- 1Department of Psychology, Faculty of Science and Technology, Lancaster University, Lancaster, United Kingdom
- 2School of Health and Life Sciences, Sport and Physical Activity Research Institute, University of the West of Scotland, Glasgow, United Kingdom
- 3Manchester Centre for Audiology and Deafness, School of Health Sciences, University of Manchester, Manchester, United Kingdom
This meta-analysis investigated the association between age-related hearing loss and structural neuroanatomy, specifically changes to gray matter volume. Hearing loss is associated with increased risk of cognitive decline. Hence, understanding the effects of hearing loss in older age on brain health is essential. We reviewed studies which compared older participants with hearing loss (age-related hearing loss: ARHL) to older adults without clinical hearing loss (no-ARHL), on neuroanatomical outcomes, specifically gray matter (GM) volume as measured by magnetic resonance imaging. A total of five studies met the inclusion criteria, three of which were included in an analysis of whole-brain gray matter volume (ARHL group n = 113; no-ARHL group n = 138), and three were included in analyses of lobe-wise gray matter volume (ARHL group n = 139; no-ARHL group n = 162). Effect-size seed-based d mapping software was employed for whole-brain and lobe-wise analysis of gray matter volume. The analysis indicated there was no significant difference between adults with ARHL compared to those with no-ARHL in whole-brain gray matter volume. Due to lacking stereotactic coordinates, the level of gray matter in specific neuroanatomical locations could only be observed at lobe-level. These data indicate that adults with ARHL show increased gray matter atrophy in the temporal lobe only (not in occipital, parietal, or frontal), compared to adults with no-ARHL. The implications for theoretical frameworks of the hearing loss and cognitive decline relationship are discussed in relation to the results. This meta-analysis was pre-registered on PROSPERO (CRD42021265375).
Systematic Review Registration: https://www.crd.york.ac.uk/prospero/display_record.php?RecordID=265375, PROSPERO CRD42021265375.
Introduction
The population is aging, meaning that health issues which affect older adults become more prevalent (1), impacting on the older population's quality of life and placing increasing pressure on health care services. Two such health concerns are hearing loss and dementia. In the UK around 70% of people aged 70+ experience hearing loss (2), and around 7.1% of over 65's, rising to 14% of those over 80, are living with dementia (3). Critically, there is evidence that these health concerns may be associated. Hearing loss has been identified as the largest potentially modifiable risk factor for dementia (4). Hearing loss could account for as much as 8% of global dementia cases (5). It is likely that many risk factors are associated, and exacerbate one another leading to increased risk of dementia in certain individuals. Considering this, understanding the neural mechanisms of hearing loss, and how they may contribute to the association between hearing loss and dementia, is a priority.
Age-related hearing loss (ARHL) is often caused by degeneration of the inner and outer hair cells within the cochlea. These cells are responsible for the transduction of sound, and their atrophy can manifest in high-frequency hearing loss (6). Age-related atrophies in the peripheral auditory system can also be observed in the stria vascularis, a cochlea structure responsible for maintaining metabolic processes (7), or in degeneration of spiral ganglion cells, the initial neurons in the pathway from the ear to the brain (8). Importantly, evidence suggests that changes and atrophies in people with ARHL do not end at the peripheral auditory system, but are also evident in the auditory pathway and auditory cortex (9, 10). Understanding the cortical changes, in auditory areas and beyond, would provide valuable insights into how the brain changes in people with ARHL, and provide evidence for the mechanisms that underpin the association between hearing loss and cognitive decline.
A number of potential explanations for the relation between hearing loss and dementia have been proposed. These include non-causal hypotheses such as: (1) The common cause hypothesis, which suggests that rather than hearing loss leading to dementia, there is a common neuro-degenerative pathology which underlies both conditions such as general aging or vascular disease (11, 12); or (2) The hearing bias in cognitive assessment hypothesis, which suggests that there may be an overestimation of the link between hearing loss and dementia, because untreated hearing loss could be a significant confound in clinical cognitive assessments that rely on auditory presentation (13, 14). However, the relation between hearing loss and cognitive decline remains after controlling for age and vascular factors (15, 16), and hearing loss has been found to be associated with poorer cognitive functioning even when the cognitive assessments do not rely on verbal communication (17). As such, a causal mechanism may be more likely. Causal hypotheses include: (1) The cognitive load (or information degradation) hypothesis, which theorizes that people with ARHL are required to use more cognitive resources for speech perception leaving fewer available for general cognitive processes, which could lead to the symptoms of dementia (18); and (2) The sensory deprivation hypothesis, which postulates that reduced sensory input from the ear leads to reduced neural activation, cortical re-organization, and atrophy across brain areas involved with speech perception (10, 19). Both these causal hypotheses make suggestions with regards to functional or structural neuro-cognitive changes that might accompany ARHL, including upregulation or reorganization of neural resources (20) or atrophy across speech perception networks [see (21) for a discussion of cortical changes]. As such, a comprehensive review of the current literature on the neural consequences of ARHL is required to generate evidence to refute or support these causal hypotheses.
The first step in the systematic examination of neural consequences of ARHL is to assess the evidence for tangible neuroanatomical changes, in both auditory and wider cortices. There is evidence from longitudinal studies that individuals with ARHL display accelerated gray matter (GM) atrophy in auditory cortex compared to individuals without ARHL (22), however these group differences have not always reached statistical significance (23). In cross-sectional studies, there is also evidence for decreases in whole brain volume in those with ARHL compared to those without (10), and in specific brain areas associated with speech perception including the anterior cingulate cortex (24). The mechanism by which ARHL leads to wider brain atrophy is unclear. One potential explanation is that over-reliance on wider brain networks involved in speech perception due to impaired auditory processing contributes to neural degeneration of these areas. There is evidence that individuals with ARHL, compared to those without, display increased functional connectivity across auditory and visual sensory cortices (25), and between auditory cortex and the cingulo-opercular network after controlling for both age and cognitive function (26). The over-reliance on these additional brain networks to support speech perception in individuals with ARHL could enable neural degeneration due to glutamate excitotoxicity (24). Through this mechanism, the neurons across the up-regulated brain networks may die due to prolonged activation of glutamate receptors beyond their natural capacity (27).
Despite evidence for potential up-regulation and cortical atrophy, it is still unclear as to whether or not ARHL exacerbates the cortical changes observed in aging. Heterogeneity in research methods, such as differences in participant age ranges, imaging techniques, or clinical definitions of hearing loss, as well as small sample sizes, can lead to ambiguity in interpretation of the results. This meta-analysis will deliver a systematic and specific analysis of the existing literature on neuroanatomical changes in ARHL, controlling for some of these confounds through appropriate inclusion criteria, and study quality assessment. We sought to investigate across cross-sectional and longitudinal evidence whether GM volume, as measured by MRI, differs in adults aged ≥60 years with ARHL compared to those without ARHL. In this paper, ARHL is defined by hearing thresholds above 25 dB HL for frequencies between 500 and 2,000 Hz in adults aged 60+, whereas “without ARHL” is defined by hearing thresholds below 25 dB HL for frequencies between 500 and 2,000 Hz in adults aged 60+, representing age-appropriate hearing function. It was hypothesized that we would observe (1) decreased whole brain GM volume, as well as (2) decreases in GM volume in the temporal lobe, in individuals with ARHL compared to those without ARHL.
Methods
This meta-analysis was pre-registered on PROSPERO (PROSPERO 2021, CRD42021265375), available from: https://www.crd.york.ac.uk/prospero/display_record.php?ID=CRD42021265375) Additionally, all materials including: search strings; references obtained at all screening stages; screening manuals and inter-rater consistency data; extracted data; and analysis files can be found in the project's repository on the Open Science Framework (OSF): https://osf.io/g5qcb/.
Literature search
An initial pilot search was conducted on PubMed and Prospero according to best practice guidance (28, 29), in order to: (1) confirm whether systematic reviews and meta-analyses on this topic already existed; (2) estimate the feasibility of the meta-analysis and availability of data; and (3) identify key papers to inform the selection of appropriate key words and criteria for the final search string. Unlike the full literature search, the pilot search was characterized by iterative searching without pre-defined search strings. In-depth engagement with the literature might introduce potential bias in the construction of the full literature search. Hence, engagement with the pilot search was limited to 2 h.
Subsequently, the full literature search was conducted following PRISMA guidelines (30) and best practice guidelines from the “Cochrane Highly Sensitive Search Strategy” (31). Nine databases were searched (Table 1). To maximize effective retrieval of relevant papers, the research question was approached, inter alia, from medical (PubMed), nursing (CINAHL), and psychological (PsycINFO) perspectives. To ensure comprehensiveness, searches were not filtered in any way, e.g., by database internal filters, such as publication date, or by full-text articles (see Table 1 for exception in Scopus). If any full text could not be accessed the research team planned to contact the authors of the papers, and allow 1 week for an initial response before re-contacting. A total of 2 weeks was granted for authors to respond and provide access to papers.
Search strings (see Supplementary materials 1 for full details) were constructed using keywords, free-text terms, and search functions (Boolean operators, near searches, truncation, wild card symbols, quotations), to ensure specificity and sensitivity across databases. An example of the search terms included: “hearing loss” or “hearing impairment” or “presbycusis,” and “voxel-based” or “morphometry” or “magnetic resonance imaging” or “cortical thickness” or “gray matter,” and “older adult.” The final search strings, selected keywords, and Boolean operators were reviewed by a librarian to ensure adherence to best practice insights. To manage resource and time constraints, the search was limited to titles and abstracts.
Prior to conducting the literature search, a strategy test of sensitivity was completed in which four key papers that satisfied the inclusion criteria were identified using a database not used in the final search to avoid bias (Google Scholar). The sensitivity of the search strings was evaluated by testing how many of these four key papers indexed in the selected databases (Table 1) could be retrieved. Once the search sensitivity was acceptable, the literature search was conducted across the selected databases. All key papers were retrieved with the search indicating high likelihood that the search would successfully identify relevant articles.
Article screening
Articles (n = 14,078) retrieved from the literature search were imported to the reference manager software CADIMA [https://www.cadima.info/; for a review, see (32)]. An overview of the articles retained at each stage of the screening process can be found in the PRISMA flow diagram presented in Figure 1 (33). Any duplicated articles, retrieved by more than one database, were removed in a two-step process: (1) automatic de-duplication based on congruity in authors, title, and year of publication; and (2) manual de-duplication by two raters based on abstracts.
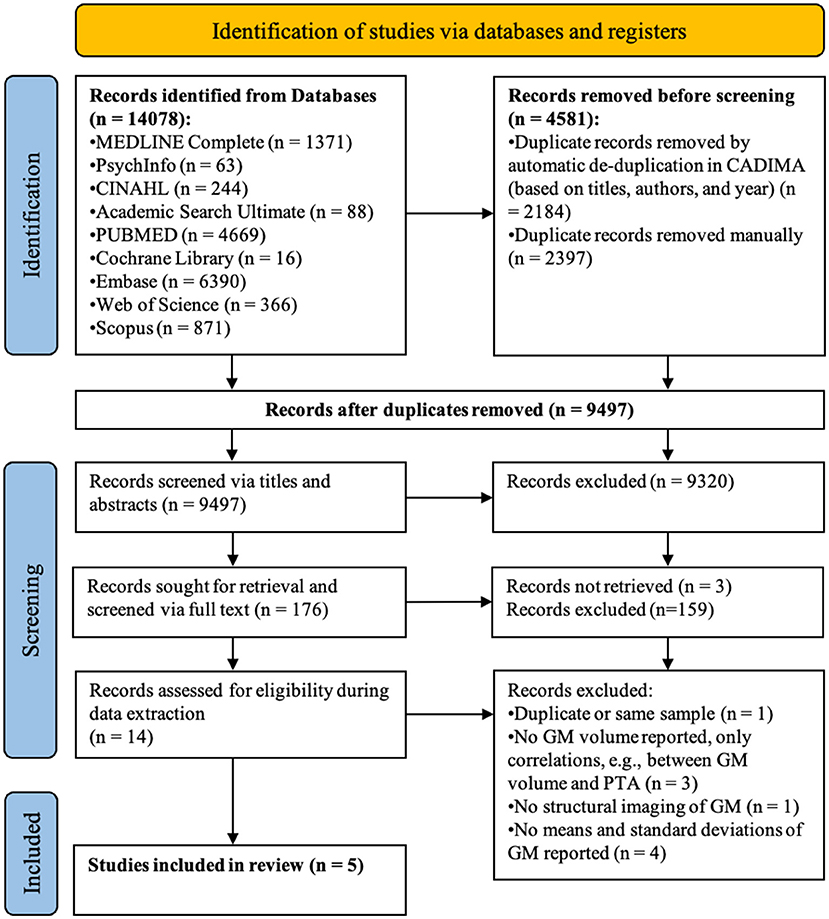
Figure 1. PRISMA flow diagram detailing the number of articles selected at each review stage. GM refers to gray matter.
Unique articles (n = 9,497) were screened by four raters for inclusion according to specific criteria (see the associated OSF repository for the full criteria used: https://osf.io/g5qcb/) in two consecutive stages: (1) title-abstract; and (2) full-text screening. Before each screening stage, the consistency between raters was checked with inter-rater reliability tests (Table 2). A subset of articles (60 at title-abstract screening stage, and 40 at full-text screening stage) were screened by two raters in parallel until at least 80% agreement was reached for each criterion. During screening, a manual with the inclusion criteria, additional background information, and guidance for the use of CADIMA was provided (manuals are also available in the OSF repository).
After consistency checks, 10% of all titles and abstracts and 30% of full-texts were double screened by two independent raters in parallel. During this initial period in screening, inconsistencies were resolved through group discussion and if necessary, information was added to the screening manual to clarify eligibility criteria. Training and extensive guidance was provided to ensure all raters fully understood the application of eligibility criteria before the remaining articles were independently screened. Throughout this process, raters met weekly to resolve outstanding questions. Raters were instructed to include rather than exclude articles if unsure, to prevent false exclusion of papers.
The final set of articles that passed title-abstract (n = 176) and full-text screening stages (n = 14), were checked for listing in the Retraction Watch Database (http://retractiondatabase.org/) to ensure that only studies not retracted were included.
Articles were screened for inclusion along a set of pre-defined eligibility criteria for (1) the title-abstract and (2) the full-text screening stages. These criteria were designed in line with the PICO/PECO framework (34, 35), which clarifies the review objectives and inclusion criteria across four domains: Population (P), Intervention/Exposure (I/E), Comparator (C), and Outcomes (O). To meet the inclusion criteria, articles were required to be original research, containing empirical data, and provided in English. Additionally, the articles needed to meet the following PICO/PECO criteria. (P) it was required that participants be older adults (average age of 60+ at the time of at least one study session) without clinical psychological or neurological illnesses, who either had or did not have age-related hearing loss (ARHL). (I/E) ARHL was defined as a pure tone average (PTA) of >25 dB HL across 0.5–2 kHz and no-ARHL was defined as a PTA of ≤ 25 dB HL averaged across 0.5–2 kHz (36, 37). (C) Studies needed to compare at least two groups, one group with ARHL and one group with no-ARHL, either longitudinally or cross-sectionally. (O) Outcome measures needed to include voxel-based morphometry data (VBM) as measured by magnetic resonance imaging (MRI) available for both groups. The outcome measures of interest were gray matter (GM) volumes for specific brain regions or for the whole brain.
Data extraction
Data extraction was performed manually by four reviewers with identically structured Microsoft Excel (2018) forms. For each study, data were extracted by at least two independent reviewers and then checked for agreement, to decrease the possibility of manual errors (38). Any inconsistencies in the extracted data were resolved through discussion. A data extraction manual was provided (available in the OSF repository). Where data were presented visually only, means and standard deviations were read from graphs. If non-significance or significance was reported without associated exact p-values, the p-value was assumed to be p = 0.05 and p = 0.04, respectively [based on Anatürk et al. (38)]. The main source of heterogeneity in analysis is likely to stem from sample characteristics, study design, and imaging technique. Therefore, data extraction included participant demographics for both ARHL and no-ARHL groups (sample size, age, sex, PTA), study design (timeframe, sampling method, timescale of longitudinal measurements), details of image acquisition (MRI field strength, smoothing kernel, slice thickness, voxel size, mask, normalization space), and outcome measures (e.g., (un)corrected p-values, effect sizes, mean and standard deviation of whole-brain and regional GM volumetric measurements). Any papers found not to meet the inclusion criteria at data extraction stage were excluded (for details, see the PRISMA flowchart, Figure 1, and materials on the OSF).
Nine papers were excluded at this stage due to the nature of the reported data or ineligibility. One was a duplicate reference. The reasons for exclusion and main findings of the remaining eight papers are reported here. Three papers (39–41) reported only correlational or regression data; due to the nature of the statistical methodology, these papers did not meet the inclusion criteria of specific group comparison between no-ARHL and ARHL groups. Across two of these studies, authors reported that ARHL only had a small effect on: GM volume in Hershel's gyrus (41); and cortical thinning in the right superior temporal and left dorsolateral frontal areas, in women only with right ear hearing loss (39). The third study reported correlations between brain volume changes and functional impairment factors within ARHL groups only (40). Another paper was ineligible as only data on white matter were reported, for which there were no differences between ARHL and no-ARHL groups (42). Finally, four papers that did not report means, standard deviations, or statistical values that could be employed in this meta-analysis were excluded due to lack of data provision following the procedure for author contact mentioned in section 2.1. Two of these papers reported no significant differences in brain volume between ARHL and no-ARHL groups (43, 44). Another reported significant differences in brain volume and thickness across temporal lobe regions, and areas of the cingulate cortex, in ARHL compared to no-ARHL (45), whereas another reported reduced GM volume in the middle frontal gyrus, but not in auditory regions, in ARHL compared to no-ARHL (46).
The remaining papers (n = 5) were included in this meta-analysis.
Critical appraisal
A framework to appraise critically the quality of the studies included in this meta-analysis was created using previously established appraisal tools. These tools typically comprise a set of questions that raters use to evaluate the research methodologies of included studies. Such frameworks allow appraisal of study quality and risk of bias, to evaluate the reliability and validity of studies' findings, and whether findings are representative and generalizable at population-level. No automation tools were used in this process. The critical appraisal tool was based on an adapted version of the trialed AXIS appraisal framework (47) and response options were based on QualSyst (48). Individual criteria of the original AXIS tool were omitted or included based on Müller et al. (28), the STROBE statement (49), and GRADE (50, 51). To minimize subjectivity (28), each paper included in the analysis was appraised by two raters independently, and disagreements resolved by discussion or ultimately, a third rater. Raters were trained and received an explanatory manual. To assess homogeneity in methods and outcomes across studies, the critical appraisal accounted for whether or not research controlled for confounding factors (e.g., sex, education, smoking status, age), as well as methodological factors that could influence data interpretation (e.g., sample size). The appraisal manual and method of calculation are available in the OSF repository.
Statistical analysis
Statistical analysis was conducted using effect-size seed-based d mapping (ES-SDM) software to perform a random-effects meta-analysis (52), the software was developed to aid the meta-analysis of voxel-based data as obtained by VBM (www.sdmproject.com). VBM is a neuroimaging technique comparing GM concentration by mapping images onto a normalized stereotactic space and extracting GM volumes, smoothing data, and finally, comparing group GM volume differences via voxel-wise comparison (53). ES-SDM is described in detail elsewhere (52, 54) and has previously been tested for reliability (55, 56), including for GM volume comparison (57). ES-SDM calculates Hedges' g effect sizes for mean analysis, based on group means, and standard deviations (54). Hedges' g uses a pooled and weighted standard deviation based on sample size and is thus more accurate for small sample sizes (<20) than Cohen's d which uses a normal standard deviation (58–60). The inclusion of non-significant findings in the analysis addresses bias toward significant overall results.
The analysis of GM volumes in ES-SDM was a mean analysis providing Hedges' g and corresponding z- and p-values, as well as standard error, the lower and upper bounds of the effect size for each study, and a mean across studies. Q statistics were used to assess inter-study heterogeneity of effect sizes. The analysis followed the ES-SDM manual (available here www.sdmproject.com). Furthermore, we verified the analysis in RStudio [R version 4.1.0, (61)], using the metafor() package to conduct a random-effects model meta-analysis (62), and to produce the associated forest and funnel plots. The data analysis obtained was the same in ES-SDM and R. The R code is provided in the OSF repository: https://osf.io/g5qcb.
Calculation of missing standard deviations
Under the assumption that data were normally distributed, missing standard deviations were calculated from confidence intervals using the following formula (30):
ES-SDM and R analysis
Sample sizes of both groups (ARHL vs. no-ARHL), means and standard deviations were entered for each study and each region of interest (ROI) into ES-SDM. Separate analyses were conducted for whole-brain and lobe-wise GM volume using the same ES-SDM “globals” calculator as it relies on mean analysis and is, therefore, also suitable for analysis of mean ROI data. To compare ROIs, ROIs were collated into frontal, temporal, parietal, and occipital lobes. The collation was completed following the papers' verbal labels of ROIs (e.g., superior temporal lobe was allocated to the temporal cortex) and widely accepted localisations, e.g., precentral gyrus is undisputedly considered to lie in the frontal lobe. If allocation to a lobe was unclear, a neuroanatomy textbook was consulted (63). The same data were entered into R and separate meta-analyses were conducted for whole-brain, frontal, temporal, parietal, and occipital lobe data, as was done in ES-SDM software.
Results
Of the 9,497 articles screened, five satisfied all inclusion criteria (see Figure 1 for the PRISMA flow diagram). During title-abstract screening, a total of 413 inter-rater inconsistencies were resolved of which only 102 affected inclusion (n = 25) or exclusion (n = 77) of the article. During full-text screening there were 37 inconsistencies of which 16 affected inclusion (n = 2) or exclusion (n = 14). The number of articles that were at first included, but through discussion of inconsistencies excluded, can be explained by the instructions to screeners to be more lenient than conservative in case of uncertainty when judging whether or not the articles fulfilled screening criteria.
Across both screening stages, the criteria that caused most inconsistencies were whether or not participants were at least 60 years old and (neurologically) healthy, as well as whether or not the study made a direct comparison of neuroanatomical differences between groups.
Heterogeneity of effect sizes and evaluation of study quality
Descriptive statistics of the meta-analyzed studies are presented in Tables 3, 4. Only one of the five included studies adopted a longitudinal approach. As such it was not possible to meta-analyze rate-of-change in GM volume over time. Therefore, all included effects reflect cross-sectional comparisons between participant groups with and without ARHL, regardless of longitudinal or cross-sectional study design.
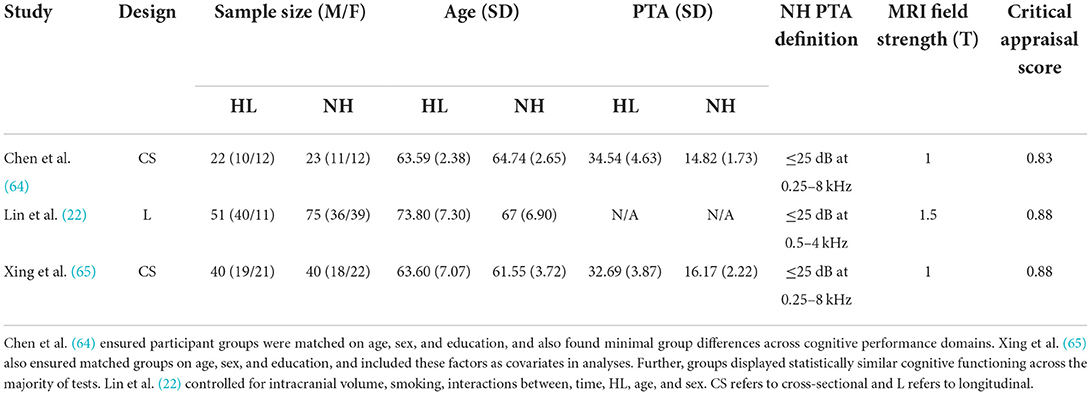
Table 3. Means, and standard deviations where applicable, of the data extracted for papers used in the whole-brain analysis.
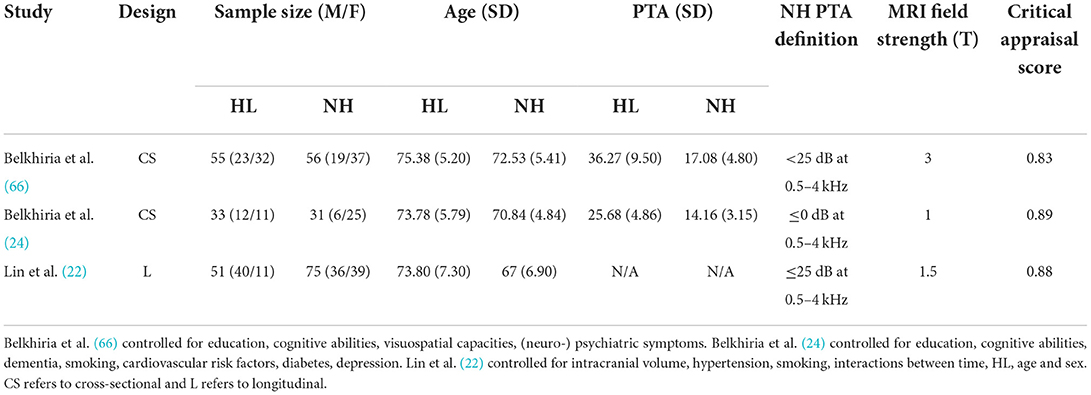
Table 4. Means, and standard deviations where applicable, of the data extracted for papers used in the lobe-wise analyses.
Critical appraisal of the included studies was conducted by a minimum of two raters to assess research quality and risk of bias to evaluate. Studies were assessed across a range of criteria, including whether or not research controlled for important confounding factors that could influence hearing status or brain structure (e.g., sex, age, education). In one study, it was unclear whether confounding variables were controlled for in analyses, but the two groups (ARHL and no-ARHL) were matched for age, sex, and education, and showed statistically similar cognitive functioning across a range of tests (64). Critically, the four remaining studies state explicitly that statistical analyses accounted for both age and sex (22, 24, 65, 66). Further, three studies controlled for education (24, 65, 66), and three controlled for total estimated intracranial volume (22, 24, 65). In the one longitudinal study included here, the additional variables of hypertension, smoking, hearing impairment, and years since baseline, were included as covariates in analyses (22). Importantly, across the studies included in this meta-analysis, all considered the impact of key confounding variables (e.g., age) on the analyzes, allowing for clearer interpretation of the relation between ARHL and GM volume.
Overall, critical appraisal scores did not lie below 0.83, indicating high methodological quality (48). In combination with the observation that all ratings fell between 0.83 and 0.89, it is unlikely that methodological inadequacies skewed results or studies formed subgroups of studies with high and low methodological quality. However, it should be noted that a source of bias might be the consistently partial fulfillment of a sampling process likely to represent the target population. All studies employed convenience sampling (recruiting from hospital settings or previous study cohorts) and acknowledged this as a limitation. The results in this meta-analysis are consequently subject to the same constraints in generalizability of results.
Across analyses, the Q statistic did not reach significance indicating no significant heterogeneity of effect sizes between studies (whole-brain, Q(2) = 3.93, p = 0.14; frontal lobe, Q(7) = 3.11, p = 0.87; temporal lobe, Q(26) = 17.59, p = 0.67; parietal lobe Q(2) = 2.78, p = 0.10; occipital lobe, Q(4) = 2.43, p = 0.66). This homogeneity, in combination with the results from the critical appraisal, suggest no significant variation in the studies' characteristics and that the heterogeneity likely stems from sampling error alone. Thus, it is unlikely that underlying variation in methodology or participant groups between studies skewed the results (67).
Whole-brain and lobe-wise analysis
A comprehensive overview and visualization of results is presented in Tables 5–8, and Figures 2–6, respectively. In comparison with group no-ARHL, group ARHL, the differences whole-brain GM volume were not significant, Hedges' g = 0.12, p = 0.52. Similar to the whole-brain GM volume, group ARHL showed lower GM volumes in lobe-wise analysis. This difference was significant in the temporal lobe (Hedges' g = −0.12, p = 0.007), but was not significant in the frontal (Hedges' g = −0.03, p = 0.64), parietal (Hedges' g = −0.17, p = 0.52), nor occipital (Hedges' g = −0.12, p = 0.14) lobes.
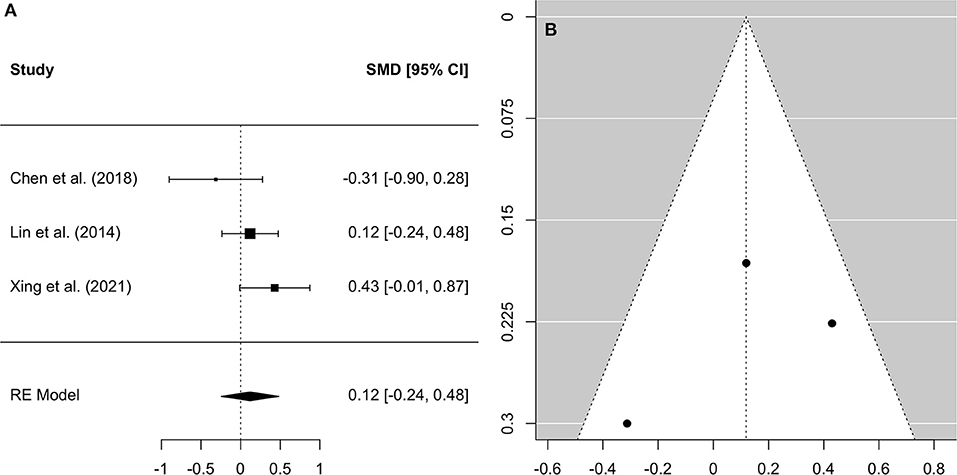
Figure 2. Forest plot (A) and funnel plot (B) of the whole-brain Analysis. In the forest plot (A) negative values on the x-axis indicate gray matter atrophy.
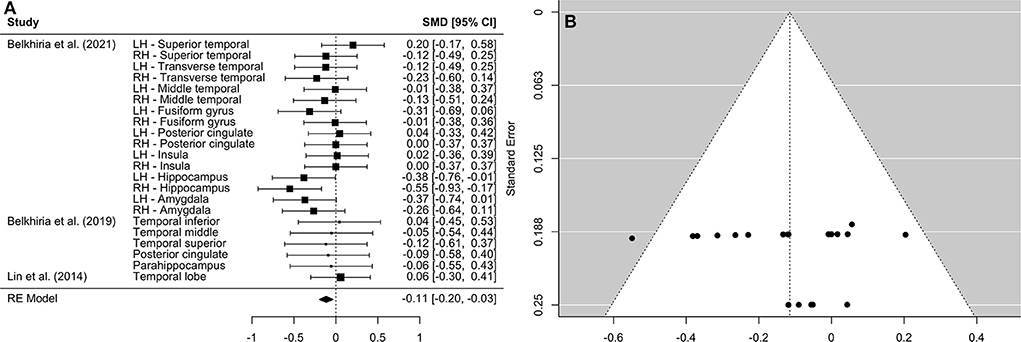
Figure 3. Forest plot (A) and funnel plot (B) of the temporal lobe analysis. In the forest plot (A) negative values on the x-axis indicate gray matter atrophy.
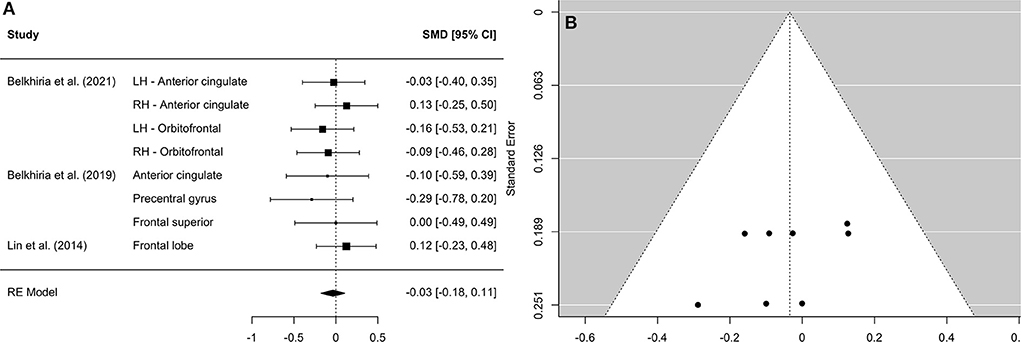
Figure 4. Forest plot (A) and funnel plot (B) of the frontal lobe analysis. In the forest plot (A) negative values on the x-axis indicate gray matter atrophy.
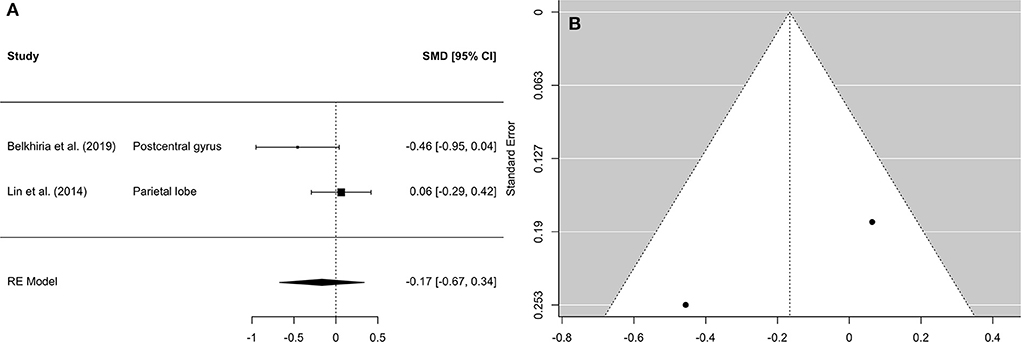
Figure 5. Forest plot (A) and funnel plot (B) of the parietal lobe analysis. In the forest plot (A) negative values on the x-axis indicate gray matter atrophy.
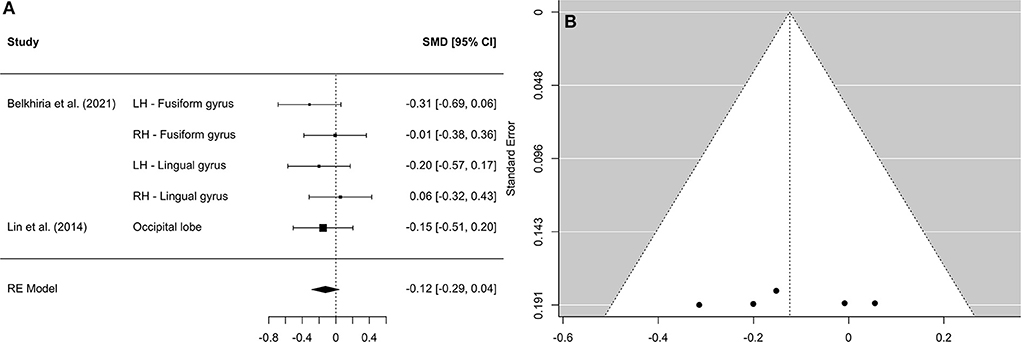
Figure 6. Forest plot (A) and funnel plot (B) of the occipital lobe analysis. In the forest plot (A) negative values on the x-axis indicate gray matter atrophy.
Discussion
This meta-analysis sought to collate and evaluate the existing evidence for a difference in brain volume, specifically GM volume, in adults (aged ≥60 years) with ARHL, compared to those without ARHL. We sought to include data from both cross-sectional and longitudinal study designs, in order to consolidate and analyse the available empirical evidence and provide a better understanding of cortical changes associated with ARHL. We employed ES-SDM software to conduct analysis of neuroanatomical data across the included studies (52).
Three studies, two of which took a cross-sectional approach and one of which took a longitudinal approach, which reported GM volumes for the whole brain were included in the analysis of global neuroanatomical changes associated with ARHL. This analysis served to investigate whether the entire brain displays significant GM atrophy in individuals with ARHL, compared to those without ARHL, in order to further understand how hearing loss contributes to brain aging. The findings did not support our hypothesis that adults with ARHL would display significantly decreased whole brain GM volume, compared to those without ARHL. While previous research suggests that cross-cortical and brain wide changes are associated with ARHL (10, 22), this meta-analysis of collated studies suggests that changes associated with ARHL are not significantly greater than changes which occur in aging. If this is the case, then it is possible, as suggested by the common cause hypothesis, that a neurodegenerative factor may underly the brain atrophies observed in both hearing loss and aging.
Elevated tau protein levels could be an indicator of a potential third factor that no meta-analyzed study has accounted for explicitly. Tau is a protein found to aggregate abnormally in Alzheimer's Disease and has, therefore, been considered as a viable biomarker (70). In a study on people with dementia, the prevalence of tau protein in the cerebral spinal fluid was found to be higher in participants who reported having hearing loss than in those who did not (71). Consequently, there may be an association between tau levels and hearing. Whilst this requires further investigation, it is possible that neuroanatomical findings in the meta-analyzed studies might be influenced by biomarkers of potential pre-clinical cognitive declines (such as tau levels) in participants with ARHL.
However, limiting neuroanatomical observations to whole brain analysis only may result in overlooking of essential information regarding lobe-wise cortical changes. Understanding in which cortical structures changes occur is important for establishing the role of potential underlying causal mechanisms. Three studies, two of which took a cross-sectional approach and one of which took a longitudinal approach, which reported GM volumes in specific brain areas were included in the lobe-wise analysis of GM volumes. This analysis enabled the investigation into cortical changes across brain lobes to establish whether GM atrophy extends beyond auditory cortex (situated in temporal lobe) in individuals with ARHL. The findings support our hypothesis that decreases in GM volume observed in individuals with ARHL compared to those without ARHL occur in the temporal lobe. This is consistent with existing literature which reports increased neural atrophy in auditory cortex in individuals with ARHL, compared to those without ARHL (22). No evidence was found that declines in GM volume in people with ARHL occur in other lobes.
Research suggests that ARHL leads to up-regulation across brain networks to support speech perception, and there is evidence from functional imaging studies to support this, showing that ARHL is associated with increased functional connectivity between auditory cortex and cognitive networks (26). Increased use of such cortical resources has been theorized to trigger neurodegeneration due to over-use of neural resources and excitotoxic cell death. Yet, our data provide no evidence for declines in GM volume beyond temporal lobe and thus do not support the hypothesis that potential compensatory activity leads to neurodegeneration. This has implications for interpretation of the causal hypotheses underlying the association between hearing loss and cognitive decline. Importantly, previous research finds that declines in cognitive functioning are also associated with greater GM volume loss in temporal regions (72). This has important implications as temporal atrophies may be an underlying mechanism in the relation between hearing loss and cognitive declines.
An additional explanation for the relation between hearing loss and cognitive declines in aging not captured by this review, is the role of the psychosocial pathway in sensory deprivation: Hearing loss does not manifest exclusively in auditory deprivation due to poor hearing, but is also accompanied by mental health and well-being consequences. Adults with hearing loss may be more likely to withdraw from social interactions due to hearing difficulties, leading to experiences of increased depression, and loneliness or isolation (73). Some authors suggest that social withdrawal may exacerbate the relation between hearing loss and wider brain and cognitive health, because it increases sensory deprivation (74). As such, there may be consequences for neural and cognitive functioning if these brain areas are less utilized for stimulating social communication.
It is important to consider, with regards to both the whole-brain and lobe-wise analyses, that the included study designs varied between cross-sectional and longitudinal. First, it is possible that global GM atrophy, or atrophy across wider cortices, only occurs after prolonged sensory deprivation. In two previous longitudinal studies, a significant association between pure-tone hearing loss and reduced GM in auditory cortex was only present after at least 5 years (22, 23). Hence, it is possible theoretically that atrophies extending further than auditory cortex, or temporal lobe, may only occur after prolonged up-regulation or cortical resource reallocation to assist speech perception due to ARHL. Second, in both designs, consideration of confounding factors is important, but particularly for cross-sectional research. As such, it is important to note that differences in the controlled variables across the included studies may affect the results, and create ambiguity for interpretation. By design, longitudinal research allows for increased control over individual factors which may influence data, and hence any observed neural changes are more easily interpreted as occurring due to HL, rather than aging or another underlying neurodegenerative variable.
Importantly, to ensure homogeneity across studies included in this meta-analysis, included studies were limited to those which classified hearing status using pure tone audiometry. This method is the current gold-standard in clinical audiology, but does not account well for supra-threshold hearing difficulties, i.e., difficulties in hearing sounds presented above the auditory threshold of the listener, such as the perception of speech in background noise. Consequently, this meta-analysis does not capture the impact of such difficulties, which may present before observable declines in the audiogram are evident, on neural structure. Some studies have investigated the relation between speech reception threshold (SRT), obtained using digits-in-noise tests, on neuroanatomy. Such research found that, in older adults, poorer speech perception was associated with lower GM volume, particularly in the left superior temporal gyrus (75). Further, in older participants with Alzheimer's dementia, poorer speech perception was associated with lower cortical thickness bilaterally across many cortices (76).
Further, as many studies did not report stereotactic coordinates, the data analysis options were limited to general lobe comparisons. Hence it is not possible to interpret exactly where GM atrophy occurs within the temporal lobe. Without exact cortical locations, it is difficult to draw strong conclusions regarding the underlying neural processes or systems. Additionally, all included studies employed opportunity sampling techniques. Therefore, any generalizations were limited to the targeted populations in the included studies. Importantly, these data should be interpreted with consideration of the sample size of studies included. In order to control for confounding variables and ensure heterogeneity in methods, strict inclusion criteria were used to select the studies meta-analyzed. In-turn this resulted in a smaller number of studies selected for analysis, which resulted in a smaller number of individual data points. It has been suggested that a large sample size of individuals (across the selected studies) is required for adequate power in whole-brain meta-analysis (77). For this to be possible, there is explicit need for future large-scale longitudinal research which seeks to observe the effects of age-related hearing loss on brain morphology.
In conclusion, this meta-analysis explored the evidence for a difference in GM volume, in older adults with ARHL, compared to those without ARHL. The analysis found evidence for reduced GM volume in temporal lobes in individuals with ARHL, compared to those without ARHL. There was no evidence that GM atrophies extended to frontal, parietal, or occipital lobes, nor was there evidence for whole brain GM declines in individuals with ARHL. It is possible that significant differences in GM volume are limited to the temporal lobe, because further cortical changes only occur after a critical time period of prolonged cortical resource re-allocation. However, this finding has important implications and further longitudinal research into how neural changes across the temporal lobe in people with ARHL affects wider brain health is essential.
Data availability statement
Publicly available datasets were analyzed in this study. This data can be found here: https://osf.io/g5qcb/.
Author contributions
KS, HN, and CP contributed to conception of the study. KS, HN, CP, and JR contributed to the design of the study. LH provided expert advice. KS, JR, KJ, and ES contributed to article screening and data extraction. KS, JR, and LH contributed to and performed the statistical analysis. KS and JR wrote the manuscript. KS, JR, HN, CP, and LH contributed to manuscript revision, read, and approved the submitted version. All authors contributed to the article and approved the submitted version.
Funding
The research was supported by the Biotechnology and Biological Sciences Research Council (BBSRC) Funding reference: BB/S008527/1.
Conflict of interest
The authors declare that the research was conducted in the absence of any commercial or financial relationships that could be construed as a potential conflict of interest.
Publisher's note
All claims expressed in this article are solely those of the authors and do not necessarily represent those of their affiliated organizations, or those of the publisher, the editors and the reviewers. Any product that may be evaluated in this article, or claim that may be made by its manufacturer, is not guaranteed or endorsed by the publisher.
Supplementary Material
The Supplementary Material for this article can be found online at: https://www.frontiersin.org/articles/10.3389/fneur.2022.950997/full#supplementary-material
References
1. Office for National Statistics (ONS). Population Estimates for the UK, England and Wales, Scotland and Northern Ireland: Mid-2020. Statistical Bulletin. (2021). Available online at: https://www.ons.gov.uk/peoplepopulationandcommunity/populationandmigration/populationestimates/bulletins/annualmidyearpopulationestimates/mid2020
2. Royal National Institute for Deaf People (RNID). Hearing Matters [Internet] (2020). Available online at: https://rnid.org.uk/wp-content/uploads/2020/05/Hearing-Matters-Report.pdf (accessed May 21, 2022).
3. Wittenberg R, Hu B, Barraza-Araiza LF, Rehill A. Projections of Older People with Dementia and Costs of Dementia Care in the United Kingdom, 2019-2040. CPEC Working Paper 5 [Internet] (2019). Available online at: https://www.alzheimers.org.uk/sites/default/files/2019-11/cpec_report_november_2019.pdf (accessed May 21, 2022).
4. Livingston G, Huntley J, Sommerlad A, Ames D, Ballard C, Banerjee S, et al. Dementia prevention, intervention, and care: 2020 report of the Lancet Commission. Lancet. (2020) 396:413–46. doi: 10.1016/S0140-6736(20)30367-6
5. Livingston G, Sommerlad A, Orgeta V, Costafreda SG, Huntley J, Ames D, et al. Dementia prevention, intervention, and care. Lancet. (2020) 390:2673–734.
6. Liberman MC, Kujawa SG. Cochlear synaptopathy in acquired sensorineural hearing loss: manifestations and mechanisms. Hear Res. (2017) 349:138–47. doi: 10.1016/j.heares.2017.01.003
7. Heeringa AN, Köppl C. The aging cochlea: Towards unraveling the functional contributions of strial dysfunction and synaptopathy. Hear Res. (2019) 376:111–24. doi: 10.1016/j.heares.2019.02.015
8. Frisina RD, Ding B, Zhu X, Walton JP. Age-related hearing loss: Prevention of threshold declines, cell loss and apoptosis in spiral ganglion neurons. Aging. (2016) 8:2081–99. doi: 10.18632/aging.101045
9. Grose JH, Buss E, Elmore H. Age-related changes in the auditory brainstem response and suprathreshold processing of temporal and spectral modulation. Trends Hear. (2019) 23:1–11. doi: 10.1177/2331216519839615
10. Rigters SC, Bos D, Metselaar M, Roshchupkin G v., Baatenburg de, Jong RJ, Ikram MA, et al. Hearing impairment is associated with smaller brain volume in aging. Front.Aging Neurosci. (2017) 9:2. doi: 10.3389/fnagi.2017.00002
11. Eckert MA, Kuchinsky SE, Vaden KI, Cute SL, Spampinato MV, Dubno JR. White matter hyperintensities predict low frequency hearing in older adults. J Assoc Res Otolaryngol. (2013) 14:425–33. doi: 10.1007/s10162-013-0381-4
12. Lockhart SN, DeCarli C. Structural imaging measures of brain aging. Neuropsychol Rev. (2014) 24:271–89. doi: 10.1007/s11065-014-9268-3
13. Füllgrabe C. On the possible overestimation of cognitive decline: the impact of age-related hearing loss on cognitive-test performance. Front Neurosci. (2020) 14:454. doi: 10.3389/fnins.2020.00454
14. Füllgrabe C. When hearing loss masquerades as cognitive decline. J Neurol Neurosurg Psychiatry. (2020) 91:1248–1248. doi: 10.1136/jnnp-2020-324707
15. Deal JA, Betz J, Yaffe K, Harris T, Purchase-Helzner E, Satterfield S, et al. Hearing impairment and incident dementia and cognitive decline in older adults: the health ABC study. J Gerontol A Biol Sci Med Sci. (2016) 72:703–9. doi: 10.1093/gerona/glw069
16. Gurgel RK, Ward PD, Schwartz S, Norton MC, Foster NL, Tschanz JT. Relationship of hearing loss and dementia. Otol Neurotol. (2014) 35:775–81. doi: 10.1097/MAO.0000000000000313
17. Lin FR. Hearing loss and cognition among older adults in the United States. J Gerontol A Biol. Sci Med Sci. (2011) 66A:1131–1136. doi: 10.1093/gerona/glr115
18. Ward KM, Shen J, Souza PE, Grieco-Calub TM. Age-related differences in listening effort during degraded speech recognition. Ear Hear. (2017) 38:74–84. doi: 10.1097/AUD.0000000000000355
19. Panouillères MTN, Möttönen R. Decline of auditory-motor speech processing in older adults with hearing loss. Neurobiol Aging. (2018) 72:89–97. doi: 10.1016/j.neurobiolaging.2018.07.013
20. Rosemann S, Thiel CM. Audio-visual speech processing in age-related hearing loss: Stronger integration and increased frontal lobe recruitment. Neuroimage. (2018) 175:425–37. doi: 10.1016/j.neuroimage.2018.04.023
21. Slade K, Plack CJ, Nuttall HE. The effects of age-related hearing loss on the brain and cognitive function. Trends Neurosci. (2020) 43:810–21. doi: 10.1016/j.tins.2020.07.005
22. Lin FR, Ferrucci L, An Y, Goh JO, Doshi J, Metter EJ, et al. Association of hearing impairment with brain volume changes in older adults. Neuroimage. (2014) 90:84–92. doi: 10.1016/j.neuroimage.2013.12.059
23. Eckert MA, Vaden KI, Dubno JR. Age-related hearing loss associations with changes in brain morphology. Trends Hear. (2019) 23:1–14. doi: 10.1177/2331216519857267
24. Belkhiria C, Vergara RC, San Martín S, Leiva A, Marcenaro B, Martinez M, et al. Cingulate cortex atrophy is associated with hearing loss in presbycusis with cochlear amplifier dysfunction. Front Aging Neurosci. (2019) 11:97. doi: 10.3389/fnagi.2019.00097/full
25. Puschmann S, Thiel CM. Changed cross modal functional connectivity in older adults with hearing loss. Cortex. (2017) 86:109–22. doi: 10.1016/j.cortex.2016.10.014
26. Fitzhugh MC, Hemesath A, Schaefer SY, Baxter LC, Rogalsky C. Functional connectivity of heschl's gyrus associated with age-related hearing loss: a resting-state fMRI study. Front Psychol. (2019) 10:2485. doi: 10.3389/fpsyg.2019.02485/full
27. Armada-Moreira A, Gomes JI, Pina CC, Savchak OK, Gonçalves-Ribeiro J, Rei N, et al. Going the extra (Synaptic) mile: excitotoxicity as the road toward neurodegenerative diseases. Front Cell Neurosci. (2020) 14:90. doi: 10.3389/fncel.2020.00090
28. Müller VI, Cieslik EC, Laird AR, Fox PT, Radua J, Mataix-Cols D, et al. Ten simple rules for neuroimaging meta-analysis. Neurosci Biobehav Rev. (2018) 84:151–61. doi: 10.1016/j.neubiorev.2017.11.012
29. Peters MDJ, Godfrey CM, Khalil H, McInerney P, Parker D, Soares CB. Guidance for conducting systematic scoping reviews. Int J Evid Based Healthc. (2015) 13:141–6. doi: 10.1097/XEB.0000000000000050
30. Moher D, Shamseer L, Clarke M, Ghersi D, Liberati A, Petticrew M, et al. Preferred reporting items for systematic review and meta-analysis protocols (PRISMA-P) 2015 statement. Syst Rev. (2015) 4:4053. doi: 10.1186/2046-4053-4-1
31. Higgins JPT, Thomas J, Chandler J, Cumpston M, Li T, Page MJ, et al. Cochrane handbook for systematic reviews of interventions. 6.2. Cochrane (2021). Available online at: www.training.cochrane.org/handbook
32. Kohl C, McIntosh EJ, Unger S, Haddaway NR, Kecke S, Schiemann J, et al. Online tools supporting the conduct and reporting of systematic reviews and systematic maps: a case study on CADIMA and review of existing tools. Environ Evid. (2018) 7:115. doi: 10.1186/s13750-018-0115-5
33. Page MJ, McKenzie JE, Bossuyt PM, Boutron I, Hoffmann TC, Mulrow CD, et al. The PRISMA 2020 statement: an updated guideline for reporting systematic reviews. BMJ. (2021) 372:n71. doi: 10.1136/bmj.n71
34. Booth A, Clarke M, Dooley G, Ghersi D, Moher D, Petticrew M, et al. The nuts and bolts of PROSPERO: an international prospective register of systematic reviews. Syst Rev. (2012) 1:4053 doi: 10.1186/2046-4053-1-2
35. Schardt C, Adams MB, Owens T, Keitz S, Fontelo P. BMC Medical Informatics and Decision Making Utilization of the PICO framework to improve searching PubMed for clinical questions. BMC Med Inform Decis Mak. (2007) 7:6947. doi: 10.1186/1472-6947-7-16
36. British Society for Audiology (BSA). Recommended procedure. Pure-tone air-conduction and Bone-conduction threshold audiometry with and without masking [Internet] (2018). Available online at: https://www.thebsa.org.uk/wp-content/uploads/2018/11/OD104-32-Recommended-Procedure-Pure-Tone-Audiometry-August-2018-FINAL.pdf (accessed May 21, 2022).
37. World Health Organization (WHO). Report of the Informal Working Group on Prevention of Deafness and Hearing Impairment Programme Planning [Internet] (1991). Available online at: https://apps.who.int/iris/bitstream/handle/10665/58839/WHO_PDH_91.1.pdf (accessed May 21, 2022).
38. Anatürk M, Demnitz N, Ebmeier KP, Sexton CE. A systematic review and meta-analysis of structural magnetic resonance imaging studies investigating cognitive and social activity levels in older adults. Neurosci Biobehav Rev. (2018) 93:71–84. doi: 10.1016/j.neubiorev.2018.06.012
39. Ha J, Cho YS, Kim SJ, Cho SH, Kim JP, Jung YH, et al. Hearing loss is associated with cortical thinning in cognitively normal older adults. Eur J Neurol. (2020) 27:1003–9. doi: 10.1111/ene.14195
40. Belkhiria C, Vergara RC, San Martin S, Leiva A, Martinez M, Marcenaro B, et al. Insula and amygdala atrophy are associated with functional impairment in subjects with presbycusis. Front Aging Neurosci. (2020) 12:102. doi: 10.3389/fnagi.2020.00102
41. Profant O, Škoch A, Tintěra J, Svobodová V, Kuchárová D, Svobodová Burianová J, et al. The influence of aging, hearing, and tinnitus on the morphology of cortical gray matter, amygdala, and hippocampus. Front Aging Neurosci. (2020) 4:553461. doi: 10.3389/fnagi.2020.553461
42. Ma W, Li M, Gao F, Zhang X, Shi L, Yu L, et al. DTI analysis of presbycusis using voxel-based analysis. Am J Neuroradiol. (2016) 37:2110–4. doi: 10.3174/ajnr.A4870
43. Profant O, Škoch A, Balogová Z, Tintěra J, Hlinka J, Syka J. Diffusion tensor imaging and MR morphometry of the central auditory pathway and auditory cortex in aging. Neuroscience. (2014) 260:87–97. doi: 10.1016/j.neuroscience.2013.12.010
44. Chen YC, Yong W, Xing C, Feng Y, Haidari NA, Xu JJ, et al. Directed functional connectivity of the hippocampus in patients with pre-sbycusis. Brain Imaging Behav. (2020) 14:917–26. doi: 10.1007/s11682-019-00162-z
45. Ren F, Ma W, Li M, Sun H, Xin Q, Zong W, et al. Gray matter atrophy is associated with cognitive impairment in patients with pre-sbycusis: A comprehensive morphometric study. Front Neurosci. (2018) 12:744. doi: 10.3389/fnins.2018.00744
46. Rosemann S, Thiel CM. Neuroanatomical changes associated with age-related hearing loss and listening effort. Brain Struct Funct. (2020) 225:2689–700. doi: 10.1007/s00429-020-02148-w
47. Downes MJ, Brennan ML, Williams HC, Dean RS. Development of a critical appraisal tool to assess the quality of cross-sectional studies (AXIS). BMJ Open. (2016) 6:e011458. doi: 10.1136/bmjopen-2016-011458
48. Kmet LM, Lee RC, Cook LS. Standard quality assessment criteria for evaluating primary research papers. Alberta Heritage Found Med Res. (2004) 3:16. doi: 10.7939/R37M04F16
49. von Elm E, Altman DG, Egger M, Pocock SJ, Gøtzsche PC, Vandenbroucke JP. The strengthening the reporting of observational studies in epidemiology (STROBE) statement: guidelines for reporting observational studies. J Clin Epidemiol. (2008) 61:344–249. doi: 10.1016/j.jclinepi.2007.11.008
50. Meader N, King K, Llewellyn A, Norman G, Brown J, Rodgers M, et al. A checklist designed to aid consistency and reproducibility of GRADE assessments: development and pilot validation. Syst Rev. (2014) 3:82. doi: 10.1186/2046-4053-3-82
51. Atkins D, Eccles M, Flottorp S, Guyatt GH, Henry D, Hill S, et al. Systems for grading the quality of evidence and the strength of recommendations I: critical appraisal of existing approaches The GRADE Working Group. BMC Health Serv Res. (2004) 4:38. doi: 10.1186/1472-6963-4-38
52. Radua J, Mataix-Cols D, Phillips ML, El-Hage W, Kronhaus DM, Cardoner N, et al. A new meta-analytic method for neuroimaging studies that combines reported peak coordinates and statistical parametric maps. Eur Psychiatry. (2012) 27:605–11. doi: 10.1016/j.eurpsy.2011.04.001
53. Ashburner J, Friston KJ. Voxel-based morphometry-the methods. Neuroimage. (2000) 11:805–21. doi: 10.1006/nimg.2000.0582
54. Albajes-Eizagirre A, Solanes A, Vieta E, Radua J. Voxel-based meta-analysis via permutation of subject images (PSI): theory and implementation for SDM. Neuroimage. (2019) 186:174–84. doi: 10.1016/j.neuroimage.2018.10.077
55. Radua J, Mataix-Cols D. Voxel-wise meta-analysis of grey matter changes in obsessive-compulsive disorder. Br J Psychiatry. (2009) 195:393–402. doi: 10.1192/bjp.bp.108.055046
56. Radua J, Rubia K, Canales-Rodríguez EJ, Pomarol-Clotet E, Fusar-Poli P, Mataix-Cols D. Anisotropic kernels for coordinate-based meta-analyses of neuroimaging studies. Front Psychiatry. (2014) 5:13. doi: 10.3389/fpsyt.2014.00013
57. Wise T, Radua J, Via E, Cardoner N, Abe O, Adams TM, et al. Common and distinct patterns of grey-matter volume alteration in major depression and bipolar disorder: evidence from voxel-based meta-analysis. Mol Psychiatry. (2017) 22:1455–63. doi: 10.1038/mp.2016.72
58. Hamman EA, Pappalardo P, Bence JR, Peacor SD, Osenberg CW. Bias in meta-analyses using Hedges' d. Ecosphere (2018) 9:2419. doi: 10.1002/ecs2.2419
59. Wasserman S, Hedges LV, Olkin I. Statistical methods for meta-analysis. J Educ Stat. (1988) 13:75–8. doi: 10.2307/1164953
60. Cooper H, Hedges L, Valentine JC. The Handbook of Research Synthesis and Meta-Analysis. 2nd ed. New York: Russell Sage Foundation (2009).
61. R Core Team. R: A language and environment for statistical computing. R Foundation for Statistical Computing. (2022). Vienna, Austria. Available online at: https://www.R-project.org/
62. Viechtbauer W. Conducting meta-analyses in R with the metafor package. J Stat Softw. (2010) 36:1–48. doi: 10.18637/jss.v036.i03
64. Chen YC, Chen H, Jiang L, Bo F, Xu JJ, Mao CN, et al. Presbycusis disrupts spontaneous activity revealed by resting-state functional MRI. Front Behav Neurosci. (2018) 12:44. doi: 10.3389/fnbeh.2018.00044
65. Xing C, Chen YC, Tong Z, Xu W, Xu JJ, Yin X, et al. Aberrant brain functional hubs and causal connectivity in presbycusis. Brain Imaging Behav. (2021) 15:453–63. doi: 10.1007/s11682-020-00386-4
66. Belkhiria C, Vergara RC, Martinez M, Delano PH, Delgado C. Neural links between facial emotion recognition and cognitive impairment in presbycusis. Int J Geriatr Psychiatry. (2021) 36:1171–8. doi: 10.1002/gps.5501
67. Bowden J, Tierney JF, Copas AJ, Burdett S. Quantifying, displaying and accounting for heterogeneity in the meta-analysis of RCTs using standard and generalized Q statistics. BMC Med Res Methodol. (2011) 11:41. doi: 10.1186/1471-2288-11-41
68. Stephani C, Fernandez-Baca Vaca G, Maciunas R, Koubeissi M, Lüders HO. Functional neuroanatomy of the insular lobe. Brain Struct Funct. (2011) 216:137–49. doi: 10.1007/s00429-010-0296-3
69. Ardila A, Bernal B, Rosselli M. The elusive role of the left temporal pole (BA38) in language: a preliminary meta-analytic connectivity study. Int J Neurosci. (2014) 946039:1–7. doi: 10.1155/2014/946039
70. Jack CR, Knopman DS, Jagust WJ, Shaw LM, Aisen PS, Weiner MW, et al. Hypothetical model of dynamic biomarkers of the Alzheimer's pathological cascade. Lancet Neurol. (2010) 9:119–28. doi: 10.1016/S1474-4422(09)70299-6
71. Xu W, Zhang C, Li JQ, Tan CC, Cao XP, Tan L, et al. Age-related hearing loss accelerates cerebrospinal fluid tau levels and brain atrophy: a longitudinal study. Aging. (2019) 11:3156–69. doi: 10.18632/aging.101971
72. Armstrong NM, An Y, Shin JJ, Williams OA, Doshi J, Erus G, et al. Associations between cognitive and brain volume changes in cognitively normal older adults. Neuroimage. (2020) 223:117289. doi: 10.1016/j.neuroimage.2020.117289
73. Dawes P, Emsley R, Cruickshanks KJ, Moore DR, Fortnum H, Edmondson-Jones M, et al. Hearing loss and cognition: The role of hearing aids, social isolation and depression. PLoS ONE. (2015) 10:9616. doi: 10.1371/journal.pone.0119616
74. Brewster K, Wall M, Stein A, Zilcha-Mano S, Rutherford BR. Age-related hearing loss, late-life depression, and risk for incident dementia in older adults. J Gerontol A Biol Sci Med Sci. (2021) 76:827–34. doi: 10.1093/gerona/glaa242
75. Rudner M, Seeto M, Keidser G, Johnson B, Rönnberg J. Poorer speech reception threshold in noise is associated with lower brain volume in auditory and cognitive processing regions. J Speech Lang Hear Res. (2019) 62:1117–30. doi: 10.1044/2018_jslhr-h-ascc7-18-0142
76. Giroud N, Pichora-Fuller MK, Mick P, Wittich W, Al-Yawer F, Rehan S, et al. Hearing loss is associated with gray matter differences in older adults at risk for and with Alzheimer's disease. Aging Brain. (2021) 1:100018. doi: 10.1016/j.nbas.2021.100018
Keywords: age-related hearing loss (ARHL), gray matter (GM), structural MRI, brain volume, hearing loss, meta-analysis
Citation: Slade K, Reilly JH, Jablonska K, Smith E, Hayes LD, Plack CJ and Nuttall HE (2022) The impact of age-related hearing loss on structural neuroanatomy: A meta-analysis. Front. Neurol. 13:950997. doi: 10.3389/fneur.2022.950997
Received: 23 May 2022; Accepted: 15 July 2022;
Published: 08 August 2022.
Edited by:
Helen Henshaw, University of Nottingham, United KingdomReviewed by:
Dona M. P. Jayakody, Ear Science Institute Australia, AustraliaIan Michael Wiggins, University of Nottingham, United Kingdom
Copyright © 2022 Slade, Reilly, Jablonska, Smith, Hayes, Plack and Nuttall. This is an open-access article distributed under the terms of the Creative Commons Attribution License (CC BY). The use, distribution or reproduction in other forums is permitted, provided the original author(s) and the copyright owner(s) are credited and that the original publication in this journal is cited, in accordance with accepted academic practice. No use, distribution or reproduction is permitted which does not comply with these terms.
*Correspondence: Kate Slade, ay5zbGFkZTJAbGFuY2FzdGVyLmFjLnVr; Helen E. Nuttall, aC5udXR0YWxsMUBsYW5jYXN0ZXIuYWMudWs=
†These authors have contributed equally to this work and share first authorship