- 1Department of Public Health, Section of Epidemiology, University of Copenhagen, Copenhagen, Denmark
- 2Department of Internal Medicine, Levanger Hospital, Health Trust Nord-Trøndelag, Levanger, Norway
- 3Departments of Family Medicine and Epidemiology, School of Public Health, University of Washington, Seattle, WA, United States
- 4Division of Aging, Department of Medicine, Brigham and Women’s Hospital and Harvard Medical School, Boston, MA, United States
- 5Department of Nutrition, Harvard T.H. Chan School of Public Health, Boston, MA, United States
- 6Department of Neurology, School of Medicine, University of Washington, Seattle, WA, United States
- 7Department of Neurology, School of Medicine, University of Pittsburgh, Pittsburgh, PA, United States
- 8Department of Laboratory Medicine and Pathology, School of Medicine, University of Washington, Seattle, WA, United States
- 9Cardiovascular Health Research Unit, Department of Medicine, University of Washington, Seattle, WA, United States
- 10Department of Medicine, Beth Israel Deaconess Medical Center, Boston, MA, United States
Background: Sphingolipids are implicated in neurodegeneration and neuroinflammation. We assessed the potential role of circulating ceramides and sphingomyelins in subclinical brain pathology by investigating their association with brain magnetic resonance imaging (MRI) measures and circulating biomarkers of brain injury, neurofilament light chain (NfL) and glial fibrillary acidic protein (GFAP) in the Cardiovascular Health Study (CHS), a large and intensively phenotyped cohort of older adults.
Methods: Brain MRI was offered twice to CHS participants with a mean of 5 years between scans, and results were available from both time points in 2,116 participants (mean age 76 years; 40% male; and 25% APOE ε4 allele carriers). We measured 8 ceramide and sphingomyelin species in plasma samples and examined the associations with several MRI, including worsening grades of white matter hyperintensities and ventricular size, number of brain infarcts, and measures of brain atrophy in a subset with quantitative measures. We also investigated the sphingolipid associations with serum NfL and GFAP.
Results: In the fully adjusted model, higher plasma levels of ceramides and sphingomyelins with a long (16-carbon) saturated fatty acid were associated with higher blood levels of NfL [β = 0.05, false-discovery rate corrected P (PFDR) = 0.004 and β = 0.06, PFDR = < 0.001, respectively]. In contrast, sphingomyelins with very long (20- and 22-carbon) saturated fatty acids tended to have an inverse association with levels of circulating NfL. In secondary analyses, we found an interaction between ceramide d18:1/20:0 and sex (P for interaction = <0.001), such that ceramide d18:1/20:0 associated with higher odds for infarcts in women [OR = 1.26 (95%CI: 1.07, 1.49), PFDR = 0.03]. We did not observe any associations with GFAP blood levels, white matter grade, ventricular grade, mean bilateral hippocampal volume, or total brain volume.
Conclusion: Overall, our comprehensive investigation supports the evidence that ceramides and sphingomyelins are associated with increased aging brain pathology and that the direction of association depends on the fatty acid attached to the sphingosine backbone.
Introduction
Dementia is a major global public health emergency, and identifying its pathophysiological risk factors remains a key scientific priority (1–3). Subclinical vascular brain injury such as covert infarction and white matter changes contribute to cognitive decline and dementia (4–7). Additionally, cerebral small vessel disease (CSVD) has emerged as a major cause of stroke and the leading contributor to vascular dementia (8).
Sphingolipids are a group of lipids characterized by a sphingosine backbone to which a fatty acid is acylated. They are especially concentrated in myelin sheath in the brain and relay important signals for intra- and intercellular events (9–11). Circulating levels of sphingolipids have been associated with both cerebrovascular and neurodegenerative diseases such as stroke and dementia (12–14). One family of sphingolipids, the ceramides (Cer) has been shown to accumulate in tissues such as the liver and brain during aging (15–17), and emerging evidence indicates that Cer are the most dysregulated lipids in cerebrovascular diseases, including stroke and CSVD (18–21). Blood levels of Cer and sphingomyelins (SM), another class of sphingolipids that can be metabolized to Cer, have both been associated with hippocampal atrophy, white matter hyperintensities, and white matter microstructural changes (22–24).
Cer and SM with different fatty acid chain lengths may exhibit distinct biological activities (25, 26). Indeed, we have shown that higher levels of circulating Cer and SM with a saturated fatty acid chain length of 16-carbon (Cer-16 and SM-16) are directly associated with cardiovascular disease and mortality, while circulating Cer and SM with 22- and 24-carbon saturated fatty acid chain length (Cer-22, Cer-24, SM-22, SM-24) are inversely associated (27–29).
Here, we address the role of Cer and SM in subclinical brain pathology by investigating their association with a broad range of measures in the Cardiovascular Health Study (CHS), a large and extensively phenotyped cohort of older adults. Based on previous observations, we hypothesize that Cer and SM with different saturated fatty acids will differ in associations, thus this study focus on 4 Cer species with the fatty acids palmitic acid, arachidic acid, behenic acid and lignoceric acid (Cer-16, Cer-20, Cer-22, Cer-24, respectively) and 4 analogous SM species (SM-16, SM-20, SM-22, SM-24). We examined several magnetic resonance imaging (MRI) measurements such as white matter hyperintensities, ventricular size, and brain atrophy, and two circulating biomarkers of brain injury, neurofilament light chain (NfL) and glial fibrillary acidic protein (GFAP), to gain a comprehensive view of the associations of 8 sphingolipid species with these measures.
Methods
Study population
The CHS began in 1989–1990 and recruited 5,201 women and men aged 65 years in 4 different US communities: Forsyth County, NC; Sacramento County, CA; Washington County, MD; Allegheny CA. In 1992–1993, an additional 687 predominantly African American participants were recruited in 3 of the 4 original communities. Potential participants were randomly identified from Medicare eligibility lists. The study design and sampling methods have been previously described in detail (30). During the first decade of the study, participants underwent annual clinic visits, followed by semiannual phone contacts thereafter. Each clinic visit involved comprehensive physical examinations and the collection of demographics, anthropometry, blood pressure, psychosocial interviews, depression assessments, medical history, health behaviors, physical function evaluations, hematology, laboratory examinations, and medication records. For the present analyses, sphingolipids were measured in plasma samples from 586 participants from 1992 to 1993 visit and 4,026 participants from the 1994–1995 visit (total N = 4,612). The clinic visit from which sphingolipids were measured (1992–1993 or 1994–1995) formed the study baseline in the analyses. Figure 1 shows the flow and number of participants included in each of our analyses.
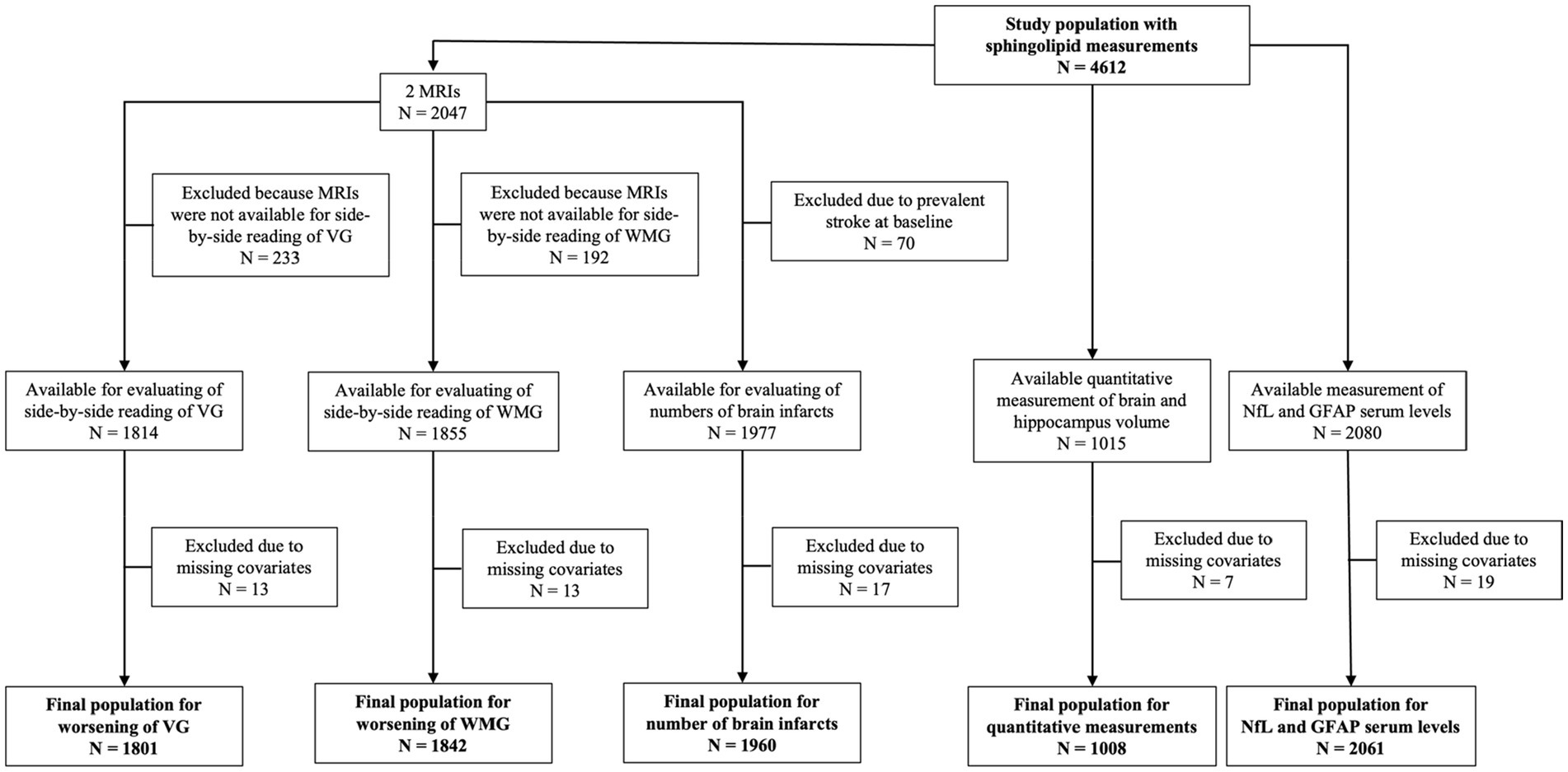
Figure 1. Flowchart of study populations. GFAP, glial fibrillary acidic protein; MRI, magnetic resonance imaging; NfL, neurofilament light chain; VG, ventricular grade; WMG, white matter grade.
Measurements of sphingolipids
Measurement of sphingolipid species were performed using EDTA-plasma samples that had been stored at −70°C. Plasma lipids were extracted, and sphingolipids quantified by liquid chromatography–tandem mass spectrometry. Previous work suggests that sphingolipid species are robust to storage at −70°C (31). A comprehensive description of the methods and quality control for sphingolipid measurement, including details on lipid extraction, sample plating, instrumentation, internal standards, and normalization, can be found in previous publications (27, 32). In the current study, we restricted analyses to 8 primary sphingolipids. This included 4 Cer species with the fatty acids palmitic acid, arachidic acid, behenic acid and lignoceric acid (Cer-16, Cer-20, Cer-22, Cer-24, respectively) and 4 analogous SM species (SM-16, SM-20, SM-22, SM-24).
Brain imaging
Brain MRI was offered twice to CHS participants in 1992–1994 and 1997–1999, as described earlier (33, 34). Results were available from both time points in 2,116 participants with a mean of 5 years between scans. Non-contrast MRI imaging included sagittal T1-weighted, spin-density, and T2-weighted images with 5-mm thickness and no interslice gaps (35). At a single reading center, neuroradiologists rated the MRI scans blinded to study information (33).
White matter hyperintensities
White matter hyperintensities (WMH) were identified on either axial T2-weighted or spin-density images scans and graded on a similar 10-point semiquantitative white matter grade (WMG), ranging from 0 (least) to 9 (most abnormal). WMG had an inter-reader interclass kappa correlation coefficient of 0.76 and intra-reader kappa coefficient of 0.89 (36, 37). To assess white matter grades over time, we used MRIs eligible for side-by-side re-reading. Because of technical problems, 197 of the original 2,116 pairs of scans could not be re-read, leaving 1,919 (91%) pairs (38). To document worsening WMG of 1 or more grades (yes vs. no), the neuroradiologists read all scans from the same participant side-by-side without knowledge of which scan was performed first (38).
Ventricular grade
Ventricular sizes were estimated from the T1-weighted axial images. The size of the lateral ventricles was estimated by the radiologist based on a referenced standard to create a ventricular grade (VG) ranging from 0 (least) to 9 (most abnormal) (39). Intra-reader agreement within 1 grade was 94% with a kappa of 0.89 for the VG (39). Worsening of VG by one or more grades (yes vs. no) was determined by neuroradiologists in side-by-side reading without knowledge of which scan was performed first.
Total brain volume and mean bilateral hippocampi volume
A subgroup of the follow-up MRIs was performed using a 1.5-tesla scanner and included 3D T1-weighted spoiled gradient-recall sequences, as described previously (40). These scans were processed with FreeSurfer software, which converted high-resolution T1-weighted images into 1x1x1 mm3 voxels and generated a surface-based reconstruction of the brain to estimate the quantitative volume of the hippocampus and total intracranial space, which were then converted to normalized brain volume (1-NBV) units. Volumetric measurements from the right and left hippocampus were averaged to compute the mean bilateral hippocampal volume.
Brain infarcts
Brain infarcts were defined as abnormal signal intensities 3 mm in size or larger, identified by neuroradiologists on MRI scans. Number of brain infarcts was defined as one or more new infarcts on the follow-up MRI.
Quantification of blood NfL and GFAP
Frozen fasting serum samples collected from participants at the 1996–1997 clinic visit were used to measure NfL and GFAP. The CHS Central Laboratory at the University of Vermont measured NfL and GFAP using the 4th generation single-molecule array Simoa™ Human Neurology 4-Plex A assay (N4PA, Quanterix™). As the measurements were funded as part of a metabolic ancillary study to CHS, they were limited to participants who underwent an oral glucose tolerance test that excluded participants with treated diabetes. The inter-assay coefficients of variation were 9.3% for NfL and 8.2% for GFAP.
Covariates
Information on age (years), race/ethnicity (Caucasian, African American), education (years), physical activity (kcal/week), alcohol intake (drinks/week), smoking status (never, former, current), medication-use, and CESD Depression score was based on self-report. Weight, height, and blood pressure were measured using standardized protocols. Body mass index (BMI) was calculated as weight in kilograms divided by height in meters squared (kg/m2). Hypertension was defined by systolic blood pressure ≥ 140 mm Hg or diastolic blood pressure ≥ 90 mm Hg or use of antihypertensive medication. Plasma lipids and glucose were measured on fasting blood samples using standard protocols (30). Prevalent diabetes mellitus was defined by a fasting glucose concentration of ≥7 mmol/L (126 mg/dL) or use of insulin or oral hypoglycemic agents. History of stroke and coronary heart disease was obtained from medical records, participant and/or proxy reports and adjudicated by committee (41, 42).
Statistical analysis
Baseline characteristics were presented for continuous variables as mean (SD) and for categorical variables as numbers (percentages). Sphingolipid species concentrations were log-transformed to reduce skewness. We used non-parametric testing to estimate correlations between sphingolipid species.
Logistic regression was used to assess associations with the dichotomous outcomes of VG and WMG worsening on follow-up MRI, using each sphingolipid specie concentration as a continuous variable [per one standard deviation (SD) in log sphingolipid species concentration (μM)]. We used ordinal logistic regression to model the associations with number of infarcts, where the number of infarcts was categorized as 0, 1 to 2, and ≥3 based on evaluation of the proportional odds assumption using a score test. We excluded all participants with prevalent stroke (N = 70) at baseline for this analysis. These analyses were initially adjusted for age (years), sex, race/ethnicity (Caucasian, African American), education (years), field center (4 sites), and baseline year (1992–1993 or 1994–1995) (Model 1). Next, we additionally included BMI (kg/m2), physical activity (kcal/week), alcohol intake (drinks/week), smoking status (never, former, current), depression score (continuous), diabetes (yes/no), coronary heart disease (yes/no), hypertension (yes/no), lipid-lowering medication use (yes/no), HDL-cholesterol (mg/dL), and LDL-cholesterol (mg/dL) (Model 2). All covariates were assessed at the respective baseline visit. Due to mutual correlation and contrasting biological properties, a third model was included in which Cer/SM with an acylated 16-carbon fatty acid were adjusted for Cer/SM with 22-carbon fatty acid, and Cer/SM with 20-, 22-, 24-carbon fatty acids were each adjusted for Cer/SM with a 16-carbon fatty acid (Model 3). This approach has been reported elsewhere (27–29, 43).
The associations with total brain volume and mean bilateral hippocampi volume were assessed using linear regression, adjusted for the same covariates as above and estimated total intracranial volume. The associations with circulating NfL and GFAP were also analyzed using linear regression models. Due to skewness, we winsorized 10 GFAP outliers to the value of 1,000 pg./mL and 9 NfL outliers to the value of 250 pg./mL and transformed their values to the natural logarithm (lognNfl or lognGFAP).
In secondary analyses, we tested for interactions with sex and race/ethnicity of the associations of sphingolipids with brain pathology by inclusion of multiplicative interaction terms in the respective models. Lastly, we performed stratified analyses based on APOE genotype, as APOEε4 carriers have increased risk of development of neurodegenerative diseases such as dementia. Analyses were limited to those with available DNA who consented to genetic studies.
To correct the analyses for multiple comparisons, we applied a false discovery rate (FDR) p-value correction in which p = 0.05 was considered significant. All statistical analyses were conducted using R version 4.1.3 (44).
Results
Figure 1 shows the number of CHS participants in each analysis, based upon outcome and exclusions ranging from N = 1,008 for quantitative analyses of brain volumes to N = 2,061 for circulating NfL and GFAP. No major differences in population characteristics between the five groups included in the different analyses were observed. Mean age was 76 years, and 57.9% to 60.4% of participants were female and 12.6% to 15.7% were African American, depending on the analyses (Supplementary Table S1). The measured sphingolipid species showed moderate inter-correlations, with stronger correlations observed between sphingolipids with very long chain fatty acid (20-, 22-, and 24-carbon) and coefficients of variation estimated over the whole study period ranged from 5.9 to 18.6% (Supplementary Table S2).
No associations with volumetric measurements, WMG, or VG worsening over 5 years
We observed no clear associations for any of the sphingolipid species with worsening in WMG, decrease in total brain volume, or mean bilateral hippocampal volume. In the fully adjusted model, elevated plasma levels of Cer-16 and SM-16 were associated with higher odds of worsening in VG, but this finding was not statistically significant upon p-value correction. In contrast, Cer-22 was associated with lower odds of worsening in VG, which also became non-significant upon p-value correction (data not shown).
Cer-20 associates with higher number of brain infarcts in women
Higher plasma levels of Cer-20 associated with increased odds for more brain infarcts in Model 1 [OR = 1.18 (1.07, 1.31), PFDR = 0.008] and 2 [OR = 1.16 (1.04, 1.29), PFDR = 0.03], which attenuated upon p-value correction in Model 3 (OR = 1.17 (1.03, 1.32), PFDR = 0.07; Figure 2; Supplementary Table S3 for estimates of Model 1 and Model 2). In sex-stratified analysis, Cer-20 was associated with 26% higher odds [OR = 1.26 (1.07, 1.49), PFDR = 0.03, P for interaction = <0.001] in women and not in men (Table 1).
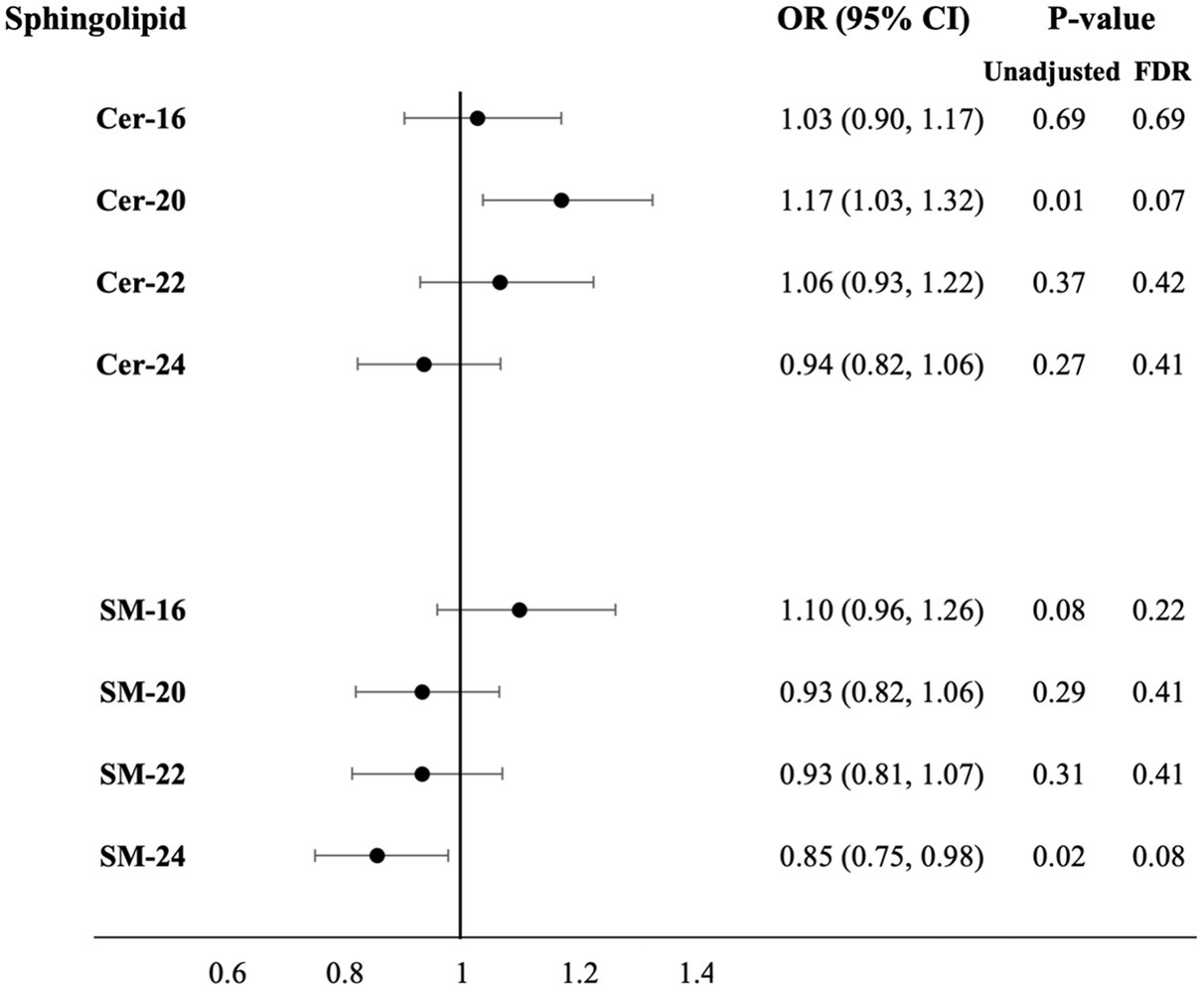
Figure 2. Adjusted odds ratios for increase in number of brain infarcts per SD higher plasma log-sphingolipid concentrations, N = 1,960. Cer, ceramide; CI, confidence interval; FDR, false discovery rate; OR, odds ratio; SD, standard deviation; SM, sphingomyelins; 16, 20, 22, and 24 stand for the number of carbons of the saturated fatty acid acylated to the sphingolipid backbone. Odds ratios adjusted for age, sex, race/ethnicity, education, field center, year of blood sample measurement, BMI, physical activity, alcohol intake, smoking status, depression score, prevalent diabetes, prevalent coronary heart disease, prevalent hypertension, lipid-lowering medication use, HDL-cholesterol, LDL-cholesterol and mutual adjustment of sphingolipids with 16-carbon fatty acid chains with corresponding sphingolipids with 22-carbon fatty acid chains and sphingolipid with 20-, 22-, 24-carbon fatty acid chains with sphingolipids with 16-carbon fatty acid chains (Model 3).
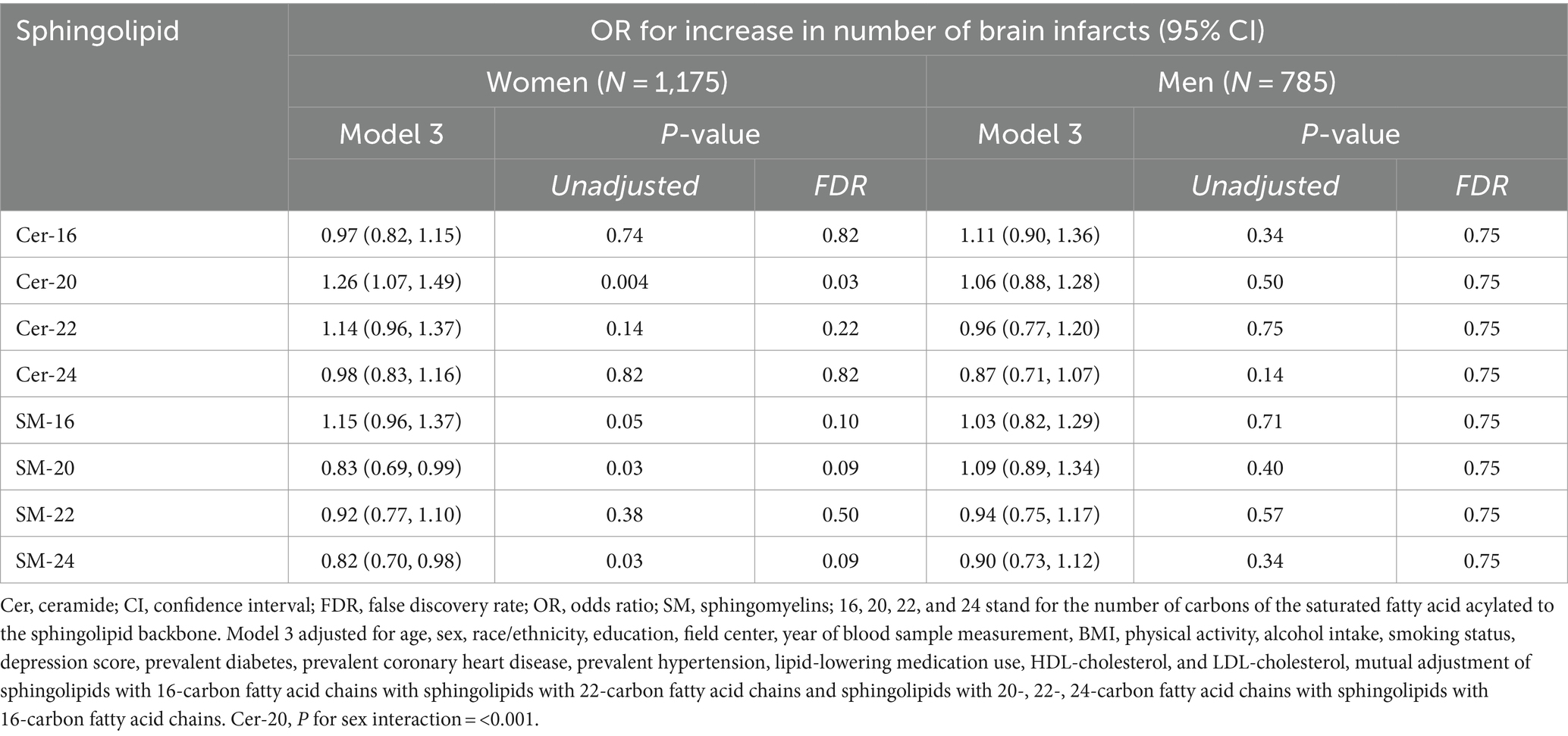
Table 1. Adjusted odds ratios for increase in number of brain infarcts per SD higher plasma log-sphingolipid concentrations in sex-stratified analysis.
Cer-16 and SM-16 associates with higher circulating NfL levels
Higher plasma levels of Cer and SM with long fatty acids were associated with higher levels of circulating NfL: Cer-16 (β = 0.05, PFDR = 0.004) and SM-16 (β = 0.06, PFDR = <0.001). In contrast, higher levels of several Cer and SM with very long chain fatty acids were associated with lower levels of circulating NfL; Cer-20 (β = −0.03, PFDR = 0.05), SM-20 (β = −0.03, PFDR = 0.03), and SM-22 (β = −0.04, PFDR = 0.02; Figure 3; Supplementary Table S4 for estimates of Model 1 and Model 2). In APOE genotype stratified analysis, higher levels of SM-16 in APOE ε4 carriers associated with higher levels of circulating NfL (β = 0.07, PFDR = 0.03), whereas the association was not statistically significant in APOE ε4 non-carriers (β = 0.03, PFDR = 0.22; Supplementary Table S5). For associations with levels of circulation GFAP, no associations were observed after covariate adjustments and p-value correction.
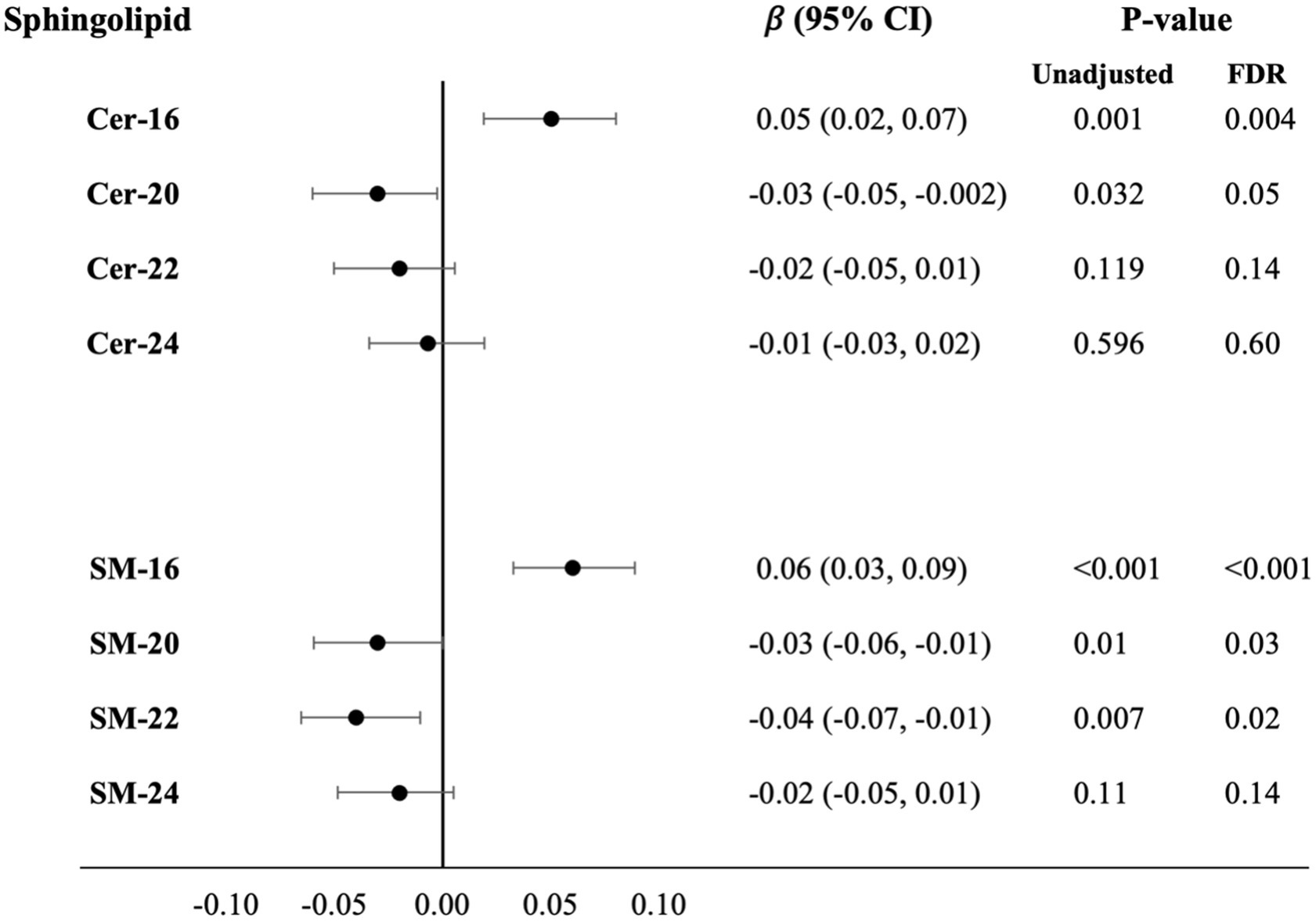
Figure 3. Cross-sectional associations with difference in serum NfL levels per SD higher plasma log-sphingolipid concentrations, N = 2,061. Cer, ceramide; CI, confidence interval; FDR, false discovery rate; NfL, neurofilament light chain; SD, standard deviation; SM, sphingomyelins; 16, 20, 22, and 24 stand for the number of carbons of the saturated fatty acid acylated to the sphingolipid backbone. Linear regression beta coefficients adjusted for age, sex, race/ethnicity, education, field center, year of blood sample measurement, BMI, physical activity, alcohol intake, smoking status, depression score, prevalent diabetes, prevalent coronary heart disease, prevalent hypertension, lipid-lowering medication use, HDL-cholesterol, LDL-cholesterol and mutual adjustment of sphingolipids with 16-carbon fatty acid chains with corresponding sphingolipids with 22-carbon fatty acid chains and sphingolipid with 20-, 22-, 24-carbon fatty acid chains with sphingolipids with 16-carbon fatty acid chains (Model 3).
Discussion
Overall, our comprehensive investigation supports the evidence that Cer and SM species with a bound (acylated) 16-carbon long fatty acid are associated with increased signs of degenerative changes on MRI. In this prospective study in older adults, higher plasma levels of Cer-16 and SM-16 were associated with higher blood levels of NfL, a biomarker of brain injury, and tended to associate with worsening of VG over 5 years, however, non-significant upon adjustment for multiple comparisons. In APOE ε4 carriers, higher levels of SM-16 associated with higher levels of circulating NfL, and no associations were observed in APOE ε4 non-carriers. Additionally, Cer and SM with a very long (20- and 22-carbon) chain fatty acids show an inverse association with levels of circulating NfL. However, Cer-20 also associated with higher odds for infarcts in women in secondary analyses. We did not observe any associations with levels of GFAP, WMG or mean bilateral hippocampal volume.
Previous epidemiological studies have found that higher plasma levels of Cer-16 associate with greater WMH volumes (23) and higher plasma levels of Cer-22 and Cer-24 associate with greater right hippocampal volume loss 1 year later in 17 patients with amnesiac mild cognitive impairment (22). We did not confirm either of these associations, although we did find that Cer-16 appears to be associated with ongoing brain injury and global atrophy. Part of the difference in these results may relate to the prospective nature of our white matter findings, which specifically examined progression late in life. Another possibility might be that Cer-16 confounded the associations of Cer-22 and Cer-24.
To the best of our knowledge, our results are novel, as no previous study has reported on the associations of sphingolipids and VG or blood levels of NfL. Neuronal cell death is a hallmark of brain atrophy, including ventricular enlargement. Ceramides have been implicated in promoting apoptosis and neuronal loss through various mechanisms, including mitochondrial dysfunction, activation of pro-apoptotic pathways, and disruption of neuronal survival signaling (45, 46). Both Cer-16 and SM-16 have been associated with neurodegenerative diseases such as dementia including Alzheimer’s disease (22, 47–55), Huntington’s and Parkinson’s disease (56–59) as well as neuroinflammation (60, 61). Our study also showed that Cer-16 and SM-16, but not other species of Cer and SM, associated with higher levels of NfL in blood, which is a nonspecific biomarker for neuronal damage. Recent studies in CHS have shown that serum NfL and GFAP were associated with substantially higher risk of incident dementia and dementia mortality (62) and WMG worsening over 5 years (37). Altered sphingolipid metabolism may similarly reflects increased neurodegeneration, neuroinflammation or both and might be valuable as a biomarker in conjunction with NfL and GFAP. The reason for the differences observed in our findings regarding NfL and GFAP is unclear. However, our previous research has shown a tendency for NfL to exhibit a higher correlation with vascular brain injuries compared to GFAP in CHS (37).
Our findings provide crucial evidence that the direction of association of Cer and SM with several brain pathology outcome depends on the fatty acid attached to the sphingosine backbone. Our research demonstrates that elevated baseline plasma levels of Cer-16 and SM-16 are associated with a higher degree of brain pathology, while higher baseline plasma levels of Cer and SM with very-long chain fatty acids are independently inversely associated. Several cell and animal studies suggest that Cer have different biological properties dependent on the length of the fatty acid chain (25, 26). Diverse Cer can alter cell membrane properties, where fluidity increases with higher levels of Cer-16 and lower amounts of Cer-22 and Cer-24 (25, 63). The enzyme ceramide synthases 2 (CerS2) produces very-long chain ceramides and a study in CerS2 null mice showed that these mice had defective myelin sheath with lower levels of Cer-24 and higher levels Cer-16 (64). Another study of downregulation of CerS2 observed induced cell autophagy with a reduction in the levels of Cer-24 and increase in Cer-16 (65). Overexpression of ceramide synthases 6 (CerS6), which produces long-chain ceramides (C14-C18), increased the secretion of the inflammatory cytokine TNF-α in the liver (66). In addition, several studies report that Cer-16 promotes apoptosis, while Cer-22 and Cer-24 appear to protect from apoptosis (14, 25, 26, 67, 68). Most studies have investigated ceramides; however, ceramides and sphingomyelins are highly interrelated and can be generated from each other.
This study has several strengths, which involve the large sample size, and the comprehensive assessment of multiple complementary subclinical brain pathology measurements including two MRIs performed 5 years apart, enabling longitudinal analysis. We also included circulating neuronal biomarkers to complement the radiological findings. Several limitations include the study participants being older and primarily Caucasian, thus our findings may not generalize to other ethnicities or younger populations. Additionally, the MRI measurements in CHS were performed in the 1990s and did not include imaging of fractional anisotropy. Also, additional brain imaging studies, such as PET scanning for proteinopathies, were not performed.
Lastly, further studies are warranted to determine whether the timing of the measurements (midlife vs. late life) is important. The levels of sphingolipids change with age (69–71), thus multiple measurements over a long time will be needed to fully understand the dynamics of sphingolipid metabolism with relation to disease.
Conclusion
In this study of older adults, Cer and SM differed in association with several measurements of subclinical brain pathology including number of brain infarcts, and NfL blood levels, which were dependent on the length of the fatty acid chain bound. Confirmation of these results in other studies with repetitive measurements of sphingolipids, MRI, levels of circulating NfL and GFAP is necessary to establish their role biomarkers of degenerative and vascular brain injury.
Data availability statement
The data analyzed in this study is subject to the following licenses/restrictions: access to data can be done through CHS. Requests to access these datasets should be directed to CHS-NHLBI.org.
Ethics statement
The studies involving humans were approved by the Institutional Review Board at University of Washington. The studies were conducted in accordance with the local legislation and institutional requirements. The participants provided their written informed consent to participate in this study.
Author contributions
KFM: Conceptualization, Formal analysis, Investigation, Visualization, Writing – original draft, Writing – review & editing. JH: Writing – original draft, Writing – review & editing. AF: Writing – original draft, Writing – review & editing. LD: Writing – original draft, Writing – review & editing. WL: Writing – original draft, Writing – review & editing. OL: Writing – original draft, Writing – review & editing. AH: Writing – original draft, Writing – review & editing. MJ: Supervision, Writing – original draft, Writing – review & editing. RL: Writing – original draft, Writing – review & editing. KJM: Supervision, Writing – original draft, Writing – review & editing.
Funding
The author(s) declare financial support was received for the research, authorship, and/or publication of this article. This research was supported by contracts HHSN268201200036C, HHSN268200800007C, HHSN268201800001C, N01HC55222, N01HC85079, N01HC85080, N01HC85081, N01HC85082, N01HC85083, N01HC85086, and 75N92021D00006 and grants U01HL080295 and U01HL130114 from the National Heart, Lung, and Blood Institute (NHLBI), with additional contribution from the National Institute of Neurological Disorders and Stroke (NINDS). Additional support was provided by R01AG023629 from the National Institute on Aging (NIA). Support for individual investigators include K24AG065525 from the NIA to KJM and the Novo Nordic Foundation Challenge Programme: Harnessing the Power of Big Data to Address the Societal Challenge of Aging (NNF17OC0027812) to KFM and MJ.
Acknowledgments
A full list of principal CHS investigators and institutions can be found at CHS-NHLBI.org.
Conflict of interest
The authors declare that the research was conducted in the absence of any commercial or financial relationships that could be construed as a potential conflict of interest.
Publisher’s note
All claims expressed in this article are solely those of the authors and do not necessarily represent those of their affiliated organizations, or those of the publisher, the editors and the reviewers. Any product that may be evaluated in this article, or claim that may be made by its manufacturer, is not guaranteed or endorsed by the publisher.
Supplementary material
The Supplementary material for this article can be found online at: https://www.frontiersin.org/articles/10.3389/fneur.2024.1385623/full#supplementary-material
Abbreviations
Cer, Ceramide; GFAP, Glial fibrillary acidic protein; MRI, Magnetic resonance imaging; NfL, Neurofilament light chain; SM, Sphingomyelin; VG, Ventricular grade; WMH, White matter hyperintensities
References
1. GBD 2017 Disease and Injury Incidence and Prevalence Collaborators. Global, regional, and national incidence, prevalence, and years lived with disability for 354 diseases and injuries for 195 countries and territories, 1990-2017: a systematic analysis for the global burden of Disease study 2017. Lancet. (2018) 392:1789–858. doi: 10.1016/S0140-6736(18)32279-7
2. 2021 Alzheimer's disease facts and figures. Alzheimers Dement. (2021) 17:327–406. doi: 10.1002/alz.12328
3. Livingston, G, Huntley, J, Sommerlad, A, Ames, D, Ballard, C, Banerjee, S, et al. Dementia prevention, intervention, and care: 2020 report of the lancet commission. Lancet. (2020) 396:413–46. doi: 10.1016/S0140-6736(20)30367-6
4. Emery, VO, Gillie, EX, and Smith, JA. Noninfarct vascular dementia and Alzheimer dementia spectrum. J Neurol Sci. (2005) 229-230:27–36. doi: 10.1016/j.jns.2004.11.016
5. Ott, A, Breteler, MMB, van Harskamp, F, Claus, JJ, van der Cammen, TJM, Grobbee, DE, et al. Prevalence of Alzheimer's disease and vascular dementia: association with education. The Rotterdam study. BMJ. (1995) 310:970–3. doi: 10.1136/bmj.310.6985.970
6. Román, GC, Tatemichi, TK, Erkinjuntti, T, Cummings, JL, Masdeu, JC, Garcia, JH, et al. Vascular dementia: diagnostic criteria for research studies. Report of the NINDS-AIREN international workshop. Neurology. (1993) 43:250–60. doi: 10.1212/WNL.43.2.250
7. Corriveau, RA, Bosetti, F, Emr, M, Gladman, JT, Koenig, JI, Moy, CS, et al. The science of vascular contributions to cognitive impairment and dementia (VCID): a framework for advancing research priorities in the cerebrovascular biology of cognitive decline. Cell Mol Neurobiol. (2016) 36:281–8. doi: 10.1007/s10571-016-0334-7
8. Lam, BYK, Cai, Y, Akinyemi, R, Biessels, GJ, van den Brink, H, Chen, C, et al. The global burden of cerebral small vessel disease in low- and middle-income countries: a systematic review and meta-analysis. Int J Stroke. (2023) 18:15–27. doi: 10.1177/17474930221137019
9. Gault, CR, Obeid, LM, and Hannun, YA. Sphingolipids as Signaling and regulatory molecules. New York: Springer (2010).
10. Zheng, W, Kollmeyer, J, Symolon, H, Momin, A, Munter, E, Wang, E, et al. Ceramides and other bioactive sphingolipid backbones in health and disease: lipidomic analysis, metabolism and roles in membrane structure, dynamics, signaling and autophagy. Biochim Biophys Acta. (2006) 1758:1864–84. doi: 10.1016/j.bbamem.2006.08.009
11. Gault, CR, Obeid, LM, and Hannun, YA. An overview of sphingolipid metabolism: from synthesis to breakdown. Adv Exp Med Biol. (2010) 688:1–23. doi: 10.1007/978-1-4419-6741-1_1
12. Yuan, H, Zhu, B, Li, C, and Zhao, Z. Ceramide in cerebrovascular diseases. Front Cell Neurosci. (2023) 17:1191609. doi: 10.3389/fncel.2023.1191609
13. Baloni, P, Arnold, M, Buitrago, L, Nho, K, Moreno, H, Huynh, K, et al. Multi-Omic analyses characterize the ceramide/sphingomyelin pathway as a therapeutic target in Alzheimer's disease. Commun Biol. (2022) 5:1074. doi: 10.1038/s42003-022-04011-6
14. Crivelli, SM, Giovagnoni, C, Visseren, L, Scheithauer, AL, de Wit, N, den Hoedt, S, et al. Sphingolipids in Alzheimer's disease, how can we target them? Adv Drug Deliv Rev. (2020) 159:214–31. doi: 10.1016/j.addr.2019.12.003
15. Cutler, RG, and Mattson, MP. Sphingomyelin and ceramide as regulators of development and lifespan. Mech Ageing Dev. (2001) 122:895–908. doi: 10.1016/S0047-6374(01)00246-9
16. Lightle, SA, Oakley, JI, and Nikolova-Karakashian, MN. Activation of sphingolipid turnover and chronic generation of ceramide and sphingosine in liver during aging. Mech Ageing Dev. (2000) 120:111–25. doi: 10.1016/S0047-6374(00)00191-3
17. Trayssac, M, Hannun, YA, and Obeid, LM. Role of sphingolipids in senescence: implication in aging and age-related diseases. J Clin Invest. (2018) 128:2702–12. doi: 10.1172/JCI97949
18. Gui, YK, Li, Q, Liu, L, Zeng, P, Ren, RF, Guo, ZF, et al. Plasma levels of ceramides relate to ischemic stroke risk and clinical severity. Brain Res Bull. (2020) 158:122–7. doi: 10.1016/j.brainresbull.2020.03.009
19. Ighodaro, ET, Graff-Radford, J, Syrjanen, JA, Bui, HH, Petersen, RC, Knopman, DS, et al. Associations between plasma ceramides and cerebral microbleeds or lacunes. Arterioscler Thromb Vasc Biol. (2020) 40:2785–93. doi: 10.1161/ATVBAHA.120.314796
20. Hannawi, Y, Yanek, LR, Kral, BG, Becker, LC, Vaidya, D, Haughey, NJ, et al. White matter Injury is associated with reduced manual dexterity and elevated serum ceramides in subjects with cerebral small vessel Disease. Cerebrovasc Dis. (2021) 50:100–7. doi: 10.1159/000511937
21. Lee, TH, Cheng, CN, Chao, HC, Lee, CH, Kuo, CH, Tang, SC, et al. Plasma ceramides are associated with outcomes in acute ischemic stroke patients. J Formos Med Assoc. (2022) 121:43–50. doi: 10.1016/j.jfma.2021.01.006
22. Mielke, MM, Haughey, NJ, Bandaru, VVR, Schech, S, Carrick, R, Carlson, MC, et al. Plasma ceramides are altered in mild cognitive impairment and predict cognitive decline and hippocampal volume loss. Alzheimers Dement. (2010) 6:378–85. doi: 10.1016/j.jalz.2010.03.014
23. Mielke, MM, Syrjanen, JA, Bui, HH, Petersen, RC, Knopman, DS, Jack, CR, et al. Elevated plasma ceramides are associated with higher white matter hyperintensity volume-brief report. Arterioscler Thromb Vasc Biol. (2019) 39:2431–6. doi: 10.1161/ATVBAHA.119.313099
24. Gonzalez, CE, Venkatraman, VK, An, Y, Landman, BA, Davatzikos, C, Ratnam Bandaru, VV, et al. Peripheral sphingolipids are associated with variation in white matter microstructure in older adults. Neurobiol Aging. (2016) 43:156–63. doi: 10.1016/j.neurobiolaging.2016.04.008
25. Mu, J, Lam, SM, and Shui, G. Emerging roles and therapeutic potentials of sphingolipids in pathophysiology --emphasis on fatty acyl heterogeneity. J Genet Genomics. (2023) 51:268–78. doi: 10.1016/j.jgg.2023.06.006
26. Grosch, S, Schiffmann, S, and Geisslinger, G. Chain length-specific properties of ceramides. Prog Lipid Res. (2012) 51:50–62. doi: 10.1016/j.plipres.2011.11.001
27. Lemaitre, RN, Jensen, PN, Hoofnagle, A, McKnight, B, Fretts, AM, King, IB, et al. Plasma ceramides and sphingomyelins in relation to heart failure risk. Circ Heart Fail. (2019) 12:e005708. doi: 10.1161/CIRCHEARTFAILURE.118.005708
28. Jensen, PN, Fretts, AM, Hoofnagle, AN, Sitlani, CM, McKnight, B, King, IB, et al. Plasma ceramides and sphingomyelins in relation to atrial fibrillation risk: the cardiovascular health study. J Am Heart Assoc. (2020) 9:e012853. doi: 10.1161/JAHA.119.012853
29. Fretts, AM, Jensen, PN, Hoofnagle, AN, McKnight, B, Sitlani, CM, Siscovick, DS, et al. Circulating ceramides and sphingomyelins and risk of mortality: the cardiovascular health study. Clin Chem. (2021) 67:1650–9. doi: 10.1093/clinchem/hvab182
30. Fried, LP, Borhani, NO, Enright, P, Furberg, CD, Gardin, JM, Kronmal, RA, et al. The cardiovascular health study: design and rationale. Ann Epidemiol. (1991) 1:263–76. doi: 10.1016/1047-2797(91)90005-W
31. Brunkhorst, R, Pfeilschifter, W, Patyna, S, Büttner, S, Eckes, T, Trautmann, S, et al. Preanalytical biases in the measurement of human blood sphingolipids. Int J Mol Sci. (2018) 19:1390. doi: 10.3390/ijms19051390
32. Lemaitre, RN, Yu, C, Hoofnagle, A, Hari, N, Jensen, PN, Fretts, AM, et al. Circulating sphingolipids, insulin, HOMA-IR and HOMA-B: the strong heart family study. Diabetes. (2018) 67:1663–72. doi: 10.2337/db17-1449
33. Longstreth, WT, Bernick, C, Manolio, TA, Bryan, N, Jungreis, CA, and Price, TR. Lacunar infarcts defined by magnetic resonance imaging of 3660 elderly people: the cardiovascular health study. Arch Neurol. (1998) 55:1217–25. doi: 10.1001/archneur.55.9.1217
34. Longstreth, WT, Dulberg, C, Manolio, TA, Lewis, MR, Beauchamp, NJ, O’Leary, D, et al. Incidence, manifestations, and predictors of brain infarcts defined by serial cranial magnetic resonance imaging in the elderly: the cardiovascular health study. Stroke. (2002) 33:2376–82. doi: 10.1161/01.STR.0000032241.58727.49
35. Bryan, RN, Manolio, TA, Schertz, LD, Jungreis, C, Poirier, VC, Elster, AD, et al. A method for using MR to evaluate the effects of cardiovascular disease on the brain: the cardiovascular health study. AJNR Am J Neuroradiol. (1994) 15:1625–33.
36. Longstreth, WT, Manolio, TA, Arnold, A, Burke, GL, Bryan, N, Jungreis, CA, et al. Clinical correlates of white matter findings on cranial magnetic resonance imaging of 3301 elderly people. Stroke. (1996) 27:1274–82. doi: 10.1161/01.STR.27.8.1274
37. Fohner, AE, Bartz, TM, Tracy, RP, Adams, HHH, Bis, JC, Djousse, L, et al. Association of Serum Neurofilament Light Chain Concentration and MRI findings in older adults: the cardiovascular health study. Neurology. (2022) 98:e903–11. doi: 10.1212/WNL.0000000000013229
38. Longstreth, WT, Arnold, AM, Beauchamp, NJ, Manolio, TA, Lefkowitz, D, Jungreis, C, et al. Incidence, manifestations, and predictors of worsening white matter on serial cranial magnetic resonance imaging in the elderly: the cardiovascular health study. Stroke. (2005) 36:56–61. doi: 10.1161/01.STR.0000149625.99732.69
39. Kuller, LH, Arnold, AM, Longstreth, WT, Manolio, TA, O’Leary, DH, Burke, GL, et al. White matter grade and ventricular volume on brain MRI as markers of longevity in the cardiovascular health study. Neurobiol Aging. (2007) 28:1307–15. doi: 10.1016/j.neurobiolaging.2006.06.010
40. Raji, CA, Lopez, OL, Kuller, LH, Carmichael, OT, Longstreth, WT, Gach, HM, et al. White matter lesions and brain gray matter volume in cognitively normal elders. Neurobiol Aging. (2012) 33:834.e7–834.e16. doi: 10.1016/j.neurobiolaging.2011.08.010
41. Longstreth, WT, Bernick, C, Fitzpatrick, A, Cushman, M, Knepper, L, Lima, J, et al. Frequency and predictors of stroke death in 5,888 participants in the cardiovascular health study. Neurology. (2001) 56:368–75. doi: 10.1212/WNL.56.3.368
42. Psaty, BM, Delaney, JA, Arnold, AM, Curtis, LH, Fitzpatrick, AL, Heckbert, SR, et al. Study of cardiovascular health outcomes in the era of claims data: the cardiovascular health study. Circulation. (2016) 133:156–64. doi: 10.1161/CIRCULATIONAHA.115.018610
43. Jensen, PN, Fretts, AM, Hoofnagle, AN, McKnight, B, Howard, BV, Umans, JG, et al. Circulating ceramides and sphingomyelins and the risk of incident cardiovascular disease among people with diabetes: the strong heart study. Cardiovasc Diabetol. (2022) 21:167. doi: 10.1186/s12933-022-01596-4
44. R Core Team. R: A language and environment for statistical computing. Vienna, Austria: R Foundation for Statistical Computing (2021).
45. Gulbins, E, and Li, PL. Physiological and pathophysiological aspects of ceramide. Am J Physiol Regul Integr Comp Physiol. (2006) 290:R11–26. doi: 10.1152/ajpregu.00416.2005
46. Pettus, BJ, Chalfant, CE, and Hannun, YA. Ceramide in apoptosis: an overview and current perspectives. Biochim Biophys Acta. (2002) 1585:114–25. doi: 10.1016/S1388-1981(02)00331-1
47. Mielke, MM, Bandaru, VVR, Haughey, NJ, Xia, J, Fried, LP, Yasar, S, et al. Serum ceramides increase the risk of Alzheimer disease: the Women's health and aging study II. Neurology. (2012) 79:633–41. doi: 10.1212/WNL.0b013e318264e380
48. Mielke, MM, Bandaru, VVR, Haughey, NJ, Rabins, PV, Lyketsos, CG, and Carlson, MC. Serum sphingomyelins and ceramides are early predictors of memory impairment. Neurobiol Aging. (2010) 31:17–24. doi: 10.1016/j.neurobiolaging.2008.03.011
49. McGrath, ER, Himali, JJ, Xanthakis, V, Duncan, MS, Schaffer, JE, Ory, DS, et al. Circulating ceramide ratios and risk of vascular brain aging and dementia. Ann Clin Transl Neurol. (2020) 7:160–8. doi: 10.1002/acn3.50973
50. Toledo, JB, Arnold, M, Kastenmüller, G, Chang, R, Baillie, RA, Han, X, et al. Metabolic network failures in Alzheimer's disease: a biochemical road map. Alzheimers Dement. (2017) 13:965–84. doi: 10.1016/j.jalz.2017.01.020
51. Huynh, K, Lim, WLF, Giles, C, Jayawardana, KS, Salim, A, Mellett, NA, et al. Concordant peripheral lipidome signatures in two large clinical studies of Alzheimer’s disease. Nat Commun. (2020) 11:5698. doi: 10.1038/s41467-020-19473-7
52. Mielke, MM, Haughey, NJ, Bandaru, VVR, Weinberg, DD, Darby, E, Zaidi, N, et al. Plasma sphingomyelins are associated with cognitive progression in Alzheimer's disease. J Alzheimers Dis. (2011) 27:259–69. doi: 10.3233/JAD-2011-110405
53. Mielke, MM, Haughey, NJ, Bandaru, VVR, Zetterberg, H, Blennow, K, Andreasson, U, et al. Cerebrospinal fluid sphingolipids, beta-amyloid, and tau in adults at risk for Alzheimer's disease. Neurobiol Aging. (2014) 35:2486–94. doi: 10.1016/j.neurobiolaging.2014.05.019
54. Mielke, MM, Haughey, NJ, Han, D, An, Y, Bandaru, VVR, Lyketsos, CG, et al. The association between plasma ceramides and sphingomyelins and risk of Alzheimer’s Disease differs by sex and APOE in the Baltimore longitudinal study of aging. J Alzheimers Dis. (2017) 60:819–28. doi: 10.3233/JAD-160925
55. Varma, VR, Oommen, AM, Varma, S, Casanova, R, An, Y, Andrews, RM, et al. Brain and blood metabolite signatures of pathology and progression in Alzheimer disease: a targeted metabolomics study. PLoS Med. (2018) 15:e1002482. doi: 10.1371/journal.pmed.1002482
56. Lin, G, Wang, L, Marcogliese, PC, and Bellen, HJ. Sphingolipids in the pathogenesis of Parkinson's Disease and parkinsonism. Trends Endocrinol Metab. (2019) 30:106–17. doi: 10.1016/j.tem.2018.11.003
57. Esfandiary, A, Finkelstein, DI, Voelcker, NH, and Rudd, D. Clinical sphingolipids pathway in Parkinson's Disease: from GCase to integrated-biomarker discovery. Cells. (2022) 11:1353. doi: 10.3390/cells11081353
58. Phillips, GR, Saville, JT, Hancock, SE, Brown, SHJ, Jenner, AM, McLean, C, et al. The long and the short of Huntington's disease: how the sphingolipid profile is shifted in the caudate of advanced clinical cases. Brain Commun. (2022) 4:fcab303. doi: 10.1093/braincomms/fcab303
59. di Pardo, A, Basit, A, Armirotti, A, Amico, E, Castaldo, S, Pepe, G, et al. De novo synthesis of sphingolipids is defective in experimental models of Huntington's Disease. Front Neurosci. (2017) 11:698. doi: 10.3389/fnins.2017.00698
60. Morrow, A, Panyard, DJ, Deming, YK, Jonaitis, E, Dong, R, Vasiljevic, E, et al. Cerebrospinal fluid sphingomyelins in Alzheimer's Disease, neurodegeneration, and neuroinflammation. J Alzheimers Dis. (2022) 90:667–80. doi: 10.3233/JAD-220349
61. Lee, JY, Jin, HK, and Bae, JS. Sphingolipids in neuroinflammation: a potential target for diagnosis and therapy. BMB Rep. (2020) 53:28–34. doi: 10.5483/BMBRep.2020.53.1.278
62. Cronjé, HT, Liu, X, Odden, MC, Moseholm, KF, Seshadri, S, Satizabal, CL, et al. Serum NfL and GFAP are associated with incident dementia and dementia mortality in older adults: the cardiovascular health study. Alzheimers Dement. (2023) 19:5672–80. doi: 10.1002/alz.13367
63. Pewzner-Jung, Y, Park, H, Laviad, EL, Silva, LC, Lahiri, S, Stiban, J, et al. A critical role for ceramide synthase 2 in liver homeostasis: I. Alterations in lipid metabolic pathways. J Biol Chem. (2010) 285:10902–10. doi: 10.1074/jbc.M109.077594
64. Imgrund, S, Hartmann, D, Farwanah, H, Eckhardt, M, Sandhoff, R, Degen, J, et al. Adult ceramide synthase 2 (CERS2)-deficient mice exhibit myelin sheath defects, cerebellar degeneration, and hepatocarcinomas. J Biol Chem. (2009) 284:33549–60. doi: 10.1074/jbc.M109.031971
65. Spassieva, SD, Mullen, TD, Townsend, DM, and Obeid, LM. Disruption of ceramide synthesis by CerS2 down-regulation leads to autophagy and the unfolded protein response. Biochem J. (2009) 424:273–83. doi: 10.1042/BJ20090699
66. Kim, MH, Ahn, HK, Lee, EJ, Kim, SJ, Kim, YR, Park, JW, et al. Hepatic inflammatory cytokine production can be regulated by modulating sphingomyelinase and ceramide synthase 6. Int J Mol Med. (2017) 39:453–62. doi: 10.3892/ijmm.2016.2835
67. Deng, X, Yin, X, Allan, R, Lu, DD, Maurer, CW, Haimovitz-Friedman, A, et al. Ceramide biogenesis is required for radiation-induced apoptosis in the germ line of C. elegans. Science. (2008) 322:110–5. doi: 10.1126/science.1158111
68. Hartmann, D, Lucks, J, Fuchs, S, Schiffmann, S, Schreiber, Y, Ferreirós, N, et al. Long chain ceramides and very long chain ceramides have opposite effects on human breast and colon cancer cell growth. Int J Biochem Cell Biol. (2012) 44:620–8. doi: 10.1016/j.biocel.2011.12.019
69. Mielke, MM, Bandaru, VVR, Han, D, An, Y, Resnick, SM, Ferrucci, L, et al. Demographic and clinical variables affecting mid- to late-life trajectories of plasma ceramide and dihydroceramide species. Aging Cell. (2015) 14:1014–23. doi: 10.1111/acel.12369
70. Mielke, MM, Bandaru, VVR, Han, D, An, Y, Resnick, SM, Ferrucci, L, et al. Factors affecting longitudinal trajectories of plasma sphingomyelins: the Baltimore longitudinal study of aging. Aging Cell. (2015) 14:112–21. doi: 10.1111/acel.12275
Keywords: white matter hyperintensity, ventricular size, hippocampal atrophy, brain infarcts, neurofilament light chain, glial fibrillary acidic protein, ceramide, sphingomyelin
Citation: Moseholm KF, Horn JW, Fitzpatrick AL, Djoussé L, Longstreth WT Jr, Lopez OL, Hoofnagle AN, Jensen MK, Lemaitre RN and Mukamal KJ (2024) Circulating sphingolipids and subclinical brain pathology: the cardiovascular health study. Front. Neurol. 15:1385623. doi: 10.3389/fneur.2024.1385623
Edited by:
Zilong Hao, Sichuan University, ChinaReviewed by:
Simone M. Crivelli, Université de Lausanne, SwitzerlandPaola Costa-Mallen, Bastyr University, United States
Copyright © 2024 Moseholm, Horn, Fitzpatrick, Djoussé, Longstreth, Lopez, Hoofnagle, Jensen, Lemaitre and Mukamal. This is an open-access article distributed under the terms of the Creative Commons Attribution License (CC BY). The use, distribution or reproduction in other forums is permitted, provided the original author(s) and the copyright owner(s) are credited and that the original publication in this journal is cited, in accordance with accepted academic practice. No use, distribution or reproduction is permitted which does not comply with these terms.
*Correspondence: Kristine F. Moseholm, kristine.moseholm@sund.ku.dk
†These authors have contributed equally to this work