- 1Centre de formation médicale du Nouveau Brunswick, University of Sherbrooke, Moncton, NB, Canada
- 2New Brunswick Center for Precision Medicine, Moncton, NB, Canada
- 3Faculty of Medicine, Dalhousie University, Halifax Regional Municipality, Halifax, NS, Canada
- 4Faculty of Medicine, Memorial University, St John's, NL, Canada
- 5Department of Neurosurgery, The Moncton Hospital, Moncton, NB, Canada
- 6Department of Diagnostic Imaging, The Moncton Hospital, Moncton, NB, Canada
- 7Vitalité Health Network, Dr. Georges-L.-Dumont University Hospital Centre, Moncton, NB, Canada
- 8Department of Neurology, Dr.-Georges-L.-Dumont University Hospital Center, Moncton, NB, Canada
The neurological impact of COVID-19 is a rising concern among medical professionals, as patients continue to experience symptoms long after their recovery. This condition, known as neurological post-acute sequelae of COVID-19 (Neuro-PASC), can last for more than 12 weeks and includes symptoms such as attention disorders, brain fog, fatigue, and memory loss. However, researchers and health professionals face significant challenges in understanding how COVID-19 affects the brain, limiting the development of effective prevention and treatment strategies. In this mini-review, we provide readers with up-to-date information on the imaging techniques currently available for measuring the neurological impact of post-SARS-CoV-2 infection. Our search of PubMed and Google Scholar databases yielded 38 articles on various brain imaging techniques, including structural MRI (magnetic resonance imaging), functional MRI, diffusion MRI, susceptibility-weighted imaging, SPECT (single-photon emission computed tomography) imaging, and PET (positron emission tomography) imaging. We also discuss the optimal usage, limitations, and potential benefits of these techniques. Our findings show that various cerebral imaging techniques have been evaluated to identify a reliable marker for Neuro-PASC. For instance, 18F-FDG-PET/CT and functional MRI have demonstrated hypometabolism in cerebral regions that are directly linked to patient symptoms. Structural MRI studies have revealed different findings, such as infarcts, white matter atrophy, and changes in gray matter volumes. One SPECT imaging study noted frontal lobe hypometabolism, while diffusion MRI showed increased diffusivity in the limbic and olfactory cortical systems. The sequence SWI showed abnormalities primarily in white matter near the gray-white matter junction. A study on 18F-amyloid PET/CT found amyloid lesions in frontal and anterior cingulate cortex areas, and a study on arterial spin labeling (ASL) found hypoperfusion primarily in the frontal lobe. While accessibility and cost limit the widespread use of 18F-FDG-PET/CT scans and functional MRI, they seem to be the most promising techniques. SPECT, SWI sequence, and 18F-amyloid PET/CT require further investigation. Nevertheless, imaging remains a reliable tool for diagnosing Neuro-PASC and monitoring recovery.
1 Introduction
Since making its debut in December of 2019, COVID-19 (SARS-CoV-2) has infected nearly 750 million people around the globe, according to the World Health Organization (1). Primarily presenting as a respiratory syndrome, many have reported neurological manifestations, termed “neuro-COVID” either in the acute setting of the disease, or neurological symptoms lingering far after respiratory recovery (2). Approximately one-third of the population affected with COVID-19 and nearly two-thirds of hospitalized COVID-19 patients have experienced neurological complications from their infection (3, 4). Common acute neurological complications, lasting <4 weeks, include anosmia, dysgeusia, altered mental status, encephalopathy, peripheral neuropathy and acute cerebrovascular events (3, 4). On the other hand, nearly 10% of people affected by COVID-19 have signs or symptoms that develop during or after COVID-19 and that persist for over 3 months (5). This was classified as post-COVID syndrome. There is some discrepancy with regard to the accepted time frame to be classified as “post-COVID”, ranging between 4 weeks and 12 weeks after the acute phase (6). To further distinguish the acute from the persistent or chronic post-COVID syndrome, the neurological symptoms associated with long-term post-COVID syndrome are different than those present in the acute phase, and they are referred to as Neuro PASC (Neurological manifestations of Post-Acute Sequelae of SARS-CoV-2 infection (7). Fatigue, memory loss, brain fog, anosmia, attentional disorders, subjective cognitive impairment, and headaches dominate the clinical board when it comes to prolonged coronavirus implications (4, 8). These have been seen to persist for over 6 months following onset (5).
1.1 Pathophysiology of neuro-COVID
Three main hypotheses are currently considered to explain Neuro-PASC (8). The first is by indirect mechanism, via peripheral inflammation, also known as the “cytokine storm” that is triggered by SARS-CoV-2. This systemic inflammatory response could alter the equilibrium of the brain, similar to a septic encephalopathy (9). Cytokine storms occur when the fine balance between pro-inflammatory and anti-inflammatory cytokines is lost, and a previously localized inflammation affects the entire system. This deregulation can be non-provoked, as in auto-inflammatory illnesses, or can be precipitated by an infectious agent, as is the case for COVID-19. In the latter, studies have shown an elevation of IL-1β, IL-2, IL-6, IL-10, IFN-γ, and TNF-α, amongst others, and that the degree of elevation was proportional to the severity of the illness (10). The second theory is also one of indirect mechanism, and rests on the basis of lack of perfusion, sepsis or hyperpyrexia that can accompany the respiratory infection, each with their respective toll on the brain's homeostasis. The third hypothesis is one of direct neurotropism (11). SARS-CoV-2 infects the human body by binding to ACE2 receptors, which are primarily found in type 2 alveoli of the lungs (12) and entering the cells. Particularly, ACE2 receptors are also present in the brain's vascular endothelium and smooth muscles, as well as in skeletal muscles, thus explaining why myalgia is a widely present symptom included in post-COVID syndrome. Skeletal muscle cells express ACE2 receptors, which makes them a direct target for SARS-CoV-2 invasion (8). In support of this third theory, previous studies demonstrated that SARS-CoV-2 enters the nervous system by latching onto ACE2 receptors of the olfactory mucosa, penetrating the neural-mucosal interface, the only part of the brain not protected by the dura (3), and migrating along neuronal structures, eventually leading to the centers controlling cardiorespiratory functions (11). Whether Neuro-PASC is caused by direct attack of the virus on the brain or indirectly from systemic secondary damages, or by a combination of these (2), needs to be clarified.
1.2 The need for a precise marker
Despite the immensely rapid response and development of COVID-19 management protocols, many gaps need to be filled when it comes to understanding the pathophysiology of the disease as a whole. For example, up to 55% of hospitalized COVID-19 patients have reported to have neurological manifestations 3 months after their infection (2). This is still not well investigated and studied. This article serves as a review of the radiological markers that are currently in development for the evaluation of neurological damages of COVID-19. We will discuss 18F-FDG-PET/CT, SPECT, and 18F-AMYLOID PET/CT imaging, as well as structural, functional, diffusion MRI, ASL, and susceptibility-weighted imaging, and their current contribution in the management of COVID-19 specifically from a neurological standpoint.
2 Methods
2.1 Search strategy
A record search was performed to identify neuro-imaging methods for tracing Neuro-PASC including 18F-FDG-PET/CT, SPECT, MRI and fMRI. Databases searched were PubMed and Google Scholars for papers published between March 2020 and April 2023. Searches were divided by neuro-imaging methods for tracing Neuro-PASC. Studies were included if they (1) examined neurological markers, (2) used neuro-imaging modalities, (3) studied patients with a COVID-19 history and, (4) were in English. We excluded articles that examined children, adolescents and pregnant women or consisted of post-vaccination studies.
2.2 Search chains
With regards to 18 F-FDG-PET/CT imaging, the search key was ((((Neuro*) OR (brain)) AND ((Covid*) OR (coronavirus) OR (SARS-CoV-2))) AND ((long covid) OR (post covid))) AND ((PET) OR (positron)). For SPECT imaging, ((((Neuro*) OR (brain)) AND ((Covid*) OR (coronavirus) OR (SARS-CoV-2))) AND ((long covid) OR (post covid))) AND ((SPECT) OR (single photon)). For 18F-amyloid PET/CT, ((((Neuro*) OR (brain)) AND ((Covid*) OR (coronavirus) OR (SARS-CoV-2))) AND ((long covid) OR (post covid))) AND ((amyloid) OR (amyloid PET)). For structural MRI, (((((Neuro*) OR (brain)) AND ((Covid*) OR (coronavirus) OR (SARS-CoV-2))) AND ((long covid) OR (post covid))) AND ((MRI) OR (Magnetic resonance imaging)). For functional MRI, ((((Neuro*) OR (brain)) AND ((Covid*) OR (coronavirus) OR (SARS-CoV-2))) AND ((long covid) OR (post covid))) AND ((fMRI) OR (functional MRI)) yielded no results addressing our topic's subject. For ASL MRI, ((((Neuro*) OR (brain)) AND ((Covid*) OR (coronavirus) OR (SARS-CoV-2))) AND ((long covid) OR (post covid))) AND ((ASL MRI) OR (arterial spin labeling)). For diffusion MRI, ((((Neuro*) OR (brain)) AND ((Covid*) OR (coronavirus) OR (SARS-CoV-2))) AND ((long covid) OR (post covid))) AND ((diffusion MRI) OR (dMRI)). For susceptibility-weighted imaging, ((((Neuro*) OR (brain)) AND ((Covid*) OR (coronavirus) OR (SARS-CoV-2))) AND ((long covid) OR (post covid))) AND ((SWI) OR (Susceptibility-weighted imaging)). A targeted search was done on Google Scholar and PubMed for each neuro-imaging modality and post-covid (i.e.: post-covid PET neuro finding) to identify specific articles that may have been omitted.
2.3 Selection strategy
Retained articles were checked for duplicates and were selected after undergoing a title, abstract and eventually a full text screening. The screening and selection process was done by two independent authors (OC and MM). Collectively, 92 abstracts were read and 38 were used to construct this review. Specifically, 1 for SPECT imaging, 9 for advances with 18F-FDG-PET/CT, 1 for 18F-Amyloid-PET/CT, 10 for structural MRI, 2 for functional MRI, 1 for diffusion MRI, 2 for SWI and the remainder for additional information on Neuro-PASC. A PRISMA flowchart can be found in Figure 1.
3 Results
For each selected article, the following data was extracted and summarized in Table 1: author, journal, study design, number of subjects, number of controls, gender distribution, age, time elapsed since COVID-19 infection, type of imaging and main findings.
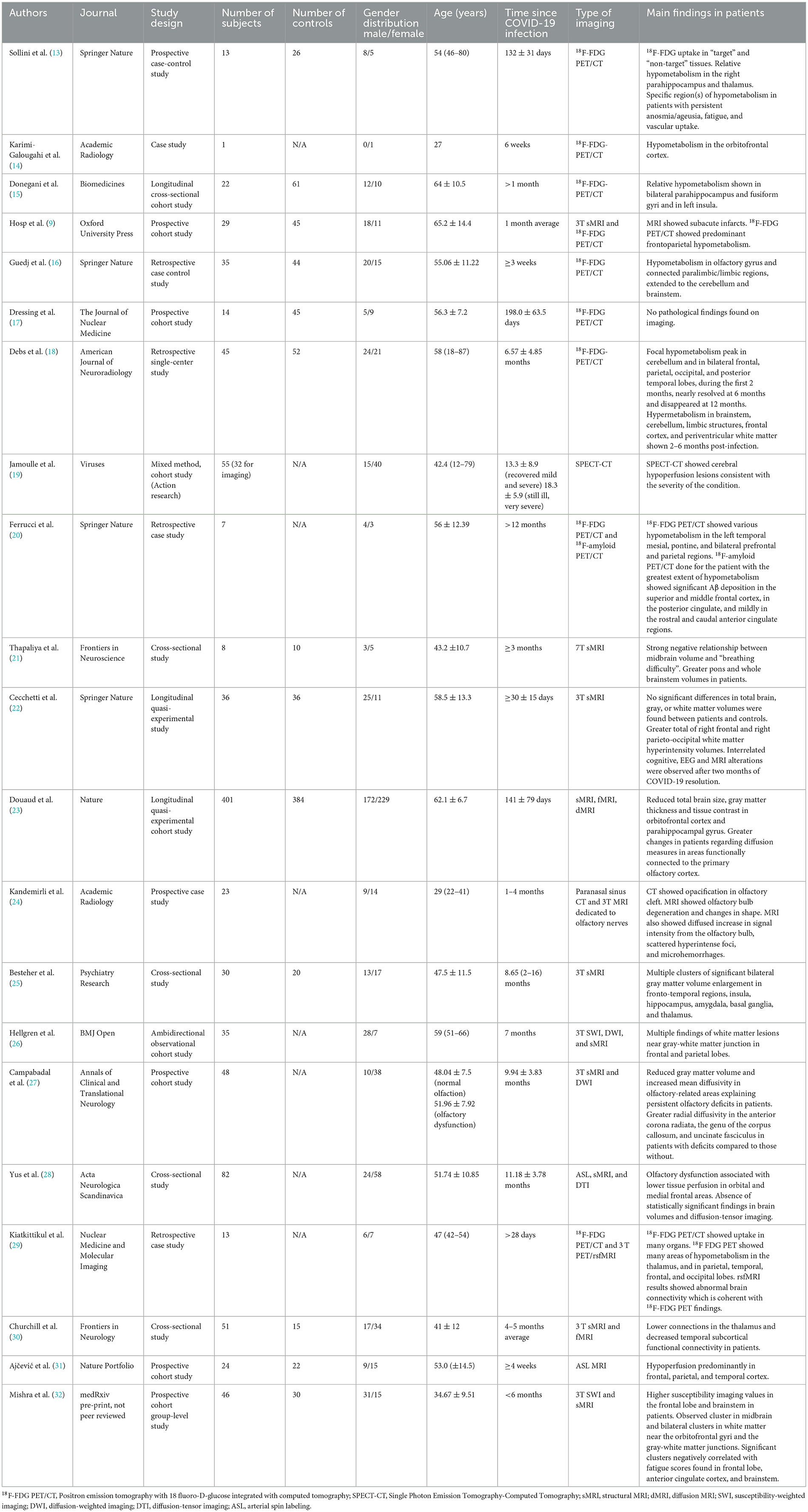
Table 1. Summary of the characteristics and findings of the imaging studies included in this review.
3.1 18F-FDG-PET/CT
Positron emission tomography (PET) measures the emission of positrons from radiomarked tracer molecules, allowing localization of metabolically active processes. The most widely used radiotracer is currently 18F-Fluorodeoxyglucose (18F-FDG), a radiolabeled glucose molecule. This method allows localization of regions of abnormal increase or decrease glucose intake in the body to better identify hyper or hypometabolic states and is currently mainly used as part of the workup and follow-up in oncology. In the Neuro-PASC scope, the expected utility of 18F-FDG-PET/CT is to identify a specific cerebral target that explains the clinical manifestations (33). This will be useful to better determine the pathophysiology, to improve the accuracy of the diagnosis, to then better develop therapeutic options and accurate follow-ups. When the intrinsic role of the hyper/hypometabolic brain loci correlates with the clinical manifestations of Neuro-PASC, while it cannot prove causality, points toward understanding the pathophysiology of COVID-19 and helps clinicians make the diagnosis of Neuro-PASC.
Sollini et al.'s case control study in 2021 evaluated post-COVID syndrome hallmarks on 18F-FDG-PET/CT and showed brain hypometabolism in limbic, paralimbic, brainstem and cerebellum. In addition, none of the post-COVID patients exhibited regions of hypermetabolism compared to controls. Limbic and orbito-frontal hypometabolism correlated with the anosmia present during acute COVID-19 infection. Sollini's team also evaluated the whole-body 18F-FDG-PET/CTscans. Despite the hypometabolic activity in the nervous system, they found hypermetabolic activity in different organs, independent of ACE-2 receptor activity, in other words, regions of hypermetabolism were seen in target (ACE-2 presenting) as well as non-target organs, supporting the hypothesis that systemic inflammation may be in play (13).
Verger et al.'s (34) study showed that 47% of the 18F-FDG-PET/CT scans of 143 patients with suspected Neuro-PASC had hypometabolism in limbic/paralimbic and fronto-orbital olfactory regions, as well as in the brainstem and cerebellum.
Several other studies have been conducted using 18F-FDG-PET/CT imaging to reveal potential Neuro-PASC biomarkers. A study by Karimi-Galougahi et al. (14) brought forth reduced metabolic activity in the orbitofrontal cortex using 18F-FDG-PET/CT. Bilateral par hippocampal, fusiform gyri, and left insula hypometabolism were seen in an 18F-FDG-PET/CT imaging study conducted by Donegani et al. (15).
A study by Hosp et al. (9) that ran its course in parallel with the Montreal Cognitive Assessment test (MoCA), a highly sensitive tool for early detection of mild cognitive impairment (MCI), correlated cognitive decline with the evident frontoparietal hypometabolism seen on scan. This study showed that while a structural MRI could not find any sign of cerebral damage, 18F-FDG-PET/CT discovered cortical fronto-parietal hypometabolism.
A study by Guedj et al. (16), demonstrated that clusters of patients with hypometabolic patterns on 18F-FDG-PET/CT scans, flagged by whole-brain statistical analysis performed using SPM8, were very distinct in patients with Neuro-PASC and allowed to reliably differentiate their brain from one of a control subject. The findings suggested that hypometabolism was seen in bilateral rectal/orbital gyrus, including the olfactory gyrus, right temporal lobe, bilateral pons/medulla, and the cerebellum bilaterally. These patients exhibited many functional complaints. Patients with cerebellar hypometabolism experienced hyposmia, anosmia and memory impairment. Patients with frontal cortex, brainstem and cerebellum hypometabolism presented pain and insomnia.
18F-FDG-PET/CT has also proven to be useful in characterizing the timeline of damages caused by SARS-CoV-2. Post-COVID syndrome patients who had increased vascular uptake (hypermetabolism) on whole-body scan done at 1-month post-infection also showed hypometabolism in the brain, thus demonstrating the different effects of COVID-19 on different regions of the body, and the chronological sequence for brain and whole-body changes throughout the disease (13).
In contrast to these studies, Dressing et al.'s (17) study revealed that post-COVID patients reporting symptoms lasting for over 3 months after the acute infection, actually only presented mild impairment on cognitive testing (MoCA) with their distinct pathologic findings on 18F-FDG-PET/CT. In this same stretch of ideas, Ferruci et al.'s review explained that not all patients who presented cognitive decline in the post-COVID timeframe showed hypometabolism on 18F-FDG-PET/CT (20).
In Debs et al. (18) study, a time-dependent brain PET hypo- and hypermetabolism was observed in patients with a history of COVID-19. Hypometabolic activity, in the bilateral frontal, parietal, occipital, and posterior temporal lobes and cerebellum, reached its peak at 2 months post-infection, then nearly resolved at 6 months, followed by disappearance at 12 months. Additionally, 2–6 months after infection onset, hypermetabolism was observed in brainstem, cerebellum, limbic structures, frontal cortex, and periventricular white matter.
3.2 SPECT/CT
Single Photon Emission Computed Tomography (SPECT/CT) is a method that allows superposition of anatomical images with metabolic activity. While PET scans detect metabolism through emission of photons, SPECT scans detect gamma rays from tracers injected into the patient (35). Brain SPECT measures brain perfusion. A limited number of studies met the particular interest of this topic. Takao et al.'s (36) study on Neuro-PASC patients described observing hypoperfusion on SPECT imaging in various areas of the brain, particularly the frontal lobes, which correlates with the previous findings in 18 F-FDG-PET/CT scans. A study conducted on 32 patients with highly impaired functional status, determined by COOP/WONCA functional ability questionnaire (Cooperative Research Network/World Organization of National Colleges, Academies and Academic Associations of General Practitioners/Family Physicians), demonstrated cerebral perfusion changes on SPECT/CT in 29 patients (90%) (19). Fifteen patients then underwent a control SPECT-CT 3–9 months after their initial imaging taken during the acute infection. The follow-up image showed marked improvement in 8 cases and worsening in 7 cases.
3.3 18F-AMYLOID PET/CT
Amyloid PET is an imaging modality that allows the visualization of amyloid plaques. A retrospective study conducted by Ferrucci et al. (20) found amyloid plaques in one Neuro-PASC patient 12 months after hospital discharge for his acute COVID-19 infection using an 18F-amyloid PET/CT. The plaques were found in the superior and middle frontal cortex, in the posterior cingulate cortex and in the rostral and caudal areas of the anterior cingulate cortex in a lower quantity. 18F- FDG PET/CT also detected hypometabolism in the left mesial temporal cortex of this patient (20). To our knowledge, this is the only published article that examined Amyloid PET.
3.4 Structural MRI
The anatomy of the brain can be studied using an MRI, an imaging modality that allows the visualization of soft tissues using a magnetic field (37). Results by Hosp et al. (9) suggest that 18F-FDG-PET/CT seems to be more effective in detecting anomalies than 3T MRI. Specifically, 18F-FDG-PET/was able to detect frontoparietal hypometabolism, whereas 3T MRI found no relevant structural or vascular anomalies. The sequences used in this article were as follow: sagittal 3D-T1 rapid gradient echo (MP-RAGE) before and after contrast infusion, sagittal 3D FLAIR SPACE (sampling perfection with application-optimized contrasts using different flip angle evolutions), SWI and diffusion mesoscopic imaging (DMI). Nonetheless, 3T MRI allowed to localize a few subacute infarcts (9).
However, in a pilot study conducted by Thapaliya et al. (21), T1-weighted 7T MRI showed an increased volume of the superior cerebella peduncle, pons, and entire brainstem in Neuro-PASC patients compared to controls. These biomarkers were also found in patients with myalgic encephalomyelitis/chronic fatigue syndrome which could explain the similarity in symptoms between these two conditions (21).
On another hand, Cecchetti et al. (22) found that COVID-19 patients showed interrelated cognitive, EEG and 3T T2-weighted structural MRI abnormalities 2 months after being discharged from the hospital. A UK Biobank longitudinal imaging study of 785 patients having been scanned by structural MRI (T1, T2 fluid attenuation inversion recovery (FLAIR) and susceptibility-weighted MRI) before, through indications for scans unrelated to COVID-19, and approximately 141 days after testing positive for COVID-19, showed statistically significant atrophy of gray matter in limbic cortical areas, directly linked to olfactory and gustatory systems 6 (23).
Another application for 3T T2-space structural MRI in post-COVID syndrome is demonstrated in Kandemirli et al.'s (24) study. Out of 23 patients with persistent COVID-19 olfactory dysfunction, nearly three-quarters had olfactory cleft opacification, a reduction in olfactory bulb volumes, change in bulb shape or signal anomalies (24). These MRI studies allowed for a better understanding of the damages inflicted on the olfactory pathway in the context of COVID-19 anosmia.
In a study conducted by Besteher et al. (25), increased gray matter volume clusters were found in post-COVID patients 8 months after their acute COVID-19 infection compared to controls using voxel-based morphometry (VBM). They used VBM with CAT12 toolbox, using T1-weighted images obtained from a 3T MRI to measure gray matter volumes. The clusters were found bilaterally in the frontal lobe, temporal lobe, insula, amygdala, hippocampus, basal ganglia, and thalamus. The volume of four of these clusters was found to decrease over time. The first cluster was in the anterior insula and some regions of the frontal lobe. The second cluster was scattered in the left temporal lobe. The third cluster was in the left post-central and precentral gyrus and finally, the fourth cluster was in parts of the temporal lobe, the right fusiform gyrus, the parahippocampal gyrus, and the hippocampus. It was hypothesized that the augmentation of the gray matter volumes was caused by compensatory processes or inflammation (25).
Hellgren et al.'s (26) observational cohort study compared the MRI imaging of post-COVID patients to the neuropsychological findings of these patients. 3T MRI (T2-FLAIR, T2-FSE, T1-FSE, T1-GRE, DWI and SWI) imaging was done on patients whose neurocognitive test results seemed concerning to researchers. The neurocognitive test performed was the Repeatable Battery for the Assessment of Neuropsychological Status (RBANS). Thirty-five post-COVID patients who had been hospitalized for their acute COVID-19 infection and who were discharged on average 6.5 months prior underwent a brain MRI which revealed subcortical white matter lesions in 71% of these patients. The lesions were primarily found in the frontal and parietal lobes near the gray-white matter junction. Compared to the patients with normal MRI results, the patients with white matter lesions had lower scores in the RBANS visuospatial index. It is worth noting that the functionality level of the patients with white matter lesions prior to their COVID-19 infection was lower compared to controls (26).
Campabadal et al. (27) using 3T structural MRI found a reduction in gray matter volume in the caudate nucleus, putamen, olfactory cortex, parahippocampal gyrus, straight gyrus, left amygdala, and the superior and inferior orbital gyri using voxel-based morphometry. A study by Yus et al. (28) using 3T structural MRI (3D-T1, T2-FLAIR) found no statistically significant findings in brain volumes.
3.5 Functional MRI
A functional MRI (fMRI) is able to estimate brain metabolism by using either blood-oxygen level dependant (BOLD) contrast or cerebral blood flow to measure activity in different regions of the brain. Resting-state fMRI (rsf-MRI) refers to an fMRI being performed while a patient is not performing any tasks (38). Limited studies have been published regarding functional MRI and its applicability in Neuro-PASC patients. Kiatkittikul et al. (29) showed abnormal brain connectivity on rsf-MRI which was concordant with 18 F-FDG-PET/CT findings. It was reported that one patient who had sensorineural hearing loss of the left ear as a consequence of COVID-19 showed hypometabolism of the left temporal region on 18 F-FDG PET/CT scans and anomalies in rsf-MRI in the same region (29). Another study using rsf-MRI, led by Churchill et al. (30), demonstrated that patients who are currently experiencing a larger number of Neuro-PASC symptoms tended to have altered connectivity between parietal, temporal, occipital and subcortical regions using BOLD rsf-MRI. Additionally, distinct patterns correlated to the intensity of PASC symptoms. They elude to the fact that this could be a useful tool to differentiate between Neuro-PASC and other non-COVID-related infections (30).
Arterial spin labeling (ASL) is an MRI technique that has been used to measure brain perfusion in Neuro-PASC patients. A study by Ajčević et al. (31) found that participants who still had cognitive impairment 2 to 10 months after the beginning of their acute COVID-19 infection had cerebral hypoperfusion patterns on ASL MRI imaging. The hypoperfusion was primarily found in the frontal cortex but was also present in the temporal and parietal cortex. These findings were more significant in the right hemisphere. They also found that overall, the cerebral blood flow was lower throughout the gray matter in Neuro-PASC patients compared to healthy controls (31). Yus et al. (28) found similar results in their ASL study on post-COVID patients where they found lower perfusion in the patient's orbital and medial frontal lobes.
3.6 Diffusion MRI
Diffusion MRI (dMRI) is an imaging modality that utilizes the diffusion of water as contrast. Diffusion tensor imaging (DTI) is a type of dMRI that creates a 3D construction of diffusion (39). Diffusivity measurements are indicators of tissue microstructure integrity. Douaud et al. (23) found a greater increase in diffusivity, an indicator of tissue damage, in areas functionally connected to the piriform cortex, olfactory tubercle and anterior olfactory nucleus, in patients who were infected with SARS-CoV-2 compared to healthy controls (23). Additionally, a study by Campabadal et al. (27) found that Neuro-PASC patients presenting with olfactory dysfunction had higher mean and radial diffusivity in certain white matter regions on DTI compared to post-acute COVID-19 patients without olfactory dysfunction. The areas of increased mean diffusivity were found in the genu of the corpus callosum, forceps minor, orbitofrontal white matter tracts, and anterior thalamic radiations. Regions with augmented radial diffusivity were found in the genu of the corpus callosum, anterior corona radiata, and uncinate fasciculus (27). In contrast, a study done by Yus et al. (28) found no statistically significant findings with DTI—in patients with Neuro-PASC.
3.7 Susceptibility-weighted imaging
Susceptibility-weighted imaging was also used to try to visualize the neurological sequela of SARS-CoV-2 infection. This imaging modality is an MRI technique that uses the patient's iron, calcium, and deoxygenated blood as a source of contrast for the image. This imaging modality is useful for detecting hemorrhages, traumatic brain injury, microvasculature, and neurodegenerative diseases (40). Hellgren et al.'s (26) study using SWI found mostly subcortical abnormalities near the gray-white matter junction in frontal and parietal lobes in 8 patients out of 35 (26).
In addition, Mishra et al. (32) found regions of abnormal susceptibility bilaterally in the brain stem, the gray-white matter junction and in the white matter of the frontal lobes in patients with post-COVID condition or patients who had recovered after COVID-19 infection. The abnormalities seen in the brainstem were primarily in the midbrain and the abnormalities in the frontal lobes were located in the uncinate fasciculus tract and the inferior frontal-occipital fasciculus tracts (32). However, it is important to note that this article has not been peer-reviewed. To our knowledge, this is the only published article where the main focus was the use of susceptibility-weighted imaging to find radiological markers in post-COVID patients.
4 Discussion
Overall, different cerebral imaging techniques have been evaluated to find a reliable and reproducible marker of the Neuro-PASC footprint. 18F-FDG-PET/CT and sMRI seem to be the most studied. SPECT scans, SWI, dMRI, fMRI, and ASL were not largely studied, however, deserve to be further investigated in the context of Neuro-PASC.
One current limitation of 18F-FDG-PET/CT and SPECT studies is that these studies' complexity, cost and radiation exposure limit the number of research cohorts. These types of imaging require prolonged periods in a scanner which is possibly not efficient when searching for a highly available, yet reliable method.
On the other hand, the use of ionizing radiation, as seen in 18F-FDG-PET/CT can only really be justified when there is high suspicion of important neurological complications. Thus, exposing patients to radiation when the benefits of such a technique remain uncertain can be perceived as questionable. Consent for such radiation exposure would be, as per usual, required.
While studies on 18F-FDG-PET/CT are ample, studies on SPECT are insufficient. Despite this, current literature suggests that because 18F-FDG-PET/CT has superior sensitivity, as well as better contrast and resolution than SPECT, currently, 18F-FDG-PET/CT imaging, is superior to SPECT. On the other hand, the cost of 18F-FDG PET/CT is significantly higher than SPECT and remains an important obstacle to its access in our healthcare system. Verger et al.'s (34) SPECT study also demonstrated that this imaging technique can be of particular interest when tracking the recovery from Neuro-PASC.
Although amyloid deposits were found in Ferrucci et al.'s study, they only conducted an amyloid PET scan on one patient. Further research is needed to conclude whether the amyloid PET scan is a useful imaging modality to visualize Neuro-PASC. A study from Sun et al. (41) showed significantly higher plasma levels of amyloid beta in participants after recovery from acute COVID-19 infection compared to controls. These results were present in participants whether they had perduring neurological symptoms or not (41). This also supports the need for further research.
With what pertains to structural MRI, findings varied depending on the strength of the MRI and the MRI technique. Structural MRI gave varying information on Neuro-PASC, such as localizing subacute infarcts and noting cortical atrophy. With a 3T MRI, some researchers were only able to localize areas of previous infarcts whilst others found atrophy of the gray matter in limbic cortical areas, a reduction in olfactory bulb volumes, or subcortical white matter lesions in the frontal and parietal lobes. On the other hand, with a 7T MRI, Thapaliya et al. found an increased volume of the brainstem in Neuro-PASC patients but the literature regarding 7T MRI in this population is limited. Clusters of increased gray matter volumes were found with voxel-based morphometry in the frontal lobe, temporal lobe, insula, amygdala, hippocampus, basal ganglia, and thalamus.
On the other hand, keeping in mind that studies are also quite limited, functional MRI shows concordant results to 18F-FDG-PET/CT, meaning findings of corresponding regions of hypometabolism and their symptomatic manifestations. ASL MRI found areas of hypoperfusion primarily in the frontal cortex but also in the temporal and parietal cortex, which concorded with SPECT findings.
Overall, most diffusion MRI studies showed changes in diffusivity in areas connected to the primary olfactory cortex. Increased diffusivity was also noted in the genu of the corpus callosum, orbitofrontal white matter tracts, anterior thalamic radiation, and forceps minor. The same study found a decrease in gray matter volumes in olfactory-related regions. These findings along with the increased mean and radial diffusivity could explain the persistent olfactory deficits found in patients.
Additionally, susceptibility-weighted imaging revealed low susceptibility mainly in the frontal lobes and brain stems, which is consistent with abnormalities detected by other imaging modalities. The anomalies found in the uncinate fasciculus tract could explain the memory and mental health problems that are often present in post-COVID patients. The orbitofrontal region of the brain has a major influence on smell and taste therefore the areas of abnormal susceptibility near the orbitofrontal gyri could explain the loss of smell and taste seen in post-COVID patients.
Combining these different neuro-imaging modalities, particularly 18F-FDG-PET/CT and sMRI, could be an interesting and rewarding approach in identifying neurological markers of Neuro-PASC. Starting with a structural imaging technique and then proceeding to functional or metabolic modalities could allow for a better grasp of the structural and metabolic long-term progression and ultimate impact of COVID-19 on the brain. A consideration to keep in mind is that pre-existing neurological conditions and neurocognitive disorders need to be considered when interpreting Neuro-PASC symptoms and imaging. Also, the reassurance of a proper diagnosis and management comforted many Neuro-PASC patients who had noticed psychological decline for a prolonged period after the initial infection, for no clear reason. Their memory, energy level and concentration were not restored post-infection, leaving them with great uncertainty regarding the cause of their psychological decline. The clear and visible perfusion defects on SPECT imaging provided great validation to patients living with these new post-infectious deficits and helped physicians make a positive diagnosis.
5 Conclusion
Overall, many studies have been conducted on the use of neuro-imaging in post-COVID syndrome patients with Neuro-PASC. Among these, 18F-FDG-PET/CT and sMRI seem to be the most studied and the most promising neuro-imaging modalities to identify Neuro-PASC markers. Further research needs to be conducted with regard to fMRI, dMRI, SPECT, amyloid PET, ASL MRI and susceptibility-weighted imaging. Although multiple structural MRI studies revealed significant abnormalities, these findings differed considerably in location within the brain and in type of abnormality from one study to another. These gaps in our knowledge do not allow us to confidently confirm the applicability of these imaging techniques in the detection, monitoring, and response to treatment. Future research should include homogenous and larger sample sizes and longitudinal data as much as possible to better identify and understand Neuro-PASC markers.
The cumulation of preliminary results of 18F-FDG-PET/CT showed that hypometabolism seen in reports could constitute a quantitative marker of cerebral damage of post-COVID syndrome. sMRI identified atrophy in white matter and changes in gray matter volumes, which could also be considered potential markers of Neuro-PASC. The possibility of having markers that allow a more accurate follow-up on brain damage, progression and response to therapy would not only allow us to better treat the increasing number of patients suffering from Neuro-PASC, but to better understand the pathophysiology of this cataclysmic disease.
Author contributions
OC and LA are the main authors of the article, doing the literature review, building the article, and sending for revisions. MM, JS, and DM helped screen the search results, with the writing and editing of the manuscript. AE and JW provided feedback and corrections, based on their expertise, in neurosurgery, and diagnostic imaging, respectively. LC-W overlooked the project and provided feedback at every stage of the article. All authors contributed to the article and approved the submitted version.
Funding
The author(s) declare that no financial support was received for the research, authorship, and/or publication of this article.
Conflict of interest
The authors declare that the research was conducted in the absence of any commercial or financial relationships that could be construed as a potential conflict of interest.
Publisher's note
All claims expressed in this article are solely those of the authors and do not necessarily represent those of their affiliated organizations, or those of the publisher, the editors and the reviewers. Any product that may be evaluated in this article, or claim that may be made by its manufacturer, is not guaranteed or endorsed by the publisher.
References
1. WHO Coronavirus (COVID-19) Dashboard. Available online at: https://covid19.who.int (accessed April 27, 2023).
2. DeKosky ST, Kochanek PM, Valadka AB, Clark RSB, Chou SHY, Au AK, et al. Blood biomarkers for detection of brain injury in COVID-19 patients. J Neurotrauma. (2021) 38:1–43. doi: 10.1089/neu.2020.7332
3. Ellul MA, Benjamin L, Singh B, Lant S, Michael BD, Easton A, et al. Neurological associations of COVID 19. Lancet Neurol. (2020) 19:767–83. doi: 10.1016/S1474-4422(20)30221-0
4. Meyer PT, Hellwig S, Blazhenets G, Hosp JA. Molecular imaging findings on acute and long-term effects of COVID-19 on the brain: a systematic review. J Nucl Med Off Publ Soc Nucl Med. (2022) 63:971–80. doi: 10.2967/jnumed.121.263085
5. Premraj L, Kannapadi NV, Briggs J, Seal SM, Battaglini D, Fanning J, et al. Mid and long-term neurological and neuropsychiatric manifestations of post-COVID-19 syndrome: a meta-analysis. J Neurol Sci. (2022) 434:120162. doi: 10.1016/j.jns.2022.120162
6. Gupta A, Madhavan MV, Sehgal K, Nair N, Mahajan S, Sehrawat TS, et al. Extrapulmonary manifestations of COVID-19. Nat Med. (2020) 26:1017–32. doi: 10.1038/s41591-020-0968-3
7. Rudroff T, Workman CD, Ponto LLB. 18F-FDG-PET imaging for Post-COVID-19 brain and skeletal muscle alterations. Viruses. (2021) 13:2283. doi: 10.3390/v13112283
8. Al-Aly Z, Xie Y, Bowe B. High-dimensional characterization of post-acute sequelae of COVID-19. Nature. (2021) 594:259–64. doi: 10.1038/s41586-021-03553-9
9. Hosp JA, Dressing A, Blazhenets G, Bormann T, Rau A, Schwabenland M, et al. Cognitive impairment and altered cerebral glucose metabolism in the subacute stage of COVID-19. Brain J Neurol. (2021) 144:1263–76. doi: 10.1093/brain/awab009
10. Zanza C, Romenskaya T, Manetti AC, Franceschi F, La Russa R, Bertozzi G, et al. Cytokine storm in COVID-19: immunopathogenesis and therapy. Medicina. (2022) 58:144. doi: 10.3390/medicina58020144
11. Heming M, Li X, Räuber S, Mausberg AK, Börsch AL, Hartlehnert M, et al. Neurological manifestations of COVID-19 feature T cell exhaustion and dedifferentiated monocytes in cerebrospinal fluid. Immunity. (2021) 54:164–175.e6. doi: 10.1016/j.immuni.2020.12.011
12. Morbelli S, Ekmekcioglu O, Barthel H, Albert NL, Boellaard R, Cecchin D, et al. COVID-19 and the brain: impact on nuclear medicine in neurology. Eur J Nucl Med Mol Imaging. (2020) 47:2487–92. doi: 10.1007/s00259-020-04965-x
13. Sollini M, Morbelli S, Ciccarelli M, Cecconi M, Aghemo A, Morelli P, et al. Long COVID hallmarks on [18F]FDG-PET/CT: a case-control study. Eur J Nucl Med Mol Imaging. (2021) 48:3187–97. doi: 10.1007/s00259-021-05294-3
14. Karimi-Galougahi M, Yousefi-Koma A, Bakhshayeshkaram M, Raad N, Haseli S. 18FDG PET/CT scan reveals hypoactive orbitofrontal cortex in anosmia of COVID-19. Acad Radiol. (2020) 27:1042–3. doi: 10.1016/j.acra.2020.04.030
15. Donegani MI, Miceli A, Pardini M, Bauckneht M, Chiola S, Pennone M, et al. Brain metabolic correlates of persistent olfactory dysfunction after SARS-Cov2 infection. Biomedicines. (2021) 9:287. doi: 10.3390/biomedicines9030287
16. Guedj E, Campion JY, Dudouet P, Kaphan E, Bregeon F, Tissot-Dupont H, et al. 18F-FDG brain PET hypometabolism in patients with long COVID. Eur J Nucl Med Mol Imaging. (2021) 48:2823–33. doi: 10.1007/s00259-021-05215-4
17. Dressing A, Bormann T, Blazhenets G, Schroeter N, Walter LI, Thurow J, et al. Neuropsychologic profiles and cerebral glucose metabolism in neurocognitive long COVID syndrome. J Nucl Med. (2022) 63:1058–63. doi: 10.2967/jnumed.121.262677
18. Debs P, Khalili N, Solnes L, Al-Zaghal A, Sair HI, Yedavalli V, et al. “Post-COVID-19 Brain [18F] FDG-PET findings: a retrospective single-center study in the United States. AJNR Am J Neuroradiol. (2023) 44:517–22. doi: 10.3174/ajnr.A7863
19. Jamoulle M, Kazeneza-Mugisha G, Zayane A. Follow-up of a cohort of patients with post-acute COVID-19 syndrome in a Belgian family practice. Viruses. (2022) 14:2000. doi: 10.3390/v14092000
20. Ferrucci R, Cuffaro L, Capozza A, Rosci C, Maiorana N, Groppo E, et al. Brain positron emission tomography (PET) and cognitive abnormalities one year after COVID-19. J Neurol. (2023) 270:1823–34. doi: 10.1007/s00415-022-11543-8
21. Thapaliya K, Marshall-Gradisnik S, Barth M, Eaton-Fitch N, Barnden L. Brainstem volume changes in myalgic encephalomyelitis/chronic fatigue syndrome and long COVID patients. Front. Neurosci. (2023) 17:1125208. doi: 10.3389/fnins.2023.1125208
22. Cecchetti G, Agosta F, Canu E, Basaia S, Barbieri A, Cardamone R, et al. Cognitive, EEG, and MRI features of COVID-19 survivors: a 10-month study. J Neurol. (2022) 269:3400–12. doi: 10.1007/s00415-022-11047-5
23. Douaud G, Lee S, Alfaro-Almagro F, Arthofer C, Wang C, McCarthy P, et al. SARS-CoV-2 is associated with changes in brain structure in UK Biobank. Nature. (2022) 604:697–707. doi: 10.1038/s41586-022-04569-5
24. Kandemirli SG, Altundag A, Yildirim D, Sanli DET, Saatci O. Olfactory bulb MRI and paranasal sinus CT findings in persistent COVID-19 anosmia. Acad Radiol. (2021) 28:28–35. doi: 10.1016/j.acra.2020.10.006
25. Besteher B, Machnik M, Troll M, Toepffer A, Zerekidze A, Rocktäschel T, et al. Larger gray matter volumes in neuropsychiatric long-COVID syndrome. Psychiatry Res. (2022) 317:114836. doi: 10.1016/j.psychres.2022.114836
26. Hellgren L, Birberg Thornberg U, Samuelsson K, Levi R, Divanoglou A, Blystad I. Brain MRI and neuropsychological findings at long-term follow-up after COVID-19 hospitalisation: an observational cohort study. BMJ Open. (2021) 11:e055164. doi: 10.1136/bmjopen-2021-055164
27. Campabadal A, Oltra J, Junqué C, Guillen N, Botí MÁ, Sala-Llonch R, et al. Structural brain changes in post-acute COVID-19 patients with persistent olfactory dysfunction. Ann Clin Transl Neurol. (2023) 10:195–203. doi: 10.1002/acn3.51710
28. Yus M, Matias-Guiu JA, Gil-Martínez L, Gómez-Ruiz N, Polidura C, Jorquera M, et al. Persistent olfactory dysfunction after COVID-19 is associated with reduced perfusion in the frontal lobe. Acta Neurol Scand. (2022) 146:194–8. doi: 10.1111/ane.13627
29. Kiatkittikul P, Promteangtrong C, Kunawudhi A, Siripongsatian D, Siripongboonsitti T, Ruckpanich P, et al. Abnormality pattern of F-18 FDG PET whole body with functional MRI brain in post-acute COVID 19. Nucl Med Mol Imaging. (2022) 56:29–41. doi: 10.1007/s13139-021-00730-6
30. Churchill NW, Roudaia E, Chen JJ, Gilboa A, Sekuler A, Ji X, et al. Effects of post-acute COVID-19 syndrome on the functional brain networks of non-hospitalized individuals. Front Neurol. (2023) 14:1136408. doi: 10.3389/fneur.2023.1136408
31. Ajčević M, Iscra K, Furlanis G, Michelutti M, Miladinović A, Buoite Stella A, et al. Cerebral hypoperfusion in post-COVID-19 cognitively impaired subjects revealed by arterial spin labeling MRI. Sci Rep. (2023) 13:5808. doi: 10.1038/s41598-023-32275-3
32. Mishra SS, Hafiz R, Misra R, Gandhi TK, Prasad A, Mahajan V, et al. Brain alterations in Covid recovered revealed by susceptibility-weighted magnetic resonance imaging. medRxiv. (2022). doi: 10.1101/2022.11.21.22282600
33. Unterrainer M, Eze C, Ilhan H, Marschner S, Roengvoraphoj O, Schmidt-Hegemann NS, et al. Recent 274 advances of PET imaging in clinical radiation oncology. Radiat Oncol. (2020) 15:88. doi: 10.1186/s13014-020-01519-1
34. Verger A, Kas A, Dudouet P, Goehringer F, Salmon-Ceron D, Guedj E. Visual interpretation of brain hypometabolism related to neurological long COVID: a French multicentric experience. Eur J Nucl Med Mol Imaging. (2022) 49:3197–202. doi: 10.1007/s00259-022-05753-5
35. Nuclear Medicine. National Institute of Biomedical Imaging and Bioengineering. Available online at: https://www.nibib.nih.gov/science-education/science-topics/nuclear-medicine (accessed April 30, 2023).
36. Takao M, Ohira M. Neurological post-acute sequelae of SARS-CoV-2 infection. Psychiatry Clin Neurosci. (2023) 77:72–83. doi: 10.1111/pcn.13481
37. National Institute of Biomedical Imaging Bioengineering. Magnetic Resonance Imaging (MRI). Available online at: https://www.nibib.nih.gov/science-education/science-topics/magnetic-resonance-imaging-mri (accessed September 23, 2023).
38. Glover GH. Overview of functional magnetic resonance imaging. Neurosurg Clin N Am. (2011) 22:133–9. doi: 10.1016/j.nec.2010.11.001
39. Le Bihan D. Diffusion MRI: what water tells us about the brain. EMBO Mol Med. (2014) 6:569–73. doi: 10.1002/emmm.201404055
40. Halefoglu AM, Yousem DM. Susceptibility weighted imaging: clinical applications and future directions. World J Radiol. (2018) 10:30–45. doi: 10.4329/wjr.v10.i4.30
Keywords: Neuro-PASC, post-COVID, MRI, fMRI, PET/CT scan, imaging, SPECT (single-photon emission computed tomography)
Citation: Cull O, Al Qadi L, Stadler J, Martin M, El Helou A, Wagner J, Maillet D and Chamard-Witkowski L (2023) Radiological markers of neurological manifestations of post-acute sequelae of SARS-CoV-2 infection: a mini-review. Front. Neurol. 14:1233079. doi: 10.3389/fneur.2023.1233079
Received: 25 July 2023; Accepted: 08 November 2023;
Published: 24 November 2023.
Edited by:
Govind Nair, National Institutes of Health (NIH), United StatesReviewed by:
Akram A. Hosseini, Nottingham University Hospitals NHS Trust, United KingdomHernan Chaves, Fundación Para la Lucha Contra las Enfermedades Neurológicas de la Infancia (FLENI), Argentina
Liane S. Canas, King's College London, United Kingdom
Copyright © 2023 Cull, Al Qadi, Stadler, Martin, El Helou, Wagner, Maillet and Chamard-Witkowski. This is an open-access article distributed under the terms of the Creative Commons Attribution License (CC BY). The use, distribution or reproduction in other forums is permitted, provided the original author(s) and the copyright owner(s) are credited and that the original publication in this journal is cited, in accordance with accepted academic practice. No use, distribution or reproduction is permitted which does not comply with these terms.
*Correspondence: Ludivine Chamard-Witkowski, ludivine.witkowski@vitalitenb.ca
†These authors have contributed equally to this work and share first authorship