- 1Beijing University of Chinese Medicine, Beijing, China
- 2Department of Neurology, Peking University People’s Hospital, Beijing, China
- 3Department of Neurology, China-Japan Friendship Hospital, Beijing, China
Background: Recent studies have shown that alkaline phosphatase to albumin ratio (APAR) is a prognostic biomarker for coronary heart disease and cancer. However, the effect of APAR on the prognosis of ischemic stroke (IS) remains unclear. We aimed to assess the association of APAR with all-cause mortality in critically ill patients with IS.
Methods: Critically ill patients with IS were identified from the Medical Information Mart for Intensive Care-IV (MIMIC-IV) Version 3.0 database, and classified into quartiles based on APAR index levels. Clinical outcomes included all-cause mortality at 28-days, 90-days and 365-days after admission. Cox proportional hazards regression analysis and restricted cubic spline method were used to clarify the relationship between APAR index and clinical outcomes in critically ill patients with IS.
Results: A total of 1,690 critically ill patients with IS were selected from the MIMIC-IV database. Multivariate Cox proportional hazard analysis showed that increased APAR index was significantly associated with all-cause mortality. After adjusting for potential confounding factors, patients with higher APAR (Q4: 1.524–2.794) had significantly increased all-cause mortality at 28-days, 90-days, and 365-days after admission (HR 2.05, 95%CI 1.47–2.86, p = 0; HR 2.09, 95%CI 1.53–2.85, p = 0; HR 2.11, 95%CI 1.55–2.87, p = 0). APAR had a linear relationship with 28-days and 365-days mortality (P for non-linearity: 0.098 and 0.051), but a nonlinear relationship with 90-days mortality (P for non-linearity: 0.042). Subgroup analyses further revealed that higher APAR was also associated with increased long-term mortality in IS patients without hypertension, DM, cardiovascular disease, liver disease or CKD. In addition, we did not observe any interaction between subgroup variables and APAR.
Conclusion: A higher APAR index was significantly associated with increased all-cause mortality at 28-days, 90-days and 365-days after admission for critically ill patients with IS. The APAR index may help identify patients with IS at high risk of all-cause death.
Introduction
Stroke is a serious global public health issue, characterized by high incidence, high disability rates, and high mortality. Stroke is the second-leading cause of death and the third-leading cause of death and disability combined worldwide in 2019 (1). Ischemic stroke (IS) is often associated with multiple complications, such as cerebral edema, epilepsy, pneumonia, and deep vein thrombosis, which are particularly likely to occur in high-risk groups such as the elderly (2–5). Despite advancements in treatment, such as intravenous thrombolysis and mechanical thrombectomy (6, 7), the risk of poor outcomes in IS patients still remains high, especially in critically ill patients. Therefore, it is crucial to identify prognostic factors associated with adverse outcomes in patients with IS.
In the prognosis assessment of IS, traditional biomarkers and scoring systems such as NIHSS (National Institutes of Health Stroke Scale), mRS (Modified Rankin Scale), CRP (C-reactive protein) and NLR (neutrophil to lymphocyte ratio) have been widely studied and applied (8–10). In recent years, however, novel biomarkers based on routine calculations of peripheral blood, such as alkaline phosphatase (ALP) and serum albumin (ALB), have also shown potential prognostic value. ALP plays an important role in regulating inflammatory processes and mineral metabolism, and may promote atherosclerosis by enhancing vascular calcification (11). Previous studies have found that ALP levels are associated with the severity and prognosis of stroke patients (12, 13). In addition, as an essential protein with anti-inflammatory, antioxidant and antithrombotic properties, ALB levels are also closely related to cardiovascular disease and stroke prognosis (14, 15).
In recent years, the ratio of ALP to ALB (APAR), as a novel indicator of inflammation and nutritional status, has been shown to be a prognostic biomarker for a variety of diseases, such as cancer and coronary heart disease (16, 17). Compared with ALP or ALB alone, APAR provides a more complete picture of the inflammatory and nutritional status of the body, which may provide better prognostic value. For example, in patients with chronic kidney disease (CKD), APAR has been shown to be independently associated with all-cause mortality and has potential value in predicting cardiovascular events (18). In addition, APAR also showed predictive power for adverse outcomes in patients with coronary heart disease (17). However, there are few studies on the relationship between APAR and IS prognosis. Given the critical role of ALP and ALB in atherosclerosis and vascular disease, APAR, as an indicator of the integration of the two information, may be associated with adverse outcomes of IS through inflammation, oxidative stress, and nutritional status. Therefore, this study aimed to investigate the association between APAR and all-cause mortality in patients with severe IS to further evaluate its potential value as a prognostic biomarker.
Methods
Study population
This study investigated health-related data obtained from the Medical Information Mart for Intensive Care IV (MIMIC-IV) version 3.0 database, which is a large database developed and managed by MIT Computational Physiology Laboratory and consists of extensive medical records of patients admitted to the intensive care unit of Beth Israel Deaconess Medical Center. Data extraction was conducted by one of the authors, Tao Zheng, who met the requirements for accessing the database (ID: 63484863). Based on the International Classification of Diseases, Ninth and Tenth Revision, this study included patients aged 18 years and older diagnosed with ischemic stroke (19). Specifically, the diagnosis of acute ischemic stroke was defined by the ICD-9 codes 43,301, 43,311, 43,321, 43,331, 43,381, 43,391, 43,401, 43,411, and 43,491, as well as the ICD-10 code I63 (20). Exclusion criteria were as follows: (1) patients who stayed in the ICU for less than 24 h; (2) patients who were admitted to the ICU multiple times due to ischemic stroke, with only their first admission data being considered; (3) patients lacking demographic data; (4) patients without sufficient data at admission (including alkaline phosphatase and albumin); (5) patients with abnormal data. Finally, a total of 1,690 patients were enrolled in this study and grouped into four groups based on the quartiles of the APAR index (Figure 1).
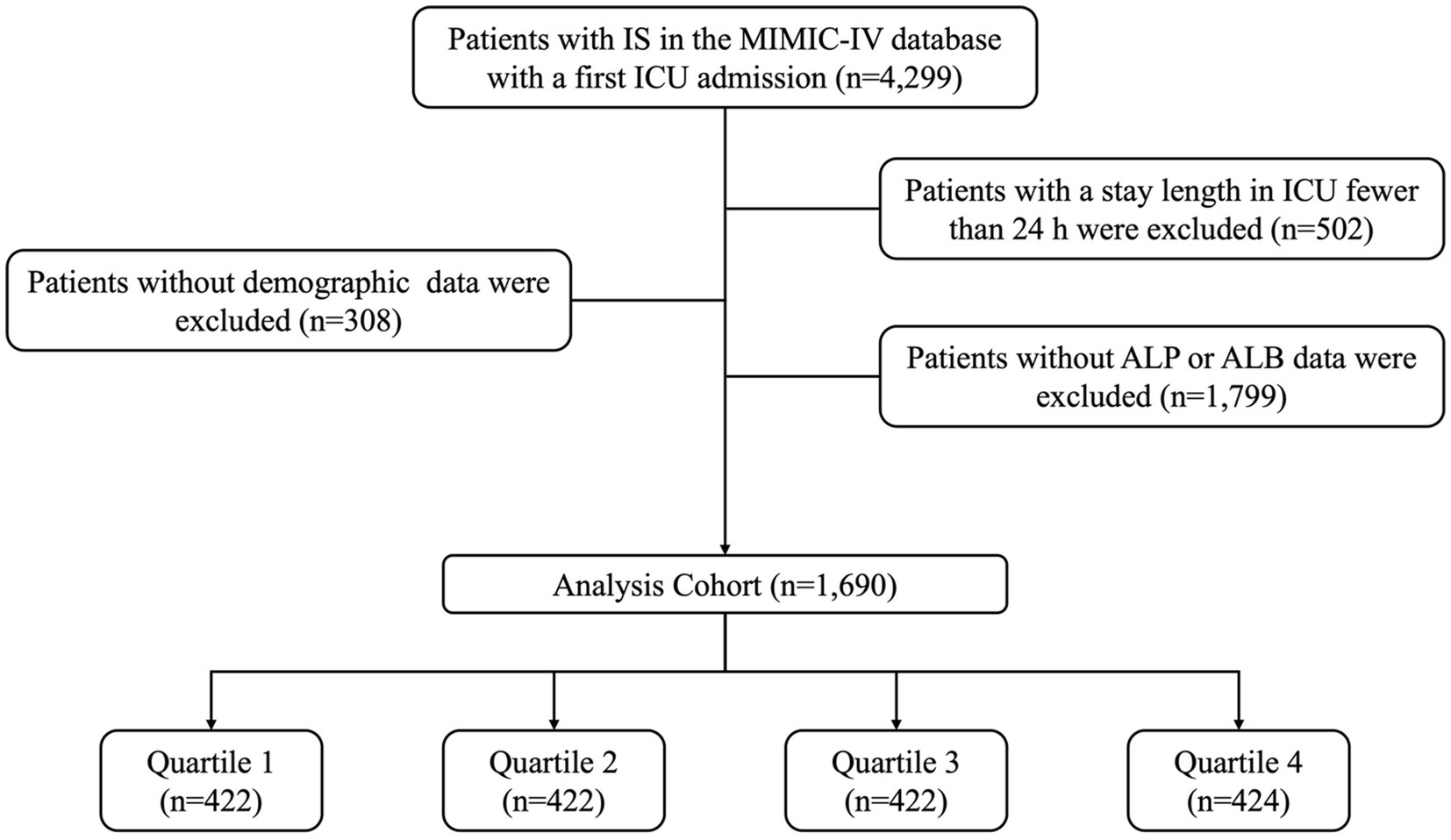
Figure 1. A flowchart illustrating the participant recruitment process. ALB, albumin; ALP, alkaline phosphatase; ICU, intensive care unit; IS, ischemic stroke. MIMIC-IV, medical information mart for intensive care IV.
Data collection
The software PostgreSQL (version 13.7.2) alongside Navicat Premium (version 16) was used to extract information with the execution of a Structured Query Language (SQL). The extraction of potential variables could be divided into six principal domains: (1) Demographic characteristics: age and gender; (2) Vital signs: heart rate (HR), systolic blood pressure (SBP), and blood oxygen saturation measured by pulse oxygen saturation (SpO2); (3) Laboratory test results: red blood cell count (RBC), white blood cell count (WBC), hemoglobin (HGB), platelet count (PLT), TC, HDL-C, glucose, serum creatinine (Scr), and serum sodium (Na); (4) Comorbidities: hypertension, diabetes mellitus (DM), hyperlipidemia, cardiovascular disease (CVD), myocardial infarction (MI), heart failure, hepatitis, liver cirrhosis, cancer, and CKD; (5) Clinical severity of illness scores: Sequential Organ Failure Assessment score (SOFA), Acute Physiology Score III (APSIII), Glasgow Coma Scale (GCS), and Charlson comorbidity Index (CCI); (6) Treatment: statins, antihypertensive drugs and mechanical ventilation (MV).
Follow-up began on the date of admission and ended on the date of death. According to previous studies, APAR is calculated by APAR: log10 (ALP/ALB). In addition, we stratified the APAR values of the participants according to the quartile method. To eliminate the missing data influence, covariates with more than 20% missing value were excluded from the analysis. For covariates with less than 20% missing data, we use multiple interpolation techniques to fill in these missing values according to previous research methods (19, 21).
Clinical outcomes
The primary endpoint of this study was 365-days all-cause mortality after admission. The secondary endpoints included all-cause mortality in 28-days and 90-days all-cause mortality after admission. Survival time was identified by the date of death in the MIMIC-IV database.
Statistical analysis
Baseline patients’ characteristics were stratified based on APAR values. Continuous variables were expressed as mean ± standard deviation (SD), while classification variables were expressed as frequency (percentage). The normally distributed continuous variables were analyzed by independent sample t-test or analysis of variance (ANOVA). Continuous variables not normally distributed were analyzed by Kruskal-Wallis test. Pearson’s Chi-square test or Fisher exact test was used to compare categorical variables.
Moreover, Kaplan–Meier survival method was used to ascertain the endpoint incidence within groups defined by APAR index. We excluded variables with variance inflation factor (VIF) ≥ 5. The Cox proportional hazards model was used to calculate the hazard ratio (HR) and 95% confidence interval (CI) between APAR index and endpoints, and also adjusted for confounding factors in three models: Model 1 (unadjusted), Model 2 (adjusted for age and sex), and Model 3 (adjusted for age, sex, HR, SBP, SpO2, WBC, RBC, HGB, PLT, Na, glucose, Scr, hypertension, DM, hyperlipidemia, CVD, MI, heart failure, hepatitis, liver cirrhosis, cancer, CKD, statins, antihypertensive drugs, GCS, MV, SOFA, APS III, and CCI). The p values for trends were calculated using the quartile level. The APAR index was entered into the models as a continuous variable or a categorical variable (the first quartile of APAR index was taken as the reference group). We also performed a restricted cubic spline regression model with four knots to investigate potential nonlinear relationships between APAR index levels and all-cause mortality. All statistical analyses were performed using R statistical package (R version 4.3). All reported p values were two-sided, and p < 0.05 were considered statistically significant.
Results
Baseline characteristics
Our study ultimately included 1,690 critically ill patients with IS, who were divided into four groups based on the APAR index quartile (Q1: 0.449–1.243; Q2: 1.243–1.36; Q3: 1.361–1.524; Q4: 1.524–2.794) (Table 1). The mean age of the patients was 68.69 ± 15.7 years, and there were 862 (51.01%) male patients. Critically ill patients with IS in the high APAR group exhibited faster HR, lower SBP, higher WBC and ALP levels, lower RBC, HGB, and ALB levels, and higher blood glucose and Scr levels than those in the low APAR group. In addition, they were more likely to have DM, CVD, MI, heart failure, CKD, and cancer, and were more likely to receive MV and antihypertensive treatment, and were less likely to receive statins.
Clinical outcomes
The Kaplan–Meier survival analysis curves were employed to analyze incidence of primary and secondary outcomes among groups based on APAR quartiles. Patients with APAR ranged from 0.449 to 1.243 (Q1) had a lowest risk of death in 28-days, at 90-days and 365-days after admission (A: log-rank p < 0.0001; B: log-rank p < 0.0001; C: log-rank p < 0.0001) (Figure 2).
Cox proportional risk analysis was used to analyze the association between APAR and mortality. When the APAR was a continuous variable, the findings indicated that APAR was a significant predictor of mortality in 28-days in the three models (Model 1: HR 5.37, 95% CI 3.48–8.31, p = 0; Model 2: HR 5.38, 95%CI 3.48–8.36, p = 0; Model 3: HR 2.39, 95% CI 1.44–3.97, p < 0.001), 90-days mortality in the three models (Model 1: HR 7.01, 95% CI 4.58–10.83, p = 0; Model 2: HR 7.04, 95%CI 4.58–10.9, p = 0; Model 3: HR 2.92, 95% CI 1.78–4.8, p = 0), and 365-days in the three models (Model 1: HR 7.78, 95% CI 5.07–12.04, p = 0; Model 2: HR 7.81, 95% CI 5.08–12.12, p = 0; Model 3: HR 3.25, 95%CI 1.99–5.33, p = 0). When APAR was a nominal variable, patients in the Quartile 4 had a significantly increased risk of death in 28-days (HR 2.05, 95%CI 1.47–2.86, p = 0), 90-days (HR 2.09, 95%CI 1.53–2.85, p = 0), and 365-days (HR 2.11, 95%CI 1.55–2.87, p = 0) mortality in the fully adjusted model 3 (Table 2).
Furthermore, we observed the APAR had a linear relationship with 28-days and 365-days mortality (P for non-linearity: 0.098, P for non-linearity: 0.051, respectively), but a nonlinear relationship with 90-days mortality (P for non-linearity: 0.042) (Figure 3).
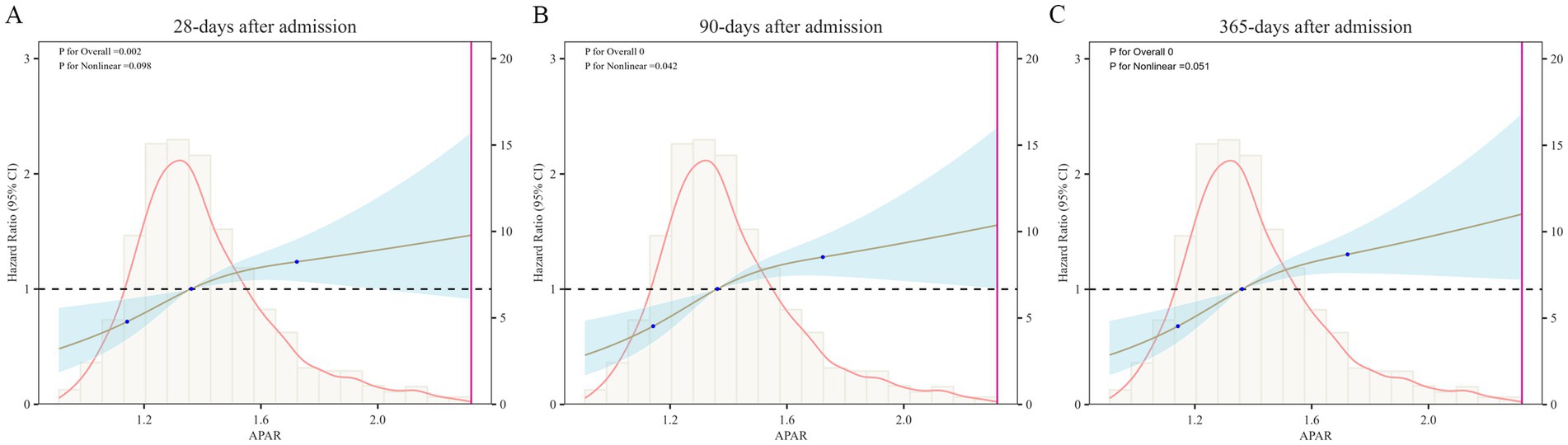
Figure 3. Restricted cubic spline regression analysis for all-cause mortality. The heavy central lines represent the estimated adjusted hazard ratios, with blue bands denoting 95% confidence intervals. APAR, alkaline phosphatase to albumin ratio.
Subgroup analysis
The prognostic value of APAR index for all-cause mortality was further analyzed across various subgroups, including age, sex, hypertension, DM, hyperlipidemia, heart failure, MI, CVD, hepatitis, liver cirrhosis, CKD, cancer, statins and GCS grades. The turning points identified by threshold effect analysis were 1.7404 in 28-days, 1.8472 in 90-days and 1.7404 in 365-days after admission. The APAR index was significantly associated with higher risk of death in female (HR 1.71, 95%CI 1.06–2.78, p = 0.029) at 365-days after admission. Higher APAR was also associated with increased 365-days after admission mortality in IS patients without hypertension, DM, cardiovascular disease, liver disease, or CKD (Figure 4). For example, it was significantly associated with higher risk of death in non-hypertension group (HR 1.52, 95%CI 1.09–2.11, p = 0.013), non-DM group (HR 1.72, 95%CI 1.2–2.46, p = 0.003), non-CVD group (HR 1.56, 95%CI 1.08–2.26, p = 0.017) at 365-days after admission. In addition, we did not observe any interaction between subgroup variables and APAR.
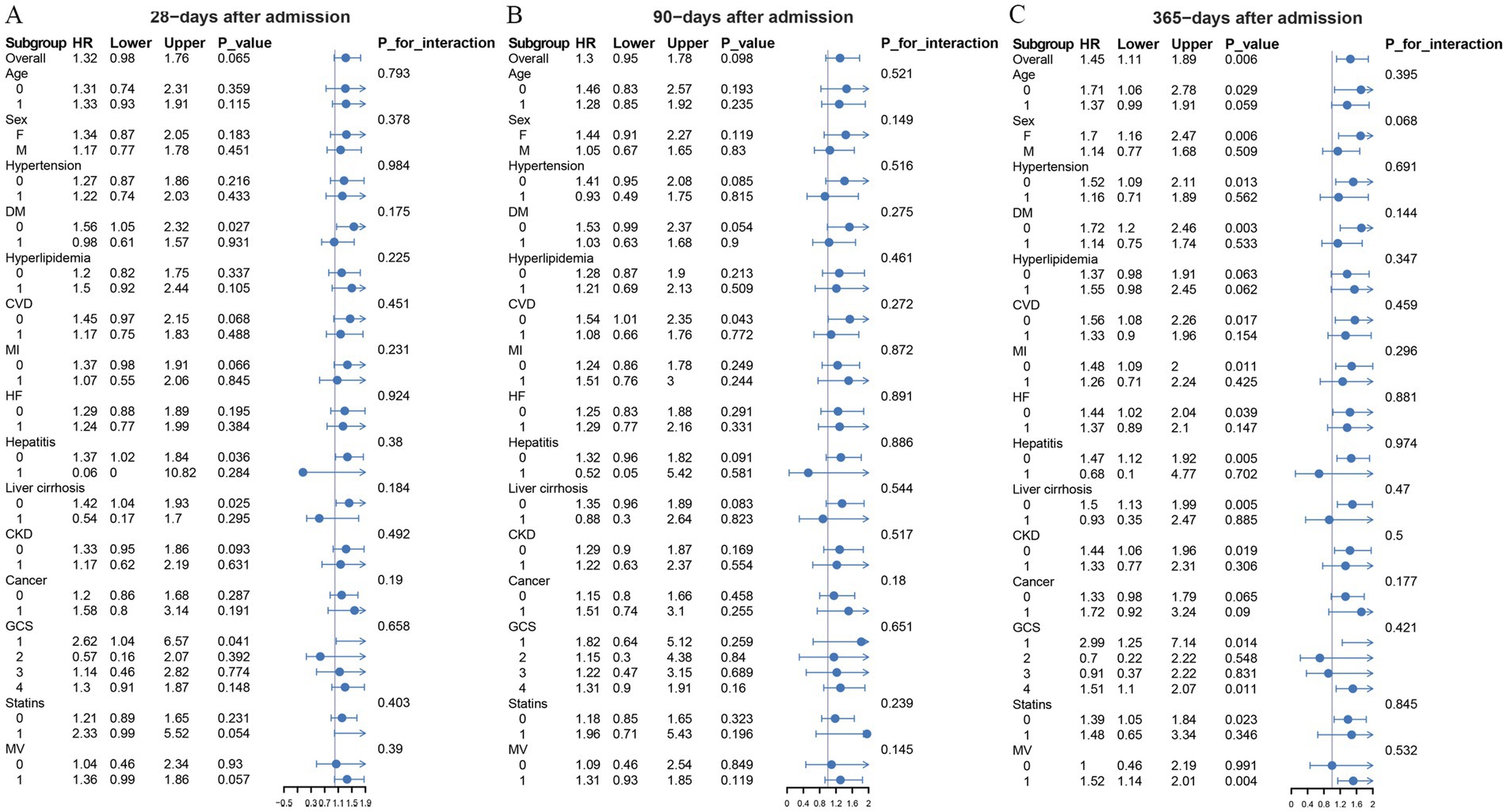
Figure 4. Forest plots of hazard ratios for mortality in different subgroups. GCS grades: grade 1, 3–5 scores; grade 2, 6–8 scores; grade 3 9–12 scores; grade 4, 13–15 scores. CI, confidence interval; HR, hazard ratio; DM, diabetes mellitus; CVD, cardiovascular disease; MI, myocardial infarction; HF, heart failure; CKD, chronic kidney disease; GCS, Glasgow coma scale; MV, mechanical ventilation.
Discussion
This study is the first to evaluate the role of APAR index in predicting short-and long-term outcomes in critically ill patients with IS. The results showed that, after controlling for potential confounding factors, higher APAR was significantly associated with increased all-cause mortality at 28-days, 90-days, and 365-days after admission. APAR had a linear relationship with 28-days and 365-days mortality (P for non-linearity: 0.098, P for non-linearity: 0.051, respectively), but a nonlinear relationship with 90-days mortality (P for non-linearity = 0.042). Subgroup analyses further revealed that higher APAR was also associated with increased long-term mortality in IS patients without hypertension, DM, cardiovascular disease, liver disease, or CKD. In addition, we did not observe any interaction between subgroup variables and APAR. Therefore, APAR has the potential to be a useful tool for clinicians in the decision-making process and may be recognized as an independent risk factor for critically ill patients with IS.
APAR is a biomarker that combines ALP and ALB, which has been gradually paid attention in medical research in recent years, especially in the prognostic evaluation of some diseases. Abnormal serum ALP levels are associated with a variety of neurological diseases, such as small cerebral vascular disease, Alzheimer’s disease, traumatic brain injury and so on. One study found that after adjusting for cardiovascular risk factors, subjects with ALP ≥ 195 IU/L had a significantly increased risk of asymptomatic lacunar infarction (SLI) and moderate to severe white matter hypersignaling (MS-cWMH) compared with ALP ≤ 155 IU/L (22). Vardy et al. found that ALP was elevated in both brain and plasma in Alzheimer’s patients and negatively correlated with cognitive function (23). In addition, serum ALP is also significantly increased in patients with traumatic brain injury (24). Many studies have found that ALP is closely related to stroke, and it has been considered as a potential diagnostic and prognostic indicator of stroke. Studies have shown that higher serum ALP levels, even if it is in the normal range, are significantly associated with a higher risk of first stroke in Chinese adults with hypertension (25). A Japanese study also found that for non-drinkers, higher ALP levels were associated with an increased risk of ischemic stroke in men and hemorrhagic stroke in women (26). In addition, the results of a meta-analysis in conjunction with the Mendelian Randomization study also confirmed that a higher ALP significantly increased the risk of stroke (27). ALP is not only associated with the risk of stroke, but also with the prognosis after stroke. A higher ALP has been found to significantly increase the 3-month all-cause mortality in patients with acute stroke (28). It has also been found that higher ALP significantly increases in-hospital mortality in patients with acute ischemic stroke (29). ALB is the most abundant circulating protein in blood, which is protective in several diseases, including CHD, heart failure, hypertension, atrial fibrillation, and peripheral artery disease (14). Some observational studies and meta-analyses suggested that hypoalbuminemia was a strong prognosticator of increased all-cause and cardiovascular mortality (30–32). In several studies, ALB was involved as a key factor for stroke risk and prognosis. ALB may serve as a correction for certain indicators to better study the relationship between these indicators and stroke. For example, numerous studies have shown that ratios such as neutrophil-to-albumin ratio (33), fibrinogen-to-albumin ratio (34), C-reactive protein to albumin ratio (35, 36) and High serum lactate dehydrogenase to albumin ratio (37) are closely related to stroke. Some studies showed that low ALB levels are associated with poor prognosis in stroke, including all-cause death, heart failure, atrial fibrillation, ventricular arrhythmias, and myocardial infarction (38).
In Model 3, APAR was positively associated with short-and long-term mortality in patients with IS, even after we included 29 covariates in our analysis, including adjustments for biochemical markers and comorbidities. In addition, subgroup analysis showed that no significant interaction was found between any particular treatment modality or comorbidities and APAR. Therefore, APAR may be a relatively independent prognostic indicator with a certain universality in predicting mortality in patients with ischemic stroke. The prognostic value of APAR in ischemic stroke may reflect unique pathophysiological mechanisms. The increase of ALP level may be closely related to the breakdown of blood–brain barrier (BBB) after IS. Studies have shown that ALP is highly expressed in cerebrovascular endothelial cells and plays an important role in the permeability, maintenance and integrity of the BBB and the transport of proteins through the barrier (39, 40). Increased ALP levels may reflect endothelial dysfunction and increased blood–brain barrier permeability, leading to increased cerebral edema and neurological deterioration. After IS, the inflammatory response of the body is activated rapidly, and the levels of inflammatory factors such as TNF-α and IL-6 are significantly increased (41). ALP may act as a regulator of inflammatory mediators during inflammation, and its elevated level may reflect the excessive neuroinflammatory response after stroke. At the same time, low albumin levels may impair its antioxidant and anti-inflammatory effects, further exacerbating neuroinflammatory damage (42). Oxidative stress plays a key role in secondary brain injury after IS. Elevated ALP levels may be associated with increased production of reactive oxygen species (43), while low albumin levels impair its ability to scavenge free radicals. This imbalance may lead to increased neuronal damage and affect the prognosis of patients. In addition, low albumin levels may reflect the acute phase reaction and worsening nutritional status after IS. Stroke-related dysphagia, metabolic alterations, and increased catabolism can lead to protein-energy malnutrition, which not only affects nerve repair, but may also increase the risk of complications. For example, ALB is inversely associated with hemorrhagic conversion in patients with acute ischemic stroke (44). However, the underlying biological mechanism of increased APAR levels and poor prognosis in patients with IS remains to be explored.
To further evaluate the prognostic value of APAR in ischemic stroke, we performed subgroup analyses that included coronary heart disease, myocardial infarction, heart failure, hepatitis, liver cirrhosis, and cancer. In previous studies, APAR has been confirmed to be closely related to the occurrence and progression of coronary heart disease, and is considered as a potential biomarker. However, in the subgroup analysis of this study, we found that the association between APAR and mortality risk has specific population characteristics. Specifically, only in patients without coronary heart disease, those with higher APAR levels had a significantly increased risk of death at 90 and 365 days after admission, while this association was not seen in patients with coronary heart disease. In addition, we did not observe a significant interaction between APAR and coronary heart disease. We also observed this phenomenon in patients with no prior myocardial infarction or heart failure at 365 days after admission. ALP is a marker of vascular calcification, which promotes the process of vascular calcification through several mechanisms, including catalyzing pyrophosphate hydrolysis, promoting vascular smooth muscle cell calcification, and participating in inflammation (45–47). Moreover, low ALB levels can promote pulmonary edema and fluid retention, leading to heart failure (48). In patients without vascular disease, a higher APAR may imply a significantly increased risk of cardiovascular disease in these patients, resulting in increased mortality.
As both ALP and ALB are key liver function indicators, we specifically examined the effect of APAR on IS patients with hepatitis or cirrhosis in our subgroup analysis. However, the results showed that a higher APAR was associated with significantly increased mortality in patients with IS during the observation period of 28 and 365 days after admission for those without hepatitis or cirrhosis. In patients without liver disease, the synthetic function of the liver is relatively normal, and the elevated level of ALP can better reflect the activation of systemic inflammatory response, while the level of ALB can better reflect the overall nutritional and metabolic status of the body. Higher APAR may indicate that the body lacks adequate anti-inflammatory and repair capacity after IS, thus increasing the risk of death. In addition, our subgroup analysis did not find an association between higher APAR and an increased risk of death in IS patients with co-existing hepatitis or cirrhosis. Moreover, in a subgroup analysis of cancer patients, we did not find a significant association between higher APAR and an increased risk of death in ischemic stroke patients with cancer. During long-term follow-up, we observed that higher APAR was associated with significantly increased mortality in ischemic stroke patients without hypertension, diabetes, or CKD. ALP can lead to increased vascular stiffness and decreased vascular compliance. A higher APAR significantly increases the risk of high blood pressure (49). It has also been found that higher serum ALP is significantly associated with an increased risk of new-onset diabetes (50). And higher ALP is also closely related to CKD and uremia (11, 45). In addition, although no significant association between APAR and risk of death was found in IS patients with hepatitis or cirrhosis and in subgroups of cancer patients, higher APAR was associated with a significantly increased risk of death in specific high-risk patient groups (e.g., GCS grade 1, non-statin users, and patients receiving mechanical ventilation). This suggests that APAR may have potential applications in identifying high-risk patients.
Although this study revealed the APAR index as an important independent predictor of all-cause mortality in patients with severe IS after adjusting for multiple confounders, some limitations remain. First, retrospective study design limits the certainty of causation and makes it difficult to establish a direct causal link between APAR and mortality. Second, despite our efforts to adjust for known confounders, given limitations in the study design, there may still be confounders that are not identified or are not adequately adjusted for, potentially affecting clinical outcomes. In addition, although we included a large amount of patient data, the integrity and accuracy of the data may be affected by the original data collection standards and methods. Finally, due to the lack of external validation of independent cohorts, the generalisability and robustness of the findings need to be further confirmed.
Conclusion
A higher APAR index was significantly associated with increased all-cause mortality at 28, 90, and 365 days after admission for critically ill patients with IS. The APAR index may help identify patients with IS at high risk of all-cause death.
Data availability statement
The datasets presented in this study can be found in online repositories. The names of the repository/repositories and accession number(s) can be found in the article/supplementary material.
Ethics statement
Ethical review and approval was not required for the study on human participants in accordance with the local legislation and institutional requirements. Written informed consent from the patients/participants or patients/participants’ legal guardian/next of kin was not required to participate in this study in accordance with the national legislation and the institutional requirements.
Author contributions
TZ: Formal analysis, Methodology, Software, Writing – original draft, Writing – review & editing. MG: Data curation, Software, Writing – original draft, Writing – review & editing. YH: Writing – original draft, Writing – review & editing. GL: Writing – review & editing. XW: Writing – review & editing. SL: Writing – review & editing. YG: Writing – review & editing. WT: Validation, Visualization, Writing – review & editing. ZL: Conceptualization, Funding acquisition, Validation, Writing – review & editing.
Funding
The author(s) declare that financial support was received for the research and/or publication of this article. This study was supported by the China-Japan Friendship Hospital Scientific Research Foundation (No: 2010-QN-08), National Natural Science Foundation of China (No: 52073310), and Peking University People’s Hospital Talent Introduction Scientific Research Launch Fund (2022-T-02).
Acknowledgments
We are grateful to the MIMIC-IV database for supplying data on summary statistics for analyses. We are also grateful to all the researchers who shared these data and the study participants.
Conflict of interest
The authors declare that the research was conducted in the absence of any commercial or financial relationships that could be construed as a potential conflict of interest.
Generative AI statement
The authors declare that no Gen AI was used in the creation of this manuscript.
Publisher’s note
All claims expressed in this article are solely those of the authors and do not necessarily represent those of their affiliated organizations, or those of the publisher, the editors and the reviewers. Any product that may be evaluated in this article, or claim that may be made by its manufacturer, is not guaranteed or endorsed by the publisher.
References
1. GBD 2019 Stroke Collaborators. Global, regional, and national burden of stroke and its risk factors, 1990-2019: a systematic analysis for the global burden of disease study 2019. Lancet Neurol. (2021) 20:795–820. doi: 10.1016/S1474-4422(21)00252-0
2. Liebeskind, DS, Jüttler, E, Shapovalov, Y, Yegin, A, Landen, J, and Jauch, EC. Cerebral edema associated with large hemispheric infarction. Stroke. (2019) 50:2619–25. doi: 10.1161/STROKEAHA.118.024766
3. Bladin, CF, Alexandrov, AV, Bellavance, A, Bornstein, N, Chambers, B, Coté, R, et al. Seizures after stroke: a prospective multicenter study. Arch Neurol. (2000) 57:1617–22. doi: 10.1001/archneur.57.11.1617
4. Banda, KJ, Chu, H, Kang, XL, Liu, D, Pien, LC, Jen, HJ, et al. Prevalence of dysphagia and risk of pneumonia and mortality in acute stroke patients: a meta-analysis. BMC Geriatr. (2022) 22:420. doi: 10.1186/s12877-022-02960-5
5. Rinde, LB, Småbrekke, B, Mathiesen, EB, Løchen, ML, Njølstad, I, Hald, EM, et al. Ischemic stroke and risk of venous thromboembolism in the general population: the Tromsø study. J Am Heart Assoc. (2016) 5:e004311. Published 2016 Nov 7. doi: 10.1161/JAHA.116.004311
6. Tsivgoulis, G, Katsanos, AH, Sandset, EC, Turc, G, Nguyen, TN, Bivard, A, et al. Thrombolysis for acute ischaemic stroke: current status and future perspectives. Lancet Neurol. (2023) 22:418–29. doi: 10.1016/S1474-4422(22)00519-1
7. Sweid, A, Hammoud, B, Ramesh, S, Wong, D, Alexander, TD, Weinberg, JH, et al. Acute ischaemic stroke interventions: large vessel occlusion and beyond. Stroke Vasc Neurol. (2019) 5:80–5. doi: 10.1136/svn-2019-000262
8. Du, J, Wang, Y, Che, B, Miao, M, Bao, A, Peng, Y, et al. The relationship between neurological function trajectory, assessed by repeated NIHSS measurement, and long-term cardiovascular events, recurrent stroke, and mortality after ischemic stroke. Int J Stroke. (2023) 18:1005–14. doi: 10.1177/17474930231180446
9. Chye, A, Hackett, ML, Hankey, GJ, Lundström, E, Almeida, OP, Gommans, J, et al. Repeated measures of modified Rankin scale scores to assess functional recovery from stroke: AFFINITY study findings. J Am Heart Assoc. (2022) 11:e025425. doi: 10.1161/JAHA.121.025425
10. Liu, F, Yang, P, Wang, Y, Shi, M, Wang, R, Xu, Q, et al. HS-CRP modifies the prognostic value of platelet count for clinical outcomes after ischemic stroke. J Am Heart Assoc. (2023) 12:e030007. doi: 10.1161/JAHA.123.030007
11. Schoppet, M, and Shanahan, CM. Role for alkaline phosphatase as an inducer of vascular calcification in renal failure? Kidney Int. (2008) 73:989–91. doi: 10.1038/ki.2008.104
12. Wang, Z, Li, J, Jing, J, Zhang, Z, Xu, Q, Liu, T, et al. Impact of alkaline phosphatase on clinical outcomes in patients with ischemic stroke: a nationwide registry analysis. Front Neurol. (2024) 15:1336069. Published 2024 Feb 14. doi: 10.3389/fneur.2024.1336069
13. Wu, G, Li, S, Luo, M, Li, X, Zhu, X, Zhang, J, et al. Role of serum alkaline phosphatase as a potential biomarker of severity and prognosis in intracerebral hemorrhage. J Stroke Cerebrovasc Dis. (2024) 33:107478. doi: 10.1016/j.jstrokecerebrovasdis.2023.107478
14. Manolis, AA, Manolis, TA, Melita, H, Mikhailidis, DP, and Manolis, AS. Low serum albumin: a neglected predictor in patients with cardiovascular disease. Eur J Intern Med. (2022) 102:24–39. doi: 10.1016/j.ejim.2022.05.004
15. Thuemmler, RJ, Pana, TA, Carter, B, Mahmood, R, Bettencourt-Silva, JH, Metcalf, AK, et al. Serum albumin and post-stroke outcomes: analysis of UK regional registry data, systematic review, and Meta-analysis. Nutrients. (2024) 16:1486. Published 2024 May 14. doi: 10.3390/nu16101486
16. An, L, Yin, WT, and Sun, DW. Albumin-to-alkaline phosphatase ratio as a promising indicator of prognosis in human cancers: is it possible? BMC Cancer. (2021) 21:247. doi: 10.1186/s12885-021-07921-6
17. Dai, XY, Zheng, YY, Tang, JN, Wang, W, Guo, QQ, Yin, SS, et al. Alkaline phosphatase-to-albumin ratio as a novel predictor of long-term adverse outcomes in coronary artery disease patients who underwent PCI. Biosci Rep. (2021) 41:BSR20203904. doi: 10.1042/BSR20203904
18. Xue, X, Li, JX, Wang, JW, Lin, LM, Cheng, H, Deng, DF, et al. Association between alkaline phosphatase/albumin ratio and the prognosis in patients with chronic kidney disease stages 1-4: results from a C-STRIDE prospective cohort study. Front Med. (2023) 10:1215318. doi: 10.3389/fmed.2023.1215318
19. Cai, W, Xu, J, Wu, X, Chen, Z, Zeng, L, Song, X, et al. Association between triglyceride-glucose index and all-cause mortality in critically ill patients with ischemic stroke: analysis of the MIMIC-IV database. Cardiovasc Diabetol. (2023) 22:138. doi: 10.1186/s12933-023-01864-x
20. Li, B, Li, J, Meng, X, Yang, S, Tian, F, Song, X, et al. The association of blood urea nitrogen-to-creatinine ratio and in-hospital mortality in acute ischemic stroke patients with atrial fibrillation: data from the MIMIC-IV database. Front Neurol. (2024) 15:1331626. doi: 10.3389/fneur.2024.1331626
21. Zheng, T, Jiang, L, Li, G, Zeng, N, Yu, B, Duan, S, et al. Association between human herpes simplex virus and severe headache or migraine among aged 20-49 years: a cross-sectional study. Front Neurol. (2024) 15:1476863. doi: 10.3389/fneur.2024.1476863
22. Lee, HB, Kim, J, Kim, SH, Kim, S, Kim, OJ, and Oh, SH. Association between serum alkaline phosphatase level and cerebral small vessel disease. PLoS One. (2015) 10:e0143355. doi: 10.1371/journal.pone.0143355
23. Vardy, ER, Kellett, KA, Cocklin, SL, and Hooper, NM. Alkaline phosphatase is increased in both brain and plasma in Alzheimer's disease. Neurodegener Dis. (2012) 9:31–7. doi: 10.1159/000329722
24. Cadosch, D, Toffoli, AM, Gautschi, OP, Frey, SP, Zellweger, R, Skirving, AP, et al. Serum after traumatic brain injury increases proliferation and supports expression of osteoblast markers in muscle cells. J Bone Joint Surg Am. (2010) 92:645–53. doi: 10.2106/JBJS.I.00097
25. Zhang, Y, Li, H, Xie, D, Li, J, Zhang, Y, Wang, B, et al. Positive association between serum alkaline phosphatase and first stroke in hypertensive adults. Front Cardiovasc Med. (2021) 8:749196. Published 2021. doi: 10.3389/fcvm.2021.749196
26. Shimizu, Y, Imano, H, Ohira, T, Kitamura, A, Kiyama, M, Okada, T, et al. Alkaline phosphatase and risk of stroke among Japanese: the circulatory risk in communities study (CIRCS). J Stroke Cerebrovasc Dis. (2013) 22:1046–55. doi: 10.1016/j.jstrokecerebrovasdis.2012.06.009
27. Li, C, Gu, L, Shi, FY, Xiong, SY, Wu, GS, Peng, JH, et al. Serum liver enzymes and risk of stroke: systematic review with meta-analyses and Mendelian randomization studies. Eur J Neurol. (2024) 31:e16506. doi: 10.1111/ene.16506
28. Guo, W, Liu, Z, Lu, Q, Liu, P, Lin, X, Wang, J, et al. Non-linear association between serum alkaline phosphatase and 3-month outcomes in patients with acute stroke: results from the Xi'an stroke registry study of China. Front Neurol. (2022) 13:859258. doi: 10.3389/fneur.2022.859258
29. Zhong, C, You, S, Chen, J, Zhai, G, du, H, Luo, Y, et al. Serum alkaline phosphatase, phosphate, and in-hospital mortality in acute ischemic stroke patients. J Stroke Cerebrovasc Dis. (2018) 27:257–66. doi: 10.1016/j.jstrokecerebrovasdis.2017.08.041
30. Shannon, CM, Ballew, SH, Daya, N, Zhou, L, Chang, AR, Sang, Y, et al. Serum albumin and risks of hospitalization and death: findings from the atherosclerosis risk in communities study. J Am Geriatr Soc. (2021) 69:2865–76. doi: 10.1111/jgs.17313
31. Akirov, A, Masri-Iraqi, H, Atamna, A, and Shimon, I. Low albumin levels are associated with mortality risk in hospitalized patients. Am J Med. (2020) 133:646. doi: 10.1016/j.amjmed.2020.02.001
32. Grimm, G, Haslacher, H, Kampitsch, T, Endler, G, Marsik, C, Schickbauer, T, et al. Sex differences in the association between albumin and all-cause and vascular mortality. Eur J Clin Investig. (2009) 39:860–5. doi: 10.1111/j.1365-2362.2009.02189.x
33. Lv, XN, Shen, YQ, Li, ZQ, Deng, L, Wang, ZJ, Cheng, J, et al. Neutrophil percentage to albumin ratio is associated with stroke-associated pneumonia and poor outcome in patients with spontaneous intracerebral hemorrhage. Front Immunol. (2023) 14:1173718. doi: 10.3389/fimmu.2023.1173718
34. Sun, S, Cheng, Y, Li, L, Zhu, H, Liu, C, and Cao, Y. A high fibrinogen-to-albumin ratio on admission is associated with early neurological deterioration following intravenous thrombolysis in patients with acute ischemic stroke. J Inflamm Res. (2024) 17:4151–61. doi: 10.2147/JIR.S459161
35. Yuan, J, Cheng, Y, Han, X, Zhu, N, Ma, W, Li, J, et al. Association between C-reactive protein/albumin ratio and all-cause mortality in patients with stroke: evidence from NHANES cohort study. Nutr Metab Cardiovasc Dis. (2024) 34:2305–14. doi: 10.1016/j.numecd.2024.05.024
36. Yu, D, Guo, G, Wan, F, and Hu, B. The association between C-reactive protein to albumin ratio and adverse outcomes in acute ischemic stroke patients: a study in the Korean population. Heliyon. (2024) 10:e39212. doi: 10.1016/j.heliyon.2024.e39212
37. Chu, M, Niu, H, Yang, N, Wang, D, Liu, Y, Mao, X, et al. High serum lactate dehydrogenase to albumin ratio is associated with increased risk of poor prognosis after ischemic stroke. Clin Neurol Neurosurg. (2024) 237:108120. doi: 10.1016/j.clineuro.2024.108120
38. Zhou, H, Wang, A, Meng, X, Lin, J, Jiang, Y, Jing, J, et al. Low serum albumin levels predict poor outcome in patients with acute ischaemic stroke or transient ischaemic attack. Stroke Vasc Neurol. (2021) 6:458–66. doi: 10.1136/svn-2020-000676
39. Nwafor, DC, Brichacek, AL, Ali, A, and Brown, CM. Tissue-nonspecific alkaline phosphatase in central nervous system health and disease: a focus on brain microvascular endothelial cells. Int J Mol Sci. (2021) 22:5257. doi: 10.3390/ijms22105257
40. Ahmadabad, MA, Naeimi, A, Keymoradzadeh, A, Faghani, S, Ahmadabad, MA, Boroujeni, NA, et al. Evaluation of De Ritis (AST/ALT), ALP/ALT, and AST/ALP ratios as prognostic factors in patients with acute ischemic stroke. BMC Neurol. (2022) 22:450. doi: 10.1186/s12883-022-02989-4
41. DeLong, JH, Ohashi, SN, O'Connor, KC, and Sansing, LH. Inflammatory responses after ischemic stroke. Semin Immunopathol. (2022) 44:625–48. doi: 10.1007/s00281-022-00943-7
42. Pompili, E, Zaccherini, G, Baldassarre, M, Iannone, G, and Caraceni, P. Albumin administration in internal medicine: a journey between effectiveness and futility. Eur J Intern Med. (2023) 117:28–37. doi: 10.1016/j.ejim.2023.07.003
43. Li, H, Zhao, Y, Li, W, Yang, J, and Wu, H. Critical role of neutrophil alkaline phosphatase in the antimicrobial function of neutrophils. Life Sci. (2016) 157:152–7. doi: 10.1016/j.lfs.2016.06.005
44. Wang, C, Deng, L, Qiu, S, Bian, H, Wang, L, Li, Y, et al. Serum albumin is negatively associated with hemorrhagic transformation in acute ischemic stroke patients. Cerebrovasc Dis. (2019) 47:88–94. doi: 10.1159/000498855
45. Lomashvili, KA, Garg, P, Narisawa, S, Millan, JL, and O'Neill, WC. Upregulation of alkaline phosphatase and pyrophosphate hydrolysis: potential mechanism for uremic vascular calcification. Kidney Int. (2008) 73:1024–30. doi: 10.1038/ki.2008.26
46. Narisawa, S, Harmey, D, Yadav, MC, O'Neill, WC, Hoylaerts, MF, and Millán, JL. Novel inhibitors of alkaline phosphatase suppress vascular smooth muscle cell calcification. J Bone Miner Res. (2007) 22:1700–10. doi: 10.1359/jbmr.070714
47. Demer, LL, and Tintut, Y. Inflammatory, metabolic, and genetic mechanisms of vascular calcification. Arterioscler Thromb Vasc Biol. (2014) 34:715–23. doi: 10.1161/ATVBAHA.113.302070
48. Arques, S. Human serum albumin in cardiovascular diseases. Eur J Intern Med. (2018) 52:8–12. doi: 10.1016/j.ejim.2018.04.014
49. Shimizu, Y, Nakazato, M, Sekita, T, Kadota, K, Yamasaki, H, Takamura, N, et al. Association between alkaline phosphatase and hypertension in a rural Japanese population: the Nagasaki Islands study. J Physiol Anthropol. (2013) 32:10. doi: 10.1186/1880-6805-32-10
50. Zhang, Y, Zhou, C, Li, J, Zhang, Y, Xie, D, Liang, M, et al. Serum alkaline phosphatase levels and the risk of new-onset diabetes in hypertensive adults. Cardiovasc Diabetol. (2020) 19:186. doi: 10.1186/s12933-020-01161-x
Glossary
HR - Heart rate
SpO2 - Blood oxygen saturation measured by pulse oxygen saturation
SBP - Systolic blood pressure
WBC - White blood cell count
RBC - Red blood cell count
HGB - Hemoglobin
PLT - Platelet count
ALB - Albumin
ALP - Alkaline phosphatase
Na - Serum sodium
Scr - Hypertension
DM - Diabetes mellitus
CVD - Cardiovascular disease
MI - Myocardial infarction
CKD - Chronic kidney disease
MV - Mechanical ventilation
APS III - Acute Physiology Score III
CCI - Charlson comorbidity Index
GCS - Glasgow coma scale
SOFA - Sequential Organ Failure Assessment score
Keywords: albumin, alkaline phosphatase, APAR, ischemic stroke, mortality, biomarker
Citation: Zheng T, Guo M, Han Y, Li G, Wang X, Li S, Gao Y, Tang W and Liu Z (2025) Association of alkaline-phosphatase/albumin ratio with all-cause mortality in critically ill patients with ischemic stroke: a retrospective study. Front. Neurol. 16:1567767. doi: 10.3389/fneur.2025.1567767
Edited by:
Mohamed F. Doheim, University of Pittsburgh Medical Center, United StatesReviewed by:
Rashi Verma, Morehouse School of Medicine, United StatesIbrahim Saleh, University of Maryland, United States
Copyright © 2025 Zheng, Guo, Han, Li, Wang, Li, Gao, Tang and Liu. This is an open-access article distributed under the terms of the Creative Commons Attribution License (CC BY). The use, distribution or reproduction in other forums is permitted, provided the original author(s) and the copyright owner(s) are credited and that the original publication in this journal is cited, in accordance with accepted academic practice. No use, distribution or reproduction is permitted which does not comply with these terms.
*Correspondence: Wenxiong Tang, dGFuZ3dlbnhpb25nQGJqbXUuZWR1LmNu; Zunjing Liu, bGl1enVuamluZ0Bwa3UuZWR1LmNu
†These authors have contributed equally to this work