- 1Hospital of Chengdu University of Traditional Chinese Medicine, Chengdu, China
- 2Deyang Hospital Affiliated Hospital of Chengdu University of Traditional Chinese Medicine, Deyang, China
Purpose: To assess the practicality and utility of employing dual post-label delay (PLD) arterial spin labeling (ASL) in transient ischemic attack (TIA) individuals exhibiting Fluid-attenuated inversion recovery (FLAIR) vascular hyperintensity (FVH).
Materials and methods: We conducted a retrospective review of clinical data from TIA patients presenting with unilateral severe atherosclerotic stenosis or obstruction of either the intracranial internal carotid artery or the middle cerebral artery. Participants were categorized into two groups based on the presence or absence of FVH: FVH positive and FVH negative. All individuals underwent pseudo-continuous ASL perfusion imaging, utilizing distinct PLD durations (1,525 and 2,525 ms) alongside qualitative visual assessments of ASL perfusion irregularities. Standardized TIA evaluations, which included medical history reviews, neuropsychological assessments, and ABCD2 scoring, were performed on all subjects. We explored the correlations between FVHs, clinical manifestations, vascular risk factors, and perfusion metrics.
Results: A total of 50 patients were included in this investigation, with FVH detected in 16 subjects (32.0%). The ABCD2 score was notably elevated within the FVH positive cohort compared to the FVH negative group. At a PLD of 1,525 ms, cerebral blood flow (CBF) values for the affected and healthy hemispheres in the FVH positive group were recorded at 19.55 ± 6.67 and 40.32 ± 6.83, respectively; corresponding values in the FVH negative group were 23.74 ± 5.03 and 46.43 ± 7.91. For a PLD of 2,525 ms, the CBF values for the affected and healthy sides in the FVH positive group were 34.11 ± 5.87 and 50.27 ± 8.57, while the FVH negative group recorded values of 42.79 ± 7.03 and 52.07 ± 7.29, respectively. The differential CBF (ΔCBF) for the affected side in the FVH positive and negative groups was 14.57 ± 4.34 and 19.05 ± 6.10, respectively. A significant negative correlation was established between ΔCBF and ABCD2 scores (Kendall’s tau-b = −0.578, p < 0.001).
Conclusion: The findings of this study indicate a strong association between the presence of FVH signs and a marked reduction in cerebral blood flow, as well as diminished blood flow reserve. This underscores the potential role of FVH as a biomarker for hemodynamic impairment in TIA patients.
1 Background
Cerebral stenotic or occlusive disorders are associated with a significant decrease in flow velocity attributable to vascular stenosis or occlusion, and the development of collateral blood flow (1). Patients suffering from atherosclerotic steno-occlusive cerebrovascular disease exhibit a considerable, albeit variable, risk of subsequent stroke. A transient ischemic attack (TIA) is typically defined by the rapid onset of a focal neurological deficit of vascular origin, which completely resolves within 24 h (2–4). TIA patients with steno-occlusive cerebrovascular conditions have long been acknowledged as being at heightened risk for subsequent stroke events.
Fluid-attenuated inversion recovery (FLAIR) vascular hyperintensities (FVHs) are described as focal, linear, or serpentine hyperintensities that correspond to arteries within the subarachnoid space and are frequently observed in patients with large artery severe stenosis or occlusion (LASO) (5, 6). FVHs are often detected in individuals with acute ischemic stroke, suggesting considerable hemodynamic impairment and sluggish retrograde flow in the ischemic region (7, 8). Possible explanations for their presence include stagnant blood flow and delayed antegrade or retrograde filling (9). The collateral circulation of the leptomeninges plays a critical role in certain clinical scenarios involving transient ischemic attack (TIA) patients, particularly those with LASO. Some individuals within this cohort exhibit FLAIR vascular hyperintensity, while others do not. However, investigations focusing on the correlation between focal vascular hyperintensities (FVHs) and cerebral perfusion remain sparse.
The use of dynamic magnetic resonance imaging with sensitivity to contrast agents may elevate the potential for complications related to the administration of exogenous contrast materials (10, 11). Arterial spin labeling (ASL) magnetic resonance perfusion imaging serves as a valuable technique for visualizing cerebral perfusion and assessing cerebral blood flow (CBF). This method employs magnetically tagged protons in arterial blood as an intrinsic tracer, thereby negating the necessity for external contrast agents or radioactive tracers (12–16). A strong correlation has been consistently identified in many studies when assessing cerebral perfusion using ASL and CT perfusion (17, 18). An essential parameter in ASL is the post-label delay (PLD) time, defined as the interval between the conclusion of the pulse sequence and the subsequent image acquisition. A brief PLD may not allow for adequate delivery of labeled blood to the target tissue, while an excessively long PLD can result in substantial T1 decay, ultimately diminishing the signal-to-noise ratio (19, 20). When utilizing a singular conventional PLD, the labeled bolus may not completely reach the parenchyma intended for examination, particularly in junctional zones, leading to significant local signal attenuation that could be misinterpreted as false hypoperfusion. Conversely, in patients with well-developed collateral circulation, a single conventional PLD may present apparent hyperperfusion in areas where collateral blood flow is stagnant, a phenomenon referred to as the arterial transit artifact (19). It is thus recommended to employ multiple PLD strategies to enhance the accuracy of CBF quantification (21, 22). The aim of this study was to assess the cerebral hemodynamic state in TIA patients experiencing severe stenosis or occlusion of large arteries, particularly those exhibiting FLAIR vascular hyperintensity.
2 Materials and methods
2.1 Subjects
We performed a retrospective analysis of TIA patients diagnosed with unilateral severe atherosclerotic stenosis (greater than 70%) or occlusion of the intracranial internal carotid artery (ICA, C6, or C7 segment) or middle cerebral artery (MCA, M1 segment) at our institution from January 2023 to December 2024. The inclusion criteria encompassed: (1) Transient neurological symptoms that a clinical neurologist assessed to potentially have a vascular origin; (2) Confirmation of unilateral stenosis or occlusion of the ICA or MCA via MRA or CTA; (3) Non-specific findings on general MRI and diffusion-weighted imaging (DWI); and (4) Completion of an MRI study incorporating ASL with PLD values of 1,525 and 2,525 ms. Exclusion criteria included: (1) Presence of intracranial hemorrhage, brain tumors, cranial trauma, psychiatric disorders, or other recognized brain abnormalities; (2) Poor quality of ASL imaging and failure to perform standard imaging; (3) Other cerebrovascular conditions such as Moyamoya disease or various cerebrovascular malformations; and (4) Incomplete or absent clinical data for patients. All subjects underwent routine screening for TIA, with ABCD2 scores evaluated by trained neurologists through the review of electronic medical records. The study received approval from the Ethics Committee of the Hospital of Chengdu University of Traditional Chinese Medicine, which waived the necessity for written informed consent due to the retrospective nature of the research.
2.2 MR imaging
All patients underwent MRI scans utilizing a Discovery MR750 3.0 T system (GE Healthcare, Milwaukee, WI, United States) outfitted with an 8-channel phased array head coil. The imaging protocol included T1-weighted imaging (T1WI), T2-weighted imaging (T2WI), T2 fluid-attenuated inversion recovery (T2-FLAIR), diffusion-weighted imaging (DWI), angiography (MRA) and pseudo-continuous arterial spin labeling (ASL) perfusion imaging were performed using two distinct post-labeling delays (PLDs) of 1,525 and 2,525 ms. The acquisition of the whole-brain three-dimensional pCASL perfusion sequence was executed utilizing a fast spin-echo methodology with background suppression, adhering to the specified parameters: labeling duration of 1,525 ms, repetition time (TR) of 4,632 ms, echo time (TE) of 10.5 ms, 36 slices, a slice thickness of 4.0 mm, a field of view of 24 cm × 24 cm, and an acquisition duration of 4 min and 29 s. Subsequently, the PLD was adjusted to 2,525 ms, while retaining all other parameters constant, resulting in an acquisition time of 5 min and 9 s.
2.3 Image evaluation
In terms of image evaluation, focal FVH was characterized as a serpentine or speckled hyperintensity located in the sulcus and subarachnoid space on T2-FLAIR imaging. Two independent neuroradiologists, each possessing a minimum of 5 years of MRI experience, scrutinized the images to identify the presence of FVH signs and ASL perfusion anomalies through qualitative visual assessment, deliberately excluding identifiable and clinical information. The ASL cerebral blood flow (CBF) maps for both patient groups underwent post-processing and were generated utilizing the Function Tool (Advanced Workstation 4.6; GE Healthcare). For each patient, a rounded region of interest (ROI) was meticulously delineated on the 3D PCASL images to symmetrically assess CBF values on both the affected and healthy hemispheres. Careful placement of the ROIs was ensured to circumvent blood vessels, cerebral sulci, or cerebral cisterns. Three ROIs were employed in each brain region to quantify CBF, and the average value was utilized for subsequent analysis. In patients experiencing hemispheric transient ischemic attacks, the assessment of perfusion disturbances was conducted to ascertain their correlation with the hemispheric localization of presenting symptoms.
2.4 Statistical analysis
Inter-observer agreement was assessed using kappa (κ) statistics. κ > 0.6 is considered to indicate good agreement, while κ > 0.8 is regarded as excellent. Continuous variables that follow a normal distribution are presented as mean ± standard deviation (SD), whereas categorical variables are reported in terms of frequency (%). The differences in each of the categorical variables between the two groups were analyzed using one-way ANOVA, the chi-square test, and Fisher’s exact test (when the expected cell frequency was < 5). Statistical significance was set at p < 0.05. All statistical analyses were performed using SPSS software (version 20.0, SPSS Inc., Chicago, IL, United States).
3 Results
3.1 Demographic and clinical information
Fifty patients (mean age: 60.5 ± 9.0 years; 27 males) satisfied the inclusion criteria and were recruited for the study. The inter-reader agreement for FVH detection was determined to be good (κ = 0.86). Among the cohort, FVH was identified in 16 patients (32.0%). The ABCD2 scores for subjects in the study were 4 (3–5) in the FVH-positive group and 2 (1–3) in the FVH-negative group, showcasing a statistically significant difference (p < 0.05). Furthermore, significant disparities were noted between the FVH negative and FVH positive groups in terms of prior stroke history and symptom duration (p < 0.05). Notably, cardiovascular risk factors, including hypertension, hyperlipidemia, and smoking history, did not exhibit significant differences between patients with and without FVH. Detailed characteristics of TIA patients, both with and without FVH signs, are outlined in Table 1.
3.2 Characteristics of cerebral perfusion parameters
The ASL markers in the current study demonstrated good reproducibility between raters (κ = 0.82). The detailed ASL perfusion data for patients from both groups is summarized in Table 2. For the PLD of 1,525 ms, the CBF values were recorded at 19.55 ± 6.67 and 40.32 ± 6.83 for the affected and healthy sides, respectively, within the FVH positive group, whereas the FVH negative group exhibited corresponding values of 23.74 ± 5.03 and 46.43 ± 7.91. In the scenario of a PLD of 2,525 ms, the CBF values were noted as 34.11 ± 5.87 and 50.27 ± 8.57 for the affected and healthy sides in the FVH positive group, whereas in the FVH negative group, the respective values were 42.79 ± 7.03 and 52.07 ± 7.29. The D-value (ΔCBF) for both groups was compared on the affected side across different PLDs, yielding values of 14.57 ± 4.34 and 19.05 ± 6.10, respectively. Representative cases of FVH positive and FVH negative patients with varying PLDs are depicted in Figures 1, 2. Notably, a significant negative correlation was identified between ΔCBF and ABCD2 scores (Kendall’s tau-b = −0.578, p < 0.001).
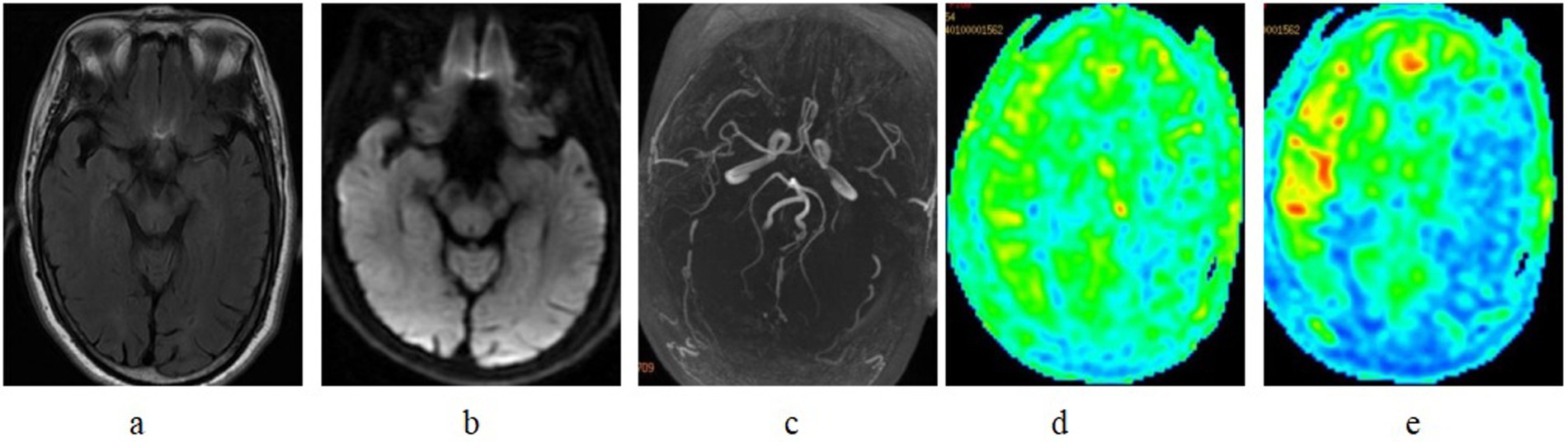
Figure 1. FLAIR vascular hyperintensity in a patient with left middle cerebral artery occlusion. (a) Serpentine hyperintense signal of the middle cerebral artery branches in the left sylvian fissure in T2 FLAIR. (b) DWI demonstrate negative finding. (c) MRA showed occlusion of the left MCA M1. (d) On ASL with a PLD of 1525 ms markedly decreased ASL signals are noted in the extended area of the left hemisphere. ASL with a PLD of 2525 ms, (e) the decreased area is somewhat improved, but there is still a slight laterality compared with the right side.
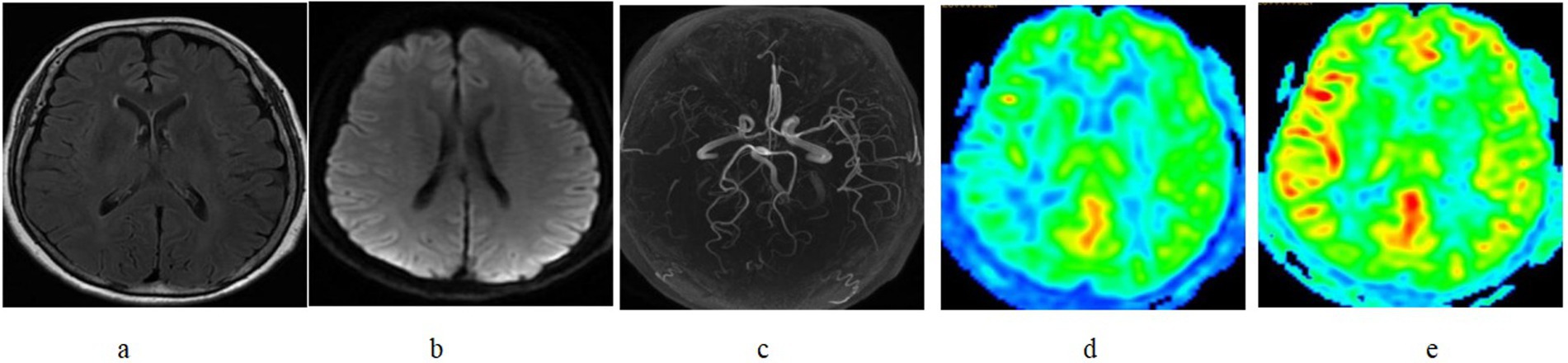
Figure 2. FLAIR vascular hyperintensity absent in a patient with right middle cerebral artery occlusion. (a) FLAIR vascular hyperintensity absent in T2 FLAIR and (b) DWI demonstrate negative finding. (c) MRA showed occlusion of the right MCA M1. The area of the right MCA demonstrated to be hypoperfused on ASLwith a PLD of 1525 ms (d) but improved on ASL with a PLD of 2525 ms (e).
4 Discussion
This investigation focused on the correlation between FVH and perfusion through ASL at varying PLDs in patients suffering from TIA and intracranial aortic stenosis or occlusion. The study presents three primary conclusions. Firstly, FVHs were identified in 32% of TIA patients who underwent LASO, aligning with findings from prior research (23). Secondly, our analysis indicated that TIA patients with LASO exhibiting positive FVH were substantially more likely to have reduced CBF and a more critically compromised blood flow reserve compared to those without FVH. Correlation assessments demonstrated significant negative relationships between ΔCBF and ABCD2 scores. Thirdly, patients with TIA and positive FVH encountered a notably elevated ABCD2 risk score, a frequently utilized metric for assessing stroke risk post-TIA. These results provide valuable insights for clinicians in understanding hemodynamic conditions and collateral compensation, which are essential for accurately identifying disease states and presenting reliable imaging evidence for both primary and secondary stroke prevention.
While much of the existing literature has concentrated on FVH manifestations in patients with acute cerebral infarctions, the prevalence of FVH is highly variable across different studies. The assessment of FVH in TIA patients remains less comprehensive compared to those with ischemic strokes. Maeda et al. (24) evaluated the progression of FVH in acute and subacute cerebral infarctions within the middle cerebral artery territory and observed that FVH appeared in 100% of evaluations conducted within 24 h of symptom onset, but only in 18% of assessments performed 5–9 days post-symptom onset. The occurrence of FVH correlates with the duration between stroke onset and MRI imaging, with its frequency diminishing over time. Ding et al. (25) noted that FVH was detected in as many as 81.6% (31/38) of hospitalized TIA patients exhibiting severe stenosis or occlusion, with MR scans executed within 48 h of symptom onset, a timeframe significantly shorter than that of our study.
Most research suggests a relationship between FVH and either large-vessel occlusion or severe stenosis, as well as hemodynamic impairment. Lyu et al. (5) examined whether FVH could serve as a prognostic indicator for ischemic events in patients with ICA or MCA occlusion. Their findings indicated that the FVH-ASPECTS was substantially lower in the asymptomatic occlusion cohort compared to the symptomatic group, implying that FVH might be predictive of stroke occurrence. Bunker and Hillis (26) reported that the typical location of FVHs matched regions of hypoperfusion in corresponding vascular territories captured via perfusion-weighted imaging. Nam et al. (27) investigated the relationship between FVH and early ischemic lesion recurrence in patients with lesion-negative TIA. Their results demonstrated that FVH is significantly associated with early ischemic lesion recurrence in this patient population. Our study quantitatively illustrates that TIA patients with significant arterial vessel occlusion who also present with FVH exhibit markedly lower CBF values and more severely diminished blood flow reserves than those without FVH. In cases of unilateral LASO, it is essential to consider the effects of delayed anterograde flow along with both primary and secondary collateral circulations when evaluating hemodynamic status.
Disruption of the blood–brain barrier (BBB) represents a critical pathological feature of ischemic stroke. Preserving BBB integrity is vital for maintaining central nervous system homeostasis (28). Cerebrovascular stenosis triggers pathological cascades via hemodynamic alterations. Atherosclerotic narrowing not only decreases cerebral perfusion pressure but also elevates turbulent flow, thereby inducing endothelial shear stress. This stress activates matrix metalloproteinases (MMPs) and compromises tight junction proteins, leading to BBB dysfunction. The resultant BBB compromise facilitates erythrocyte extravasation and hemoglobin degradation, releasing ferrous iron that catalyzes Fenton reactions. The subsequent production of reactive oxygen species (ROS) perpetuates neuroinflammation and exacerbates BBB disruption through vascular endothelial growth factor (VEGF)-mediated vascular remodeling. Importantly, hemosiderin deposition in perivascular spaces generates paramagnetic susceptibility effects detectable on susceptibility-weighted imaging (SWI) MRI. Furthermore, chronic iron overload accelerates tau phosphorylation and amyloid-β aggregation via ferroptosis pathways, establishing a self-sustaining cycle of neurodegeneration (29). Uchida et al. (30) conducted a combined quantitative MRI analysis using QSM and R2* relaxometry, demonstrating that increased iron concentration in ischemic lesions is associated with reduced improvement in neurological outcomes following stroke rehabilitation.
Conventional magnetic resonance imaging has a satisfactory resolution ratio for components of brain tissue, but cannot provide blood flow perfusion information. ASL imaging enables detection of absolute perfusion values. PLD is the most important parameter contributing to the accurate assessment of CBF (31, 32). Cerebral blood flow imaging can sensitively and specifically detect abnormal perfusion changes (33). The present study targeted the noninvasive assessment of cerebral hemodynamics with a quantitative measure of CBF perfusion using the ASL technique. This technique has good reproducibility and does not require radiation or gadolinium-based tracers, thereby avoiding potential adverse effects. CBF readings obtained from a single PLD scan may not accurately reflect the true cerebral perfusion status, particularly in patients with extensive vascular lesions. A PLD of 2,525 ms may detect slower blood flow through secondary collateral circulation, while a PLD of 1,525 ms may not capture such dynamics. This study validated dual-PLD settings of 1,525 and 2,525 ms for assessing the hemodynamic condition of patients with transient ischemic attack (TIA) who underwent large artery stenosis occlusion. Moreover, we explored the clinical significance of supplementary ASL and FVH signs. Patients experiencing transient ischemic attacks frequently exhibit similar pathophysiological mechanisms to individuals suffering from other cerebrovascular stenotic or obstructive diseases, characterized by a pronounced reduction in blood flow velocity resulting from arterial stenosis or occlusion, alongside the establishment of collateral circulation. Intracranial atherosclerosis is a notable contributor to cerebral stenosis and insufficient cerebral perfusion, playing a crucial role in both the initial onset and the recurrence of ischemic strokes (5). Individuals exhibiting transient neurological symptoms alongside confirmed evidence of LASO are at an increased risk of subsequent strokes due to compromised CBF and disturbed cerebral perfusion. The ABCD2 scoring system, which is based on specific risk factors and clinical presentations of TIA, revealed that patients with TIA and FLAIR hyperintensities (FVH) had significantly elevated ABCD2 risk scores, potentially correlating with adverse outcomes. Collateral circulation can sustain brain tissue viability for extended periods following the occlusion of major cerebral arteries, thereby serving a vital function in TIA patients. Therefore, enhancing or preserving collateral circulation emerges as a promising therapeutic target. Given that chronic hemodynamic impairment often leads to progressive cortical neuronal degeneration, early intervention is advocated for TIA patients presenting with FVH to avert stroke occurrence.
The application of ASL magnetic resonance imaging with dual PLD holds significant potential for evaluating cerebral hemodynamics in TIA patients. This method addresses limitations of single-PLD ASL by capturing both early and delayed perfusion phases, which is critical for assessing collateral-dependent blood flow and cerebrovascular reserve (CVR) in regions with prolonged arterial transit times (ATT). For instance, studies in patients with internal carotid artery steno-occlusion demonstrated that hypoperfusion observed at PLD 1.5 s often improved at PLD 2.5 s due to delayed collateral flow, as validated by digital subtraction angiography (DSA) (19, 34). This dual-PLD approach also differentiates stagnant collateral pathways from functional hyperperfusion, which is essential for identifying tissue at risk of ischemic injury in TIA patients (19). Furthermore, dual-PLD ASL correlates with acetazolamide-challenged SPECT in assessing CVR, highlighting its utility for noninvasive evaluation of hemodynamic compromise (35). In periictal hyperperfusion studies, dual PLD revealed distinct hemodynamic patterns (“fast flow” vs. “gradual flow”), suggesting its adaptability to dynamic perfusion changes (36). For TIA patients, combining these capabilities could enable precise stratification of cerebral hemodynamic status, guiding interventions to prevent stroke progression. The method’s repeatability and lack of contrast agents further support its practicality in serial monitoring of TIA-related perfusion alterations (34, 35).
Despite meticulous participant selection and data scrutiny, certain limitations must be acknowledged. Firstly, this investigation was a retrospective study conducted at a single center, which might introduce selection bias. Further prospective research is warranted to elucidate the underlying pathophysiological mechanisms. Secondly, while the study cohort was relatively small, it encompassed a homogenous group of TIA patients who underwent dual PLD assessments. Future research should aim to replicate this investigation with a larger sample size. Thirdly, longitudinal studies are essential to ascertain the prognostic significance of FVH.
5 Conclusion
In conclusion, this study establishes that FVH signs exhibit a strong correlation with diminished cerebral blood flow and a significantly impaired blood flow reserve, potentially reflecting the underlying pathomechanisms associated with stroke. The dual-PLD approach is a noninvasive and straightforward method for evaluating cerebral hemodynamics in TIA patients. We are optimistic that this methodology will evolve into a valuable instrument for prevention and early intervention in the future.
Data availability statement
The raw data supporting the conclusions of this article will be made available by the authors, without undue reservation.
Ethics statement
The studies involving humans were approved by the Ethics Committee of the Hospital of Chengdu University of Traditional Chinese Medicine. The studies were conducted in accordance with the local legislation and institutional requirements. The ethics committee/institutional review board waived the requirement of written informed consent for participation from the participants or the participants’ legal guardians/next of kin due to the retrospective nature of the study.
Author contributions
LZ: Conceptualization, Data curation, Writing – original draft, Writing – review & editing. JW: Data curation, Writing – original draft. QW: Data curation, Writing – original draft. ZY: Data curation, Writing – original draft. HL: Data curation, Investigation, Methodology, Supervision, Writing – review & editing. WW: Conceptualization, Data curation, Investigation, Supervision, Writing – original draft, Writing – review & editing.
Funding
The author(s) declare that financial support was received for the research and/or publication of this article. This research was funded by the National Natural Science Foundation of China (No. 82174358) and the “Xing-lin Scholars” Project of Chengdu University of Traditional Chinese Medicine (No. CCYB2022004).
Conflict of interest
The authors declare that the research was conducted in the absence of any commercial or financial relationships that could be construed as a potential conflict of interest.
Generative AI statement
The authors declare that no Gen AI was used in the creation of this manuscript.
Publisher’s note
All claims expressed in this article are solely those of the authors and do not necessarily represent those of their affiliated organizations, or those of the publisher, the editors and the reviewers. Any product that may be evaluated in this article, or claim that may be made by its manufacturer, is not guaranteed or endorsed by the publisher.
References
1. Li, Z, Li, N, Qu, Y, Gai, F, Zhang, G, and Zhang, G. Application of 3.0T magnetic resonance arterial spin labeling (ASL) technology in mild and moderate intracranial atherosclerotic stenosis. Exp Ther Med. (2016) 12:297–301. doi: 10.3892/etm.2016.3318
2. Khan, F, Yogendrakumar, V, Lun, R, Ganesh, A, Barber, PA, Lioutas, VA, et al. Long-term risk of stroke after transient ischemic attack or minor stroke: A systematic review and meta-analysis[J]. JAMA. (2025). doi: 10.1001/jama.2025.2033
3. Wolf, M-E, Held, V-E, and Hennerici, M-G. Risk scores for transient ischemic attack[J]. Front Neurol Neurosci. (2014) 3341–68. doi: 10.1159/000351891
4. Okada, Y. Transient ischemic attack as a medical emergency. Front Neurol Neurosci. (2014) 33:19–29. doi: 10.1159/000351889
5. Lyu, J, Hu, J, Wang, X, Bian, X, Wei, M, Wang, L, et al. Association of fluid-attenuated inversion recovery vascular hyperintensity with ischaemic events in internal carotid artery or middle cerebral artery occlusion. Stroke Vasc Neurol. (2023) 8:69–76. doi: 10.1136/svn-2022-001589
6. Zeng, L, Wang, Q, Liao, H, Ren, F, Zhang, Y, du, J, et al. FLAIR vascular Hyperintensity: an important MRI marker in patients with transient ischemic attack. Int J Gen Med. (2022) 15:6165–71. doi: 10.2147/IJGM.S371894
7. Lv, B, Ran, Y, Lv, J, Lou, X, and Tian, C. Individualized interpretation for the clinical significance of fluid-attenuated inversion recovery vessel hyperintensity in ischemic stroke and transient ischemic attack: a systematic narrative review. Eur J Radiol. (2023) 166:111010. doi: 10.1016/j.ejrad.2023.111010
8. Legrand, L, Le Berre, A, Seners, P, Benzakoun, J, Hassen, WB, Lion, S, et al. FLAIR vascular Hyperintensities as a surrogate of collaterals in acute stroke: DWI matters. AJNR Am J Neuroradiol. (2023) 44:26–32. doi: 10.3174/ajnr.A7733
9. Lee, KY, Kim, JW, Park, M, Suh, SH, and Ahn, SJ. Interpretation of fluid-attenuated inversion recovery vascular hyperintensity in stroke. J Neuroradiol. (2021) 49:258–66. doi: 10.1016/j.neurad.2021.01.009
10. Souillard-Scemama, R, Tisserand, M, Calvet, D, Jumadilova, D, Lion, S, Turc, G, et al. An update on brain imaging in transient ischemic attack. J Neuroradiol. (2015) 42:3–11. doi: 10.1016/j.neurad.2014.11.001
11. Tung, CE, Olivot, JM, and Albers, GW. Radiological examinations of transient ischemic attack. Front Neurol Neurosci. (2014) 33:115–22. doi: 10.1159/000351913
12. Lv, Y, Wei, W, Song, Y, Han, Y, Zhou, C, Zhou, D, et al. Non-invasive evaluation of cerebral perfusion in patients with transient ischemic attack: an fMRI study. J Neurol. (2019) 266:157–64. doi: 10.1007/s00415-018-9113-3
13. Havsteen, I, Willer, L, Ovesen, C, Nybing, JD, Ægidius, K, Marstrand, J, et al. Significance of arterial spin labeling perfusion and susceptibility weighted imaging changes in patients with transient ischemic attack: a prospective cohort study. BMC Med Imaging. (2018) 18:24. doi: 10.1186/s12880-018-0264-6
14. Grams, RW, Kidwell, CS, Doshi, AH, Drake, K, Becker, J, Coull, BM, et al. Tissue-negative transient ischemic attack: is there a role for perfusion MRI? AJR Am J Roentgenol. (2016) 207:157–62. doi: 10.2214/AJR.15.15447
15. Hartkamp, NS, van Osch, MJ, Kappelle, J, and Bokkers, RPH. Arterial spin labeling magnetic resonance perfusion imaging in cerebral ischemia. Curr Opin Neurol. (2014) 27:42–53. doi: 10.1097/WCO.0000000000000051
16. Lyu, J, Duan, Q, Xiao, S, Meng, Z, Wu, X, Chen, W, et al. Arterial spin labeling-based MRI estimation of penumbral tissue in acute ischemic stroke. J Magn Reson Imaging. (2023) 57:1241–7. doi: 10.1002/jmri.28364
17. Xu, X, Tan, Z, Fan, M, Ma, M, Fang, W, Liang, J, et al. Comparative study of multi-delay Pseudo-continuous arterial spin labeling perfusion MRI and CT perfusion in ischemic stroke disease. Front Neuroinform. (2021) 15:719719. doi: 10.3389/fninf.2021.719719
18. Xu, H, Han, H, Liu, Y, Huo, R, Lang, N, Yuan, H, et al. Perioperative cerebral blood flow measured by arterial spin labeling with different postlabeling delay in patients undergoing carotid endarterectomy: a comparison study with CT perfusion. Front Neurosci. (2023) 17:1200273. doi: 10.3389/fnins.2023.1200273
19. Akiyama, T, Morioka, T, Shimogawa, T, Haga, S, Sayama, T, Kanazawa, Y, et al. Arterial spin-labeling magnetic resonance perfusion imaging with dual Postlabeling delay in internal carotid artery Steno-occlusion: validation with digital subtraction angiography. J Stroke Cerebrovasc Dis. (2016) 25:2099–108. doi: 10.1016/j.jstrokecerebrovasdis.2016.06.005
20. Golay, X, and Ho, ML. Multidelay ASL of the pediatric brain. Br J Radiol. (2022) 95:20220034. doi: 10.1259/bjr.20220034
21. Woods, JG, Achten, E, Asllani, I, Bolar, DS, Dai, W, Detre, JA, et al. Recommendations for quantitative cerebral perfusion MRI using multi-timepoint arterial spin labeling: acquisition, quantification, and clinical applications. Magn Reson Med. (2024) 92:469–95. doi: 10.1002/mrm.30091
22. Jaafar, N, and Alsop, DC. Arterial spin labeling: key concepts and Progress towards use as a clinical tool. Magn Reson Med Sci. (2024) 23:352–66. doi: 10.2463/mrms.rev.2024-0013
23. Yoshioka, K, Ishibashi, S, Shiraishi, A, Yokota, T, and Mizusawa, H. Distal hyperintense vessels on FLAIR images predict large-artery stenosis in patients with transient ischemic attack. Neuroradiology. (2013) 55:165–9. doi: 10.1007/s00234-012-1092-y
24. Maeda, M, Koshimoto, Y, Uematsu, H, Yamada, H, Kimura, H, Kawamura, Y, et al. Time course of arterial hyperintensity with fast fluid-attenuated inversion-recovery imaging in acute and subacute middle cerebral arterial infarction. J Magn Reson Imaging. (2001) 13:987–90. doi: 10.1002/jmri.1142
25. Ding, B, Chen, Y, Jiang, H, Zhang, H, Huang, J, and Ling, HW. Fluid-attenuated inversion recovery vascular Hyperintensities in transient ischemic attack within the anterior circulation. Biomed Res Int. (2020) 2020:7056056. doi: 10.1155/2020/7056056
26. Bunker, LD, and Hillis, AE. Location of Hyperintense vessels on FLAIR associated with the location of perfusion deficits in PWI. J Clin Med. (2023) 12:1554. doi: 10.3390/jcm12041554
27. Nam, KW, Kim, CK, Kim, TJ, Oh, K, Han, MK, Ko, SB, et al. FLAIR vascular hyperintensities predict early ischemic recurrence in TIA. Neurology. (2018) 90:e738–44. doi: 10.1212/WNL.0000000000005034
28. Liang, Y, Jiang, Y, Liu, J, Li, X, Cheng, X, Bao, L, et al. Blood-brain barrier disruption and imaging assessment in stroke. Transl Stroke Res. (2024). doi: 10.1007/s12975-024-01300-6
29. Montagne, A, Barnes, SR, Sweeney, MD, Halliday, MR, Sagare, AP, Zhao, Z, et al. Blood-brain barrier breakdown in the aging human hippocampus. Neuron. (2015) 85:296–302. doi: 10.1016/j.neuron.2014.12.032
30. Uchida, Y, Kan, H, Kano, Y, Onda, K, Sakurai, K, Takada, K, et al. Longitudinal changes in Iron and myelination within ischemic lesions associate with neurological outcomes: a pilot study. Stroke. (2024) 55:1041–50. doi: 10.1161/STROKEAHA.123.044606
31. Zaidat, OO, Yoo, AJ, Khatri, P, Tomsick, TA, von Kummer, R, Saver, JL, et al. Recommendations on angiographic revascularization grading standards for acute ischemic stroke: a consensus statement. Stroke. (2013) 44:2650–63. doi: 10.1161/STROKEAHA.113.001972
32. Bivard, A, Stanwell, P, Levi, C, and Parsons, M. Arterial spin labeling identifies tissue salvage and good clinical recovery after acute ischemic stroke. J Neuroimaging. (2013) 23:391–6. doi: 10.1111/j.1552-6569.2012.00728.x
33. He, Q, Li, G, Jiang, M, Zhou, Q, Gao, Y, and Yan, J. Predicting a favorable (mRS 0-2) or unfavorable (mRS 3-6) stroke outcome by arterial spin labeling and amide proton transfer imaging in post-thrombolysis stroke patients. J Pers Med. (2023) 13:248. doi: 10.3390/jpm13020248
34. Zhang, GR, Zhang, YY, Liang, WB, and Ding, D. Cerebral perfusion in patients with unilateral internal carotid artery occlusion by dual post-labeling delays arterial spin labeling imaging. World J Radiol. (2024) 16:429–38. doi: 10.4329/wjr.v16.i9.429
35. Haga, S, Morioka, T, Shimogawa, T, Akiyama, T, Murao, K, Kanazawa, Y, et al. Arterial spin labeling perfusion magnetic resonance image with dual Postlabeling delay: a correlative study with acetazolamide loading (123)I-Iodoamphetamine single-photon emission computed tomography. J Stroke Cerebrovasc Dis. (2016) 25:1–6. doi: 10.1016/j.jstrokecerebrovasdis.2015.08.025
Keywords: arterial spin labeling, post label delay, FLAIR vascular hyperintensity, hyperintense vessel, magnetic resonance imaging
Citation: Zeng L, Wang J, Wang Q, Zhang Y, Liao H and Wu W (2025) Cerebral hemodynamics evaluation of FLAIR vascular hyperintensity in TIA patients with large artery severe stenosis or occlusion. Front. Neurol. 16:1589198. doi: 10.3389/fneur.2025.1589198
Edited by:
Jieqiong Wang, Chinese Academy of Sciences, ChinaReviewed by:
Yuto Uchida, Johns Hopkins University, United StatesMinglu Yan, University of Texas Southwestern Medical Center, United States
Copyright © 2025 Zeng, Wang, Wang, Zhang, Liao and Wu. This is an open-access article distributed under the terms of the Creative Commons Attribution License (CC BY). The use, distribution or reproduction in other forums is permitted, provided the original author(s) and the copyright owner(s) are credited and that the original publication in this journal is cited, in accordance with accepted academic practice. No use, distribution or reproduction is permitted which does not comply with these terms.
*Correspondence: Wenbin Wu, d3diMTIwMUB2aXAuc2luYS5jb20=; Huaqiang Liao, NzY3MDMwODM3QHFxLmNvbQ==
†These authors have contributed equally to this work