lühmann K., Aars J., Ask A., Goksøyr A., et al. (2021). Concentrations and endocrine disruptive potential of phthalates in marine mammals from the Norwegian Arctic. Environ. Int. 152,, 106458. doi: 10.1016/j.envint.2021.106458
Runkel A. A., Mazej D., Snoj Tratnik J., Tkalec Ž., Kosjek T., Horvat M. (2022). Exposure of men and lactating women to environmental phenols, phthalates, and DINCH. Chemosphere 286, 131858. doi: 10.1016/J.CHEMOSPHERE.2021.131858
Schrader E. L. (1991). Remediation of floating, open water oil spills: Comparative efficacy of commercially available polypropylene sorbent booms. Environ. Geol. Water Sci. 17, 157–166. doi: 10.1007/BF01701571/METRICS
Schwacke L. H., Marques T. A., Thomas L., Booth C. G., Balmer B. C., Barratclough A., et al. (2022). Modeling population effects of the Deepwater Horizon oil spill on a long-lived species. Conserv. Biol. 36, e13878. doi: 10.1111/COBI.13878
Schwacke L. H., Smith C. R., Townsend F. I., Wells R. S., Hart L. B., Balmer B. C., et al. (2014). Health of common bottlenose dolphins (Tursiops truncatus) in Barataria Bay, Louisiana, following the Deepwater Horizon oil spill. Environ. Sci. Technol. 48, 93–103. doi: 10.1021/ES403610F/SUPPL_FILE/ES403610F_SI_001.PDF
Schwacke L. H., Thomas L., Wells R. S., Mcfee W. E., Hohn A. A., Mullin K. D., et al. (2017). Quantifying injury to common bottlenose dolphins from the Deepwater Horizon oil spill using an age-, sex-and class-structured population model. ENDANGERED SPECIES Res. Endang. Species Res. 33, 265–279. doi: 10.3354/esr00777
Schwacke L. H., Thomas L., Wells R. S., Rowles T. K., Bossart G. D., Townsend F., et al. (2024). An expert-based system to predict population survival rate from health data. Conserv. Biol. 38, e14073. doi: 10.1111/COBI.14073
Sengupta A., Lyons J. M., Smith D. J., Drewes J. E., Snyder S. A., Heil A., et al. (2014). The occurrence and fate of chemicals of emerging concern in coastal urban rivers receiving discharge of treated municipal wastewater effluent. Environ. Toxicol. Chem. 33, 350–358. doi: 10.1002/ETC.2457
Seyoum A., Pradhan A. (2019). Effect of phthalates on development, reproduction, fat metabolism and lifespan in Daphnia magna. Sci. Total Environ. 654, 969–977. doi: 10.1016/J.SCITOTENV.2018.11.158
Shoaff J. R., Romano M. E., Yolton K., Lanphear B. P., Calafat A. M., Braun J. M. (2016). Prenatal phthalate exposure and infant size at birth and gestational duration. Environ. Res. 150, 52–58. doi: 10.1016/J.ENVRES.2016.05.033
Smith C., Rowles T., Hart L., Townsend F., Wells R., Zolman E., et al. (2017). Slow recovery of Barataria Bay dolphin health following the Deepwater Horizon oil spill, (2013-2014), with evidence of persistent lung disease and impaired stress response. Endanger Species Res. 33, 127–142. doi: 10.3354/esr00778
Staples C. A., Peterson D. R., Parkerton T. F., Adams W. J. (1997). The environmental fate of phthalate esters: A literature review. Chemosphere 35, 667–749. doi: 10.1016/S0045-6535(97)00195-1
Stojanoska M. M., Milosevic N., Milic N., Abenavoli L. (2017). The influence of phthalates and bisphenol A on the obesity development and glucose metabolism disorders. Endocrine 55, 666–681. doi: 10.1007/s12020-016-1158-4
Suaria G., Perold V., Lee J. R., Lebouard F., Aliani S., Ryan P. G. (2020). Floating macro- and microplastics around the Southern Ocean: Results from the Antarctic Circumnavigation Expedition. Environ. Int. 136, 105494. doi: 10.1016/J.ENVINT.2020.105494
Sun J., Huang J., Zhang A., Liu W., Cheng W. (2013). Occurrence of phthalate esters in sediments in Qiantang River, China and inference with urbanization and river flow regime. J. Hazard Mater. 248–249, 142–149. doi: 10.1016/j.jhazmat.2012.12.057
Takeshita R., Balmer B. C., Messina F., Zolman E. S., Thomas L., Wells R. S., et al. (2021). High site-fidelity in common bottlenose dolphins despite low salinity exposure and associated indicators of compromised health. PloS One 16, 1–25. doi: 10.1371/journal.pone.0258031
Teil M. J., Tlili K., Blanchard M., Labadie P., Alliot F., Chevreuil M. (2014). Polychlorinated biphenyls, polybrominated diphenyl ethers, and phthalates in roach from the Seine River basin (France): Impact of densely urbanized areas. Arch. Environ. Contam. Toxicol. 66, 41–57. doi: 10.1007/S00244-013-9955-8/FIGURES/5
Teitelbaum S. L. L., Britton J. A. A., Calafat A. M. M., Ye X., Silva M. J. J., Reidy J. A. A., et al. (2008). Temporal variability in urinary concentrations of phthalate metabolites, phytoestrogens and phenols among minority children in the United States. Environ. Res. 106, 257–269. doi: 10.1016/j.envres.2007.09.010
Thushari G. G. N., Senevirathna J. D. M. (2020). Plastic pollution in the marine environment. Heliyon 6 , e04709. doi: 10.1016/J.HELIYON.2020.E04709/ASSET/406BF0D5-AC94-4756-85E6-737CAAB05DE2/MAIN.ASSETS/GR3.JPG
Toft G., Jönsson B. A. G., Lindh C. H., Jensen T. K., Hjollund N. H., Vested A., et al. (2012). Association between pregnancy loss and urinary phthalate levels around the time of conception. Environ. Health Perspect. 120, 458–463. doi: 10.1289/ehp.1103552
Tranfo G., Caporossi L., Paci E., Aragona C., Romanzi D., De Carolis C., et al. (2012). Urinary phthalate monoesters concentration in couples with infertility problems. Toxicol. Lett. 213, 15–20. doi: 10.1016/J.TOXLET.2011.11.033
Tyson R. B., Wells R. S. (2016). Sarasota Bay/Little Sarasota Bay bottlenose dolphin abundance estimates: 2015. doi: 10.7289/V5/RD-PRBD-2016-02
US Census Bureau (2024a). Grand Isle town, Louisiana - Census Bureau Profile. Available online at: https://data.census.gov/profile/Grand_Isle_town,_Louisiana?g=160XX00US2230830 (Accessed December 30, 2024).
US Census Bureau (2024b). Sarasota city, Florida - Census Bureau Profile. Available online at: https://data.census.gov/profile/Sarasota_city,_Florida?g=160XX00US1264175 (Accessed December 30, 2024).
USF (2020). Sarasota bay watershed (Sarasota County, USF Water Institute). Available online at: https://www.sarasota.wateratlas.usf.edu/watershed/geography.asp?wshedid=5&wbodyatlas=watershed (Accessed February 13, 2021).
Valton A. S., Serre-Dargnat C., Blanchard M., Alliot F., Chevreuil M., Teil M. J. (2014). Determination of phthalates and their by-products in tissues of roach (Rutilus rutilus) from the Orge river (France). Environ. Sci. Pollution Res. 21, 12723–12730. doi: 10.1007/s11356-014-3213-0
Viljoen S. J., Brailsford F. L., Murphy D. V., Hoyle F. C., Chadwick D. R., Jones D. L. (2023). Leaching of phthalate acid esters from plastic mulch films and their degradation in response to UV irradiation and contrasting soil conditions. J. Hazard Mater. 443, 130256. doi: 10.1016/J.JHAZMAT.2022.130256
Wang J., Bo L., Li L., Wang D., Chen G., Christie P., et al. (2014). Occurrence of phthalate esters in river sediments in areas with different land use patterns. Sci. Total Environ., 500–501. doi: 10.1016/J.SCITOTENV.2014.08.092
Wang L., Liu M., Tao W., Zhang W., Wang L., Shi X., et al. (2018). Pollution characteristics and health risk assessment of phthalate esters in urban soil in the typical semi-arid city of Xi’an, Northwest China. Chemosphere 191, 467–476. doi: 10.1016/J.CHEMOSPHERE.2017.10.066
Wang J., Zhang X., Li Y., Liu Y., Tao L. (2021). Exposure to dibutyl phthalate and reproductive-related outcomes in animal models: evidence from rodents study. Front. Physiol. 12. doi: 10.3389/FPHYS.2021.684532/BIBTEX
Wardlaw C. M., Corcoran P. L., Neff B. D. (2022). Factors influencing the variation of microplastic uptake in demersal fishes from the upper Thames River Ontario. Environ. Pollution 313, 120095. doi: 10.1016/J.ENVPOL.2022.120095
Weaver J. A., Beverly B. E. J., Keshava N., Mudipalli A., Arzuaga X., Cai C., et al. (2020). Hazards of diethyl phthalate (DEP) exposure: A systematic review of animal toxicology studies. Environ. Int. 145, 105848. doi: 10.1016/J.ENVINT.2020.105848
Weizhen Z., Xiaowei Z., Peng G., Ning W., Zini L., Jian H., et al. (2020). Distribution and risk assessment of phthalates in water and sediment of the Pearl River Delta. Environ. Sci. Pollution Res. 27, 12550–12565. doi: 10.1007/s11356-019-06819-y
Wells R. S., Rhinehart H. L., Hansen L. J., Sweeney J. C., Townsend F. I., Stone R., et al. (2004). Bottlenose dolphins as marine ecosystem sentinels: developing a health monitoring system. EcoHealth 1, 246–254.
Wells R. S. (2009). Learning from nature: Bottlenose dolphin care and husbandry. Zoo Biol. 28, 635–651. doi: 10.1002/zoo.20252
Wells R. S. (2014). “Social Structure and Life History of Bottlenose Dolphins Near Sarasota Bay, Florida: Insights from Four Decades and Five Generations,” in Primates and Cetaceans. Eds. Yamagiwa J., Karczmarski L. (Springer, Tokyo), 149–172. doi: 10.1007/978-4-431-54523-1_8
Wells R. S., Hohn A. A., Scott M. D., Sweeney J. C., Townsend F. I., Allen J. B., et al. (2025). Life history, reproductive, and demographic parameters for bottlenose dolphins (Tursiops truncatus) in Sarasota Bay, Florida. Front. Mar. Sci. 12. doi: 10.3389/FMARS.2025.1531528/PDF
Wells R. S., Scott M. D., Irvine A. B. (1987). The social structure of free-ranging bottlenose dolphins. Curr. Mammal. 247–305, 247–305. doi: 10.1007/978-1-4757-9909-5_7
Wormuth M., Scheringer M., Vollenweider M., Hungerbühler K. (2006). What are the sources of exposure to eight frequently used phthalic acid esters in Europeans? Risk Anal. 26, 803–824. doi: 10.1111/J.1539-6924.2006.00770.X
Yang S., Arcanjo R. B., Nowak R. A. (2021). The effects of the phthalate DiNP on reproduction. Biol. Reprod. 104, 305–316. doi: 10.1093/BIOLRE/IOAA201
Yang P., Deng L. J., Xie J. Y., Li X. J., Wang X. N., Sun B., et al. (2023). Phthalate exposure with sperm quality among healthy Chinese male adults: The role of sperm cellular function. Environ. Pollution 331, 121755. doi: 10.1016/J.ENVPOL.2023.121755
Yang Y., Wan S., Yu L., Liu W., Song J., Shi D., et al. (2025). Phthalates exposure, biological aging, and increased risks of insulin resistance, prediabetes, and diabetes in adults with metabolic dysfunction-associated steatotic liver disease. Diabetes Metab. 51, 101602. doi: 10.1016/J.DIABET.2024.101602
Ye T., Kang M., Huang Q., Fang C., Chen Y., Shen H., et al. (2014). Exposure to DEHP and MEHP from hatching to adulthood causes reproductive dysfunction and endocrine disruption in marine medaka (Oryzias melastigma). Aquat. Toxicol. 146, 115–126. doi: 10.1016/j.aquatox.2013.10.025
Zhang S., Cheng X., Jia C., An J., Zhang X., Li P., et al. (2023). Association of serum phthalates exposure with incident type 2 diabetes risk in Chinese population: A nested case-control study. Ecotoxicol. Environ. Saf. 265, 115493. doi: 10.1016/J.ECOENV.2023.115493
Zhang Y., Liang Q., Gao R., Hou H., Tan W., He X., et al. (2015b). Contamination of phthalate esters (PAEs) in typical wastewater-irrigated agricultural soils in Hebei, north China. PloS One 10, e0137998. doi: 10.1371/journal.pone.0137998
Zhang X., Qi W., Xu Q., Li X., Zhou L., Ye L. (2021). Di(2-ethylhexyl) phthalate (DEHP) and thyroid: biological mechanisms of interference and possible clinical implications. Environ. Sci. Pollution Res. 29, 1634–1644. doi: 10.1007/S11356-021-17027-Y
Zhang H., Zhang Z., Nakanishi T., Wan Y., Hiromori Y., Nagase H., et al. (2015a). Structure-dependent activity of phthalate esters and phthalate monoesters binding to human constitutive androstane receptor. Chem. Res. Toxicol. 28, 1196–1204. doi: 10.1021/ACS.CHEMRESTOX.5B00028/SUPPL_FILE/TX5B00028_SI_001.PDF
Zhang Z. M., Zhang H. H., Zhang J., Wang Q. W., Yang G. P. (2018). Occurrence, distribution, and ecological risks of phthalate esters in the seawater and sediment of Changjiang River Estuary and its adjacent area. Sci. Total Environ. 619–620, 93–102. doi: 10.1016/j.scitotenv.2017.11.070
Zhong X., Yi X., Cheng F., Tong H., Xu W., Yang X. (2023). Leaching of di-2-ethylhexyl phthalate from biodegradable and conventional microplastics and the potential risks. Chemosphere 311, 137208. doi: 10.1016/J.CHEMOSPHERE.2022.137208
Keywords: marine mammal, pollution, Sarasota Bay, Barataria Bay, plastic
Citation: Dziobak MK, Curtin T, Wells RS, Takeshita R, Smith CR, Zolman E, Toms CN, Allen RF and Hart LB (2025) Comparing phthalate exposure between bottlenose dolphins (Tursiops truncatus) residing in urban and rural environments. Front. Mar. Sci. 12:1554075. doi: 10.3389/fmars.2025.1554075
Received: 31 December 2024; Accepted: 09 April 2025;
Published: 12 May 2025.
Edited by:
James Scott Maki, Marquette University, United StatesReviewed by:
Cristina Panti, University of Siena, ItalyYujiang Hao, Chinese Academy of Sciences (CAS), China
Copyright © 2025 Dziobak, Curtin, Wells, Takeshita, Smith, Zolman, Toms, Allen and Hart. This is an open-access article distributed under the terms of the Creative Commons Attribution License (CC BY). The use, distribution or reproduction in other forums is permitted, provided the original author(s) and the copyright owner(s) are credited and that the original publication in this journal is cited, in accordance with accepted academic practice. No use, distribution or reproduction is permitted which does not comply with these terms.
*Correspondence: Miranda K. Dziobak, ZHppb2Jha21rQGNvZmMuZWR1