- 1Inner Mongolia Kunming Cigarette Limited Liability Company, Hohhot, China
- 2Inner Mongolia Autonomous Region Tobacco Company Chifeng Company, Chifeng, China
- 3Tobacco Research Institute of Chinese Academy of Agricultural Sciences, Qingdao, China
Introduction: Excessive chloride ion content in tobacco leaves adversely affects the flammability of flue-cured tobacco, particularly in tobacco-growing regions of northern China, where this issue is more pronounced. Consequently, this poses a challenge for finding economically and environmentally friendly control methods. Vibrio natriegens, as a non-pathogenic, moderately halophilic bacterium, can reduce chloride ion content in soil. Zn²+, as a chloride ion channel inhibitor, can effectively reduce the accumulation of chloride ions in plants.
Methods: In this study, we established two experimental groups, T1 and T2, where T1 contained V. natriegens as a chloride ion inhibitor and T2 contained Zn²+ as a chloride ion inhibitor. We investigated the effects of T1 and T2 on chloride ion content in tobacco, soil environmental factors, and microbial community structure. Various methods were employed to measure soil physicochemical properties, and the impact of different treatments on microbial community structure was elucidated through soil microbial DNA extraction, PCR amplification, PE library construction, Illumina sequencing, and subsequent bioinformatics analysis.
Results and discussion: The results indicate that both inhibitors significantly reduced chloride ion levels in tobacco (p < 0.05) and altered the abundance of certain soil microorganisms. Specifically, the chloride ion content in upper leaves decreased from 1.33% to 0.83% under T1 treatment, while the T2 experimental group showed a reduction to 0.99%. Additionally, we evaluated the agronomic traits of tobacco plants and found that the application of chloride ion inhibitors promoted plant growth. Field trials further validated the efficacy of these inhibitors, with treated tobacco leaves exhibiting significantly lower chloride ion content compared to the control group. This study, based on the source of chloride ion uptake by tobacco plants from soil, employed strategies to control soil chloride ion content or reduce plant uptake, thereby effectively managing chloride ion levels in tobacco leaves. This provides an effective strategy for controlling chloride ion content in tobacco leaves, contributing to the high-quality development of tobacco production.
1 Introduction
Chloride, an essential element, serves to maintain electrical neutrality in the extra-cellular fluid. It works synergistically with sodium to maintain osmotic pressure balance and collaborates with bicarbonate to upload acid-base balance (1). As the most abundant anion in living organisms, chloride ions play diverse crucial roles in organism function, including controlling membrane potential and preserving intracellular acid-base balance.
Undoubtedly, chloride is a vital nutrient for plants (2). On one hand, chloride ions significantly influence plant biomass, leaf size and quantity, as well as root morphology and distribution. Generally, the presence of chloride ions positively affects the normal growth and development of plants, resulting in increased biomass, yield, and quality. Moreover, chloride ions contribute positive to the overall growth and development of plants. On the other hand, the concentration of chloride ions can impact the stomata of the plant, thereby affecting photosynthesis (3, 4). Excessive concentration of chloride ions can become toxic, leading to cellular dehydration, diminished stomatal conductance, and consequently limiting net photosynthesis. This toxicity may also induce hormonal imbalances, inactivate ribosomal enzymes, cause photoinhibitory damage, and disrupt mitochondrial respiration, ultimately resulting in impaired growth or even plant mortality (4, 5). Furthermore, the regulation of chloride ion flux is essential for the proper development of pollen tubes. Increased chloride ion concentrations, which possess a negative charge, can alter the electric field surrounding pollen tubes, thereby influencing the direction growth (6). Cation-chloride cotransporters facilitate the active uptake of chloride ions from the root xylem into the plant tissue, a process that is closely linked to the plant’s salt tolerance mechanisms (7–9). To optimize plant growth, it is crucial to provide both Cl− and NO3–N simultaneously, as their synergistic interaction improves the efficiency of water and nitrogen utilization within plants. Additionally, specific chloride (Cl−) transporter protein genes, such as ZmNPF 6.4 and AtSLAH3, have been identified as key player in the processes of nutrient uptake and long-distance translocation within plants (10).
Tobacco, a significant cash crop cultivated extensively in the world, exhibits high sensitivity to Cl− (11). Optimal levels of Cl− not only promote tobacco growth and increase yields, but also alleviate oxidative stress induced by cadmium toxicity. This mitigation is achieved by reducing cadmium accumulation within the plant while enhancing the activity of antioxidant enzymes (12). Conversely, an excessive concentration of Cl− in tobacco can detrimentally affect the plant by inducing water loss, significantly impairing photo-synthesis, and inhibiting the biosynthesis of growth regulators like hormones and gibberellins (11). Historically labeled as a “chlorine-avoiding crop”, tobacco manifests symptoms of leaf poisoning upon surpassing a critical threshold of chlorine ions, resulting in leaf hypertrophy and deformities that compromise cigarette flavor and combustibility, increasing the likelihood of flameout (13). Chlorine content in tobacco is intimately linked to soil chloride levels and influenced by irrigation water quality, fertilizer application, and preceding crops (14). Therefore, Currently, various strategies have been devised to manage chloride ions in tobacco cultivation. These include intercropping techniques, development of low Cl− uptake cultivars, adoption of suitable planting systems, and judicious use of microbial fertilizers. Noteworthy among emerging techniques is the utilization of ion-exchange columns containing anion-exchange resins to curtail chloride accumulation in tobacco plants during irrigation (15, 16). However, the intricate nature of field irrigation water systems harboring diverse anions may diminish the efficacy of Cl− adsorption by resins (17). Although intercropping with chlorine-tolerant species diminishes Cl− uptake by tobacco to some extent, it adversely impacts overall cash crop yields, thus posing economic challenges (18). Given the limitations of existing technologies and the challenges in practical applications, developing an efficient, economical, and easily implementable method for chloride ion management is of paramount importance. Chloride ion inhibitors can effectively address the gaps in current technologies, offering a more efficient and cost-effective solution for tobacco cultivation.
Zn²+ functions as an anion channel inhibitor, effectively suppressing the uptake of anions by plants from the soil, a mechanism that has been experimentally confirmed in Arabidopsis thaliana (19, 20). In tobacco studies, Zn²+ exhibits antioxidant effects (21), promotes nitrate nitrogen uptake (22), and reduces chloride ion accumulation in tobacco leaves, thereby alleviating the toxic effects of chloride ions on tobacco (23). However, research on the application of Zn²+ as a chloride ion inhibitor in the Haradaokou tobacco-growing region of Chifeng remains limited. Moreover, excessive zinc can accumulate in plant tissues, leading to phytotoxicity symptoms, and high concentrations of zinc may interfere with the uptake and utilization of other essential nutrients by plants (24). Additionally, high levels of zinc ions can negatively impact soil microbial communities, reducing the efficiency of nutrient cycling and soil organic matter decomposition (25). In contrast, the use of microbial inoculants does not exhibit significant adverse effects on soil or microbial communities. Studies have shown (26) that the additional application of microorganisms can significantly enhance the yield and quality of flue-cured tobacco. Specifically, the applied microorganisms provide sufficient nutrients to tobacco leaves through mechanisms such as biological nitrogen fixation, phosphorus solubilization, and potassium release, thereby promoting leaf growth, development, and photosynthesis, ultimately improving tobacco yield and quality. Research has also demonstrated that the cell mass of halophilic bacteria is related to chloride ion concentration, and their chloride ion uptake often increases with accelerated growth rates (27). The moderately halophilic bacterium Vibrio costicola can increase organic anions within cells to counteract the toxicity caused by high intracellular chloride ion concentrations (28), and all halophilic bacteria possess inwardly oriented light-driven Cl− pumps (29). Vibrio natriegens (V. natriegens), as a non-pathogenic, moderately halophilic bacterium (30), is characterized by its rapid growth rate, low mutation rate, and natural presence in high-sodium and high-chlorine marine environments (30, 31) (32) (33). Although no studies have shown that V. natriegens can reduce chloride ion content in plants, we hypothesize, based on the ability of halophilic bacteria to increase chloride ion uptake with faster growth rates and their capacity to elevate intracellular organic anions to resist chloride ion toxicity, that V. natriegens could serve as a chloride ion inhibitor. This approach would effectively save energy and time costs while reducing soil chloride ion concentration through its own metabolism, thereby decreasing chloride ion content in tobacco leaves. This holds significant economic potential (31).
In this study, we identified a novel strain inhibitor (V. natriegens) and a reagent (Zn2+) that effectively attenuates chloride uptake in tobacco. Employing high-throughput sequencing techniques for16S rRNA and ITS rRNA genes, we conducted a comprehensive analysis of the microbial community’s abundance, diversity, and composition. Additionally, we explored the intricate network of interactions between the rhizosphere soil environment, the associated microbial community, and the dynamics of soil chloride ions in relation to these uptake inhibitors. We hypothesized that the introduction of chloride uptake inhibitors during the vigorous growth phase of tobacco plants would significantly diminish chloride ions absorption, thereby decreasing the chloride concentration within the tobacco leaves. This study is underpinned by two primary objectives: (1) to elucidate a new class of tobacco chloride ion uptake inhibitors characterized by ease of application, cost-effectiveness, environmental sustainability, and potential for widespread dissemination in agricultural settings; and (2) to examine the correlations between soil properties and microbial communities in response to the application of different chloride ion inhibitors.
2 Materials and methods
2.1 Description of study site
The pot trial took place at the Qingdao Experimental Base of the Tobacco Research Institute, Chinese Academy of Agricultural Sciences, situated in Shimen Village, Longquan Street, Jimo District, Qingdao City, Shandong Province, China. The precise coordinates for the location are 120°58’E and 36°44’N.
2.2 Experimental design and treatment
The pot experiment was conducted in a greenhouse at the experimental base, using tobacco plants of similar quality and seedling age that were at the vigorous growth stage. The experiment included one control group and two experimental groups. The CK did not receive any chloride ion inhibitors and was only treated with 100 mL of sterile water. The experimental group T1 was treated with a chloride ion inhibitor containing V. natriegens. Based on preliminary dose-gradient experiments, considering time, chloride ion content in tobacco leaves, and economic efficiency, we found that inoculating each tobacco plant with 100 mL of V. natriegens solution at OD=1.0, with an inoculation concentration of 6.4×108 CFU/mL, yielded the best control effect on chloride ions in tobacco leaves. The experimental group T2 was treated with a chloride ion inhibitor containing Zn²+. Preliminary experiments indicated that applying ZnSO4·7H2O at a concentration of 600 μmol/L achieved optimal control of chloride ions in tobacco leaves. Therefore, the T2 group was treated with 100 mL of ZnSO4·7H2O solution at this concentration. Fertilization and agronomic management practices for both experimental and control groups were consistent with those at the Haladaokou Tobacco Station. Each treatment group in the pot experiment consisted of three replicates, with each replicate containing five pots, resulting in a total of 15 pots per treatment group. The experimental containers were plastic pots with an inner diameter of 40 cm and a height of 35 cm, each filled with 10.0 kg of loam soil.
2.3 Soil sample collection and soil physicochemical analysis
Fifteen well-grown tobacco plant soils were randomly selected for each treatment and control group, and well-grown plants were randomly selected for the test, and inter-root soils in the 10-20 cm soil layer were selected, collected along the plant roots with an earth auger, removed with a sterile brush and placed in a sampling bag, and transported to the laboratory chilled in ice packs and stored at -80 °C for testing (34). Nine samples were collected at a time and evenly mixed into thirds to ensure homogeneity of the collected samples. The obtained potting soil was screened through a 60-mesh sieve (0.25 mm diameter) to avoid the presence of soot, debris, etc. in the test soil, and the filtered soil was stored in an ultra-low temperature refrigerator at -80°C (35, 36). The samples were then transported to the laboratory and stored in ice packs. We utilized a glass electrode digital pH meter to blend 10 g of air-dried soil with 25 mL of distilled water for pH determination in the sample. Soil quick-acting phosphorus (AP) was extracted using 0.5 M sodium bicarbonate and quantified through the molybdenum blue colorimetric method. Leaching of soil with ammonium acetate and measurement of available potassium (AK) content using flame photometry (37). Soil chloride content was measured by titrating soil leachate for chloride ions using silver nitrate according to the Agricultural Industry Standard of the People’s Republic of China (NY/T 1378-2007). Measurement of nitrate nitrogen (NO3–N) by phenol-2,4-disulfonic acid colorimetric method; measurement of soil ammonium nitrogen (NH4+-N) by KCl leaching-indophenol blue colorimetric method.
2.4 Tobacco sample collection
For each treatment and control group, five leaves from each of the upper and middle and lower parts of the tobacco plant with good and uniform growth were selected, placed in sampling bags and transported to the laboratory refrigerated in ice packs for immediate drying.
2.5 Analysis of soil microbial diversity
Detection of soil microorganisms involved several steps, including soil collection, DNA extraction, PCR amplification, PE library construction, Illumina sequencing, and subsequent biological analyses. Soil DNA was extracted from microbial communities using the DNeasy® PowerSoil® Pro Kit (QIAGEN, United States). The DNA was assessed for purity and concentration using a NanoDrop 2000 spectrophotometer (Thermo Fisher Scientific, United States), and its integrity was evaluated through 1% agarose gel electrophoresis. The highly variable region of bacterial 16S rRNA (V3-V4) was amplified using primers 338F (5’-ACTCCTACGGGGAGCAGCAGC-3’) and 806R (5’-GGACTACHVGGGTWTCTAAT-3’) (38). PCR amplification system: 4 µL of 5×FastPfu Buffer, 2 µL of 2.5 mM dNTPs, 0.8 µL of up-stream primer (5 µM), 0.8 µL of downstream primer (5 µM), 0.4 µL of FastPfu DNA Polymerase, 0.2 µL of BSA, 10 ng of genomic DNA, and ddH2O up to 20 µL. The PCR conditions included an initial denaturation at 95°C for 3 min, followed by denaturation at 95°C for 30 s, extension at 72°C for 45 s, a final extension at 72°C for 10 min, and storage at 10°C. The total number of cycles conducted was 30.
PCR products were purified and mixed proportionally using the AxyPrep DNA Gel Extraction Kit and subsequently sequenced using Illumina’s Miseq PE300 platform. The raw data underwent quality checks using FASTP software and were merged with FLASH software based on the following criteria: (1) Trimming reads at positions where the aver-age quality score within a 50 bp sliding window dropped below 20. (2) Merging sequences with overlapping stack lengths longer than 10 bp while allowing up to 2 bp mismatches. (3) Combining sequences according to barcodes (exact matches) and primers (allowing for 2-nucleotide mismatches). Subsequently, sequences from each sample were separated, and reads containing ambiguous bases were excluded (39, 40).
The coverage of the microbial community analysis exceeded 99%, essentially encompassing all bacterial species present in the soil. The average sequencing depth for the control group was over 1200 reads. For the T1 experimental group, the average sequencing depth was over 1400 reads, while for the T2 experimental group, it was over 1000 reads.
2.6 Bioinformatics, data analysis and statistical analysis
Use fastp (41) The software performs quality control of the paired-end raw sequencing sequence, using FLASH (39) The software performs splicing: (1) filter the bases below 20 in the tail of reads, set a window of 50bp, if the average quality value in the window is lower than 20, cut off the back-end bases from the window, filter the reads below 50bp after quality control, and remove the reads containing N bases; (2) According to the overlap relationship between PE reads, the pairs of reads are spliced into a sequence, and the minimum overlap length is 10bp; (3) The maximum allowable mismatch ratio of the overlap region of the splicing sequence is 0.2 to screen the non-conforming sequences; (4) The samples are distinguished according to the barcode and primers at the beginning and end of the sequence, and the sequence orientation is adjusted, the number of mismatches allowed by the barcode is 0, and the maximum number of primer mismatches is 2.
Using UPARSE v7.1 (42) (http://drive5.com/uparse/), operational taxonomic units (OTUs) were clustered at 97% similarity from the quality-controlled and merged sequences, and chimeras were removed. Sequences annotated as chloroplast or mitochondrial origins were excluded from all samples. To minimize the impact of sequencing depth on subsequent alpha and beta diversity analyses, the sequence count for all samples was normalized to 20,000. After normalization, the average sequence coverage for each sample remained at 99.09%. Taxonomic annotation of OTUs was performed using the RDP classifier (43) (http://rdp.cme.msu.edu/, version 2.11) against the Silva 16S rRNA gene database (v138) with a confidence threshold of 70%, and the community composition of each sample was statistically analyzed at different taxonomic levels. Functional prediction analysis of 16S data was conducted using PICRUSt2 (version 2.2.0) (44).
Rarefaction curve analysis was used to assess whether the sequencing depth was sufficient. The curves plateaued at their ends, indicating that the sequencing depth was adequate to cover the major microbial community composition in the samples. Alpha diversity knowledge Chao 1, Shannon index, etc. were calculated using mothur software, and Wilxocon rank sum test was used for the analysis of between-group differences in Alpha diversity; PCoA analysis based on the braycurtis distance algorithm was used to test the similarity of microbial community structure between samples, and combined with the PERMANOVA non-parametric test to analyze whether the differences in microbial community structure between sample groups were significant or not (45). LEfSe analysis (46) (http://huttenhower.sph.harvard.edu/LEfSe) (LDA > 2, P < 0.05) was employed to identify bacterial taxa with significantly different abundances from the phylum to genus levels among different groups. Distance-based redundancy analysis (db-RDA) was used to investigate the influence of soil physicochemical properties/clinical indicators on soil/gut bacterial community structure. Linear regression analysis was applied to assess the impact of key soil physicochemical properties/clinical indicators identified in the db-RDA analysis on microbial alpha diversity indices (47). Significant differences were analyzed using analysis of variance (ANOVA), where 0.01 < P ≤ 0.05 was marked as *, 0.001 < P ≤ 0.01 as **, 0.001 < P ≤ 0.0001 as *** and P ≤ 0.0001 as ****. Alternatively, the same letters indicate no significant difference (P > 0.05), different letters indicate significant differences (0.01 < P ≤ 0.05), and more distinct letters indicate highly significant differences (P ≤ 0.01).
2.7 The field validation experiment
The field validation experiment was conducted at the Haladaokou Tobacco Station experimental field in Hongshan District, Chifeng City, Inner Mongolia Autonomous Region, China. The tobacco varieties, quality, and seedling age used were similar to those in the pot experiment. The experiment included three treatments: a CK, FT1, and FT2, with three replicates each. Each replicate consisted of 30 tobacco plants. In FT1, the concentration of V. natriegens was 6.4×108 CFU/mL, while in FT2, the concentration of ZnSO4·7H2O was 600 μmol/L. Chloride ion inhibitors were applied during the early vigorous growth stage, and chloride ion content in the soil was measured during the late vigorous growth stage and the maturation stage, as well as in the leaves during the maturation stage.
3 Results
3.1 Chloride ion contents in different leaf segments of tobacco
The experiment results illustrated in Figure 1 revealed that the chlorine ion content in various leaf segments of tobacco (upper leaves, middle leaves, and lower leaves) exhibited distinct responses to treatments with T1 and T2, resulting in differential impacts on chlorine ion uptake by the tobacco plants. As shown in the figure, the chloride ion content in tobacco leaves from the experimental groups was significantly lower than that in the control group, particularly in the upper leaves, with values of 0.99% (T2), 0.83% (T1), and 1.33% (CK). Both the T1 and T2 experimental groups exhibited highly significant differences compared to the CK. These treatments resulted in varying effects on chloride ion concentrations across the three leaf positions, with the most significant impact observed in the upper leaves, followed by the middle leaves, and a relatively minor effect on the lower leaves. In the upper leaves, the inhibitory effect of the T1 experimental group was superior to that of the T2 experimental group, whereas in the middle leaves, the T2 experimental group showed better inhibition than the T1 experimental group. The inhibitory effects on the lower leaves were relatively consistent between the two groups. Additionally, significant differences (P ≤ 0.01) in chloride ion levels were observed between the two experimental groups in the upper and middle leaves, but no significant differences were found in the lower leaves. Overall, both inhibitor experimental groups showed discernible influences on chlorine ion absorption in tobacco leaves. Additionally, the growth performance of tobacco plants was optimal in T1 group, followed by T2 group, both surpassing the control group, as detailed in Table 1. The maximum leaf length in T1 group has achieved 63.1 cm, contrasting significantly with 56.3 cm of the control group. Moreover, the maximum leaf width also surged to 22.5 cm from 17.7 cm, thereby illustrating the augmented morphological attributes facilitated by the experimental treatments.
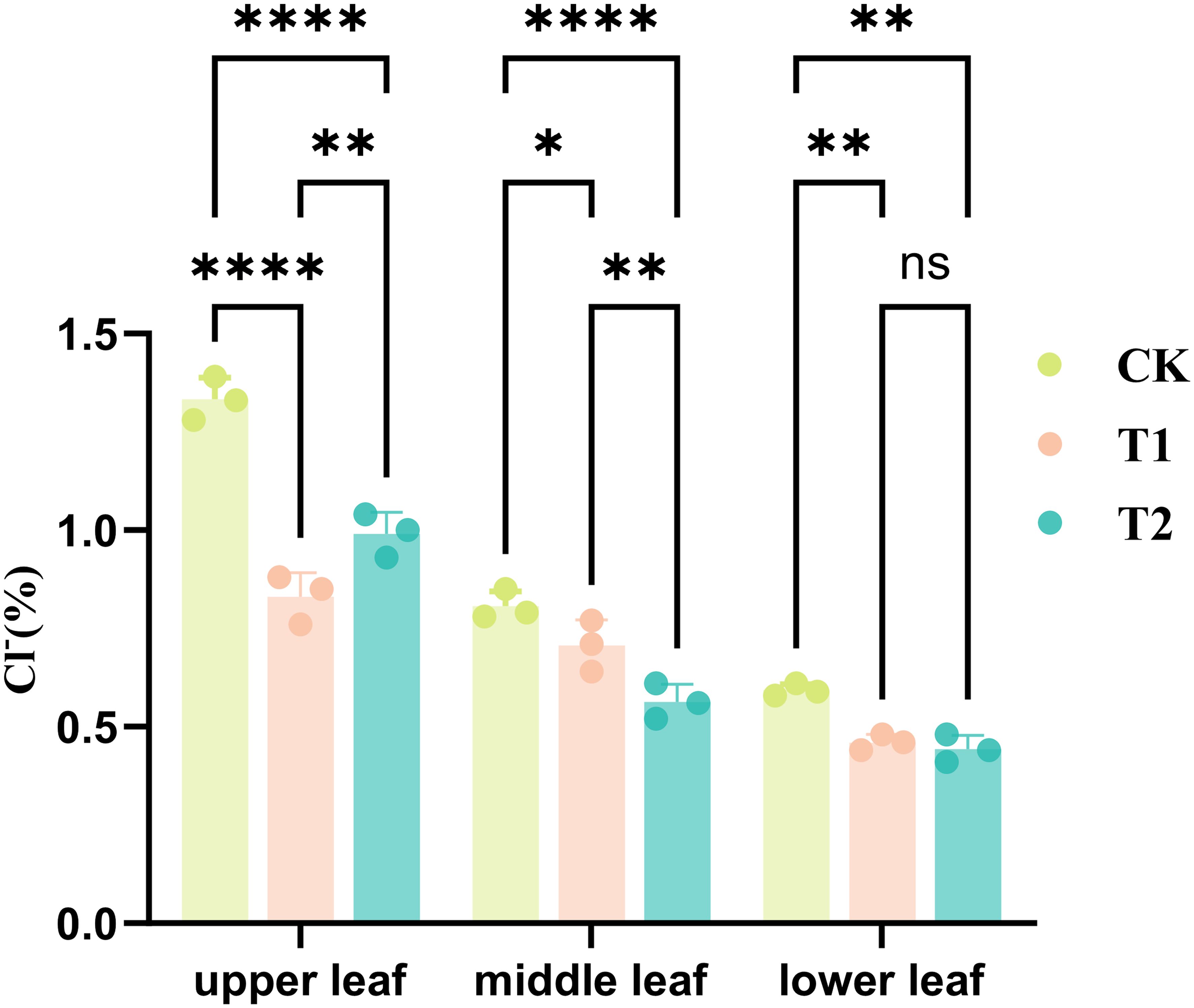
Figure 1. Chloride ion content in different parts of tobacco leaves in different treatments (*p < 0.05, **p < 0.01, ****p < 0.0001, ns: not significant).
3.2 Differences in soil physicochemical properties after the addition of inhibitors
Subsequent to the addition of inhibitors, notable differences in soil physicochemical properties emerged between the inhibitor-added treatments and the control group (Table 2). As the pivotal indices in this study, soil chloride ion concentrations presented a significantly increase in the T1 and T2 compared to the control group, up to 108.65 and 106.25 mg/kg, respectively, representing nearly twice the content observed in the control group (56.69 mg/kg). This indicated that fewer chloride ions were absorbed by the tobacco plants after the addition of the two inhibitors, which might be correlated with the lower chloride ion concentrations in tobacco leaves. Furthermore, the contents of available potassium (AK) in the T1and T2 were also significantly higher than the control group, also approaching twice as high as the control group. Conversely, the soil available phosphorus (AP) contents were almost the same as that of the control group. Additionally, the contents of nitrate nitrogen (NO3−-N) and ammonium nitrogen (NH4+-N) exhibited divergent trends in the T2 while remaining relatively stable in the T1experimental group. The NO3−-N content increased a lot in the T2 compared to the control group, while the NH4+-N decreased by nearly half when contrasted with the control group. These results indicated the different influences on soil physicochemical properties raised by the two types of inhibitors.
3.3 Effects on microbial community structures of the rhizospheric soil
To investigate the impact of inhibitors on soil microbial composition, the diversity and richness of microbial communities in the rhizospheric soil in different treatment and control groups were analyzed using alpha diversity analysis (Table 3). The results indicated that more than 99% of the sequencing samples exhibited sufficient data coverage. The Shannon index of the T1 group was notably higher at 5.56 compared to the control (5.08) and T2 (4.97), implying that the introduction of Halophilic microorganisms could enhance microbial community diversity. The Simpson’s index of the T1 and T2 treatments exhibited a modest increase when compared to the control group. Moreover, the Ace index and Chao value were significantly higher in the T1 than in the other treatments. These results indicated that the incorporation of salt-tolerant micro-organisms could contribute to increased diversity and abundance of soil microorganisms with a certain extent. Conversely, the addition of zinc sulphate in the T2 had a minimal impact on soil microbial diversity.
Ternary analysis (Figure 2) revealed distinct variations in the composition and distribution of microorganisms following the inhibitor addition, presenting an increased prevalence of Pseudarthrobacter in the V. natriegens-treated group and a heightened proportion of Thermoactinomyces in the Zn2+-treated group. Based on the analysis results shown in Figure 2, we further analyzed the differences in species composition at the phylum level among the different samples. In Figure 3, notable differences in microbial structural composition at the phylum level across the soil samples were demonstrated. The dominant phyla, including Proteobacteria, Firmicutes, Actinobacteria, and Bacteroidota, consistently ranked among the top four. Moreover, the combined relative abundance of Proteobacteria and Firmicutes exceeded 50%. The proportion of Proteobacteria significantly increased in the T1. Proteobacteria encompasses numerous nitrogen-fixing bacteria, which play a stabilizing role in soil residual nitrogen during the nitrogen cycle. Additionally, they exhibit strong adaptability and can decompose complex organic matter, thereby promoting soil nutrient cycling. Furthermore, certain strains within the Proteobacteria can participate in the transformation and migration of chloride ions through synergistic metabolism with other microorganisms. Certain groups within Firmicutes can break down complex organic substances, releasing nutrients such as nitrogen, phosphorus, and sulfur, thereby increasing the availability of nutrients in the soil and enhancing soil fertility. The proportions of Firmicutes were 11.7% ± 3.3% in the T1, 20.4% ± 4% in the T2 experimental group, and 16.7% ± 5% in the control group. Furthermore, changes in the proportions of other phyla were observed to some extent, indicating that the addition of inhibitors indeed disrupted the bacterial community structure in the rhizosphere soil. Based on the analysis results shown in Figure 3, the top four dominant phyla were consistent across all treatment groups. Therefore, we analyzed the principal component differences among the different samples. Additionally, principal component analysis (PCA) performed at the phylum level demonstrated significant differences between the three experimental groups (Figure 4). The PCA analyses (PERMANOVA, R= 0.523, P = 0.0010) highlighted that 31.45% of disparities could be attributed to the PC1 axis and 20.79% to the PC2 axis regarding phylum-level discrepancies. Moreover, to further elucidate the distinctive microbial communities in the soil following the inhibitor addition, linear discriminant analysis effect size (LEfSe) analysis was conducted on the T1 and T2, respectively, in comparison with the control group (Figure 5). Results revealed significant enrichment of Sphingomonadaceae, Rhizobiaceae, and Xanthobacteraceae at the family level in the T1 when compared to the control group. Conversely, Cellvibrionaceae, Sphingobacteriaceae, and Nocardioidaceae were enriched in the control samples. Similarly, LEFSe analysis of soil microorganisms from the T2 and control groups showed significant enrichments of Alcaligenaceae and Legionellaceae in the soil samples from the T2, while Micrococcaceae and Cellvibrionaceae were enriched in the control group. These findings together suggested that the addition of inhibitors has resulted in substantial structural changes in the bacterial community.
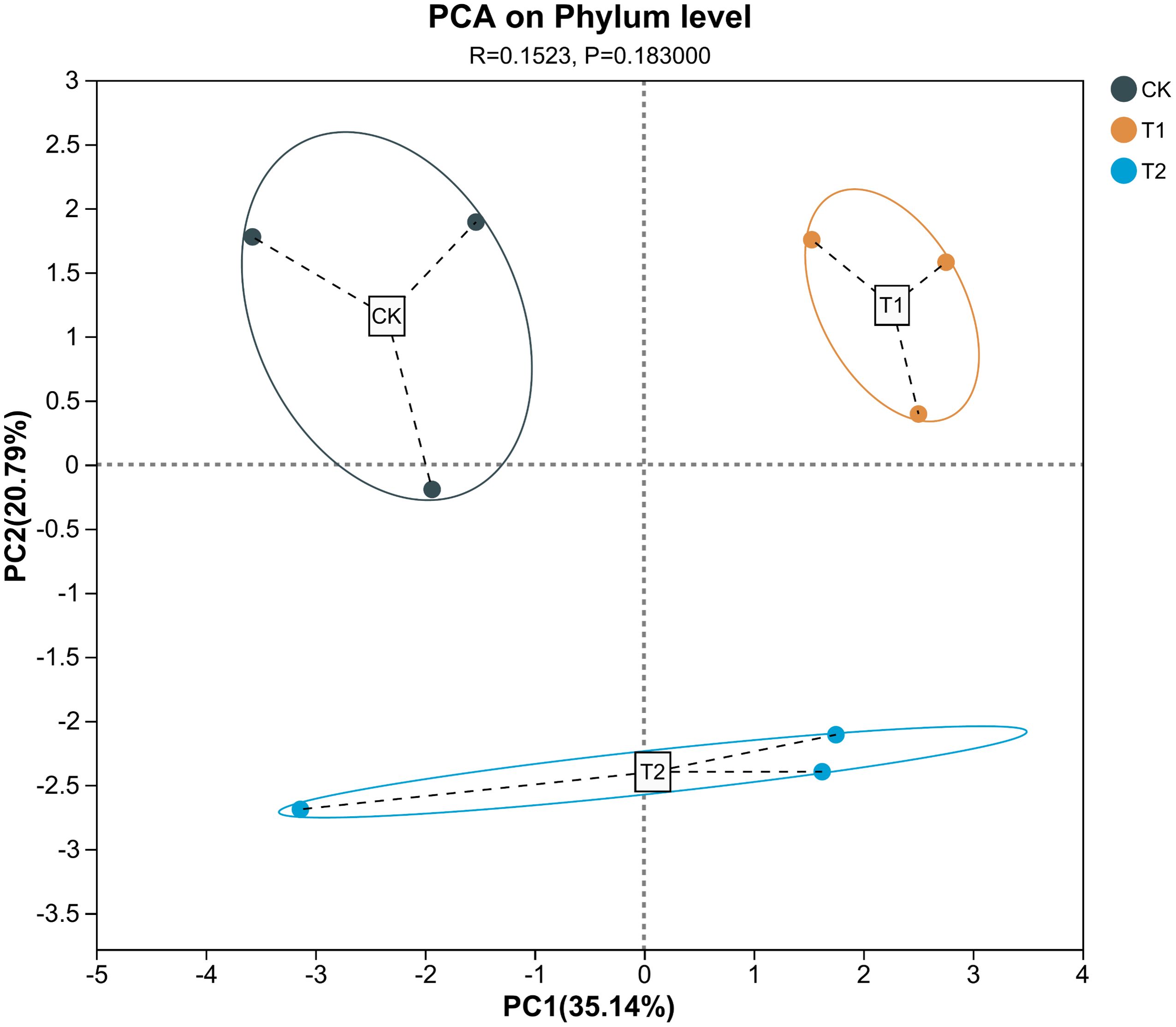
Figure 4. Microbiology community diversity in the analysis of soil samples (PERMANOVA, R2 = 0.523, P=0.001).
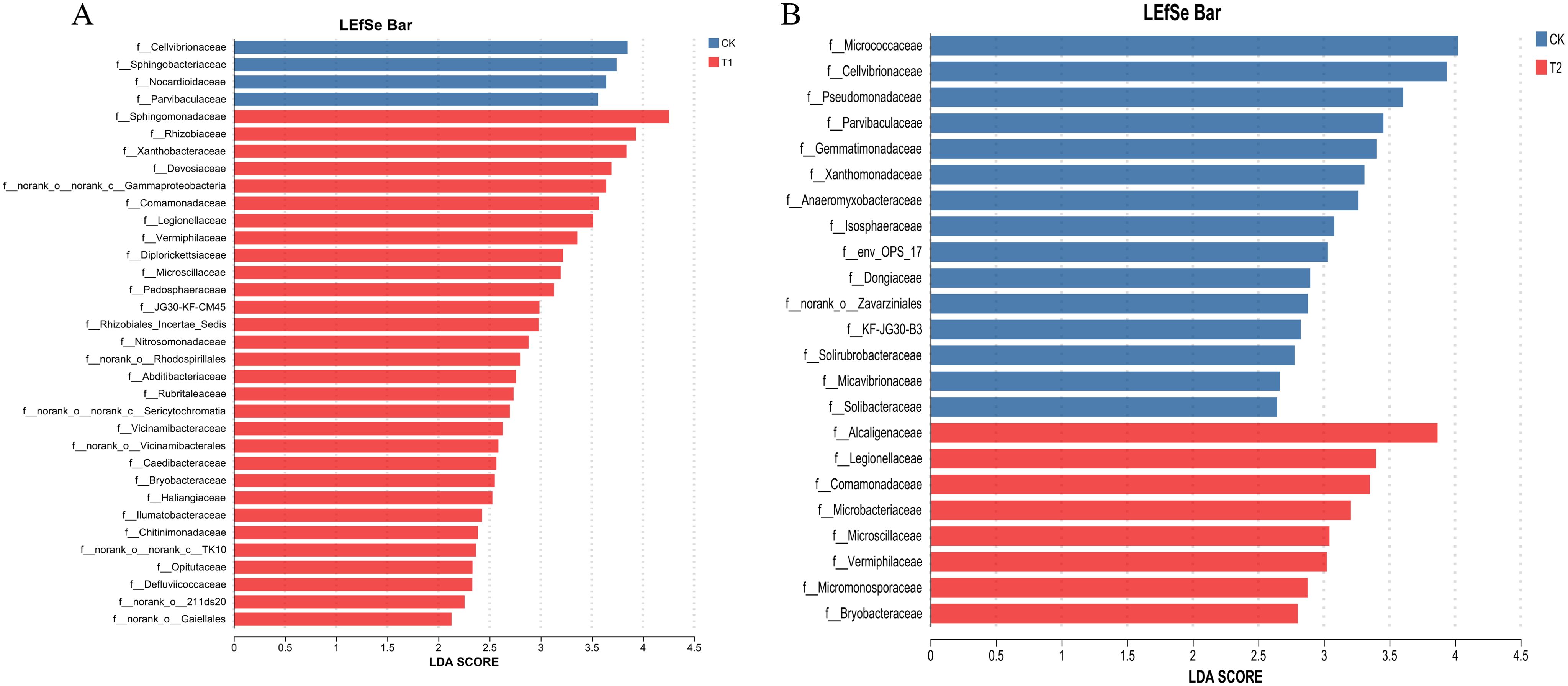
Figure 5. Microbiology community linear discriminant in the analysis of effect size (LEfSe) in soil samples. (A) Comparison of rhizosphere bacterial communities between the T1 experimental group and the CK control group using LEfSe (LDA=2). (B) Comparison of rhizosphere bacterial 1 communities between the T2 experimental group and the CK control group using LEfSe (LDA=2).
3.4 The inhibitor addition influenced the interaction between soil physicochemical properties and microbial communities
To analyze the correlation between soil microorganisms and soil physicochemical properties, we conducted a correlation analysis based on the microbial community analysis results for the three sample groups. This analysis aimed to elucidate the relationship between soil physicochemical properties and the microbial community structure in tobacco-growing soil (Figure 6A). The results of distance-based redundancy analysis (db-RDA) indicated that the composition of bacterial communities in the T2 and control groups was positively correlated with AP, NH4+-N, and NO3–N, while negatively correlated with AK. Conversely, AK revealed a positively correlation with the bacterial communities in the T1. However, the Cl− content showed no significant correlations with the bacterial diversity. Additionally, CAP1 and CAP2 explained 24.67% and 18.62% of the variation in bacterial communities, respectively. To address potential confounding effects arising from variations in microbial abundance, the correlation between microbial taxa and soil physicochemical properties across the initial 30 taxonomic order levels was subsequently analyzed (Figure 6B). Clear distinctions were observed among different taxonomic orders in relation to physicochemical properties. Specially, Rhizobiales and Sphingomonadales exhibited a significant positive correlation with AK. Cytophagales and Gammaproteobacteria showed positive associations with Cl− and AK, whereas Parvibaculales displayed the exact opposite correlation with these two physicochemical properties. Moreover, Pseudomonadales demonstrated a notable negative correlation with NH4+-N and NO3–N. To sum up, these results suggest that a subset of the bacterial community collectively regulates the levels of chloride ions and other nutrients included in the planting soil.
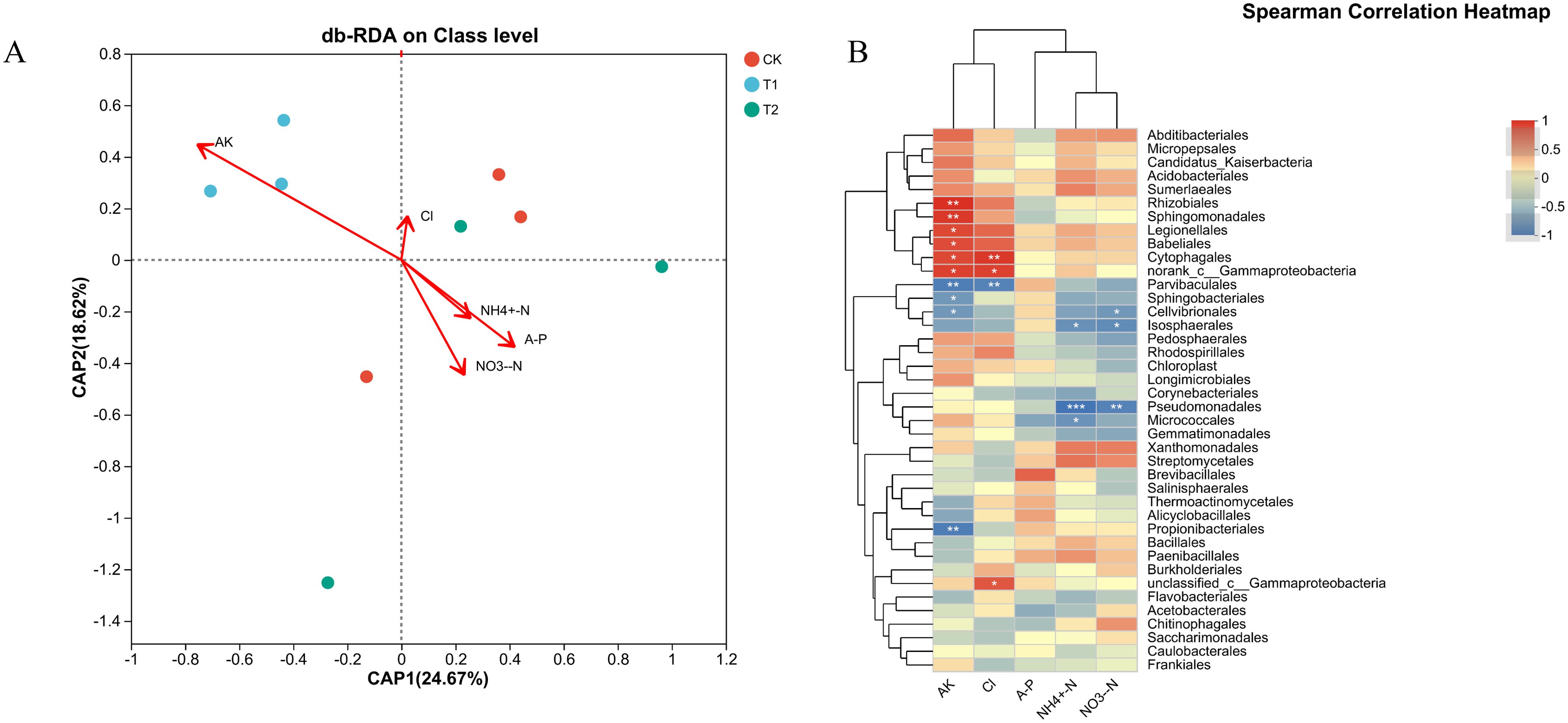
Figure 6. Correlation analysis of microbial community structure and environmental factors soil. (A) Nutrient content (Cl, AK, NH4+-N, NO3--N, A-P), redundancy analysis of different inhibitors (db-RDA) (B) Correlation plot analysis of nutrient content (Cl-, AK, NH4+-N, NO3-+N, A-P) with different inhibitors (*p < 0.05, **p < 0.01, ***p < 0.001).
Additionally, to further explore the effect of inhibitor addition on the functioning of soil microbial communities in tobacco rhizosphere soil, the FAPROTAX database was adopted to conduct functional predictions, respectively (Figures 7A, B). The photoheterotropgy, phototrophy and nitrite-denitrification of the soil in the T1 showed significant enhancement. The photoheterotropgy in the tobacco-planted soil of the T2 t exhibited significant improvement.
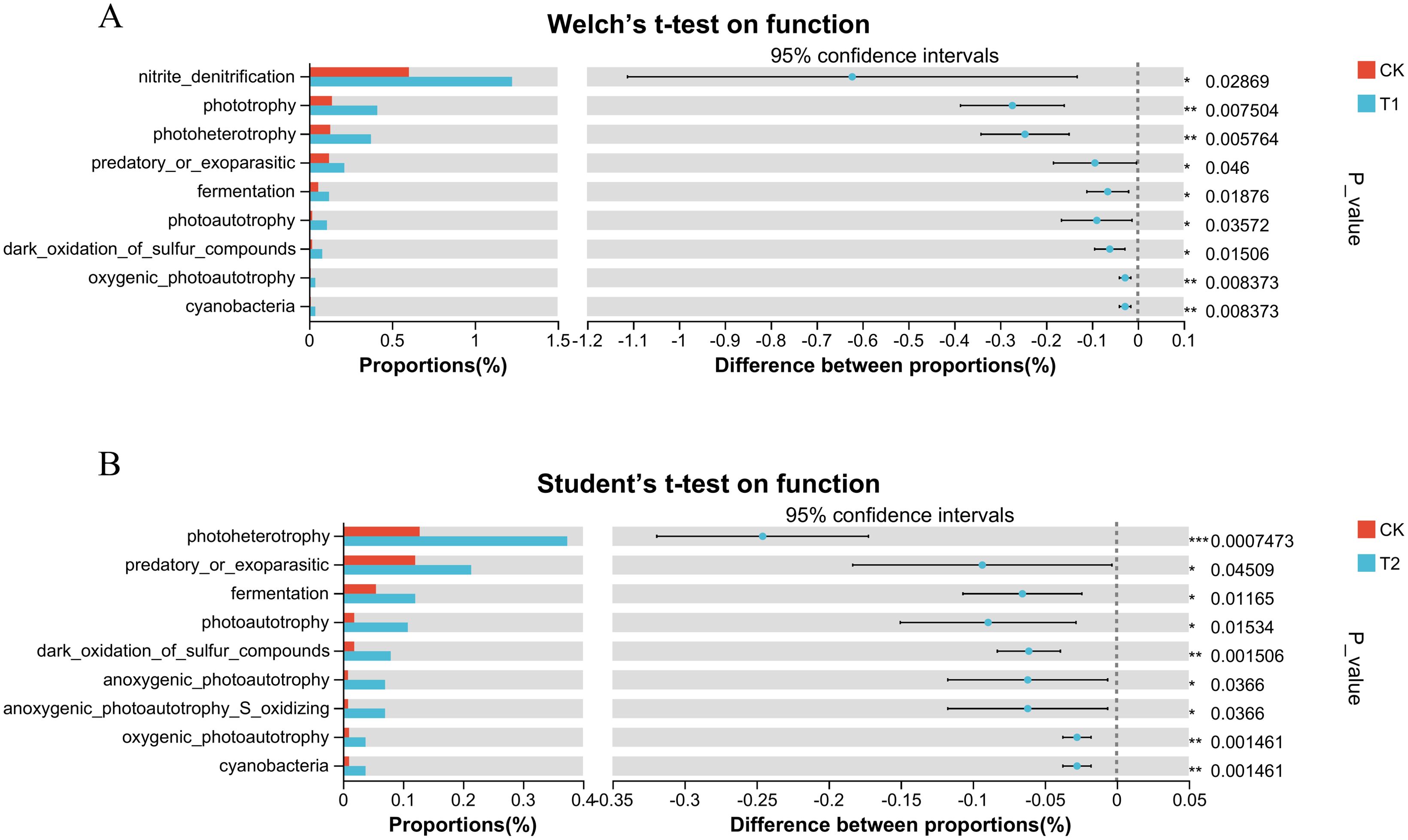
Figure 7. Functional and phenotypic prediction of soil microbial communities (A) Significance test for the addition of sodium-demanding Vibrio natriegens for soil diversity prediction of FAPROTAX functional group differences in tobacco planting (*p < 0.05, **p < 0.01, *** p < 0.001) (B) Significance test for differences between FAPROTAX functional groups for predicting soil diversity in planted tobacco with the addition of zinc sulphate heptahydrate (*p < 0.05, **p < 0.01, ***p < 0.001).
3.5 Field experiment validation of chloride ion absorption inhibitors
To further investigate the practical application effectiveness of the inhibitors FT1 and FT2 in tobacco cultivation, field experiments was conducted. The chloride ion content in tobacco leaves was analyzed (Figure 8), and the results demonstrated that the chloride ion content in various parts of the tobacco leaves in the FT1 was lower than that in the FT2 and the control group, with a significant reduction observed in the upper leaves. The chloride ion content in the tobacco leaves of the FT2 was also lower than that in the control group, indicating its positive role in inhibiting the absorption of chloride ions in tobacco plants. Moreover, the chloride ion content in tobacco soil from the field experiment was also investigated (Table 4). During the vigorous stage, the control group had the highest chloride ion content (113.79 mg/kg) in tobacco soil, followed by the FT2 (108.54 mg/kg), and lastly the FT1 (105.03 mg/kg). Conversely, during the mature stage, the best-performing experimental group in terms of tobacco soil chloride ion content was the FT1, followed by the FT2, with the lowest content observed in the control group. Overall, the field experiment results were consistent with those of the pot experiment.
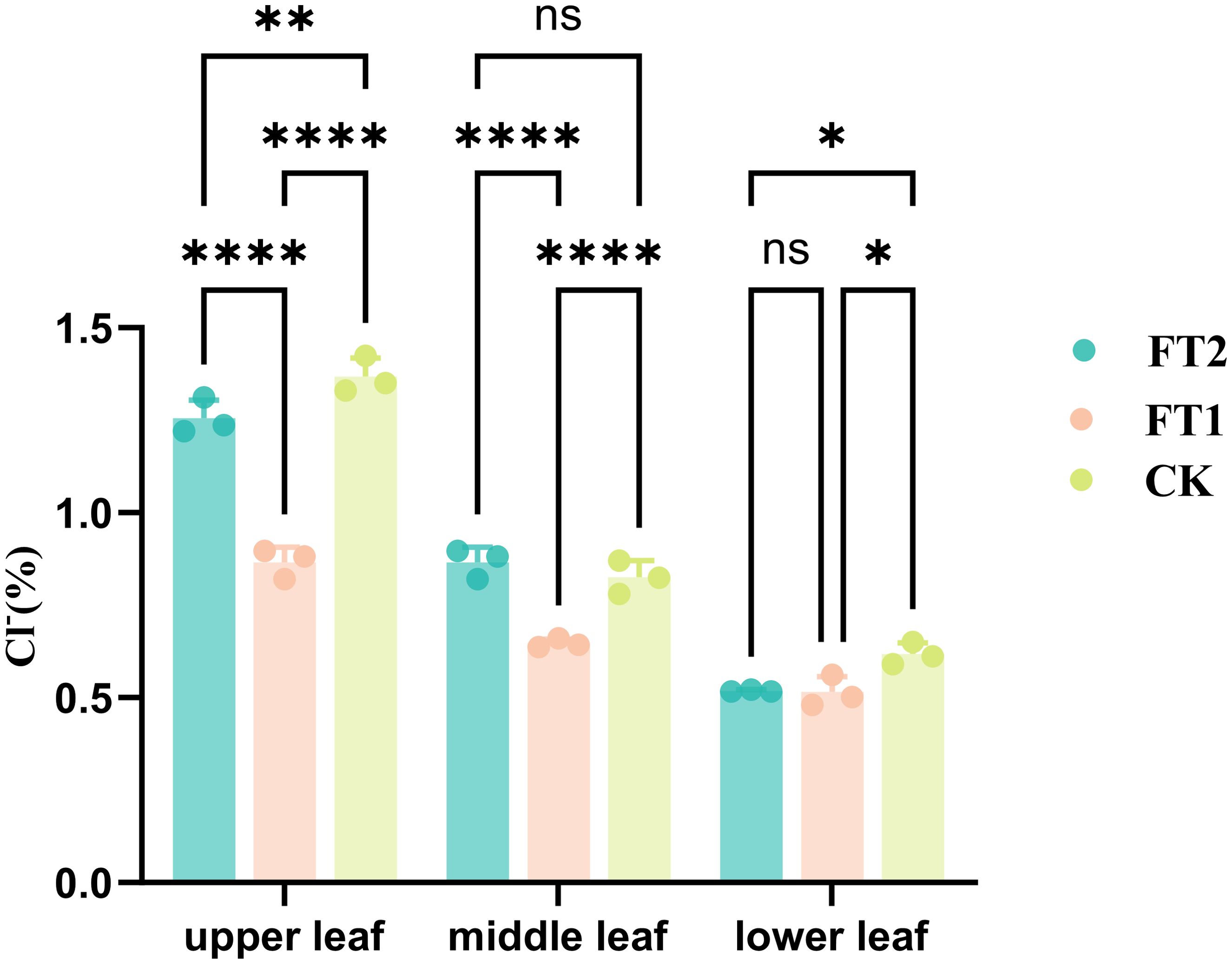
Figure 8. Chloride ion content in tobacco leaves in field experiment. (*p < 0.05, **p < 0.01, ****p < 0.0001, ns: not significant).
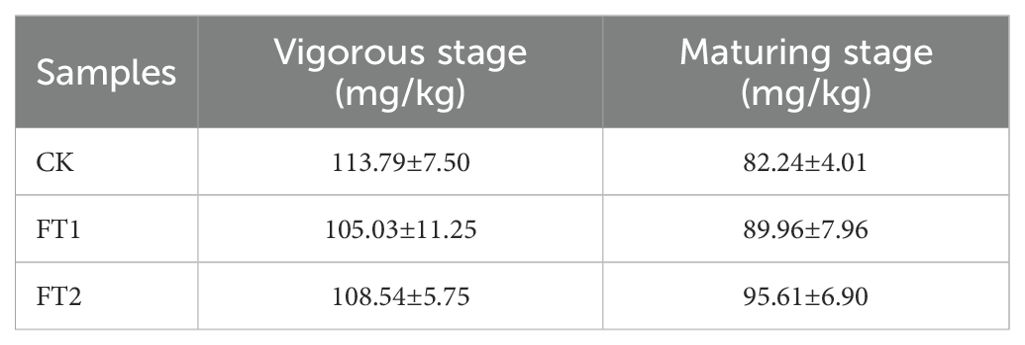
Table 4. Chloride ion contents in tobacco-planting soil at different growth stages in field experiment.
4 Discussion
In this study, we observed that the introduction of the halophilic microorganism V. natriegens and zinc sulfate resulted in diminished chlorine ion content in tobacco leaves, and the deposition of chlorine ions within the tobacco rhizospheric soil. It has been reported that elevated levels of chlorine ion could impede plant growth, while simultaneously augment water uptake and decrease tobacco combustion rates (48, 49). Beside the decrease of chlorine ion content in tobacco leaves, the application of inhibitors correspondingly increased the levels of AK and NH4+-N in the tobacco rhizospheric soil. As well known, both nitrogen and potassium are vital nutrients essential for tobacco growth (50). In this study, the addition of the V. natriegens inhibitor increased the content of available potassium in the soil. V. natriegens can decompose minerals such as potassium feldspar in the soil, releasing potassium ions into the soil solution, thereby increasing the available potassium content. Additionally, the sodium ions absorbed by V. natriegens are replaced by potassium ions, which are then released into the soil solution, further increasing the available potassium content (30). Moreover, as shown in Figure 3, the addition of the inhibitor increased the abundance of Actinobacteria in the soil. This phylum can enhance the available potassium content in the soil through synergistic interactions with other microbial species (51).
Soil microorganisms are known to be crucial in enhancing crop growth by facilitating biotransformation and improving nutrient utilization (52). Research has demonstrated that a subset of microorganisms employ salt solubilization mechanism to maintain osmotic balance between intra and extracellular environments, resulting in high intracellular levels of Cl− (53). Although there is currently no research demonstrating the mechanism by which V. natriegens reduces chloride ion content in tobacco leaves, we hypothesize, based on the fact that the rapid growth rate of halophilic bacteria increases chloride ion uptake and that V. natriegens can regulate ion balance inside and outside cells through ion transport proteins on its cell membrane (30), that V. natriegens may absorb large amounts of chloride ions from the soil during its rapid growth, thereby reducing chloride ion content in tobacco leaves. This study shows that the introduction of sodium-dependent V. natriegens reduces tobacco plants’ uptake of chloride ions from the soil, which aligns with previous findings on the ability of halophilic microorganisms to mitigate free Cl− in high-salinity wastewater (54).
Zn2+ functions as an anion channel inhibitor, effectively suppressing the uptake of anions by plants from soil, and the mechanism has been experimentally confirmed in Arabidopsis thaliana (19, 20). In this work, the comparatively low chloride content in tobacco leaves and the increased chloride deposition in the soil were achieved after the addition of Zn2+. The results were generally consistent the findings from studies on Zn2+ as an inhibitor affecting anionic protein channel transport.
As mentioned above, increased abundance of soil microflora promotes plant growth and improves biomass accumulation to some extent (55). Here different inhibitors have resulted in significant differences in soil microbial composition. The addition of V. natriegens promoted an increase in soil bacterial abundance, among which the proportion of Proteobacteria increased significantly. Actually, V. natriegens belongs to Proteobacteria, the raised proportion might to be relative to the colonization of V. natriegens in the soil. However, the T2 was essentially the same as the control group in terms of soil bacterial abundance. Ternary analyses also confirmed this, with little difference in bacterial species between the control and T2, suggesting that there are still some differences in the improvement of soil microflora by chemical reagents compared to microbial agents. In tobacco rhizospheric soil ecosystems, the addition of microbial agents has obviously promoted changes in microbial community structure, which further affected soil nutrients. Through the db-RDA environmental factor analysis, it could be deduced that the contents of NH4+-N, NO3–N and AP were positively correlated with the bacterial diversity when Zn2+ was added versus the control group. Conversely, Cl− and AK appeared to be positively correlated with the bacterial diversity the soil microbiota following the addition of V. natriegens, highlighting a complex association between soil physicochemical properties and microbial composition. The significant negative relationship between Pseudomonadales bacteria abundance and NH4+-N, NO3–N content might be attributed to the influence of this bacterium on the nitrogen assimilation rate of the tobacco plant, which is consistent with previous study. LEfSe analyses were more helpful in identifying bacterial communities that were significantly correlated with certain functions after the addition of inhibitors (56). For instance, the Sphingomonadaceae community was significantly characterized by the addition of V. natriegens, known for its ability to degrade aromatic compounds, thereby improving soil quality and mitigating soil toxicity (57). Meanwhile, the ubiquitous rhizobacteria function as nitrogen-fixers and phosphorus solubilizers (58), potentially accounting for the higher levels of NH4 +-N and lower content of AP in the T1. The enrichment of Micrococcaceae and Cellvibrionaceae in T2 treated soils displayed significant correlations with various soil physicochemical properties. Notably, the two taxa have been reported to participate in the processes including fermentation, respiratory metabolism, and the degradation of chemically energetic organic matter in soil (59, 60). This result was also verified in the agronomic trait growth of tobacco plants, which was superior in the T2 compared to the control. Obviously, the microbial abundance in tobacco planting soil significantly influences the growth and vigor of tobacco plants (61, 62). Especially, the T1 has greatly increased the abundance and variability of soil microorganisms, and this treatment also presented the optimal growth of tobacco plants. These results are consistent with the results of previous studies (63), indicating V. natriegens has various potential functions in addition to inhibiting chloride ion uptake. In summary, to investigate the effects of inhibitors on soil microbial composition, alpha diversity analysis was used to assess the diversity and richness of microbial communities in the rhizosphere soil of different treatment groups and the control group. Additionally, ternary plots were employed to analyze the composition and distribution among different treatment groups. Further analysis at the phylum level revealed significant differences in species composition between the treatment groups and the control group. Moreover, LEfSe analysis was conducted to identify significantly enriched microbial taxa at the family level in each treatment group, and FAPROTAX was used to analyze functional changes. Finally, we examined the relationships between samples and environmental factors to observe the impact of soil microbial community changes on soil nutrients. The results indicated that the addition of the V. natriegens inhibitor did not increase chloride ion content in the soil. Furthermore, based on the above analysis, the addition of V. natriegens led to an increase in Proteobacteria in the soil. Certain strains of Proteobacteria can participate in the transformation and migration of chloride ions through synergistic metabolism with other microorganisms. Additionally, the addition of V. natriegens significantly enhanced the denitrification function of the microbial community. The protons (H+) produced during this process can influence soil pH balance. An acidic environment may increase the solubility of chloride ions, thereby raising their concentration in the soil solution. H+ may also compete with chloride ions for adsorption sites, affecting the adsorption and migration of chloride ions in the soil (64).
Through pot and field experiments, we found that the chloride ion inhibitor containing V. natriegens outperformed Zn²+ in reducing chloride ion content in upper tobacco leaves. To explain this phenomenon, we analyzed it in the context of existing reports. First, the mechanisms of action may differ. Zn²+ regulates ion uptake and transport by modulating the structure of the xylem in the plant roots and leaf veins, as well as the ratio of root cortex thickness, thereby reducing chloride ion uptake by the plant and subsequently lowering chloride ion content in the leaves. However, Zn²+ cannot reduce chloride ion content in the soil, leading to an accumulation of chloride ions in the soil and increasing the likelihood of plant uptake. In contrast, V. natriegens rapidly grows and absorbs chloride ions into its cells, reducing soil chloride ion levels and thereby decreasing the likelihood of plant uptake. Second, it is possible that Zn²+ is more susceptible to interference from anions in the soil, whereas V. natriegens, as a microbial strain, remains stable in the soil, resulting in superior efficacy compared to Zn²+. In summary, compared to using cations as anion channel blockers (19), employing V. natriegens as a Cl− uptake inhibitor offers greater stability, superior efficacy, and the ability to actively reduce chloride ion content in the soil.
As reported, effective microorganisms play a crucial role in enhancing plant leaf photosynthesis and improving yield and quality traits (65). In this study, the predicted functions, the oxygenated phototrophic autotrophic and fermentation functions, were significantly enhanced in the T1 group, consistent with previous studies (66). Notably, based on the FAPROTAX functional prediction results, we found that the addition of V. natriegens significantly enhanced the nitrite denitrification function compared to the CK. Nitrite denitrification refers to the microbial process of reducing nitrite to nitrogen gas or other nitrogen oxides. During this process, the production of protons can influence soil pH balance. An acidic environment may increase the solubility of chloride ions, thereby raising their concentration in the soil solution. Additionally, H+ may compete with Cl− for adsorption sites, affecting the adsorption and migration of chloride ions in the soil (67). However, these hypotheses have not yet been experimentally validated, which may lead to discrepancies with actual functional outcomes (68). Therefore, further detailed experiments are essential to confirm the effect of changes in the tobacco-planted soil microbial community on its functionality. Notably, limitations exist in this study conducted in greenhouse potted plants. A more accurate approach would involve precise control over a large field area, and the field trial would provide a more accurate judgement on the feasibility of the inhibitor products. Therefore, the field experiment was performed in this study, whose results suggested to be basically consistent with the findings from the plot experiment. Nevertheless, microbial inoculants utilizing microorganisms as inhibitors still face several challenges in soil improvement under field experimental conditions. Primarily, factors such as soil nutrient levels, temperature, and humidity may attenuate the beneficial effects of microbial inoculants. Secondly, the production costs of microbial inoculants are relatively high, particularly when employing lyophilization and cryopreservation techniques to sustain cellular viability. Additionally, due to variations in soil type, climatic conditions, and crop species, the efficacy of chloride ion inhibitors may differ across different field conditions, leading to increased uncertainty in their performance. Moreover, in field experiments, temperature is an uncontrollable factor, and excessively high or low temperatures may adversely affect the performance of the inhibitors. The amount and distribution of rainfall can influence the concentration and persistence of inhibitors in the soil. Furthermore, the chloride ion content in irrigation water may also impact the effectiveness of the inhibitors. In future studies, the mechanism of V. natriegens, Zn2+ and tobacco interaction at different stages can be studied, and large-scale field validation can be carried out to evaluate the overall impact on tobacco under different conditions. Moving forward, further comprehensive analyses on soil microorganisms and tobacco plants should be carried out.
5 Conclusions
In this study, the addition of both inhibitors of sodium-demanding V. natriegens and zinc sulphate reduced the chloride ion content of tobacco leaves and both raised the effect of inhibiting the chloride ion uptake, but there were differences between the two inhibitors. The T2, although effective in inhibiting the uptake of chloride ions, was slightly inferior to the T1experimental group in altering the soil microbial community and enhancing the growth of tobacco plants. V. natriegens, as a non-pathogenic halophilic bacterium, is ecologically safe. Additionally, due to its rapid growth rate, V. natriegens significantly reduces cultivation time and costs, making it highly economically valuable. In tobacco experiments, the addition of V. natriegens not only reduces chloride ion content in tobacco but also improves the physicochemical properties and microbial community structure of the rhizosphere soil, promoting tobacco growth. These findings have been further validated by field trials. These results may help to study the inhibitors to inhibit the uptake of chloride ions in tobacco plants, reduce the chloride ion content of tobacco, and enhance the quality of tobacco by providing theoretical references. However, this study still has certain limitations. Although fertilization and agronomic management were consistent between the pot and field experiments, the pot experiments were conducted in a greenhouse, while the field experiments were subject to more external influencing factors. As a result, the inhibitor concentration that was optimal in the pot experiments may not be optimal in the field experiments. Additionally, there are differences in chloride ion content in the irrigation water used for pot and field experiments. Furthermore, the mechanism by which V. natriegens interacts with soil nutrients to influence chloride ion uptake has not been investigated in greater detail. In future research, it is essential to delve deeper into the molecular mechanisms of V. natriegens, including gene expression and protein functions, which will facilitate the development of more efficient chloride ion inhibitors and enhance the adaptability and functionality of V. natriegens in diverse environments. Additionally, field experiments should be conducted across different regions, soil types, and tobacco varieties to evaluate the long-term effects and ecological impacts of V. natriegens in various soils. Furthermore, exploring the mechanisms of composite inhibitors is crucial to developing more efficient and cost-effective chloride ion inhibitors.
Data availability statement
The datasets presented in this study can be found in online repositories. The names of the repository/repositories and accession number(s) can be found below: https://www.ncbi.nlm.nih.gov/, PRJNA1205122.
Author contributions
HD: Conceptualization, Writing – review & editing. SW: Conceptualization, Writing – review & editing. YZ: Investigation, Writing – review & editing. JD: Investigation, Writing – review & editing. RZ: Investigation, Writing – review & editing. BZ: Investigation, Writing – review & editing. SM: Investigation, Writing – review & editing. DL: Investigation, Writing – review & editing. RC: Investigation, Writing – review & editing. BC: Investigation, Writing – review & editing. QW: Investigation, Writing – review & editing. JS: Data curation, Writing – review & editing. SX: Conceptualization, Data curation, Formal analysis, Investigation, Resources, Validation, Writing – review & editing. ZW: Conceptualization, Data curation, Formal analysis, Investigation, Resources, Supervision, Validation, Writing – review & editing. MS: Data curation, Supervision, Writing – review & editing. HZ: Conceptualization, Investigation, Supervision, Writing – review & editing. WW: Conceptualization, Data curation, Formal analysis, Investigation, Resources, Validation, Writing – original draft, Writing – review & editing.
Funding
The author(s) declare that financial support was received for the research and/or publication of this article. This research was funded by Inner Mongolia Kunming Cigarette Limited Liability Company, grant number 202315010534-JS-061 and 202315010534-JS-062.
Acknowledgments
The authors would also like to thank the “Laboratory for Agricultural Molecular Biology” of Qingdao Agricultural University for providing laboratory apparatus and the support of Shandong Provincial Colleges and Universities “Youth Innovation Team Program” (2022KJ167).
Conflict of interest
Authors HD, SW, YZ, BZ, SM, DL, RC, BC, QW were employed by the company Inner Mongolia Kunming Cigarette Limited Liability Company. Authors JD, RZ were employed by the company Inner Mongolia Autonomous Region Tobacco Company Chifeng Company.
The remaining authors declare that the research was conducted in the absence of any commercial or financial relationships that could be construed as a potential conflict of interest.
Generative AI statement
The author(s) declare that no Generative AI was used in the creation of this manuscript.
Publisher’s note
All claims expressed in this article are solely those of the authors and do not necessarily represent those of their affiliated organizations, or those of the publisher, the editors and the reviewers. Any product that may be evaluated in this article, or claim that may be made by its manufacturer, is not guaranteed or endorsed by the publisher.
References
1. Bohn AA, De Morais HA. A quick reference on chloride. Vet Clin North Am Small Anim Pract. (2017) 47:219–22. doi: 10.1016/j.cvsm.2016.10.008
2. Jawad HA, Hussein NH. Effect of sodium chloride on stevia plant growth in vitro. MINAR Int J Appl Sci Technol. (2022) 4:171.
3. Flowers TJ, Rana M, Colmer TD. Sodium Chloride Toxicity and the Cellular basis of salt Tolerance in Halophytes. Ann Bot. (2015) 3:419–31. doi: 10.1093/aob/mcu217
4. Stefanie W, Matthew G, Henderson SW. Chloride: not simply a ‘cheap osmoticum’, but a beneficial plant macronutrient. J Exp Bot. (2017) 12:12. doi: 10.1093/jxb/erx050
5. Geilfus C-M. Chloride: from nutrient to toxicant. Plant Cell Physiol. (2018) 59:877–86. doi: 10.1093/pcp/pcy071
6. larke TLH, Hepler PK. Control of pollen tube growth: role of ion gradients and fluxes. New Phytol. (2003) 159:539–63. doi: 10.1046/j.1469-8137.2003.00847.x
7. Henderson SW, Wege S, Qiu J, Blackmore DH, Walker AR, Tyerman S, et al. Grapevine and arabidopsis cation-chloride cotransporters localize to the golgi and trans-golgi network and indirectly influence long-distance ion transport and plant salt tolerance. Plant Physiol. (2015) 169:2215–29. doi: 10.1104/pp.15.00499
8. Tam NC, Agorio A, Jossier M, Filleur S. Characterization of the chloride channel-like, atCLCg, involve. Plant Cell Physiol. (2015) 57:764–75. doi: 10.1093/pcp/pcv169
9. Nguyen CT, Agorio A, Jossier M, Depré S, Thomine S, Filleur S. Characterization of the chloride channel-like, atCLCg, involved in chloride tolerance in arabidopsis thaliana. Plant Cell Physiol. (2016) 57:764–75. doi: 10.1093/pcp/pcv169
10. Colmenero-Flores JM, Franco-Navarro JD, Cubero-Font P, Peinado-Torrubia P, Rosales MA. Chloride as a beneficial macronutrient in higher plants: new roles and regulation. Int J Mol Sci. (2019) 20:4686. doi: 10.3390/ijms20194686
11. Wang L, Xu JY, Jia W, Chen Z, Xu ZC. Chloride salinity in a chloride-sensitive plant: Focusing on photosynthesis, hormone synthesis and transduction in tobacco. Plant Physiol Biochem. (2020) 153:119–30. doi: 10.1016/j.plaphy.2020.05.021
12. Zhang BL, Shang SH, Zhang HT, Jabeen Z, Zhang GP. Sodium chloride enhances cadmium tolerance through reducing cadmium accumulation and increasing anti-oxidative enzyme activity in tobacco. Environ Toxicol Chem. (2013) 32:1420–5. doi: 10.1002/etc.2183
13. Meixia S. Effect of different soil moistures in tobacco maturing stage on water contents and chemical components of flue-cured tobacco leaf. J Anhui Agric Sci. (2002) 2:280–2.
14. Peng L, Jin-Feng Z, Li G, Kai J, Hui-Fang S, Wu-Xing H, et al. Research progress in source and control measures of chlorine ion in flue-cured tobacco. Jiangxi J Agric Sci. (2013) 74-77:82.
15. Yang YH, Chen DM, Jin Y, Wang HB, Duan YQ, Guo XK, et al. Effect of different fertilizers on functional diversity of microbial flora in rhizospheric soil under tobacco monoculture. Acta Agron Sin. (2011) 37:105–11. doi: 10.1016/S1875-2780(11)60003-5
16. Lei Y, Xiao Y, Li L, Jiang C, Zu C, Li T, et al. Impact of tillage practices on soil bacterial diversity and composition under the tobacco-rice rotation in China. J Microbiol. (2017) 55:349–56. doi: 10.1007/s12275-017-6242-9
17. Maletskyi Z, Mitchenko T, Hoell WH, Makarova N. Properties of anion exchange resins exhausted by humic compounds. Desalination Water Treat. (2011) 25:78–83. doi: 10.5004/dwt.2011.1524
18. Tai-Wen Y, Wen-Yu Y, Da-Bing X, Xiao-Rong C, Yan W. Production and N nutrient performance of wheat-maize-soybean relay strip intercropping system and evaluation of interspecies competition. Acta Pratacult Sin. (2012) 1:50–8.
19. Barbier-Brygoo H, Vinauger M, Colcombet J, Ephritikhine G, Frachisse JM, Maurel C. Anion channels in higher plants: functional characterization, molecular structure and physiological role. Biochim Biophys Acta. (2000) 1465:199–218. doi: 10.1016/S0005-2736(00)00139-5
20. Bingjun YU, Youliang L. Effects of salt stress on the metabolism of active oxygen in seedlings of annual halophyte Glycine soja. Acta Botanica Boreali-occidentalia Sin. (2003) (01):18–22.
21. Fang Y, Hongbo Z, Yue W, Guoqing H, Jiechen W, Daliang G, et al. The role of antioxidant mechanism in photosynthesis under heavy metals Cd or Zn exposure in tobacco leaves. J Plant Interact. (2021) 16:354–66. doi: 10.1080/17429145.2021.1961886
22. Hu C, Ning L, Zou L, Ming P. Research progress on nitrogen utilization and nitrate nitrogen transport proteins in plants JST/Kyoto University machine translation. Fenzi Zhiwu Yuzhong. (2016) 14:2188–96.
23. Bo L, Mark T, Matthew G. Chloride on the move. Trends Plant Science. (2017) 22:236–48. doi: 10.1016/j.tplants.2016.12.004
24. Camilla S, Dale S, Ute K, Dorina P. Zinc in plants: Integrating homeostasis and biofortification. Mol Plant. (2022) 15:65–85. doi: 10.1016/j.molp.2021.12.008
25. Davis MRH, Zhao FJ, Mcgrath SP. Pollution-induced community tolerance of soil microbes in response to a zinc gradient. Environ Toxicol Chem. (2004) 23:2665–72. doi: 10.1897/03-645
26. Ruan YN, Tang ZX, Xia TY, Chen ZB. Application progress of microbial fertilizers in flue-cured tobacco production in China. IOP Conf Series: Earth Environ Sci. (2020) 615:012084 (012089pp). doi: 10.1088/1755-1315/615/1/012084
27. Kevin CL, Stephen A, Mayada KK, Dina MA-M. Bioremediation of oily hypersaline soil via autochthonous bioaugmentation with halophilic bacteria and archaea. Sci Total Environment. (2024) 922:171279. doi: 10.1016/j.scitotenv.2024.171279
28. Kamekura M, Kushner DJ. Effect of chloride and glutamate ions on in vitro protein synthesis by the moderate halophile Vibrio costicola. J Bacteriology. (1984) 160:385–90. doi: 10.1128/jb.160.1.385-390.1984
29. Goo Y,A. Proteomic analysis of an extreme halophilic archaeon, Halobacterium sp. NRC-1. Mol Cell Proteomics. (2003) 2:506–24. doi: 10.1074/mcp.M300044-MCP200
30. Matthew TW, Eric DH, Christopher MW, Daniel GG. Vibrio natriegens as a fast-growing host for molecular biology. Nat Methods. (2016) 13:849–51. doi: 10.1038/nmeth.3970
31. Biener R, Horn T, Komitakis A, Schendel I, Knig L, Hauenstein A, et al. High-cell-density cultivation of Vibrio natriegens in a low-chloride chemically defined medium. Appl Microbiol Biotechnol. (2023) 107:12. doi: 10.1007/s00253-023-12799-4
32. Lima M, Muddana C, Xiao Z, Bandyopadhyay A, Wangikar PP, Pakrasi HB, et al. The new chassis in the flask: Advances in Vibrio natriegens biotechnology research. Biotechnol Adv. (2024) 77:108464. doi: 10.1016/j.biotechadv.2024.108464
33. Zerulla K, Soppa JR. Polyploidy in haloarchaea: advantages for growth and survival. Front Microbiol. (2014) 5:274. doi: 10.3389/fmicb.2014.00274
34. Yu X, Zhang Y, Shen M, Dong S, Zhang F, Gao Q, et al. Soil conditioner affects tobacco rhizosphere soil microecology. Microbial Ecol. (2022) 86:460–73.
35. Brow CN, Johnson ROB, Mouzhong XU, Johnson RL, Simon HM. Effects of cryogenic preservation and storage on the molecular characteristics of microorganisms in sediments. Environ Sci Technol. (2010) 44:8243. doi: 10.1021/es101641y
36. Rissanen AJ, Kurhela E, Aho T, Oittinen T, Tiirola M. Storage of environmental samples for guaranteeing nucleic acid yields for molecular microbiological studies. Appl Microbiol Biotechnol. (2010) 88:977. doi: 10.1007/s00253-010-2838-2
37. Hao X, Zhu P, Zhang H, Liang Y, Yin H, Liu X, et al. Mixotrophic acidophiles increase cadmium soluble fraction and phytoextraction efficiency from cadmium contaminated soils. Sci Total Environ. (2019) 655:347–55. doi: 10.1016/j.scitotenv.2018.11.221
38. Liu C, Zhao D, Ma W, Guo Y, Lee DJ. Denitrifying sulfide removal process on high-salinity wastewaters in the presence of Halomonas sp. Appl Microbiol Biotechnol. (2016) 100:1421–6. doi: 10.1007/s00253-015-7039-6
39. Mago T, Salzberg SL. FLASH: fast length adjustment of short reads to improve genome assemblies. Bioinformatics. (2011) 27:2957–63. doi: 10.1093/bioinformatics/btr507
40. Wilkins O, Capitanchik C, Luscombe N, Ule J. Ultraplex- A rapid, flexible, all-in-one fastq demultiplexer. Bioinformatics. (2021) 6:141. doi: 10.12688/wellcomeopenres
41. Shifu C, Yanqing Z, Yaru C, Jia G. fastp: an ultra-fast all-in-one FASTQ preprocessor. Bioinformatics. (2018) 34:884–90. doi: 10.1093/bioinformatics/bty560
42. Robert CE. UPARSE: highly accurate OTU sequences from microbial amplicon reads. Nat Methods. (2013) 10:996–8. doi: 10.1038/nmeth.2604
43. Qiong W, George MG, James MT, James RC. Naïve Bayesian Classifier for Rapid Assignment of rRNA Sequences into the New Bacterial Taxonomy. Appl Environ Microbiol. (2007) 73:16. doi: 10.1128/aem.00062-07
44. Gavin MD, Vincent JM, Jesse Z, Svetlana NY, James RB, Christopher MT, et al. PICRUSt2 for prediction of metagenome functions. Nat Biotechnol. (2020) 38:685–8. doi: 10.1038/s41587-020-0548-6
45. Schloss PD, Westcott SL, Ryabin T, Hall JR, Hartmann M, Hollister EB, et al. Introducing mothur: open-source, platform-independent, community-supported software for describing and comparing microbial communities. Appl Environ Microbiol. (2009) 75:7537. doi: 10.1128/AEM.01541-09
46. Nicola S, Jacques I, Levi W, Dirk G, Larisa M, Wendy SG, et al. Metagenomic biomarker discovery and explanation. GenomeBiology.com (London. Print). (2011) 12:R60. doi: 10.1186/gb-2011-12-6-r60
47. Albert B, Scott TB, Emilio OC, Noah F. Using network analysis to explore co-occurrence patterns in soil microbial communities. ISME J. (2012) 6:343–51. doi: 10.1038/ismej.2011.119
48. Jian L, Han X, Jian P, Wan Z. Effects of Potassium Application Methods on Potassium Supply Capa bility of Soil and Potassium Accumulation in Flue cured Tobacco. J Hunan Agric Univ (Nat Sci). (2000) 5:352–4.
49. Cui Y-N, Fang-Zhen, Yuan J-Z, Guo H, Wang S-M, Ma. High concentrations of sodium and chloride ions have opposing effects on the growth of the xerophyte Pugionium cornutum under saline conditions. J Plant Nutr Soil Sci. (2021) 184:88–97. doi: 10.1002/jpln.202000148
50. Jiayi F, Shuangshuang C, Jing W, Daoming WU, Qifeng MO, Shucai Z. Comprehensive evaluation of soil fertility of five typical forest stands in South China. J South China Agric Univ. (2018) 39:73–81.
51. Hua Z, Farman U, Rafiq A, Sayed Usman Ali S, Abdullah K, Muhamamd A. Response of Soil Proteobacteria to Biochar Amendment in Sustainable Agriculture- A mini review. J Soil Plant Environment. (2022) 1:16–30. doi: 10.56946/jspae.v1i2.56
52. Baldi E. Soil–plant interaction: effects on plant growth and soil biodiversity. Agronomy. (2021) 11:2378. doi: 10.3390/agronomy11122378
53. Mana OL. Amino acid composition of bulk protein and salt relationships of selected enzymes of Salinibacter ruber, an extremely halophilic bacterium. Extremophiles. (2002) 6:217–23. doi: 10.1007/s007920100241
54. Tan S, Cui C, Hou Y, Chen X, Xu A, Li W, et al. Cultivation of activated sludge using sea mud as seed to treat industrial phenolic wastewater with high salinity. Mar Pollut Bull. (2016) 114:867–70. doi: 10.1016/j.marpolbul.2016.11.026
55. Gabre VV, Venancio WS, Moraes BA, Furmam FDG, Galvo CW, Gonalves DRP, et al. Multiple effect of different plant growth promoting microorganisms on beans. Braz Arch Biol Technol. (2020) 63:e20190493. doi: 10.1590/1678-4324-solo-2020190493
56. Du TY, He HY, Zhang Q, Lu L, Mao WJ, Zhai MZ. Positive effects of organic fertilizers and biofertilizers on soil microbial community composition and walnut yield. Appl Soil Ecol. (2022) 175:104457. doi: 10.1016/j.apsoil.2022.104457
57. Seo JS, Keum YS, Li QX. Bacterial degradation of aromatic compounds. Biodegrad Environ Sci. (2009) 6:278–309. doi: 10.3390/ijerph6010278
58. Oyedoh OP, Yang W, Dhanasekaran D, Santoyo G, Glick BR, Babalola OO. Rare rhizo-Actinomycetes: A new source of agroactive metabolites. Biotechnol Adv. (2023) 67:108205. doi: 10.1016/j.biotechadv.2023.108205
59. Marin MAL, Strejcek M, Junkova P, Suman J, Santrucek J, Uhlik O. Exploring the potential of micrococcus luteus culture supernatant with resuscitation-promoting factor for enhancing the culturability of soil bacteria. Front Microbiol. (2021) 12:1715. doi: 10.3389/fmicb.2021.685263
60. Verma S, Kumar A, Joshi S, GAngola S, Rani A. Chapter 9 - Role of microorganisms in agricultural waste management. In: Advanced Microbial Technology for Sustainable Agriculture and Environment (2023). Amsterdam in Netherlands: Elsevier Inc.
61. Roriz M, Pereira SIA, Castro PML, Carvalho SMP, Vasconcelos MW. Impact of soybean-associated plant growth-promoting bacteria on plant growth modulation under alkaline soil conditions. Heliyon. (2023) 9:e14620. doi: 10.1016/j.heliyon.2023.e14620
62. Yang J, Schrader S, Tebbe CC. Legacy effects of earthworms on soil microbial abundance, diversity, and community dynamics. Soil Biol Biochem. (2024) 190:109294. doi: 10.1016/j.soilbio.2023.109294
63. Sugden AM. Microbes’ role in soil decomposition. Science. (2019) 365:769.769–771. doi: 10.1126/science.365.6455.769-i
64. Campillo-Cora C, Conde-Cid M, Arias-Estévez M, Fernández-Calviño D, Alonso-Vega F. Specific Adsorption of Heavy Metals in Soils: Individual and Competitive Experiments. Agronomy. (2020) 10(8):1113. doi: 10.3390/agronomy10081113
65. Iriti M, Scarafoni A, Pierce S, Castorina G, Vitalini S. Soil application of effective microorganisms (EM) maintains leaf photosynthetic efficiency, increases seed yield and quality traits of bean (Phaseolus vulgaris L.) plants grown on different substrates. Int J Mol Sci. (2019) 20:2327. doi: 10.3390/ijms20092327
66. Xindi Z, Hui C, Dongqing XU, Yefei J, Yinke C. Effects of photosynthetic bacteria and organic fertilizer on soil microorganisms and soil enzyme activities. Soil. (2008) 40:443–7.
67. Leffelaar PA. Dynamics of partial anaerobiosis, denitrification and water in a soil aggregate: Experimental. Soil Sci. (1987) 146:352–66.
Keywords: chloride ion, inhibitors, vibrio natriegens, Zn2+, microbiota
Citation: Ding H, Wang S, Zhang Y, Dong J, Zhang R, Zhang B, Ma S, Liu D, Cui R, Chen B, Wang Q, Sun J, Xing S, Wang Z, Shen M, Wang W and Zhang H (2025) Investigation into the impact of chloride ion uptake inhibitors on tobacco plants. Front. Soil Sci. 5:1554922. doi: 10.3389/fsoil.2025.1554922
Received: 03 January 2025; Accepted: 26 March 2025;
Published: 28 April 2025.
Edited by:
Amitava Rakshit, Banaras Hindu University, IndiaReviewed by:
Digvijay Verma, Babasaheb Bhimrao Ambedkar University, IndiaAtif Khurshid Wani, Lovely Professional University, India
Shuaibing Wang, Yuxi Normal University, China
Chaosheng Luo, Yunnan Agricultural University, China
Copyright © 2025 Ding, Wang, Zhang, Dong, Zhang, Zhang, Ma, Liu, Cui, Chen, Wang, Sun, Xing, Wang, Shen, Wang and Zhang. This is an open-access article distributed under the terms of the Creative Commons Attribution License (CC BY). The use, distribution or reproduction in other forums is permitted, provided the original author(s) and the copyright owner(s) are credited and that the original publication in this journal is cited, in accordance with accepted academic practice. No use, distribution or reproduction is permitted which does not comply with these terms.
*Correspondence: Huaibao Zhang, emhhbmdodWFpYmFvQGNhYXMuY24=; Weitao Wang, d3d0MTc4NTIzMjIwMThAMTYzLmNvbQ==; Minchong Shen, c2hlbm1pbmNob25nQGNhYXMuY24=