- 1International Center for Tropical Agriculture (CIAT), Cali, Colombia
- 2Departamento de Producción Agraria, Escuela Técnica Superior de Ingeniería Agronómica, Alimentaria y de Biosistemas (ETSIAAB), Universidad Politécnica de Madrid (UPM), Madrid, Spain
Accurate and cost-effective soil bulk density (BD) measurements are crucial for robust climate mitigation and adaptation strategies by ensuring reliable estimation of key soil properties, such as soil organic carbon (SOC) stocks. This study evaluates the effectiveness of the power probe method compared to the traditional core metal ring method (reference method) for measuring BD in acidic soils (Oxisols and Ultisols) in Colombia. Soil samples were collected from eight locations at soil depths of up to 1 meter, with fine fraction content ranging from 29% to 57% silt and 31% to 41% clay, covering two land uses: natural savanna (n=2) and planted pastures (n=6) (Urochloa humidicola cv. Tully). We found minimal differences between the use of metal ring and probe methods for BD measurements, indicating that both methods generate similar results. Out of 32 observations, only four showed significant differences at specific soil depths with varying silt or clay content. While the probe method showed higher variability, particularly in the 0–10 cm and 50–100 cm layers, which is likely due to soil compaction or stretching, it offers significant advantages in terms of cost reduction and improved work efficiency. While further validation is recommended for use in different soil types and conditions, particularly in clay-rich soils, integrating the use of power probe method in soil assessments can significantly enhance the efficiency of BD measurements. This is particularly relevant to support agricultural and SOC management initiatives while streamlining monitoring efforts for climate finance, where reliable, efficient, and cost-effective soil measurements are crucial.
1 Introduction
Soil bulk density (BD) refers to the mass of undisturbed soil, including its pore space. This property is key to understanding soil compaction and its influence on essential processes such as water infiltration, root growth, and nutrient retention. Accurate BD measurements are also essential for evaluating soil health and determining soil organic carbon (SOC) stocks in both agricultural and natural ecosystems (1, 2). Additionally, BD measurement is critical for agricultural management, environmental monitoring, and the development of effective climate finance strategies (3).
Traditionally, BD is measured using soil cores (in a volumetric cylinder) sampled using metal rings (4). But this technique, while accurate, is labor-intensive, time consuming, and thus unsuitable for large-scale assessments. As agricultural and environmental monitoring efforts expand, the demand for more efficient soil sampling methods has grown, prompting researchers to explore alternatives that facilitate rapid and reliable data collection.
Many researchers have sought alternatives to improve BD measurement efficiency (5–8). Al-shammary et al. (6) highlights that the metal ring method, though widely used, faces challenges related to sampling depth, cylinder size, moisture content, and operator expertise, making it time-consuming. The clod method, commonly used in heavy clay soils, requires precise calibration but it is also labor-intensive, while excavation techniques are limited to soils with large pores, and these vary depending on soil texture. As a result, the need for testing alternative methods tailored to specific contexts and regions has become increasingly important.
Recent advancements, such as the use of soil probes, offer efficiency and cost advantages over metal ring methods, especially in large-scale soil surveys (9). This method has even been proposed for carbon projects in voluntary carbon markets, such as those managed by Verra (10), as a cost-effective solution to enhance monitoring processes. By reducing the financial burden of tracking SOC changes, this approach could help attract more investments into the agricultural sector, further advancing sustainability and climate mitigation efforts.
Accuracy of probes compared to traditional methods remains a concern, particularly in soils of varying soil textures (5, 11). Compaction effects in clay-rich soils can skew probe-based measurements, impacting reliability of the results (12). Validating and refining these techniques will improve the cost-effectiveness of soil assessments, making them more accessible for climate finance mechanisms like carbon markets.
This study aims to compare the effectiveness of a power probe method for BD measurement relative to the core metal ring method across different soil depths in the Colombian pastures with varying silt (29-57%) and clay content (31-41%), including both natural and planted pastures of different establishment ages. The goal was to assess the accuracy of the probe method and explore its potential for large-scale soil assessments for management and carbon market applications. We hypothesized that, while both methods provide comparable soil BD measurements, the probe method offers greater efficiency and cost-effectiveness, particularly for large-scale carbon monitoring projects.
2 Materials and methods
2.1 Experimental site and design
Soil sampling sites were selected across different land uses at Hacienda San José and neighboring farms in La Primavera, Vichada, Colombia (5°54’52.48” N, 69°37’12.54” W), to estimate bulk density (BD) using two methods: the metal ring and the power probe (Figures 1, 2). The study covered approximately 5,600 hectares and included two land-use types: (i) native savanna (Savanna A and Savanna B) and (ii) cultivated pastures of Urochloa humidicola CIAT 679, cultivar Tully (hereafter U. humidicola cv. Tully). The cultivated pastures were established in different years (2021, 2022, and 2023), with “A” and “B” distinguishing two separate sites where pastures were established within the same year. These pastures were formerly native savanna areas that were converted into planted grasslands and are now managed under rotational grazing.
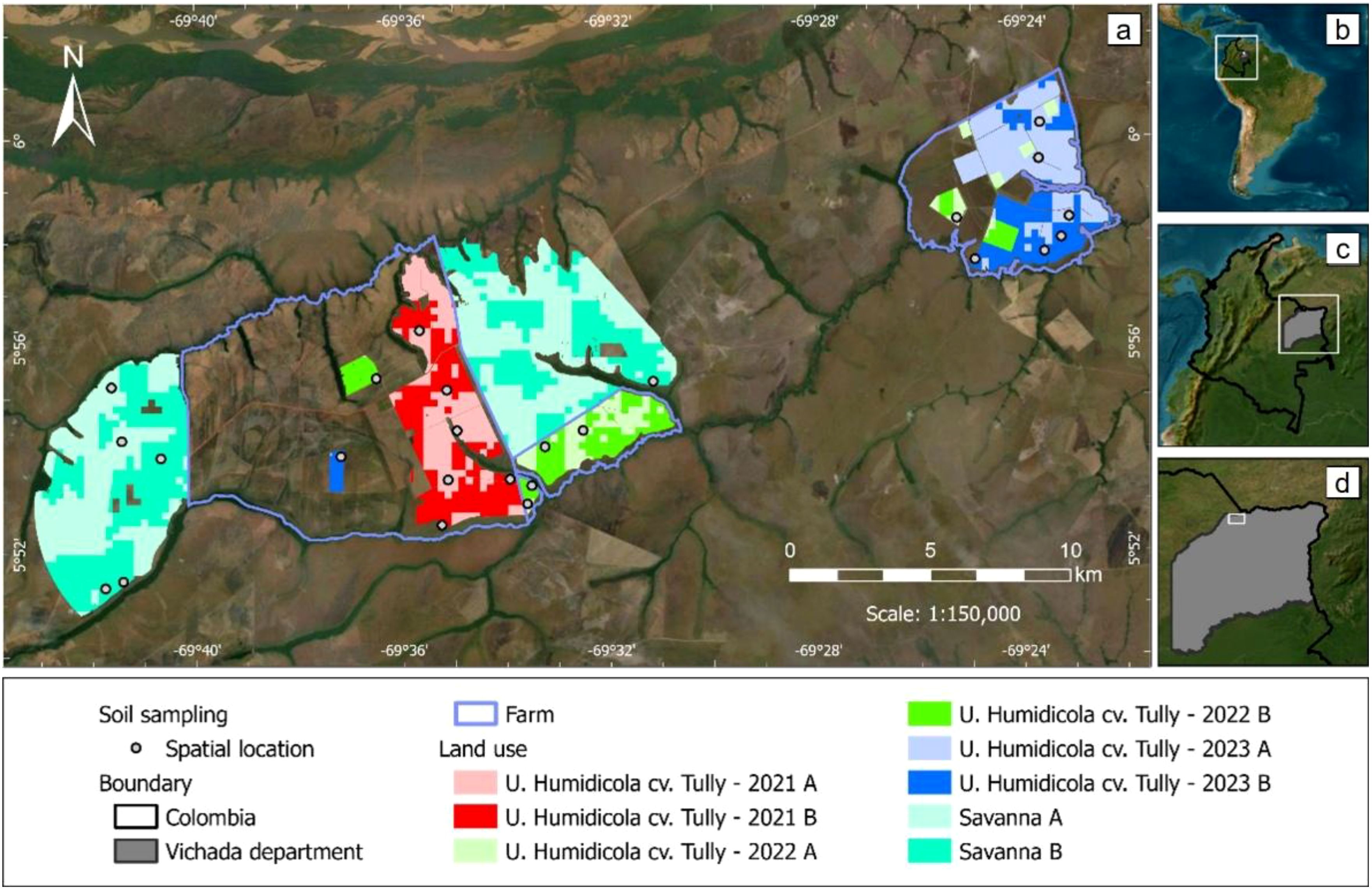
Figure 1. Soil sampling points at Hacienda San José and neighboring farms (a) in La Primavera (d), Vichada (c), Colombia (b), across two land-use types: (i) native savanna (Savanna A and B) and (ii) cultivated Urochloa humidicola cv. Tully pastures, established in 2021, 2022, and 2023. ‘A’ and ‘B’ denote separate pasture areas established within the same year.
A stratified random sampling design was implemented to ensure representative coverage of both land-use types. Within each land use type, soil sampling points were randomly distributed, maintaining a minimum distance between points to reduce spatial autocorrelation (Figure 1). This approach aimed to capture variability across the landscape while minimizing potential bias in bulk density measurements. The climate in this tropical region is warm and humid with an average annual rainfall of 2,223 mm in anunimodal regime and with a mean temperature of 27.3°C (13). The soils in this tropical savanna ecosystem are predominantly acidic and of low fertility. The main soil classes are Xanthic Haplustox, Typic Kandiustults, Oxyaquic Haplustepts, Plinthic Kandiustults, Fluvaquentic Humaquepts, and Typic Acrustox (14, 15).
2.2 Soil sampling methods
In 2024, a total of 48 sampling points were selected across two native savanna areas and six cultivated pasture areas. At each area, 3 soil samples were collected using the metal ring method and 3 using the power probe method, maintaining a one-meter distance between methods to enable direct comparison (8 areas × 2 methods × 3 replicates). Sampling covered 4 soil depth intervals (0–10, 10–30, 30–50, and 50–100 cm). In total, 192 undisturbed soil samples were collected to estimate bulk density (BD) (Figure 1). This design resulted in 32 paired observations (metal ring vs. power probe), derived from the 8 areas and 4 depths, leading to 64 BD observations across the study - 32 measurements from each method.
The traditional metal ring core sampling method involved excavating soil pits of 1x1x1 meter (length, width and depth) to access soil at different depths (Figure 2). Soil samples were collected at 10-cm intervals up to a depth of 100 cm by inserting a metal ring (5 cm diameter x 10 cm height) vertically into the ground (Figure 2). This process ensures minimal disturbance to the surrounding soil. Samples from each 10 cm interval were then grouped into four main depth intervals (0–10 cm, 10–30 cm, 30–50 cm, and 50–100 cm) to optimize the comparative analysis of soil properties. Although labor-intensive, this method served as the reference due to its validation in previous studies and its ability to provide consistent measurements of soil BD.
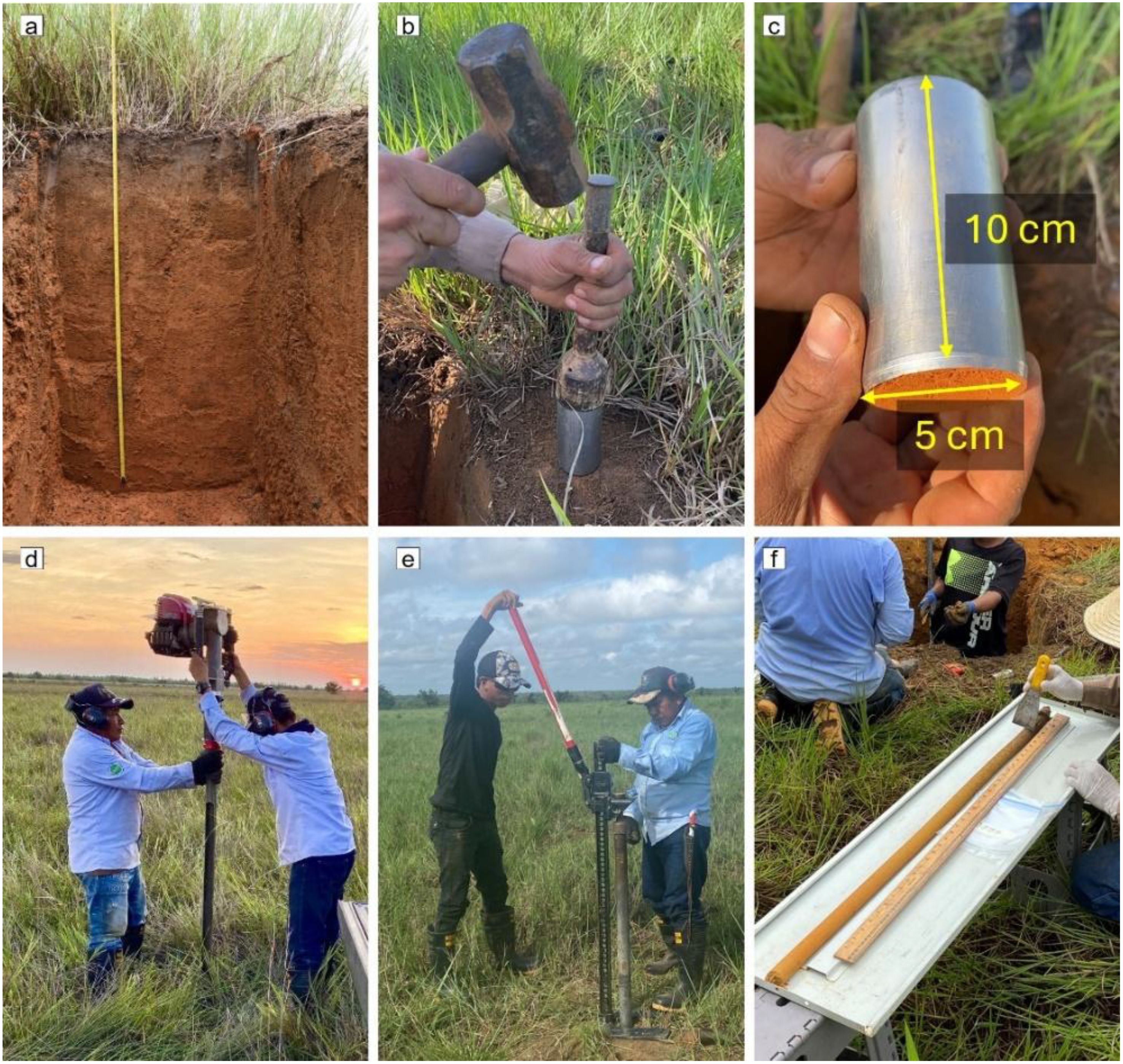
Figure 2. Photos of the metal ring (a–c) and power probe (d–f) methods used for soil sampling to measure bulk density in the two land-use types in La Primavera, Vichada, Colombia. The power probe is a mechanized tool that drives the probe into the soil using a gasoline-powered hammer, allowing the collection of intact soil cores without requiring complex excavations, as needed for sampling with the metal ring method.
The power probe used in this study is a mechanized tool driven into the soil by a gasoline-powered hammer, providing a direct push (Figure 2). Specifically, the AMS Gas Powered Core Sampling Kit was employed, consisting of a REDI Boss Hammer and slotted tubes (AMS Inc. American Falls, Idaho 83211, USA). The power probe has a diameter of 3.057 cm and allows for the extraction of undisturbed soil columns to different depths, using tubes that are connected as needed. In this case, a 1-meter-deep soil column was extracted, which was then precisely cut into four depth intervals (0-10, 10-30, 30-50, and 50–100 cm). This method facilitated efficient sampling without the need for extensive soil pit excavation.
Samples were stored in plastic bags, labeled with relevant information such as sample depth and sampling site location for identification, and sent to the Analytical Services Laboratory at the International Center for Tropical Agriculture (CIAT) in Cali, Colombia. At the laboratory, soil samples were first weighed to obtain their wet mass, and then samples were oven-dried at 100 °C to determine their dry mass. The BD value at each soil depth was calculated by dividing the dry mass of the soil by the volume of the soil ring or probe (16), allowing for accurate assessments of soil BD in relation to its structure and the extent of compaction. Soil texture analysis was carried out using the Bouyoucos hydrometer method (17). This analysis made it possible to determine the percentages of sand, silt and clay for each of the different native and cultivated pasture areas and soil depth intervals considered in this study (Table 1; Figure 2).
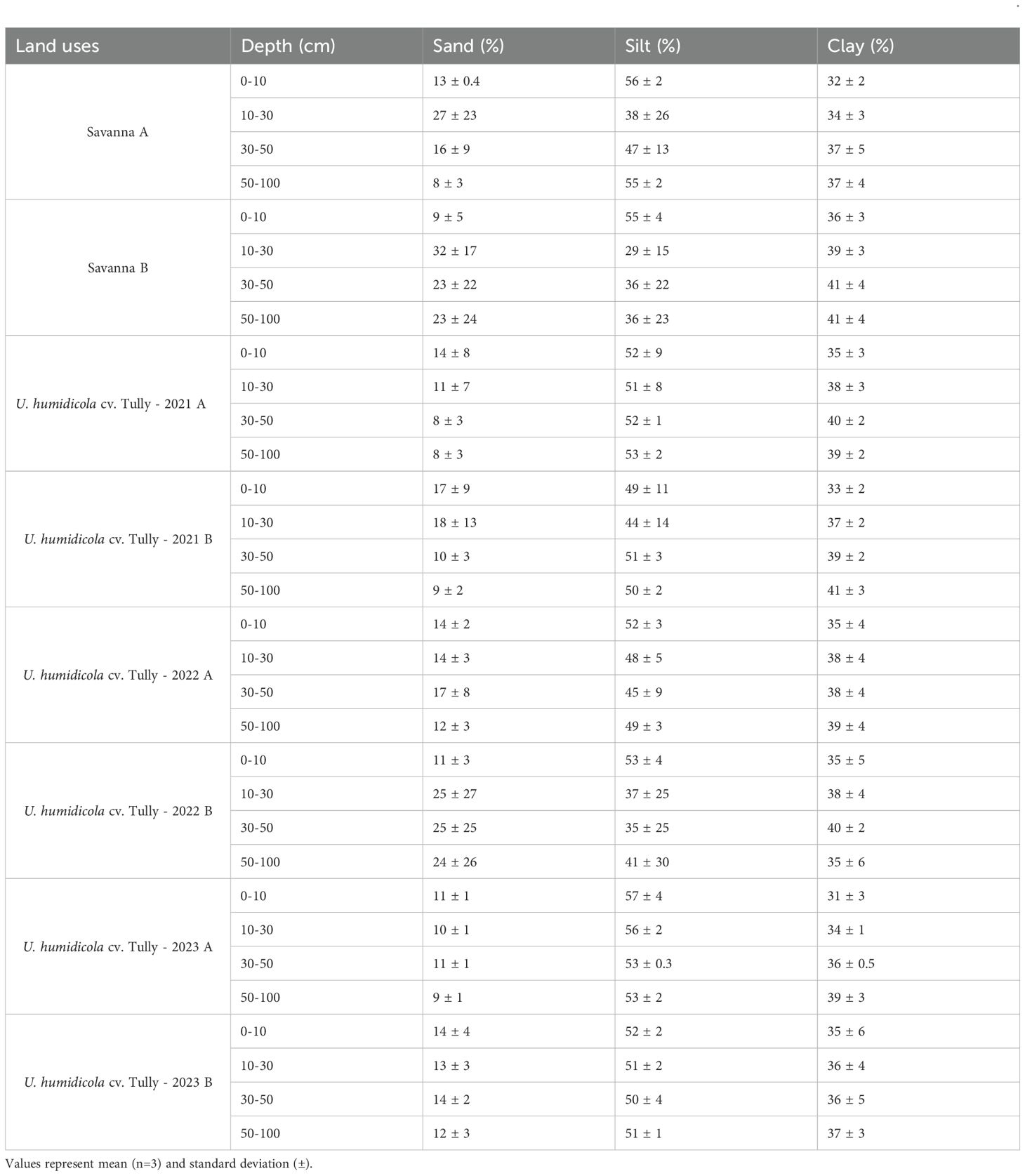
Table 1. Soil texture in different native savanna and cultivated pasture areas in the La Primavera municipality in the department of Vichada, Colombia.
2.3 Statistical analysis
Soil bulk density (BD) was analyzed separately for each soil layer and pasture system type (native and cultivated). To validate the probe method against the standard metal ring method, a paired t-test (α < 0.05) was conducted, comparing BD measurements between the two methods across 32 observations. Data normality was verified beforehand using the Shapiro-Wilk test. The performance of the probe method at each soil depth was further assessed by calculating systematic error (SyE), mean absolute error (MAE), and root mean square error (RMSE), with BD values from the metal ring method serving as the reference. SyE quantified the average bias between methods, while MAE and RMSE captured the magnitude and variability of the errors, respectively. All statistical analyses were conducted in R version 4.4.1 (18), using the packages dplyr (19), rstatix (20), and ggplot2 (21).
3 Results and discussion
Figure 3 presents the results of soil BD (g/cm³) evaluations using metal ring and probe sampling across different soil depths in native and cultivated (U. humidicola cv. Tully) pasture areas in La Primavera municipality in the department of Vichada, Colombia. The evaluations aimed to compare the reliability and effectiveness of the metal ring core (reference method) and probe methods in measuring soil BD under different land uses. The soil BD measured by the metal ring method (reference method) presented values ranging from 1.21 to 1.65 g/cm³ across the soil layers evaluated in this work (0-10, 10-30, 30–50 and 50–100 cm) with higher values observed in the deeper soil layers (Figure 3). These higher values are likely due to increased soil compaction and decreased soil organic matter content with depth, resulting in a denser soil matrix. These values are consistent with findings from previous studies that show increasing soil BD values with depth due to reduced soil organic matter and increased compaction (22–24).
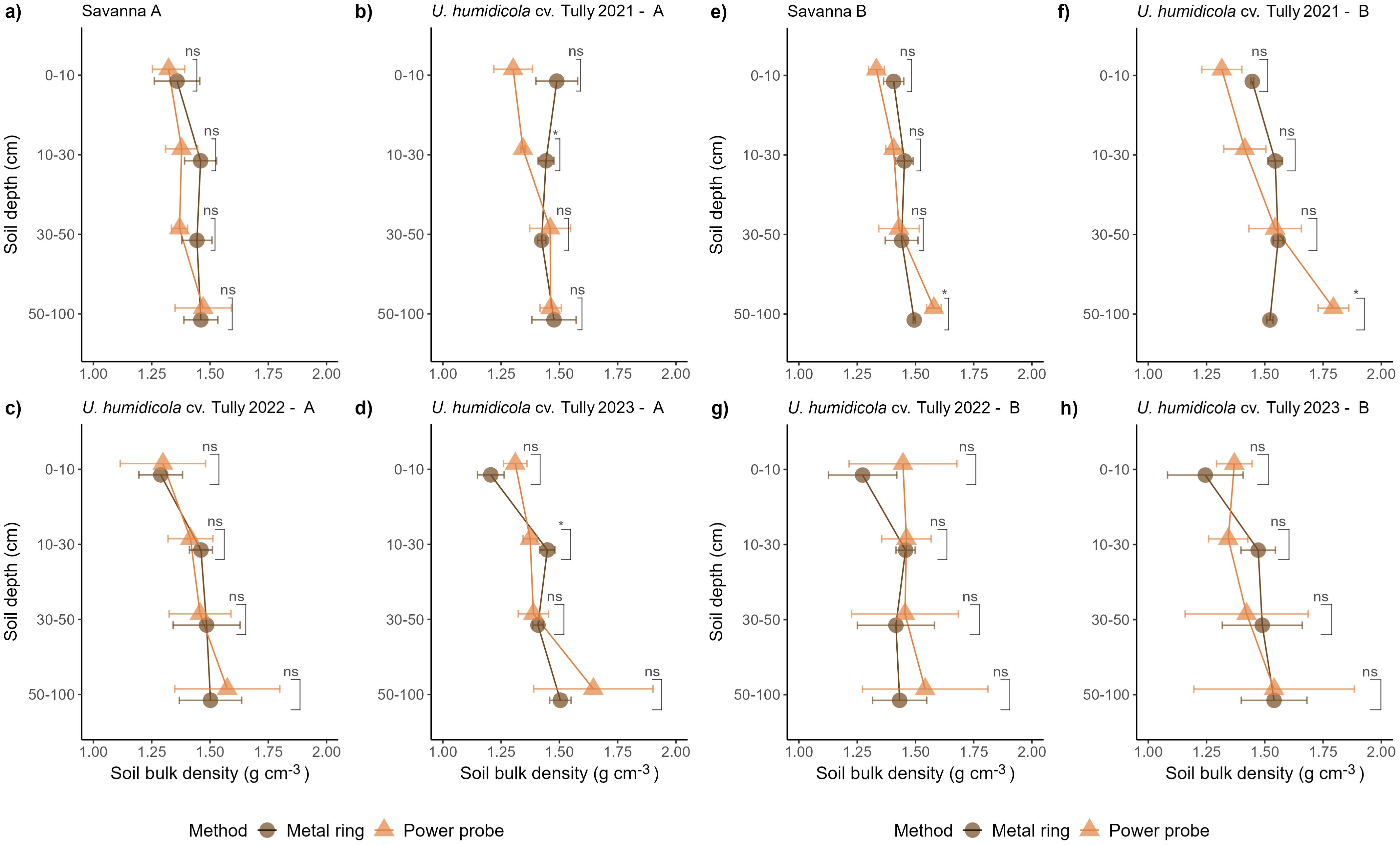
Figure 3. Comparison of soil bulk density (g/cm³) between metal ring sampling and probe sampling methods (n = 3) across different soil depths in native savanna (a, e) and cultivated pasture areas (b–d, f–h) with U. humidicola cv. Tully in the La Primavera municipality, Vichada department, Colombia.
Soil BD values also tended to increase over time after the establishment of U. humidicola cv. Tully pastures, likely driven by soil preparation practices during pasture establishment, with a notable rise in soil BD occurring within the three years after pasture establishment (Table 2). However, this compaction is supposed to decrease over time as deep-rooted forages such as U. humidicola cv. Tully contribute significantly to soil organic matter accumulation mitigating compaction and improving soil structure (25).
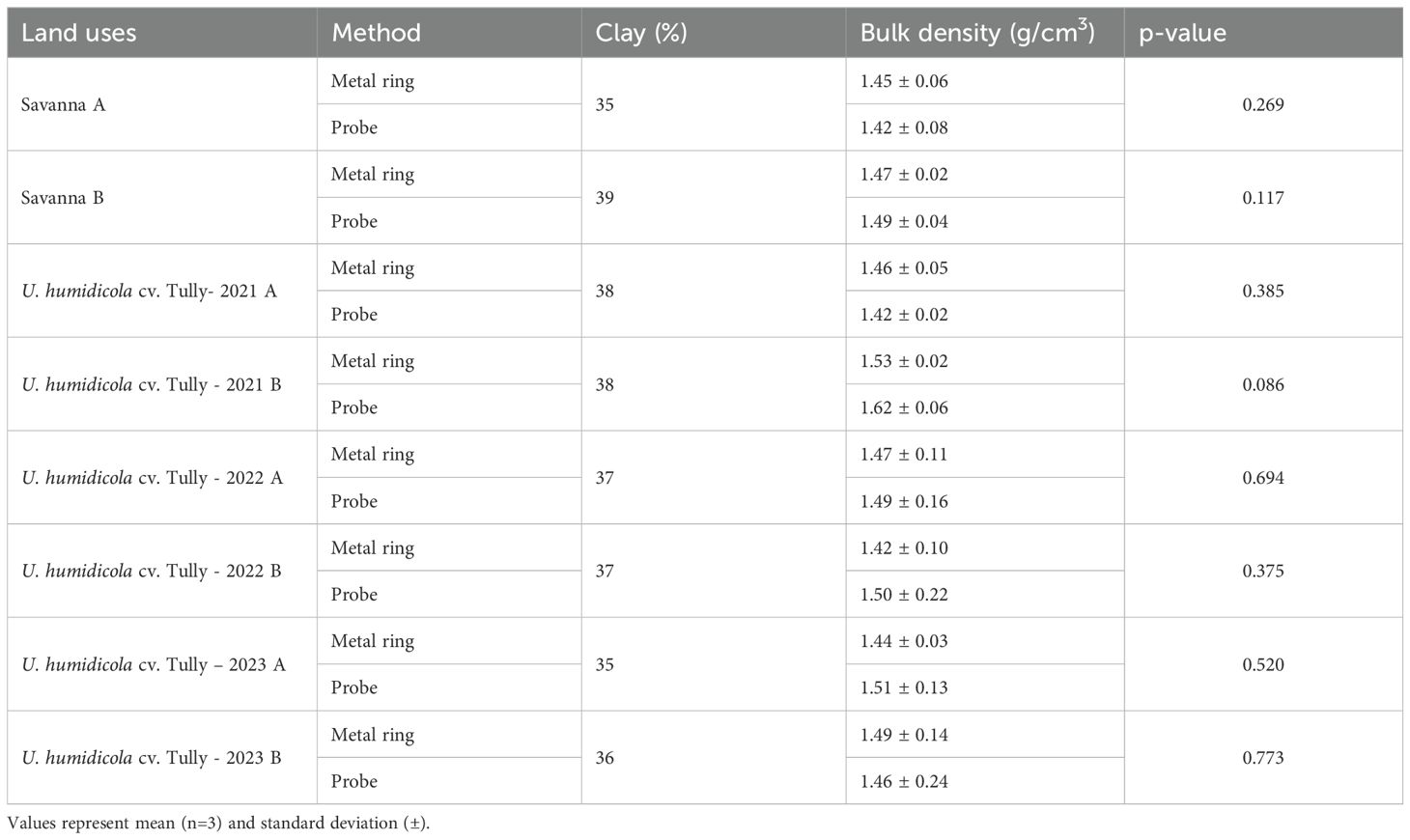
Table 2. Paired-t-test comparing soil bulk density (g/cm³) between metal ring sampling and probe sampling for the 0–100 cm soil layer in native savanna and cultivated (U. humidicola cv. Tully) pasture areas in the La Primavera municipality in the department of Vichada, Colombia.
When analyzing each soil layer individually, the differences in soil BD values between the metal ring and probe methods were minimal (Figure 3). Of the 32 observations, only four showed statistically significant differences: two at a depth of 10–30 cm in soils with 34–38% clay content, and two at 50–100 cm in soils with 41% clay content (Table 2; Figure 3). For the entire 0–100 cm soil profile, soil BD measurements using the metal ring method ranged from 1.42 to 1.53 g/cm³, while the probe method recorded values between 1.42 and 1.62 g/cm³, with no significant differences in soil BD values between the two methods (Table 2).
The minimal differences in soil BD values observed between the metal ring and probe methods across most soil layers indicate that the probe used in this study provides a reliable and efficient alternative for BD measurements in the soil type and the region evaluated. However, it is important to acknowledge the context-specific reliability of the probe method, which may not universally apply to all soil types and conditions. While a few significant differences (Figure 3) may be attributed to handling techniques during sampling, these discrepancies highlight the need for continuous improvements in operational protocols. These errors can be minimized through standardized protocols and proper training as well as increasing the number of samplings. The higher variability in measurements (Figure 3) during soil sampling with probes highlights the need for careful calibration and application, especially in soils that are prone to compaction, to ensure accurate soil BD assessments.
The probe method showed minimal overall bias across the 0–100 cm soil profile (-0.002 g/cm³) and low deviation (± 0.11 g/cm³), indicating strong agreement with the metal ring as a reference method (Table 3). However, performance varied by soil depth. The 30–50 cm soil layer demonstrated the best accuracy, with the lowest MAE (0.074 g/cm³) and RMSE (0.09 g/cm³). The 10–30 cm layer also showed good precision (MAE: 0.084 g/cm³ and RMSE: 0.097 g/cm³), despite a slight underestimation (-0.075 g/cm³). In contrast, the 0–10 cm and 50–100 cm layers exhibited greater variability, with higher MAE (0.14 g/cm³) and RMSE (0.17 g/cm³) values, and a shift to positive bias (0.085 g/cm³) in the deepest layer, which is probably associated with soil compaction or stretching during soil sampling (Table 3). Soil compaction occurs when mechanical pressure from the probe reduces pore space, increasing bulk density estimates, while soil stretching results from sample disturbance and vibrations during extraction, artificially expanding the soil structure and leading to underestimation (5).
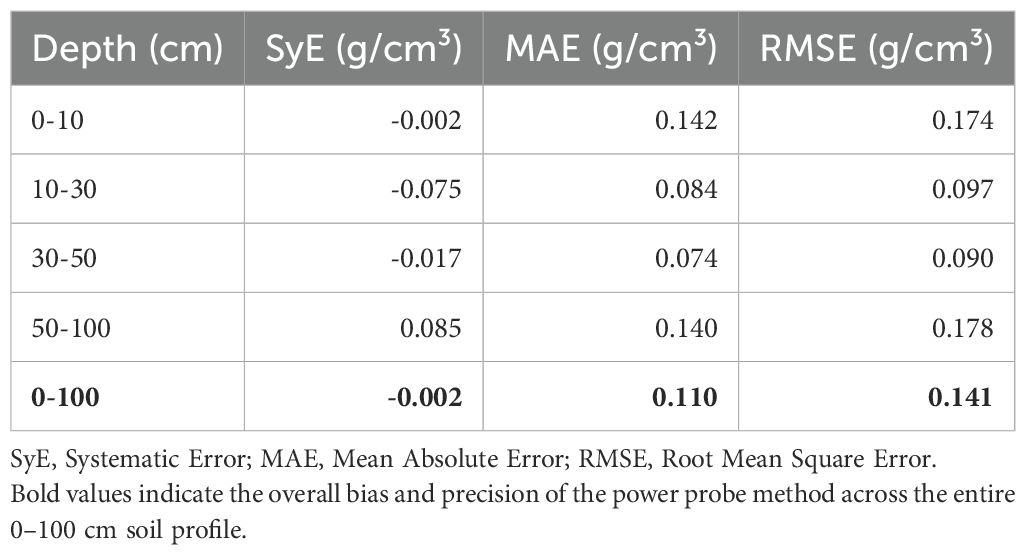
Table 3. Bias and precision analysis of the power probe method compared to the metal ring method by soil depth.
Similar to our findings, Walter et al. (5) demonstrated that both metal ring and probe methods provided reliable soil BD measurements with minimal systematic error. In clayey soils, they found that use of probes showed a systematic error of 0.05–0.08 g/cm3 compared to the reference method (metal ring), but the random error from the use of probes was 50% higher when corrected for compaction or stretching. Sample ring method proved to be the most reliable, particularly for shallow sampling, small depth increments, and repeated measurements. In contrast, probe method was more efficient for deep sampling and capturing spatial heterogeneity. Additionally, they also found that probe method facilitates BD determination without extensive soil pit excavation, potentially reducing uncertainties in SOC stock inventories, although their accuracy can vary depending on soil type and depth (5).
In another comparative study of soil sampling probes, Sharma et al. (7) found that the small-diameter probe compacted the surface soil, resulting in higher BD values than use of probes. Their study demonstrated that differences in soil BD values among probes decreased with depth and that using the equivalent soil mass correction method minimized discrepancies in SOC stocks calculation across probes, achieving consistency in 90% of the sites measured. These findings underscore the importance of probe selection for soil BD measurements, while also noting that factors such as soil depth variations, residue removal, and land management practices contribute to measurement uncertainties (7).
A potential limitation of the probe method is the diameter difference between the metal ring (5 cm) and the probe (3.057 cm), which could influence soil compaction during sampling. Although the probe method exhibited slightly higher variability — particularly in the upper (0–10 cm) and deeper (50–100 cm) soil layers — this only resulted in a deviation of ±0.11 g/cm³ across the entire soil profile (Table 3). This suggests that any compaction effect introduced by the smaller probe diameter did not substantially impact the overall BD estimates. These findings align with prior research (5, 7), which acknowledged minor compaction effects with small-diameter probes but considered them an acceptable trade-off for improved sampling efficiency in large-scale soil assessments. Nevertheless, future studies could further investigate diameter-related effects, particularly in soils with higher silt and clay content, where compaction may be more pronounced.
While our study demonstrated that the use of probe method provides an efficient alternative to the metal ring method in Oxisols and Ultisols with moderate levels of silt (29-57%) and clay content (31–41%), its application may be challenging in certain soil conditions. In high clay soils (>40%), probe insertion may induce compaction, leading to overestimation of BD. Similarly, in very dry or highly compacted soils, the probe may encounter resistance, affecting sample integrity. In sandy soils, the loose structure can lead to sample loss during extraction. Further studies should evaluate probe performance across a wider range of soil types and soil humidity conditions to refine its applicability for large-scale soil carbon monitoring (5, 7).
The overall performance of power probe method remains highly promising, particularly in terms of efficiency. In our study, the sampling time was reduced by 75% compared to the metal ring method. Based on our experience, a two-person team using the metal ring method can complete approximately four soil pits per workday. Given that our sampling required 32 pits, the full protocol took a total of eight days. In contrast, the power probe method enables up to 20 sampling points per day, allowing the same 32 points to be completed in just two days. This substantial reduction in sampling time highlights the notable operational efficiency of the power probe method, which translates into significant savings in labor and logistical costs, particularly in large-scale soil surveys where time constraints are a critical factor. While the initial investment in probe equipment (approximately USD 5,000) may be higher than that of metal rings, this cost is rapidly offset over time through reduced fieldwork durations and streamlined operations, such as minimizing soil pit excavation and eliminating the need for use of metal rings.
This time-saving advantage, combined with their cost-effectiveness, makes the use of probe method particularly valuable for large-scale soil surveys and agricultural management practices where rapid and frequent soil assessments are needed as well as those required by climate financial mechanisms, such as in carbon markets (10). However, it is important to recognize the limitations in probe performance under certain soil conditions. Although our study demonstrated that probe method performed well under our specific conditions, our field sites encompassed a relatively narrow range of variation in clay content (31-41%; Table 1). Caution should be exercised when using probes in soils with higher clay content, as this can lead to increased soil compaction and potential inaccuracies (26). This suggests that metal ring sampling may yield more accurate results on soil BD under such conditions, as it better captures the effects of soil compaction.
Therefore, to further validate the reliability of these two methods, additional comparisons are recommended to test under varying soil types and climatic conditions, and different land uses. Such studies could provide deeper insights into the performance of these measurement techniques across diverse soil types and management practices. As the effectiveness of the probe method is further refined and validated across different soil conditions, use of probe method could become an essential tool for cost-effective soil assessments and monitoring, potentially helping toward unlocking greater attention and investments in climate-smart agriculture.
4 Conclusions
This study demonstrates that both the metal ring and power probe methods are effective for measuring soil bulk density (BD), with no significant differences observed across various soil layers in Colombian pasturelands with silt content ranging from 29% to 57% and clay content ranging from 31% to 41%. The probe method offers a cost-effective and efficient alternative for large-scale soil assessments, maintaining accuracy comparable to the traditional metal ring method, with a deviation of ±0.11 g/cm³. However, the probe method showed higher variability, particularly in the 0–10 cm and 50–100 cm layers, emphasizing the importance of proper calibration and operator training to ensure consistent results.
We acknowledge the study’s limited spatial coverage and sample size. Therefore, we clarify that our findings are primarily applicable to acidic, silt- or clay-rich tropical soils and should not be extrapolated to regions with substantially different soil types such as sandy soils without further validation. We recommend additional studies across a wider range of soil textures, compaction levels, land uses, and climatic conditions to confirm the broader applicability of probe-based BD measurements.
Despite these limitations, integrating power probe-based method into soil assessments holds significant potential to streamline BD measurements, supporting agricultural management, carbon monitoring, and larger-scale climate finance initiatives.
Data availability statement
The raw data supporting the conclusions of this article will be made available by the authors, without undue reservation.
Author contributions
LR: Investigation, Writing – original draft, Writing – review & editing, Data curation, Formal analysis, Methodology. MB: Writing – original draft, Writing – review & editing, Data curation, Formal analysis, Investigation, Methodology. MS: Data curation, Funding acquisition, Investigation, Resources, Supervision, Writing – original draft, Writing – review & editing. JuA: Writing – original draft, Writing – review & editing, Formal analysis, Investigation, Methodology. JM-L: Writing – original draft, Writing – review & editing, Methodology. NM-R: Writing – original draft, Writing – review & editing, Methodology. SL: Writing – original draft, Writing – review & editing, Data curation, Formal analysis, Investigation, Methodology, Validation. IR: Writing – original draft, Writing – review & editing, Conceptualization, Data curation, Formal analysis, Investigation, Methodology, Supervision, Validation. JA: Writing – original draft, Writing – review & editing, Conceptualization, Funding acquisition, Methodology, Resources, Supervision, Validation. CCJ: Conceptualization, Data curation, Investigation, Methodology, Supervision, Writing – original draft, Writing – review & editing, Project administration, Validation, Visualization.
Funding
The author(s) declare that financial support was received for the research and/or publication of this article. This work was carried out as part of the CGIAR Initiative Livestock & Climate (L&C) and the CGIAR Science Program on Climate Action (CA) and Sustainable Animal & Aquatic Foods (SAAF). We thank all donors who globally support our work through their contributions to the CGIAR Trust Fund. CGIAR is a global research partnership for a food-secure future, dedicated to transforming food, land, and water systems in a climate crisis. We also acknowledge the support of the Bezos Earth Fund project “Using genetic diversity to capture carbon through deep root systems in tropical soils”.
Conflict of interest
The authors declare that the research was conducted in the absence of any commercial or financial relationships that could be construed as a potential conflict of interest.
Generative AI statement
The author(s) declare that no Generative AI was used in the creation of this manuscript.
Publisher’s note
All claims expressed in this article are solely those of the authors and do not necessarily represent those of their affiliated organizations, or those of the publisher, the editors and the reviewers. Any product that may be evaluated in this article, or claim that may be made by its manufacturer, is not guaranteed or endorsed by the publisher.
References
2. De Vos B, Cools N, Ilvesniemi H, Vesterdal L, Vanguelova E, Carnicelli S. Benchmark values for forest soil carbon stocks in Europe: Results from a large scale forest soil survey. Geoderma. (2015) 251-252:33–46. doi: 10.1016/j.geoderma.2015.03.008
3. Paustian K, Larson E, Kent J, Marx E, Swan A. Soil C sequestration as a biological negative emission strategy. Front Climate. (2019) 1:8. doi: 10.3389/fclim.2019.00008
4. Nelson DW, Sommers LE. Total carbon, organic carbon, and organic matter. In: Sparks DL, editor. Methods of Soil Analysis: Part 3 - Chemical Methods. Soil Science Society of America (1996). p. 961–1010. doi: 10.2136/sssabookser5.3.c34
5. Walter K, Don A, Tiemeyer B, Freibauer A. Determining soil bulk density for carbon stock calculations: a systematic method comparison. Soil Sci Soc America J. (2016) 80:579–91. doi: 10.2136/sssaj2015.11.0407
6. Al-shammary AAG, Kouzani AZ, Kaynak A, Khoo SY, Norton M, Gates W. Soil bulk density estimation methods: A review. Pedosphere. (2018) 28:581–96. doi: 10.1016/S1002-0160(18)60034-7
7. Sharma S, Wilson T, Ochsner T, Warren JG. Sampling probes affect bulk density and soil organic carbon measurements. Agric Environ Lett. (2020) 5(1):e20005. doi: 10.1002/ael2.20005
8. Pepers KHJ, Koomans R, Teuling K, Staats G. Validation of a new gamma ray soil bulk density sensor. Eur J Soil Sci. (2024) 75:e13542. doi: 10.1111/ejss.13542
9. Baker R, Carter MR, Gregorich EG. Soil Sampling and Methods of Analysis. 2nd ed. Boca Raton, FL: CRC Press (2006). doi: 10.1201/9781420005271
10. Verra. VCS Program Guide: Verified Carbon Standard for Agricultural Projects (2022). Available online at: https://www.verra.org (Accessed October 1, 2024).
11. Carter MR, Gregorich EG. Soil Sampling and Methods of Analysis. 2nd ed. Boca Raton, FL: CRC Press (2008). doi: 10.1201/9781420005271
12. Soil Science Society of America. Soil Science Glossary. Madison, WI: Soil Science Society of America (2015).
13. Institute of Hydrology, Meteorology and Environmental Studies of Colombia (IDEAM). Citizen services. IDEAM (2024). Available at: http://dhime.ideam.gov.co/atencionciudadano/ (Accessed October 1, 2024).
14. Instituto Geográfico Agustín Codazzi (IGAC). Suelo y tierras de Colombia. Sixth ed. Bogota, Colombia: Editorial IGAC (2014).
15. Soil Survey Staff. Soil taxonomy: A basic system of soil classification for making and interpreting soil surveys. 2nd edition. Washington, DC: Natural Resources Conservation Service. U.S. Department of Agriculture (1999). p. 436.
16. Blake GR. Bulk density. En: Black CA, editor. Methods of soil analysis: Part I Physical and mineralogical properties, including statistics of measurement and sampling. Madison, WI: American Society of Agronomy (1965) p. 374–390.
17. Bouyoucos GJ. Directions for making mechanical analyses of soils by the hydrometer method. Soil Sci. (1936) 42(3):225–230. doi: 10.1097/00010694-193609000-00007
18. R Development Core Team. R: A Language and Environment for Statistical Computing. Vienna, Austria: R Foundation for Statistical Computing (2024).
19. Wickham H, François R, Henry L, Müller K. dplyr: A Grammar of Data Manipulation. R package version 1.1.3 (2023). Available online at: https://CRAN.R-project.org/package=dplyr (Accessed October 1, 2024).
20. Kassambara A. rstatix: Pipe-Friendly Framework for Basic Statistical Tests. Version 0.7.2 (2023). Available online at: https://CRAN.R-project.org/package=rstatix (Accessed October 1, 2024).
21. Wickham H, Chang W, Henry L, Pedersen TL, Takahashi K, Wilke C, et al. ggplot2: create elegant data visualisations using the grammar of graphics. R package version 3.5.1. (2022). doi: 10.32614/CRAN.package.ggplot2.
22. Costa C Jr., Villegas D, Bastidas M, Rubio N, Rao I, Arango J. Soil carbon stocks and nitrous oxide emissions of pasture systems in Orinoquía Region of Colombia: Potential for developing land-based greenhouse gas removal projects. Front Climate. (2022) 4:916068. doi: 10.3389/fclim.2022.916068
23. Hillel D. Introduction to Environmental Soil Physics. San Diego: Elsevier Academic Press (2004), 494. doi: 10.1016/B978-012348655-4/50000-9
24. Weil RR, Brady N. The Nature and Properties of Soils. 15th edition. Columbus: Pearson (2016). Available at: http://lccn.loc.gov/20160085.
25. Ayarza MA, Rao I, Vilela L, Lascano C, Vera-Infanzón R. Soil carbon accumulation in crop-livestock systems in acid soil savannas of South America: A review. Adv Agron. (2022) 173:163–226. doi: 10.1016/bs.agron.2022.02.003
Keywords: soil bulk density, sampling methods, grasslands, probes, large-scale assessments
Citation: Rodriguez L, Bastidas M, Da Silva M, Arias J, Martín-López JM, Matiz-Rubio N, Loaiza S, Rao I, Arango J and Costa Junior C (2025) Comparative assessment of soil bulk density measurements using core metal ring and power probe methods in acidic soils of Colombian pasturelands. Front. Soil Sci. 5:1527807. doi: 10.3389/fsoil.2025.1527807
Received: 13 November 2024; Accepted: 03 April 2025;
Published: 06 May 2025.
Edited by:
Emmanuel Arthur, Aarhus University, DenmarkReviewed by:
Peter Ogban, University of Calabar, NigeriaDiana Vigah Adetsu, Aarhus University, Denmark
Copyright © 2025 Rodriguez, Bastidas, Da Silva, Arias, Martín-López, Matiz-Rubio, Loaiza, Rao, Arango and Costa Junior. This is an open-access article distributed under the terms of the Creative Commons Attribution License (CC BY). The use, distribution or reproduction in other forums is permitted, provided the original author(s) and the copyright owner(s) are credited and that the original publication in this journal is cited, in accordance with accepted academic practice. No use, distribution or reproduction is permitted which does not comply with these terms.
*Correspondence: Ciniro Costa Junior, Yy5jb3N0YWpyQGNnaWFyLm9yZw==