- 1Department of Neurology, The Second Xiangya Hospital, Central South University, Changsha, China
- 2China National Clinical Research Center on Mental Disorders, Changsha, China
- 3Department of Medical Genetics, The Second Xiangya Hospital, Central South University, Changsha, China
- 4Key Laboratory of Hunan Province in Neurodegenerative Disorders, Central South University, Changsha, China
Dizziness and postural instability are frequently observed symptoms in patient with Parkinson’s disease (PD), potentially linked to vestibular dysfunction. Despite their significant impact on quality of life, these symptoms are often overlooked and undertreated in clinical practice. This review aims to summarize symptoms associated with vestibular dysfunction in patients with PD and discusses vestibular-targeted therapies for managing non-specific dizziness and related symptoms. We conducted searches in PubMed and Web of Science using keywords related to vestibular dysfunction, Parkinson’s disease, dizziness, and postural instability, alongside the reference lists of relevant articles. The available evidence suggests the prevalence of vestibular dysfunction-related symptoms in patients with PD and supports the idea that vestibular-targeted therapies may be effective in improving PD symptoms.
1 Introduction
Parkinson’s disease (PD) is a neurodegenerative disease with a slow rate of progression primarily characterized by movement disorders, including resting tremors, rigidity, and bradykinesia. These symptoms often stand out prominently and have consistently been the primary focus of attention and treatment for most healthcare professionals. In contrast, dizziness and balance disorders are often overlooked as common symptoms in the elderly. Nevertheless, the prevalence of dizziness in patients with PD is twice higher than in normal elderly individuals (1), and the prevalence of balance disorders is indisputably higher, which greatly affects their quality of life (QoL) and urgently requires adequate attention and treatment.
The vestibular system, which integrates visual, proprioceptive, and vestibular signals, is the largest sensory system in the human body and is crucial for maintaining postural equilibrium and spatial orientation (2). Vestibular dysfunction is prevalent in PD (3–6) increasing the risk of falls (2, 7, 8). It is associated with dizziness, imbalance, gaze instability, and spatial disorientation, significantly affecting the QoL of patients (9, 10). The symptoms associated with vestibular dysfunction in patients with neurodegenerative diseases, including impaired balance, dizziness, and spatial disorientation, have recently been discussed (10). In addition, the anatomical and functional correlations between PD and vestibular system dysfunction have also been assessed (4). However, few reviews have focused on vestibular dysfunction-associated symptoms in patients with PD. Moreover, by integrating vestibular assessment and targeted therapeutic approaches into clinical practice, healthcare professionals can provide more nuanced and comprehensive care for patients with PD. Therefore, in this review, we summarize the clinical characteristics of vestibular dysfunction-related symptoms in patients with PD and explore vestibular-targeted therapies for nonspecific dizziness of PD with the aim of bridging the gap between identifying vestibular dysfunction and its effective management in PD.
2 Symptoms of vestibular dysfunction in patients with PD
Vestibular dysfunction in PD might be associated with motor symptoms, including postural instability, Pisa syndrome, freezing of gait, abnormal eye movement, and non-motor symptoms such as dizziness, sleep disturbances, mood abnormalities, and cognitive impairment (Figure 1).
2.1 Associations between vestibular dysfunction and motor symptoms of PD
Resting tremors, limb rigidity, bradykinesia, postural abnormalities, gait dysfunction, and axial symptoms are established markers of disability in patients with PD. They usually manifest as a stooped or externally flexed posture, with reduced or increased variability in stride length and gait speed. As the main sensory system in maintaining balance and gait, the vestibular system might be involved in the mechanisms that drive such anomalies. Accordingly, vestibular laboratory tests have substantiated various such abnormalities in patients with PD (Table 1).
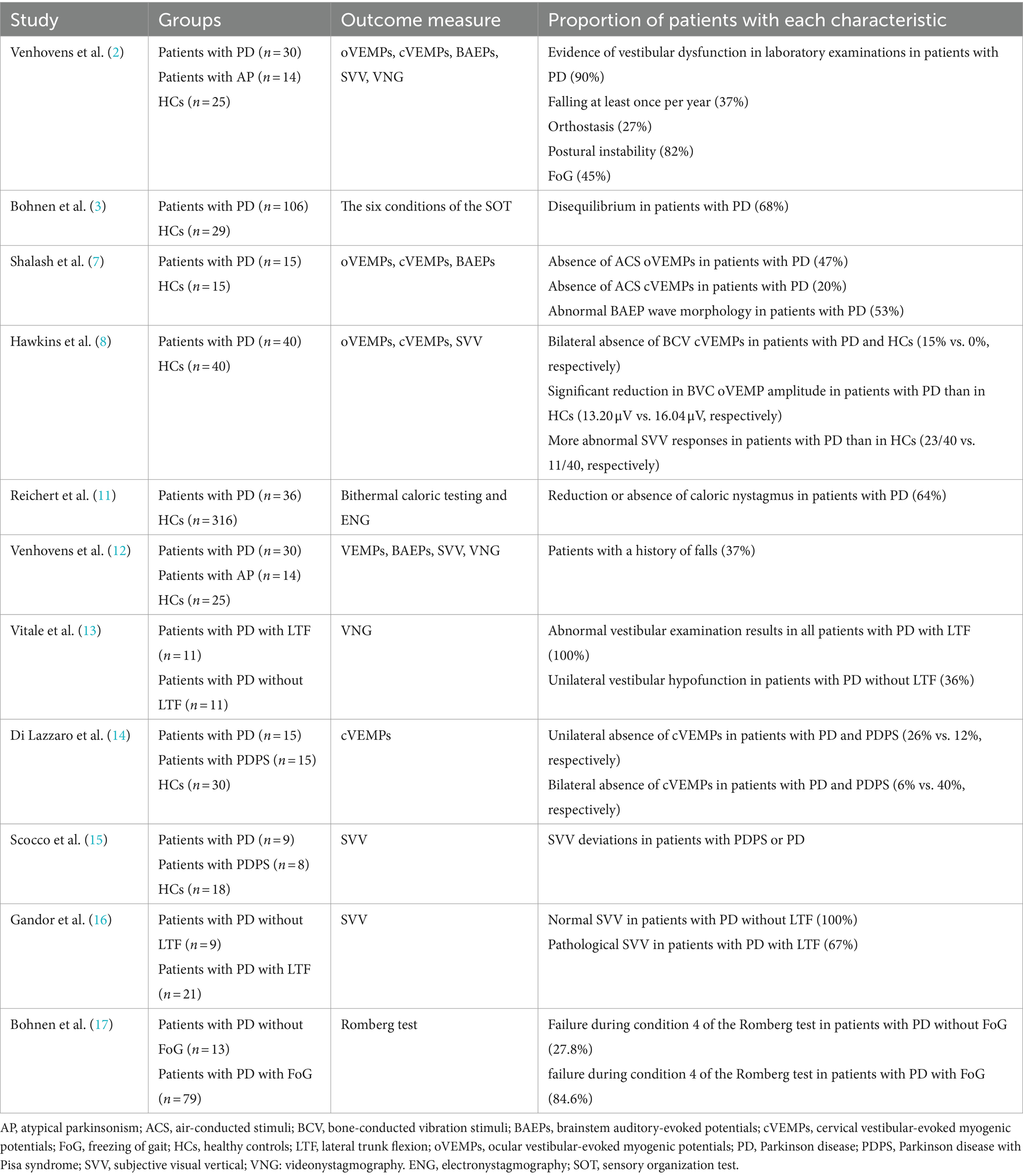
Table 1. Summary of studies associated with abnormal vestibular laboratory findings in Parkinson’s disease (PD).
2.1.1 Postural instability
Postural instability (PI) is one of the most distressing motor symptoms in PD and significantly increases the risk of falls (18, 19). Approximately 70% of patients with PD fall at least once annually (2, 20); moreover, this instability is known to increase with PD progression. PI occurs in approximately one-third of patients 2 years after the diagnosis of PD, rising to 71% after 10 years, and reaching 92% after 15 years (21). Vestibular signaling plays a crucial role in processing ego-motion information and regulating posture and balance (10), with vestibular dysfunction being an independent risk factor for falls in patients with PD and animal models (2–4). Six sensory integration tests using the NeuroCom Dynamic Postural Balance Instrument revealed that disrupted balance in patients with PD resulted from the inability to effectively interpret vestibular information independent of visual and proprioceptive integration and the loss of nigrostriatal dopamine during disease progression (3).
Altered vestibular-evoked myogenic potentials (VEMPs) increase as PD progresses to advanced stages and correlate with PI (22, 23), suggesting that impaired vestibular activity is a critical underlying factor. A prospective one-year follow-up study found that neurovestibular dysfunction might predict falls in at-risk patients with PD and imbalanced posture (12), suggesting that a baseline assessment using VEMPs may help predict future PI occurrence. Overall, PI in PD is associated with vestibular dysfunction.
Regarding anatomical localization, vestibular dysfunction in PD is associated with changes in the substantia nigra and pedunculopontine nucleus (PPN)–thalamic cholinergic innervation. For example, altered levels of vesicular acetylcholine transporters in the medial geniculate body are associated with PI and gait abnormalities in patients with PD (24). Cholinergic nerve endings in the vestibular brainstem nuclei include the caudal medial vestibular nucleus (25). Therefore, vestibular sensory information processing could be modulated by cholinergic signaling, further implicating vestibular dysfunction in PI development.
2.1.2 Pisa syndrome
Pisa syndrome (PS), a disabling complication of PD, is defined as a specific set of recoverable postural changes involving ≥10° of lateral trunk flexion (LTF) and vestibular defects (13, 26, 27). The estimated prevalence of PS in patients with PD (PDPS) is 7.4–10.3% (28); these patients are more likely to have disordered balance and experience falls than those without PS (27). Therefore, PS severely reduces the QoL of patients and can lead to fractures and death, especially during advanced stages. As the primary system involved in regulating postural balance, vestibular dysfunction might be associated with PS development in patients with PD (29, 30).
Unilateral damage to the vestibulospinal reflex arc in experimental animals causes an imbalance in the descending motor regulation system of the spinal cord, resulting in scoliosis (31). Bilateral vestibulospinal reflexes are defective in patients with PDPS, who tend to have more severe cervical VEMP (cVEMP) abnormalities than healthy controls, suggesting an association between the vestibulospinal pathway and PS pathophysiology in PD (14). Scocco et al. (15) found that 14 of 21 patients with PD and LTF had pathological subjective visual vertical (SVV) perception; Gandor et al. (16) reported similar results. Therefore, vestibular balance disorders might be involved in LTF pathophysiology. Vitale et al. examined 11 patients with PD and LTF and found vestibular hypofunction in all of them (13). These findings suggested a potential connection between the postural changes in PD and a combination of vestibular dysfunction and altered somatosensory integration, with vestibular dysfunction driving the mechanisms leading to scoliosis in PD.
2.1.3 Freezing of gait
Freezing of gait (FoG) is a unique and disabling clinical phenomenon characterized by brief episodes of inability to step forward or by extremely short steps that typically occur on initiating gait or turning while walking. Symptoms of FoG in patients with PD are also associated with vestibular dysfunction. Approximately 84.6% of patients experiencing FoG failed the modified Romberg 4 subtest, which focuses more specifically on altered vestibular function (17). Thus, vestibular processing deficits might pathophysiologically correlate with FoG in patients with PD.
Postural sensory processing, particularly of vestibular information, is poorer in patients with FoG, possibly due to impaired central processing of vestibular signals (32). Primates have a high vestibular response in the PPN (33). In addition, PPN connectivity can be enhanced via galvanic vestibular stimulation (GVS) in patients with PD (34), while rotational stimulation of the vestibular system might alleviate FoG (35). A study of four patients with PD who had undergone bilateral PPN and subthalamic (STN) electrode implantation found that deep brain stimulation (DBS) of the PPN improved vestibular perceptual thresholds, further confirming the response of PPN neurons to vestibular stimuli (36). Thus, the PPN might be a vestibular signal processing center within the brainstem, and its stimulation might improve posture and gait in patients with PD by modulating vestibular signals, thereby reducing the risk of falling. However, definitive proof of a causal relationship between FoG and impaired vestibular processing is lacking and further investigation is required.
2.1.4 Eye movement abnormalities
The vestibular system controls not only gait and posture but also eye movements, mainly through the vestibular-ocular reflex (VOR), which detects head movements and maintains image stability on the retinal fovea. Pathological changes involving the formation of Lewy bodies induced by the immune response to α-synuclein, the vestibular nucleus, oculomotor nucleus, and basal and upper ganglia affect saccadic and microsaccadic eye movements (37). Coincidentally, evidence of VOR abnormalities have been reported in many early studies of PD (4, 38).
A study of eye movements in 35 patients with idiopathic PD using videonystagmography (VNG) revealed visual and vestibular motoneuron abnormalities (39). Similarly, patients with PD exhibited poorer stereopsis and impaired oculomotor behaviors, as assessed using a three-dimensional active shutter system and Tobii Eye Tracker, respectively (40). Large meta-analyses have also confirmed the occurrence of increased response latencies in pro- and anti-saccade tasks in patients with PD (41, 42). A cross-sectional study of oculomotor performance found prolonged saccadic latencies, poorer saccadic accuracy, and lower gain in smooth pursuit eye movement (SPEM) in patients with de novo PD compared with those in healthy individuals (43). In addition, vestibular rehabilitation (VR) was shown to help improve the VOR gain (44). Therefore, eye movement abnormalities in patients with PD appear to be closely associated with vestibular system changes. However, extensive case–control studies are needed to confirm this.
2.2 Associations between vestibular dysfunction and common nonmotor symptoms of PD
The impact of non-motor symptoms on the QoL of patients with advanced PD can be more significant than that of motor symptoms due to a lower treatment response. Some non-motor symptoms associated with vestibular dysfunction are dizziness, sleep and mood disorders, and cognitive issues.
2.2.1 Dizziness
The prevalence of dizziness complaints among patients with PD (48–68%) is twice as high as that among older individuals (20–30%) (1, 45). A case–control study reported that patients with de novo PD sometimes experience dizziness for many years before disease diagnosis (46). Dizziness in patients with PD is most often attributed to orthostatic hypotension (OH) (47, 48). Accordingly, various cross-sectional studies have shown that 30–50% of patients with PD have OH (49, 50). However, many patients with PD without OH also complain of dizziness. This type of dizziness is called non-specific dizziness and refers to a symptom of feeling dizzy or lightheaded without a specific identifiable cause. Of note, non-specific dizziness is the second most prevalent type of dizziness after OH. A retrospective study including 80 patients with early-stage PD (disease duration ≤5 years) found that 37 (46.3%) presented with dizziness, which was non-specific in 11 (29.7%) of the cases (1).
Although the mechanism leading to non-specific dizziness in patients with PD is unclear, it may be affected by vestibular dysfunction. A prospective study of 84 patients with PD with and without OH found greater impairment of vestibular function in patients with dizziness compared with those without dizziness (51). An electronystagmographic assessment of 30 patients with PD found vestibular disorders in 83% of them, with a 43.3% prevalence of dizziness despite the absence of complaints (52).
In summary, dizziness is prevalent among patients with PD; the symptoms of non-specific dizziness might be closely associated with vestibular system dysfunction. Thus, targeted vestibular therapy or improving dysfunctional vestibular-related neural circuits might help relieve non-specific dizziness in patients with PD.
2.2.2 Sleep disorders
Vestibular dysfunction might also be linked with sleep disorders. A study of nine adults with abnormal sleep patterns and shorter sleep durations uncovered greater bilateral vestibular hypofunction in these patients than in healthy controls (53). A cross-sectional study of 20,950 patients also found that 30% of individuals with vestibular vertigo had abnormal sleep durations; those with vestibular symptoms were more likely to experience insomnia or lethargy (54). Changes in VEMPs were found to directly correlate with rapid eye movement (REM), sleep behavior disorder, and PI (22). cVEMP abnormalities directly correlated with sleep scores in patients assessed using the REM Sleep Behavior Disorder Screening Questionnaire (55). Additionally, a small randomized controlled trial of 20 adults found that repeated electrical vestibular stimulation administered for 30 min/day improved total Insomnia Severity Index scores (56). These results indicated that vestibular pathways could project into multiple sleep and circadian-regulating nuclei in the brainstem and hypothalamus.
Mechanistically, the vestibular system regulates circadian rhythms and influences sleep behaviors by converging inputs from the visual and somatosensory systems (57). The higher centers of the vestibular system include many subcortical and cortical structures (58), which correlate with the function of the nerve center that regulates sleep; the hypothalamic circadian rhythm in animals is modulated through a neuroanatomical pathway between the medial vestibular nuclei and suprachiasmatic nucleus (59). In addition to sending projections to the cerebellar and brainstem nuclei, glutamatergic neurons in the vestibular nucleus project to the sleep–wake centers associated with brain regions that might be involved in sleep regulation (60).
Although the precise molecular mechanisms remain unknown, orexin expression increases after sleep deprivation (61). Interestingly, sleep regulation has been reported to depend on the activity of orexin-producing neurons in experimental animals. Orexin-producing neurons are closely associated with the vestibular system (62), supporting the correlation between the vestibular system and sleep regulation.
2.2.3 Mood disorders
Mood disorders are a group of psychological disorders predominantly characterized by abnormal emotional regulation. Anxiety, fear, depression, and other neuropsychiatric symptoms are common in the general population and among patients with PD, although the underlying neurobiological mechanisms are complex and unclear (63). Many patients with impaired vestibular function also have emotional disorders, including those related to anxiety and fear (64). These relationships are not unidirectional, as mood disorders such as anxiety can lead to vestibular dysfunction and vice versa.
Ventricles synthesize adrenocorticotropic hormones, participate in processes associated with stress and anxiety, act on the vestibulolateral nucleus, and help regulate postural balance. Thus, stress, anxiety, and balance control are closely related to vestibular nuclei activity (65). Clinical findings have shown that caloric vestibular stimulation modulates mood and affective control (66); for instance, unrealistic optimism was selectively reduced during cold caloric stimulation of the left ear (67). The underlying mechanism might involve the vestibular cortex, and laterality may also be significant.
The right hemisphere is superior in dealing with negative emotions (68, 69). Similarly, vestibular cortical regulation is also affected by hemispheric lateralization, with the right hemisphere being more active than the vestibular cortex projection area in the left hemisphere in right-handed individuals (70). Hence, the right hemisphere might specialize in processes associated with interpreting or regulating negative emotions. More specifically, the right prefrontal region plays a role in negative emotional regulation associated with vestibular stimulation (71, 72). These findings indicated that cerebral hemisphere lateralization plays essential roles in vestibular and emotional processing, thus providing a theoretical basis for the interaction between the vestibular system and emotional processing centers.
2.2.4 Cognitive challenges
Vestibular cognitive (also known as advanced vestibular) function is associated with visuospatial interaction, attention, executive function, and memory; accumulating evidence have supported a link between vestibular disorders and cognitive impairment in humans (73–75). Thus, cognitive decline in patients with PD might be associated with vestibular dysfunction.
Patients with PD exhibit visuospatial processing capacity deficits associated with aberrant neural circuitry in the frontal basal ganglia (76). However, this alone does not fully explain the cause of visuospatial deficits in PD. Similar to the basal ganglia, the vestibular system is also involved in spatial processing; vestibular dysfunction has been identified as the cause of decreased visuospatial competence in patients with PD (77). Patients with PD are more likely to experience cognitive dysfunction if they have gait and balance disorders; moreover, both symptoms worsen with disease progression (78). Dizziness might also be associated with cognitive decline in PD. For example, dizziness was closely associated with low Montreal Cognitive Assessment scores in patients with early-stage PD (1). Conversely, patients with refractory dizziness experienced significant improvement in cognitive function and dizziness-related indicators following VR therapy (79).
Vestibular function and cognitive processing might be linked to hippocampal activity (80). The hippocampus is involved in emotional processing closely associated with cognitive processes such as spatial memory (81). The human posterior hippocampus is involved in information processing and spatial memory, whereas the ventral hippocampus is responsible for emotional regulation (82).
The mechanisms underlying cognitive dysfunction in PD appear to be inextricably linked to the function of the vestibular system. However, further investigation is required to delineate these relationships.
2.2.5 Other non-motor symptoms
Vestibular signal transmission and reception frequently coincide with those of other types of sensory information; central vestibular processing is promiscuous, contributing to its ubiquitous nature. Hearing loss has recently been recognized as an additional non-motor symptom of PD (83, 84). The factors affecting hearing loss are multifaceted, and common diseases such as otitis media with effusion also play a role in vestibular dysfunction (85). Hearing and balance function have certain neural connections and pathophysiologic mechanisms with motor control. Thus, neurodegeneration in PD may affect these common neural pathways, leading to hearing and balance problems. Although other non-motor symptoms, such as perception deficits, urinary problems, and cardiovascular and sexual dysfunction, are seemingly unrelated to vestibular function, some studies have suggested associations between these symptoms and VEMPs (7, 22). Thus, symptoms associated with vestibular system dysfunction are prevalent in patients with PD. While the underlying mechanisms are not fully understood, current pathologic, physiologic, and anatomic evidence support the idea that patients with PD have vestibular dysfunction (Table 2).
3 Treatment options for relieving non-specific dizziness associated with vestibular dysfunction in patients with PD
3.1 Vestibular rehabilitation therapy
Although medications are indispensable for treating certain diseases, they can also cause serious side effects that might limit their use. In contrast, non-invasive VR therapy has been associated with better compliance than pharmacotherapy. Due to its consistently recognized effectiveness (93–95), it is now recommended in United States clinical practice guidelines (96). VR therapy can improve many PD symptoms, increasing gait speed, reducing dizziness, resolving balance disorders, and decreasing the frequency of falls (97–99). VR can also improve fatigue and enhance activities of daily living in patients with PD (100). VR works on the vestibular system through repetition of specific physical exercises that activate central neuroplastic mechanisms to achieve adaptive compensation of the impaired functions (101, 102). Synaptic inhibition or membrane hyperpolarization of neurons in the medial vestibular nucleus leads to a sustained increase in intrinsic excitability, a phenomenon referred to as “firing rate potentiation.” This mechanism could potentially be employed in vivo to facilitate behavioral plasticity (103, 104). Besides, balance training increases cortical thickness in visual and vestibular cortical regions, which favors vestibular compensation (105). In summary, a growing body of evidence has confirmed the positive effects of VR in patients with PD experiencing dizziness and balance disturbances, including (1) augmenting vision and proprioception to compensate for the vestibular loss, (2) developing compensatory strategies in situations of imbalance, and (3) developing substitution strategies to assist with gaze stability. Moreover, virtual reality and other technologies will continue to evolve and become more frequently applied in VR therapy, offering promising clinical applications.
3.2 Galvanic vestibular stimulation
The application of GVS for treating PD has attracted considerable attention because of its ease of management, non-intrusive nature, affordability, favorable safety profile, and minimal side effects. It is currently recognized as enhancing PD symptom improvement through vestibular-targeted therapy. Vestibular stimulation has been shown to result in the activation of multiple cortical regions (106), with GVS repeatedly achieving PD symptom amelioration (4, 107). Near-threshold stochastic vestibular stimulation can improve postural control in patients with PD (108, 109). Case reports have also suggested that suprathreshold GVS could improve postural reflexes (110), with repetitive caloric vestibular stimulation resulting in lasting motor and non-motor symptom improvement (111). Both noisy and sinusoidal GVS patterns can aid balance control by increasing the PPN connection amplitude (34).
Galvanic vestibular stimulation has also been used to treat dizziness (112) in patients with bilateral vestibular disorders. Significant neural activity in cortical areas involved in vestibular processing is associated with the severity of dizziness-related disability (113). The underlying mechanism likely involves a central role of the vestibular cortical network. These results were further validated in a functional imaging study (114). Therefore, GVS can not only help improve postural imbalance in PD, but also help reduce non-specific dizziness.
3.3 Repetitive transcranial magnetic stimulation
Repetitive transcranial magnetic stimulation (rTMS) is a non-invasive, painless, and non-destructive extracranial neuromodulation technology that has become the primary adjuvant therapy for PD in clinical practice (115), especially for complications arising from pharmacotherapies. Of note, rTMS has been shown to reduce motor symptoms and improve psychiatric symptoms, including anxiety and depression (116–118). Coincidentally, anxiety, depression, and stress commonly coexist with PD and can exacerbate dizziness symptoms. Psychological factors can amplify perceived dizziness severity and contribute to functional impairment. Therefore, rTMS may be effective in patients with PD who have non-specific dizziness, especially those with comorbid depression.
Moreover, rTMS has also been clinically applied to treat dizziness from other causes. A case study found that symptom severity was reduced by at least 50% after rTMS treatment over 3 months in a patient with chronic dizziness and persistent postconcussive symptoms (119). Eight women with a history of classic mal de débarquement syndrome were administered rTMS for 3 weeks in a prospective, double-blind, placebo-controlled study. They experienced significant improvements in various symptoms, including dizziness, mood disturbances, and anxious behaviors, achieving an approximate ten-point reduction in their Dizziness Handicap Inventory (DHI) scores (120). Therefore, we suggest that for patients with PD experiencing significant dizziness and depressive symptoms may benefit from daily sessions of rTMS lasting half an hour each, administered over a course of 1 to 3 months. This treatment approach could potentially help improve the patients’ symptoms of dizziness. However, the use of rTMS for treating non-specific dizziness in patients with PD has not been extensively studied. It is hoped that future large-scale clinical trials will be designed to confirm its effectiveness in this regard.
3.4 Deep brain stimulation
Deep brain stimulation (DBS) is an effective treatment for advanced PD (121–123). The relationship between DBS and vestibular function has recently attracted the interest of researchers. The cerebellum and vestibule work in tandem to assist in gaze stabilization and orientation for motion perception, thereby maintaining balance, with the cerebellum projecting directly to the ventroposterior and ventrolateral thalamus through the vestibulothalamic pathway (124). A study investigating DBS-induced changes in the thalamic ventral intermediate nucleus confirmed that SVV perception was altered during active electrical stimulation (125). DBS significantly improved vestibular discrimination accuracy and threshold in the rightward direction in patients with PD compared with that in healthy controls (126). Therefore, subthalamic DBS might exert differential effects on the vestibular and visual perception of linear motion in patients with PD. The effects of STN stimulation have also been investigated in a study of medial and caudal DBS electrode contact in five patients with PD (127). The patients described compromised rotational motion perception in the plane of the horizontal semicircular canal; one stated having the feeling of sitting on a swing. The latter form of complex perception impairment might be due to the combined stimulation of fibers from the vertical semicircular canals and otolith-derived signals, representing pitch and fore-aft motion, respectively. These findings provided new insights into the counterintuitive implementation of DBS for treating vertigo and imbalance caused by abnormal motion perception. Collectively, these studies suggested that treatment for enhancing vestibular function might be helpful for such patients.
4 Conclusion
Vestibular dysfunction is prevalent among patients with PD; however, the underlying mechanisms are poorly understood. Targeted treatment of vestibular-related symptoms might alleviate PD symptoms and enhance the QoL of patients.
Two major themes have emerged in the literature. One emphasizes the clinical challenges of vestibular dysfunction in PD, with an urgent need for better characterization of its symptoms. Vestibular dysfunction in PD may be linked to motor symptoms like PI, PS, FoG, abnormal eye movements, as well as non-motor symptoms such as dizziness, sleep disturbances, mood changes, and cognitive impairment. The second emphasizes what vestibular dysfunction reveals about PD regarding disease staging and distribution, and its role as an adjunctive assessment of PD prognosis. Further investigation in this area will have implications beyond the symptoms themselves to broader issues, such as non-specific dizziness in PD, cognitive decline, and developing new treatments for such symptoms in PD and other clinical situations. Future studies could use this as a starting point and focus on assessing whether targeted treatment of the vestibular system can delay PD progression, potentially facilitating the exploration of improved clinical approaches.
Author contributions
MG: Funding acquisition, Methodology, Resources, Writing – original draft. LL: Data curation, Investigation, Methodology, Writing – original draft. LQ: Conceptualization, Data curation, Formal analysis, Writing – review & editing, Supervision. CW: Conceptualization, Investigation, Project administration, Validation, Visualization, Writing – review & editing.
Funding
The author(s) declare financial support was received for the research, authorship, and/or publication of this article. This work was supported by the National Natural Science Foundation of China [No. 82101342, 81971201], the National Science Foundation of Hunan province [No. 2022JJ30833], and the Scientific Research Launch Project for new employees of the Second Xiangya Hospital of Central South University.
Conflict of interest
The authors declare that the research was conducted in the absence of any commercial or financial relationships that could be construed as a potential conflict of interest.
Publisher’s note
All claims expressed in this article are solely those of the authors and do not necessarily represent those of their affiliated organizations, or those of the publisher, the editors and the reviewers. Any product that may be evaluated in this article, or claim that may be made by its manufacturer, is not guaranteed or endorsed by the publisher.
References
1. Kwon, K-Y, Park, S, Lee, M, Ju, H, Im, K, Joo, B-E, et al. Dizziness in patients with early stages of Parkinson's disease: prevalence, clinical characteristics and implications. Geriatr Gerontol Int. (2020) 20:443–7. doi: 10.1111/ggi.13894
2. Venhovens, J, Meulstee, J, Bloem, BR, and Verhagen, WIM. Neurovestibular analysis and falls in Parkinson's disease and atypical parkinsonism. Eur J Neurosci. (2016) 43:1636–46. doi: 10.1111/ejn.13253
3. Bohnen, NI, Roytman, S, Griggs, A, David, SM, Beaulieu, ML, and Müller, MLTM. Decreased vestibular efficacy contributes to abnormal balance in Parkinson's disease. J Neurol Sci. (2022) 440:120357. doi: 10.1016/j.jns.2022.120357
4. Smith, PF. Vestibular functions and Parkinson's disease. Front Neurol. (2018) 9:1085. doi: 10.3389/fneur.2018.01085
5. Seidel, K, Mahlke, J, Siswanto, S, Krüger, R, Heinsen, H, Auburger, G, et al. The brainstem pathologies of Parkinson's disease and dementia with Lewy bodies. Brain Pathology (Zurich, Switzerland). (2015) 25:121–35. doi: 10.1111/bpa.12168
6. Wellings, TP, Brichta, AM, and Lim, R. Altered Neurofilament protein expression in the lateral vestibular nucleus in Parkinson's disease. Exp Brain Res. (2017) 235:3695–708. doi: 10.1007/s00221-017-5092-3
7. Shalash, AS, Hassan, DM, Elrassas, HH, Salama, MM, Mendez-Hernandez, E, Salas-Pacheco, JM, et al. Auditory- and vestibular-evoked potentials correlate with motor and non-motor features of Parkinson's disease. Front Neurol. (2017) 8:55. doi: 10.3389/fneur.2017.00055
8. Hawkins, KE, Chiarovano, E, Paul, SS, MacDougall, HG, and Curthoys, IS. Static and dynamic otolith reflex function in people with Parkinson's disease. Eur Arch Otorrinolaringol. (2021) 278:2057–65. doi: 10.1007/s00405-020-06446-1
9. Chow, MR, Ayiotis, AI, Schoo, DP, Gimmon, Y, Lane, KE, Morris, BJ, et al. Posture, gait, quality of life, and hearing with a vestibular implant. N Engl J Med. (2021) 384:521–32. doi: 10.1056/NEJMoa2020457
10. Cronin, T, Arshad, Q, and Seemungal, BM. Vestibular deficits in neurodegenerative disorders: balance, dizziness, and spatial disorientation. Front Neurol. (2017) 8:538. doi: 10.3389/fneur.2017.00538
11. Reichert, WH, Doolittle, J, and McDowell, FH. Vestibular dysfunction in Parkinson disease. Neurology. (1982) 32:1133–8. doi: 10.1212/WNL.32.10.1133
12. Venhovens, J, Meulstee, J, Bloem, BR, and Verhagen, WIM. Neurovestibular dysfunction and falls in Parkinson's disease and atypical parkinsonism: a prospective 1 year follow-up study. Front Neurol. (2020) 11:580285. doi: 10.3389/fneur.2020.580285
13. Vitale, C, Marcelli, V, Furia, T, Santangelo, G, Cozzolino, A, Longo, K, et al. Vestibular impairment and adaptive postural imbalance in parkinsonian patients with lateral trunk flexion. Mov Disord. (2011) 26:1458–63. doi: 10.1002/mds.23657
14. Di Lazzaro, G, Schirinzi, T, Giambrone, MP, Di Mauro, R, Palmieri, MG, Rocchi, C, et al. Pisa syndrome in Parkinson's disease: evidence for bilateral Vestibulospinal dysfunction. Parkinsons Dis. (2018) 2018:8673486. doi: 10.1155/2018/8673486
15. Scocco, DH, Wagner, JN, Racosta, J, Chade, A, and Gershanik, OS. Subjective visual vertical in Pisa syndrome. Parkinsonism Relat Disord. (2014) 20:878–83. Epub 20140509. doi: 10.1016/j.parkreldis.2014.04.030
16. Gandor, F, Basta, D, Gruber, D, Poewe, W, and Ebersbach, G. Subjective visual vertical in Pd patients with lateral trunk flexion. Parkinson's Disease. (2016) 2016:1–4. doi: 10.1155/2016/7489105
17. Bohnen, NI, Kanel, P, van Emde, BM, Roytman, S, and Kerber, KA. Vestibular sensory conflict during postural control, freezing of gait, and falls in Parkinson's disease. Mov Disord. (2022) 37:2257–62. doi: 10.1002/mds.29189
18. Crouse, JJ, Phillips, JR, Jahanshahi, M, and Moustafa, AA. Postural instability and falls in Parkinson's disease. Rev Neurosci. (2016) 27:549–55. doi: 10.1515/revneuro-2016-0002
19. Kwon, K-Y, Lee, M, Ju, H, and Im, K. Risk factors for falls in patients with De novo Parkinson's disease: a focus on motor and non-motor symptoms. J Mov Disord. (2020) 13:142–5. doi: 10.14802/jmd.20009
20. Young, DE, Wagenaar, RC, Lin, C-C, Chou, Y-H, Davidsdottir, S, Saltzman, E, et al. Visuospatial perception and navigation in Parkinson's disease. Vis Res. (2010) 50:2495–504. doi: 10.1016/j.visres.2010.08.029
21. Kim, SD, Allen, NE, Canning, CG, and Fung, VSC. Postural instability in patients with Parkinson's disease. Epidemiology, pathophysiology and management. CNS Drugs. (2013) 27:97–112. doi: 10.1007/s40263-012-0012-3
22. de Natale, ER, Ginatempo, F, Paulus, KS, Manca, A, Mercante, B, Pes, GM, et al. Paired neurophysiological and clinical study of the brainstem at different stages of Parkinson's disease. Clin Neurophysiol. (2015) 126:1871–8. doi: 10.1016/j.clinph.2014.12.017
23. Park, JH, Kim, MS, and Kang, SY. Initial vestibular function may be associated with future postural instability in Parkinson's disease. J Clin Med. (2022) 11:5608. doi: 10.3390/jcm11195608
24. Bohnen, NI, Kanel, P, Koeppe, RA, Sanchez-Catasus, CA, Frey, KA, Scott, P, et al. Regional cerebral cholinergic nerve terminal integrity and cardinal motor features in Parkinson's disease. Brain Commun. (2021) 3:fcab109. doi: 10.1093/braincomms/fcab109
25. Barmack, NH. Central Vestibular System: Vestibular Nuclei and Posterior Cerebellum. Brain Res Bull. (2003) 60:511–41. doi: 10.1016/s0361-9230(03)00055-8
26. Doherty, KM, Davagnanam, I, Molloy, S, Silveira-Moriyama, L, and Lees, AJ. Pisa syndrome in Parkinson's disease: a Mobile or fixed deformity? J Neurol Neurosurg Psychiatry. (2013) 84:1400–3. doi: 10.1136/jnnp-2012-304700
27. Michele Tinazzi, M, Alfonso Fasano, M, Christian Geroin, P, Francesca Morgante, M, Smania, N, Tamburin, S, et al. Pisa syndrome in Parkinson's disease: An integrated approach from pathophysiology to management. Mov Disord. (2016) 31:1785–95. doi: 10.1002/mds.26829
28. Artusi, CA, Montanaro, E, Tuttobene, S, Romagnolo, A, Zibetti, M, and Lopiano, L. Pisa syndrome in Parkinson's disease is associated with specific cognitive alterations. Front Neurol. (2019) 10:577. doi: 10.3389/fneur.2019.00577
29. Castrioto, A, Piscicelli, C, Pérennou, D, Krack, P, and Debû, B. The pathogenesis of Pisa syndrome in Parkinson's disease. Mov Disord. (2014) 29:1100–7. doi: 10.1002/mds.25925
30. Barone, P, Santangelo, G, Amboni, M, Pellecchia, MT, and Vitale, C. Pisa syndrome in Parkinson's disease and parkinsonism: clinical features, pathophysiology, and treatment. Lancet Neurol. (2016) 15:1063–74. doi: 10.1016/s1474-4422(16)30173-9
31. Lambert, FM, Malinvaud, D, Gratacap, M, Straka, H, and Vidal, PP. Restricted neural plasticity in Vestibulospinal pathways after unilateral Labyrinthectomy as the origin for scoliotic deformations. J Neurosci. (2013) 33:6845–56. doi: 10.1523/JNEUROSCI.4842-12.2013
32. Huh, YE, Hwang, S, Kim, K, Chung, W-H, Youn, J, and Cho, JW. Postural sensory correlates of freezing of gait in Parkinson's disease. Parkinsonism Relat Disord. (2016) 25:72–7. doi: 10.1016/j.parkreldis.2016.02.004
33. Aravamuthan, BR, and Angelaki, DE. Vestibular responses in the macaque Pedunculopontine nucleus and central mesencephalic reticular formation. Neuroscience. (2012) 223:183–99. doi: 10.1016/j.neuroscience.2012.07.054
34. Cai, J, Lee, S, Ba, F, Garg, S, Kim, LJ, Liu, A, et al. Galvanic vestibular stimulation (Gvs) augments deficient Pedunculopontine nucleus (Ppn) connectivity in mild Parkinson's disease: Fmri effects of different stimuli. Front Neurosci. (2018) 12:101. doi: 10.3389/fnins.2018.00101
35. Van Vaerenbergh, J, Vranken, R, and Baro, F. The influence of rotational exercises on freezing in Parkinson's disease. Funct Neurol. (2003) 18:11–6.
36. Yousif, N, Bhatt, H, Bain, PG, Nandi, D, and Seemungal, BM. The effect of Pedunculopontine nucleus deep brain stimulation on postural sway and vestibular perception. Eur J Neurol. (2016) 23:668–70. doi: 10.1111/ene.12947
37. Archibald, NK, Hutton, SB, Clarke, MP, Mosimann, UP, and Burn, DJ. Visual exploration in Parkinson's disease and Parkinson's disease dementia. Brain. (2013) 136:739–50. doi: 10.1093/brain/awt005
38. Guo, L, Normando, EM, Shah, PA, De Groef, L, and Cordeiro, MF. Oculo-visual abnormalities in Parkinson's disease: possible value as biomarkers. Mov Disord. (2018) 33:1390–406. doi: 10.1002/mds.27454
39. Kaźmierczak, H, Gospodarek, T, Harat, M, Pawlak-Osińska, K, and Gospodarek, G. Visual-oculomotor and vestibular-oculomotor abnormalities in Parkinson's disease. Otolaryngol Pol. (2006) 60:21–4.
40. Ba, F, Sang, TT, He, W, Fatehi, J, Mostofi, E, and Zheng, B. Stereopsis and eye movement abnormalities in Parkinson's disease and their clinical implications. Front Aging Neurosci. (2022) 14:783773. doi: 10.3389/fnagi.2022.783773
41. Chambers, JM, and Prescott, TJ. Response times for visually guided saccades in persons with Parkinson's disease: a Meta-analytic review. Neuropsychologia. (2010) 48:887–99. doi: 10.1016/j.neuropsychologia.2009.11.006
42. Waldthaler, J, Stock, L, Student, J, Sommerkorn, J, Dowiasch, S, and Timmermann, L. Antisaccades in Parkinson's disease: a Meta-analysis. Neuropsychol Rev. (2021) 31:628–42. doi: 10.1007/s11065-021-09489-1
43. Zhou, M-X, Wang, Q, Lin, Y, Xu, Q, Wu, L, Chen, Y-J, et al. Oculomotor impairments in De novo Parkinson's disease. Front Aging Neurosci. (2022) 14:985679. doi: 10.3389/fnagi.2022.985679
44. Meldrum, D, and Jahn, K. Gaze stabilisation exercises in vestibular rehabilitation: review of the evidence and recent clinical advances. J Neurol. (2019) 266:11–8. doi: 10.1007/s00415-019-09459-x
45. Lasisi, AO, and Gureje, O. Disability and quality of life among community elderly with dizziness: report from the Ibadan study of ageing. J Laryngol Otol. (2010) 124:957–62. doi: 10.1017/S0022215110000538
46. Schrag, A, Horsfall, L, Walters, K, Noyce, A, and Petersen, I. Prediagnostic presentations of Parkinson's disease in primary care: a case-control study. Lancet Neurol. (2015) 14:57–64. doi: 10.1016/S1474-4422(14)70287-X
47. Barone, P, Antonini, A, Colosimo, C, Marconi, R, Morgante, L, Avarello, TP, et al. The Priamo study: a multicenter assessment of nonmotor symptoms and their impact on quality of life in Parkinson's disease. Mov Disord. (2009) 24:1641–9. doi: 10.1002/mds.22643
48. Yilmaz, LÇ, Tunç, T, and İnan, LE. The causes of dizziness in Parkinson’s disease. Int J Clin Med. (2014) 5:667–73. doi: 10.4236/ijcm.2014.512092
49. Palma, J-A, Gomez-Esteban, JC, Norcliffe-Kaufmann, L, Martinez, J, Tijero, B, Berganzo, K, et al. Orthostatic hypotension in Parkinson disease: how much you fall or how low you go? Mov Disord. (2015) 30:639–45. doi: 10.1002/mds.26079
50. Velseboer, DC, de Haan, RJ, Wieling, W, Goldstein, DS, and de Bie, RMA. Prevalence of orthostatic hypotension in Parkinson's disease: a systematic review and Meta-analysis. Parkinsonism Relat Disord. (2011) 17:724–9. doi: 10.1016/j.parkreldis.2011.04.016
51. Park, J-H, and Kang, SY. Dizziness in Parkinson's disease patients is associated with vestibular function. Sci Rep. (2021) 11:18976. doi: 10.1038/s41598-021-98540-5
52. Bassetto, JM, Zeigelboim, BS, Jurkiewicz, AL, and Klagenberg, KF. Neurotological findings in patients with Parkinson's disease. Braz J Otorhinolaryngol. (2008) 74:350–5. doi: 10.1016/S1808-8694(15)30567-X
53. Martin, T, Moussay, S, Bulla, I, Bulla, J, Toupet, M, Etard, O, et al. Exploration of circadian rhythms in patients with bilateral vestibular loss. PLoS One. (2016) 11:e0155067. doi: 10.1371/journal.pone.0155067
54. Albathi, M, and Agrawal, Y. Vestibular Vertigo is associated with abnormal sleep duration. J Vestib Res. (2017) 27:127–35. doi: 10.3233/VES-170617
55. Ampar, N, Mehta, A, Mahale, RR, Javali, M, Pradeep, R, Acharya, P, et al. Electrophysiological evaluation of Audiovestibular pathway dysfunction in Parkinson's disease and its correlates: a case control study. Ann Indian Acad Neurol. (2021) 24:531–5. doi: 10.4103/aian.AIAN_1011_20
56. Goothy, SSK, and McKeown, J. Modulation of sleep using electrical vestibular nerve stimulation prior to sleep onset: a pilot study. J Basic Clin Physiol Pharmacol. (2020) 32:19–23. doi: 10.1515/jbcpp-2020-0019
57. Martin, T, Mauvieux, B, Bulla, J, Quarck, G, Davenne, D, Denise, P, et al. Vestibular loss disrupts daily rhythm in rats. J Appl Physiol (1985). (2015) 118:310–8. doi: 10.1152/japplphysiol.00811.2014
58. Dieterich, M, and Brandt, T. The bilateral central vestibular system: its pathways, functions, and disorders. Ann N Y Acad Sci. (2015) 1343:10–26. doi: 10.1111/nyas.12585
59. Horowitz, SS, Blanchard, JH, and Morin, LP. Intergeniculate leaflet and ventral lateral geniculate nucleus afferent connections: an anatomical substrate for functional input from the Vestibulo-Visuomotor system. J Comp Neurol. (2004) 474:227–45. doi: 10.1002/cne.20125
60. Shi, X, Wei, H, Chen, Z, Wang, J, Qu, W, Huang, Z, et al. Whole-brain monosynaptic inputs and outputs of glutamatergic neurons of the vestibular nuclei complex in mice. Hear Res. (2021) 401:108159. doi: 10.1016/j.heares.2020.108159
61. Pan, L, Qi, R, Wang, J, Zhou, W, Liu, J, and Cai, Y. Evidence for a role of orexin/Hypocretin system in vestibular lesion-induced locomotor abnormalities in rats. Front Neurosci. (2016) 10:355. doi: 10.3389/fnins.2016.00355
62. Ciriello, J, and Caverson, MM. Hypothalamic orexin-a (Hypocretin-1) neuronal projections to the vestibular complex and cerebellum in the rat. Brain Res. (2014) 1579:20–34. doi: 10.1016/j.brainres.2014.07.008
63. Weintraub, D, Aarsland, D, Chaudhuri, KR, Dobkin, RD, Leentjens, AF, Rodriguez-Violante, M, et al. The neuropsychiatry of Parkinson's disease: advances and challenges. Lancet Neurol. (2022) 21:89–102. doi: 10.1016/S1474-4422(21)00330-6
64. Brandt, T, and Dieterich, M. 'Excess Anxiety' and 'Less Anxiety': both depend on vestibular function. Curr Opin Neurol. (2020) 33:136–41. doi: 10.1097/wco.0000000000000771
65. Wang, Y, Chen, Z-P, Yang, Z-Q, Zhang, X-Y, Li, J-M, Wang, J-J, et al. Corticotropin-releasing factor depolarizes rat lateral vestibular nuclear neurons through activation of Crf receptors 1 and 2. Neuropeptides. (2019) 76:101934. doi: 10.1016/j.npep.2019.05.005
66. Preuss, N, Hasler, G, and Mast, FW. Caloric vestibular stimulation modulates affective control and mood. Brain Stimul. (2014) 7:133–40. doi: 10.1016/j.brs.2013.09.003
67. McKay, R, Tamagni, C, Palla, A, Krummenacher, P, Hegemann, SCA, Straumann, D, et al. Vestibular stimulation attenuates unrealistic optimism. Cortex. (2013) 49:2272–5. doi: 10.1016/j.cortex.2013.04.005
68. Hartikainen, KM. Emotion-attention interaction in the right hemisphere. Brain Sci. (2021) 11:1006. doi: 10.3390/brainsci11081006
69. Gainotti, G. Hemispheric asymmetries for emotions in non-human Primates: a systematic review. Neurosci Biobehav Rev. (2022) 141:104830. doi: 10.1016/j.neubiorev.2022.104830
70. Arshad, Q. Dynamic interhemispheric competition and Vestibulo-cortical control in humans; a theoretical proposition. Neuroscience. (2017) 353:26–41. doi: 10.1016/j.neuroscience.2017.04.013
71. Bednarczuk, NF, Casanovas Ortega, M, Fluri, A-S, and Arshad, Q. Vestibulo-cortical hemispheric dominance: the link between anxiety and the vestibular system? Eur J Neurosci. (2018) 47:1517–24. doi: 10.1111/ejn.13948
72. Riccelli, R, Indovina, I, Staab, JP, Nigro, S, Augimeri, A, Lacquaniti, F, et al. Neuroticism modulates brain Visuo-vestibular and anxiety systems during a virtual rollercoaster task. Hum Brain Mapp. (2017) 38:715–26. doi: 10.1002/hbm.23411
73. Smith, PF, and Zheng, Y. From ear to uncertainty: vestibular contributions to cognitive function. Front Integr Neurosci. (2013) 7:84. doi: 10.3389/fnint.2013.00084
74. Chari, DA, Madhani, A, Sharon, JD, and Lewis, RF. Evidence for cognitive impairment in patients with vestibular disorders. J Neurol. (2022) 269:5831–42. doi: 10.1007/s00415-022-11289-3
75. Ayar, DA, Kumral, E, and Celebisoy, N. Cognitive functions in acute unilateral vestibular loss. J Neurol. (2020) 267:153–9. doi: 10.1007/s00415-020-09829-w
76. Wylie, KP, Kluger, BM, Medina, LD, Holden, SK, Kronberg, E, Tregellas, JR, et al. Hippocampal, basal ganglia and olfactory connectivity contribute to cognitive impairments in Parkinson's disease. Eur J Neurosci. (2023) 57:511–26. doi: 10.1111/ejn.15899
77. Crucian, GP, Barrett, AM, Schwartz, RL, Bowers, D, Triggs, WJ, Friedman, W, et al. Cognitive and Vestibulo-proprioceptive components of spatial ability in Parkinson's disease. Neuropsychologia. (2000) 38:757–67. doi: 10.1016/S0028-3932(99)00143-8
78. Lichter, DG, Benedict, RHB, and Hershey, LA. Importance of balance-gait disorder as a risk factor for cognitive impairment, dementia and related non-motor symptoms in Parkinson's disease. J Parkinsons Dis. (2018) 8:539–52. doi: 10.3233/JPD-181375
79. Sugaya, N, Arai, M, and Goto, F. Changes in cognitive function in patients with intractable dizziness following vestibular rehabilitation. Sci Rep. (2018) 8:9984. doi: 10.1038/s41598-018-28350-9
80. Smith, PF. The vestibular system and cognition. Curr Opin Neurol. (2017) 30:84–9. doi: 10.1097/WCO.0000000000000403
81. Brandt, T, Schautzer, F, Hamilton, DA, Brüning, R, Markowitsch, HJ, Kalla, R, et al. Vestibular loss causes hippocampal atrophy and impaired spatial memory in humans. Brain. (2005) 128:2732–41. doi: 10.1093/brain/awh617
82. Besnard, S, Machado, ML, Vignaux, G, Boulouard, M, Coquerel, A, Bouet, V, et al. Influence of vestibular input on spatial and nonspatial memory and on hippocampal Nmda receptors. Hippocampus. (2012) 22:814–26. doi: 10.1002/hipo.20942
83. Vitale, C, Marcelli, V, Abate, T, Pianese, A, Allocca, R, Moccia, M, et al. Speech discrimination is impaired in parkinsonian patients: expanding the Audiologic findings of Parkinson's disease. Parkinsonism Relat Disord. (2016) 22:S138–43. doi: 10.1016/j.parkreldis.2015.09.040
84. Vitale, C, Marcelli, V, Allocca, R, Santangelo, G, Riccardi, P, Erro, R, et al. Hearing impairment in Parkinson's disease: expanding the nonmotor phenotype. Mov Disord. (2012) 27:1530–5. doi: 10.1002/mds.25149
85. Iannella, G, Magliulo, G, Lechien, JR, Maniaci, A, Perrone, T, Frasconi, PC, et al. Impact of Covid-19 pandemic on the incidence of otitis media with effusion in adults and children: a multicenter study. Eur Arch Otorrinolaringol. (2022) 279:2383–9. doi: 10.1007/s00405-021-06958-4
86. Pötter-Nerger, M, Reich, MM, Colebatch, JG, Deuschl, G, and Volkmann, J. Differential effect of Dopa and subthalamic stimulation on vestibular activity in Parkinson's disease. Mov Disord. (2012) 27:1268–75. doi: 10.1002/mds.25061
87. Tighilet, B, Bourdet, A, Péricat, D, Timon-David, E, Rastoldo, G, and Chabbert, C. Sk channels modulation accelerates equilibrium recovery in unilateral vestibular Neurectomized rats. Pharmaceuticals (Basel). (2021) 14:1226. doi: 10.3390/ph14121226
88. Putcha, D, Ross, RS, Rosen, ML, Norton, DJ, Cronin-Golomb, A, Somers, DC, et al. Functional correlates of optic flow motion processing in Parkinson's disease. Front Integr Neurosci. (2014) 8:57. doi: 10.3389/fnint.2014.00057
89. Cardin, V, and Smith, AT. Sensitivity of human visual and vestibular cortical regions to Egomotion-compatible visual stimulation. Cereb Cortex. (2010) 20:1964–73. Epub 20091224. doi: 10.1093/cercor/bhp268
90. Messa, LV, Ginanneschi, F, Momi, D, Monti, L, Battisti, C, Cioncoloni, D, et al. Functional and brain activation changes following specialized upper-limb exercise in Parkinson's disease. Front Hum Neurosci. (2019) 13:350. doi: 10.3389/fnhum.2019.00350
91. Lai, H, Tsumori, T, Shiroyama, T, Yokota, S, Nakano, K, and Yasui, Y. Morphological evidence for a Vestibulo-Thalamo-striatal pathway via the Parafascicular nucleus in the rat. Brain Res. (2000) 872:208–14. doi: 10.1016/S0006-8993(00)02457-4
92. Kim, N, Choi, MA, Koo, H, Park, BR, Han, SW, Cheong, C, et al. Activation of the thalamic Parafascicular nucleus by electrical stimulation of the peripheral vestibular nerve in rats. Exp Brain Res. (2017) 235:1617–25. doi: 10.1007/s00221-016-4864-5
93. Tramontano, M, Russo, V, Spitoni, GF, Ciancarelli, I, Paolucci, S, Manzari, L, et al. Efficacy of vestibular rehabilitation in patients with neurologic disorders: a systematic review. Arch Phys Med Rehabil. (2021) 102:1379–89. doi: 10.1016/j.apmr.2020.11.017
94. Dunlap, PM, Holmberg, JM, and Whitney, SL. Vestibular rehabilitation: advances in peripheral and central vestibular disorders. Curr Opin Neurol. (2019) 32:137–44. doi: 10.1097/WCO.0000000000000632
95. Yardley, L, Barker, F, Muller, I, Turner, D, Kirby, S, Mullee, M, et al. Clinical and cost effectiveness of booklet based vestibular rehabilitation for chronic dizziness in primary care: single blind, parallel group, pragmatic, randomised controlled trial. BMJ. (2012) 344:e2237. doi: 10.1136/bmj.e2237
96. Hall, CD, Herdman, SJ, Whitney, SL, Anson, ER, Carender, WJ, Hoppes, CW, et al. Vestibular rehabilitation for peripheral vestibular hypofunction: an updated clinical practice guideline from the academy of neurologic physical therapy of the American Physical Therapy Association. J Neurol Phys Ther. (2022) 46:118–77. doi: 10.1097/NPT.0000000000000382
97. Hirsch, MA, Toole, T, Maitland, CG, and Rider, RA. The effects of balance training and high-intensity resistance training on persons with idiopathic Parkinson's disease. Arch Phys Med Rehabil. (2003) 84:1109–17. doi: 10.1016/S0003-9993(03)00046-7
98. Rossi-Izquierdo, M, Soto-Varela, A, Santos-Pérez, S, Sesar-Ignacio, A, Labella-Caballero, T, Rossi-Izquierdo, M, et al. Vestibular rehabilitation with computerised dynamic Posturography in patients with Parkinson's disease: improving balance impairment. Disabil Rehabil. (2009) 31:1907–16. doi: 10.1080/09638280902846384
99. Sulway, S, and Whitney, SL. Advances in vestibular rehabilitation. Adv Otorhinolaryngol. (2019) 82:164–9. doi: 10.1159/000490285
100. Abasi, A, Raji, P, Friedman, JH, Hadian, M-R, Hoseinabadi, R, Abbasi, S, et al. Effects of vestibular rehabilitation on fatigue and activities of daily living in people with Parkinson's disease: a pilot randomized controlled trial study. Parkinson's Disease. (2020) 2020:8624986. doi: 10.1155/2020/8624986
101. Lacour, M, Helmchen, C, and Vidal, PP. Vestibular compensation: the neuro-Otologist's best friend. J Neurol. (2016) 263:S54–64. doi: 10.1007/s00415-015-7903-4
102. Zhang, S, Liu, D, Tian, E, Wang, J, Guo, Z, and Kong, W. Central vestibular dysfunction: Don't forget vestibular rehabilitation. Expert Rev Neurother. (2022) 22:669–80. doi: 10.1080/14737175.2022.2106129
103. Nelson, AB, Krispel, CM, Sekirnjak, C, and du Lac, S. Long-lasting increases in intrinsic excitability triggered by inhibition. Neuron. (2003) 40:609–20. doi: 10.1016/s0896-6273(03)00641-x
104. Nelson, AB, Faulstich, M, Moghadam, S, Onori, K, Meredith, A, and du Lac, S. Bk channels are required for multisensory plasticity in the oculomotor system. Neuron. (2017) 93:211–20. doi: 10.1016/j.neuron.2016.11.019
105. Rogge, AK, Röder, B, Zech, A, and Hötting, K. Exercise-induced neuroplasticity: balance training increases cortical thickness in visual and vestibular cortical regions. NeuroImage. (2018) 179:471–9. doi: 10.1016/j.neuroimage.2018.06.065
106. Lopez, C, Blanke, O, and Mast, FW. The human vestibular cortex revealed by coordinate-based activation likelihood estimation Meta-analysis. Neuroscience. (2012) 212:159–79. doi: 10.1016/j.neuroscience.2012.03.028
107. Kumar Goothy, SS, Gawarikar, S, Choudhary, A, Gajanan Govind, P, Purohit, M, Pathak, A, et al. Effectiveness of electrical vestibular nerve stimulation as adjunctive therapy to improve the cognitive functions in patients with Parkinson's disease. J Basic Clin Physiol Pharmacol. (2022) 34:77–82. doi: 10.1515/jbcpp-2022-0066
108. Samoudi, G, Jivegård, M, Mulavara, AP, and Bergquist, F. Effects of stochastic vestibular galvanic stimulation and Ldopa on balance and motor symptoms in patients with Parkinson's disease. Brain Stimul. (2015) 8:474–80. doi: 10.1016/j.brs.2014.11.019
109. Wuehr, M, Schmidmeier, F, Katzdobler, S, Fietzek, UM, Levin, J, and Zwergal, A. Effects of low-intensity vestibular noise stimulation on postural instability in patients with Parkinson's disease. J Parkinsons Dis. (2022) 12:1611–8. doi: 10.3233/JPD-213127
110. Kataoka, H, Okada, Y, Kiriyama, T, Kita, Y, Nakamura, J, Morioka, S, et al. Can postural instability respond to galvanic vestibular stimulation in patients with Parkinson's disease? J Mov Disord. (2016) 9:40–3. doi: 10.14802/jmd.15030
111. Wilkinson, D, Podlewska, A, and Sakel, M. A durable gain in motor and non-motor symptoms of Parkinson's disease following repeated caloric vestibular stimulation: a single-case study. NeuroRehabilitation. (2016) 38:179–82. doi: 10.3233/NRE-161308
112. Woll, J, Sprenger, A, and Helmchen, C. Postural control during galvanic vestibular stimulation in patients with persistent perceptual-postural dizziness. J Neurol. (2019) 266:1236–49. doi: 10.1007/s00415-019-09255-7
113. Helmchen, C, Rother, M, Spliethoff, P, and Sprenger, A. Increased brain responsivity to galvanic vestibular stimulation in bilateral vestibular failure. Neuroimage Clin. (2019) 24:101942. doi: 10.1016/j.nicl.2019.101942
114. Helmchen, C, Machner, B, Rother, M, Spliethoff, P, Göttlich, M, and Sprenger, A. Effects of galvanic vestibular stimulation on resting state brain activity in patients with bilateral Vestibulopathy. Hum Brain Mapp. (2020) 41:2527–47. doi: 10.1002/hbm.24963
115. Lefaucheur, J-P, Aleman, A, Baeken, C, Benninger, DH, Brunelin, J, Di Lazzaro, V, et al. Evidence-based guidelines on the therapeutic use of repetitive transcranial magnetic stimulation (Rtms): an update (2014-2018). Clin Neurophysiol. (2020) 131:474–528. doi: 10.1016/j.clinph.2019.11.002
116. Chou, YH, Hickey, PT, Sundman, M, Song, AW, and Chen, NK. Effects of repetitive transcranial magnetic stimulation on motor symptoms in Parkinson disease: a systematic review and Meta-analysis. JAMA Neurol. (2015) 72:432–40. doi: 10.1001/jamaneurol.2014.4380
117. Chung, CL, and Mak, MKY. Effect of repetitive transcranial magnetic stimulation on physical function and motor signs in Parkinson's disease: a systematic review and Meta-analysis. Brain Stimul. (2016) 9:475–87. doi: 10.1016/j.brs.2016.03.017
118. Xie, C-L, Chen, J, Wang, X-D, Pan, J-L, Zhou, Y, Lin, S-Y, et al. Repetitive transcranial magnetic stimulation (Rtms) for the treatment of depression in Parkinson disease: a Meta-analysis of randomized controlled clinical trials. Neurol Sci. (2015) 36:1751–61. doi: 10.1007/s10072-015-2345-4
119. Paxman, E, Stilling, J, Mercier, L, and Debert, CT. Repetitive transcranial magnetic stimulation (Rtms) as a treatment for chronic dizziness following mild traumatic brain injury. BMJ Case Rep. (2018) 2018:bcr2018226698. doi: 10.1136/bcr-2018-226698
120. Cha, Y-H, Deblieck, C, and Wu, AD. Double-blind sham-controlled crossover trial of repetitive transcranial magnetic stimulation for mal De Debarquement syndrome. Otol Neurotol. (2016) 37:805–12. doi: 10.1097/MAO.0000000000001045
121. Pinkhardt, EH, Jürgens, R, Lulé, D, Heimrath, J, Ludolph, AC, Becker, W, et al. Eye movement impairments in Parkinson's disease: possible role of Extradopaminergic mechanisms. BMC Neurol. (2012) 12:5. doi: 10.1186/1471-2377-12-5
122. Limousin, P, Krack, P, Pollak, P, Benazzouz, A, Ardouin, C, Hoffmann, D, et al. Electrical stimulation of the subthalamic nucleus in advanced Parkinson's disease. N Engl J Med. (1998) 339:1105–11. doi: 10.1056/NEJM199810153391603
123. Benabid, AL, Chabardes, S, Mitrofanis, J, and Pollak, P. Deep brain stimulation of the subthalamic nucleus for the treatment of Parkinson's disease. Lancet Neurol. (2009) 8:67–81. doi: 10.1016/S1474-4422(08)70291-6
124. Meng, H, and Angelaki, DE. Responses of ventral posterior thalamus neurons to three-dimensional vestibular and optic flow stimulation. J Neurophysiol. (2010) 103:817–26. doi: 10.1152/jn.00729.2009
125. Baier, B, Vogt, T, Rohde, F, Cuvenhaus, H, Conrad, J, and Dieterich, M. Deep brain stimulation of the nucleus Ventralis intermedius: a thalamic site of Graviceptive modulation. Brain Struct Funct. (2017) 222:645–50. doi: 10.1007/s00429-015-1157-x
126. Beylergil, SB, Noecker, AM, Petersen, M, Gupta, P, Ozinga, S, Walker, MF, et al. Subthalamic deep brain stimulation affects heading perception in Parkinson's disease. J Neurol. (2022) 269:253–68. doi: 10.1007/s00415-021-10616-4
Keywords: dizziness, neuropathology, Parkinson’s disease, quality of life, vestibular system
Citation: Gui M, Lv L, Qin L and Wang C (2024) Vestibular dysfunction in Parkinson’s disease: a neglected topic. Front. Neurol. 15:1398764. doi: 10.3389/fneur.2024.1398764
Edited by:
Aasef G. Shaikh, Case Western Reserve University, United StatesReviewed by:
Salvatore Cocuzza, University of Catania, ItalyArun Singh, University of South Dakota, United States
Copyright © 2024 Gui, Lv, Qin and Wang. This is an open-access article distributed under the terms of the Creative Commons Attribution License (CC BY). The use, distribution or reproduction in other forums is permitted, provided the original author(s) and the copyright owner(s) are credited and that the original publication in this journal is cited, in accordance with accepted academic practice. No use, distribution or reproduction is permitted which does not comply with these terms.
*Correspondence: Lixia Qin, cWlubGl4aWExMDI3QGNzdS5lZHUuY24=; Chunyu Wang, d2FuZ2NodW55dUBjc3UuZWR1LmNu