- 1Department of Neurology, Cardinal Tien Hospital, New Taipei City, Taiwan
- 2Institute of Public Health, National Yang-Ming Chiao Tung University, Taipei, Taiwan
- 3Department of Radiology, Cardinal Tien Hospital, New Taipei City, Taiwan
- 4Medical School of Fu-Jen University, New Taipei City, Taiwan
- 5Geriatric Behavioral Neurology Project, Tohoku University New Industry Hatchery Center (NICHe), Sendai, Japan
Background and objectives: Among individuals with Alzheimer's disease (AD), APOE e4 carriers with increased white matter hyperintensities (WMHs) may selectively be at increased risk of cognitive impairment. Given that the cholinergic system plays a crucial role in cognitive impairment, this study aimed to identify how APOE status modulates the associations between dementia severity and white matter hyperintensities in cholinergic pathways.
Methods: From 2018 to 2022, we recruited participants (APOE e4 carriers, n = 49; non-carriers, n = 117) from the memory clinic of Cardinal Tien Hospital, Taipei, Taiwan. Participants underwent brain MRI, neuropsychological testing, and APOE genotyping. In this study, we applied the visual rating scale of the Cholinergic Pathways Hyperintensities Scale (CHIPS) to evaluate WMHs in cholinergic pathways compared with the Fazekas scale. Multiple regression was used to assess the influence of CHIPS score and APOE carrier status on dementia severity based on Clinical Dementia Rating—Sum of Boxes (CDR-SB).
Results: After adjusting for age, education and sex, higher CHIPS scores tended to be associated with higher CDR-SB in APOE e4 carriers but not in the non-carrier group.
Conclusions: Carriers and non-carriers present distinct associations between dementia severity and WMHs in cholinergic pathways. In APOE e4 carriers, increased white matter in cholinergic pathways are associated with greater dementia severity. In non-carriers, WMHs exhibit less predictive roles for clinical dementia severity. WMHs on the cholinergic pathway may have a different impact on APOE e4 carriers vs. non-carriers.
1. Introduction
Sporadic Alzheimer's disease (AD) is the most common cause of neurodegenerative diseases in older adults, accounting for ~10% of cases in the population above the age of 65 years in the US (1) and 7–8% of the same population worldwide, with a considerable growing prevalence noted in the Asia Pacific region (2). The progressive loss of cholinergic neurons in the basal forebrain (3, 4) is associated with cognitive impairment in AD dementia. Current evidence indicates that the widely distributed acetylcholine in the central nervous system plays a critical role in modulating cognitive function and memory processes (5, 6). The reduction in cholinergic receptor density or binding affinity was more significant in older adults with mild cognitive impairment (MCI) and AD dementia (7) compared with cognitively unimpaired older adults. The complex molecular interaction of the cholinergic system, amyloid precursor protein, and proinflammatory cytokines modulates the formation of β-amyloid plaques and deposition, contributing more to the pathogenesis of Alzheimer's disease (8).
White matter hyperintensities (WMHs) in cholinergic pathways can also impair the integrity of the cholinergic network (9). WMHs are the most noticeable and easily accessible marker of small vessel diseases and cerebral amyloid angiopathy on MRI (10). The prevalence of WMH is highly associated with age and AD pathologies. A more severe WMH burden is associated with worse cognitive function, although this association weakened while controlling for age and other risk factors (11). Additionally, few data have demonstrated that greater dementia severity is associated with higher WMH burdens in cholinergic pathways compared with the whole brain (12, 13).
Among risk factors for AD, such as age, sex, and education, the APOE e4 allele is the strongest known genetic risk factor for Alzheimer's disease despite unclear neurostructural substrates (14, 15). Both heterozygous and homozygous e4 alleles increase the risk for AD dementia by 3–6- and 8–10-fold, respectively (16). The apolipoprotein e4 allele is associated with greater burdens of amyloid deposition (17), cerebral microbleeds (18), and white matter hyperintensities (19–21). Previous studies indicated that numerous factors may interact with APOE e4 synergically to facilitate the progression of WMH (22). The WMH and APOE e4 alleles may not directly influence each other but both contribute to cognitive impairment, especially memory loss, in AD dementia. An interactive effect of WMH and the APOE e4 gene on clinical cognitive impairment has been reported by some studies (23); however, the exact mechanism or etiology remains unclear. By analyzing the association between WMH in the cholinergic pathway and dementia severity among APOE e4 carriers and non-carriers primarily with memory problems, our goal is to examine the potential alterations on WMH that e4 alleles might contribute to in the formation of AD dementia syndrome.
In this study, we adopted and focused on the Cholinergic Pathways Hyperintensities Scale (CHIPS) (13, 24) to evaluate the degree of WMH in cholinergic pathways. As visual rating method counterparts, besides CHIPS, we also applied the Fazekas scale and medial temporal lobe atrophy (MTA) as predictive variables for dementia severity. In this study, we examined the association among three visual rating scores and dementia severity in APOE e4 carriers and non-carriers. We hypothesize that greater WMHs in cholinergic pathways would be associated with greater dementia severity and that the APOE e4 gene plays a facilitating role in the increased severity of clinical dementia.
2. Methods
2.1. Participants
From January 2018 to July 2022, patients aged 50–90 years with either subjective or objective memory impairment for more than 6 months were recruited from the memory clinic in Cardinal Tien Hospital, Taipei, Taiwan. Participants received brain MRI, completed standardized neuropsychological tests, and APOE genotyping. Age, years of education, and diagnoses were documented. After medical evaluation and laboratory testing, participants with any possible reversible causes of dementia were excluded, such as those with brain tumors, metabolic diseases, and psychiatric history. All participants provided written informed consent, and the study was approved by the Research Ethics Committee/Institutional Review Board of Cardinal Tien Hospital, Taipei, Taiwan (CTH-110-2-1-014).
2.2. Neuropsychological tests
In this study, to evaluate general objective cognitive function, a unified standardized Mandarin Chinese version of the Mini-Mental Status Examination (MMSE) (25) was performed on each patient during the first clinic encounter. MMSE served as a baseline cognitive evaluation for the diagnosis of dementia.
The functional severity of dementia was evaluated by the global Clinical Dementia Rating (CDR) scale and the Clinical Dementia Rating scale Sum of Boxes (CDR-SB). The evaluation of CDR was based on five levels (0, 0.5, 1, 2, and 3) in six domains (memory, orientation, judgment, problem solving, community affairs, home and hobbies, and personal care). The CDR-SB score was calculated by adding six domains of functioning scores.
Compared to MMSE, CDR-SB incorporates evaluations on the domain of self-care, social impairment, and daily function, and is better at detecting prodromal dementia (26). As opposed to global CDR, CDR-SB provides a quantitative evaluation of impairment and can be treated as interval data in statistical analysis rather than the global CDR scale (27). In this study, CDR-SB is the primary outcome variable that we analyzed for dementia severity. All clinical information was provided by the caregivers and occasionally the patients themselves at the first clinic encounter to avoid the influence of medical treatment.
2.3. Diagnosis of dementia
Participants with subjective or objective memory impairment for more than 6 months were recruited from the memory clinic using the 2011 National Institute on Aging—Alzheimer's Association (NIA-AA) criteria (28). Participants with a global CDR scale of 0 are classified as normal participants; subjects with a CDR score of 0.5 are classified as having mild cognitive impairment (MCI); and subjects with a CDR score of 1 or 2 are diagnosed with probable Alzheimer's disease.
2.4. Brain MRI acquisition and visual rating scale in our study
All participants received brain MRI (GE, 3T DISCOVERY 750, GE Taiwan) with trans-axial T2 weighted scans, and 3D fluid-attenuated inversion recovery images and high-resolution sagittal T1-weighted images were obtained. We minimized the variations in MRI machines by using the same MRI machine at Cardinal Tien Hospital during participant enrollment. The image analysis included three visual rating methods that are described as follows.
2.5. Evaluation of white matter hyperintensities
The periventricular white matter hyperintensities measured based on the Fazekas scale were graded and evaluated by the author in T2-FLAIR axial view (29, 30). The periventricular Fazekas score (PV Fazekas) was rated on a 4-point scale as follows: 0, absent; 1, mild; 2, moderate; and 3, severe; in addition, deep white matter Fazekas score (DWM) was evaluated on the same 4-point scale as an alternative to white matter hyperintensity visual rating scores.
2.6. Evaluation of cholinergic pathway hyperintensities scale
White matter hyperintensities in cholinergic pathways were graded visually using the Cholinergic Pathways HyperIntensity Scale (CHIPS) by the first author on a single-rater basis. First reported by Bocti et al. (24), CHIPS is a visual rating scale developed based on published immunohistochemical tracings of the cholinergic pathways in humans (24, 31), and was previously used by some studies for the relationship between WMH and cognition (12, 13). According to Selden (31), the cholinergic pathways include the medial pathway and lateral pathway. The medial pathway is closely associated with the adjacent cingulate gyrus and rostrum of the corpus callosum; the lateral pathway courses through the external capsule and claustrum within the white matter (31). Accordingly, four axial planes of T2-FLAIR images were identified by major anatomical landmarks—low external capsule, high external capsule, corona radiata, and centrum semiovale (Figure 1). Medial pathway is included in two of the axial planes as anterior cingulate gyrus and posterior cingulate gyrus. A total of 10 regions are illustrated in Figures 1A–D. White matter hyperintensity of each region was determined visually on a 3-point scale for each region (0 = normal; 1 = minimal; 2 = confluent or moderate to severe). To account for the decreasing concentration of cholinergic fibers, each slice was weighted sequentially from 1 to 4 with one being the centrum semiovale and four being the lower external capsules (Table 1). The total CHIPS score (both hemispheres) ranged from 1 to 100. The lowest CHIPS score is 0, indicating no burden of WMH in cholinergic pathways, and the highest CHIPS score is 100 (24). The corresponding author independently rated CHIPS scores of random 65 participants to ascertain the inter-rater reliability of CHIPS. Controversial images were rated based on the consensus of the first author, the corresponding author, and a radiologist (Cheng-Feng Ho). The consensus CHIPS scores were used in our regression analyses. Intra-rater reliability was calculated by two independent ratings of the first author. The inter-rater reliability and intra-rater reliability were analyzed by inter-class correlation coefficient (ICC).
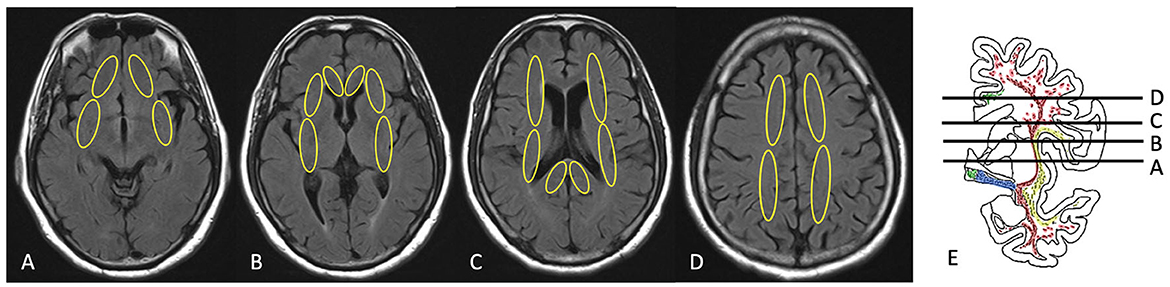
Figure 1. Illustration of CHIPS scoring on brain MRI. (A) Low external capsule, (B) high external capsule and anterior cingulate gyrus, (C) corona radiata and posterior cingulate gyrus, (D) centrum semiovale, (E) coronal view [immunohistochemical tracings of the cholinergic pathways with levels for selected slices (A–D) presented in the axial plane; drawing from Selden (31)].
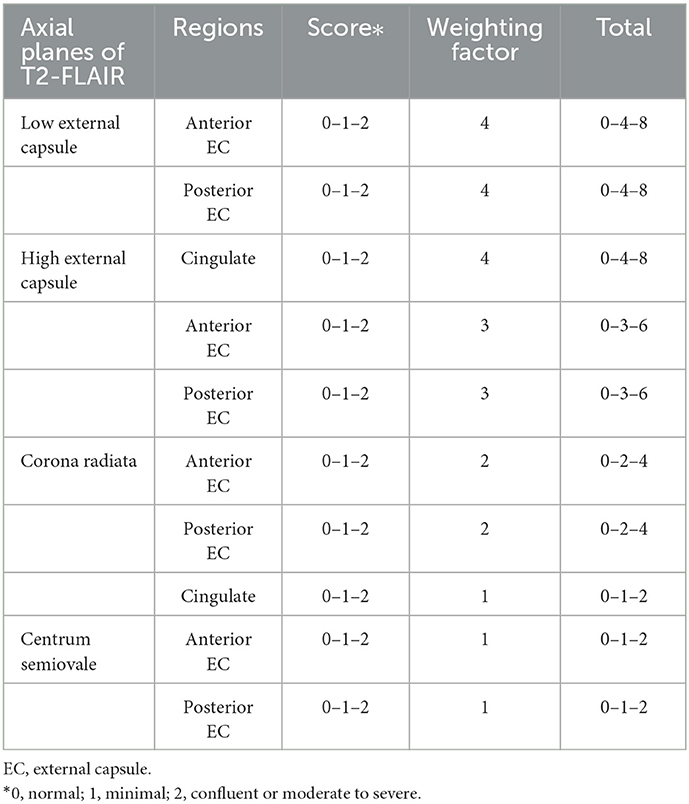
Table 1. The Cholinergic Pathways Hyperintensities Scale (CHIPS) evaluation of unilateral hemisphere.
2.7. Evaluation of medial temporal lobe atrophy
Medial temporal lobe atrophy, also known as the Scheltens' scale, is a sign of neurodegenerative disease and a strong predictor of clinical cognitive impairment (32, 33). MTA was evaluated by the first author in the coronal cut through T1-weighted images, and was rated on a 5-point scale based on the height of the hippocampal formation and the width of the choroid fissure and the temporal horn as follows: 0, absent; 1, minimal; 2, mild; 3, moderate; and 4, severe (32). The MTA score was applied to both the right and left medial temporal lobes, separately. In our study, both sides were summed to determine the degree of atrophy.
2.8. Statistical analyses
All analyses were performed using STATA version 16 (College Station, TX, USA). Among the two groups, including carriers and non-carriers, demographic variables were compared using two-tailed Student's t-test for continuous variables, the chi-square independent test for categorical variables, and Fisher's exact test as appropriate. Pearson's correlations were analyzed between different visual rating scores. An alpha of 0.05 was set for statistical significance. General cognition was measured using the MMSE. The MMSE score and CDR-SB were used to compare cognition in APOE e4 carriers and non-carriers at baseline. For analyses, we used CDR-SB as the primary outcome variable due to its detailed quantitative nature for dementia severity scores. Additional regression analyses using MMSE as an outcome variable were performed as a comparison to CDR-SB.
First, we tested associations between visual rating scores and dementia severity using simple linear regression in APOE e4 carriers and non-carriers, separately. Second, multiple well-studied dementia predictors, such as age and years of education, were used to assess the effects of WMH in cholinergic pathways on CDR-SB scores. We performed multiple linear regressions for CHIPS, Fazekas scale, and MTA in separate regression models, further including their interactions with APOE e4 status as predictors. In previous literature, more evidence suggests that periventricular white matter lesions, rather than deep white matter hyperintensities, are associated with cognitive impairment (34). We choose periventricular Fazekas scale as a visual rating scale counterpart for CHIPS due to their anatomical overlaps and higher association with cognitive performance. CDR-SB was the primary outcome variable. In all models, age, sex, and education were included as predictor variables. Regression Model 0 exclusively includes age, sex, and education as predictive factors. Due to the high correlation between visual rating scores, CHIPS, MTA, and the Fazekas scale were separately added to Model 0 to decrease statistical collinearity. In Model 1, CHIPS and CHIPS*e4 status were included as predictors. Model 2 included the Fazekas scale and Fazekas*e4 status as predictors. Model 3 included the MTA scores and MTA*e4 status as predictors. In Model 4, we included CHIPS scores, CHIPS*e4 status, and MTA scores despite the potential collinearity of CHIPS and MTA, for MTA and CHIPS are two distinctive visual rating measurements anatomically and etiologically. Third, additional multiple linear regression models using MMSE as the outcome variable were analyzed in a similar manner with predictive variables (CHIPS, PV Fazekas, MTA) respectively added into models.
3. Results
3.1. Demographic data and image evaluation
The demographic data of APOE e4 carriers and non-carriers are presented in Table 2. Of the 166 subjects, 49 were APOE e4 carriers (29.5%), and 117 were non-carriers (70.5%). Among the 49 e4 carriers, 44 had the e3e4 genotype, 3 had the e4e4 genotype, and 2 had the e2e4 genotype. The diagnoses included Alzheimer's disease (AD, 22.891%, n = 38), mild cognitive impairment (MCI, 47.590%, n = 79), and normal (29.518%, n = 49). In ADs, 19 subjects were e4 carriers, and 19 subjects were non-carriers. For MCIs, 19 subjects were e4 carriers, and 62 subjects were non-carriers. In total, 13 normal participants were carriers, and 36 were non-carriers. Fisher's exact test revealed an association between participants' diagnoses and the APOE e4 allele (Fisher's exact test p-value = 0.008). Age, sex, and years of education did not differ between APOE e4 carriers and non-carriers. The average MMSE of the carriers was significantly lower than that of the non-carriers (Student's t-test p-value = 0.003). Regarding global CDR scores, the numbers of APOE e4 carriers and non-carriers differed significantly (Fisher's exact test p-value = 0.009). Higher CDR-SB scores were observed in carriers than in non-carriers (Student's t-test p-value < 0.001). Regarding visual rating scores, the mean periventricular Fazekas scores were significantly lower in carriers (Student's t-test p-value = 0.038); carriers also have a lower mean of deep white matter Fazekas scale without statistical significance (Student's t-test p-value = 0.232). Neither CHIPS nor MTA scores exhibited between-group differences. In all participants, there is a strong correlation between periventricular Fazekas scale and deep white matter Fazekas scale (Pearson's r = 0.693, p-value < 0.001). Either periventricular or DWM Fazekas scale presented high correlations with CHIPS. There is a small to mediate correlation between CHIPS and MTA in our participants (Pearson's r = 0.285, p-value < 0.001). For total CHIPS scores, the inter-rater reliability (ICC = 0.934, 95% confidence interval 0.894 < ICC < 0.959) and intra-rater reliability (ICC = 0.985, 95% confidence interval 0.975 < ICC < 0.991) were both high.
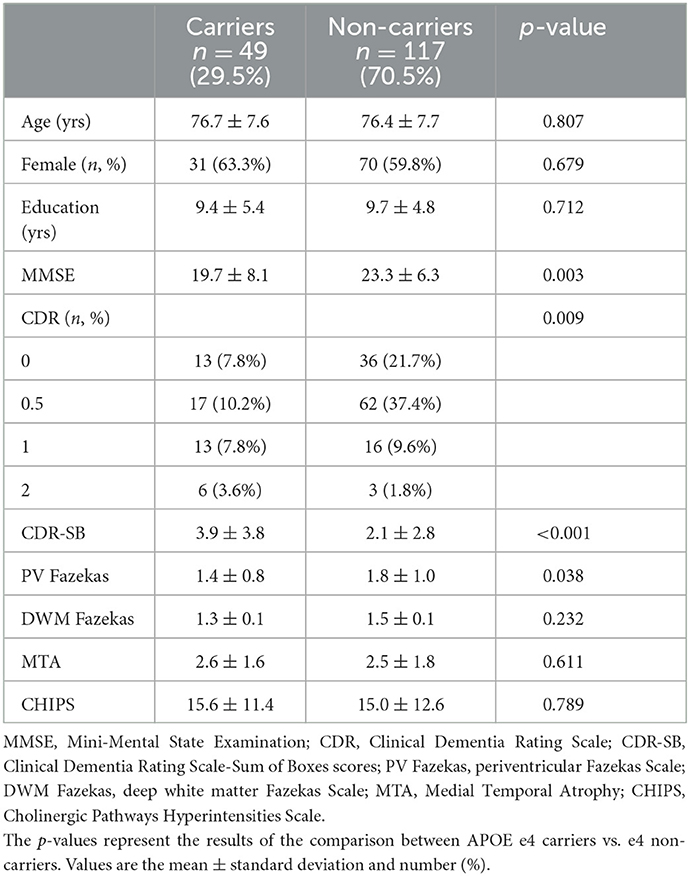
Table 2. Demographic and clinical data in two groups of study participants defined as carriers and non-carriers based on APOE e4 genotype.
3.2. Results of simple linear regression and multiple linear regression analyses
Our findings of correlations for all participants indicated that CDR-SB correlates with MTA (standardized beta coefficient = 0.322, p-value < 0.001) and that CDR-SB is not correlated with CHIPS (Figure 2). However, when assessing CHIPS and CDR-SB separately in the APOE e4 carrier group and the non-carrier group, two distinct correlations are noted (Figure 3). CHIPS scores were associated with CDR-SB scores (p-value = 0.027) in the carriers but not in non-carriers. No significant associations between Fazekas scores and CDR-SB scores were observed in either carriers (p-value = 0.409) or non-carriers (p-value = 0.739).
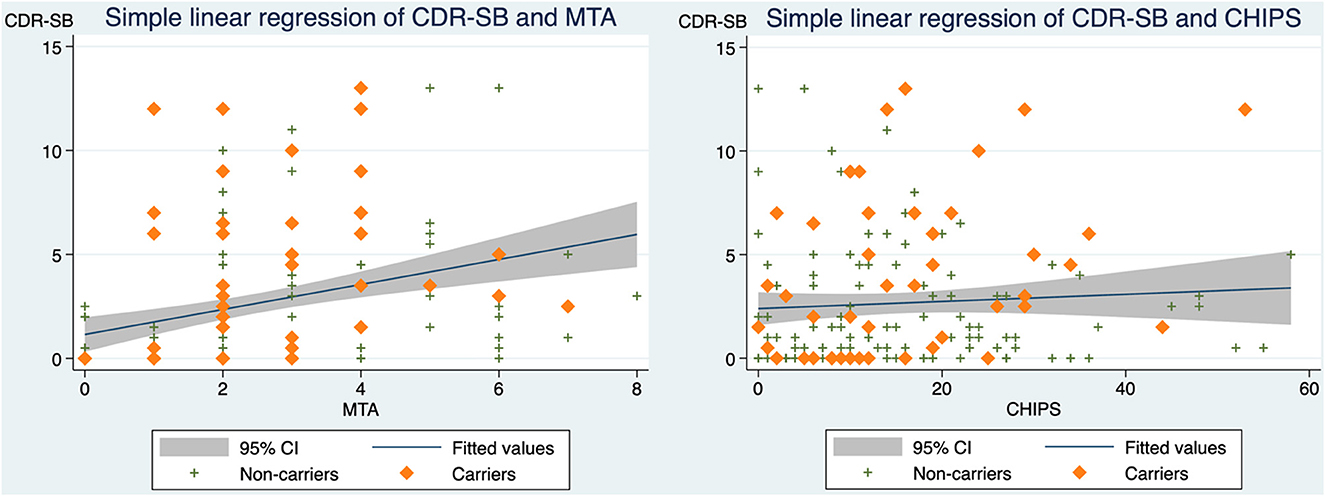
Figure 2. The correlations between CDR-SB and MTA as well as CDR-SB and CHIPS in all participants. (Left) Scatterplot depicting CDR-SB scores and MTA with a simple linear regression line and 95% confidence interval (standardized beta coefficient = 0.322, R2 = 0.104, adjusted R2 = 0.098, p-value < 0.001). (Right) Scatterplot depicting CDR-SB scores and CHIPS scores with a simple linear regression line and 95% confidence interval (standardized beta coefficient = 0.066, R2 = 0.004, adjusted R2 = −0.002, p-value = 0.397). CDR-SB, clinical dementia rating scale-sum of boxes scores; MTA, medial temporal atrophy; CHIPS, Cholinergic Pathways Hyperintensities Scale.
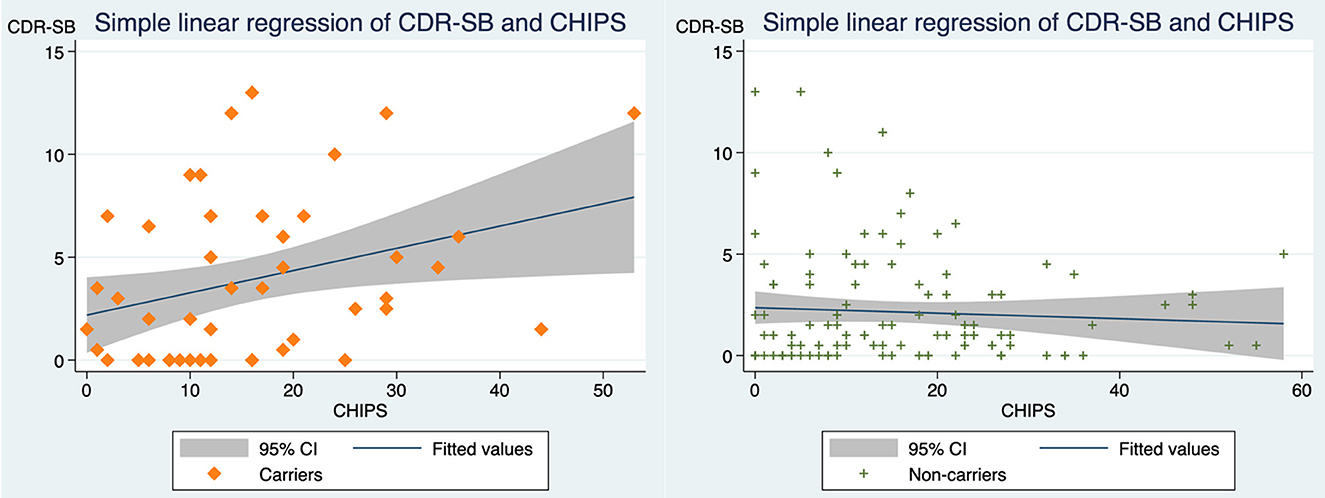
Figure 3. The correlations between CDR-SB and CHIPS in APOE4 carriers vs. non-carriers. (Left) In carriers, scatterplot depicting CDR-SB scores and CHIPS scores with a simple linear regression line and 95% confidence interval (standardized beta coefficient = 0.319, R2 = 0.101, adjusted R2 = 0.082, p-value = 0.026). (Right) In non-carriers, scatterplot depicting CDR-SB scores and CHIPS with simple linear regression line and 95% confidence interval (standardized beta coefficient = −0.062, R2 = 0.004, adjusted R2 = −0.005, p-value = 0.509). CDR-SB, clinical dementia rating scale-sum of boxes scores; CHIPS, Cholinergic Pathways Hyperintensities Scale; Carriers, APOE e4 heterozygous or homozygous carriers; Non-carriers, APOE e4 non-carriers.
Multiple linear regression analyses, using CDR-SB as the outcome variable, revealed that older age was a significant predictive factor in Models 0, 1, 2, 3, and 4 (Table 3). Model 1 analysis indicated that CHIPS was not significantly associated with CDR-SB, but an interaction of APOE e4 on CHIPS was demonstrated to be significant (p-value = 0.044). In Model 1, a greater CHIPS score was associated with higher CDR-SB in APOE e4 carriers only (beta = 0.064, p-value = 0.097); no such associations were observed in non-carriers (beta = −0.024, p-value = 0.270). In Model 2, the Fazekas scale showed no significance in prediction and no interaction with APOE e4. Model 3 indicated that the MTA score was significantly predictive of CDR-SB in all participants (p-value = 0.008) without an interactive effect between the MTA and e4 alleles (p-value = 0.427). In Model 4, where both CHIPS and MTA were included into regression analysis, the significant predictive factors are age, MTA, and the APOE e4 interactions on CHIPS (p-value = 0.043).
Multiple linear regression analyses, using MMSE as the outcome variable, suggested that age and education were significantly predictive in all models. In the model that includes MTA, MTA demonstrated significant predictive effect for MMSE. There was no APOE e4 interactions in any of the models using MMSE as the outcome variable. Neither CHIPS nor Fazekas scale had predictive power for MMSE.
4. Discussion
This is a cross-sectional study comparing the association between white matter hyperintensities in cholinergic pathways and the severity of dementia among two groups, namely, apolipoprotein e4 carriers and non-carriers. Our findings suggest two distinct associations between WMHs and clinical dementia severity across carriers and non-carriers. Under the context of a similar demographic background, the e4 carriers presented lower MMSE and higher CDR-SB scores, which is consistent with previous literature indicating that the e4 phenotype is associated with greater dementia severity (35–37). Other visual rating scores (CHIPS, MTA score, periventricular Fazekas scale, and DWM Fazekas scale) were analyzed by correlation and regression models. This finding suggests that CDR-SB correlates with WHMs in cholinergic pathways exclusively in e4 carriers and not in non-carriers. An interaction for the APOE e4 gene between dementia severity and CHIPS was noted.
The average CHIPS values of the two study groups were not significantly different, indicating that the degree of white matter changes in cholinergic pathways is visually similar among carriers and non-carriers. CHIPS alone does not demonstrate a clear and significant association with dementia severity (CDR-SB) as the MTA score does. Next, we separated participants into e4 carriers and non-carriers. We noticed that it is only in the carriers that present significant correlations between WMHs in cholinergic pathway and dementia severity as depicted in Figure 3. After adjusting for age, sex, and education in our regression models, CHIPS is only predictive for CDR-SB with the facilitating role of APOE e4. Even when MTA was added as an additional predictive variable, the e4 interaction is still significant for CHIPS and CDR-SB. Namely, for carriers, more WMH burden in cholinergic pathway associates with worse CDR-SB. CHIPS has no predictive role for CDR-SB for non-carriers. A similar result on WMHs was demonstrated by Mirza et al. (23), where general WMH volumes were associated with worse cognitive performance exclusively in APOE e4 carriers but not in non-carriers. Apolipoprotein E is one of the primary apolipoproteins in the lipid metabolism of the central nervous system (CNS) (38). Its role in lipid homeostasis and its anti-inflammatory effects have been investigated and supported for decades (17). Neuroinflammation is one of the key pathogenic factors in Alzheimer's disease (39). In e4 carriers, the increased level of inflammation may cause more covert damage to the neurons and nerve tracts, leading to earlier or worse dementia presentation (23, 40, 41).
In our analysis, both periventricular Fazekas and deep white matter Fazekas were correlated with CHIPS. Periventricular Fazekas score is the predictive variable we used in our regression models, yet in our analyses, neither periventricular Fazekas scale nor DWM Fazekas scale had predictive power for clinical dementia severity in either group. The Fazekas scale provides a general estimation of white matter change by grading the brain as a whole entity by a scale of 0–3 (30), but it does not compare or separate cerebral regions based on their functionality. This may limit its clinical utility due to non-specificity. Of note, in our analysis, the non-carrier group with better cognitive performance exhibits higher Fazekas scores. This finding suggests that WHMs in specific pathways instead of WMHs in general may be a better indicator of clinical dementia severity (42). In addition to the Fazekas scale, another commonly used WMH visual measurement, the Age-Related White Matter Change (ARWMC) score, has not been routinely applied as a clinical predictor for clinical dementia severity. This scale separates both the left and right hemispheres into five anatomical regions with a total sum-up score of 0–30 (43, 44). Although ARWMCs are easy and more detailed to apply to radiological images, this score still separates brain regions anatomically instead of functionally.
It is current knowledge that white matter changes are etiologically heterogeneous but present similar signals under brain magnetic resonance (10). Hyperintensities on MRI T2 FLAIR represent subtle water content differences from the “non-hyperintense” regions, which can result from different etiologies, such as small vessel diseases, microbleeds, ischemia, axonal loss, or demyelination (16). Over decades, measurements of white matter lesions have evolved from visual rating methods to semi-automated volumetry to tractography. Some evidence suggests high reliability and more efficacy of semi-automatic volume measurement than visual rating methods (45, 46). We used visual rating scale in this study, CHIPS in particular, which was validated by high correlation to the volumetric analysis of the cholinergic pathways (24). CHIPS evaluates hyperintensity signals in cholinergic pathways within the white matter. Previous DTI investigation substantiated the correlation between cognitive impairment and volume reduction or integrity reduction of cholinergic tracts (47–49). However, evidence of nerve tract atrophy or damage can not necessarily be interpreted as definite white matter signal changes or vice versa. The fact that carriers and non-carriers present similar CHIPS scores but only carriers demonstrate stronger clinical correlation further corroborates the heterogeneity of WMH and shed a light on possible alterations in WMH formation caused by e4 alleles.
Based on different models of multiple linear regression, MTA was identified as a significant independent variable for dementia severity in both carriers and non-carriers. The e4 interaction with MTA was not observed in the MTA models. Medial temporal lobe atrophy has long been demonstrated to be associated with amnestic cognitive impairment, and its correlation to Alzheimer's disease severity is substantial (32, 33). Nonetheless, dementia results from a multifactorial process. It is also known that MTA per se is insufficient to predict Alzheimer's pathologic change (50). In addition to neurodegeneration, other factors also contribute to clinical cognitive impairment, such as years of education, hearing impairment, and APOE genotype (51). The findings of this study suggest different pathogenicities for clinical dementia severity across e4 carriers and non-carriers. Regardless of neurodegeneration, we assume that the involvement of the e4 gene alters the formation of WMHs. With the e4 alleles conduces worse lipid metabolism and increased CNS inflammation, white matter lesions are more detrimental and destructive to neurons in carriers. Consequently, the destruction of cholinergic pathways manifests clinically as greater dementia severity. In our study population, two primary distinct pathoetiologies of WMH formation are proposed. For carriers, white matter lesions may, to a greater degree, be derived from neuronal inflammation, lipid metabolic dysfunction, and amyloid angiopathy; for non-carriers, WMHs could be the result of aging.
One of the limitations of this study involves the use of a visual rating method for WMHs. Despite high inter-/intra-rater reliability and time-saving characteristics, visual measurement in neuroimaging is still subjective and qualitative in nature. Some studies have indicated that visual rating methods are less sensitive to WMH volume measurements (52). Although Bocti et al. (24), has demonstrated high correlation of CHIPS and semi-automatic volumetric measurement, more objective methods may be adopted for future studies. The application of semi-automatic WMH volume measuring in cholinergic pathways may be worth investigating across e4 carriers and non-carriers. Further analysis comparing DTI and white matter signals in cholinergic pathways, examining e4 status and clinical severity, would be valuable for the etiology in clinical dementia syndrome. Additional limitation of this study is the absence of biomarker information from our participants, such as CSF study, amyloid PET, and tau distribution. It is possible that a proportion of patients in the normal group and MCI group eventually reflect a non-AD pathology. Other limitations of this study include the cross-sectional study design and the clinic-based recruitment. A community-based longitudinal cohort study design may further examine the temporal and causal relationships with less selection bias. Further community-based cohort study to examine the associations between WMHs in cholinergic pathways and clinical dementia staging would be conducive.
5. Conclusions
This study demonstrates the presence of an APOE e4 gene interaction on the associations between dementia severity (CDR-SB) and WMHs in cholinergic pathways. More white matter lesions in cholinergic pathways correlate with greater dementia severity in e4 carriers but not in non-carriers. This finding supports the importance of the cholinergic system in cognitive performance, the heterogeneity of WMHs in neuroimaging, and the lipid homeostasis and anti-inflammatory effects of apolipoprotein e4. In e4 carriers, due to possible e4 alterations in WMH formation, it is possible that WMHs are more detrimental, so lesions on the cholinergic pathways present greater dementia severity. General WMH gradings that do not consider functionality, such as the Fazekas scale or ARWMC, may play a less significant role in clinical symptoms in subjects with MCI and AD. After adjusting for age and education, CHIPS was only predictive for CDR-SB under APOE e4 interaction. The role of the APOE e4 genotype in cognitive outcome requires further investigation.
Data availability statement
The raw data supporting the conclusions of this article will be made available by the authors, without undue reservation.
Ethics statement
The studies involving human participants were reviewed and approved by the Institutional Review Board of Cardinal Tien Hospital, Taipei, Taiwan (IRB: CTH-110-2-1-014). The patients were given a complete description of the study, and each patient signed an informed consent form. The patients/participants provided their written informed consent to participate in this study.
Author contributions
M-CY, Y-FC, C-JC, and Y-CL contributed to manuscript writing and revision. M-CY and S-CW contributed to preparing and sorting the data. M-CY, Y-CL, and C-FH contributed to interpreting the neuroimages. All authors have read and approved the final manuscript.
Funding
Funding for this project was obtained from the Ministry of Science and Technology of Taiwan (MOST 110-2321-B-418-001) and Cardinal Tien Hospital (CTH-110-2-1-014).
Conflict of interest
The authors declare that the research was conducted in the absence of any commercial or financial relationships that could be construed as a potential conflict of interest.
Publisher's note
All claims expressed in this article are solely those of the authors and do not necessarily represent those of their affiliated organizations, or those of the publisher, the editors and the reviewers. Any product that may be evaluated in this article, or claim that may be made by its manufacturer, is not guaranteed or endorsed by the publisher.
Supplementary material
The Supplementary Material for this article can be found online at: https://www.frontiersin.org/articles/10.3389/fneur.2023.1100322/full#supplementary-material
Abbreviations
MCI, mild cognitive impairment; MRI, magnetic resonance imaging; WMHs, white matter hyperintensities; CHIPS, Cholinergic Pathways Hyperintensities Scale; Fazekas, Fazekas Scale; MTA, medial temporal lobe atrophy, Schelten scale; ARWMC, age-related white matter change; MMSE, mini-mental status examination; CDR, clinical dementia rating; CDR-SB, clinical dementia rating-sum of boxes; APOE, apolipoprotein E.
References
1. Alzheimer's Association. 2018 Alzheimer's disease facts and figures. Alzheimer's Dementia. (2018) 14:367–429. doi: 10.1016/j.jalz.2018.02.001
2. Wu Y, Brayne C, Matthews FE. Prevalence of dementia in East Asia: a synthetic review of time trends. Int J Geriatr Psychiatry. (2015) 30:793–801. doi: 10.1002/gps.4297
3. Bierer LM, Haroutunian V, Gabriel S, Knott PJ, Carlin LS, Purohit DP, et al. Neurochemical correlates of dementia severity in Alzheimer's disease: relative importance of the cholinergic deficits. J Neurochem. (2002) 64:749–60. doi: 10.1046/j.1471-4159.1995.64020749.x
4. Mesulam M. The cholinergic lesion of Alzheimer's Disease: pivotal factor or side show? Learn Mem. (2004) 11:43–9. doi: 10.1101/lm.69204
5. Schliebs R, Arendt T. The significance of the cholinergic system in the brain during aging and in Alzheimer's disease. J Neural Transm. (2006) 113:1625–44. doi: 10.1007/s00702-006-0579-2
6. Schliebs R. Basal forebrain cholinergic dysfunction in Alzheimer's disease: interrelationship with β-amyloid, inflammation and neurotrophin signaling. Neurochem Res. (2005) 30:895–908. doi: 10.1007/s11064-005-6962-9
7. Sultzer DL, Lim AC, Gordon HL, Yarns BC, Melrose RJ. Cholinergic receptor binding in unimpaired older adults, mild cognitive impairment, and Alzheimer's disease dementia. Alz Res Therapy. (2022) 14:25. doi: 10.1186/s13195-021-00954-w
8. Kar S, Slowikowski SPM, Westaway D, Mount HTJ. Interactions between β-amyloid and central cholinergic neurons: implications for Alzheimer's disease. J Psychiatry Neurosci. (2004) 29:427–41.
9. Liu Q, Zhu Z, Teipel SJ, Yang J, Xing Y, Tang Y, et al. White matter damage in the cholinergic system contributes to cognitive impairment in subcortical vascular cognitive impairment, no dementia. Front Aging Neurosci. (2017) 9:47. doi: 10.3389/fnagi.2017.00047
10. Wardlaw JM, Smith EE, Biessels GJ, Cordonnier C, Fazekas F, Frayne R, et al. Neuroimaging standards for research into small vessel disease and its contribution to ageing and neurodegeneration. Lancet Neurol. (2013) 12:822–38. doi: 10.1016/S1474-4422(13)70124-8
11. Arfanakis K, Evia AM, Leurgans SE, Cardoso LFC, Kulkarni A, Alqam N, et al. Neuropathologic correlates of white matter hyperintensities in a community-based cohort of older adults. J Alzheimer's Dis. (2020) 73:333–45. doi: 10.3233/JAD-190687
12. Kimura Y, Sato N, Ota M, Maikusa N, Maekawa T, Sone D, et al. A structural MRI study of cholinergic pathways and cognition in multiple sclerosis. eNeurologicalSci. (2017) 8:11–6. doi: 10.1016/j.ensci.2017.06.008
13. Shin J, Choi S, Lee JE, Lee HS, Sohn YH, Lee PH. Subcortical white matter hyperintensities within the cholinergic pathways of Parkinson's disease patients according to cognitive status. J Neurol Neurosurg Psychiatry. (2012) 83:315–21. doi: 10.1136/jnnp-2011-300872
14. Tsuang D, Leverenz JB, Lopez OL, Hamilton RL, Bennett DA, Schneider JA, et al. APOE ϵ4 increases risk for dementia in pure synucleinopathies. JAMA Neurol. (2013) 70:223. doi: 10.1001/jamaneurol.2013.600
15. Lutz MW, Crenshaw DG, Saunders AM, Roses AD. Genetic variation at a single locus and age of onset for Alzheimer's disease. Alzheimer's Dementia. (2010) 6:125–31. doi: 10.1016/j.jalz.2010.01.011
16. Querfurth HW, LaFerla FM. Alzheimer's disease. N Engl J Med. (2010) 362:329–44. doi: 10.1056/NEJMra0909142
17. Belloy ME, Napolioni V, Greicius MD. A quarter century of APOE and Alzheimer's disease: progress to date and the path forward. Neuron. (2019) 101:820–38. doi: 10.1016/j.neuron.2019.01.056
18. Schilling S, DeStefano AL, Sachdev PS, Choi SH, Mather KA, DeCarli CD, et al. APOE genotype and MRI markers of cerebrovascular disease: systematic review and meta-analysis. Neurology. (2013) 81:292–300. doi: 10.1212/WNL.0b013e31829bfda4
19. Lyall DM, Cox SR, Lyall LM, Celis-Morales C, Cullen B, Mackay DF, et al. Association between APOE e4 and white matter hyperintensity volume, but not total brain volume or white matter integrity. Brain Imag Behav. (2020) 14:1468–76. doi: 10.1007/s11682-019-00069-9
20. Sudre CH, Cardoso MJ, Frost C, Barnes J, Barkhof F, Fox N, et al. APOE ε4 status is associated with white matter hyperintensities volume accumulation rate independent of AD diagnosis. Neurobiol Aging. (2017) 53:67–75. doi: 10.1016/j.neurobiolaging.2017.01.014
21. Rojas S, Brugulat-Serrat A, Bargalló N, Minguillón C, Tucholka A, Falcon C, et al. Higher prevalence of cerebral white matter hyperintensities in homozygous APOE-ε4 allele carriers aged 45–75: results from the ALFA study. J Cereb Blood Flow Metab. (2018) 38:250–61. doi: 10.1177/0271678X17707397
22. Cox SR, Ritchie SJ, Dickie DA, Pattie A, Royle NA, Corley J, et al. Interaction of APOE e4 and poor glycemic control predicts white matter hyperintensity growth from 73 to 76. Neurobiol Aging. (2017) 54:54–8. doi: 10.1016/j.neurobiolaging.2017.02.014
23. Mirza SS, Saeed U, Knight J, Ramirez J, Stuss DT, Keith J, et al. APOE ε4, white matter hyperintensities, and cognition in Alzheimer and Lewy body dementia. Neurology. (2019) 93:e1807–19. doi: 10.1212/WNL.0000000000008377
24. Bocti C, Swartz RH, Gao FQ, Sahlas DJ, Behl P, Black SE, et al. new visual rating scale to assess strategic white matter hyperintensities within cholinergic pathways in dementia. Stroke. (2005) 36:2126–31. doi: 10.1161/01.STR.0000183615.07936.b6
25. Shyu YIL, Yip PK. Factor structure and explanatory variables of the mini-mental state examination (MMSE) for elderly persons in Taiwan. J Formos Med Assoc. (2001) 100:8.
26. Balsis S, Benge JF, Lowe DA, Geraci L, Doody RS. How do scores on the ADAS-Cog, MMSE, and CDR-SOB correspond? Clin Neuropsychol. (2015) 29:1002–9. doi: 10.1080/13854046.2015.1119312
27. O'Bryant SE. Staging dementia using clinical dementia rating scale sum of boxes scores: a Texas Alzheimer's research consortium study. Arch Neurol. (2008) 65:1091. doi: 10.1001/archneur.65.8.1091
28. McKhann GM, Knopman DS, Chertkow H, Hyman BT, Jack CR, Kawas CH, et al. The diagnosis of dementia due to Alzheimer's disease: recommendations from the National Institute on Aging-Alzheimer's Association workgroups on diagnostic guidelines for Alzheimer's disease. Alzheimer's Dementia J Alzheimer's Assoc. (2011) 7:263–9. doi: 10.1016/j.jalz.2011.03.005
29. Kim KW, MacFall JR, Payne ME. Classification of white matter lesions on magnetic resonance imaging in elderly persons. Biol Psychiatry. (2008) 64:273–80. doi: 10.1016/j.biopsych.2008.03.024
30. Fazekas F, Chawluk J, Alavi A, Hurtig H, Zimmerman R. MR signal abnormalities at 15 T in Alzheimer's dementia and normal aging. Am J Roentgenol. (1987) 149:351–6.
31. Selden N. Trajectories of cholinergic pathways within the cerebral hemispheres of the human brain. Brain. (1998) 121:2249–57.
32. Duara R, Loewenstein DA, Potter E, Appel J, Greig MT, Urs R, et al. Medial temporal lobe atrophy on MRI scans and the diagnosis of Alzheimer disease. Neurology. (2008) 71:1986–92. doi: 10.1212/01.wnl.0000336925.79704.9f
33. Torisson G, van Westen D, Stavenow L, Minthon L, Londos E. Medial temporal lobe atrophy is underreported and may have important clinical correlates in medical inpatients. BMC Geriatr. (2015) 15:65. doi: 10.1186/s12877-015-0066-4
34. Griffanti L, Jenkinson M, Suri S, Zsoldos E, Mahmood A, Filippini N, et al. Classification and characterization of periventricular and deep white matter hyperintensities on MRI: a study in older adults. Neuroimage. (2018) 170:174–81. doi: 10.1016/j.neuroimage.2017.03.024
35. Farrer LA. Effects of age, sex, and ethnicity on the association between apolipoprotein E genotype and Alzheimer disease. A meta-analysis APOE and Alzheimer disease meta analysis consortium. JAMA J Am Med Assoc. (1997) 278:1349–56.
36. Corder EH. Gene dose of apolipoprotein E type 4 allele and the risk of Alzheimer's disease in late onset families. Science. (1993) 261:921–3.
37. Evans S, Dowell NG, Tabet N, Tofts PS, King SL, Rusted JM. Cognitive and neural signatures of the APOE E4 allele in mid-aged adults. Neurobiol Aging. (2014) 35:1615–23. doi: 10.1016/j.neurobiolaging.2014.01.145
38. Pitas RE, Boyles JK, Lee SH, Hui D, Weisgraber KH. Lipoproteins and their receptors in the central nervous system. Characterization of the lipoproteins in cerebrospinal fluid and identification of apolipoprotein B,E(LDL) receptors in the brain. J Biol Chem. (1987) 262:14352–60.
39. Broussard GJ, Mytar J, Li R, Klapstein GJ. The role of inflammatory processes in Alzheimer's disease. Inflammopharmacology. (2012) 20:109–26. doi: 10.1007/s10787-012-0130-z
40. Rebeck GW. The role of APOE on lipid homeostasis and inflammation in normal brains. J Lipid Res. (2017) 58:1493–9. doi: 10.1194/jlr.R075408
41. Ringman JM, Elashoff D, Geschwind DH, Welsh BT, Gylys KH, Lee C, et al. Plasma signaling proteins in persons at genetic risk for Alzheimer disease: influence of APOE genotype. Arch Neurol. (2012) 69:757–64. doi: 10.1001/archneurol.2012.277
42. Behl P, Bocti C, Swartz RH, Gao F, Sahlas DJ, Lanctot KL, et al. Strategic subcortical hyperintensities in cholinergic pathways and executive function decline in treated Alzheimer patients. Arch Neurol. (2007) 64:266. doi: 10.1001/archneur.64.2.266
43. Kapeller P, Barber R, Vermeulen RJ, Adèr H, Scheltens P, Freidl W, et al. Visual rating of age-related white matter changes on magnetic resonance imaging: scale comparison, interrater agreement, and correlations with quantitative measurements. Stroke. (2003) 34:441–5. doi: 10.1161/01.STR.0000049766.26453.E9
44. de Leeuw FE. Progression of cerebral white matter lesions in Alzheimer's disease: a new window for therapy? J Neurol Neurosurg Psychiatry. (2005) 76:1286–8. doi: 10.1136/jnnp.2004.053686
45. Iorio M, Spalletta G, Chiapponi C, Luccichenti G, Cacciari C, Orfei MD, et al. White matter hyperintensities segmentation: a new semi-automated method. Front Aging Neurosci. (2013) 5:76. doi: 10.3389/fnagi.2013.00076
46. Andere A, Jindal G, Molino J, Collins S, Merck D, Burton T, et al. Volumetric white matter hyperintensity ranges correspond to fazekas scores on brain. MRI J Stroke Cerebrovasc Dis. (2002) 31:106333. doi: 10.1016/j.jstrokecerebrovasdis.2022.106333
47. Schumacher J, Ray NJ, Hamilton CA, Donaghy PC, Firbank M, Roberts G, et al. Cholinergic white matter pathways in dementia with Lewy bodies and Alzheimer's disease. Brain. (2022) 145:1773–84. doi: 10.1093/brain/awab372
48. Nemy M, Cedres N, Grothe MJ, Muehlboeck JS, Lindberg O, Nedelska Z, et al. Cholinergic white matter pathways make a stronger contribution to attention and memory in normal aging than cerebrovascular health and nucleus basalis of Meynert. Neuroimage. (2020) 211:116607. doi: 10.1016/j.neuroimage.2020.116607
49. Teipel SJ, Meindl T, Grinberg L, Grothe M, Cantero JL, Reiser MF, et al. The cholinergic system in mild cognitive impairment and Alzheimer's disease: an in vivo MRI and DTI study. Hum Brain Mapp. (2011) 32:1349–62. doi: 10.1002/hbm.21111
50. Jeong HE, Shin DH, Lee DC. Medial temporal atrophy alone is insufficient to predict underlying Alzheimer's disease pathology. Korean J Fam Med. (2020) 41:352–8. doi: 10.4082/kjfm.18.0144
51. Elias-Sonnenschein LS, Viechtbauer W, Ramakers IHGB, Verhey FRJ, Visser PJ. Predictive value of APOE-4 allele for progression from MCI to AD-type dementia: a meta-analysis. J Neurol Neurosurg Psychiatry. (2011) 82:1149–56. doi: 10.1136/jnnp.2010.231555
Keywords: mild cognitive impairment, Alzheimer's disease, white matter hyperintensities (WMHs), Cholinergic Pathways Hyperintensities Scale (CHIPS), apolipoprotein E (APOE)
Citation: Yu M-C, Chuang Y-F, Wu S-C, Ho C-F, Liu Y-C and Chou C-J (2023) White matter hyperintensities in cholinergic pathways are associated with dementia severity in e4 carriers but not in non-carriers. Front. Neurol. 14:1100322. doi: 10.3389/fneur.2023.1100322
Received: 16 November 2022; Accepted: 24 January 2023;
Published: 14 February 2023.
Edited by:
Christopher Mark Filley, University of Colorado Denver, United StatesReviewed by:
Murat Bilgel, National Institute on Aging (NIH), United StatesAndrea Mendez Colmenares, Colorado State University, United States
Copyright © 2023 Yu, Chuang, Wu, Ho, Liu and Chou. This is an open-access article distributed under the terms of the Creative Commons Attribution License (CC BY). The use, distribution or reproduction in other forums is permitted, provided the original author(s) and the copyright owner(s) are credited and that the original publication in this journal is cited, in accordance with accepted academic practice. No use, distribution or reproduction is permitted which does not comply with these terms.
*Correspondence: Yi-Chien Liu, milkgen@gmail.com
†These authors have contributed equally to this work