- 1Department of Neurology, The Second Hospital, Cheeloo College of Medicine, Shandong University, Jinan, China
- 2Department of Pediatric Surgery, Qilu Hospital of Shandong University, Jinan, China
- 3Cheeloo College of Medicine, Shandong University, Jinan, China
- 4Department of Human Genetics, School of Medicine, Emory University, Atlanta, GA, United States
Objective: Serum klotho protein is a protein with anti-aging effects. Since the relationship between serum klotho and Stroke remains rather ambiguous, this research probed into the potential correlation between serum klotho concentration and Stroke.
Methods: This study employed a cross-sectional design and incorporated population data from the NHANES from 2007 to 2016. Weighted univariate and multivariate logistic regression models were utilized to inspect the relationship between klotho and Stroke. Stratified analyses and interaction tests were carried out to explore the latent correlation between klotho and Stroke. Finally, a fitted smooth curve was adopted to depict the non-linear relationship.
Results: In this study, after excluding all the missing data, a total of 12,414 participants were encompassed, including 450 Stroke individuals. After adjusting for all covariates, higher klotho was associated with a lower prevalence of Stroke. According to the subgroup analyses and interaction tests, age, gender, race, BMI, hypertension, diabetes mellitus, family members, drinker and smoker were not significantly correlated with the influence of klotho and Stroke. After adjusting for all covariates, higher klotho was associated with a lower prevalence of stroke [OR: 0.68, 95% CI: 0.47–0.99].
Conclusion: This study disclosed the negative correlation between serum klotho protein levels and the prevalence of Stroke. Further prospective studies are requisite to investigate the impact of serum klotho protein levels on Stroke and determine the causal relationship.
1 Introduction
Stroke represents the second leading global cause of mortality, surpassed only by acute ischemic heart disease (1). This severe acute cerebrovascular disease not only threatens survival but frequently induces debilitating sequelae including motor deficits, cognitive impairment, and affective disorders, imposing substantial socioeconomic burdens and quality-of-life deterioration (2). With the current development of an aging population, the prevalence rate of stroke is continuously rising (3). Furthermore, stroke is currently emerging gradually among the young population (attributed to factors including patent foramen ovale, dyslipidemia, hormonal therapies, and genetic predisposition) (4, 5). Since stroke is a global public health problem, researchers have never stopped their research on the specific etiology, pathogenesis, predictive means and treatment measures of stroke. And they have been endeavoring to prevent the occurrence of stroke and handle it in a timely manner through some laboratory test indicators and other approaches.
The klotho gene, first identified through longevity studies in murine models (1997), has emerged as a pleiotropic regulator of aging processes. In mice, the overexpression of the klotho gene prolongs lifespan, while its mutation reduces it (6). The human klotho gene is situated at chromosome 13q12. It demonstrates effects such as anti-aging and anti-inflammation and is correlated with numerous age-related diseases (7, 8). The klotho protein encoded by the klotho gene is predominantly a single-channel transmembrane protein, and its soluble component can be cleaved to form α-klotho and dissolve in the blood, featuring three distinct functional types: membrane-bound α-klotho, truncated soluble α-klotho, and secreted α-klotho (6). Unless otherwise specified, the term “klotho” specifically refers to α-klotho. In previous studies, it has been found to be associated with kidney diseases (9), cardiovascular diseases (10, 11), and diabetes mellitus (12). Additionally, in the field of neurology, it has also been discovered that its reduction might be related to Alzheimer’s disease and other degenerative disorders (13). In 2014, Dubal and colleagues discovered that mice with higher serum klotho levels exhibited stronger cognitive function across all age groups, independent of the aging process (14). Subsequently, the team of Castner demonstrated through experiments in rodents and primates that klotho enhances synaptic plasticity and cognitive capacity. Notably, their research revealed that a single low-dose (but not high-dose) administration of klotho could improve memory function in aged non-human primates (15). Emerging evidence from animal studies has demonstrated that beyond its well-documented cardiovascular effects, klotho protein plays a significant role in mediating ischemic preconditioning mechanisms. This endogenous neuroprotective function not only reduces stroke prevalence but also exhibits therapeutic potential through multifaceted cerebroprotective actions, including attenuation of oxidative stress and modulation of apoptotic pathways (16, 17).
Despite these advances, critical knowledge gaps persist regarding klotho’s clinical relevance in cerebrovascular disease. Whether serum klotho levels demonstrate an independent association with stroke risk beyond traditional cardiovascular risk factors. The number of related clinical studies is limited, and the sample sizes therein are relatively small. We hypothesize that: Serum klotho concentration inversely correlates with stroke prevalence in adults. This association remains significant after comprehensive adjustment for confounders including lipid profiles and metabolic comorbidities. Hence, we carried out this research, conducting a large-sample study in the NHANES, which is representative of the national population, in the hope of testing these hypotheses between serum klotho and the prevalence of Stroke in the clinical setting.
2 Materials and methods
2.1 Study population
The data for this article were derived from the National Health and Nutrition Examination Survey (NHANES), which is a nationwide cross-sectional examination carried out by the National Center for Health Statistics (NCHS) of the US Centers for Disease Control and Prevention (CDC) to evaluate the health and nutrition status of the US population. The complete research design and data can be accessed on the NCHS website.1 All participants provided written informed consent. The Research Ethics Review Board of the CDC and NCHS sanctioned the survey protocol.
We collected data from 50,588 participants in the NHANES database between 2007 and 2016. After excluding participants with missing Stroke data (n = 21,388) and klotho data (n = 15,436). Since klotho protein is primarily secreted by the kidneys, we excluded participants with chronic kidney disease (CKD), defined as an estimated glomerular filtration rate (eGFR) < 60 ml/min. As the NHANES database does not directly provide eGFR values, we calculated eGFR using established formulas from previous literature (18).
The CKD-EPI equations for estimating the GFR are as follows:
where SCr is μmol/L. ① a-values: 166 for black females and 163 for black males; 144 for white females of other races and 141 for white males of other races; ② b-values: 0.7 for females and 0.9 for males; and ③ c-values: −0.329 for females with SCr ≤ 0.7 mg/dl and −1.209 for females with SCr > 0.7 mg/dl; −0.411 for males with SCr ≤ 0.9 mg/dl and −1.209 for males with SCr > 0.9 mg/dl.
12,414 participants were ultimately encompassed in the current study. A total of 450 participants were defined as Stroke. The NHANES data utilized in this study were gathered in accordance with standard operating procedures. By conducting repeated measurements under the same conditions, intra-batch variability can be minimized, while inter-batch variability can be reduced through standardized procedures and periodic equipment calibration. We also employed sample weighting and multiple imputation methods in the analysis to further augment the reliability of the results (Figure 1).
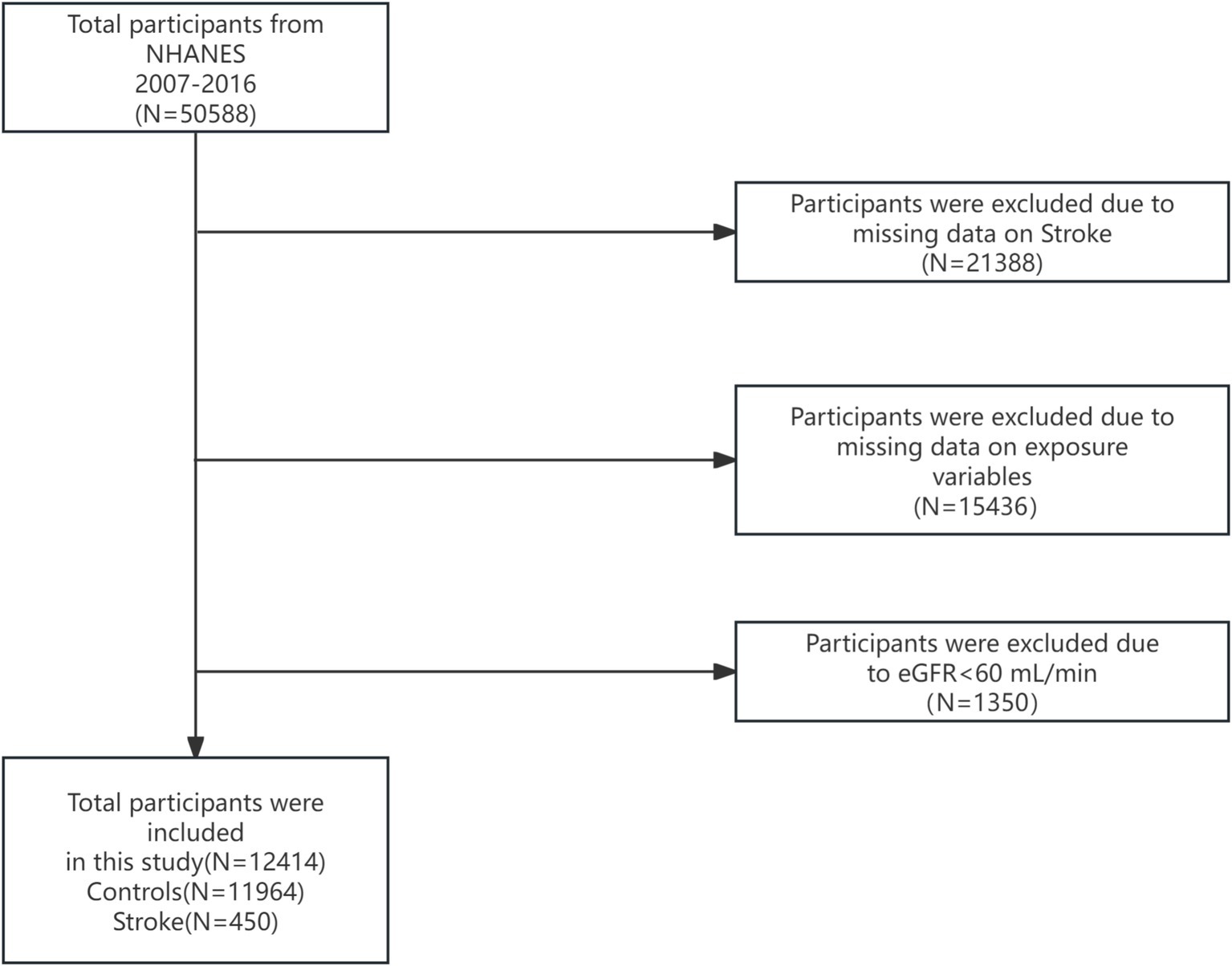
Figure 1. Flow chart of participants selection. NHANES, National Health and Nutrition Examination Survey.
2.2 Assessment of stroke
The definition of Stroke is based on previous studies (19–21). Stroke was defined by self-reported previous diagnosis by a physician. “Have you ever been told by a physician or a health professional that you had stroke?” If you answered yes, you were considered having stroke. Given that previous studies have shown the relatively high prevalence of ischemic stroke among stroke patients and its closer association with the klotho gene, despite the lack of information on stroke types in the NHANES database, it is highly likely that the majority of stroke participants included in this study were ischemic stroke cases (22).
2.3 Exposure
According to prior research, klotho measurements can be directly obtained from NHANES with established validity (23). All participants’ blood samples were collected and preserved at −80°C. Then klotho concentration in each participant was determined by enzyme-linked immunosorbent assay (ELISA) kit provided by IBL International. Each sample underwent duplicate testing, and the validation results were shared with the study investigators. The final value was calculated as the average of the two results. In order to more clearly define the relationship between klotho and stroke, we discussed the relationship between every 1,000 pg./ml increase in klotho protein and the prevalence rate of stroke in this article. The specific laboratory measurement process and standards for exposure variables can be traced at the NHANES.2
2.4 Assessment of covariates
Our analysis incorporated multiple covariates across three domains: sociodemographic characteristics, biochemical parameters, and chronic comorbidities. Sociodemographic data including age, gender, race, and family members were collected through standardized questionnaires. Weight (kg) divided by height squared (m2) was used to determine BMI. Biochemical measurements comprised serum phosphorus levels and lipid profile components: high-density lipoprotein (HDL), total cholesterol (TC), triglycerides (TG), and low-density lipoprotein (LDL). “Have you smoked at least 100 cigarettes in your entire life?” If you answered yes, you were defined as a smoker. “Had at least 12 alcohol drinks/1 year?” If you answered yes, you were defined as a drinker. Chronic comorbidities encompass hypertension (HBP) and diabetes mellitus (DM). Hypertension was defined as having an average systolic blood pressure of ≥ 140 mmHg or an average diastolic blood pressure of ≥ 90 mmHg and a prior diagnosis of hypertension by a physician. The diabetes mellitus was defined by fasting glucose (mmol/L) ≥ 7.0 and a prior diagnosis of diabetes mellitus by a physician. Moreover, as previous studies have demonstrated associations between phosphorus/phosphate and cardiovascular mortality, but phosphate data are unavailable in NHANES, we adjusted for phosphorus in the covariates (24). All detailed measurement procedures for these variables can be accessed at www.cdc.gov/nchs/nhanes/.
2.5 Statistical analyses
R software (version 4.2) and EmpowerStats (version 4.2) were employed for data analysis, and all calculations were weighted in accordance with the guidelines of NHANES. In the stage of demographic analysis, the data were categorized into Stroke and HC groups. For continuous variables, characteristics were described as mean ± standard error (SE), while for categorical variables, they were described as proportions. Categorical variables were analyzed by means of chi-square test, and continuous variables were analyzed via t-test. A multivariate logistic regression analysis was utilized to explore the association between klotho and Stroke. In Model 1, no covariates were incorporated for adjustment. In Model 2, adjustment variables encompassed age, gender, race, and education. In Model 3, all factors were taken into consideration and adjusted, and a weighted generalized additive model was adopted. Additionally, the smoothed curve fits were generated to examine potential nonlinear relationships. Finally, further stratified analyses and interaction tests were conducted to determine the association between klotho and Stroke. Statistical significance was determined if the two-sided p-value was less than 0.05.
3 Result
3.1 Participants characteristics at baseline
The baseline of all participants characteristics are presented in the Table 1. In total of 12,414 participants were comprised in our research, of which 450 were stroke patients. The average age of the Stroke cohort was 61.79 years, with 49.19% being male and 50.81% female. And the average klotho/1000 was 0.81. Notably, comparative analysis revealed significantly lower klotho values in the stroke group compared with HC (p < 0.05). Significant intergroup differences were observed in established biomarkers including TC, LDL, and AGE (all p < 0.05). Furthermore, the stroke cohort demonstrated significant disparities in multiple categorical variables: race (p < 0.05), family members (p < 0.05), smoker (p < 0.05), HBP (p < 0.05), and DM (p < 0.05) all showed statistically meaningful variations compared to the control group.
3.2 Association between klotho and stroke
Table 2 summarizes our comprehensive analysis examining the inverse association between klotho/1000 and stroke risk. Using multivariate logistic regression with three progressively adjusted models, we consistently observed statistically significant negative correlations across all analytical frameworks. The fully adjusted model (Model 3) revealed that each unit increase in klotho/1000 was associated with a 32% reduction in stroke risk (OR: 0.68; 95% CI: 0.47–0.99).
When stratifying stroke risk into quartiles, significant associations were identified in Quartiles 2 and 4 of the crude model (Model 1). However, these quartile-based associations attenuated to non-significance in the fully adjusted model. Model calibration was confirmed through Hosmer-Lemeshow testing (χ2 = 14.6928, df = 12, p = 0.1437), with the non-significant p-value indicating adequate goodness-of-fit when data were stratified into 12 quantile groups (Table 3).
Complementary analyses using generalized additive models (GAM) with smooth curve fitting (Figure 2) reinforced the consistent inverse relationship pattern across all models. This multimodal analytical approach substantiates the robustness of the observed negative correlation between klotho/1000 expression and stroke susceptibility.
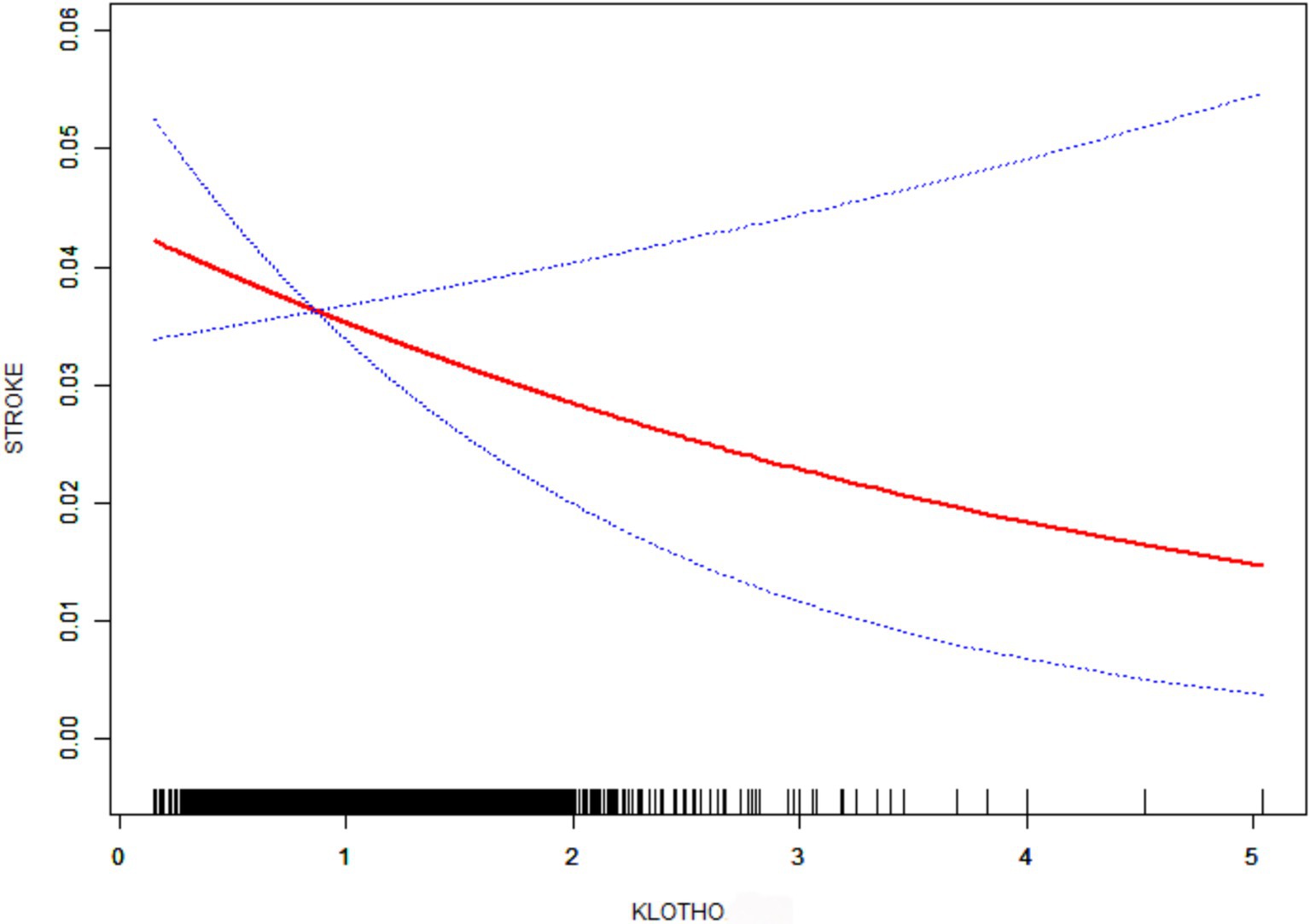
Figure 2. Smooth curve fitting. Age, Gender, Race, BMI, Family members, DM, HBP, HDL, LDL, Phosphorus, TC, TG, Drinker, and Smoker were adjusted.
3.3 Subgroup analysis
As delineated in Table 4, we conducted stratified subgroup analyses and multiplicative interaction testing across clinically relevant demographic and lifestyle parameters (age, gender, race, BMI, hypertension status, diabetes mellitus, family members, alcohol consumption patterns, and smoking status) to evaluate the robustness of the klotho-stroke association and identify potential effect modifiers. Notably, likelihood ratio tests revealed non-significant interaction effects (p > 0.05) across all pre-specified subgroups. This pattern of null heterogeneity suggests the observed association exhibits stability independent of these demographic stratifications, with no evidence of differential effects modulated by subgroup-level characteristics.
4 Discussion
In this research, we selected a total of 12,414 eligible participants from the NHANES from 2007 to 2016, among whom 450 were stroke patients, and explored the correlation between serum klotho and the prevalence of stroke. Notably, this represents the first large-scale epidemiological study establishing an independent negative correlation between serum klotho levels and stroke risk using nationally representative data. Even after eliminating all possible confounding factors, this negative correlation remained highly significant in the fully adjusted model (p < 0.05), with a 32% reduction in the risk of having stroke for every 1,000 pg./ml increase in klotho concentration. When comparing continuous variables and quartile-based categorical variables, the continuous variables show significance while the quartile variables do not. This discrepancy may arise due to the following reasons: (1) Information loss during variable categorization: Converting continuous variables into categorical variables (e.g., quartiles) may lead to information loss. For instance, the original linear relationship of the continuous variable could become obscured after categorization, as internal variations are averaged out and original trends are disrupted. Categorical variables may fail to capture subtle changes inherent in continuous variables, thereby reducing statistical power and resulting in a larger p-value. (2) Sample size limitations: Although the study includes 450 patients, this sample size may still be insufficient relative to the broader population. When categorized into quartiles, the number of stroke patients within each subgroup becomes smaller, further diminishing the statistical power of hypothesis testing. This reduction in power may hinder the detection of true differences or trends, even if they exist in the population. Finally, through subgroup analysis, we discovered that in the majority of subgroups, the negative correlation between serum klotho levels and the prevalence of stroke was remarkable.
Stroke is a globally prevalent public health concern. It is not only the second leading cause of death globally but also the third major cause of death and disability, and incurs substantial costs during the rehabilitation process following a stroke (1). Numerous researchers have been persistently dedicated to investigations in the direction of identifying the specific pathogenic mechanisms and are constantly in pursuit of effective clinical therapeutic approaches for alleviating or preventing Stroke (25, 26). As the population structure changes and the trend of younger strokes emerges, more effective diagnostic and preventive measures, as well as novel and efficacious treatment approaches, are required.
The klotho protein is a significant co-carrier of fibroblast growth factor 23 (FGF23), and their combination exhibits a certain degree of stability. The klotho protein participates in the regulation of calcium and phosphorus, as well as numerous metabolic regulations such as oxidative stress, through the FGF-klotho axis, and is likely to exert a crucial role in senile diseases (27, 28). klotho protein can be generated by ependymal cells of the choroid plexus, Purkinje EC cells, and hippocampal neurons and exists abundantly in the brain (29). In the field of neurology, especially in diseases associated with cognition, overexpressed klotho protein is capable of ameliorating cognitive impairments represented by Alzheimer’s disease. Furthermore, artificial injection of klotho protein is also able to enhance synaptic plasticity and cognitive capacity in non-human primates (13, 15). In another study in 2023, klotho protein can treat sarcopenia by inhibiting transforming growth factor β through binding to ligands and type I and type II serine/threonine kinase receptors (30). An increasing amount of evidence suggests that klotho exerts a neuroprotective effect in the central nervous system and plays a significant role in neurological disorders. Then, with regard to stroke, the most common disorder in the field of neurology, whether klotho plays a significant role in its pathogenesis and offers a certain degree of neuroprotection, or whether klotho can act as a predictive factor or contribute to the rehabilitation of stroke patients, are the issues that researchers aim to address.
In an analysis of a large-scale database, it was also discovered that the reduction of klotho levels has a certain correlation with the high prevalence of hyperlipidemia. It is considered that the potential mechanisms underlying this negative correlation may include three possibilities: anti-inflammatory action, insulin resistance, and antioxidant effects (31). klotho protein is capable of participating in and influencing lipid metabolism, and elevated blood lipids (particularly the increase of LDL) constitute a crucial factor for atherosclerosis and cerebral artery stenosis, which subsequently give rise to stroke. Cerebral ischemic preconditioning (CIP) is capable of inducing cerebral ischemic tolerance and protecting neurons against potential ischemic damage (32, 33). Previous studies have revealed that the upregulation of klotho is capable of preventing ischemic injury in the brain (34, 35). Consequently, two animal experiments conducted in 2022 and 2024 revealed that the upregulation of klotho was capable of suppressing apoptosis and was conducive to the neuroprotection induced by CIP (16, 17). This could also be the reason why klotho contributes to neuroprotection and the prevention of stroke, and there may exist a potential therapeutic approach. Ferroptosis is a type of cell death where iron overload results in the accumulation of lethal levels of lipid hydroperoxides, thereby causing cell death (36, 37). Previous studies have also indicated that following cerebral ischemia, inflammation promptly occurs in the vascular system, generating pro-inflammatory signals and activating immune cells, thereby aggravating neuronal injury (38, 39). However, the augmented expression of klotho is capable of regulating energy metabolism within the brain, mitigating ferroptosis of neurons, and suppressing inflammatory responses, etc. (40–42). The klotho protein, functioning as a coreceptor for fibroblast growth factor 21 (FGF21), plays a critical role in modulating cellular aging processes mediated by human cerebral vascular smooth muscle cells. This cooperative interaction enhances mitochondrial pathway activity while suppressing p53 signaling, ultimately contributing to reduced prevalence of stroke and other neurological disorders (43). Emerging evidence from these investigations and subsequent human studies reveals that klotho’s unique neuroprotective mechanism against stroke pathogenesis appears to be multifactorial. The protein primarily exerts its effects through coordinated suppression of oxidative stress and inflammatory cascades (44–47). Specifically, klotho demonstrates the capacity to regulate endothelial cell homeostasis and attenuate reactive oxygen species (ROS) generation, and inhibit apoptotic signaling while downregulating the expression of adhesion molecules and pro-inflammatory cytokines through multiple intercellular pathways.
However, whether serum klotho protein can act as a clinically applicable indicator for predicting Stroke, or whether the artificial and rational overexpression of klotho protein can prevent, treat or ameliorate the sequelae brought about by Stroke, these are issues that might require resolution in subsequent clinical studies. Compared to previous studies, our research presents several advantages in the following aspects: (1) This is the first clinical data study based on a large sample size from the NHANES database. As a comprehensive dataset collected over an extended period, NHANES encompasses objective health data from all racial groups within the U.S. population, ensuring representativeness and mitigating selection bias. Furthermore, we have thoroughly considered sample design and weighting in our data analysis. This enhances the representativeness and credibility of our research findings. (2) Due to the influence of potential confounding factors in observational studies, we employed a multivariable logistic regression model in our data analysis. This approach allowed us to control for a range of relevant covariates and comprehensively elucidate the correlation between serum klotho levels and the prevalence of stroke. (3) We also conducted stratified and interaction effect analyses, which yielded consistent conclusions regarding the correlation between serum klotho levels and stroke prevalence across different subgroups. This demonstrates the robustness of the results in the present investigation. However, our study does have certain limitations: (1) This study has limitations as a non-prospective investigation. The detection method employed for klotho was not the gold standard (immunoprecipitation immunoblotting), thus introducing potential measurement bias (48). Due to the cross-sectional design of the research, we can only establish a correlation between serum klotho levels and the prevalence of stroke, without being able to determine a causal relationship. Further prospective studies with larger sample sizes are needed to clarify this causality. (2) The selection of stroke patients was limited by the absence of stroke subtype classification in the NHANES database, resulting in potential heterogeneity of cerebrovascular pathology that precluded definitive confirmation of ischemic stroke diagnoses, thereby introducing potential selection bias. (3) The limitations of the data included in the database prevent us from accounting for all potential confounding variables that may influence the results, as well as other possible contributing factors. (4) Given that NHANES primarily relies on data from the U.S. population and its ethnic groups, further investigations are required to ascertain whether our findings are applicable to specific circumstances in other countries and among different ethnicities.
5 Conclusion
In summary, our research indicates a significant negative correlation between serum klotho levels and the prevalence of stroke. Furthermore, lower serum klotho levels are independently associated with a higher prevalence of stroke. This raises the possibility that supplementation with klotho protein may prevent or treat stroke. However, it is important to note that the current findings only demonstrate correlation and do not establish causation. Therefore, further large-scale, multi-ethnic prospective studies are needed to confirm the causal relationship between serum klotho levels and stroke prevalence.
Data availability statement
The original contributions presented in the study are included in the article/supplementary material, further inquiries can be directed to the corresponding authors.
Ethics statement
The studies involving humans were approved by National Center for Health Statistics (NCHS) of the US Centers for Disease Control and Prevention (CDC). The studies were conducted in accordance with the local legislation and institutional requirements. The participants provided their written informed consent to participate in this study.
Author contributions
HX: Conceptualization, Data curation, Formal analysis, Investigation, Methodology, Writing – original draft, Writing – review & editing. YD: Data curation, Formal analysis, Validation, Writing – review & editing. YZ: Conceptualization, Data curation, Investigation, Writing – original draft. JL: Data curation, Writing – original draft. SZ: Software, Writing – original draft. DW: Formal analysis, Writing – review & editing. XX: Supervision, Writing – review & editing. XM: Validation, Writing – review & editing. CW: Data curation, Supervision, Writing – original draft. SX: Data curation, Formal analysis, Supervision, Writing – review & editing.
Funding
The author(s) declare that no financial support was received for the research and/or publication of this article.
Conflict of interest
The authors declare that the research was conducted in the absence of any commercial or financial relationships that could be construed as a potential conflict of interest.
Generative AI statement
The author(s) declare that no Gen AI was used in the creation of this manuscript.
Publisher’s note
All claims expressed in this article are solely those of the authors and do not necessarily represent those of their affiliated organizations, or those of the publisher, the editors and the reviewers. Any product that may be evaluated in this article, or claim that may be made by its manufacturer, is not guaranteed or endorsed by the publisher.
Footnotes
2. ^See footnote 1.
References
1. Feigin, VL, Brainin, M, Norrving, B, Martins, S, Sacco, RL, Hacke, W, et al. World stroke organization (WSO): global stroke fact sheet 2022. Int J Stroke. (2022) 17:18–29. doi: 10.1177/17474930211065917
2. Herpich, F, and Rincon, F. Management of Acute Ischemic Stroke. Crit Care Med. (2020) 48:1654–63. doi: 10.1097/ccm.0000000000004597
3. Ekker, MS, Verhoeven, JI, Vaartjes, I, van Nieuwenhuizen, KM, Klijn, CJM, and de Leeuw, F-E. Stroke incidence in young adults according to age, subtype, sex, and time trends. Neurology. (2019) 92:7533. doi: 10.1212/wnl.0000000000007533
4. Bhatt, N, Malik, AM, and Chaturvedi, S. Stroke in young adults. Neurol Clin Pract. (2018) 8:501–6. doi: 10.1212/cpj.0000000000000522
5. Potter, TBH, Tannous, J, and Vahidy, FS. A contemporary review of epidemiology, risk factors, etiology, and outcomes of premature stroke. Curr Atheroscler Rep. (2022) 24:939–48. doi: 10.1007/s11883-022-01067-x
6. Xu, Y, and Sun, Z. Molecular basis of klotho: from gene to function in aging. Endocr Rev. (2015) 36:174–93. doi: 10.1210/er.2013-1079
7. Makoto Kuro-o, YM, Aizawa, H, Kawaguchi, H, Suga, T, Utsugi, T, Ohyama, Y, et al. Mutation of the mouse klotho gene leads to a syndrome resembling ageing. Nature. (1997) 390:45–51. doi: 10.1038/36285
8. Yutaka Matsumura, HA, Shiraki-Iida, T, Kuro-o, M, and Nabeshima, Y-i. HumanklothoGene and its two transcripts encoding membrane and SecretedklothoProtein. Biochem Biophys Res Commun. (1997) 242:626–30. doi: 10.1006/bbrc.1997.8019
9. Neyra, JA, Hu, MC, and Moe, OW. klotho in clinical nephrology. Clin J Am Soc Nephrol. (2021) 16:162–76. doi: 10.2215/cjn.02840320
10. Cai, J, Zhang, L, Chen, C, Ge, J, Li, M, Zhang, Y, et al. Association between serum klotho concentration and heart failure in adults, a cross-sectional study from NHANES 2007–2016. Int J Cardiol. (2023) 370:236–43. doi: 10.1016/j.ijcard.2022.11.010
11. Lanzani, C, Citterio, L, and Vezzoli, G. klotho: a link between cardiovascular and non-cardiovascular mortality. Clin Kidney J. (2020) 13:926–32. doi: 10.1093/ckj/sfaa100
12. Tang, A, Zhang, Y, Wu, L, Lin, Y, Lv, L, Zhao, L, et al. klotho’s impact on diabetic nephropathy and its emerging connection to diabetic retinopathy. Front Endocrinol. (2023) 14:1180169. doi: 10.3389/fendo.2023.1180169
13. Zhao, Y, Zeng, CY, Li, XH, Yang, TT, Kuang, X, and Du, JR. klotho overexpression improves amyloid-β clearance and cognition in the APP/PS1 mouse model of Alzheimer's disease. Aging Cell. (2020) 19:e13239. doi: 10.1111/acel.13239
14. Dubal, DB, Yokoyama, JS, Zhu, L, Broestl, L, Worden, K, Wang, D, et al. Life extension factor klotho enhances cognition. Cell Rep. (2014) 7:1065–76. doi: 10.1016/j.celrep.2014.03.076
15. Castner, SA, Gupta, S, Wang, D, Moreno, AJ, Park, C, Chen, C, et al. Longevity factor klotho enhances cognition in aged nonhuman primates. Nat Aging. (2023) 3:931–7. doi: 10.1038/s43587-023-00441-x
16. Liu, X-Y, Zhang, L-Y, Wang, X-Y, Li, S-C, Hu, Y-Y, Zhang, J-G, et al. STAT4-mediated klotho up-regulation contributes to the brain ischemic tolerance by cerebral ischemic preconditioning via inhibiting neuronal pyroptosis. Mol Neurobiol. (2023) 61:2336–56. doi: 10.1007/s12035-023-03703-2
17. Zhang, L-Y, Liu, X-Y, Su, AC, Hu, Y-Y, Zhang, J-G, Xian, X-H, et al. klotho upregulation via PPARγ contributes to the induction of brain ischemic tolerance by cerebral ischemic preconditioning in rats. Cell Mol Neurobiol. (2022) 43:1355–67. doi: 10.1007/s10571-022-01255-y
18. Zhu, M, Wang, X, Peng, Z, Yan, W, Deng, Q, Li, M, et al. The role of the estimated glomerular filtration rate and body roundness index in the risk assessment of uric acid-lowering therapy-resistant gout in U.S. adults: evidence from the National Health and nutrition examination survey (2007–2018). Ren Fail. (2025) 47:1398. doi: 10.1080/0886022x.2024.2441398
19. Jiang, YA, Shen, J, Chen, P, Cai, J, Zhao, Y, Liang, J, et al. Association of triglyceride glucose index with stroke: from two large cohort studies and Mendelian randomization analysis. Int J Surg. (2024) 110:5409–16. doi: 10.1097/js9.0000000000001795
20. Shi, R, Tian, Y, Tian, J, Liu, Q, Zhang, J, Zhang, Z, et al. Association between the systemic immunity-inflammation index and stroke: a population-based study from NHANES (2015–2020). Sci Rep. (2025) 15:381. doi: 10.1038/s41598-024-83073-4
21. Zheng, Y, Huang, C, Jin, J, Zhao, Y, Cui, H, and Wei, C. Association between stroke and relative fat mass: a cross-sectional study based on NHANES. Lipids Health Dis. (2024) 23:354. doi: 10.1186/s12944-024-02351-2
22. Mao, Y, Weng, J, Xie, Q, Wu, L, Xuan, Y, Zhang, J, et al. Association between dietary inflammatory index and stroke in the US population: evidence from NHANES 1999–2018. BMC Public Health. (2024) 24:50. doi: 10.1186/s12889-023-17556-w
23. Jiang, L, Guo, T, Zhong, X, Cai, Y, Yang, W, and Zhang, J. Serum protein α-klotho mediates the association between lead, mercury, and kidney function in middle-aged and elderly populations. Environ Health Prev Med. (2025) 30:10–09. doi: 10.1265/ehpm.24-00296
24. Campos-Obando, N, Lahousse, L, Brusselle, G, Stricker, BH, Hofman, A, Franco, OH, et al. Serum phosphate levels are related to all-cause, cardiovascular and COPD mortality in men. Eur J Epidemiol. (2018) 33:859–71. doi: 10.1007/s10654-018-0407-7
25. Han, Z, Song, Y, Qin, C, Zhou, H, Han, D, Yan, S, et al. S-Nitrosylation of Dexras1 controls post-stroke recovery via regulation of neuronal excitability and dendritic remodeling. CNS Neurosci Ther. (2025) 31:e70199. doi: 10.1111/cns.70199
26. Sun, E, Torices, S, Osborne, OM, and Toborek, M. Microvascular dysfunction, mitochondrial reprogramming, and inflammasome activation as critical regulators of ischemic stroke severity induced by chronic exposure to prescription opioids. J Neurosci. (2025) 45:e0614242024. doi: 10.1523/jneurosci.0614-24.2024
27. Chen, G, Liu, Y, Goetz, R, Fu, L, Jayaraman, S, Hu, M-C, et al. α-klotho is a non-enzymatic molecular scaffold for FGF23 hormone signalling. Nature. (2018) 553:461–6. doi: 10.1038/nature25451
28. Sun, F, Liang, P, Wang, B, and Liu, W. The fibroblast growth factor–klotho axis at molecular level. Open Life Sci. (2023) 18:655. doi: 10.1515/biol-2022-0655
29. Wang, X, and Sun, Z. RNAi silencing of brain klotho potentiates cold-induced elevation of blood pressure via the endothelin pathway. Physiol Genomics. (2010) 41:120–6. doi: 10.1152/physiolgenomics.00192.2009
30. Ohsawa, Y, Ohtsubo, H, Munekane, A, Ohkubo, K, Murakami, T, Fujino, M, et al. Circulating α-klotho counteracts transforming growth factor-β–induced sarcopenia. Am J Pathol. (2023) 193:591–607. doi: 10.1016/j.ajpath.2023.01.009
31. Yan, S, Luo, W, Lei, L, Zhang, Q, and Xiu, J. Association between serum klotho concentration and hyperlipidemia in adults: a cross-sectional study from NHANES 2007–2016. Front Endocrinol. (2023) 14:1280873. doi: 10.3389/fendo.2023.1280873
32. Gidday, JM. Cerebral preconditioning and ischaemic tolerance. Nat Rev Neurosci. (2006) 7:437–48. doi: 10.1038/nrn1927
33. Steiger, HJ, and Hänggi, D. Ischaemic preconditioning of the brain, mechanisms and applications. Acta Neurochir. (2006) 149:1–10. doi: 10.1007/s00701-006-1057-1
34. Karizmeh, MS, Shabani, M, Shabani, M, Sardari, M, Babaei, JF, Nabavizadeh, F, et al. Preconditioning exercise reduces hippocampal neuronal damage via increasing klotho expression in ischemic rats. Brain Res Bull. (2022) 188:133–42. doi: 10.1016/j.brainresbull.2022.07.022
35. Long, F-Y, Shi, M-Q, Zhou, H-J, Liu, D-L, Sang, N, and Du, J-R. klotho upregulation contributes to the neuroprotection of ligustilide against cerebral ischemic injury in mice. Eur J Pharmacol. (2018) 820:198–205. doi: 10.1016/j.ejphar.2017.12.019
36. Gowtham, A, Chauhan, C, Rahi, V, and Kaundal, RK. An update on the role of ferroptosis in ischemic stroke: from molecular pathways to neuroprotection. Expert Opin Ther Targets. (2024) 28:1149–75. doi: 10.1080/14728222.2024.2446319
37. Wu, X, Li, Y, Zhang, S, and Zhou, X. Ferroptosis as a novel therapeutic target for cardiovascular disease. Theranostics. (2021) 11:3052–9. doi: 10.7150/thno.54113
38. Alsbrook, DL, Di Napoli, M, Bhatia, K, Biller, J, Andalib, S, Hinduja, A, et al. Neuroinflammation in acute ischemic and hemorrhagic stroke. Curr Neurol Neurosci Rep. (2023) 23:407–31. doi: 10.1007/s11910-023-01282-2
39. He, Y, Wang, J, Ying, C, Xu, KL, Luo, J, Wang, B, et al. The interplay between ferroptosis and inflammation: therapeutic implications for cerebral ischemia-reperfusion. Front Immunol. (2024) 15:1482386. doi: 10.3389/fimmu.2024.1482386
40. Orellana, AM, Mazucanti, CH, dos Anjos, LP, de Sá Lima, L, Kawamoto, EM, and Scavone, C. klotho increases antioxidant defenses in astrocytes and ubiquitin–proteasome activity in neurons. Sci Rep. (2023) 13:15080. doi: 10.1038/s41598-023-41166-6
41. Roig-Soriano, J, Griñán-Ferré, C, Espinosa-Parrilla, JF, Abraham, CR, Bosch, A, Pallàs, M, et al. AAV-mediated expression of secreted and transmembrane αklotho isoforms rescues relevant aging hallmarks in senescent SAMP8 mice. Aging Cell. (2022) 21:e13581. doi: 10.1111/acel.13581
42. Xu, T, Zhu, Q, Huang, Q, Gu, Q, Zhu, Y, Tang, M, et al. FGF21 prevents neuronal cell ferroptosis after spinal cord injury by activating the FGFR1/β-klotho pathway. Brain Res Bull. (2023) 202:110753. doi: 10.1016/j.brainresbull.2023.110753
43. Wang, X-M, Xiao, H, Liu, L-L, Cheng, D, Li, X-J, and Si, L-Y. FGF21 represses cerebrovascular aging via improving mitochondrial biogenesis and inhibiting p53 signaling pathway in an AMPK-dependent manner. Exp Cell Res. (2016) 346:147–56. doi: 10.1016/j.yexcr.2016.06.020
44. Chen, O, Woo, HG, Chang, Y, Ryu, D-R, and Song, T-J. Plasma klotho concentration is associated with the presence, burden and progression of cerebral small vessel disease in patients with acute ischaemic stroke. PLoS One. (2019) 14:796. doi: 10.1371/journal.pone.0220796
45. Cui, W, Leng, B, Liu, W, and Wang, G. Suppression of apoptosis in human umbilical vein endothelial cells (HUVECs) by klotho protein is associated with reduced endoplasmic reticulum oxidative stress and activation of the PI3K/AKT pathway. Med Sci Monit. (2018) 24:8489–99. doi: 10.12659/msm.911202
46. Rakugi, H, Matsukawa, N, Ishikawa, K, Yang, J, Imai, M, Ikushima, M, et al. Anti-oxidative effect of klotho on endothelial cells through cAMP activation. Endocrine. (2007) 31:82–7. doi: 10.1007/s12020-007-0016-9
47. Wei, H, Li, H, Song, X, Du, X, Cai, Y, Li, C, et al. Serum klotho: a potential predictor of cerebrovascular disease in hemodialysis patients. BMC Nephrol. (2019) 20:63. doi: 10.1186/s12882-019-1232-2
Keywords: klotho, stroke, atherosclerosis, cross-sectional study, NHANES
Citation: Xu H, Ding Y, Zhang Y, Li J, Zhou S, Wang D, Xing X, Ma X, Wang C and Xu S (2025) The association between serum klotho protein and stroke: a cross-sectional study from NHANES 2007–2016. Front. Neurol. 16:1573027. doi: 10.3389/fneur.2025.1573027
Edited by:
Haipeng Liu, Coventry University, United KingdomReviewed by:
Senthil Kumaran Satyanarayanan, Hong Kong Institute of Innovation and Technology, Hong Kong SAR, ChinaLeiluo Geng, The University of Hong Kong, Hong Kong SAR, China
Natalia Campos-obando, Erasmus Medical Center, Netherlands
Copyright © 2025 Xu, Ding, Zhang, Li, Zhou, Wang, Xing, Ma, Wang and Xu. This is an open-access article distributed under the terms of the Creative Commons Attribution License (CC BY). The use, distribution or reproduction in other forums is permitted, provided the original author(s) and the copyright owner(s) are credited and that the original publication in this journal is cited, in accordance with accepted academic practice. No use, distribution or reproduction is permitted which does not comply with these terms.
*Correspondence: Cunfu Wang, ODEyOTg1NzNAcXEuY29t; Shunliang Xu c2x4dUBsaXZlLmNvbQ==
†These authors have contributed equally to this work