- 1Department of Neurology, Monash Health, Melbourne, VIC, Australia
- 2NEUR Research Group, Center for Neurosciences, Vrije Universiteit Brussel, Brussels, Belgium
- 3Faculty of Medicine, Dentistry and Health Sciences, The University of Melbourne, Melbourne, VIC, Australia
- 4Department of Medicine, School of Clinical Sciences at Monash Health, Monash University, Melbourne, VIC, Australia
Background: The search for neuroprotective treatments for Parkinson’s disease (PD) still relies largely on motor disability scales. A limitation of these tools is the strong influence of symptomatic dopaminergic treatment effects. Drawing on a wealth of published information, we conducted a systematic review and meta-analysis of motor progression in PD and its relationships with dopaminergic therapy.
Methods: We searched Medline, Embase, and Central to identify 84 publications with adequate serial motor scores to calculate progression, expressed as an increase in the percentage of maximum disability.
Results: A random-effects model showed motor progression at 2.0% p.a. (95% CI 1.7–2.4%). There were no significant differences by baseline age, sample size, or observation period. However, untreated patients, in 8 publications, progressed at 4.5% p.a. compared to 1.6% p.a. in 76 studies containing individuals on dopaminergic drugs (p = 0.0004, q = 0.003). This was supported by research on phenoconversion in prodromal PD, where motor progression exceeded 5% p.a. in the 2 years before diagnosis. Starting levodopa improved pre-treatment disability by 40.3 ± 15.2%. Practically defined off state measurements increase faster than on scores by a modest degree (p = 0.05).
Conclusion: This survey suggests that accurate long-term measurements of motor progression to assess disease-modifying therapies can be conducted despite the sequential commencement of dopaminergic drugs and sample attrition over time. While study designs involving prodromal or untreated PD avoid confounding effects of symptomatic treatment, different assumptions about motor progression may be needed. A defined off state with the levodopa test dose method maximizes information about the medication cycle once dopaminergic therapy has begun.
1 Introduction
While there are effective symptomatic drug treatments for Parkinson’s disease (PD), there is no proven way to alter the slow progression of its underlying pathology. A 2022 survey identified more than 150 clinical trials of neuroprotective agents (1). None have convincingly shown a disease-modifying effect. Objective motor assessment has been the mainstay of clinical trial methodology for PD. It is used to evaluate both symptomatic treatments—pharmacological or non-pharmacological—as well as potential disease-modifying drugs.
Debate about the shortcomings of disease-modifying research in PD has focused on two main areas—drug development, particularly therapeutic target and experimental agent choices, and clinical trial design (2). Trial design issues include population selection (manifest early PD as opposed to possible latent PD, identified by non-motor clinical features or genetic risk) and outcome measurements (3). Measuring motor disability means making allowance for symptomatic effects, both for experimental agents that might have symptomatic and protective benefits and for the ways in which standard dopaminergic treatments influence motor scoring. Inconclusive results of large trials such as DATATOP (4) and ADAGIO (5) may be traced to assumptions about symptomatic treatment effects. The tradition of reliance on objective motor scales has been questioned. Possible alternatives are composite endpoints by weighting scores from multiple scales, the use of wearable sensors to collect electronic movement data (6), and the identification of dependable clinical milestones of disease progression (7). Laboratory biomarkers as symptom-unrelated outcome measures would be ideal (8). None as yet are sufficiently sensitive to disease progression.
The Braak model of progression of alpha-synuclein pathology explains why olfactory or sleep-related non-motor symptoms can be premonitory PD features, and why cognitive and neuropsychiatric non-motor symptoms attend its advanced stages (9). However, at its core, PD is a motor disorder, and quantitative histological studies show why this is true. Lewy pathology, containing aggregated alpha-synuclein protein, denotes populations of neurons that are selectively vulnerable in PD. These are anatomically and neurochemically diverse, though there are certain morphological similarities. The pars compacta region of the substantia nigra is the most consistently, most severely affected region. Approximately 50% of these neurons have been lost at the time of first motor symptoms, rising to 90% by the end of the disease course (a small subpopulation always survives) (10). Many other regions are affected and may contribute to non-motor symptoms, but cell loss in relation to Lewy pathology is generally less (11). The UPDRS motor scale shows a linear correlation with neuronal density in the substantia nigra (12). A sufficiently sensitive objective clinical motor scale should still be the best way to prove that an investigational agent modifies the pathological progression of PD.
The influence of symptomatic treatments on the measurement of motor disability needs to be addressed in clinical trial design. One approach has been to try to exploit the pre-treatment phase, allowing pure active and placebo comparative arms. Participants are temporarily denied the benefit of dopaminergic drugs, restricting such trials to short time scales. An alternative is long-term assessments guided by a good understanding of the effects of symptomatic treatments on the measurement scale.
The purpose of this article is to examine rates of progression in the various phases of PD and in relation to the commencement and continuation of dopaminergic therapy. There is a wealth of published data that can inform this inquiry. In a systematic review and meta-analysis of serial motor scoring in PD, we will focus on four main areas:
1. Rate of motor progression, both before and during chronic pharmacological treatment;
2. Influence of timing of dopaminergic medication doses on measurements of motor progression, particularly the use of defined off and on states;
3. Short- and medium-term effects of commencement of dopaminergic treatment on motor disability scores;
4. Appearance and progression of motor disability in the very earliest stage of PD.
The last two points are highly relevant to future trials involving “preclinical” or “prodromal” PD—individuals with strong risk indicators (clinical, genetic, and neuroimaging) for the development of PD yet no sign of motor manifestation. Neuroprotective agents may have their strongest effects before the neurodegeneration of PD is far advanced, prior to detectable motor signs (3). Actual proof of disease modification, though, will probably rely on the measurement of motor disability when symptomatic treatment effects are present.
2 Methods
2.1 Study design
The study, a systematic review and meta-analysis, was registered with PROSPERO (CRD42023410326). The Preferred Reporting Items for Systematic Reviews and Meta-Analyses (PRISMA) guidelines were followed (13).
2.2 Eligibility criteria
Articles were included if they reported on at least 20 individuals diagnosed with or at risk for idiopathic PD who had serial (two or more) measurements of motor function over a minimum interval of 6 months. These motor assessments had to be performed using a recognized objective PD motor disability scale with a range of 20 or more points (excluding less sensitive instruments such as the 5-point Hoehn and the Yahr scale). Separate reporting of motor scores was required. An article was ineligible if there was no English full-text available, or if only an abstract was published.
All study designs yielding longitudinal data, prospective or retrospective, were considered. This included cohort and population-based studies. For clinical trial research, data from control arm patients receiving standard-of-care pharmacological treatment, levodopa monotherapy, or no medication were included. Excluded were data from patients in a clinical trial or comparison who received a trial pharmacological agent, or a non-pharmacological intervention. The purpose of these restrictions was to focus as much as possible on the natural history of PD before and during normal pharmacological therapy. While we accepted that placebo effects would be present in clinical trial results, we excluded studies where this was excessive, as manifested by motor disability scores that were stable or improved during a period of observation (any calculated progression rate ≤ 0%). Studies that subdivided participants to examine certain characteristics, such as genetic factors, were included as long as the whole sample was representative of PD. In cases where multiple studies reported overlapping observations on the same cohort, we selected the study with the longest follow-up and/or largest sample size, which was generally the most recent study.
2.3 Data sources
On 15 March 2023, we conducted literature searches in Medline (Ovid interface), Embase (Ovid interface), Cochrane Central Register of Clinical Trials, and clinicaltrials.gov. See Appendix 1 for the search strategy and Figure 1 for the PRISMA flowchart.
2.4 Article selection and classification
Two authors (AP and PK) independently performed title/abstract and full-text screening to determine eligible papers and categorize key study attributes. In cases of missing information, the nature and cause (if specified) of omissions were registered. Disagreements were discussed with the aim of consensus, and the opinion of the third author (CD) was sought if required.
2.5 Data extraction and processing
Data were extracted from tables or graphical plots to obtain the maximum number of time points of motor disability for each paper. In clinical trials with a washout phase at the end of the study period, the washout was excluded. If insufficient data were provided to determine spread—standard deviation (SD) or standard error of the mean—authors were contacted by email to request this information. Where values of two subgroups of PD patients were provided separately (e.g., carriers and non-carriers of a specific gene), the weighted mean was used to calculate the progression rate for the whole sample. One other paper reported on two separate cohorts (14), which both were used.
Where some or all patients received dopaminergic treatment, the relationship of motor scoring with the timing of medication was noted, usually specified as on or off states. If a study was silent on this point, it was assumed that assessments were performed in prevailing motor states not temporally linked to the medication cycle. The use of practically defined off states with levodopa test doses was also recorded. All specified on and off scoring was used to derive separate progression rates for these motor states. Where a study provided both on and off scores, an average of on and off was taken to calculate overall progression. This was done to avoid over-weighting a cohort by double representation, or one type of motor assessment at the expense of the other.
Response to commencement of levodopa therapy was ascertained from studies that met the following conditions: (i) pre-treatment motor disability recorded; (ii) levodopa started at a single time point; (iii) ≥3 motor measurements conducted during the first 2 years of treatment. The initial treatment response was calculated by expressing the amount of motor improvement at the time point of the lowest recorded disability score after starting treatment as a percentage of pre-treatment disability. This nadir of disability was then used as the first point for the calculation of motor progression in those articles.
Three additional classifications of articles were made:
• Sequential treatment commencement > 25%: a study in which dopaminergic treatment was not commenced at a single time point but it was estimated that more than 25% of participants had initiated treatment during a study period.
• Fixed sample size: a study with the same number of subjects at baseline and final observation point (retrospective cohort analyses).
• Sample attrition: a study where the number at baseline exceeded the final observation point because of loss to follow-up (clinical trial and prospective cohort research).
2.6 Statistical methods
Statistical analyses were performed with R, version 4.3.1. The raw data were converted to mean ± SD as this was the effect size measure most commonly reported. The median and interquartile range (IQR) were changed to mean and SD using the methods of Luo and Wan (15, 16). SD was obtained from the 95% confidence interval (CI) by dividing it by 3.92 before multiplying it by the square root of the sample size (17). For sample attrition studies that did not provide a patient number for every time point, we assumed a constant attrition rate based on sample size at the first and last time points. If the SD of the rate of progression was not given, it was imputed from the first and last measurements, assuming a conservative correlation coefficient of 0.7 (17, 18). Descriptive statistics are presented as mean ± SD or median ± IQR.
The progression rate in individual articles was determined by linear regression. To standardize the various motor scoring systems, the results are presented as percentage change per annum (p.a.) of the maximum disability score of the relevant scale (19). All studies with a calculable rate of PD motor progression were analyzed according to study attributes using the Wilcoxon rank-sum test with correction for multiple comparisons following the Benjamini-Hochberg principle, with a p-value adjusted for false discovery rate (q-value) of ≤0.05 considered significant. As an additional check for bias from sample size, study duration, and number of time points, we calculated annual motor progression weighted for each of these factors.
All studies yielding statistics on both the rate of motor progression and its standard error were pooled in a meta-analysis to synthesize evidence and assess for heterogeneity. We employed a DerSimonian and Laird random-effects meta-analysis model, using the meta package in R (20). Heterogeneity between studies was assessed with the I2 metric. Study characteristics and bias assessment were explored as potential sources for heterogeneity using multivariate linear models (rma.mv function, metafor package) (21).
Studies with mean and SD statistics on serial time points contributed to a data simulation. We used the truncnorm package (22), with a truncated normal distribution model (range 0 to 100). For studies providing both on and off scores, the off measurements were simulated.
2.7 Study quality assessment
Quality was independently assessed by two authors (AP and PK), using the Critical Appraisal and Skills Programme (CASP) quality assessment tool (23). We adapted the cohort study version of the CASP tool and examined all studies for their longitudinal cohort properties, irrespective of the research question or method.
Studies were critically assessed on five points: 1. study population; 2. selection bias; 3. ascertainment of diagnosis; 4. outcomes; 5. results. Ascertainment of diagnosis refers to the use of published PD diagnostic criteria. See Supplementary Table S1 for a detailed overview of our applied scoring system.
3 Results
3.1 Search results
Figure 1 shows the disposition of 12,765 initially identified articles in a PRISMA flowchart. Following a full-text review, 90 studies remained for qualitative analysis. Of these, 84 studies had a calculable progression rate, 75 had statistics on the spread of the progression rate and were suitable for meta-analysis of pooled results, and 61 allowed raw progression data simulation. All included studies (4, 5, 14, 19, 24–108) are summarized in Supplementary Table S2.
3.2 Rate of motor progression of PD
At baseline in studies with a calculable rate of motor progression, the mean age was 63.4 ± 4.1 and the median PD duration was 2.4 ± 3.9 years. The median study duration was 3.0 ± 3.5 years, and the median sample size was 92 ± 159. We estimated that that our article selection encompassed approximately 5.7 × 104 patient-years of PD progression. The following motor scoring systems were used to calculate the progression rate: UPDRS-III (including modifications) (109), 54 studies; MDS-UPDRS-III (110), 20 studies; Webster Rating Scale (including modifications) (111), 6 studies; Columbia University Rating Scale (including modifications) (112), 3 studies; and Scales for Outcomes in Parkinson’s disease (113), 1 study. Eligible studies employed different ways to present their motor progression data. We show progression rates in three formats to maximize the amount of source data and to make the best use of information on statistical spread, where provided.
3.2.1 All progression rates
The average increase of the maximum disability score per year in all 84 studies (including 85 cohorts) with a calculable motor progression rate was 2.2% (median = 1.7 ± 1.6%).
3.2.2 Progression rate by random-effects modeling
Enough data were obtained from 75 articles for random-effects modeling. The pooled rate of change was 2.0% (95% CI = 1.7–2.4%) of the maximum disability score per year. This is shown in the forest plot (Figure 2).
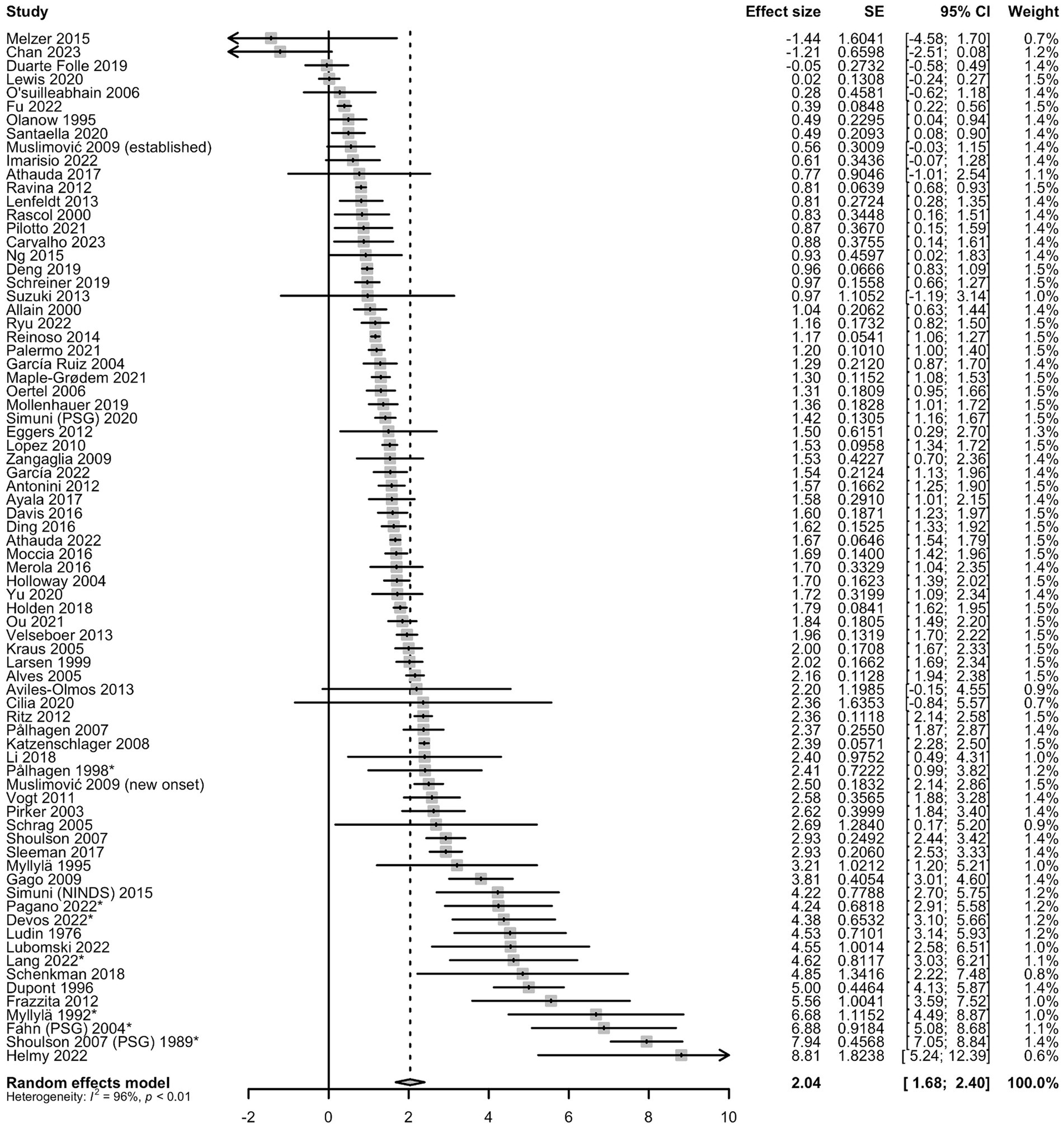
Figure 2. Random forest plot with pooled annual percentage change of maximum disability score. *Articles with untreated patients; SE, standard error.
3.2.3 Data simulation approach
Figure 3 presents a data simulation of 61 studies for which we were able to extract sufficient statistical information on individual time point observations. This illustrates the variety of selected studies in terms of relative motor disability and observation period. The use of different objective motor scales and the paucity of observations extending beyond 10 years can both be appreciated. The linear function of best fit has a slope that indicates motor progression of 1.5% p.a. It should be noted that only 4 of the 8 pre-treatment studies were included in the data simulation, which may have contributed to a lower progression rate than in the summary metric of all studies combined.
3.3 Determinants of motor progression
Table 1 presents a breakdown by study attribute for all 84 articles with a calculable PD progression rate. The most significant difference concerned untreated motor disability, in 8 articles, which was greater than for all other studies that contained individuals on dopaminergic agents (Comparison 5). The untreated disability rate was based on a combined sample of 1,571 clinical trial participants with a mean baseline PD duration of 1.3 ± 0.7 years, observed for a mean period of 0.9 ± 0.1 years. As all untreated disability articles presented clinical trial results in early PD, they contributed to differences in progression rates shown in Comparisons 2 and 4. Excluding the 8 untreated studies from both analyses narrowed those differences and removed their significance (Comparison 2: median 2.0 ± 1.31% p.a. vs. 1.5 ± 1.4% p.a., p = 0.07, q = 0.14; Comparison 4: median 2.0 ± 1.9% p.a. vs. 1.6 ± 1.2% p.a., p = 0.11, q = 0.18).
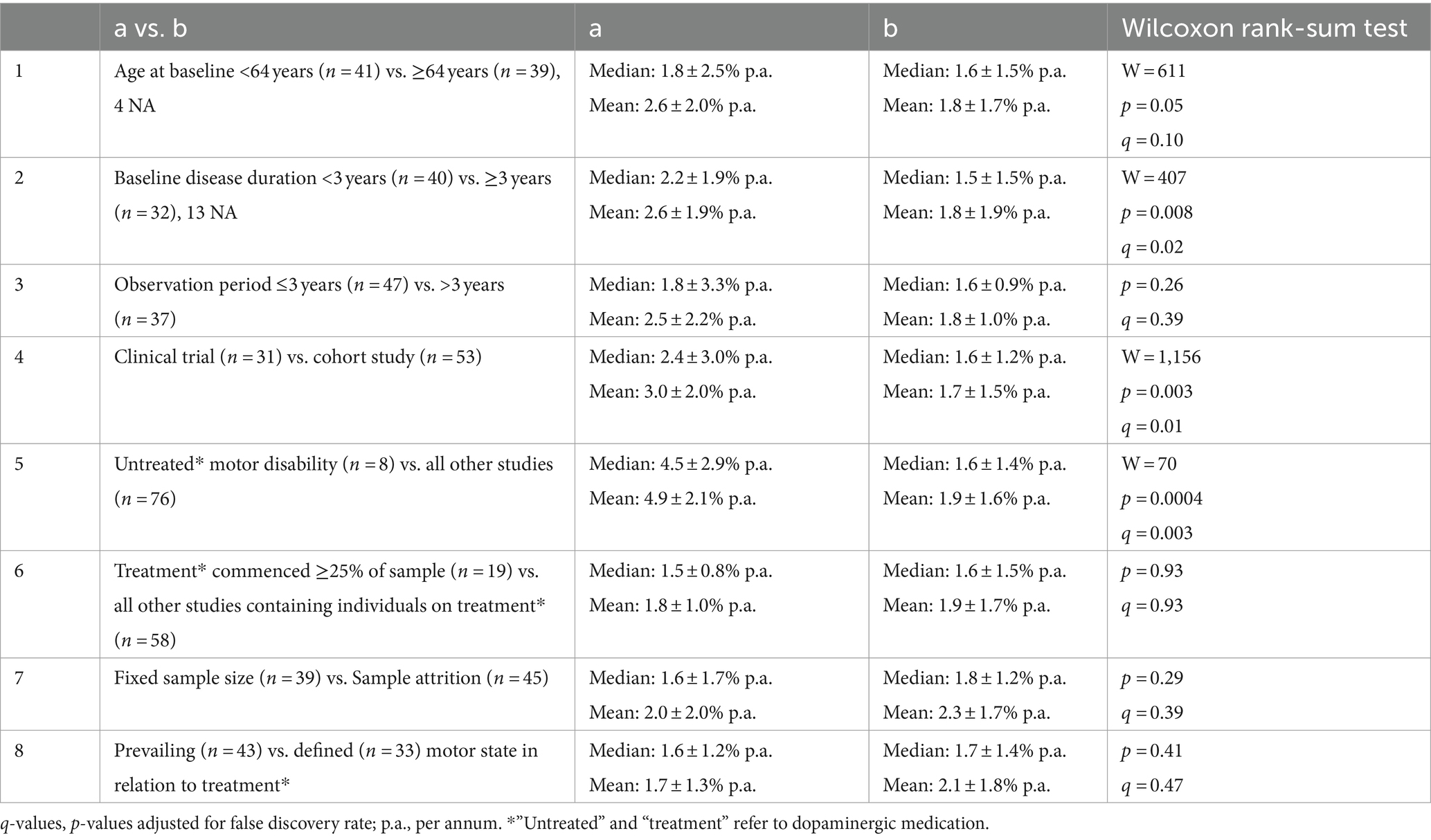
Table 1. Studies with a calculable rate of motor progression, analyzed according to study attributes using the Wilcoxon rank-sum test with correction for multiple comparisons.
Comparison 6 addresses the potentially distorting effect of the commencement of dopaminergic drugs in a substantial number of subjects during an observation period. Comparison 7 looks at ‘real world’ prospective research in which patients are lost to follow-up.
Weighting the progression rate for sample number rendered a mean progression of 1.8% p.a. Weighting for study duration and number of time points gave 1.9% p.a. for each. None of these variables created important bias. Supplementary Figure S2 presents data on progression rate with respect to baseline PD duration.
3.4 On or off scoring
Forty-three studies assessed patients in prevailing motor states (Comparison 8). They showed a small but insignificant reduction in progression rate compared to any study that defined a relationship between motor scoring and the medication cycle. In 24 studies, scoring was performed either on or off state (see Supplementary Table S2). The average on progression rate was 2.1% p.a. (median = 1.6 ± 1.8%), and the average off progression was 2.1% p.a. (median = 1.6 ± 1.1%). In a further 9 studies, defined off state and levodopa test dose assessments were conducted. Here, off exceeded on progression in every case but one (average on 1.8% p.a., average off 2.4% p.a.; median on = 1.3 ± 1.2%, median off = 2.0 ± 1.2%; paired Wilcoxon rank-sum test V = 39, p = 0.05, n = 18).
3.5 Commencement of levodopa treatment
Sixteen studies with concurrent commencement of levodopa met the conditions for calculation of initial response. The mean initial levodopa response was 40.3 ± 15.2% of the pre-treatment disability score. The mean time to maximum improvement in motor score was 7.0 ± 2.3 months in the 10 studies where multiple early time points permitted this to be estimated.
Fifteen articles with concurrent commencement of levodopa had sufficient follow-up to track the progression of motor disability in relation to pre-treatment scores. Pre-treatment disability was exceeded in five studies, after a mean time of 4.5 years. In one of these studies with practically defined off state methodology, the intercept of off motor score with pre-treatment disability was at 4.9 years. The pre-treatment score had not been reached at the end of the observation period in the other 10 studies (after a mean interval of 3.0 years).
3.6 Motor progression of prodromal Parkinson’s disease
Only a handful of studies addressed this topic. Although insufficient for statistical analysis, they provide interesting data on motor function leading up to the time of diagnosable idiopathic PD. One study used olfaction and dopamine transporter scans to define an at-risk sample, back-analyzing motor scores from the time point of phenoconversion (90). The UPDRS motor score worsened at a rate of 7.5% p.a. in the 2 years before diagnosis, whereas progression had been 0.9% p.a. three and more years before diagnosis (90). A cohort identified by REM sleep disorder progressed at 5.2% p.a. over 2 years before phenoconversion (which included individuals who fulfilled diagnostic criteria for dementia with Lewy bodies), compared to 0.6% p.a. three and more years prior to diagnosis (38). Another REM sleep disorder cohort showed a progression rate of 3.2% p.a. for phenoconverters on a 5-year time scale not aligned with the time of PD diagnosis (51). In a study of community-dwelling older adults, subjects with minimal parkinsonian signs by the criteria of Louis et al. (114) progressed only at 0.13% p.a. (63).
3.7 Risk of bias assessment
Seventy-seven studies were judged to have a low risk of bias and 13 studies to have a moderate risk (Supplementary Figure S1). Potential sources of bias were related to selection, diagnosis, and outcomes.
3.8 Meta-regression and heterogeneity
The annual rate of change data was highly heterogeneous between studies, with I2 being 95.6% (CI = 95.0–96.2). Using multivariate linear models, we found no significant influence from motor scale, study duration, study type, sample number, risk of bias assessment, presence of levodopa treatment, relationship to levodopa medication cycle, baseline disease duration, or baseline age on heterogeneity (test of moderator p-value >0.05 for all variables).
4 Discussion
4.1 Motor progression of PD
The long-term rate of motor progression of idiopathic PD is close to 2% p.a. Different methods of statistical handling converged around this number, and factors such as baseline age, sample size, and period of observation had insignificant effects.
Parkinsonian motor disability reflects the underlying loss of neurons in the substantia nigra pars compacta and the resultant dopaminergic deficit. The clinical progression rate from our survey aligns quite well with pathological evidence. In four studies that correlated nigral cell counts with disease duration, the rate of nigral cell loss ranged between 1.2 and 1.9% p.a. with respect to control cell populations (10, 12, 115, 116). While biological processes of growth or decay are often governed by exponential laws, nearly all studies with multiple time points reviewed here showed the linear rate of change and that is how we calculated progression. Linear motor decline accords with most clinicians’ impressions of PD. Exponentiality might be expected to increase the progression rate in longer studies, in studies with greater PD duration at baseline, and in older patients at baseline. In Table 1, the trend is in the opposite direction for each of these subgroup analyses. The main caveat to this conclusion is that few observations on the advanced disease were captured by our publication search.
We tried to examine factors that long-term prospective clinical trials of disease-modifying agents need to contend with. Recruitment in the prodromal or early clinical disease phase anticipates sequential commencement of dopaminergic therapy in a substantial proportion of participants, and eventual attrition of sample size from disability or death. Both might be expected to depress the disease trajectory, through treatment benefit from the first factor and by removal of more rapidly progressing cases by the second. Neither, however, greatly affected the progression rate.
4.2 Effect of on and off scoring
Defined on and off serial motor assessments were available only from a minority of articles. Prevailing motor state studies showed an insignificant reduction in progression rate compared to defined motor states. Studies that classified motor function as either on or off showed, perhaps surprisingly, similar progression rates. Some of these measurements were obtained by labeling prevailing motor function on usual treatments as off or on. Such an approach may result in underscoring of off or overscoring of on. The more rigorous method used in nine other studies employed practically defined off states followed by levodopa test doses with ascertainment of maximum benefit. These articles do suggest that off motor progression exceeds on progression by a modest degree. The on and off comparisons also show that the magnitude of the levodopa response does not decline with disease progression, despite increasing disability in both on and off states.
The motor benefit of levodopa has two components—the short-duration response (SDR), which follows the pharmacokinetic profile of the drug and represents the difference between on and off scores; and the long-duration response (LDR), which outlasts the elimination of the drug at least by days to weeks, composes 30–50% of the overall response, and is much more difficult to measure (117). While shaped by aggregate data obtained in this review, Figure 4 should not be considered part of the statistical analysis. It is presented with a hypothetical depiction of the LDR as a framework to discuss the motor progression of PD in terms of on and off measurements of the levodopa response.
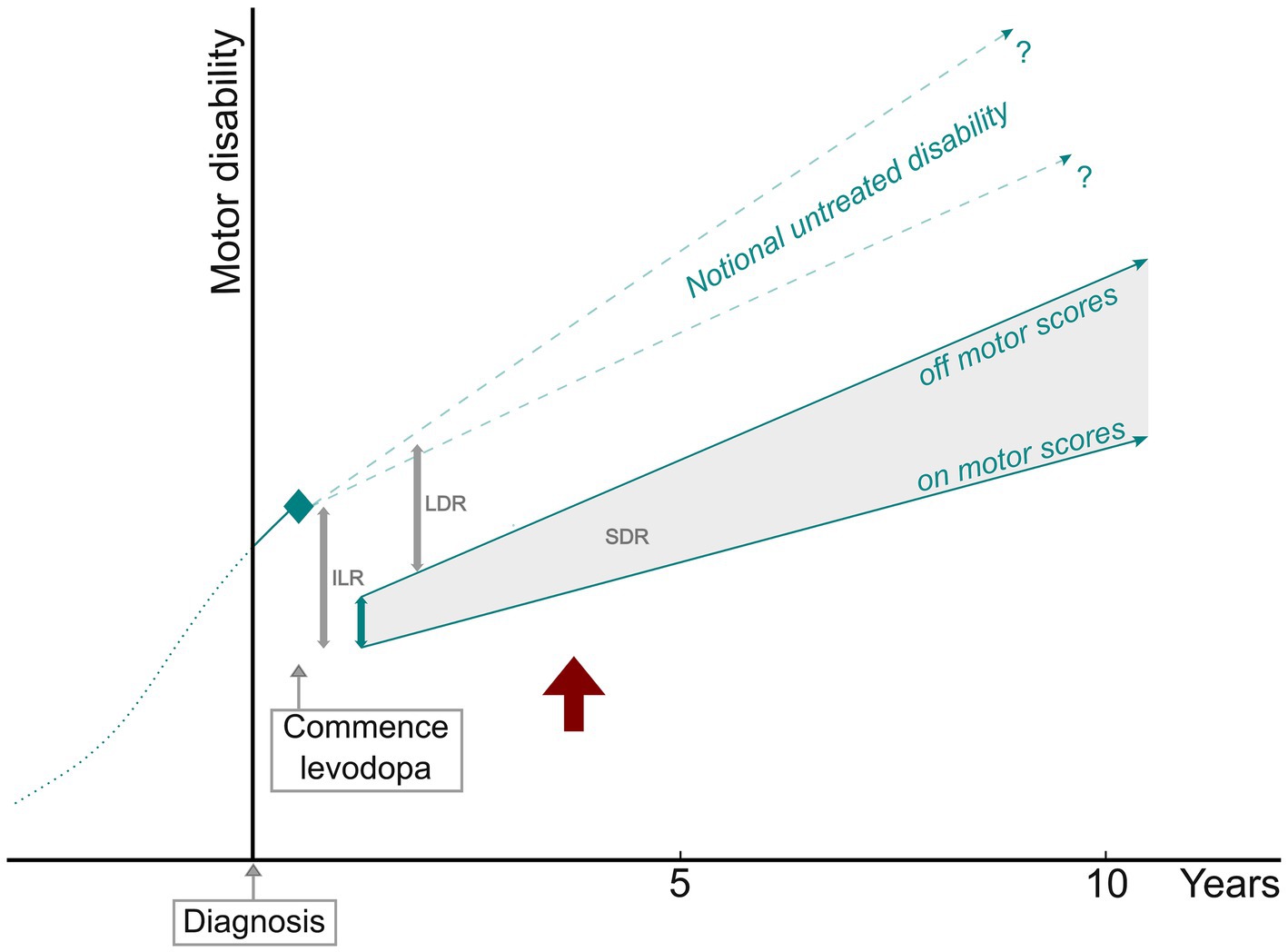
Figure 4. PD motor progression in relation to parameters of the levodopa response. ILR, initial levodopa response; SDR, short-duration response; LDR, long-duration response. Off and on motor score slopes based on the studies with defined off state and levodopa test dose measurements. The red arrow indicates the point at which pre-treatment disability is exceeded.
4.3 Motor progression before dopaminergic treatment and before diagnosis
Although based on a limited number of clinical trials with untreated placebo control participants, there is evidence that motor progression is faster before dopaminergic drugs are commenced. The most statistically significant difference presented in Table 1 is that motor disability increased more than twice as rapidly before treatment. This is unlikely to be an artifact of the lower section of motor scales because, as illustrated in Figure 4, measurements of progression after initial treatment benefit traverse much the same range of scores. While it might be concluded that dopaminergic treatment modifies the motor progression of PD, this does not necessarily mean that some aspect of the underlying pathology is changed. More likely, compensatory processes for nigral cell loss and dopamine deficiency are augmented in some way by exogenous dopamine receptor stimulation.
The idea that certain non-motor features could identify a premonitory phase of PD arose from the Braak model of progression of Lewy pathology and its implication that the substantia nigra is not initially affected (9). With time taken to assemble cohorts with strong risk factors and to follow them long enough to accrue sufficient phenoconversion numbers, there is a relatively small body of research outcomes in this area. Two retrospective back-analyses of phenoconversion in at-risk populations defined in different ways reached a similar conclusion—disability scores in these groups progressed slowly for a time then much more quickly in the 2 years before clinical diagnosis (38, 90). These observations add support to the measurements of untreated PD patients in clinical trials—progression occurs at a rate of more than 4% p.a. in the early motor phase of the disorder (40, 92). In one longitudinal dopamine transporter imaging study, striatal 123-I Ioflupane binding showed greater deterioration in the first year after diagnosis than in subsequent years (118).
4.4 Short-and medium-term effects of dopaminergic treatment
After the commencement of levodopa treatment, we calculated that motor scores declined by 40.3% for pre-treatment disability. Lowest scores were recorded at approximately 7 months, after which they begin to track upward with disease progression. A substantial presence of a LDR can be inferred from the fact that progression measurements do not exceed pre-treatment disability for a number of years.
Nutt et al., employing hand tapping as the sole motor assessment, examined the SDR and LDR at the start of dopaminergic treatment. They measured the SDR for the very first exposure to levodopa; this did not greatly change over the next 12 months, during which time the LDR was progressively established (119). Conversely, one study selected for this meta-analysis conducted UPDRS-III scoring for an initial levodopa test dose and then off and on measurements after 12 months of treatment. The SDR fell to below 50% of the levodopa-naïve magnitude, with a sizable LDR estimated to have developed (32). As shown in Figure 4, a line of notional untreated disability, which would be expected to progress at a certain rate over time, can theoretically help to define the size of the LDR. The gradient of this line is uncertain, though not likely to be less than 2% p.a.
In the medium term, cross-sectional studies of the SDR suggest that its magnitude roughly doubles over 5–10 years of treatment, a trend more pronounced in patients with motor fluctuations (120–122). This predicts that an evolving SDR should delay the onset and reduce the trajectory of the progression of on disability, consistent with those articles that scored both off and on states. A small number of studies identified for this review contained prospective on and off state measurements extending into the second decade of the disease course (35, 48) and suggested that on and off scores eventually deteriorate in parallel.
4.5 Performance of the motor scales
The older UPDRS-III motor scale (maximum disability score = 108) was the most widely used assessment. The UPDRS-III was discriminatory at scores of less than 10 in prodromal PD and in individuals with minimal parkinsonian signs. In studies that recruited untreated subjects with early PD, there was good consistency at approximately 20% of the maximum disability score (median = 19.3 ± 8.9%). The average UPDRS-III score at phenoconversion to diagnosable PD in two prodromal disease studies was 18.4 (38, 90). Five motor scales were included in our review. There were no significant differences in progression rate according to scale type.
4.6 Study limitations
Our broad inclusion criteria selected some studies that were small, were relatively short, had been conducted many years ago, or presented data with insufficient statistical detail for meta-analysis. Nevertheless, we found a consistent pattern of PD progression across a wide range of study types.
The finding that the rate of PD progression appears to be twice as fast in untreated compared to treated patients is both interesting and unexpected. These findings were drawn from methodologically different studies and diverse cohorts. The question is worthy of future examination by double-blind trials designed specifically to address it.
We found a high degree of heterogeneity. Between-study variability can be caused by clinical or methodological diversity (123). With published diagnostic criteria for PD employed by most included articles, clinical heterogeneity from patient selection seems unlikely. We performed mixed model analyses with several moderator variables, none of which could explain a large amount of heterogeneity. Presumably, non-quantifiable between-study methodological diversities were responsible. Although we found no statistically significant influence of motor scale on heterogeneity, it is still possible that combining scoring systems with different scaling properties had some effect. For instance, the UPDRS-III and MDS-UPDRS-III differ from some older scales in emphasis given to bradykinesia over other motor signs, and in their balance of “lateralized” versus “midline” motor items.
Many articles had to be screened for this review, and it is possible that some eligible studies were overlooked. Our study quality assessment, which identified no studies with a high risk of bias, employed a version of the CASP cohort checklist. This had to be applied to studies that did not have our research question as a primary objective.
4.7 Practical implications for long-term measurement of motor progression
1. PD progresses at roughly 2% p.a. in the long term. A neuroprotective treatment that slows disease progression by, say, 30% would be hard to detect over a short observation period.
2. While study designs involving prodromal or untreated PD avoid some confounding effects of symptomatic treatment, different assumptions about the rate of disease progression may be needed. Motor progression appears to be faster before than after dopaminergic drugs are commenced.
3. The effects of starting dopaminergic treatment are substantial and complex. They include the establishment of the LDR, though its presence and magnitude can only be inferred in most circumstances.
4. Practically defined off state measurements probably give the best guide to progression once dopaminergic therapy has begun. A defined off state with levodopa test dose method maximizes information about the medication cycle.
5. It is possible to conduct accurate long-term observations of motor progression that span prodromal, early, and treated disease phases and allow for serial commencement of dopaminergic drugs and sample attrition over time.
6. While simultaneously registering symptomatic treatment effects and underlying disease progression, the objective motor scales are sensitive to small changes in disability. Motor scores show linear temporal relationships throughout the early and middle stages of PD. Significant floor effects in these assessment tools were not apparent in our analysis.
Data availability statement
The original contributions presented in the study are included in the article/Supplementary material, further inquiries can be directed to the corresponding author.
Author contributions
AP: Writing – review & editing, Writing – original draft, Visualization, Project administration, Methodology, Investigation, Formal analysis, Data curation. ALGP: Writing – review & editing, Visualization, Validation, Methodology, Formal analysis, Data curation. CD: Writing – review & editing, Validation, Data curation. TP: Writing – review & editing, Visualization, Validation, Supervision, Methodology, Formal analysis. PK: Writing – review & editing, Writing – original draft, Visualization, Validation, Supervision, Software, Resources, Project administration, Methodology, Investigation, Funding acquisition, Formal analysis, Data curation, Conceptualization.
Funding
The author(s) declare that no financial support was received for the research, authorship, and/or publication of this article.
Acknowledgments
We thank Michael Lawton (29), Louis Tan (34, 81), Samuel Ng (81), Xiao Deng (34), Carl Counsell (33), Regina Katzenschlager (52), Andrew Lees (52), Jenny Head (52), Padraig O’Suilleabhain (69), Aline Duarte Folle (39), Beate Ritz (39), Margaret Schenkman (87), Charity Patterson (87), Daniel Corcos (87), and any other person that helped to provide additional data to their original publications.
Conflict of interest
The authors declare that the research was conducted in the absence of any commercial or financial relationships that could be construed as a potential conflict of interest.
The author(s) declared that they were an editorial board member of Frontiers, at the time of submission. This had no impact on the peer review process and the final decision.
Publisher’s note
All claims expressed in this article are solely those of the authors and do not necessarily represent those of their affiliated organizations, or those of the publisher, the editors and the reviewers. Any product that may be evaluated in this article, or claim that may be made by its manufacturer, is not guaranteed or endorsed by the publisher.
Supplementary material
The Supplementary material for this article can be found online at: https://www.frontiersin.org/articles/10.3389/fneur.2024.1452741/full#supplementary-material
References
1. King, E, Zeissler, ML, Sharpe, P, Boey, T, O’Brian, F, Chapman, D, et al. Neuroprotective trial design methodology for Parkinson’s: a scoping review [abstract]. Mov Disord. (2022) 37
2. Lungu, C, Cedarbaum, JM, Dawson, TM, Dorsey, ER, Faraco, C, Federoff, HJ, et al. Seeking progress in disease modification in Parkinson disease. Parkinsonism Relat Disord. (2021) 90:134–41. doi: 10.1016/j.parkreldis.2021.09.006
3. Lang, AE, Melamed, E, Poewe, W, and Rascol, O. Trial designs used to study neuroprotective therapy in Parkinson’s disease. Mov Disord. (2013) 28:86–95. doi: 10.1002/mds.24997
4. Shoulson, I, Fahn, S, Oakes, D, Odoroff, C, Lang, A, Langston, WJ, et al. Effect of deprenyl on the progression of disability in early Parkinson’s disease. N Engl J Med. (1989) 321:1364–71.
5. Rascol, O, Fitzer-Attas, CJ, Hauser, R, Jankovic, J, Lang, A, Langston, JW, et al. A double-blind, delayed-start trial of rasagiline in Parkinson’s disease (the ADAGIO study): prespecified and post-hoc analyses of the need for additional therapies, changes in UPDRS scores, and non-motor outcomes. Lancet Neurol. (2011) 10:415–23. doi: 10.1016/S1474-4422(11)70073-4
6. Espay, AJ, Bonato, P, Nahab, FB, Maetzler, W, Dean, JM, Klucken, J, et al. Technology in Parkinson disease: challenges and opportunities. Mov Disord. (2016) 31:1272–82. doi: 10.1002/mds.26642
7. Bjornestad, A, Pedersen, KF, Tysnes, OB, and Alves, G. Clinical milestones in Parkinson’s disease: a 7-year population-based incident cohort study. Parkinsonism Relat Disord. (2017) 42:28–33. doi: 10.1016/j.parkreldis.2017.05.025
8. Chahine, LM, Stern, MB, and Chen-Plotkin, A. Blood-based biomarkers for Parkinson’s disease. Parkinsonism Relat Disord. (2014) 20:S99–S103. doi: 10.1016/S1353-8020(13)70025-7
9. Braak, H, Bohl, JR, Müller, CM, Rüb, U, de Vos, RAI, and Del Tredici, K. Stanley Fahn lecture 2005: the staging procedure for the inclusion body pathology associated with sporadic Parkinson’s disease reconsidered. Mov Disord. (2006) 21:2042–51. doi: 10.1002/mds.21065
10. Fearnley, JM, and Lees, AJ. Ageing and Parkinson’s disease: substantia nigra regional selectivity. Brain. (1991) 114:2283–301.
11. Giguère, N, Nanni, SB, and Trudeau, LE. On cell loss and selective vulnerability of neuronal populations in Parkinson’s disease. Front Neurol. (2018) 9:455. doi: 10.3389/fneur.2018.00455
12. Greffard, S, Verny, M, Bonnet, AM, Beinis, JY, Gallinari, C, Meaume, S, et al. Motor score of the unified Parkinson disease rating scale as a good predictor of Lewy body-associated neuronal loss in the substantia nigra. Arch Neurol. (2006) 63:584–8. doi: 10.1001/archneur.63.4.584
13. Page, MJ, McKenzie, JE, Bossuyt, PM, Boutron, I, Hoffmann, TC, Mulrow, CD, et al. The PRISMA 2020 statement: an updated guideline for reporting systematic reviews. BMJ. (2021) 372:n71. doi: 10.1136/bmj.n71
14. Muslimović, D, Post, B, Speelman, JD, De Haan, RJ, and Schmand, B. Cognitive decline in Parkinson’s disease: a prospective longitudinal study. J Int Neuropsychol Soc. (2009) 15:426–37. doi: 10.1017/S1355617709090614
15. Luo, D, Wan, X, Liu, J, and Tong, T. Optimally estimating the sample mean from the sample size, median, mid-range, and/or mid-quartile range. Stat Methods Med Res. (2018) 27:1785–805. doi: 10.1177/0962280216669183
16. Wan, X, Wang, W, Liu, J, and Tong, T. Estimating the sample mean and standard deviation from the sample size, median, range and/or interquartile range. BMC Med Res Methodol. (2014) 14:1–13. doi: 10.1186/1471-2288-14-135
17. Higgins, JPT, and Green, S (editors). Cochrane handbook for systematic reviews of interventions version 5.1.0 [updated march 2011]. The Cochrane collaboration (2011). Available at: https://www.handbook.cochrane.org
18. Rosenthal, R . Meta-analytic procedures for social research. Thousand Oaks, CA: Sage Publications, Inc. (1991).
19. Evans, JR, Mason, SL, Williams-Gray, CH, Foltynie, T, Brayne, C, Robbins, TW, et al. The natural history of treated Parkinson’s disease in an incident, community based cohort. J Neurol Neurosurg Psychiatry. (2011) 82:1112–8. doi: 10.1136/jnnp.2011.240366
20. Balduzzi, S, Rücker, G, and Schwarzer, G. How to perform a meta-analysis with R: a practical tutorial. Evid Based Ment Health. (2019) 22:153–60. doi: 10.1136/ebmental-2019-300117
21. Viechtbauer, W . Conducting meta-analyses in R with the metafor package. J Stat Softw. (2010) 36:1–48. doi: 10.18637/jss.v036.i03
22. Mersmann, O, Trautmann, H, Steuer, D, and Bornkamp, B. Truncnorm: truncated normal distribution. (2023). Available at: https://CRAN.R-project.org/package=truncnorm (Accessed November 16, 2023).
23. CASP Critical appraisal skills Programme (2022). Available at: https://casp-uk.net/ (Accessed July 14, 2023)
24. Alarcón, F, Cevallos, N, and Lees, AJ. Does combined levodopa and bromocriptine therapy in Parkinson’s disease prevent late motor complications? Eur J Neurol. (1998) 5:255–63. doi: 10.1046/j.1468-1331.1998.530255.x
25. Allain, H, Destée, A, Petit, H, Patay, M, Schück, S, Bentué-Ferrer, D, et al. Five-year follow-up of early lisuride and levodopa combination therapy versus levodopa monotherapy in de novo Parkinson’s disease. The French Lisuride study group. Eur Neurol. (2000) 44:22–30. doi: 10.1159/000008188
26. Alves, G, Wentzel-Larsen, T, Aarsland, D, and Larsen, JP. Progression of motor impairment and disability in Parkinson disease: a population-based study. Neurology. (2005) 65:1436–41. doi: 10.1212/01.wnl.0000183359.50822.f2
27. Antonini, A, Barone, P, Marconi, R, Morgante, L, Zappulla, S, Pontieri, FE, et al. The progression of non-motor symptoms in Parkinson’s disease and their contribution to motor disability and quality of life. J Neurol. (2012) 259:2621–31. doi: 10.1007/s00415-012-6557-8
28. Athauda, D, Maclagan, K, Skene, SS, Bajwa-Joseph, M, Letchford, D, Chowdhury, K, et al. Exenatide once weekly versus placebo in Parkinson’s disease: a randomised, double-blind, placebo-controlled trial. Lancet. (2017) 390:1664–75. doi: 10.1016/S0140-6736(17)31585-4
29. Athauda, D, Evans, J, Wernick, A, Virdi, G, Choi, ML, Lawton, M, et al. The impact of type 2 diabetes in Parkinson’s disease. Mov Disord. (2022) 37:1612–23. doi: 10.1002/mds.29122
30. Ayala, A, Triviño-Juárez, JM, Forjaz, MJ, Rodríguez-Blázquez, C, Rojo-Abuin, JM, Martínez-Martín, P, et al. Parkinson’s disease severity at 3 years can be predicted from non-motor symptoms at baseline. Front Neurol. (2017) 8:551. doi: 10.3389/fneur.2017.00551
31. Carvalho de Abreu, DC, Pieruccini-Faria, F, Sarquis-Adamson, Y, Black, A, Fraser, J, Van Ooteghem, K, et al. White matter hyperintensity burden predicts cognitive but not motor decline in Parkinson’s disease: results from the Ontario neurodegenerative diseases research initiative. Eur J Neurol. (2023) 30:920–33. doi: 10.1111/ene.15692
32. Cilia, R, Cereda, E, Akpalu, A, Sarfo, FS, Cham, M, Laryea, R, et al. Natural history of motor symptoms in Parkinson’s disease and the long-duration response to levodopa. Brain. (2020) 143:2490–501. doi: 10.1093/brain/awaa181
33. Davidson, MB, McGhee, DJM, and Counsell, CE. Comparison of patient rated treatment response with measured improvement in Parkinson’s disease. J Neurol Neurosurg Psychiatry. (2012) 83:1001–5. doi: 10.1136/jnnp-2012-302741
34. Deng, X, Xiao, B, Allen, JC, Ng, E, Foo, JN, Lo, YL, et al. Parkinson’s disease GWAS-Linked Park 16 carriers show greater motor progression. J Med Genet. (2019) 56:765–8. doi: 10.1136/jmedgenet-2018-105661
35. Ding, C, Ganesvaran, G, Alty, JE, Clissold, BG, Mccoll, CD, Reardon, KA, et al. Study of levodopa response in Parkinson’s disease: observations on rates of motor progression. Mov Disord. (2016) 31:589–92. doi: 10.1002/mds.26497
36. Dupont, E, Andersen, A, Boas, J, Boisen, E, Borgmann, R, Helgetveit, AC, et al. Sustained-release Madopar HBS compared with standard Madopar in the long-term treatment of de novo parkinsonian patients. Acta Neurol Scand. (1996) 93:14–20. doi: 10.1111/j.1600-0404.1996.tb00163.x
37. Eggers, C, Pedrosa, DJ, Kahraman, D, Maier, F, Lewis, CJ, Fink, GR, et al. Parkinson subtypes progress differently in clinical course and imaging pattern. PLoS One. (2012) 7:e46813. doi: 10.1371/journal.pone.0046813
38. Fereshtehnejad, SM, Yao, C, Pelletier, A, Montplaisir, JY, Gagnon, JF, and Postuma, RB. Evolution of prodromal Parkinson’s disease and dementia with Lewy bodies: a prospective study. Brain. (2019) 142:2051–67. doi: 10.1093/brain/awz111
39. Duarte Folle, A, Paul, KC, Bronstein, JM, Keener, AM, and Ritz, B. Clinical progression in Parkinson’s disease with features of REM sleep behavior disorder: a population-based longitudinal study. Parkinsonism Relat Disord. (2019) 62:105–11. doi: 10.1016/j.parkreldis.2019.01.018
40. Frazzitta, G, Bertotti, G, Riboldazzi, G, Turla, M, Uccellini, D, Boveri, N, et al. Effectiveness of intensive inpatient rehabilitation treatment on disease progression in parkinsonian patients: a randomized controlled trial with 1-year follow-up. Neurorehabil Neural Repair. (2012) 26:144–50. doi: 10.1177/1545968311416990
41. Fu, XY, Zhang, YC, Ding, CW, Zhao, P, Liu, QY, Yang, M, et al. Association between asymmetry of substantia nigra hyperechogenicity and characteristics in different Parkinson disease subtypes: a 5-year follow-up study. Ultrasound Med Biol. (2022) 48:2139–45. doi: 10.1016/j.ultrasmedbio.2022.06.006
42. Gago, MF, Garrett, MC, Fonseca, MR, Rosas, MJ, Simões, MF, Vieira, S, et al. How do cognitive and axial motor signs correlate in Parkinson’s disease? A 6-year prospective study. J Neurol. (2009) 256:1655–62. doi: 10.1007/s00415-009-5174-7
43. García Ruiz, PJ, Meseguer, E, Del Val, J, Vázquez, A, Sanchez Bernardos, V, and Vázquez, A. Motor complications in Parkinson disease: a prospective follow-up study. Clin Neuropharmacol. (2004) 27:49–52. doi: 10.1097/00002826-200403000-00001
44. Helmy, A, Hamid, E, Salama, M, Gaber, A, El-Belkimy, M, and Shalash, A. Baseline predictors of progression of Parkinson’s disease in a sample of Egyptian patients: clinical and biochemical. Egypt J Neurol Psychiatr Neurosurg. (2022) 58:9. doi: 10.1186/S41983-022-00445-1
45. Hely, MA, Morris, JGL, Reid, WGJ, O’Sullivan, DJ, Williamson, PM, Rail, D, et al. The Sydney multicentre study of Parkinson’s disease: a randomised, prospective five year study comparing low dose bromocriptine with low dose levodopa-carbidopa. J Neurol Neurosurg Psychiatry. (1994) 57:903–10. doi: 10.1136/jnnp.57.8.903
46. Holden, SK, Finseth, T, Sillau, SH, and Berman, BD. Progression of MDS-UPDRS scores over five years in de novo Parkinson disease from the Parkinson’s progression markers initiative cohort. Mov Disord Clin Pract. (2018) 5:47–53. doi: 10.1002/mdc3.12553
47. Holloway, RG, Kieburtz, K, McDermott, M, Tariot, P, Kamp, C, Day, D, et al. Pramipexole vs levodopa as initial treatment for Parkinson disease: a 4-year randomized controlled trial. Arch Neurol. (2004) 61:1044–53. doi: 10.1001/archneur.61.7.1044
48. Hughes, AJ, Frankel, JP, Kempster, PA, Stem, GM, and Lees, AJ. Motor response to levodopa in patients with parkinsonian motor fluctuations: a follow-up study over three years. J Neurol Neurosurg Psychiatry. (1994) 57:430–4. doi: 10.1136/jnnp.57.4.430
49. Imarisio, A, Pilotto, A, Garrafa, E, Conforti, F, Masciocchi, S, Turrone, R, et al. Plasma cystatin C correlates with plasma NfL levels and predicts disease progression in Parkinson’s disease. Neurodegener Dis. (2022) 21:109–16. doi: 10.1159/000523982
50. Jankovic, J, and Kapadia, AS. Functional decline in Parkinson disease. Arch Neurol. (2001) 58:1611–5. doi: 10.1001/archneur.58.10.1611
51. Joza, S, Hu, MT, Jung, KY, Kunz, D, Stefani, A, Dušek, P, et al. Progression of clinical markers in prodromal Parkinson’s disease and dementia with Lewy bodies: a multicentre study. Brain. (2023) 146:3258–72. doi: 10.1093/brain/awad072
52. Katzenschlager, R, Head, J, Schrag, A, Ben-Shlomo, Y, Evans, A, and Lees, AJ. Fourteen-year final report of the randomized PDRG-UK trial comparing three initial treatments in PD. Neurology. (2008) 71:474–80. doi: 10.1212/01.wnl.0000310812.43352.66
53. Kraus, PH, Klotz, P, Hoffmann, A, Lewe, J, and Przuntek, H. Analysis of the course of Parkinson’s disease under dopaminergic therapy: performance of “fast tapping” is not a suitable parameter. Mov Disord. (2005) 20:348–54. doi: 10.1002/mds.20265
54. Larsen, JP, Boas, J, and Erdal, JE. Does selegiline modify the progression of early Parkinson’s disease? Results from a five-year study. The Norwegian-Danish study group. Eur J Neurol. (1999) 6:539–47. doi: 10.1046/j.1468-1331.1999.650539.x
55. Lenfeldt, N, Hansson, W, Larsson, A, Nyberg, L, Birgander, R, and Forsgren, L. Diffusion tensor imaging and correlations to Parkinson rating scales. J Neurol. (2013) 260:2823–30. doi: 10.1007/s00415-013-7080-2
56. Lewis, MM, Harkins, E, Lee, EY, Stetter, C, Snyder, B, Corson, T, et al. Clinical progression of Parkinson’s disease: insights from the NINDS common data elements. J Parkinsons Dis. (2020) 10:1075–85. doi: 10.3233/JPD-201932
57. Li, W, Lao-Kaim, NP, Roussakis, AA, Martín-Bastida, A, Valle-Guzman, N, Paul, G, et al. 11 C-PE2I and 18 F-Dopa PET for assessing progression rate in Parkinson’s: a longitudinal study. Mov Disord. (2018) 33:117–27. doi: 10.1002/mds.27183
58. López, IC, Ruiz, PJG, Del Pozo, SVF, and Bernardos, VS. Motor complications in Parkinson’s disease: ten year follow-up study. Mov Disord. (2010) 25:2735–9. doi: 10.1002/mds.23219
59. Louis, ED, Tang, MX, Cote, L, Alfaro, B, Mejia, H, and Marder, K. Progression of parkinsonian signs in Parkinson disease. Arch Neurol. (1999) 56:334–7. doi: 10.1001/archneur.56.3.334
60. Lubomski, M, Xu, X, Holmes, AJ, Muller, S, Yang, JYH, Davis, RL, et al. The gut microbiome in Parkinson’s disease: a longitudinal study of the impacts on disease progression and the use of device-assisted therapies. Front Aging Neurosci. (2022) 14:875261. doi: 10.3389/fnagi.2022.875261
61. Ludin, HP, and Bass-Verrey, F. Study of deterioration in long-term treatment of parkinsonism with L-dopa plus decarboxylase inhibitor. J Neural Transm. (1976) 38:249–58. doi: 10.1007/BF01249442
62. Merola, A, Espay, AJ, Romagnolo, A, Bernardini, A, Rizzi, L, Rosso, M, et al. Advanced therapies in Parkinson’s disease: long-term retrospective study. Parkinsonism Relat Disord. (2016) 29:104–8. doi: 10.1016/j.parkreldis.2016.05.015
63. Miller-Patterson, C, Han, J, Yaffe, K, Rosso, AL, Launer, LJ, Kritchevsky, SB, et al. Clinical and neuroimaging correlates of progression of mild parkinsonian signs in community-dwelling older adults. Parkinsonism Relat Disord. (2020) 75:85–90. doi: 10.1016/j.parkreldis.2020.05.023
64. Moccia, M, Erro, R, Picillo, M, Vitale, C, Longo, K, Amboni, M, et al. Caffeine consumption and the 4-year progression of de novo Parkinson’s disease. Parkinsonism Relat Disord. (2016) 32:116–9. doi: 10.1016/j.parkreldis.2016.08.005
65. Mollenhauer, B, Zimmermann, J, Sixel-Döring, F, Focke, NK, Wicke, T, Ebentheuer, J, et al. Baseline predictors for progression 4 years after Parkinson’s disease diagnosis in the De novo Parkinson cohort (DeNoPa). Mov Disord. (2019) 34:67–77. doi: 10.1002/mds.27492
66. Myllylä, VV, Sotaniemi, KA, Vuorinen, JA, and Heinonen, EH. Selegiline as initial treatment in de novo parkinsonian patients. Neurology. (1992) 42:339–43. doi: 10.1212/WNL.42.2.339
67. Myllylä, VV, Heinonen, EH, Vuorinen, JA, Kilkku, OI, and Sotaniemi, KA. Early selegiline therapy reduces levodopa dose requirement in Parkinson’s disease. Acta Neurol Scand. (1995) 91:177–82. doi: 10.1111/j.1600-0404.1995.tb00429.x
68. Ng, A, Chander, RJ, Tan, LCS, and Kandiah, N. Influence of depression in mild Parkinson’s disease on longitudinal motor and cognitive function. Parkinsonism Relat Disord. (2015) 21:1056–60. doi: 10.1016/j.parkreldis.2015.06.014
69. O’Suilleabhain, PE, Oberle, R, Bartis, C, Dewey, RB, Bottiglieri, T, and Diaz-Arrastia, R. Clinical course in Parkinson’s disease with elevated homocysteine. Parkinsonism Relat Disord. (2006) 12:103–7. doi: 10.1016/j.parkreldis.2005.10.002
70. Oertel, WH, Wolters, E, Sampaio, C, Gimenez-Roldan, S, Bergamasco, B, Dujardin, M, et al. Pergolide versus levodopa monotherapy in early Parkinson’s disease patients: the PELMOPET study. Mov Disord. (2006) 21:343–53. doi: 10.1002/mds.20724
71. Olanow, CW, Hauser, RA, Gauguster, L, Malapira, T, Koller, W, Hubble, J, et al. The effect of deprenyl and levodopa on the progression of Parkinson’s disease. Ann Neurol. (1995) 38:771–7. doi: 10.1002/ana.410380512
72. Olanow, CW, Schapira, AH, LeWitt, PA, Kieburtz, K, Sauer, D, Olivieri, G, et al. TCH346 as a neuroprotective drug in Parkinson’s disease: a double-blind, randomised, controlled trial. Lancet Neurol. (2006) 5:1013–20. doi: 10.1016/S1474-4422(06)70602-0
73. Ou, R, Hou, Y, Wei, Q, Lin, J, Liu, K, Zhang, L, et al. Longitudinal evolution of non-motor symptoms in early Parkinson’s disease: a 3-year prospective cohort study. NPJ Parkinsons Dis. (2021) 7:58. doi: 10.1038/s41531-021-00207-5
74. Palermo, G, Giannoni, S, Giuntini, M, Belli, E, Frosini, D, Siciliano, G, et al. Statins in Parkinson’s disease: influence on motor progression. J Parkinsons Dis. (2021) 11:1651–62. doi: 10.3233/JPD-212655
75. Pålhagen, SE, and Heinonen, E. Use of selegiline as monotherapy and in combination with levodopa in the management of Parkinson’s disease: perspectives from the MONOCOMB study. Prog Neurother Neuropsychopharmacol. (2007) 3:49–71.
76. Pålhagen, S, Heinonen, EH, Hägglund, J, Kaugesaar, T, Kontants, H, Mäki-Ikola, O, et al. Selegiline delays the onset of disability in de novo parkinsonian patients. Neurology. (1998) 51:520–5. doi: 10.1212/wnl.51.2.520
77. Pirker, W, Holler, I, Gerschlager, W, Asenbaum, S, Zettinig, G, and Brücke, T. Measuring the rate of progression of Parkinson’s disease over a 5-year period with β-CIT SPECT. Mov Disord. (2003) 18:1266–72. doi: 10.1002/mds.10531
78. Simuni, T, Oakes, D, Biglan, K, Galpern, WR, Hauser, R, Hodgeman, K, et al. Isradipine versus placebo in early Parkinson disease a randomized trial. Ann Intern Med. (2020) 172:591–8. doi: 10.7326/M19-2534
79. Rascol, O, Brooks, DJ, Korczyn, AD, De Deyn, PP, Clarke, CE, and Lang, AE. A five-year study of the incidence of dyskinesia in patients with early Parkinson’s disease who were treated with ropinirole or levodopa. N Engl J Med. (2000) 342:1484–91. doi: 10.1056/NEJM200005183422004
80. Ravina, B, Marek, K, Eberly, S, Oakes, D, Kurlan, R, Ascherio, A, et al. Dopamine transporter imaging is associated with long-term outcomes in Parkinson’s disease. Mov Disord. (2012) 27:1392–7. doi: 10.1002/mds.25157
81. Reinoso, G, Allen, JC, Au, WL, Seah, SH, Tay, KY, and Tan, LCS. Clinical evolution of Parkinson’s disease and prognostic factors affecting motor progression: 9-year follow-up study. Eur J Neurol. (2014) 22:457–63. doi: 10.1111/ene.12476
82. Rinne, UK, Bracco, F, Chouza, C, Dupont, E, Gershanik, O, Marti Masso, JF, et al. Cabergoline in the treatment of early Parkinson’s disease: results of the first year of treatment in a double-blind comparison of cabergoline and levodopa. The PKDS009 collaborative study group. Neurology. (1997) 48:363–8. doi: 10.1212/WNL.48.2.363
83. Rinne, UK, Bracco, F, Chouza, C, Dupont, E, Gershanik, O, Marti Masso, JF, et al. Early treatment of Parkinson’s disease with cabergoline delays the onset of motor complications. Results of a double-blind levodopa controlled trial. The PKDS009 study group. Drugs. (1998) 55:23–30. doi: 10.2165/00003495-199855001-00004
84. Ryu, DW, Yoo, SW, Oh, YS, Lee, KS, Ha, S, and Kim, JS. Comparison of disease progression between brain-predominant Parkinson’s disease versus Parkinson’s disease with body-involvement phenotypes. Neurobiol Dis. (2022) 174:105883. doi: 10.1016/j.nbd.2022.105883
85. Santaella, A, Kuiperij, HB, van Rumund, A, Esselink, RAJ, van Gool, AJ, Bloem, BR, et al. Cerebrospinal fluid monocyte chemoattractant protein 1 correlates with progression of Parkinson’s disease. NPJ Parkinsons Dis. (2020) 6:21. doi: 10.1038/s41531-020-00124-z
86. García, DS, Canfield, H, De Deus, FT, Bartolomé, CC, Ríos, LN, Roca, LG, et al. Parkinson’s disease motor subtypes change with the progression of the disease: results from the COPPADIS cohort at 2-year follow-up. J Parkinsons Dis. (2022) 12:935–55. doi: 10.3233/JPD-213004
87. Schenkman, M, Moore, CG, Kohrt, WM, Hall, DA, Delitto, A, Comella, CL, et al. Effect of high-intensity treadmill exercise on motor symptoms in patients with de novo Parkinson disease: a phase 2 randomized clinical trial. JAMA Neurol. (2018) 75:219–26. doi: 10.1001/jamaneurol.2017.3517
88. Schrag, A, Dodel, R, Spottke, A, Bornschein, B, Siebert, U, and Quinn, NP. Rate of clinical progression in Parkinson’s disease. A prospective study. Mov Disord. (2007) 22:938–45. doi: 10.1002/mds.21429
89. Schreiner, SJ, Imbach, LL, Werth, E, Poryazova, R, Baumann-Vogel, H, Valko, PO, et al. Slow-wave sleep and motor progression in Parkinson disease. Ann Neurol. (2019) 85:765–70. doi: 10.1002/ana.25459
90. Siderowf, A, Jennings, D, Stern, M, Seibyl, J, Eberly, S, Oakes, D, et al. Clinical and imaging progression in the PARS cohort: long-term follow-up. Mov Disord. (2020) 35:1550–7. doi: 10.1002/mds.28139
91. Simuni, T, Kieburtz, K, Tilley, B, Elm, J, Ravina, B, Babcock, D, et al. Pioglitazone in early Parkinson’s disease: a phase 2, multicentre, double-blind, randomised trial. Lancet Neurol. (2015) 14:795–803. doi: 10.1016/S1474-4422(15)00144-1
92. Sleeman, I, Aspray, T, Lawson, R, Coleman, S, Duncan, G, Khoo, TK, et al. The role of vitamin D in disease progression in early Parkinson’s disease. J Parkinsons Dis. (2017) 7:669–75. doi: 10.3233/JPD-171122
93. Suzuki, M, Yoshioka, M, Hashimoto, M, Murakami, M, Noya, M, Takahashi, D, et al. Randomized, double-blind, placebo-controlled trial of vitamin D supplementation in Parkinson disease. Am J Clin Nutr. (2013) 97:1004–13. doi: 10.3945/ajcn.112.051664
94. Velseboer, DC, Broeders, M, Post, B, Van Geloven, N, Speelman, JD, Schmand, B, et al. Prognostic factors of motor impairment, disability, and quality of life in newly diagnosed PD. Neurology. (2013) 80:627–33. doi: 10.1212/WNL.0b013e318281cc99
95. Vogt, T, Kramer, K, Gartenschlaeger, M, and Schreckenberger, M. Estimation of further disease progression of Parkinson’s disease by dopamin transporter scan vs clinical rating. Parkinsonism Relat Disord. (2011) 17:459–63. doi: 10.1016/j.parkreldis.2011.04.002
96. Yu, HX, Guo, MR, Li, G, and Zhang, B. Association between fatigue and motor progression in Parkinson’s disease in southern Chinese. Neurol Sci. (2020) 41:161–4. doi: 10.1007/s10072-019-04059-z
97. Zangaglia, R, Pacchetti, C, Pasotti, C, Mancini, F, Servello, D, Sinforiani, E, et al. Deep brain stimulation and cognitive functions in Parkinson’s disease: a three-year controlled study. Mov Disord. (2009) 24:1621–8. doi: 10.1002/mds.22603
98. Pilotto, A, Imarisio, A, Conforti, F, Scalvini, A, Masciocchi, S, Nocivelli, S, et al. Plasma NfL, clinical subtypes and motor progression in Parkinson’s disease. Parkinsonism Relat Disord. (2021) 87:41–7. doi: 10.1016/j.parkreldis.2021.04.016
99. Aviles-Olmos, I, Dickson, J, Kefalopoulou, Z, Djamshidian, A, Ell, P, Soderlund, T, et al. Exenatide and the treatment of patients with Parkinson’s disease. J Clin Invest. (2013) 123:2730–6. doi: 10.1172/JCI68295
100. Davis, MY, Johnson, CO, Leverenz, JB, Weintraub, D, Trojanowski, JQ, Chen-Plotkin, A, et al. Association of GBA mutations and the E326K polymorphism with motor and cognitive progression in Parkinson disease. JAMA Neurol. (2016) 73:1217–24. doi: 10.1001/jamaneurol.2016.2245
101. Shoulson, I, Schwid, S, Hyson, C, Oakes, D, Gorbold, E, Rudolph, A, et al. Mixed lineage kinase inhibitor CEP-1347 fails to delay disability in early Parkinson disease. Neurology. (2007) 69:1480–90. doi: 10.1212/01.wnl.0000277648.63931.c0
102. Maple-Grødem, J, Dalen, I, Tysnes, OB, Macleod, AD, Forsgren, L, Counsell, CE, et al. Association of GBA genotype with motor and functional decline in patients with newly diagnosed Parkinson disease. Neurology. (2021) 96:e1036–44. doi: 10.1212/WNL.0000000000011411
103. Chan, L, Chung, CC, Hsieh, YC, Wu, RM, and Hong, CT. Plasma extracellular vesicle tau, β-amyloid, and α-synuclein and the progression of Parkinson’s disease: a follow-up study. Ther Adv Neurol Disord. (2023) 16:1–14. doi: 10.1177/17562864221150329
104. Ritz, B, Rhodes, SL, Bordelon, Y, and Bronstein, J. α-Synuclein genetic variants predict faster motor symptom progression in idiopathic Parkinson disease. PLoS One. (2012) 7:e36199. doi: 10.1371/journal.pone.0036199
105. Pagano, G, Taylor, KI, Anzures-Cabrera, J, Marchesi, M, Simuni, T, Marek, K, et al. Trial of prasinezumab in early-stage Parkinson’s disease. N Engl J Med. (2022) 387:421–32. doi: 10.1056/NEJMoa2202867
106. Devos, D, Labreuche, J, Rascol, O, Corvol, J-C, Duhamel, A, Guyon Delannoy, P, et al. Trial of deferiprone in Parkinson’s disease. N Engl J Med. (2022) 387:2045–55. doi: 10.1056/NEJMoa2209254
107. Lang, AE, Siderowf, AD, Macklin, EA, Poewe, W, Brooks, DJ, Fernandez, HH, et al. Trial of cinpanemab in early Parkinson’s disease. N Engl J Med. (2022) 387:408–20. doi: 10.1056/NEJMoa2203395
108. Melzer, TR, Myall, DJ, Mac Askill, MR, Pitcher, TL, Livingston, L, Watts, R, et al. Tracking Parkinson’s disease over one year with multimodal magnetic resonance imaging in a group of older patients with moderate disease. Plo S One. (2015) 10:e0143923. doi: 10.1371/journal.pone.0143923
109. Fahn, S, and Elton, RLUPDRS Program Members. Unified Parkinsons disease rating scale In: S Fahn, C Marsden, M Goldstein, and D Calne, editors. Recent developments in Parkinsons disease. Florham Park, NJ: Macmillan Healthcare Information (1987). 153–63.
110. Goetz, CG, Tilley, BC, Shaftman, SR, Stebbins, GT, Fahn, S, Martinez-Martin, P, et al. Movement Disorder Society-sponsored revision of the unified Parkinson’s disease rating scale (MDS-UPDRS): scale presentation and clinimetric testing results. Mov Disord. (2008) 23:2129–70. doi: 10.1002/mds.22340
111. Webster, DD . Clinical analysis of the disability in Parkinson’s disease. Mod Treatm. (1968) 5:257–82.
112. Duvoisin, R. The evaluation of extrapyramidal disease. In: J Ajuriaguerrade and G Gauthier, editors. Monoamines, noyaux gris centraux et syndrome de Parkinson. Paris: Masson (1971). p. 313–325.
113. Marinus, J, Visser, M, Stiggelbout, AM, Rabey, JM, Martínez-Martín, P, Bonuccelli, U, et al. A short scale for the assessment of motor impairments and disabilities in Parkinson’s disease: the SPES/SCOPA. J Neurol Neurosurg Psychiatry. (2004) 75:388–95. doi: 10.1136/jnnp.2003.017509
114. Louis, ED, Tang, MX, and Mayeux, R. Parkinsonian signs in older people in a community-based study: risk of incident dementia. Arch Neurol. (2004) 61:1273–6. doi: 10.1001/archneur.61.8.1273
115. Ma, SY, Röyttä, M, Rinne, JO, Collan, Y, and Rinne, UK. Correlation between neuromorphometry in the substantia nigra and clinical features in Parkinson’s disease using disector counts. J Neurol Sci. (1997) 151:83–7. doi: 10.1016/s0022-510x(97)00100-7
116. Kordower, JH, Olanow, CW, Dodiya, HB, Chu, Y, Beach, TG, Adler, CH, et al. Disease duration and the integrity of the nigrostriatal system in Parkinson’s disease. Brain. (2013) 136:2419–31. doi: 10.1093/brain/awt192
117. Nutt, JG, Carter, JH, and Woodward, WR. Long-duration response to levodopa. Neurology. (1995) 45:1613–6. doi: 10.1212/WNL.45.8.1613
118. Simuni, T, Siderowf, A, Lasch, S, Coffey, CS, Caspell-Garcia, C, Jennings, D, et al. Longitudinal change of clinical and biological measures in early Parkinson’s disease: Parkinson’s progression markers initiative cohort. Mov Disord. (2018) 33:771–82. doi: 10.1002/mds.27361
119. Nutt, JG, Carter, JH, Van Houten, L, and Woodward, WR. Short-and long-duration responses to levodopa during the first year of levodopa therapy. Ann Neurol. (1997) 42:349–55. doi: 10.1002/ana.410420311
120. Nutt, JG, Woodward, WR, Carter, JH, and Gancher, ST. Effect of long-term therapy on the pharmacodynamics of levodopa. Relation to on-off phenomenon. Arch Neurol. (1992) 49:1123–30. doi: 10.1001/archneur.1992.00530350037016
121. Pieterman, MM . Investigating the acute levodopa response in early to advanced Parkinson’s disease. Electronic Thesis and Dissertation Repository. (2017). Available at: https://ir.lib.uwo.ca/etd/4641 (Accessed November 8, 2023).
122. Kempster, PA, Frankel, JP, Bovingdon, M, Webster, R, Lees, AJ, and Stern, GM. Levodopa peripheral pharmacokinetics and duration of motor response in Parkinson’s disease. J Neurol Neurosurg Psychiatry. (1989) 52:718–23. doi: 10.1136/jnnp.52.6.718
Keywords: Parkinson’s disease, levodopa, progression, natural history, meta-analysis
Citation: Pauwels A, Phan ALG, Ding C, Phan TG and Kempster PA (2024) Rate of motor progression in Parkinson’s disease: a systematic review and meta-analysis. Front. Neurol. 15:1452741. doi: 10.3389/fneur.2024.1452741
Edited by:
David Eidelberg, Feinstein Institute for Medical Research, United StatesReviewed by:
Tomaz Rus, University Medical Centre Ljubljana, SloveniaChris C. Tang, Feinstein Institute for Medical Research, United States
Copyright © 2024 Pauwels, Phan, Ding, Phan and Kempster. This is an open-access article distributed under the terms of the Creative Commons Attribution License (CC BY). The use, distribution or reproduction in other forums is permitted, provided the original author(s) and the copyright owner(s) are credited and that the original publication in this journal is cited, in accordance with accepted academic practice. No use, distribution or reproduction is permitted which does not comply with these terms.
*Correspondence: Peter A. Kempster, cGV0ZXIua2VtcHN0ZXJAbW9uYXNoaGVhbHRoLm9yZw==