- 1Department of Neurology, Xuanwu Hospital, Capital Medical University, Beijing, China
- 2The Affiliated Hospital and the Second Clinical Medical College of North Sichuan Medical University, Nanchong Central Hospital, Nanchong, China
- 3National Clinical Research Center for Geriatric Disorders, Beijing, China
- 4Center of Alzheimer's Disease, Beijing Institute for Brain Disorders, Beijing, China
Since late stage dementia, including Alzheimer's disease (AD), cannot be reversed by any available drugs, there is increasing research interest in the preclinical stage of AD, i.e., subjective cognitive decline (SCD). SCD is characterized by self-perceptive cognitive decline but is difficult to detect using objective tests. At SCD stage, the cognitive deficits can be more easily reversed compared to that of mild cognitive impairment (MCI) and AD only if accurate diagnosis of SCD and early intervention can be developed. In this paper, we review the recent progress of SCD research including current assessment tools, biomarkers, neuroimaging, intervention and expected prognosis, and the potential relevance to traumatic brain injury (TBI)-induced cognitive deficits.
Background
In 1982, Reisberg (1) classified the course of Alzheimer's disease (AD) progression into the following seven stages according to the patients' clinical manifestations: (1) no cognitive decline, (2) very mild cognitive decline, (3) mild cognitive decline, (4) moderate cognitive decline, (5) moderately severe cognitive decline, (6) severe cognitive decline, and (7) very severe cognitive decline. The second stage was regarded as the earliest description of AD-related subjective cognitive decline (SCD). With the increased understanding of AD etiology detected by neuropathology, neuropsychology, pathophysiology, neuroimaging, and other techniques, the National Institute on Aging and Alzheimer's Association (NIA-AA) suggested that AD be subdivided into AD-preclinical stage, AD-MCI stage, and AD-dementia stage (2, 3). Of them, the preclinical phase of AD can be further divided into 3 substages: (1) amyloid deposition, (2) amyloid deposition and neuronal degeneration, and (3) neuronal degeneration and amyloid deposition with subtle cognitive decline (i.e., SCD stage). Until now SCD has had multiple names: subjective memory impairment (SMI), subjective cognitive complain (SCC), and subjective memory complaint (SMC) etc., with memory decline as the most prominent manifestation. With the recent research progress and understanding of the clinical symptoms of SCD from basic research and clinical studies, it is now recognized that visual space damage, language impairment, attention deficit, and other symptoms could co-exist in SCD patients. Therefore, “cognition” was the more appropriate word to describe the clinical characteristics of SCD rather than “memory” (4–6). Thus, the Subjective Cognitive Decline Initiative (SCD-I) Working Group has advocated the use of the name-SCD (6).
In 2014, the SCD-I working group proposed the SCD conceptual framework, and by redefining SCD, emphasized that the subjective perception of cognitive function decline of SCD patients is not a state and thus does not need to be confirmed by objective cognitive tests. Thus, the purpose of neuropsychological examination of SCD is to exclude the possibility of objective cognitive impairment. SCD could be characterized as memory impairment or multiple cognitive domains impairment. The SCD-I conceptual framework also described important clinical features and accompanying symptoms of SCD, emphasizing that key information—such as age of onset, onset manifestation, symptom fluctuation, accompanying symptoms, circumstantial evidence, and comorbidity—should be collected in future research. The conceptual framework has also emphasized that the following features can increase the likelihood of preclinical AD in individuals with SCD: subjective perceptive memory decline, onset of disease within 5 years, onset at over 60 years of age, worrying about cognition decline, or subjectively feeling worse memory than the same age group. Specificity of the SCD diagnosis increases if the following conditions are present: informative confirmation, carrying ApoEe4 allele, and with positive AD pathophysiological biomarkers. In short, SCD has gradually become better understood.
Stewart and others (4, 7) have suggested that toward the end of the preclinical stage of AD, the subjective perception of cognitive decline in the elderly is more sensitive than objective neuropsychological tests. Pathological changes in AD could have occurred years ahead of the manifestation of MCI (8), which suggests that there should be a “pre-MCI” phase before the manifestation of MCI. The cognitive functional deficits of the patients could be reversible to normal at this stage. Thus, SCD may be a critical stage for early diagnosis and intervention of AD.
SCD is an intermediate state between normal cognition and MCI that may predict the development of objective cognitive decline (9–11). AD is a progressive disease and an abnormal deposition of Aβ and Tau in the brain may occur 15–20 years before the clinical diagnosis of AD, whereas MCI often occur 2 to 3 years before the onset of AD (12). Therefore, the extended SCD concept fills the gap between the manifestation of initial decline in cognitive function of AD and occurrence of MCI. The inclusion of the SCD concept completes the full picture of AD development (from normal state to SCD to MCI and to AD) and provides the basis for the diagnosis and intervention of AD at the very early stage of the disease (2, 6, 13).
Epidemiology
Epidemiological studies showed the prevalence of SCD ranged from 10 to 88%, with the highest prevalence found in the most advanced age group: 20% among people aged 65 years old and younger, 25 to 50% among people older than 65, and 88% among people older than 85 (4, 7, 14, 15). Jonker et al. (16) reported a 34.3% prevalence of SCD in community-based people aged 65–85 in the Netherlands, whereas Luck et al. (17) showed 12.3% prevalence of SCD in 117 of the 953 elderly participants (based on the general population sample). In a Spanish population-based cohort (ALFA project), 572 out of the 2670 participants studied reported SCD, showing a prevalence of 21.4% (18). Han et al. (19) explored the prevalence of SCD in the Shun Yi District in Beijing, China, by two standards. They found that the prevalence of SCD was 18.8% based on standard 1 (the Alzheimer's Disease Neuroimaging Initiative, ADNI) and 14.4% according to standard 2 (Jak/Bondi criterion), in their well-designed study. Another German study included 1,467 patients from clinics, wherein 792 (54.0%) reported SCD (20). In view of longitudinal studies, a multi-center longitudinal observational study (3-year follow-up) of the German Dementia Competence Network (DCN) revealed that 22% SCD patients progressed to MCI and 12% to AD, while only 3.7% subjects developed to a non-AD dementia (21). Some Swedish researchers found that in 122 SCD patients, over a follow-up period of 48 months, 39% declined cognitively and 10% converted to AD, in a clinical prospective single-center Gothenburg MCI study (22). Mitchell et al. (23) displayed that the conversion rate of SCD to MCI was 34.2% based on the community population over the mean period of 5 years through review of 28 studies. A recent 7-year follow-up study (24) showed that 212 subjects (109 patients with SCD) were considered: 26 out of 109 SCD subjects converted to MCI, 15 developed to AD, and 68 patients remained stable. A large-scale, 10-year-follow-up epidemiological survey conducted among 2,043 non dementia subjects showed that 372 old people developed dementia within 10 years (25, 26), of which 208 (55.9%) were diagnosed as AD. Cox regression analysis showed that SCD predicted all causes of dementia. From the results mentioned above, we can see that epidemiological datum of SCD differs from different studies because no common definition, criteria, and screening tools are available (27). Consequently, unified terminology and methodology and different populations (different age, community or clinic-based, etc.) are significant parts of further research.
Clinical Characteristics
Subjective memory impairment (SMI), such as recent memory decline, is a common clinical manifestation of SCD. In comparison, only a few SCD patients reported subjective language impairment.
Emotional state and individual heterogeneities could affect SCD diagnosis. People with depression and anxiety tend to over worry about their cognitive ability, memory, or other cognitive decline (10, 25). These patients tend to regard normal physiological forgetfulness as severe memory decline and believe their cognitive functions are getting worse, increasing the physical and mental burden. And this kind of memory decline is often age-related, non-pathological, and without objective evidence. However, recent studies suggest that late-life depression is associated with increased risk of all causes of dementia, including AD (28, 29). Moreover, depression has been shown to be related to objective changes to brain structure and function (30), including gray matter abnormalities within frontal-subcortical and limbic networks (31) and loss of white matter integrity (32). Together, these findings suggest that arbitrary exclusion of persons with depression from SCD studies can result in incomplete understanding of the mechanisms by which SCD subjects progress to cognitive decline and dementia.
Furthermore, like depression and anxiety, enhanced stress levels and neuroticism may accelerate AD pathological progression and cognitive decline (33, 34). Therefore, it is often difficult to make the clinical diagnosis or differential diagnosis of SCD for people with neuro-emotional comorbidity. They are more prone to be considered for an exclusionary diagnosis, in which comprehensive factors including age, gender, education, experience, emotional state, etc. are considered. Including such patients in SCD research would require quantification of the impact of depression and other psychiatric symptoms so that they can be adjusted as potential moderator variables (33, 34).
As mentioned above, SCD is characterized by self-experienced cognitive decline. Thus, informant confirmation has been speculated to increase the accuracy of diagnosis for preclinical AD spectrum. Many researchers also reckon that self- and informant-reports represent complementary approaches (33).
Neuropsychological Assessment
Mini-Mental State Examination (MMSE) and Montreal Cognitive Assessment (MoCA) are two widely used screening tools that evaluate overall cognitive function, with high sensitivity for detecting MCI and AD. However, for detection and assessment of SCD, their validations or alternatives are needed. Sahlgrenska Academy Self-Reposed Cognitive Impairment Questionnaire (SASCI-Q) has been used to distinguish SCD from normal cognitive population and to evaluate daily cognitive ability in several studies (37). The Memory Alteration Test (M@T) has been reported to have distinguished SCD from amnestic mild cognitive impairment (aMCI) and early AD with higher sensitivity and specificity in comparison with MMSE (37). However, M@T is not a comprehensive tool for evaluating the overall level of cognition (38, 39). The Subjective Cognitive Decline Questionnaire (SCD-Q) is a more informative test that can distinguish SCD patients from the normal cognition population (37, 40). The test places an emphasis on the concept of “cognitive-decline-as-chief-complaint in different sources.” The complementary confirmation of cognitive decline by both subjective and objective perception makes The Subjective Cognitive Decline Questionnaire (SCD-Q) a great screening tool in the diagnosis and prognosis of SCD (41). Other neuropsychological examination methods evaluated by different study groups include the Memory Complaint Questionnaire (MAC-Q) and Everyday Cognition (ECog) (42, 43). The most common cognitive impairment of SCD is episodic memory, followed by executive function. Researchers tried to screen SCD patients from elderly people with normal cognition using the Stroop Color Word Test (CWT) and found significant differences in the outcomes between the SCD and normal cognition individuals by using the CWT rather than general neuropsychological tests, which indicated that CWT could be used as an independent indicator of SCD in clinical management (44). Recently, Ismail et al. (45) developed the mild behavioral impairment checklist (MBI-C) questionnaire for SCD and MCI, with the aim to develop more accurate assessment tools for screening the clinical manifestations of patients at early-stage-AD. Some recent research indicated that neuropsychological assessment may aid clinical diagnosis, especially memory neuropsychological tests which may also be useful for evaluating the risk of progression to AD among SCD subjects (24, 46). However, most scales and evaluation methods are still in the development stage or in their initial testing stage. More large-scale, multi-center, and standardized studies are needed to determine the suitability for clinical application as well as unified screening tools.
Biomarkers
Valid SCD biomarkers may reflect the intermediate stage between clinically normal older adults (yet still with potential SCD) and MCI individuals, which is in line with how SCD is currently conceptualized. Any established associations between SCD and biomarkers would further validate SCD as a valid stage prior to the onset of clinical impairment along the early AD trajectory (33). In this section, biomarkers of cerebrospinal fluid (CSF) are emphatically introduced.
Biomarkers of CSF are also significant auxiliary diagnoses and predictors of SCD. Biomarkers in the CSF of SCD patients may occur well before any significant changes in brain MRI. The low level of Aβ protein in CSF indicates increased amyloid protein deposition in the brain, while high levels of T-tau and P-tau proteins suggest neurodegeneration. Leoni et al. (47) reported that as the cognitive function declined, T-tau and P-tau proteins in CSF of SCD patients increased significantly whereas Aβ protein decreased significantly. These findings are consistent with the 2011-National Institute on Aging and Alzheimer's Association (NIA-AA) diagnosis standard for SCD (48). Although changes in Aβ protein and tau protein in the CSF of SCD population could be a possible diagnostic basis for SCD, other potential independent causative factors, such as severe anxiety and depression, should be excluded. A 5-year follow-up study of 149 patients (94 MCI patients, 55 SCD patients) conducted by Sierra-Rio et al. (49) showed that during the follow-up period, the ratio of CSFAβ42/P-tau decreased in 72.4% of the patients (MCI83%, SCD27%). Because all of these patients progressed to AD eventually, these results suggest that the decreased Aβ42/P-tau ratio in CSF could be a critical risk factor for SCD/MCI progressing to AD. Compared to tau protein or other related predictors, Aβ42 could better predict the progression of SCD to MCI or to AD as proposed by Van Harten et al. (50). They suggested that Aβ42 level is a better predictor for clinical progression of SCD whereas T-tau is a predictor for MCI progression. Another cohort study conducted in Holland by the same research group found that SCD patients with initial abnormal CSF levels of Aβ42, T-tau, and P-tau have increased the risk of progression into MCI or AD within 2 years by 16, 2.8, and 2.6 times, respectively, compared to those with normal levels, suggesting that low level Aβ42 is a strong predictive factor for cognitive decline in SCD patients (50). Indeed, patients with detectable biomarkers are more likely to undergo cognitive decline during the course of SCD progression.
Jia et al. (51) found that the exosomal concentrations of Ab42, T-tau, and P-T181-tau in AD patients were higher than those in aMCI and control groups and the level of each exosomal biomarker was highly correlated with that in CSF. Their multicenter study with two-stage design verified the consistence between CSF and blood exosomal biomarkers and confirmed that exosomal Ab42, T-tau, and P-T181-tau have the same capacity as those in CSF for the diagnosis of AD and aMCI. This kind of peripheral blood will play a significant role in non-invasive detection at the early stage of AD. However, these findings, including CSF and peripheral blood, still need further confirmation in longitudinal studies.
Neuroimaging Examination
Many neuroimaging methods have been applied to diagnose SCD. Among them, Structural MRI (sMRI) can be applied to measure the volume and thickness of the patients' cortex (38). There is evidence to show that at SCD stage, the degree of the cortex atrophy is associated with the severity of cognitive impairment. Saykin et al. (52) reported that, compared to the normal population, the level of medial temporal lobes atrophy and frontal lobes atrophy of SCD patients is related to the degree of cognitive decline. Recent MRI studies also showed that people with MCI differed from very mild cognitive decline and normal controls in the right hippocampus volume (53, 54). People with low-volume right hippocampus and cognitive impairment are at greater risk of advancing to AD (53, 54). Overall, sMRI is expected to be a useful tool for diagnosis of SCD.
Functional MRI (fMRI) studies also show a weakened resting state in some of the brain regions of AD patients, such as the right hippocampus and insular subregions, indicating damage in these regions. Whereas, the increased activities in certain brain regions may suggest functional compensation in these areas at the early stage of AD (see Figure 1). Regarding SCD, the fMRI study by Ying's groups showed that (55), compared to the control group, there was a significant increase in the activity of the resting state in the following brain regions of SCD patients: inferior parietal lobule, right superior temporal gyrus, right inferior temporal gyrus, fusiform gyrus, and right posterior lobe of the cerebellum. This confirms the existence of compensatory mechanisms in spontaneous activities of SCD patients at the resting state. Another study suggested that patients with APOEε4 presented both increased and decreased functional connectivity as reflected in default mode network (DMN), which is correlated with clinical cognitive performances based on the regions of interest (ROI)-based functional connectivity analyses and voxel-based analyses (56). Thus, altered functional connectivity may be an early sign of cognitive decline. A resting-state fMRI study mainly revealed that SCD individuals had reduced correlations between centrality frequency (i.e., across the entire time window, the proportion of time that the hub appeared was defined as centrality frequency) of the anterior cortical regions and cognitive performance, compared with normal controls (NCs). In contrast, correlations between centrality frequency of the posterior cortical regions and cognitive performance were stronger in SCD participants than NCs. Besides, the alterations mainly concentrated on the anterior and posterior regions associated with the default mode network (DMN) (36). See Figure 2.
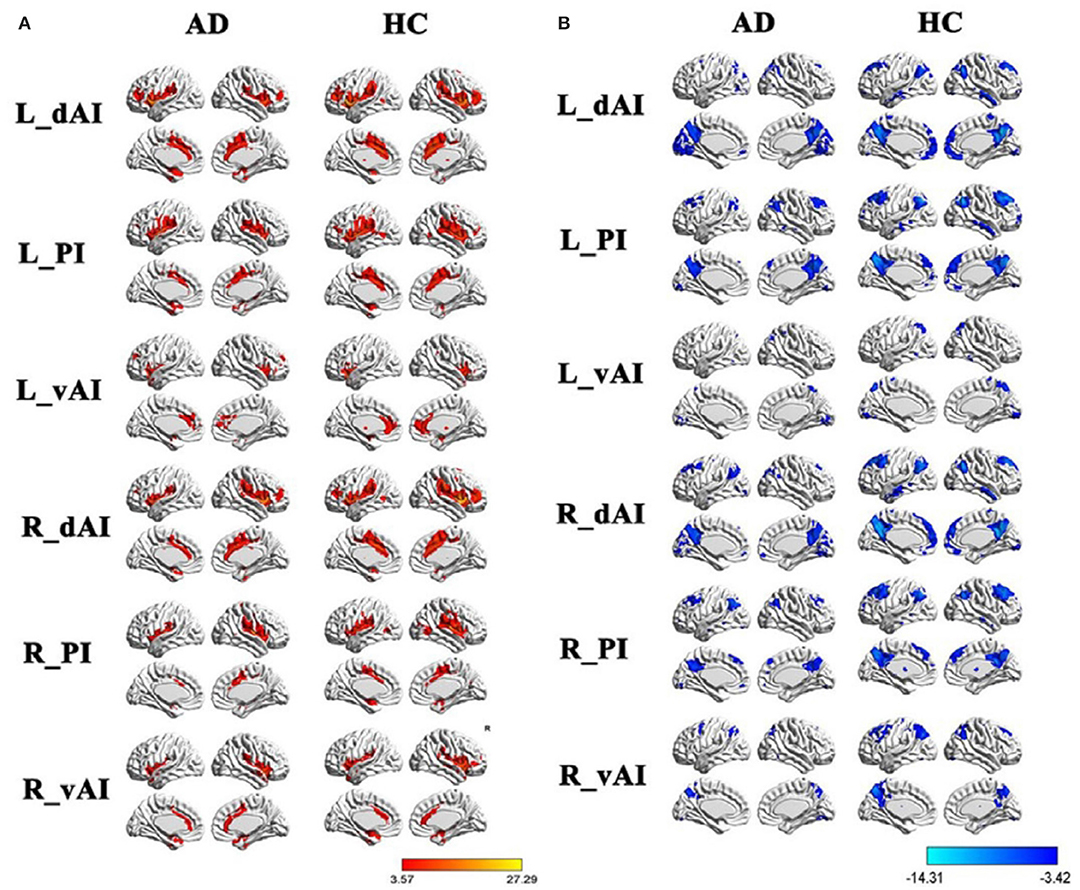
Figure 1. Within group resting-state functional connectivity (RSFC) analysis. (A) The positive RSFC patterns of the insular subregions in each group including healthy controls and AD patients. (B) The negative RSFC patterns of the insular subregions in each group including healthy controls and AD patients. PI, posterior insula; dAI, the dorsal anterior insula; vAI, the ventral anterior insula. Figure is reproduced from He et al. (35), with authors permission.
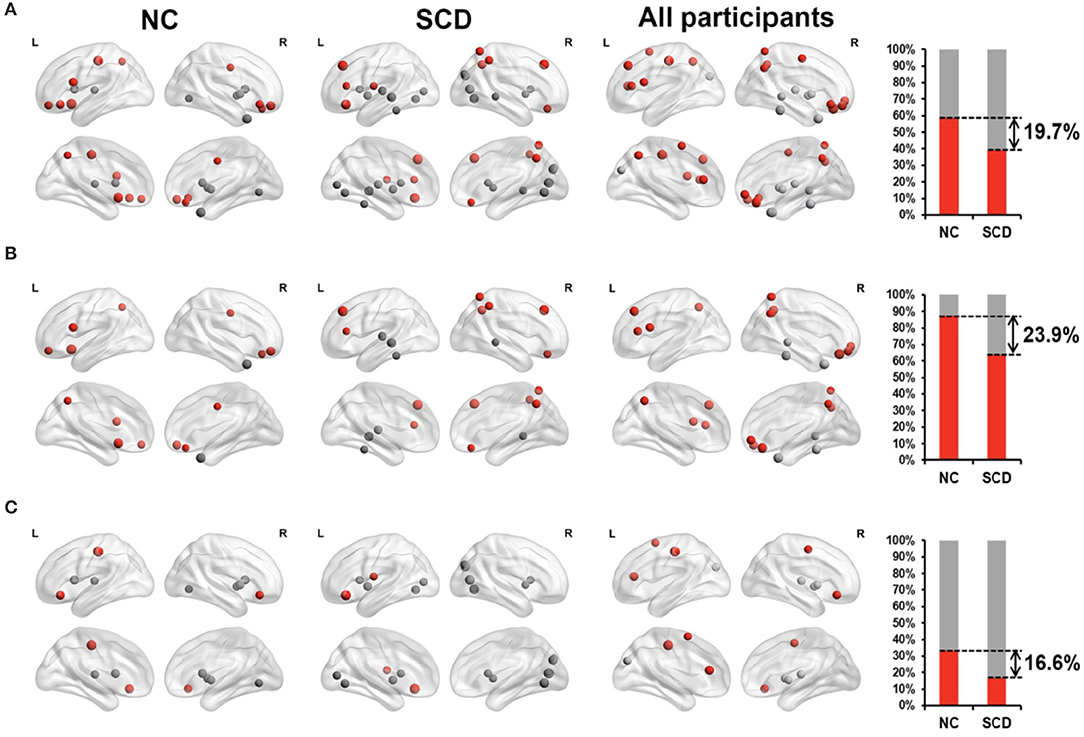
Figure 2. Relationship between degree of centrality (reflecting the dynamic functional connectivity) and neuropsychological tests in NC individuals (the first 2-columns), SCD patients (the second 2-columns), and all participants (the third 2-columns). The far-right column displayed the percentage of significantly correlated regions (p < 0.05) located in anterior (red) cortical and other (gray) cortical regions in the two groups. The nodes covered by red are located in anterior cortical regions while nodes covered by gray are located in posterior cortical regions or subcortical regions. (A) All the significant correlated regions (p < 0.05). (B) The regions in the DMN. (C) The regions out of the DMN. Figure is reproduced from Han et al. (36), with authors permission.
Glucose is an important energy source for brain function. Abnormal glucose uptake in different regions may be related to the extent of altered brain function. 18F-fluorodeoxyglucose position emission tomography (FDG-PET) can reflect changes in glucose metabolism in different parts of the brain (57). Scheef et al. (57) reported that, compared to the control group, metabolic rates in right precuneus and left parietal cortex are lower in SCD patients, while metabolic rates in medial temporal lobe and right para-hippocampal gyrus are higher, suggesting simultaneous abnormal energy metabolism and neuronal dysfunction in certain regions of SCD patients. And the decline in executive ability of SCD patients was associated with Aβ deposition in a Pittsburgh Compound B PET (PIB-PET) study (58). In recent years, breakthroughs in SCD research have been achieved by applying Flobetapir F-18 to Florbetapir-PET (amyloid-PET) techniques with improved sensitivity. Flobetapir F-18 has a higher affinity for Aβ accumulated in the brain and the results of Florbetapir-PET are highly consistent with that of Aβ in CSF that may be used as an early clinical diagnosis (59). Clark et al. (60), found an association between amyloid -PET imaging and the density of brain β amyloid protein. These findings show that PET can be an effective tool for detecting abnormalities in brains at SCD stage from function and Aβ deposit perspective.
Mallio et al. (61) demonstrated an epicentral disruption of structural connectivity in aMCI and AD around entorhinal and hippocampal regions, using diffusion-weighted imaging (DWI) consistent with the transneuronal spread hypothesis. Hereafter, researchers gradually paid attention to investigating brain network by Magnetic Resonance Diffusion Tensor Imaging (MR-DTI), and found that structural network properties might be preserved in patients with SCD but disrupted in aMCI stage, which may contribute to a better understanding of pathological mechanisms of AD (62). Li et al. (63) analyzed the DTI data in SCD patients by using Tract Based Spatial Statistics (TBSS), and found extensive white matter damage in SCD patients. These studies suggested that these pathological changes in SCD subjects were undetectable by conventional tests. Han et al. (64) also used DTI and graph theory approaches to demonstrate disrupted topologic efficiency of the brains' structural connectome of SCD. Thus, connectome-based biomarkers could be potentially used for detection of SCD in an elderly population. Another DTI study (65) revealed that rich club organization (some certain cortical regions highly connected to each other, with other regions referred to as peripheral) was destroyed, with less structural connectivity among rich club nodes in persons with MCI or AD but remained stable in SCD patients, which can show the development of AD and be viewed as a biomarker for early diagnosis. A recent systematic review including 16 studies (using neuroimaging tools containing Magnetoencephalography, structural and functional MRI, DTI, etc.) concluded that patients with SCD exhibited a significant abnormality of the brain network, compared to healthy controls, which was damaged in a similar approach as in Mild Cognitive Impairment (66). Magnetic Resonance Diffusion Kurtosis Imaging (DKI) is a relatively new technique that is an extension of DTI and is based on the non-Gauss-field of the water molecular diffusion. DKI can solve the problem of nerve-fibers-crossing and is more sensitive in observing subtle changes in brain white matter and gray matter (36, 56). Although only a few SCD studies reported using DKI, DKI will play an increasingly important role in the study of SCD in the future.
Together, comprehensive application of neuroimaging technology provides a valid way of capturing early brain alterations, and as such, these approaches may complement the absence of other neuropsychological tests and CSF biomarkers available for SCD diagnosis.
Prognosis of SCD
The development of SCD could follow any of these three directions: (1) remission and symptoms fade away, (2) stabilized, (3) become worse and progress to MCI or AD. The conversion rate of SCD to MCI and AD in diagnosed SCD was reported to be 54.2%, of which 78.9% progressed to MCI in a 7-year follow-up study (4). In comparison, the risk of developing MCI or AD in the normal group was 14.9%, of which 71.4% developed to MCI. After adjusting for confounding factors, the risk for SCD group to develop to MCI or AD within 7 years was 4.5 times greater than that of no-SCD group. A meta-analysis by Mitchell et al. (23), showed that the risk of progression to AD in the elderly with SCD was 2 times greater than the elderly without SCD, with the annual conversion rate of SCD to MCI and AD as 6.6 and 2.3%, respectively. Importantly, many studies also suggested that low CSF Aβ42 and high t-tau or p-tau may predict cognitive decline (progression to MCI) in SCD subjects (67).
Intervention
So far there is no FDA-approved pharmacologic interventions for SCD. A few supplements-based interventions have been reported. A 12-week dietary supplements treatment was conducted among 30 SCD patients (68), using active ingredients including Vinpocetine, uridine-5 monophosphate, hops, ginger, rosemary, Ashwaganda, grape seed, wild blueberry extract, L-alpha, Omega-glycerophosphate choline-3 phosphatidylserine, etc. The results showed significantly improved cognitive function in all subjects at the second week assessment, but no further improvement was found at the end of the study. Another report by Williams et al. (69) proposed that cognition training, vegetable intake, Mediterranean diet, Omega-3 fatty acid, physical activity, and non-physical leisure activities, when combined, could prevent cognitive decline. Among various nutritional markers related to aging and cognition, experts found that there may be an association between low-level Vitamin D and likelihood of functional deficits, such as coexisting or separate physical and cognitive decline in patients with subjective memory complaints (70). Researchers also found that a 4-week simultaneous memory training and aerobic exercise program can contribute to improving memory, reasoning abilities, and attention in a recent study (71). At the 2017 London Alzheimer's Association International Conference (AAIC), “Lancet” International Disease Prevention, Intervention and Nursing Committee released a new analysis system that identified nine modifiable risk factors, including <12 years of education, hypertension, obesity, hearing loss, depression, diabetes, lack of exercise, smoking, and social isolation. Most of these risk factors could be modified from childhood to middle age and account for 35% of AD. Using the stratified cluster random sampling method, Han et al. (19) investigated the prevalence and risk factors of SCD in Shun-Yi District of Beijing, China, and found high prevalence of SCD among people who were of an old age, had a low education, less social support, and daily drinking habits, highlighting these as independent risk factors. Because most of these factors are modifiable except age, the preservation and mobilization of brain plasticity by cognitive training, healthy diet, active aerobic exercise, smoking cessation, alcohol restriction, control of diseases such as diabetes, hypertension, dyslipidemia, anxiety and depression, enriched social activities, alone or combined, could be potential directions of intervention. Recently, numerous systematic reviews and meta-analyses (72) indicate that non-pharmacologic intervention (NPI) can be an effective intervention for SCD, particularly, cognitive interventions can benefit objective cognitive functioning, offset some normal age-related cognitive decline, support productive aging, and enhance quality of life for individuals who may otherwise develop MCI or dementia, such as mind-body exercise, especially Tai Chi, a well-known type of traditional Chinese martial arts (73). Some researchers also found that ketogenic diet may be another early non-pharmacologic intervention in AD (74). NPI could be more useful than medication due to its cost-effectiveness, lack of side effects, the fact that it can be readily adapted by a wide diversity of appropriately trained and experienced health professionals (72), and it is administrable before symptom onset (75). However, there is still a lack of high-quality research (such as randomization, blinding of participants and clinicians, use of “intention to treat” analysis for incomplete outcome data, etc.) in this direction (76).
Relevance to Traumatic Brain Injury-Induced Cognitive Deficits
The past decades have seen traumatic brain injury (TBI) rush into the forefront of neurology due to the increasing incidence of falls, motor vehicle accidents, sports injuries, and wars (77). TBI is defined as altered brain function or other evidence of brain pathology caused by an external force (jolt to the head, blow, or other such penetrating head injury) and, more importantly, has been increasingly recognized as a risk factor for cognitive decline, dementia, and AD (78). Numerous studies also showed that there was a linear relationship between TBI severity and cognitive consequences after TBI, more specifically, the greater the severity, the greater the outcomes. To date, some links and details of mechanisms by which TBI leads to cognitive impairment remains to be fully elucidated. However, it is believed that excitotoxicity mediated by an overproduction of excitatory neurotransmitters like glutamate extracellularly, is hypothesized to be the sentinel event after TBI (79). Neuroinflammation is also activated in response to TBI, and has both beneficial and hazardous consequences. Some of the released pro-inflammatory cytokines, anti-inflammatory cytokines, and chemokines may induce Aβ plaque deposition in the brain (80, 81). After TBI, some brain areas have been shown to suffer from hypoxic damage and secondary ischemia (82), which is involved in the pathogenesis of AD by accelerating the accumulation of Aβ and increasing the hyper-phosphorylation of tau, resulting in chronic neurodegeneration (82). Furthermore, a growing number of studies have focused on the cerebrovascular link between TBI and AD. Cerebrovascular outcomes of TBI include edema, hemorrhages, vasospasms, changes in cerebral blood flow (CBF), blood-brain barrier (BBB) breakdown, coagulopathy, and chronic inflammation (83). TBI is a trigger of neurodegeneration and is a useful model for studying certain pathological features of AD, such as Aβand tau deposition. Conversely, Aβ and tau release can lead to cerebrovascular injury, and their accumulation around cerebral micro-vessels has a deleterious chronic impact (84, 85). In addition, pericyte dysfunction and alterations in endothelial cell after TBI are contributors to the neuropathology and cognitive deficits. Indeed, TBI is intimately related to cognitive decline.
As mentioned above, patients who report a history of TBI are more likely to precede an onset of AD and enough data support some overlapping distributions of pathology between them. In each of these two disorders, the final common pathway into clinical symptomatology includes the malfunctioning and death of neurons. Therefore, it is considered that, for AD investigators, it might be better to pay attention to different research directions that potentially offer opportunities to outflank TBI rather than frontally assault, AD, because of failure of related clinical trials before, in more depth, TBI as a possible model could contribute to better understanding the puzzle of AD. Specifically, a TBI provides investigators with the opportunity to induce many of the shared neuropathologies by animal models for TBIs or study them in patients who have suffered a head injury. TBI also offers basic and clinical investigators a temporally condensed microenvironment potentially reflecting within days and weeks of neuropathological progressions that can be studied in AD animal models or patients only by cross-sectional sampling of at risk subjects chosen from a population spread across decades (78). And we speculate that some neuropathologies may be observed at early stages of cognitive impairment because head injuries can be viewed as a starting point. Also, we are supposed to focus on prevention at the early stage, SCD, due to disappointing outcomes of AD therapy (78, 86, 87). Thus, TBI may be a definite breakthrough-point for studies of SCD. Additionally, it is noteworthy that subjective cognitive decline complaints after TBI and post-traumatic stress disorder (PTSD) have frequently been reported (88, 89), however, high anxiety and low mood resulting from TBI and PTSD may significantly influence subjective cognitive function, especially subjective appraisal of memory without any objective decline. Thus, clinicians should take that into account when distinguishing between TBI-induced affective disorders (anxiety, depression, etc.) and AD-related SCD, especially in old patients (89). Overall, there are differences and interrelations between TBI-induced SCD and AD-related SCD, and TBI is a significant focus of dementia research, with both basic and clinical research.
A summary of recent studies of TBI and cognitive decline is listed in Table 1.
Conclusion
The concept of SCD could shift the ineffective late-stage diagnosis and ineffective treatment of AD into a more effective prophylactic strategy. To better explore the field of preclinical AD, several significant issues should be resolved. First, standard terminology and assessment practices should be unified and adopted. Second, due to SCD's heterogeneity and subjectivity, comprehensive assessment methods can enhance the predictive validity of SCD as a marker for preclinical AD, including neuroimaging, CSF, blood, informants' complaints, and so on. Third, intervention trail, especially well-designed NPI for patients with SCD, is still a hotspot. Last but not least, studies of TBI animal models or patients may contribute to better understanding of more complex mechanisms of SCD, resulting from many neuropathologies similar to AD. Consequently, large-cohort, multi-centered, and longitudinal studies are needed to achieve more breakthroughs in the field of cognitive impairment.
A summary of the main findings or conclusions for each section is listed in Table 2.
Author Contributions
TS wrote the first draft of this manuscript. YH and GX critically revised the manuscript. All authors read and approved the final manuscript.
Funding
This review was supported by: National Natural Science Foundation of China (61633018), The affiliated hospital and the second clinical medical college of the North Sichuan Medical University, Nanchong Central Hospital (internal research grants).
Conflict of Interest
The authors declare that the research was conducted in the absence of any commercial or financial relationships that could be construed as a potential conflict of interest.
Acknowledgments
We would like to thank Drs. Yong He and Zhiqun Wang for their permission of using the Figure 1 from their previously published paper. We would also like to thank the reviewers (Carlo Augusto Mallio and Oliver Peters) for their constructive comments on the manuscript.
References
1. Reisberg B. The global deterioration scale for assessment of primary degenerative dementia. Am J Psychiatry. (1982) 139:1136–9. doi: 10.1176/ajp.139.9.1136
2. Sperling RA, Aisen PS, Beckett LA, Bennett DA, Craft S, Fagan AM, et al. Toward defining the preclinical stages of Alzheimer's disease: recommendations from the national institute on aging-Alzheimer's association workgroups on diagnostic guidelines for Alzheimer's disease. Alzheimer's Dement. (2011) 7:280–92. doi: 10.1016/j.jalz.2011.03.003
3. Valech N, Mollica MA, Olives J, Tort A, Fortea J, Lleo A, et al. Informants' perception of subjective cognitive decline helps to discriminate preclinical Alzheimer's disease from normal aging. J Alzheimer's Dis. (2015) 48:S87–98. doi: 10.3233/JAD-150117
4. Reisberg B, Prichep L, Mosconi L, John ER, Glodzik-Sobanska L, Boksay I, et al. The pre–mild cognitive impairment, subjective cognitive impairment stage of Alzheimer's disease. Alzheimer's Dement J Alzheimer's Assoc. (2008) 4(Suppl. S1):S98–108. doi: 10.1016/j.jalz.2007.11.017
5. Mitchell AJ. Is it time to separate subjective cognitive complaints from the diagnosis of mild cognitive impairment? Age Ageing. (2008) 37:497–9. doi: 10.1093/ageing/afn147
6. Jessen F, Amariglio RE, Boxtel MV, Breteler M, Ceccaldi M, Gaël Chételat, et al. A conceptual framework for research on subjective cognitive decline in preclinical Alzheimer's disease. Alzheimer's Dement. (2014) 10:844–52. doi: 10.1016/j.jalz.2014.01.001
7. Stewart R. Subjective cognitive impairment. Curr Opin Psychiatry. (2012) 25:445–50. doi: 10.1097/YCO.0b013e3283586fd8
8. Jessen F, Wiese B, Bachmann C, Eifflaender-Gorfer S, Bickel H. Prediction of dementia by subjective memory impairment effects of severity and temporal association with cognitive impairment. Arch Gen Psychiatry. (2010) 67:414–22. doi: 10.1001/archgenpsychiatry.2010.30
9. Reisberg B, Shulman MB. Commentary on “a roadmap for the prevention of dementia ii: leon thal symposium 2008.” subjective cognitive impairment as an antecedent of Alzheimer's dementia: policy import. Alzheimer's Dement. (2009) 5:154–6. doi: 10.1016/j.jalz.2009.01.011
10. Crowe M, Andel R, Wadley V, Cook S, Ball K. Subjective cognitive function and decline among older adults with psychometrically defined amnestic MCI. Int J Geriatr Psychiatry. (2006) 21:1187–92. doi: 10.1002/gps.1639
11. Rodda JE, Dannhauser TM, Cutinha DJ, Shergill SS, Walker Z. Subjective cognitive impairment: increased prefrontal cortex activation compared to controls during an encoding task. Int J Geriatr Psychiatry. (2009) 24:865–74. doi: 10.1002/gps.2207
12. Amieva H, Le Goff M, Millet X, Orgogozo JM, Pérès K, Dartigues JF, et al. Prodromal Alzheimer's disease: successive emergence of the clinical symptoms. Ann Neurol. (2008) 64:492–8. doi: 10.1002/ana.21509
13. Sperling RA, Jack CR, Aisen PS. Testing the right target and right drug at the right stage. Sci Transl Med. (2011) 3:111cm33. doi: 10.1126/scitranslmed.3002609
14. Norden AGWV, Fick WF, Laat KFD, Uden IWMV, Leeuw FED. Subjective cognitive failures and hippocampal volume in elderly with white matter lesions. Neurology. (2008) 71:1152–9. doi: 10.1212/01.wnl.0000327564.44819.49
15. Paradise MB, Glozier NS, Naismith SL, Davenport TA, Hickie IB. Subjective memory complaints, vascular risk factors and psychological distress in the middle-aged: a cross-sectional study. BMC Psychiatry. (2011) 11:108. doi: 10.1186/1471-244X-11-108
16. Jonker C, Launer LJ, Hooijer C, Lindeboom J. Memory complaints and memory impairment in older individuals. J Am Geriatr Soc. (1996) 44:44–9. doi: 10.1111/j.1532-5415.1996.tb05636.x
17. Luck T, Roehr S, Jessen F, Villringer A, Angermeyer MC, Riedel-Heller SG, et al. Mortality in individuals with subjective cognitive decline: results of the leipzig longitudinal study of the aged (lEILA75+). J Alzheimer's Dis. (2015) 48:S33–42. doi: 10.3233/JAD-150090
18. Sánchez-Benavides G, Grau-Rivera O, Suárez-Calvet M, Minguillon C, Cacciaglia R, Gramunt N, et al. Brain and cognitive correlates of subjective cognitive decline-plus features in a population-based cohort. Alzheimer's Res Ther. (2018) 10:123. doi: 10.1186/s13195-018-0449-9
19. Hao L, Wang X, Zhang L, Xing Y, Guo Q, Hu X, et al. Prevalence, risk factors, and complaints screening tool exploration of subjective cognitive decline in a large cohort of the Chinese population. J Alzheimer's Dis. (2017) 60:371–88. doi: 10.3233/JAD-170347
20. Roehr S, Riedel-Heller SG, Kaduszkiewicz H, Wagner M, Fuchs A, Carolin VDL, et al. Is function in instrumental activities of daily living a useful feature in predicting Alzheimer's disease dementia in subjective cognitive decline? Int J Geriatr Psychiatry. (2018) 34:193–203. doi: 10.1002/gps.5010
21. Wolfsgruber S, Polcher A, Koppara A, Kleineidam L, Fr Lich L, Peters O, et al. (2017). Cerebrospinal fluid biomarkers and clinical progression in patients with subjective cognitive decline and mild cognitive impairment. J Alzheimer's Dis. 58:939–50. doi: 10.3233/JAD-161252
22. Wallin A, Nordlund A, Jonsson M, Lind K, Edman A, Gothlin M, et al. The gothenburg MCI study: design and distribution of Alzheimer's disease and subcortical vascular disease diagnoses from baseline to 6-year follow-up. J Cerebr Blood Flow Metab. (2015) 36:114–31. doi: 10.1038/jcbfm.2015.147
23. Mitchell AJ, Beaumont H, Ferguson D, Yadegarfar M, Stubbs B. Risk of dementia and mild cognitive impairment in older people with subjective memory complaints: meta-analysis. Acta Psychiatrica Scand. (2014) 130:439–51. doi: 10.1111/acps.12336
24. Bessi V, Mazzeo S, Padiglioni S, Piccini C, Nacmias B, Sorbi S, et al. From subjective cognitive decline to Alzheimer's disease: the predictive role of neuropsychological assessment, personality traits, and cognitive reserve. A 7-year follow-up study. J Alzheimer's Dis. (2018) 63:1523–35. doi: 10.3233/JAD-171180
25. Reid LM, Maclullich AMJ. Subjective memory complaints and cognitive impairment in older people. Dement Geriatr Cogn Disord. (2006) 22:471–85. doi: 10.1159/000096295
26. Rönnlund M, Sundström A, Adolfsson R, Nilsson LG. Subjective memory impairment in older adults predicts future dementia independent of baseline memory performance: evidence from the Betula prospective cohort study. Alzheimer's Dementia. (2015) 11:1385–92. doi: 10.1016/j.jalz.2014.11.006
27. Parnetti L, Chipi E, Salvadori N, D'Andrea K, Eusebi P. Prevalence and risk of progression of preclinical Alzheimer's disease stages: a systematic review and meta-analysis. Alzheimer's Res Ther. (2019) 11:7. doi: 10.1186/s13195-018-0459-7
28. Diniz BS, Butters MA, Albert SM, Dew MA, Reynolds CF. Late-life depression and risk of vascular dementia and Alzheimer's disease: systematic review and meta-analysis of community-based cohort studies. B J Psychiatry. (2013) 202:329–35. doi: 10.1192/bjp.bp.112.118307
29. Da Silva J, Goncalves-Pereira M, Xavier M, Mukaetova-Ladinska EB. Affective disorders and risk of developing dementia: systematic review. B J Psychiatry. (2013) 202:177–86. doi: 10.1192/bjp.bp.111.101931
30. Butters MA, Young JB, Lopez O, Aizenstein HJ, Becker JT. Pathways linking late-life depression to persistent cognitive impairment and dementia. Dial Clin Neurosci. (2008) 10:345–57. doi: 10.3389/fphar.2020.00279
31. Sexton CE, Mackay CE, Ebmeier KP. A systematic review and meta-analysis of magnetic resonance imaging studies in late-life depression. Am J Geriatr Psychiatry. (2013) 21:184–95. doi: 10.1016/j.jagp.2012.10.019
32. Allan CL, Sexton CE, Filippini N, Topiwala A, Mahmood A, Zsoldos E, et al. Sub-threshold depressive symptoms and brain structure: a magnetic resonance imaging study within the whitehall II cohort. J Affect Disord. (2016) 204:219–25. doi: 10.1016/j.jad.2016.06.049
33. Rabin LA, Smart CM, Amariglio RE. Subjective cognitive decline in preclinical Alzheimer's disease. Annu Rev Clin Psychol. (2017) 13:369. doi: 10.1146/annurev-clinpsy-032816-045136
34. Molinuevo JL, Rabin LA, Amariglio R, Buckley R, Dubois B, Ellis KA, et al. Implementation of subjective cognitive decline criteria in research studies. Alzheimer's Dement. (2017) 13:296–311. doi: 10.1016/j.jalz.2016.09.012
35. Liu X, Chen X, Zheng W, Xia M, Han Y, Song H, et al. Altered Functional Connectivity of Insular Subregions in Alzheimer's Disease. Front. Aging Neurosci. (2018) 10:107. doi: 10.3389/fnagi.2018.00107
36. Xie Y, Liu T, Ai J, Chen D, Zhuo Y, Zhao G, et al. Changes in centrality frequency of the default mode network in individuals with subjective cognitive decline. Front Aging Neurosci. (2019) 11:118. doi: 10.3389/fnagi.2019.00118
37. Eckerstrom M, Skoogh J, Rolstad S, Gothlin M, Steineck G, Johansson B, et al. Sahlgrenska academy self-reported cognitive impairment questionnaire (SASCI-Q) – a research tool discriminating between subjectively cognitively impaired patients and healthy controls. Int Psychogeriatr. (2013) 25:420–30. doi: 10.1017/S1041610212001846
38. Pan PL, Zhu L, Yu TT, Shi HC, Zhang B, Qin R, et al. Aberrant spontaneous low-frequency brain activity in amnestic mild cognitive impairment: a meta-analysis of resting-state FMRI studies. Ageing Res Rev. (2017) 35:12–21. doi: 10.1016/j.arr.2016.12.001
39. Rami L, Bosch B, Sanchez-Valle R, Molinuevo JL. The memory alteration test (M@T) discriminates between subjective memory complaints, mild cognitive impairment and Alzheimer's disease. Arch Gerontol Geriatr. (2010) 50:171–4. doi: 10.1016/j.archger.2009.03.005
40. Rami L, Mollica MA, García-Sanchez C, Saldaña J, Molinuevo JL. The subjective cognitive decline questionnaire (SCD-Q): a validation study. J Alzheimer's Dis. (2014) 41:453–66. doi: 10.3233/JAD-132027
41. Di Stefano F, Epelbaum S, Coley N, Cantet C, Ousset PJ, Hampel H, et al. Prediction of Alzheimer's disease dementia: data from the guidage prevention trial. J Alzheimer's Dis. (2015) 48:793–804. doi: 10.3233/JAD-150013
42. Vogel A, Salem LC, Andersen BB, Waldemar G. Differences in quantitative methods for measuring subjective cognitive decline: results from a prospective memory clinic study. Alzheimer's Dementia. (2016) 28:1513–20. doi: 10.1017/S1041610216000272
43. Farias ST, Mungas D, Reed BR, Cahn-Weiner D, Jagust W, Baynes K, et al. The measurement of everyday cognition (ECog): scale development and psychometric properties. Neuropsychology. (2008) 22:531–44. doi: 10.1037/0894-4105.22.4.531
44. Schroeter ML, Vogt B, Frisch S, Becker G, Barthel H, Mueller K, et al. Executive deficits are related to the inferior frontal junction in early dementia. Brain. (2012) 135:201–15. doi: 10.1093/brain/awr311
45. Ismail Z, Agüera-Ortiz L, Brodaty H, Cieslak A, Cummings J, Fischer CE, et al. The mild behavioral impairment checklist (MBI-C): a rating scale for neuropsychiatric symptoms in pre-dementia populations. J Alzheimer's Dis. (2017) 56:929–38. doi: 10.3233/JAD-160979
46. David DA, Prigatano GP. Subjective ratings of cognitive and emotional functioning in patients with mild cognitive impairment and patients with subjective memory complaints but normal cognitive functioning. J Clin Exp Neuropsychol. (2019) 41:565–75. doi: 10.1080/13803395.2019.1588229
47. Leoni V, Solomon A, Lövgren-Sandblom A, Minthon L, Björkhem I, et al. Diagnostic power of 24s-hydroxycholesterol in cerebrospinal fluid: candidate marker of brain health. J Alzheimer's Dis. (2013) 36:739–47. doi: 10.3233/JAD-130035
48. Meyer PF, Savard M, Poirier J, Morgan D, Breitner J. Hypothesis: cerebrospinal fluid protein markers suggest a pathway toward symptomatic resilience to AD pathology. Alzheimer's Dement. (2019) 15:1160–71. doi: 10.1016/j.jalz.2019.05.007
49. Sierra-Rio A, Balasa M, Olives J, Antonell A, Iranzo A, Castellvi M, et al. Cerebrospinal fluid biomarkers predict clinical evolution in patients with subjective cognitive decline and mild cognitive impairment. Neurodegener Dis. (2015) 16:69–76. doi: 10.1159/000439258
50. Van Harten AC, Visser PJ, Pijnenburg YAL, Teunissen CE, Blankenstein MA, Scheltens P, et al. Cerebrospinal fluid aβ42 is the best predictor of clinical progression in patients with subjective complaints. Alzheimer's Dementia. (2013) 9:481–7. doi: 10.1016/j.jalz.2012.08.004
51. Jia L, Qiu Q, Zhang H, Chu L, Zhang J, Zhou C, et al. Concordance between the assessment of Abeta42, T-tau, and P-T181-tau in peripheral blood neuronal-derived exosomes and cerebrospinal fluid. Alzheimer's Dement. (2019) 15:1071–80. doi: 10.1016/j.jalz.2019.05.002
52. Saykin AJ, Wishart HA, Rabin LA, Santulli RB, Flashman LA, West JD, et al. Older adults with cognitive complaints show brain atrophy similar to that of amnestic MCI. Neurology. (2006) 67:834–42. doi: 10.1212/01.wnl.0000234032.77541.a2
53. Peter J, Scheef L, Abdulkadir A, Boecker H, Heneka M, Wagner M, et al. Gray matter atrophy pattern in elderly with subjective memory impairment. Alzheimer's Dementia. (2014) 10:99–108. doi: 10.1016/j.jalz.2013.05.1764
54. Perrotin A, De Flores R, Lamberton F, Poisnel G, La Joie R, de la Sayette V, et al. Hippocampal subfield volumetry and 3D surface mapping in subjective cognitive decline. J Alzheimer's Dis. (2015) 48:S141–50. doi: 10.3233/JAD-150087
55. Ying H, Su L, Weihong K, Qi L, Ling Z, Jianping J, et al. Anatomical and functional deficits in patients with amnestic mild cognitive impairment. PloS ONE. (2012) 7:e28664. doi: 10.1371/journal.pone.0028664
56. Haiqing S, Haixia L, Xiumei Z, Chunshui Y, Bing L, Zhiqun W, et al. APEO effects on default mode network in Chinese cognitive normal elderly: relationship with clinical cognitive performance. PloS ONE. (2015) 10:e0133179. doi: 10.1371/journal.pone.0133179
57. Wagner M, Jessen F, Scheef L. Glucose metabolism, gray matter structure and memory decline in subjective memory impairment. Alzheimers Dement. (2012). 8:P259. doi: 10.1212/WNL.0b013e31826c1a8d
58. Amariglio RE, Becker JA, Carmasin J, Wadsworth LP, Lorius N, Sullivan C, et al. Subjective cognitive complaints and amyloid burden in cognitively normal older individuals. Neuropsychologia. (2012) 50:2880–6. doi: 10.1016/j.neuropsychologia.2012.08.011
59. Camus V, Payoux P, Barré L, Desgranges B, Voisin T, Guilloteau D, et al. Using PET with 18F-AV-45 (florbetapir) to quantify brain amyloid load in a clinical environment. Eur J Nucl Med Mol Imaging. (2012) 39:621–31. doi: 10.1007/s00259-011-2021-8
60. Clark CM, Pontecorvo MJ, Beach TG, Bedell BJ, Coleman RE, Doraiswamy PM, et al. Cerebral PET with florbetapir compared with neuropathology at autopsy for detection of neuritic amyloid-β plaques: a prospective cohort study. Lancet Neurol. (2012) 11:669–78. doi: 10.1016/S1474-4422(12)70142-4
61. Mallio CA, Schmidt R, De Reus MA, Vernieri F, Quintiliani L, Curcio G, et al. Epicentral disruption of structural connectivity in Alzheimer's disease. CNS Neurosci Ther. (2015) 21:837–45. doi: 10.1111/cns.12397
62. Wang XN, Zeng Y, Chen GQ, Zhang YH, Li XY, Hao XY, et al. Abnormal organization of white matter networks in patients with subjective cognitive decline and mild cognitive impairment. Oncotarget. (2016) 7:48953–62. doi: 10.18632/oncotarget.10601
63. Li H-Y, Han Y, Tang Z. Tract-based spatial statistics analysis of white matter changes in subjects with subjective cognitive decline. J China Clinic Med Imaging. (2015) 12:1140. doi: 10.1016/j.jalz.2016.07.023
64. Shu N, Wang X, Bi Q, Zhao T, Han Y. Disrupted topologic efficiency of white matter structural connectome, in individuals with subjective cognitive decline. Radiology. (2017) 286:229–38. doi: 10.1148/radiol.2017162696
65. Tianyi Y, Wenhui W, Liu Y, Kewei C, Rong C, Ying H. Rich club disturbances of the human connectome from subjective cognitive decline to Alzheimer's disease. Theranostics. (2018) 8:3237–55. doi: 10.7150/thno.23772
66. Lazarou I, Nikolopoulos S, Dimitriadis SI, Kompatsiaris IY, Spilioti M, Tsolaki M. Is brain connectome research the future frontier for subjective cognitive decline? A systematic review. Clin Neurophysiol. (2019) 130:1762–80. doi: 10.1016/j.clinph.2019.07.004
67. Adalberto SN, Ricardo N. Subjective cognitive decline: the first clinical manifestation of Alzheimer's disease? Dementia Neuropsychol. (2016) 10:170–7. doi: 10.1590/S1980-5764-2016DN1003002
68. Richter Y, Herzog Y, Eyal I, Cohen T. Cognitex supplementation in elderly adults with memory complaints: an uncontrolled open label trial. J Diet Suppl. (2011) 8:158–68. doi: 10.3109/19390211.2011.569514
69. Williams JW, Plassman BL, Burke J, Holsinger T, Benjamin S. Preventing Alzheimer's Disease and Cognitive Decline. Evidence Reports/Technology Assessments, No. 193. Rockville, MD: Agency for Healthcare Research and Quality (2010). doi: 10.7326/0003-4819-154-3-201102010-00015
70. Chhetri JK, De SBP, Soriano G, Gennero I, Cantet C, Vellas B. Vitamin D, homocysteine and n-3PUFA status according to physical and cognitive functions in older adults with subjective memory complaint: results from cross-sectional study of the MAPT trial. Exp Gerontol. (2018) 111:71–7. doi: 10.1016/j.exger.2018.07.006
71. McEwen SC, Siddarth P, Rahi B, Kim Y, Mui W, Wu P, et al. Simultaneous aerobic exercise and memory training program in older adults with subjective memory impairments. J Alzheimer's Dis. (2018) 62:795–806. doi: 10.3233/JAD-170846
72. Smart CM, Karr JE, Areshenkoff CN, Rabin LA, Hudon C, Gates N, et al. Non-pharmacologic interventions for older adults with subjective cognitive decline: systematic review, meta-analysis, and preliminary recommendations. Neuropsychol Rev. (2017) 27:245–57. doi: 10.1007/s11065-017-9342-8
73. Lyu J, Li W, Rong X, Wei L, Huang N, Champ M, et al. Efficacy of practising Tai Chi for older people with mild dementia: protocol for a randomised controlled study. BMJ Open. (2018) 8:e019940. doi: 10.1136/bmjopen-2017-019940
74. Taylor MK, Sullivan DK, Mahnken JD, Burns JM, Swerdlow RH. Feasibility and efficacy data from a ketogenic diet intervention in Alzheimer's disease. Alzheimer's Dementia. (2017) 4:28–36. doi: 10.1016/j.trci.2017.11.002
75. Doniger GM, Beeri MS, Bahar-Fuchs A, Gottlieb A, Tkachov A, Kenan H, et al. Virtual reality–based cognitive-motor training for middle-aged adults at high Alzheimer's disease risk: a randomized controlled trial. Alzheimer's Dementia. (2018) 4:118–29. doi: 10.1016/j.trci.2018.02.005
76. Rohan B, Berry AJ, Huntley JD, Howard RJ. Interventions for subjective cognitive decline: systematic review and meta-analysis. BMJ Open. (2018) 8:e021610. doi: 10.1136/bmjopen-2018-021610
77. Flanagan SR. Invited Commentary on “Centers for disease control and prevention report to congress: traumatic brain injury in the united states: epidemiology and rehabilitation”. Arch Phys Med Rehabil. (2015) 96:1753–5. doi: 10.1016/j.apmr.2015.07.001
78. Becker RE, Kapogiannis D, Greig NH. Does traumatic brain injury hold the key to the Alzheimer's puzzle? Alzheimer's Dementia. (2017) 14:431–43. doi: 10.1016/j.jalz.2017.11.007
79. Stocchetti N, Zanier ER. Chronic impact of traumatic brain injury on outcome and quality of life: a narrative review. Crit Care. (2016) 20:148. doi: 10.1186/s13054-016-1318-1
80. Ziebell JM, Morganti-Kossmann MC. Involvement of pro- and anti-inflammatory cytokines and chemokines in the pathophysiology of traumatic brain injury. Neurotherapeutics. (2010) 7:22–30. doi: 10.1016/j.nurt.2009.10.016
81. Breunig JJ, Marie-Victoire GS, Terrence T. Brain injury, neuroinflammation and Alzheimer's disease. Front Aging Neurosci. (2013) 5:26. doi: 10.3389/fnagi.2013.00026
82. Huang RQ, Cheng HL, Zhao XD, Dai W, Shi JX. Preliminary study on the effect of trauma-induced secondary cellular hypoxia in brain injury. Neurosci Lett. (2010) 473:22–7. doi: 10.1016/j.neulet.2010.02.011
83. Salehi A, Zhang JH, Obenaus A. Response of the cerebral vasculature following traumatic brain injury. J Cereb Blood Flow Metabo. (2017) 37:2320–39. doi: 10.1177/0271678X17701460
84. Ramos-Cejudo J, Wisniewski T, Marmar C, Zetterberg H, Blennow K, De Leon MJ, et al. Traumatic brain injury and Alzheimer's disease: the cerebrovascular link. Ebiomedicine. (2018) 28:21–30. doi: 10.1016/j.ebiom.2018.01.021
85. Hay JR, Johnson VE, Young AM, Stewart W. Blood-brain barrier disruption is an early event that may persist for many years after traumatic brain injury in humans. J Neuropathol Exp Neurol. (2015) 74:1147–57. doi: 10.1093/jnen/74.12.1147
86. Becker RE, Greig NH, Giacobini E, Schneider LS, Ferrucci L. A new roadmap for drug development for Alzheimer's disease. Nat Rev Drug Discov. (2013) 13:156. doi: 10.1038/nrd3842-c2
87. Becker RE, Greig NH. A new regulatory road-map for Alzheimer's disease drug development. Curr Alzheimer Res. (2014) 11:215. doi: 10.2174/156720501103140329210642
88. Byrne C, Coetzer R, Addy K. Investigating the discrepancy between subjective and objective cognitive impairment following acquired brain injury: the role of psychological affect. Neurorehabilitation. (2017) 41:501–12. doi: 10.3233/NRE-162015
89. Jamora CW, Young A, Ruff RM. Comparison of subjective cognitive complaints with neuropsychological tests in individuals with mild vs. more severe traumatic brain injuries. Brain Injury. (2012) 26:36–47. doi: 10.3109/02699052.2011.635352
90. Chen C-C, Wei S-T, Tsaia S-C, Chen X-X, Cho D-Y. Cerebrolysin enhances cognitive recovery of mild traumatic brain injury patients: double-blind, placebocontrolled, randomized study. Br J Neurosurg. (2013). 27:803–7. doi: 10.3109/02688697.2013.793287
91. Covassin T, Elbin RJ, Harris W, Parker T, Kontos A. The role of age and sex in symptoms, neurocognitive performance, and postural stability in athletes after concussion. Am J Sports Med. (2012) 40:1303–12. doi: 10.1177/0363546512444554
92. Dikmen S, Machamer J, Temkin N. Mild traumatic brain injury: longitudinal study of cognition, functional status, and post-traumatic symptoms. J Neurotrauma (2016) 7. doi: 10.1089/neu.2016.4618
93. Failla MD, Myrga JM, Ricker JH, Edward Dixon C, Conley YP, Wagner AK. Posttraumatic brain injury cognitive performance is moderated by variation within ANKK1 and DRD2 Genes. J Head Trauma Rehabil. (2015) 30:E54–66. doi: 10.1097/HTR.0000000000000118
94. Farbota KD, Bendlin BB, Alexander AL, Rowley HA, Dempsey RJ, Johnson SC. Longitudinal diffusion tensor imaging and neuropsychological correlates in traumatic brain injury patients. Front Hum Neurosci. (2012) 6. doi: 10.3389/fnhum.2012.00160
95. Kontos AP, Elbin RJ, Kotwal RS, Lutz RH, Kane S, Benson PJ, et al. The effects of combat-related mild traumatic brain injury (mTBI): does blast mTBI history matter? J Trauma Acute Care Surg. (2015) 79:S146–51. doi: 10.1097/TA.0000000000000667
96. Meier TB, Bellgowan PSF, Singh R, Kuplicki R, Polanski DW, Mayer AR. Recovery of cerebral blood flow following sports-related concussion. JAMA Neurol. (2015) 72:530. doi: 10.1001/jamaneurol.2014.4778
97. Ponsford J, Cameron P, Fitzgerald M, Grant M, Mikocka-Walus A. Long-term outcomes after uncomplicated mild traumatic brain injury: a comparison with trauma controls. J Neurotrauma. (2011) 28:937–46. doi: 10.1089/neu.2010.1516
98. Register-Mihalik JK, Guskiewicz KM, Mihalik JP, Schmidt JD, Kerr ZY, McCrea MA. Reliable change, sensitivity, and specificity of a multidimensional concussion assessment battery: implications for caution in clinical practice. J Head Trauma Rehabil. (2013) 28:274–83. doi: 10.1097/HTR.0b013e3182585d37
99. Robertson K, Schmitter-Edgecombe M. Self-awareness and traumatic brain injury outcome. Brain Inj. (2015) 29:848–58. doi: 10.3109/02699052.2015.1005135
100. Sandhaug M, Andelic N, Langhammer B, Mygland A. Functional level during the first 2 years after moderate and severe traumatic brain injury. Brain Inj. (2015) 29:1431–8. doi: 10.3109/02699052.2015.1063692
101. Schmitter-Edgecombe M, Seelye AM. Recovery of content and temporal order memory for performed activities following moderate to severe traumatic brain injury. J Clin Exp Neuropsychol. (2012) 34:256–68. doi: 10.1080/13803395.2011.633497
102. Sours C, Rosenberg J, Kane R, Roys S, Zhuo J, Shanmuganathan K, et al. Associations between interhemispheric functional connectivity and the Automated Neuropsychological Assessment Metrics (ANAM) in civilian mild TBI. Brain Imaging Behav. (2015) 9:190–203. doi: 10.1007/s11682-014-9295-y
103. Vanderploeg RD, Donnell AJ, Belanger HG, Curtiss G. Consolidation deficits in traumatic brain injury: the core and residual verbal memory defect. J. Clin. Exp. Neuropsychol. (2014) 36:58–73. doi: 10.1080/13803395.2013.864600
104. Veeramuthu V, Narayanan V, Ramli N, Hernowo A, Waran V, Bondi MW, et al. Neuropsychological outcomes in patients with complicated versus uncomplicated mild traumatic brain injury: 6-Month follow-up. World Neurosurg. (2017) 97:416–23. doi: 10.1016/j.wneu.2016.10.041
105. Wang S, Cheng H, Dai G, Wang X, Hua R, Liu X, et al. Umbilical cord mesenchymal stem cell transplantation significantly improves neurological function in patients with sequelae of traumatic brain injury. Brain Res. (2013) 1532:76–84. doi: 10.1016/j.brainres.2013.08.001
106. Wylie GR, Freeman K, Thomas A, Shpaner M, OKeefe M, Watts R, et al. Cognitive improvement after mild traumatic brain injury measured with functional neuroimaging during the acute period. PLoS One (2015) 10. doi: 10.1371/journal.pone.0126110
Keywords: subjective cognitive decline, mild cognitive impairment, Alzheimer's disease, traumatic brain injury, diagnosis, intervention
Citation: Si T, Xing G and Han Y (2020) Subjective Cognitive Decline and Related Cognitive Deficits. Front. Neurol. 11:247. doi: 10.3389/fneur.2020.00247
Received: 22 August 2019; Accepted: 13 March 2020;
Published: 19 May 2020.
Edited by:
Tony L. Strickland, Sports Concussion Institute, United StatesReviewed by:
Carlo Augusto Mallio, Campus Bio-Medico University, ItalyOliver Peters, Charité – Universitätsmedizin Berlin, Germany
Copyright © 2020 Si, Xing and Han. This is an open-access article distributed under the terms of the Creative Commons Attribution License (CC BY). The use, distribution or reproduction in other forums is permitted, provided the original author(s) and the copyright owner(s) are credited and that the original publication in this journal is cited, in accordance with accepted academic practice. No use, distribution or reproduction is permitted which does not comply with these terms.
*Correspondence: Guoqiang Xing, gxing99@yahoo.com; Ying Han, hanying@xwh.ccmu.edu.cn