- 1Department of Neurosurgery, Beijing Tiantan Hospital, Capital Medical University, Beijing, China
- 2Department of Functional Neurosurgery, Beijing Neurosurgical Institute, Capital Medical University, Beijing, China
Background: Reduction of medication in Parkinson's disease (PD) following subthalamic nucleus deep brain stimulation (STN-DBS) has been recognized, but the optimal timing for medication adjustments remains unclear, posing challenges in postoperative patient management.
Objective: This study aimed to provide evidence for the timing of medication reduction post-DBS using propensity score matching (PSM).
Methods: In this study, initial programming and observation sessions were conducted over 1 week for patients 4–6 weeks postoperatively. Patients were subsequently categorized into medication reduction or non-reduction groups based on their dyskinesia evaluation using the 4.2-item score from the MDS-UPDRS-IV. PSM was employed to maintain baseline comparability. Short-term motor and neuropsychiatric symptom assessments for both groups were conducted 3–6 months postoperatively.
Results: A total of 123 PD patients were included. Baseline balance in motor and non-motor scores was achieved between the two groups based on PSM. Short-term efficacy revealed a significant reduction in depression scores within the non-reduction group compared to baseline (P < 0.001) and a significant reduction compared to the reduction group (P = 0.037). No significant differences were observed in UPDRS-III and HAMA scores between the two groups. Within-group analysis showed improvements in motor symptoms, depression, anxiety, and subdomains in the non-reduction group, while the reduction group exhibited improvements only in motor symptoms.
Conclusion: This study provides evidence for the timing of medication reduction following DBS. Our findings suggest that early maintenance of medication stability is more favorable for improving neuropsychiatric symptoms.
Introduction
Deep brain stimulation (DBS) of the subthalamic nucleus (STN) is the first-line surgical treatment for Parkinson's disease (PD), it can effectively improve Parkinson's disease patients' motor and non-motor symptoms (1, 2), reduce motor fluctuations, reduce dopaminergic drug use and adverse drug reactions, and improve patients' quality of life (3–5). In recent years, an increasing number of studies have discovered that non-motor symptoms, particularly emotional aspects such as anxiety and depression, have a significant impact on the quality of life of Parkinson's disease patients (6).
The success of DBS is critically dependent on delivering the appropriate dose of stimulation at the best location within the target region (7). DBS programming is the process of choosing the appropriate electrical stimulation dose at the individual electrode placement and anatomy to achieve the greatest clinical benefit (8). DBS programming is a process that relies on clinical observation and repeated empirical attempts by programming doctors (9). A large number of parameter combinations, including frequency, pulse width, and stimulation voltage selection, as well as contact selection, are usually insufficient to test the best curative effect in a clinical routine treatment (10, 11). If the patient experiences drug side effects such as dyskinesias, apart from the stimulation parameters, the drug may also be adjusted (12). This will result in a greater number of test parameters being combined. Furthermore, while real-time observation and adjustment of stimulation parameters and contacts can improve motor symptoms, most non-motor symptoms cannot be effectively adjusted through real-time observation (13, 14). As a result, the curative effect of programming is highly dependent on the programming doctor's experience and the number of times the patient has gone through trial and error. This has resulted in patients' uncontrollable postoperative clinical benefit, with individual differences in clinical benefit.
In addition to improving the clinical symptoms of patients, STN-DBS has the effect of reducing dopamine drug use (15). Existing research indicates that after DBS, a high dose of LEDD reduces quality of life, and that there is a negative correlation between the two (16). As a result, postoperative drug adjustment, especially the reduction of drug, is critical. Compared with drug therapy alone, the adjustment of drug dosage and type after STN-DBS can better improve the patients' motor and non-motor symptoms (4, 17, 18). For example, after surgery, appropriate medication reductions improved the patient's depressive symptoms and reduced motor fluctuations (19, 20). Medication is reduced based on response to stimulation according to existing drug reduction strategies. If there is a clear response to stim, even a partial response, medication can be gradually reduced. If there is not a response to stimulation because the stimulation parameters are still being determined, medication should not be reduced (21). However, the current drug reduction strategy only relies on clinical response and doctor experience, which has some limitations. If the patient's first-time programming effect is satisfactory, it is an unresolved issue whether the drug dosage should be reduced immediately or maintained in the early stage. If the dosage, type, or timing of the adjustment of the drug is improper, it may cause dopamine dysregulation syndrome (DDS), mental symptoms, depression, mania, etc. (22–24). Improper adjustment of dopamine agonists within 3 months after DBS can lead to the development of manic symptoms or impairment of impulse control (25). In addition, reducing dopamine agonist medications and levodopa immediately after surgery can lead to depression and anxiety (26). As a result, the current DBS postoperative drug adjustment is also based on trial-and-error results of the programmed parameters and the experience of the programmed doctor, and the current postoperative drug adjustment lacks clinical evidence support. When the curative effect of the patient's first programming is acceptable or dyskinesias occur, the programming doctor usually chooses to reduce the drug dosage or stimulation parameters, however, there is no evidence to suggest which method is superior.
In the present study, we included 123 patients treated with STN-DBS and compared early drug reduction (drug reduction at the initial programming after surgery) and late drug reduction (reduction of drug after 3–6 months after surgery) differences in motor and mental symptoms, with the goal of providing clinical evidence support for drug adjustment after DBS and reducing the number of programming trial and error.
Methods
Study design and ethical approval
This study was a single center retrospective study. Baseline visit was conducted between August 2018 and February 2021 in Beijing Tiantan Hospital. The programming parameter of the first time was high frequency stimulation, as used by most centers.
The patients in this study were all treated at Tiantan Hospital, and the study was conducted in accordance with the Declaration of Helsinki and authorized by Tiantan Hospital Ethics Committees. Our study followed the STROBE guidelines, and the selection of clinical problems followed the PICOS principle.
Patients
The PD diagnosis was based on UK Brain Bank criteria (27). Patients were screened for bilateral STN-DBS according to the International PD and Movement Disorders Society and National Neurological Society guidelines (28). Levodopa challenge tests were considered satisfactory if there was >30% Movement Disorders Society-Unified Parkinson's Disease Rating Scale (MDS-UPDRS)-III improvement, and the patients were treated with DBS.
Patient medication adjustments were jointly decided by neurologists and programming doctors. Initial programming for patients commenced at 4–6 weeks post-surgery (T1) and spanned a duration of 1 week, involving 2–3 programming sessions. During this period, medication adjustments were based on the patients' MDS-UPDRS Part IV item 4.2 scores. Patients with scores ≥2 points after 2–3 programming sessions underwent medication reduction, while those scoring 0–1 points had their medication maintained at a stable level. Short-term follow-up at 3–6 months post-surgery (T2) involved maintaining medication dosages unchanged from T1. Patients were categorized into reduced and non-reduced groups based on whether medication adjustments were made at T1. We excluded patients with clinically relevant severe neuropsychiatric disorders and if neuropsychological impairments were found in the preoperative multi-disciplinary assessments by specialized neuropsychologists and neuropsychiatrists (29). All participants provided written informed consent to take part in the study.
Clinical assessment
Clinical assessments were conducted at preoperative baseline (within 1 month before surgery) in the ON-medication state (MedON) and OFF-medication state (MedOFF), with at 3–6 months follow-up (medication and stimulation ON/medication OFF and stimulation ON, MedON-StimON/MedOFF-StimON). Due to the best efficacy of DBS achieved within 3–6 months after surgery, 3–6 months was the time point for short-term efficacy analyses of this cohort study at our center (30).
With the following scales:
1. Motor disorder: MDS-UPDRS-III (31) and Hoehn-Yahr Stage were used to investigate motor impairment. MDS-UPDRS-III involved video collections and was scored by two experienced doctors in a single-blind way, back-to-back (32, 33). Higher scores indicated higher impairment in all scales.
2. The therapeutic medical regimen was recorded to calculate the levodopa equivalent daily dose (LEDD) according to the method of Tomlinson et al. (34).
3. The Hamilton Rating Scale for Anxiety/Depression (anxiety/depression, HAM-A/-D) were used for anxiety and depression (35). The 24 items of HAMD were grouped into the following seven factors: (1) anxiety/somatization (six items: psychic anxiety, somatic anxiety, gastrointestinal symptoms, hypochondriasis, insight, and general symptoms); (2) weight loss (one item); (3) cognitive disturbances (six items: self-guilt, suicide, agitation, depersonalization and derealization, paranoid, and obsessive compulsive symptom); (4) circadian fluctuations (one item); (5) retardation symptoms (four items: depression, work and interests, retardation, and sexual symptoms); (6) sleep disturbances (three items: difficulty falling asleep, superficial sleep and early awakening); (7) hopelessness symptoms (three items: helplessness, hopelessness, and worthlessness) (36). The 14 items of HAMA were grouped into the following two factors: (1) somatic anxiety (seven items: somatic anxiety muscular, somatic anxiety sensory, cardiovascular symptoms, respiratory symptoms, gastro-intestinal symptoms, genito-urinary symptoms, and autonomic symptoms); (2) psychic anxiety (seven items: anxious mood, tension, fears, insomnia, cognitive, depressed mood, and behavior at interview). Higher scores indicated higher impairment in all scales.
Programming and follow-up
In Tiantan Center, there is a special team responsible for PD patients' evaluation. They did not participate to the programming or surgery procedure. So they were unaware of the programming parameters or whether the drug was reduced. At each follow-up, an appointment was made for the patients to be evaluated first, followed by programming. We considered these results were less bias for acute programming parameter changes. As a result, the evaluation results were guaranteed to reflect the patient's true effects within 3–6 months.
The programming doctor performs trial and error on the patient's programming parameters based on the patient's motor symptoms, non-motor symptoms, dyskinesias, and other side effects. The initial programming parameters primarily employ monopolar stimulation of the STN's dorsal contact, 130 Hz high-frequency stimulation, 60 μs pulse width, and a voltage of approximately 2.0 V. Adjust the combination of contacts and parameters based on the patient's immediate motor response and stimulus-related side effects to the programming when the patient is not taking anti-parkinsonian drugs.
Following 1 week of programming and observation, patients conclude their initial programming phase. Subsequently, they return to the hospital for short-term follow-up 3–6 months postoperatively. Throughout the T1-T2 timeframe, the patients' programming parameters and medication regimens remain consistent with those at T1. After the conclusion of the short-term follow-up, patients undergo another 1 week programming and medication adjustment session. We define 1-year post-surgery as the milestone for long-term follow-up, at which point we conduct an assessment of the patients' long-term therapeutic efficacy.
Statistical analysis
The normality of distribution was assessed using the Shapiro-Wilk test. Differences of baseline characteristics and the follow-up between the two groups were analyzed using Mann-Whitney U-tests or unpaired t-tests, if parametric tests were applicable. Outcome changes from baseline to follow-up of each group were tested using Wilcoxon signed-rank tests or paired samples t-tests, and the results from the two groups were tested with Mann-Whitney U-tests or unpaired t-tests. The threshold was P < 0.05, unless stated otherwise. All statistical tests were two-tailed. The magnitude of clinical responses was evaluated with relative changes [(mean Testfollow − up-mean Testbaseline)/mean Testbaseline] and Cohen's effect size [(mean Testbaseline-mean Testfollow − up)/SD Testchangescores]. Confidence intervals were calculated for effect sizes based on non-central t- distribution (29). Multiple comparisons of the domines of HAMA and HAMD, resulting from the two groups and the use of multiple tests, were corrected using the FDR method. Corrected P-values adjusted to the significance threshold of P < 0.05 are presented unless otherwise stated.
Furthermore, as our study included data from an observational perspective cohort study of MDS-UPDRS-III, we screened all patients who met the enrollment criteria of this study, and who were enrolled from August 2018 to February 2021, and excluded patients who were lost to follow-up. We used propensity score matching (PSM) to increase causal inference and minimize selection bias. PSM is therefore also called a post-random method. The aim here was to find sub-cohorts of patients in the reducing LEDD group and non-reducing LEDD group with accurately balanced preoperative demographic and clinical characteristics. Variables included for propensity score matching were baseline MDS-UPDRS-III (MedOFF), LEDD, HAMD, HAMA, age at intervention, sex, and duration to reduce confounding factors between categories. Nearest-neighbor matching with a 0.03 caliper without replacement was conducted using a 1:1 ratio (37–39). Subsequently, all analyses of clinical changes from baseline to follow-up were also conducted for the identified matched cohort. Statistical analyses were performed using SPSS statistical software for Windows, version 25.0 (SPSS, Chicago, IL, USA) and R 3.6.1 (R Foundation for Statistical Computing. Analyses were performed using the R statistical software version 3.6.1 (available online at http://www.R-project.org) and propensity score analysis using the “MatchIt” package (40).
Results
A total of 123 patients were included, and one patient who was not returning for the 3–6 months follow-up were excluded. Finally, a total of 122 patients (64 males) with PD patients undergoing bilateral STN-DBS were included in the statistical analyses (Figure 1). The patients were divided in two groups involving the reducing LEDD group (n = 75) and non-reducing LEDD group (n = 47). The 122 patients in the final analysis were aged 61.93 ± 8.41 years with 13.21 ± 4.75 years disease durations. The Hoehn and Yahr was 2.9 ± 0.2. The average MMSE was 26.19 ± 4.04 and the cores of PDQ-39 were 60.83 ± 24.21 for all patients.
Baseline characteristics
Comparing baseline parameters of the two groups in the original cohort (Table 1), we observed significantly less LEDD in the non-reduced LEDD group (P = 0.001). In addition, there was no significant difference in the baseline age, sex, and duration of disease between the two groups.
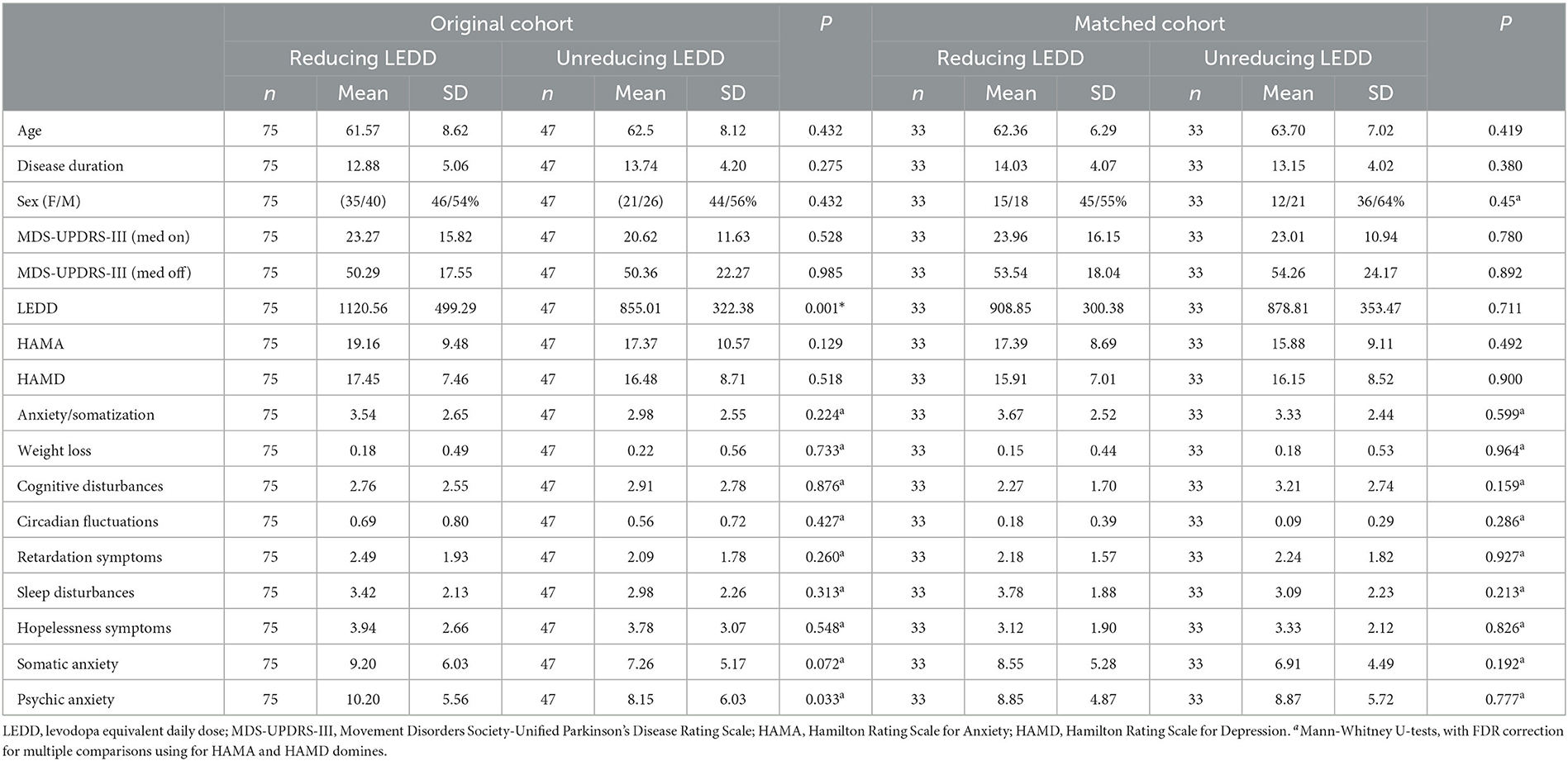
Table 1. Demographic characteristics and outcome parameters at baseline in unmatched and matched cohorts.
Based on propensity score matching, a sub-cohort of 66 patients was obtained, including 33 patients for each treatment group in a 1:1 ratio (Table 1). Diagnostic statistics indicated a good balance of all demographic and main clinical baseline parameters between the reducing LEDD and non-reducing LEDD groups of the matched cohort. Accordingly, no significant difference was found for these parameters between the two groups. Furthermore, no significant difference was observed between the groups regarding HAMA and HAMD domain scores. The programming parameters are balanced in the two groups (Supplementary Table 2).
Differences of outcomes at follow-up
Both groups showed improvement in HAMD total scores and HAMD sleep disturbance scores improved, but more improvement was found in the non-reducing group. Compared to the group with reduced LEDD, the group without LEDD reduction exhibited a significant decrease in the total HAMD score (P = 0.037). Additionally, the overall improvement rate of the HAMD score was significantly higher in the group without LEDD reduction when compared to the LEDD reduction group (P = 0.030).
The sleep disturbances domain of HAMD was significantly lower at the short-term follow-up in the non-reducing LEDD group (P = 0.009, post-hoc). As expected, the LEDDs were significantly lower (Table 2). Programming parameters are listed in Table 3 and were balanced in both groups. Long-term follow-up is shown in Supplementary Table 1. The patient's anxiety (P = 0.011) and depression (P = 0.013) improved significantly, but there is no different significantly between the two groups.
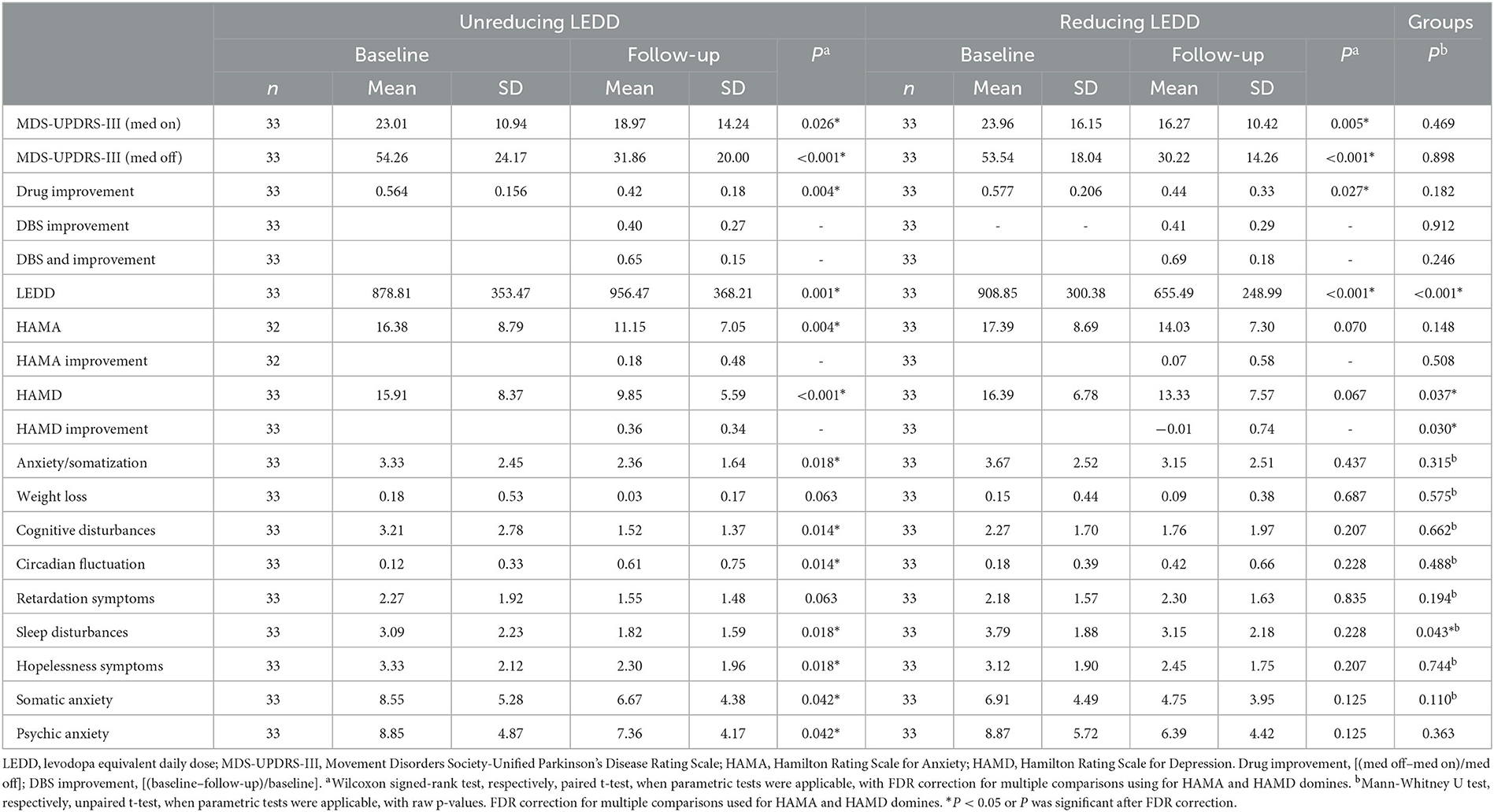
Table 2. Outcomes at baseline and at 3–6 months follow-up in unreducing and reducing LEDD groups for the matched cohort.
UPDRS-III (MedOFF) and UPDRS-III (MedON) significantly improved from baseline to follow-up in the non-reducing LEDD (P < 0.001, P = 0.026; Table 2) and reducing LEDD groups (P < 0.001, P = 0.005; see Table 2). LEDD was significantly reduced by ~28% in the reducing LEDD group (P < 0.001). In addition, the drug improvement rate was significantly decreased in the two groups (non-reducing LEDD, P = 0.004; reducing LEDD, P = 0.027). HAMA and HAMD scores were significantly improved in the non-reducing LEDD group (HAMA, P = 0.004; HAMD, P < 0.001). No significant difference was found in the reducing LEDD group dealing with anxiety and depression (HAMA, P = 0.070; HAMD, P = 0.067).
Post-hoc analyses of HAMD domain scores revealed differential effects for the two treatment groups (Figure 2): In the non-reducing LEDD group, significant beneficial effects were observed for cognitive disturbances (P = 0.003) and sleep disturbances (P = 0.012), but the circadian fluctuation was significantly worse than before (P = 0.004). In the reducing LEDD group, we found no significant change between baseline and follow-up.
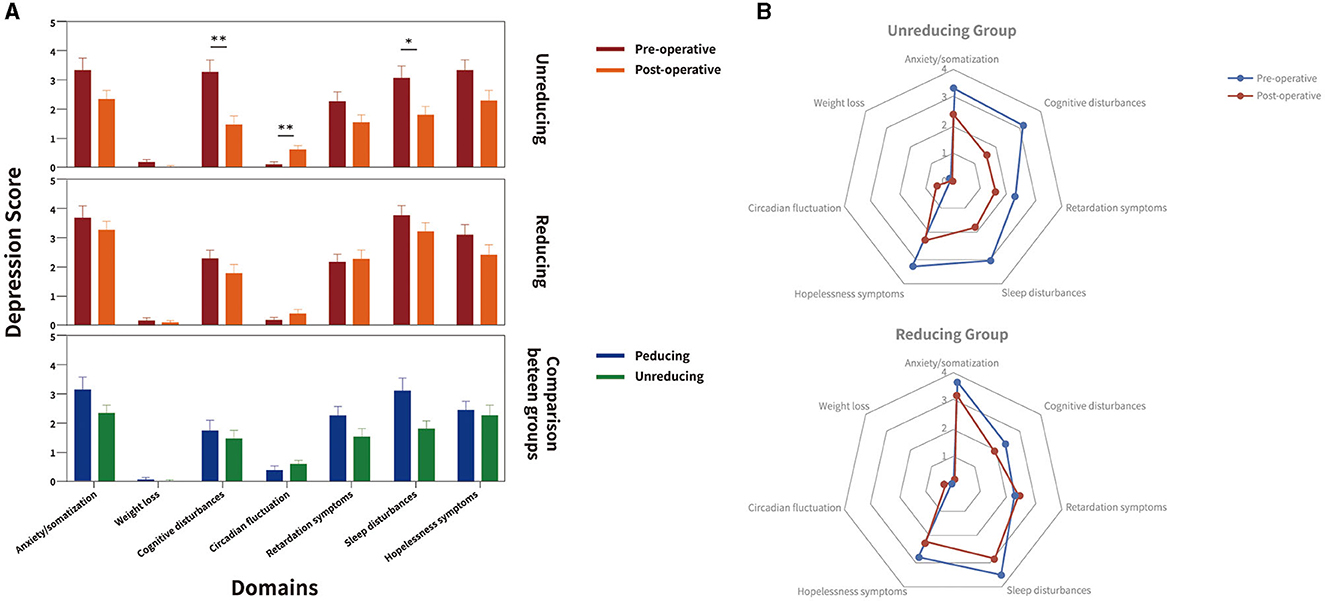
Figure 2. Depression domains at baseline and follow-up in the matched cohort for unreducing and reducing LEDD group. Figure illustrates Hamilton Rating Scale for Depression (HAMD) domains at baseline (red) and follow-up (orange) from baseline to follow-up, and the reducing (blue) and unreducing (green) groups in comparison between groups in (A) clustered box-plots and (B) radar charts. Significant intragroup improvements of HAMD domains from baseline to follow-up and between reducing and unreducing group are highlighted with black stars. In unreducing LEDD group, significant beneficial effects were observed for cognitive disturbances, sleep disturbances, the circadian fluctuation was significantly worse than before. In the reducing LEDD group, no significant change was observed between baseline and follow-up. The sleep disturbances domain of HAMD was significantly lower in unreducing LEDD group. *P < 0.05.
Effect sizes (CI) were large for MDS-UPDRS-III (MedOFF) and small for HAMA in both groups. In the non-reducing LEDD group, confidence intervals (CIs) were small for MDS-UPDRS-III (MedON) and moderate for HAMD. In the reducing LEDD group, effect sizes were moderate for MDS-UPDRS-III (MedON) and small for HAMD. In the non-reducing LEDD group, all effect sizes of depression domains were larger. Comparing relative changes of the two groups, we observed more beneficial effects of the non-reducing LEDD group regarding the total scores of depressions and anxiety, domains of depression including anxiety/somatization, weight loss, cognitive disturbances, retardation symptoms, sleep disturbances, and hopelessness symptoms (Table 3). The adjustment of various classes of drugs in the drug-reducing group is shown in Table 4.
Discussion
The current longitudinal cohort study shows that: (1) bilateral STN-DBS has significant beneficial effects on global and specific aspects of neuropsychiatric symptoms and UPDRS-III at short-term follow-up; (2) the short-term effects of DBS on neuropsychiatric symptoms are closely related to LEDD adjustment.
This is the first report of neuropsychiatric symptoms in PD patients during short-term follow-ups. We supplemented the existing medication adjustment model and found that maintaining early medication stability is more favorable for improving patients' psychiatric symptoms. PSM could balance the differences between the two groups and achieve RCT-like results. It represents the effects of these treatments as seen in clinical practice, and as such, we believe the data has real clinical significance.
STN-DBS improved motor symptoms, which was consistent with previous research (41). At the 1-year follow-up, STN-DBS improved neuropsychiatric symptoms (Supplementary Table 1). STN-DBS improves depression in PD patients in a multifactorial way that is related to motor function, anti-PD drugs, and contact location. First and foremost, improvement in motor symptoms promotes emotional relief (42). Furthermore, STN-DBS may influence monoaminergic structures (serotonergic raphe nucleus and noradrenergic locus coeruleus) to reduce depression (43, 44). Furthermore, disruption of DA transmission is important in PD patients with neuropsychiatric symptoms. Stimulation after STN-DBS may gradually restore the patient's DA interruption by increasing GABAergic transmission (45). However, neural remodeling may necessitate long-term stimulation to achieve therapeutic effects, which is consistent with our findings of long-term improvement in anxiety and depression. Finally, the anterior, medial, and ventral STN were more conducive to alleviating patients' neuropsychiatric symptoms (46). Stimulating these areas can have a preferential effect on the limbic pathways, improving the patient's depression symptoms. These systems are involved in the regulation of neuropsychiatric symptoms and may explain why long-term depression is improving.
STN-DBS can reduce anxiety and depression in the short term (47). Non-reducing LEDD outperformed reducing LEDD when relative changes and effect sizes were considered. The non-reducing LEDD group was more conducive to improving patients' neuropsychiatric symptoms in the short term, which could be due to a variety of factors. Depression is caused by depleted levels of serotonin, dopamine, and norepinephrine (NE). Furthermore, the hypothalamic-pituitary adrenal axis and hypothalamic-pituitary thyroid axis both contribute to depression pathophysiology (48, 49). Among the several types of drugs used to treat PD patients, MAO inhibitors can inhibit neurotransmitter reuptake [5-HT, NE, and dopamine (DA)], and levodopa can directly increase the patient's dopaminergic levels (50, 51). Therefore, dopaminergic treatment can alleviate anxiety and depression. However, COMT-I and amantadine may exacerbate depressive symptoms (52). According to our findings, an early medication reduction was primarily focused on the reduction of levodopa. The primary target of early postoperative drug reduction is not COMT-I and amantadine (Table 4). As a result, early drug discontinuation is not beneficial to the improvement of neuropsychiatric symptoms. This finding provides a rationale for the timing of drug reduction after DBS and opposes premature drug reduction after DBS.
Finally, there was no significant change in anxiety and depression symptoms in the early drug reduction group in the short-term postoperative period. This group's mood was not affected by the medication reduction. This finding could be attributed to the improvement of the patient's motor symptoms following DBS, which can reduce psychological distress and improve clinical symptoms, and the improvement of motor disorders allows for mindfulness-based interventions, which can reduce depression (53).
Although existing drug reduction strategies are primarily based on programmed efficacy, there was no significant difference in the programming parameters between the two groups of patients (Supplementary Table 2). Patients with mid-to-advanced PD who receive STN-DBS are frequently bothered by financial issues and levodopa-induced dyskinesia caused by high-dose, multi-type drug therapy (54, 55). Early programming is made more difficult by patients' urgent need to reduce drug dosage to improve quality of life (18, 56, 57). Most programming doctors struggle with how to quickly find the balance between optimal stimulation parameters and drug adjustment timing in a massive combination of a large number of programmed parameters and drug adjustments (10, 55). Our study has revealed that a substantial reduction in medication during the initial programming can impede the psychiatric outcomes of STN-DBS. To mitigate this scenario, it is essential to conduct multiple programming sessions and medication adjustments over the course of several weeks, gradually working toward optimizing result.
Limitations
This retrospective study primarily elucidates the phenomenon that maintaining early medication stability yields greater clinical benefits. However, it does not provide a definitive optimal timeframe for medication reduction. The practice of reducing medication only at two time points, during the initial programming and 3–6 months later, is evidently suboptimal. A more physiologically approach to postoperative programming and medication adjustment would involve gradual changes. Unfortunately, due to a large number of patients and geographical reasons related to patient residence, such an approach was not feasible in our study. In the future, remote programming may offer a solution to this issue.
Conclusions
In conclusion, (1) It was discovered that maintaining drug stability within 3–6 months is more conducive to neuropsychiatric symptom improvement; (2) Providing evidence for drug reduction timing and opposing early drug reduction after surgery; (3) one step and probably a major drug reduction at the first post-op programming will hamper the mental outcome in STN-DBS. To avoid this, the process needs fine tune individually.
Data availability statement
The original contributions presented in the study are included in the article/Supplementary material, further inquiries can be directed to the corresponding authors.
Ethics statement
The studies involving humans were approved by Beijing Tiantan Ethics Committee. The studies were conducted in accordance with the local legislation and institutional requirements. The participants provided their written informed consent to participate in this study.
Author contributions
YD: Data curation, Formal analysis, Methodology, Software, Writing—original draft. TH: Writing—review and editing, Data curation. HX: Data curation, Writing—review and editing. HF: Data curation, Writing—review and editing. FM: Project administration, Writing—review and editing. AY: Methodology, Visualization, Writing—review and editing. YB: Data curation, Formal analysis, Investigation, Software, Visualization, Writing—review and editing. JZ: Conceptualization, Funding acquisition, Project administration, Resources, Writing—review and editing.
Funding
The author(s) declare that financial support was received for the research, authorship, and/or publication of this article. The work was funded by National Natural Science Foundation of China (81830033, 81671104, and 61761166004).
Acknowledgments
We thank all the subjects who participated in this study.
Conflict of interest
The authors declare that the research was conducted in the absence of any commercial or financial relationships that could be construed as a potential conflict of interest.
Publisher's note
All claims expressed in this article are solely those of the authors and do not necessarily represent those of their affiliated organizations, or those of the publisher, the editors and the reviewers. Any product that may be evaluated in this article, or claim that may be made by its manufacturer, is not guaranteed or endorsed by the publisher.
Supplementary material
The Supplementary Material for this article can be found online at: https://www.frontiersin.org/articles/10.3389/fneur.2023.1270746/full#supplementary-material
References
1. Krack P, Batir A, Van Blercom N, Chabardes S, Fraix V, Ardouin C, et al. Five-year follow-up of bilateral stimulation of the subthalamic nucleus in advanced Parkinson's disease. N Engl J Med. (2003) 349:1925–34. doi: 10.1056/NEJMoa035275
2. Diao Y, Bai Y, Hu T, Yin Z, Liu H, Meng F, et al. A meta-analysis of the effect of subthalamic nucleus-deep brain stimulation in Parkinson's disease-related pain. Front Hum Neurosci. (2021) 15:688818. doi: 10.3389/fnhum.2021.688818
3. Odekerken VJJ, van Laar T, Staal MJ, Mosch A, Hoffmann CFE, Nijssen PCG, et al. Subthalamic nucleus versus globus pallidus bilateral deep brain stimulation for advanced Parkinson's disease (NSTAPS study): a randomised controlled trial. Lancet Neurol. (2013) 12:37–44. doi: 10.1016/S1474-4422(12)70264-8
4. de Almeida Marcelino L, Horn A, Krause P, Kühn AA, Neumann W-J. Subthalamic neuromodulation improves short-term motor learning in Parkinson's disease. Brain. (2019) 142:2198–206. doi: 10.1093/brain/awz152
5. Yin Z, Bai Y, Zou L, Zhang X, Wang H, Gao D, et al. Balance response to levodopa predicts balance improvement after bilateral subthalamic nucleus deep brain stimulation in Parkinson's disease. NPJ Parkinsons Dis. (2021) 7:47. doi: 10.1038/s41531-021-00192-9
6. Gothwal M, Arumugham SS, Yadav R, Pal PK, Hegde S. Deficits in emotion perception and cognition in patients with Parkinson's disease: a systematic review. Ann Indian Acad Neurol. (2022) 25:367–75. doi: 10.4103/aian.aian_573_21
7. Boutet A, Madhavan R, Elias GJB, Joel SE, Gramer R, Ranjan M, et al. Predicting optimal deep brain stimulation parameters for Parkinson's disease using functional MRI and machine learning. Nat Commun. (2021) 12:3043. doi: 10.1038/s41467-021-23311-9
8. Gülke E, Paz LJ, Scholtes H, Gerloff C, Kühn AA, Pötter-Nerger M. Multiple input algorithm-guided deep brain stimulation-programming for Parkinson's disease patients. NPJ Parkinsons Dis. (2022) 8:144. doi: 10.1038/s41531-022-00396-7
9. Kleinholdermann U, Bacara B, Timmermann L, Pedrosa DJ. Prediction of movement ratings and deep brain stimulation parameters in idiopathic Parkinson's disease. Neuromodulation. (2022) 26:356–63. doi: 10.1016/j.neurom.2022.09.010
10. Roediger J, Dembek TA, Wenzel G, Butenko K, Kühn AA, Horn A. StimFit-A data-driven algorithm for automated deep brain stimulation programming. Mov Disord. (2022) 37:574–84. doi: 10.1002/mds.28878
11. Xu SS, Lee W-L, Perera T, Sinclair NC, Bulluss KJ, McDermott HJ, et al. Can brain signals and anatomy refine contact choice for deep brain stimulation in Parkinson's disease? J Neurol Neurosurg Psychiatry. (2022). doi: 10.1136/jnnp-2021-327708
12. Bove F, Mulas D, Cavallieri F, Castrioto A, Chabardès S, Meon S, et al. Long-term outcomes (15 years) after subthalamic nucleus deep brain stimulation in patients with Parkinson disease. Neurology. (2021). doi: 10.1212/WNL.0000000000012246
13. Hu T, Xie H, Diao Y, Fan H, Wu D, Gan Y, et al. Effects of subthalamic nucleus deep brain stimulation on depression in patients with Parkinson's disease. J Clin Med. (2022) 11:5844. doi: 10.3390/jcm11195844
14. Zhang F, Wang F, Xing Y-J, Yang M-M, Wang J-W, Li C-H, et al. Correlation between electrode location and anxiety depression of subthalamic nucleus deep brain stimulation in Parkinson's disease. Brain Sci. (2022). 12:755. doi: 10.3390/brainsci12060755
15. Hvingelby VS, Pavese N. Surgical advances in Parkinson's disease. Curr Neuropharmacol. (2022). doi: 10.2174/1570159X21666221121094343
16. Cernera S, Eisinger RS, Wong JK, Ho KWD, Lopes JL, To K, et al. Long-term Parkinson's disease quality of life after staged DBS: STN vs GPi and first vs second lead. NPJ Parkinsons Dis. (2020) 6:13. doi: 10.1038/s41531-020-0115-3
17. Jost ST, Sauerbier A, Visser-Vandewalle V, Ashkan K, Silverdale M, Evans J, et al. A prospective, controlled study of non-motor effects of subthalamic stimulation in Parkinson's disease: results at the 36-month follow-up. J Neurol Neurosurg Psychiatry. (2020) 91:687–94. doi: 10.1136/jnnp-2019-322614
18. Hacker ML, Turchan M, Heusinkveld LE, Currie AD, Millan SH, Molinari AL, et al. Deep brain stimulation in early-stage Parkinson disease: five-year outcomes. Neurology. (2020) 95:e393–401. doi: 10.1212/WNL.0000000000009946
19. Mansouri A, Taslimi S, Badhiwala JH, Witiw CD, Nassiri F, Odekerken VJJ, et al. Deep brain stimulation for Parkinson's disease: meta-analysis of results of randomized trials at varying lengths of follow-up. J Neurosurg. (2018) 128:1199–213. doi: 10.3171/2016.11.JNS16715
20. Dietrich AD, Koeppen JA, Buhmann C, Pötter-Nerger M, Pinnschmidt HO, Oehlwein C, et al. Sex disparities in the self-evaluation of subthalamic deep brain stimulation effects on mood and personality in Parkinson's disease patients. Front Neurol. (2020) 11:776. doi: 10.3389/fneur.2020.00776
21. Shah H, Usman O, Ur Rehman H, Jhaveri S, Avanthika C, Hussain K, et al. Deep brain stimulation in the treatment of Parkinson's disease. Cureus. (2022) 14:e28760. doi: 10.7759/cureus.28760
22. Mosley PE, Akram H. Neuropsychiatric effects of subthalamic deep brain stimulation. Handb Clin Neurol. (2021) 180:417–31. doi: 10.1016/B978-0-12-820107-7.00026-4
23. Witt K, Daniels C, Volkmann J. Factors associated with neuropsychiatric side effects after STN-DBS in Parkinson's disease. Parkinsonism Relat Disord. (2012) 18:S168–70. doi: 10.1016/S1353-8020(11)70052-9
24. Volkmann J, Daniels C, Witt K. Neuropsychiatric effects of subthalamic neurostimulation in Parkinson disease. Nat Rev Neurol. (2010) 6:487–98. doi: 10.1038/nrneurol.2010.111
25. Lamy F, Lagha-Boukbiza O, Wirth T, Philipps C, Longato N, Gebus O, et al. Early hyperdopaminergic state following sub-thalamic nucleus deep brain stimulation in Parkinson disease. Rev Neurol. (2022) 178:896–906. doi: 10.1016/j.neurol.2022.07.003
26. Thobois S, Ardouin C, Lhommée E, Klinger H, Lagrange C, Xie J, et al. Non-motor dopamine withdrawal syndrome after surgery for Parkinson's disease: predictors and underlying mesolimbic denervation. Brain. (2010) 133:1111–27. doi: 10.1093/brain/awq032
27. Kalia LV, Lang AE. Parkinson's disease. Lancet. (2015) 386:896–912. doi: 10.1016/S0140-6736(14)61393-3
28. Benabid AL, Deuschl G, Lang AE, Lyons KE, Rezai AR. Deep brain stimulation for Parkinson's disease. Mov Disord. (2006) 21:S168–70. doi: 10.1002/mds.20954
29. Dafsari HS, Ghilardi MGD, Visser-Vandewalle V, Rizos A, Ashkan K, Silverdale M, et al. Beneficial nonmotor effects of subthalamic and pallidal neurostimulation in Parkinson's disease. Brain Stimul. (2020) 13:1697–705. doi: 10.1016/j.brs.2020.09.019
30. Bronstein JM, Tagliati M, Alterman RL, Lozano AM, Volkmann J, Stefani A, et al. Deep brain stimulation for Parkinson disease: an expert consensus and review of key issues. Arch Neurol. (2011) 68:165. doi: 10.1001/archneurol.2010.260
31. Zhang W-S, Gao C, Tan Y-Y, Chen S-D. Prevalence of freezing of gait in Parkinson's disease: a systematic review and meta-analysis. J Neurol. (2021) 268:4138–50. doi: 10.1007/s00415-021-10685-5
32. Lin Z, Zhang X, Wang L, Zhang Y, Zhou H, Sun Q, et al. Revisiting the L-Dopa response as a predictor of motor outcomes after deep brain stimulation in Parkinson's disease. Front Hum Neurosci. (2021) 15:604433. doi: 10.3389/fnhum.2021.604433
33. Persson RS, Nordin T, Hariz G-M, Wårdell K, Forsgren L, Hariz M, et al. Deep brain stimulation of caudal zona incerta for Parkinson's disease: one-year follow-up and electric field simulations. Neuromodulation. (2021) 25:935–44. doi: 10.1111/ner.13500
34. Tomlinson CL, Stowe R, Patel S, Rick C, Gray R, Clarke CE. Systematic review of levodopa dose equivalency reporting in Parkinson's disease. Mov Disord. (2010) 25:2649–53. doi: 10.1002/mds.23429
35. Rodriguez-Blazquez C, Frades-Payo B, Forjaz MJ, de Pedro-Cuesta J, Martinez-Martin P, Longitudinal Parkinson's Disease Patient Study Group. Psychometric attributes of the hospital anxiety and depression scale in Parkinson's disease. Mov Disord. (2009) 24:519–25. doi: 10.1002/mds.22321
36. Lian T-H, Guo P, Zuo L-J, Hu Y, Yu S-Y, Liu L, et al. An investigation on the clinical features and neurochemical changes in Parkinson's disease with depression. Front Psychiatry. (2018) 9:723. doi: 10.3389/fpsyt.2018.00723
37. Nagler A, Labopin M, Houhou M, Aljurf M, Mousavi A, Hamladji R-M, et al. Outcome of haploidentical versus matched sibling donors in hematopoietic stem cell transplantation for adult patients with acute lymphoblastic leukemia: a study from the acute leukemia working party of the European society for blood and marrow transplantation. J Hematol Oncol. (2021) 14:53. doi: 10.1186/s13045-021-01065-7
38. Gopalakrishnan C, Bykov K, Fischer MA, Connolly JG, Gagne JJ, Fralick M. Association of fluoroquinolones with the risk of aortic aneurysm or aortic dissection. JAMA Intern Med. (2020) 180:1596–605. doi: 10.1001/jamainternmed.2020.4199
39. Ouldali N, Toubiana J, Antona D, Javouhey E, Madhi F, Lorrot M, et al. Association of intravenous immunoglobulins plus methylprednisolone vs immunoglobulins alone with course of fever in multisystem inflammatory syndrome in children. JAMA. (2021) 325:855–64. doi: 10.1001/jama.2021.0694
40. Ho DE, Imai K, King G, Stuart EA. Matching as nonparametric preprocessing for reducing model dependence in parametric causal inference. Political Anal. (2017) 15:199–236. doi: 10.1093/pan/mpl013
41. Lachenmayer ML, Mürset M, Antih N, Debove I, Muellner J, Bompart M, et al. Subthalamic and pallidal deep brain stimulation for Parkinson's disease-meta-analysis of outcomes. NPJ Parkinsons Dis. (2021) 7:77. doi: 10.1038/s41531-021-00223-5
42. Huang LC, Chen L-G, Wu P-A, Pang C-Y, Lin S-Z, Tsai S-T, et al. Effect of deep brain stimulation on brain network and white matter integrity in Parkinson's disease. CNS Neurosci Ther. (2021) 28:92–104. doi: 10.1111/cns.13741
43. Birchall EL, Walker HC, Cutter G, Guthrie S, Joop A, Memon RA, et al. The effect of unilateral subthalamic nucleus deep brain stimulation on depression in Parkinson's disease. Brain Stimul. (2017) 10:651–6. doi: 10.1016/j.brs.2016.12.014
44. Faggiani E, Delaville C, Benazzouz A. The combined depletion of monoamines alters the effectiveness of subthalamic deep brain stimulation. Neurobiol Dis. (2015) 82:342–8. doi: 10.1016/j.nbd.2015.07.010
45. Lemos JC, Friend DM, Kaplan AR, Shin JH, Rubinstein M, Kravitz AV, et al. Enhanced GABA transmission drives bradykinesia following loss of dopamine D2 receptor signaling. Neuron. (2016) 90:824–38. doi: 10.1016/j.neuron.2016.04.040
46. Dafsari HS, Petry-Schmelzer JN, Ray-Chaudhuri K, Ashkan K, Weis L, Dembek TA, et al. Non-motor outcomes of subthalamic stimulation in Parkinson's disease depend on location of active contacts. Brain Stimul. (2018) 11:904–12. doi: 10.1016/j.brs.2018.03.009
47. Eisenstein SA, Dewispelaere WB, Campbell MC, Lugar HM, Perlmutter JS, Black KJ, et al. Acute changes in mood induced by subthalamic deep brain stimulation in Parkinson disease are modulated by psychiatric diagnosis. Brain Stimul. (2014) 7:701–8. doi: 10.1016/j.brs.2014.06.002
48. Khatri DK, Choudhary M, Sood A, Singh SB. Anxiety: an ignored aspect of Parkinson's disease lacking attention. Biomed Pharmacother. (2020) 131:110776. doi: 10.1016/j.biopha.2020.110776
49. Maillet A, Krack P, Lhommée E, Météreau E, Klinger H, Favre E, et al. The prominent role of serotonergic degeneration in apathy, anxiety and depression in de novo Parkinson's disease. Brain. (2016) 139:2486–502. doi: 10.1093/brain/aww162
50. Hillhouse TM, Porter JH. A brief history of the development of antidepressant drugs: from monoamines to glutamate. Exp Clin Psychopharmacol. (2015) 23:1–21. doi: 10.1037/a0038550
51. Smith KM, Eyal E, Weintraub D, Investigators A. Combined rasagiline and antidepressant use in Parkinson disease in the ADAGIO study: effects on nonmotor symptoms and tolerability. JAMA Neurol. (2015) 72:88–95. doi: 10.1001/jamaneurol.2014.2472
52. Santos-Garcia D, de Deus Fonticoba T, Castro ES, Díaz AA, Bartolomé CC, Panceiras MJF, et al. Quality of life and non-motor symptoms in Parkinson's disease patients with subthreshold depression. J Neurol Sci. (2020) 418:117109. doi: 10.1016/j.jns.2020.117109
53. van der Heide A, Meinders MJ, Speckens AEM, Peerbolte TF, Bloem BR, Helmich RC. Stress and mindfulness in Parkinson's disease: clinical effects and potential underlying mechanisms. Mov Disord. (2021) 36:64–70. doi: 10.1002/mds.28345
54. Sharma JC, Macnamara L, Hasoon M, Vassallo M, Ross I. Cascade of levodopa dose and weight-related dyskinesia in Parkinson's disease (LD-WD-PD cascade). Parkinsonism Relat Disord. (2006) 12:499–505. doi: 10.1016/j.parkreldis.2006.07.002
55. Pintér D, Járdaházi E, Balás I, Harmat M, Makó T, Juhász A, et al. Antiparkinsonian drug reduction after directional versus omnidirectional bilateral subthalamic deep brain stimulation. Neuromodulation. (2022). doi: 10.1016/j.neurom.2022.01.006
56. Dafsari HS, Martinez-Martin P, Rizos A, Trost M, Ghilardi MGD, Reddy P, et al. EuroInf 2: subthalamic stimulation, apomorphine, and levodopa infusion in Parkinson's disease. Mov Disord. (2019) 34:353–65. doi: 10.1002/mds.27626
Keywords: Parkinson's disease, short-term effects, follow-up, deep brain stimulation, propensity score matching, depression, non-motor symptoms, medicine reduction
Citation: Diao Y, Hu T, Xie H, Fan H, Meng F, Yang A, Bai Y and Zhang J (2023) Premature drug reduction after subthalamic nucleus deep brain stimulation leading to worse depression in patients with Parkinson's disease. Front. Neurol. 14:1270746. doi: 10.3389/fneur.2023.1270746
Received: 07 August 2023; Accepted: 26 September 2023;
Published: 19 October 2023.
Edited by:
Mya C. Schiess, University of Texas Health Science Center at Houston, United StatesReviewed by:
Xianlun Zhu, The Chinese University of Hong Kong, ChinaTatsuya Yamamoto, Chiba Prefectural University of Health Sciences, Japan
Copyright © 2023 Diao, Hu, Xie, Fan, Meng, Yang, Bai and Zhang. This is an open-access article distributed under the terms of the Creative Commons Attribution License (CC BY). The use, distribution or reproduction in other forums is permitted, provided the original author(s) and the copyright owner(s) are credited and that the original publication in this journal is cited, in accordance with accepted academic practice. No use, distribution or reproduction is permitted which does not comply with these terms.
*Correspondence: Yutong Bai, baiyutong88@qq.com; Jianguo Zhang, zjguo73@126.com