- 1Department of Agriculture, School of Agriculture, Mwalimu Julius K. Nyerere University of Agriculture and Technology, Butiama, Tanzania
- 2Department of Soil and Geological Sciences, College of Agriculture, Sokoine University of Agriculture, Morogoro, Tanzania
Introduction: Salt-affected soils are a global issue, affecting 1 billion hectares worldwide, including 80 million hectares in Africa. In sub-Saharan Africa (SSA), these soils originate from marine, geological, and hydrogeological sources, as well as human activities and arid climatic condition-induced salinization.
Methods: This systematic review, conducted using the PRISMA framework, provides an in-depth analysis of salt-affected soils in SSA from 1970 to the present. It highlights historical trends and emerging patterns of salinization in the region.
Results and Discussion: The review estimates that 65.6 million hectares of land in SSA are salt-affected, with key hotspots in coastal zones, river deltas like the Nile Delta, and arid areas with intensive irrigation. Generally, the coastal areas of Eastern Africa, Southwest Africa, and the West African and inland areas of the Nile Delta and Lake Chad Basin are the most vulnerable. Ethiopia is the most affected country, with 11 million hectares affected, primarily due to poor irrigation and drainage infrastructure. The study also highlights research gaps, revealing that coastal countries such as Senegal, Tanzania, and Kenya are better studied than inland areas like Chad and Mali. The in-depth review found that available estimates of salt-affected soils heavily rely on the FAO report of 1988, based on Solonchaks (saline soils) and Solonetz (sodic soils). This report was produced from the FAO Soil Map of the World at a scale of 1:5,000,000, which was created between 1970 and 1981. Due to its coarse resolution, high generalization, and environmental changes that have occurred over the decades, it may be considered outdated, presenting the need for updated data. The creation of digital fine-scale maps by integrating field and laboratory data, as well as soil data from FAO Soil Map of the World, HWSD, and WoSIS databases with remote sensing data, is highly suggested in this regard. Saline agriculture utilizing brackish water and salt-tolerant crops, improved salinity detection and monitoring, improved irrigation practices, application of gypsum and organic amendments (e.g., pressmud), and phytoremediation with halophytes are recommended. The study projects that these efforts could double agriculturally yields in affected areas, improving food security and economic resilience.
1 Introduction
Salt-affected soils pose a major global threat to agricultural productivity (1–7). Estimates indicate that approximately 1 billion hectares of land worldwide are salt-affected (3, 8, 9). Singh (10) stressed that over 3% of the world’s soils are salt-affected as a result of degradation due to salinization. Salt-affected soils are largely reported in places such as India, Pakistan, China, the United States, Argentina, Ethiopia, and Central and Western Asia (2). Agriculturally potential lands with poor irrigation water quality, low-permeability soils, and shallow saline groundwater are among the most vulnerable areas to be salt-affected (11). About 20% of total cultivated land worldwide is already salt-affected, and for irrigated land, about 33% is already affected globally and the trend of increase is projected to accelerate by the year 2050 (12). Furthermore, projections suggest that by 2050, salt-affected soils will impact 50% of the world’s arable land, thereby worsening food insecurity (2). Qadir et al. (13) and Smaoui et al. (14) claims that in 2014 it was assessed that salt-affected areas are responsible for economic losses of 27.3 billion each year worldwide. In Africa, according to Smaoui et al. (14), around 80 million hectares are salinized, with 68.8 million hectares in sub-Saharan Africa (SSA).
Salt-affected soils are generally referred to as soils with high salinity or saline soils. High salinity in these soils is a result of the presence of major cations such as sodium (Na+), potassium (K+), calcium (Ca2+), and magnesium (Mg2+), along with anions like chloride (Cl−), sulfate (SO42−), bicarbonate (HCO3−), carbonate (CO32−), and nitrate (NO3−) in quantities intolerable to most plants (5, 15–24). The impact of soil salinization varies significantly based on climatic and soil conditions, light intensity, and the growth tolerance of different plant species (25–27). The ongoing climate change is expected to amplify the detrimental effects of soil salinization (4, 28, 29). For instance, according to Hagage et al. (30), in places like the northern Nile Delta in Egypt, climate change has been and still is one of the major drivers of rising groundwater levels, thus consequently leading to salt-affected soils. Results include substantial declines in crop growth and productivity. This could lead to yield reductions exceeding 50%, particularly in arid and semi-arid regions (3, 31).
In SSA, as in other regions globally, the issue of salt-affected soils is becoming increasingly problematic. In this region, according to Tully et al. (32) salt-affected soils have already covered up to 19 million hectares of land. Other reports by Kebede (33) and Smaoui et al. (14) suggested that salt-affected soils in SSA cover up to 68.8 million hectares. However, Ivushkin et al. (3) asserted that there is enormous inadequacy of detailed information on salt-affected soils at both country and regional levels in SSA. Although salt-affected soils have significant negative impacts on agricultural production, turning productive lands into barren areas and degrading water quality and ecosystem services, research indicates that with proper management, these soils can be highly productive and have substantial economic potential (5, 8, 34–37). Realizing the economic potential of salt-affected soils requires a thorough understanding of their characteristics, extent and distribution to select appropriate technologies for effective management. However, in SSA, information on the distribution, extent and characteristics of salt-affected soils is limited and fragmented (2, 3, 14). This poses challenges for effective management and sustainable agricultural production. This systematic review aims to assess the current state of knowledge on extent and distribution of salt-affected soils in SSA from 1970 to the present as valuable information for policymaking in monitoring and managing salt-affected soils in SSA.
2 Material and methods
2.1 Geography and nature of climate of SSA
SSA consists of 49 countries, covering 24.3 million square kilometers and spanning four time zones. This expansive region occupies over 15% of the Earth’s land surface and stretches across all four hemispheres. More than half of SSA lies between the Tropics of Cancer and Capricorn, making it predominantly influenced by a tropical climate, though significant regional variations exist. The SSA climate generally is characterized by warm temperatures and distinct wet and dry seasons. The region’s climate exhibits a wide range of variability, from arid and semi-arid regions to the dense tropical rainforests of Central Africa, such as the Congo Basin, and the vast savannas that dominate much of the landscape south of the Sahel and in East Africa. SSA is generally divided into four main regions (Figure 1): West Africa, spanning from Senegal to Chad; Central Africa, covering Cameroon to the Democratic Republic of Congo; East Africa, extending from Sudan to Tanzania; and Southern Africa, stretching from Angola to South Africa.
Topographically, SSA consists largely of a plateau that slopes downward to the west, but each region has distinct landforms, climates, and vegetation zones. West Africa features humid equatorial rainforests along the coast, transitioning into semi-arid steppe lands inland. The northernmost parts, bordering the Sahara Desert, experience frequent droughts. Semi-arid zones such as the Sahel and parts of Southern Africa contrast with the arid deserts of the Namib and Kalahari. Central Africa, positioned along the equator, has a humid equatorial climate that supports extensive tropical rainforests and provides ideal conditions for cultivating high-value crops such as bananas, coffee, oil palm, and cacao.
East Africa experiences diverse climatic conditions that shape its landscapes and ecosystems. The equatorial/tropical climate, dominant in regions near the Equator, brings heavy rainfall of about 1500mm annually, particularly in areas around large water bodies like Lake Victoria and Lake Kyoga, where onshore and offshore breezes enhance precipitation. Moving away from the Equator, the savannah climate emerges between 5 to 15 degrees north and south, acting as a transitional zone between the humid equatorial regions and the arid deserts. In the drier parts of East Africa, the arid and semi-arid climate prevails, particularly in the subtropical belt, where regions like Karamoja, Turkana, and the Chalbi Desert in Northern Kenya experience arid conditions. Contrasting with these low-lying areas, the montane climate is found in the highlands and mountainous regions of East Africa, such as Kikuyu land (Mt. Kenya), Chagga land (Mt. Kilimanjaro), Bugisu land (Mt. Elgon), and Kigezi land, where cooler temperatures and increased rainfall support diverse vegetation and agricultural activities. Meanwhile, highland climates in regions like the Ethiopian Highlands experience cooler temperatures due to elevation. Southern Africa presents a diverse landscape of coastal mountains—the Cape Fold range in the west and the Drakensberg Mountains in the east—separated by an interior plateau that includes significant geographical features like the Zambezi River, Victoria Falls, and the Namib and Kalahari deserts. This climatic variability is shaped by factors such as latitude, altitude, proximity to oceans, and seasonal shifts of atmospheric systems like the Intertropical Convergence Zone (ITCZ).
2.2 Review methodology
This review utilized the Preferred Reporting Items for Systematic Reviews and Meta-Analyses (PRISMA) framework, which, according to Page et al. (38), provides a clear methodology, ensures scientific rigor, and maintains transparency in conducting systematic reviews. Consequently, this review followed a modified PRISMA flowchart, a widely recognized checklist aimed at enhancing the reporting quality of comprehensive literature reviews and meta-analyses (39).
As illustrated in Figure 2, the process consisted of four phases: identification, screening, eligibility, and inclusion. Seven databases— ScienceDirect, Google Scholar, Web of Science, Scopus, AGRIS, African Journals Online (AJOL) and SpringerLink—were employed to locate and gather articles published in peer-reviewed journals. In regions where published literature was scarce, relevant unpublished reports were also taken into account. Title’s keywords were utilized during the search to reduce the inclusion of irrelevant papers and to minimize the risk of overlooking pertinent studies.
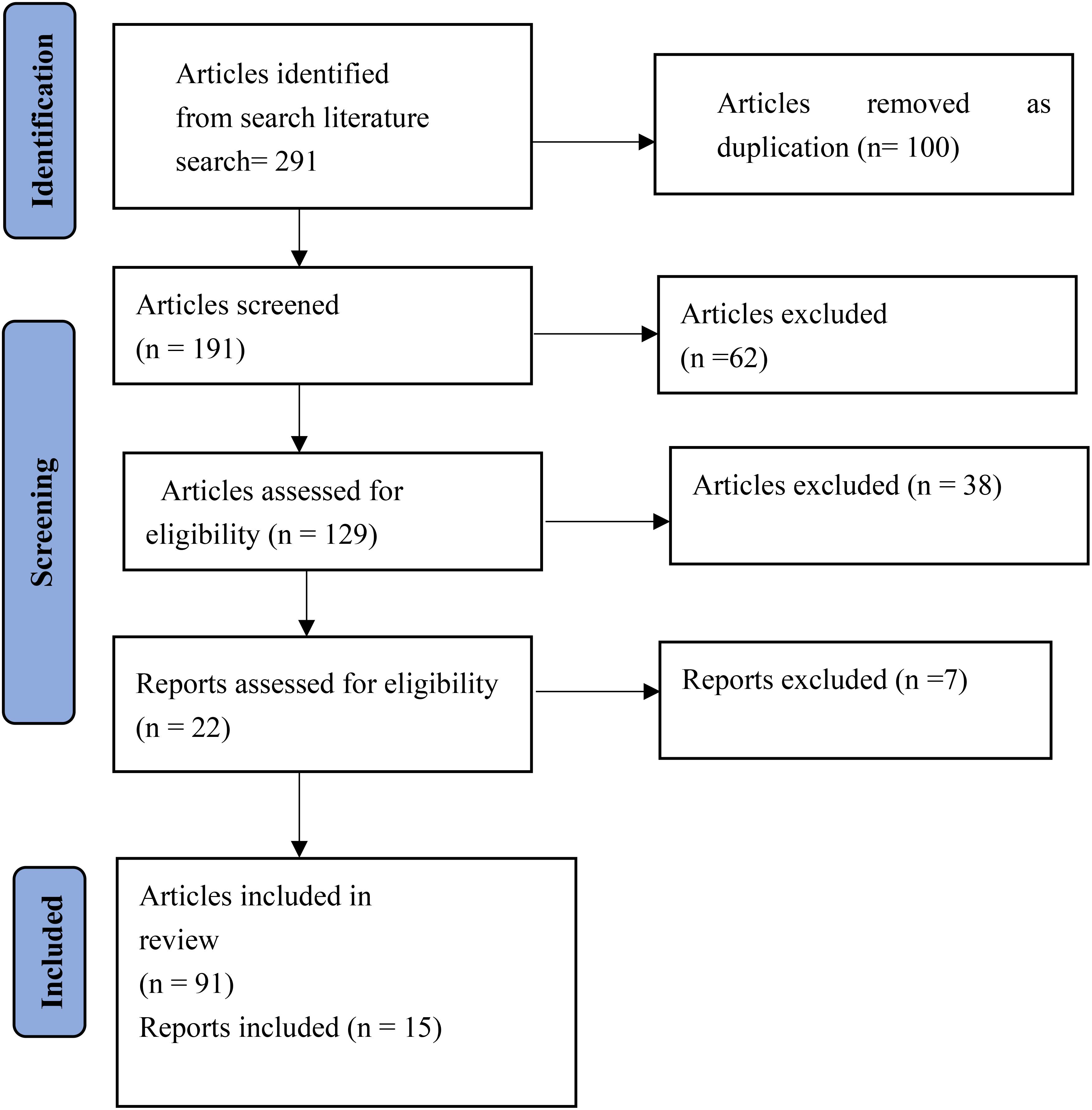
Figure 2. A modified updated guideline for reporting systematic reviews adopted from Page et al. (38).
In total, the search identified 291 journal articles and 22 reports from those databases. Among the 291 articles, 100 were duplicates and were subsequently removed. The remaining 191 articles underwent further screening by examining whether their titles, abstracts and full texts aligned with the review’s focus, and as a result, 62 papers were rejected during this process for not meeting the necessary criteria. Further screening was conducted to assess whether these articles contained relevant information, and due to the absence of directly pertinent content in their main texts, 38 articles were excluded from the remaining 129. In the case of 22 reports, 7 reported were excluded followed screening for eligibility. Ultimately, 91 articles and 15 reports were selected for examining and discussing various aspects of salt-affected soils in SSA. Figure 2 depicts the review process, from initial search to the final selection of articles, following the PRISMA approach by Page et al. (38).
3 Results and discussion
3.1 The origin of salt-affected soils in SSA
3.1.1 Salt-affected soils with marine origin
Soils affected by marine salts primarily arise from tidal influences. These soils often display a poorly developed salt crust or a pseudo-sand horizon at the surface, composed of fine aggregates of saline-alkali clay. They form when seawater extends far inland, sometimes over 100 km, in estuaries, deltas, and their tributaries, particularly in areas with alternating wet and dry periods (40, 41). During dry spells, saline water covers the land until rainy seasons push it back towards the sea. Over time, salts infiltrate the soil and enter the groundwater system. Examples include the pseudo-delta of Senegal, as well as regions in Madagascar, Tanzania, and Southwest Africa (40).
In many coastal areas, marine water interacts directly with groundwater, leading to salinization through capillary action when water tables are shallow. This process is common in arid and semi-arid regions like Senegal, Sierra Leone, Togo, Ghana, and southern Madagascar (40). Additionally, soils formed from ancient marine deposits during the Quaternary period are widespread across Africa. Where the climate favors salt accumulation, saline parent materials give rise to saline soils, as observed in the Senegal River Valley and Southwest Africa (40, 42).
Another marine source of salt-affected soils in SSA is transported marine salts. These salts, often gypsum, sodium, and magnesium sulfates, and chlorides, originate from the leaching of ancient marine rocks. In Zimbabwe and South Africa, Permian rocks are significant sources of these soluble salts (40). These salts accumulate in depressions or lower parts of valley systems where runoff water collects. During dry periods, evaporation brings these salts to the surface, which then accumulate at lower levels after floods. Even in areas with high rainfall, impermeable soils can become saline if evaporation rates are high, a common scenario in tropical areas of SSA.
In SSA, transported marine salts are a common cause of salt-affected soils in regions like northern Nigeria, Swaziland, Botswana, and South Africa (33, 40). These soils often form solonchaks with a cation exchange complex dominated by calcium, magnesium, or sodium. They may exhibit a thick salt crust or pseudo-sand horizon. Similar soils, known as alkali soils, occur in northern Nigeria, while solonetz soils are found in Botswana (40). These soils are primarily composed of chlorides and sulfates due to gypsiferous soils, with sodium being the dominant cation. In some cases, hydroscopic soils rich in calcium and magnesium chlorides have been identified, such as in the Sabi Valley of Zimbabwe, where they exhibit acidic properties (40, 43).
3.1.2 Salt-affected soils with geological and hydrogeological origin
The weathering of sodium-rich minerals such as feldspars and amphiboles releases soluble sodium salts, including carbonates, bicarbonates, sulfates, and, in rare cases, chlorides and silicates (40, 44). These minerals are widespread across SSA, and under specific climatic and topographic conditions, their breakdown can lead to the formation of saline or sodic soils. Additionally, some areas such as Ga’etel in Ethiopia undergo seismic activities which create thermal springs that introduce salts into soils (45).
In arid regions, closed drainage basins like Lake Chad frequently develop saline soils due to the accumulation of salts transported by inflowing rivers. Also, capillary rise of saline groundwater in low-lying Lake Chad Basin transports salts to root zones (33). The dominant salt-affected soils in these environments are Solonchaks, which often exhibit salt crusts on the surface or highly saline layers at shallow depths, as observed in Chad, Kenya, Botswana, and Mali (40). Some of these salt crusts, enriched with sodium carbonate or sodium chloride, are commercially extracted. Aubert (40) further reported that in marshy environments, particularly in organic-rich soils and parts of Lake Chad, diverse types of salt-affected soils are likely to emerge.
In subhumid and certain semiarid regions, excess water accumulation alters soil conditions. Dissolved sodium is leached vertically or laterally and accumulates in the B horizon, leading to the development of sodic soils such as Solonetz, solodized soils, and solodized planosols (43). These soils form under hydromorphic conditions and elevated soil temperatures and are prevalent in countries including Burkina Faso, northern Togo, northern Nigeria, northern Cameroon, Chad, Malawi, Botswana, Zimbabwe, Swaziland, and Lesotho (40). Solonetz and solodized soils are particularly vulnerable to water erosion and are often found in association with higher-lying leached tropical ferruginous soils (Chromic or Ferralitic Luvisols) and lower-lying Vertisols.
3.1.3 Climate- and human activity-induced salt-affected soils
Arid and semi-arid climates with high evaporation rates, such as those in Sudan and Botswana, cause salts to accumulate in the topsoil as water evaporates (46). Additionally, low rainfall in some areas of the Horn of Africa like Somalia limits salt leaching, worsening natural salinity and leading to the expansion of salt-affected soils (47).
Human activities including excessive use of brackish irrigation water in Nile Delta farms and Ethiopian lowlands deposits salts that accumulate as water evaporates (48). Over-irrigation in water-scarce regions like Sudan’s Gezira Scheme raises water tables, mobilizing subsoil salts (49). Inadequate drainage infrastructure in Ethiopia’s Wabi Shebelle Basin causes waterlogging, enabling salt accumulation near plant roots (48, 50). Rice cultivation in West African mangroves traps saline water, worsening topsoil salinity (51). Furthermore, over-fertilization in Kenyan and Tanzanian farms introduces soluble salts in the soils increasing salinization (14, 24).
3.2 The current state of scientific knowledge and an overview on the extent and distribution of salt-affected soils in SSA
The extent and distribution of salt-affected soils have been subjects of considerable study, yet the precise measurements remain uncertain due to the lack of reliable data and only rough estimates are available (32, 52). Estimates of salt-affected soils in this region vary significantly, ranging from 19 million hectares (32) to 68 million hectares (33). FAO (43) estimated the salt-affected soils in SSA to be about 63.9 million ha. This discrepancy, shown in Table 1, underscores the imprecision in current measurements and highlights the limited availability of comprehensive data on the subject.
Scientific knowledge on salt-affected soils is unevenly distributed across SSA. Generally, there is enormous disparity in documentation of salt-affected soils across SSA countries. Coastal countries like Senegal in West Africa and Tanzania, Kenya, and Ethiopia in East Africa are relatively better documented than inland countries such as Chad or Mali. In the case of groundwater induced salt-affected soils, countries such as Senegal, Burkina Faso, Kenya, and Ethiopia have been relatively well studied, whereas others like Mali, Chad, and South Sudan lack comprehensive analyses. In SSA, coastal areas and river deltas have the most salt-affected soils, with Ethiopia emerging as the most affected country (53). However, the apparent scarcity of data on salt-affected soils in arid and semi-arid inland areas suggests that these regions may be underrepresented in existing research. Inadequacy of knowledge of inland areas therefore presents enormous obstacle for a clear estimation of the distribution of salt-affected soils across SSA.
This review also noted a significant resemblance between the data from the older FAO (43) report and findings from recent studies on salt-affected soils in SSA. The obvious consistency of older data, such as those compiled by FAO (43), with current studies across the region further highlights the significant slowness in updating and expanding the knowledge base, as many authors continue to reference previous studies instead of generating new field and laboratory observational data, and mapping of recent salt-affected soils. Thus, due to the lack of reliable and comprehensive data on salt-affected soils in SSA, the use of information compiled by FAO (43) remains a valuable baseline for understanding the extent and distribution of these soils. FAO’s pioneering work in 1988, based on the FAO/Unesco Soil Map of the World, continues to be dependable by current literature on salinity studies in various countries within SSA. For instance, salt-affected soils data for Ethiopia (22, 32, 33, 53, 54), Sudan (49), Chad, Nigeria, Botswana, Somalia, Kenya and Mali (33) and Tanzania (55) show a greater resemblance with the findings presented by FAO (43).This resemblance highlights the dependability of FAO’s data of 1988 as a foundational reference point, especially in a region like SSA where updated and precise data are sparse and very inadequate. The type of soils identified by FAO (43) as indicators of salt-affected areas, such as Solonchaks for saline soils and Solonetz for sodic soils remain critical in identifying and mapping salt-affected soils in SSA. Van Oort (56) supports this classification, noting that these soil types are still used to indicate the presence of salt-affected soils in the region. However, overdependence on the FAO (43) report as a reference for salt-affected soils presents serious problems. The FAO (43) report relies on the FAO Soil Map of the World at a scale of 1:5,000,000, which was produced between 1970 and 1981 with a much coarser resolution and high generalization. Thus, considering the dynamic nature of soil salinity, the FAO (43) data may be considered outdated in terms of presenting recent data on salt-affected soils. Furthermore, there is a lack of time-series data on salt-affected soils, which is essential for tracking their dynamics over time for effective monitoring and mapping.
The Harmonized World Soil Database (HWSD v 1.2) of 2012 (https://www.fao.org/soils-portal/data-hub/soil-maps-and-databases/harmonized-world-soil-database-v12/en/) has presented another important opportunity for studying the extent and distribution of salt-affected soils in various regions around the world, including SSA. Van Oort (56), when using HWSD v 1.2 observed that certain soil types have a closer correlation with salinity and sodicity. Specifically, Van Oort used Solonchaks and Salic Fluvisols (SCFLs) from the HWSD v 1.2 to represent salinized soils/areas and combined Solonchaks, Salic Fluvisols (saline), and Solonetz (SCFLsSN) to represent saline or sodic soils. This approach was based on the close correlation between the electrical conductivity (EC) and exchangeable sodium percentage (ESP) values of these soil types with salinity as indicated in the HWSD soil map. The results from the HWSD v 1.2 were consistent with other data on salt-affected soils in several SSA countries, including Tanzania, Guinea, Senegal, Mozambique, Sierra Leone, and Guinea-Bissau, particularly in rice-growing soils. However, in most countries, no comparable studies on salt-affected soils were available for verification. The inherent properties of these soils contribute significantly to salinization, making them reliable indicators. For instance, Solonchaks and Solonetz are known for their high salt content and poor drainage, which favor salinization.
However, the use of HWSD v 1.2 as the source for salt-affected soils in SSA and on a global scale presents serious limitations. Although this database remains a crucial source for salinity data in various regions around the world, particularly in SSA, it has significant shortcomings. The database consists of soil mapping units, with each unit representing a single value of soil salinity and some of these units may span hundreds of kilometers. Consequently, the maps have a much coarser resolution and thus high generalization and limited details in representing spatial variability of salt-affected soils. Additionally, the database primarily relies on the FAO/UNESCO Soil Map of the World, produced between 1970 and 1981 at a coarse scale of 1:5,000,000. Given the dynamic nature of salt-affected soils, this reliance renders the data outdated. Despite these challenges, there is a great similarity in results between historical and current studies and the HWSD and FAO soil maps data, where Solonchaks and Solonetz soils were used as indicators of salt-affected soils, with their coverage representing these areas. This validates the FAO approach and reinforces the importance of Solonchaks and Solonetz soils in understanding the distribution of salt-affected soils across SSA and the whole world at large. Therefore, the FAO (43) and all its sister databases, including the World Soil Information Soil and Terrain (SOTER) database, ISRIC – World Soil Information (WoSIS), and HWSD v 1.2, serve as important baselines for studies on salt-affected soils in SSA, providing a solid foundation for future research.
3.3 Extent and distribution of salt-affected soils in SSA
The causes of salt-affected soils are diverse and complex, encompassing salts released through the weathering of rocks and soils, airborne salts, saline groundwater from underground sources, and human activities like mining, and railway development, road construction (21, 23, 47, 57–59). The extent and distribution of salt-affected soils in SSA vary significantly across different countries, with some regions affected severely while others remain relatively unaffected. Ethiopia ranks highest in Africa in terms of the extent of salt-affected soils, with an estimated 11 million hectares impacted, corresponding to 9% of the total land area and 13% of the irrigated area of the country (50, 54). These soils are concentrated in the Rift Valley, Wabi Shebelle River Basin, the Denakil Plains, and various other lowlands and valleys. Chad, Nigeria, and Botswana also face significant challenges, with 8,267,000 hectares, 6,502,000 hectares, and 5,679,000 hectares of salt-affected soils, respectively (33).
Generally, in SSA, salt-affected soils are predominantly found in Eastern African countries, along the Western African coast, within the Lake Chad Basin, and in specific areas of Southern Africa (Figure 3). Negacz et al. (60) found that arid areas of East and Southern Africa are among the most salt-affected areas. East Africa (including Horn of Africa) is the most affected sub-region in SSA (Figure 4). In East Africa Lake/river salinity is among the major drivers of salt-affected soils in this region. Areas near lakes and rivers, especially in East Africa, have some of the most salt-affected soils in SSA (61). In Table 2, countries including Ethiopia, Somalia, Kenya, and Tanzania rank among the most affected due to salinity from lakes, deltas and rivers, and similar findings were reported by Hammer (61) and Dubois (67). Schagerl and Renaut (68) also reported that lakes in Ethiopia, Kenya, and Tanzania exhibit saline conditions which accelerate the occurrence of salt-affected soils in such areas. Some saline lakes were formed through the evaporation of marine-based water sources, as seen with Lake Assal in Djibouti, where geothermal heat drove the evaporation process, resulting in a highly saline environment (45). Moreover, salt-affected soils in areas with thermal springs such as Ga’etel in Ethiopia have been contributed by earthquakes (45).
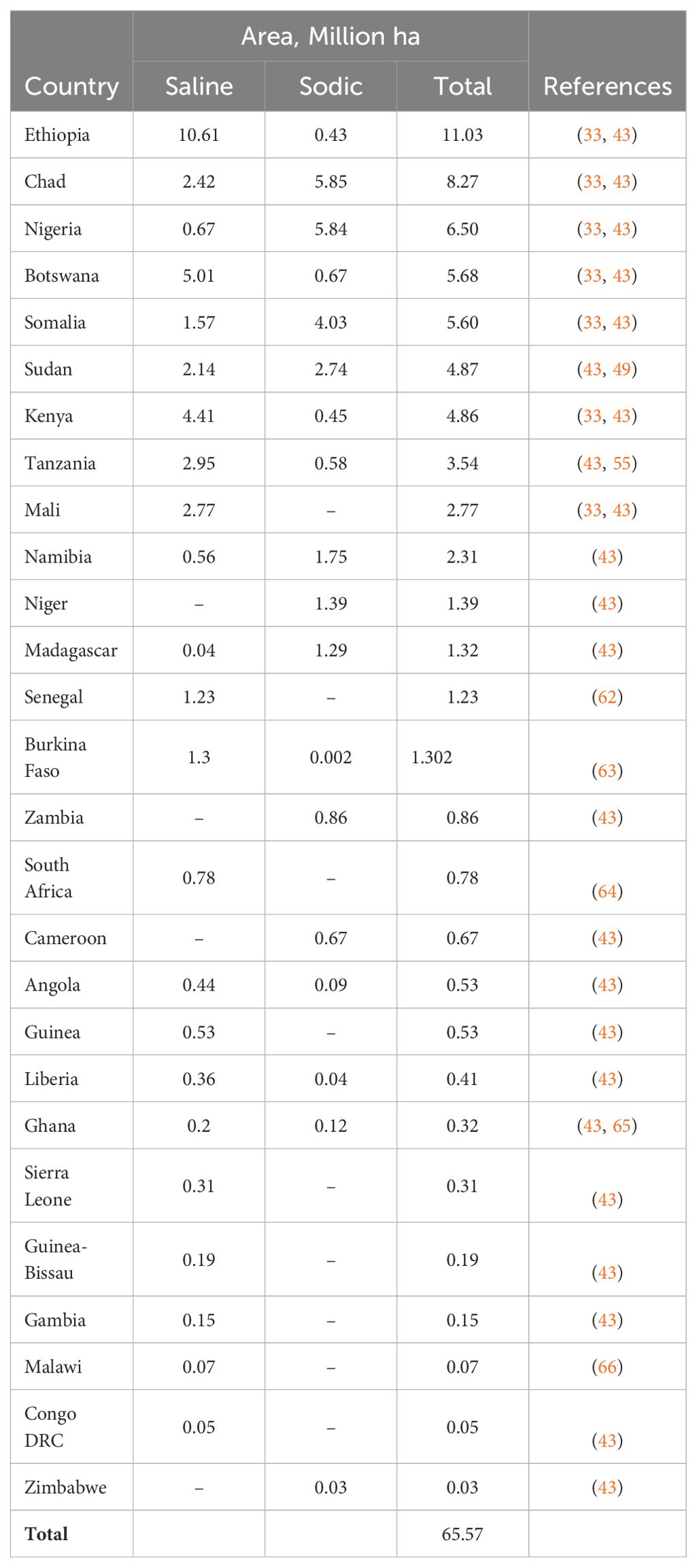
Table 2. Extent of the distribution of salt-affected soils in various SSA countries, listed in descending order from most to least affected (Million ha) as per reviewed data.
Kebede (33) and Qureshi et al. (54) highlighted that prominent countries with widespread salt-affected soils include Angola, Botswana, Burundi, Cameroon, Chad, Ethiopia, Kenya, Madagascar, Malawi, Mali, Mozambique, Niger, Nigeria, Somalia, Sudan, Rwanda, and Tanzania. In the case of the Horn of Africa which includes northeast Kenya, eastern Ethiopia, Djibouti, Eritrea, and Somalia, key drivers of salt-affected soils include precipitation patterns, evaporation, low groundwater recharge rates, proximity to the ocean and the presence of fractured rocks (47, 69). Additionally, the region has experienced repeated droughts. With many countries in this area having an arid climate and low groundwater recharge, the prevalence of salt-affected soils is expected. In contrast, countries such as Congo DRC, Congo, Gabon and Ivory Coast are almost free of saline soils, highlighting the uneven distribution of salt-affected soils across the SSA region (32, 56).
The types of soils that are most susceptible to salinity and sodicity include Solonchaks, Salic Fluvisols, and Solonetz. Van Oort (56) used these soil types, contained in HWSD v 1.2, to represent salinized and sodic areas. Solonchaks and Salic Fluvisols are particularly correlated with high electrical conductivity (EC) and exchangeable sodium percentage (ESP) values, making them reliable indicators of salt-affected soils. This classification is consistent with data on salt-affected soils in countries such as Tanzania, Guinea, Senegal, Mozambique, Sierra Leone, and Guinea-Bissau (56).
Low-lying topography and poor drainage significantly contribute to the development of salt-affected soils in SSA (70–72). Regions with extensive wetlands, such as river and coastal floodplains, deltas, and depressions, are particularly susceptible (73–76). These areas, found in West and Central Africa, include both drylands on upper and mid-slopes and wetlands in valley bottoms, where poor drainage intensifies the extent of salt-affected soils. The transition from dryland to wetland on lower slopes traps salts in the soil, hindering agricultural productivity (62). Additionally, approximately 30 million hectares of wetland areas in SSA are situated in river floodplains, which are periodically inundated and often exhibit moderate to poor drainage, further contributing to salt-affected soils (32). Irrigation can induce the development of salt-affected soils (77). Irrigation practices with poor drainage in lowland areas also play a crucial role in the development of salt-affected soils in SSA. In Ethiopia, for example, the gradual rise of the groundwater table caused by the development of large irrigation schemes in the Awash Valley, without appropriate drainage systems, has led to increased salt-affected soils (48). High evapotranspiration rates in these areas further exacerbate the problem. Similarly, in Sudan, poor soil and water management, combined with inadequate drainage in irrigated areas, contribute significantly to the increasing salt-affected soils, particularly in low rainfall regions along the Nile River terraces and agricultural schemes near Khartoum and Gezira (49). These examples illustrate the critical need for improved irrigation and drainage practices to mitigate salt-affected soils in SSA. The presence of mangrove rice cultivation in coastal areas also contributes to salt-affected soils (78–82). Countries such as Nigeria, Guinea, Sierra Leone, and Guinea-Bissau, which have significant mangrove rice areas, also face notable problems associated with salt-affected soils (51). However, a country like Tanzania does not have mangrove rice, but it still experiences salinity and sodicity issues due to inland sodic soils (56). The correlation between mangrove rice cultivation and salinity highlights the need for targeted interventions in these areas to manage and reduce the impact of salt-affected soils on agricultural productivity.
Climate is another fundamental factor influencing the distribution and extent of salt-affected soils in SSA. The region’s arid and semi-arid areas, such as Ethiopia, Somalia, Botswana and even Sudan, are particularly susceptible to soil salinization due to their climatic conditions (46). High temperatures and low rainfall in these areas lead to elevated rates of evapotranspiration, which concentrates salts in the soil as water evaporates. This process is exacerbated by the natural weathering of salt-bearing rocks and the capillary rise of saline groundwater to the soil surface (48). In Ethiopia, for instance, the Rift Valley and Wabi Shebelle River Basin experience significant salinization due to these climatic factors, compounded by inadequate drainage systems (50). Climate change further intensifies salt-affected soil issues in SSA by increasing temperatures and altering precipitation patterns, leading to more frequent and severe droughts (4). These changes exacerbate salt-affected soil problems in already vulnerable regions, such as the Nile River terraces in Sudan and the low-lying topographies of Botswana. In these areas, poor soil and water management practices, combined with climatic stressors, lead to widespread soil degradation. The impacts of climate on salt-affected soils in these areas necessitate comprehensive management strategies, including improving irrigation efficiency, implementing effective drainage systems, and adopting salt-tolerant crops to sustain agricultural productivity under changing climatic conditions (32, 33).
Coastal salt-affected soils exist in some areas in SSA resulting from various factors including seawater intrusion, flooding during high tides, intrusion through rivers and estuaries, groundwater inflows, and salt-laden aerosols (83). The West and Central African coastal zone stretches from Mauritania to Namibia and the Eastern African coastal zone including coastal areas of the island states Madagascar, Mauritius, Reunion, and Seychelles. These areas are particularly vulnerable to salinization due to their low-lying topographies and diverse ecosystems such as estuaries, deltas, wetlands, mangroves, and coral reefs (33). In many arid and semi-arid coastal areas, and sometimes humid areas, evaporation from shallow water tables exacerbates salinization, bringing saline groundwater to the surface through capillary action. (84, 85). This process is particularly severe in countries like Senegal, Sierra Leone, Togo, Ghana, and southern Madagascar (33). In humid tropical regions, coastal saline soils found in estuaries and deltas are rich in organic matter and exhibit unique characteristics when associated with mangrove vegetation such as Avicennia and Rhizophora (86). When these soils are drained, they become highly acidic and are classified as Thionic Fluvisols (87). These soils are prevalent in countries such as Sierra Leone, southern Senegal, the Gambia, Guinea Bissau, Guinea, Liberia, Nigeria, Cameroon, Gabon, Kenya, Tanzania, Mozambique, and western Madagascar, covering an estimated area of approximately 3.35 million hectares (33). The presence of soluble salts in tidal marshes and recently reclaimed sulfidic soils can inhibit water and nutrient uptake due to osmotic effects, and the toxicity of sodium (Na+) and chloride (Cl−) ions is also common. In countries like Senegal, the Gambia, and Guinea Bissau, the combination of a pronounced dry season and decreased annual rainfall over the past 20 years has led to dramatic increase in salinity levels in the topsoil with ECe values reaching 80 dS m−1 (88). Effective management strategies are essential to mitigate these impacts and sustain agricultural productivity in these coastal regions.
3.4 Salt-affected soils in irrigated areas in SSA
Irrigated areas in SSA are currently predominant salt-affected area, particularly in regions like the Nile Delta countries including Ethiopia and Sudan (22, 32, 56, 89). Salt accumulation in irrigated soils is a significant issue in SSA, driven by mechanisms such as seawater intrusion, rising groundwater in low-lying areas, and the use of saline irrigation water (90). As soil dries, salts concentrate in the soil solution, intensifying salt stress. According to Kebede (33) in regions with hot and dry climates, soils are often naturally salty, but inefficient irrigation and poor drainage intensify the problem by causing waterlogging, which raises the water table and brings salts closer to the surface. When water evaporates, salts accumulate around plant roots, preventing water absorption and stunting plant growth. The more irrigation is used to boost food production, the more soils become saline, leading to degraded soils, reduced crop productivity, increased poverty, and social instability. The observation by Dewitte et al. (91) stressed that the area covered by salt-affected soils is likely to increase when irrigation is expanded. Therefore, if irrigated agriculture expands in SSA semi-arid soil regions, it could exacerbate salinity problems in the future especially, when necessary, measures are not taken into consideration.
In addition, irrigation induced salt-affected soils are prevalent in low-rainfall regions along the Nile River terraces and agricultural schemes like those near Khartoum and Gezira (49). Poor soil and water management, coupled with inadequate drainage in irrigated areas, exacerbate the salt-affected soil issues. Similarly, Ethiopia has significant salinization problems, with an estimated 13% of the irrigated area in the country affected, particularly in the Rift Valley, Wabi Shebelle River Basin, the Denakil Plains, and other lowlands and valleys (48, 50). High evapotranspiration rates and the lack of proper drainage systems further contribute to the development of salt-affected soils in these areas, causing substantial crop losses and the abandonment of farmlands (92). Effective irrigation and drainage practices are crucial to mitigating the impact of salt-affected soils on agricultural productivity and sustainability in SSA.
3.5 The role and application of remote sensing in assessing distribution of salt-affected soils in SSA
The current review noted a lack of remote sensing applications in assessing and mapping salt-affected soils in SSA from 1970 to the present. The assessment of salt-affected soils in SSA has, for a long time, relied on conventional methods, which involve labor-intensive soil sampling and laboratory analysis. These methods, while accurate at local scales, are time-consuming, expensive, and impractical for large-scale and continuous monitoring (93). The advancement of remote sensing has revolutionized salinity mapping by overcoming these limitations. Remote sensing enables large-scale, continuous, and cost-effective assessment of salinity distribution using multispectral and hyperspectral data from satellites such as Landsat, Sentinel, MODIS, and GaoFen (93–96). These sensors detect salinity-induced spectral variations, allowing the generation of digital maps of salt-affected soils with high accuracy. Environmental covariates, including salinity indices such as the Normalized Difference Salinity Index (NDSI), vegetation indices such as the Normalized Difference Vegetation Index (NDVI) and Soil-Adjusted Vegetation Index (SAVI), and moisture indices such as the Normalized Difference Water Index (NDWI), derived from remote sensing imagery are used to predict salinity distribution with machine learning models such as Random Forest (RF) and Support Vector Machine (SVM) with enhanced predictive accuracy (93, 95).
Unlike spatial interpolation, which often produces approximate distributions, remote sensing-based models offer more precise spatial characterization of salt-affected soils, enabling effective land management decisions. Additionally, remote sensing provides frequent updates, facilitating progressive monitoring of salinity dynamics across diverse landscapes, including remote and inaccessible areas such as wetlands and coastal zones (97, 98). Its integration with digital soil mapping further enhances predictive accuracy, reducing reliance on intensive field sampling. The widespread availability of satellite imagery ensures that salt-affected soil assessments can be conducted at different spatial and temporal scales, offering a more efficient alternative to conventional methods. Given the increasing soil salinization due to human activities and climate change, remote sensing offers a crucial solution for generating reliable, updatable, transferable, and reproducible data. This enables faster, cost-effective and more accurate assessment and mapping of salt-affected soils, supporting sustainable agricultural and land management strategies in SSA. Nevertheless, the application of remote sensing in accessing salt-affected soils does not operate completely free of shortcomings. According to Wen et al. (29), the spatiotemporal and spectral resolution of current satellites are still significant limitations in accessing salt-affected soils through monitoring plant traits in response to salinity stress. Although this has greatly improved with the launch of the Sentinel satellites, insufficient spatiotemporal resolution and revisit periods remain major constraints for current satellite sensor applications in accessing and monitoring salt-affected soils through crop monitoring.
3.6 Reclamation of salt-affected soils in SSA
To achieve sustainable reclamation of salt-affected soils, management strategies should be designed according to the specific type and severity of soil salt-affected soils, while also considering the availability and affordability of site-specific amendment materials for farmers. Current salt-affected soil reclamation strategies in SSA include various direct and adaptive approaches. The type and degree of severity largely dictate the methods and strategies of reclaiming salt-affected soils in SSA. In some countries, such as Sudan, saline soils are reclaimed through leaching and drainage, while sodic soils require calcium amendments, such as gypsum, to replace sodium and improve soil structure for enhanced crop productivity (99). Salt-tolerant pasture crops are widely promoted, as seen in Ethiopia, where farmers are educated on their benefits for livestock production, particularly in nomadic pastoralist communities (14). In Tanzania, Omar et al. (100) reported that farmers have adopted indigenous methods such as farmyard manure (FYM), burned and unburned rice husks, burned rice straw, and flushing techniques to manage salt-affected soils, but their knowledge of salt-tolerant cultivars in irrigation schemes remains limited. Portable soil sensors and remote sensing for real-time salinity monitoring are being employed, as demonstrated in Mozambique, to improve land management decisions (14, 101).
Agroforestry and composting help enhance soil organic matter and mitigate salinity effects, exemplified by Kenya and Senegal, where these methods have been encouraged despite resource limitations (14, 102). Management strategies for salt-affected soils through land use planning can improve resilience in saline environments. A notable example is raised-bed planting and irrigation modifications are used to reduce salt-affected soil impacts on crops, with Senegal serving as a case study for their implementation (102). The use of salt-tolerant crop cultivars is another approach that is implemented in several SSA countries, including Ethiopia and Gambia. However, in Gambia, land abandonment remains a challenge due to increasing soil-affected soils (14).
Failure to address salt-affected lands can result in revenue losses ranging from 15% to 69%, depending on factors such as crop type, land degradation severity, irrigation water quality, drainage efficiency, and soil and water management practices (13). The economic impact of salt-induced land degradation has been assessed in countries such as Australia, India, the United States, Iraq, Pakistan, Kazakhstan, and Spain (103–109). However, limited research, methodological constraints, and inconsistencies in results make it challenging to determine the reliability and accuracy of these estimates.
For irrigated areas, the economic losses caused by salt-affected soils can be inferred from Qadir et al. (13), who in 2013 estimated that, the annual global cost of salt-induced land degradation in irrigated areas was US$ 441 per hectare. Various studies attempting to assess the net benefits of restoring salt-affected lands have shown significant variability, influenced by differences in methodology, materials used, salinity levels, and input costs. Despite these variations, research consistently demonstrates that reclaiming salt-affected soils can double agricultural yields (106, 108, 110, 111). Thus, although precise projections for SSA remain uncertain, existing literature strongly suggests that reclaiming salt-affected lands in the SSA could double the yield in areas with salt-affected soils.
4 Conclusions and recommendations
Salt-affected soils in SSA originate from marine, geological, hydrogeological, and climate-induced factors. Marine salts infiltrate coastal soils through tidal action, seawater intrusion, and ancient marine deposits. Geological weathering releases sodium-rich minerals, while hydrogeological processes transport salts via groundwater and rivers, forming saline basins. Climate factors, including high evaporation in arid regions, cause salt accumulation, while human activities such as irrigation with brackish water, poor drainage, and over-fertilization accelerate soil salinization. These processes result in widespread salinization across SSA, affecting agricultural productivity in countries like Sudan, Ethiopia, Nigeria, Senegal, Madagascar, Botswana, and Zimbabwe.
Salt-affected soils in SSA cover approximately 65.6 million hectares. Key affected regions include Eastern Africa, the Western African coast, the Lake Chad Basin, and some parts of Southern Africa such as Botswana. Notable hotspots include Ethiopia’s Rift Valley and Sudan’s Nile River terraces. Salt-affected soils are most common in arid climates, low-lying areas, and regions with intensive irrigation. Major causes include natural factors like saline groundwater movement and human-induced issues such as inefficient irrigation and poor water management.
Data on salt-affected soils in SSA remain fragmented and rely heavily on old sources, such as the FAO (43) report. Despite their high generalization, databases like HWSD, FAO Global Soil Map and Database, World Soil Information Soil and Terrain (SOTER) databases, and ECe point data from the WoSIS database are valuable sources of information on salt-affected soils in SSA for both older and current literature. The estimation of salt-affected soil coverage, as represented by Solonchaks (saline soils) and Solonetz (sodic soils) in the FAO World Soil Map and HWSD, has shown strong alignment with findings in recent literature. This consistency suggests that Solonchaks and Solonetz serve as a crucial starting point and foundational baseline for studying and estimating the extent and distribution of salt-affected soils, not only in SSA but globally.
There is a significant lack of new field and laboratory observational data and time-series studies to monitor changes over time, due to the shortage of use of advanced remote sensing techniques, which could address the limitations of traditional soil mapping. These technologies enable high-resolution and dynamic salinity maps by integrating data on thermal infrared imagery, soil properties, and environmental covariates in a machine learning environment for fine-scale mapping of salt-affected soils. The current strategies for the reclamation of salt-affected soils across SSA include leaching, gypsum application, agroforestry, composting, and the use of salt-tolerant crops. Research projects that these measures can double yields in areas with salt-affected soils, improving food security and economic resilience. The above conclusions lead to the following recommendations:
● Improve data collection and mapping by enhancing new field and laboratory observations and utilizing advanced technologies like GIS and remote sensing to achieve accurate and dynamic mapping of salt-affected soils at a fine scale.
● Conduct targeted research in under-explored arid and semi-arid regions to better understand the distribution of salt-affected soils.
● Adopt sustainable irrigation practices by improving irrigation efficiency, using saline water carefully, and upgrading drainage systems to reduce the risks of salinization.
● Implement land management techniques such as promoting mulching and crop rotation to conserve soil moisture and reduce evaporation, particularly in areas with high evapotranspiration relative to precipitation.
● Encourage saline agriculture by cultivating salt-tolerant crops and employing brackish water irrigation, particularly in high-salinity areas like the Nile Delta and coastal regions, to restore degraded soils.
● Application of Gypsum (calcium sulfate) which effectively displaces sodium ions in sodic soils through cation exchange, reducing soil sodicity and improving permeability.
● Designing effective drainage systems for salt-affected lands is essential. Drainage systems and leaching help mitigate salt accumulation and waterlogging by requiring multiple pore volumes of water to flush displaced salts, particularly in calcareous soils, while subsurface drainage prevents the capillary rise of salts in waterlogged areas by effectively managing shallow wetting zones.
● Application of organic amendments which play a crucial role in combating salt-affected soils by improving soil structure and nutrient availability. For instance, press mud supplies calcium sulfate and organic carbon to reduce reliance on pure gypsum, while compost and farmyard manure enhance microbial activity and water retention, collectively contributing to better soil health and salinity management.
● Phytoremediation using halophytic plants, such as Suaeda maritima and Salicornia europaea, to directly uptake sodium and chloride ions or indirectly improve soil structure through root-induced cation exchange, achieving comparable efficiency to chemical treatments while being more cost-effective.
● Provide policy and institutional support by creating incentives for adopting advanced technologies and sustainable practices to ensure long-term soil health and agricultural productivity.
Author contributions
FM: Conceptualization, Data curation, Formal Analysis, Investigation, Methodology, Resources, Validation, Visualization, Writing – original draft, Writing – review & editing.
Funding
The author declare that no financial support was received for the research and/or publication of this article.
Acknowledgments
The author would like to thank Sokoine University of Agriculture for being the hosting institution during the course of writing this paper.
Conflict of interest
The author declares that the research was conducted in the absence of any commercial or financial relationships that could be construed as a potential conflict of interest.
Generative AI statement
The author(s) declare that Generative AI was used in the creation of this manuscript. During the preparation of this work the corresponding author used GPT-4o in order to improve language and readability. After using this tool, the author reviewed and edited the content as needed and takes full responsibility for the content of the publication.
Publisher’s note
All claims expressed in this article are solely those of the authors and do not necessarily represent those of their affiliated organizations, or those of the publisher, the editors and the reviewers. Any product that may be evaluated in this article, or claim that may be made by its manufacturer, is not guaranteed or endorsed by the publisher.
References
1. Setia R, Gottschalk P, Smith P, Marschner P, Baldock J, Setia D, et al. Soil salinity decreases global soil organic carbon stocks. Sci Total Environ. (2013) 465:267–72. doi: 10.1016/J.SCITOTENV.2012.08.028
2. Butcher K, Wick AF, DeSutter T, Chatterjee A, Harmon J. Soil salinity: A threat to global food security. Agron J. (2016) 108:2189–200. doi: 10.2134/agronj2016.06.0368
3. Ivushkin K, Bartholomeus H, Bregt AK, Pulatov A, Kempen B, De Sousa L. Global mapping of soil salinity change. Remote Sens Environ. (2019) 231:111260. doi: 10.1016/j.rse.2019.111260
4. Corwin DL, Scudiero E. Review of soil salinity assessment for agriculture across multiple scales using proximal and/or remote sensors. Adv Agron. (2019) 158:1–130. doi: 10.1016/bs.agron.2019.07.001
5. Hopmans JW, Qureshi AS, Kisekka I, Munns R, Grattan SR, Rengasamy P, et al. Critical knowledge gaps and research priorities in global soil salinity. Adv Agron. (2021) 169:1–191. doi: 10.1016/BS.AGRON.2021.03.001
6. Khasanov S, Kulmatov R, Li F, van Amstel A, Bartholomeus H, Aslanov I, et al. Impact assessment of soil salinity on crop production in Uzbekistan and its global significance. Agric Ecosyst Environ. (2023) 342:108262. doi: 10.1016/J.AGEE.2022.108262
7. Rui LI, Wenyou HU, Zhongjun JIA, Hanqiang LIU, Zhang C, Huang B, et al. Soil degradation: a global threat to sustainable use of black soils. Pedosphere. (2024) 35(1):264–79. doi: 10.1016/J.PEDSPH.2024.06.011
8. Wicke B, Smeets E, Dornburg V, Vashev B, Gaiser T, Turkenburg W, et al. The global technical and economic potential of bioenergy from salt-affected soils. Energy Environ Sci. (2011) 4:2669–81. doi: 10.1039/C1EE01029H
9. Singh A. Soil salinization management for sustainable development: A review. J Environ Manage. (2021) 277:111383. doi: 10.1016/J.JENVMAN.2020.111383
10. Singh A. Salinization of agricultural lands due to poor drainage: A viewpoint. Ecol Indic. (2018) 95:127–30. doi: 10.1016/J.ECOLIND.2018.07.037
11. Hagage M, Abdulaziz AM, Elbeih SF, Hewaidy AGA. Monitoring soil salinization and waterlogging in the northeastern Nile Delta linked to shallow saline groundwater and irrigation water quality. Sci Rep. (2024) 14:27838. doi: 10.1038/s41598-024-77954-x
12. Mukhopadhyay R, Sarkar B, Jat HS, Sharma PC, Bolan NS. Soil salinity under climate change: Challenges for sustainable agriculture and food security. J Environ Manage. (2021) 280:111736. doi: 10.1016/J.JENVMAN.2020.111736
13. Qadir M, Quillérou E, Nangia V, Murtaza G, Singh M, Thomas RJ, et al. “Economics of salt-induced land degradation and restoration”. In: Natural resources forum. Hoboken, New Jersey, US: John Wiley & Sons Ltd, vol. 38. (2014). p. 282–95. doi: 10.1111/1477-8947.12054
14. Smaoui J, Negacz KK, van Tongeren P. Salinity in African Countries: From Local Challenges to Global Solutions. AMSTERDAM, The Netherlands: Vrije Universiteit Amsterdam (2024). doi: 10.17605/OSF.IO/MCB48.
15. Shannon MC, Grieve CM. Tolerance of vegetable crops to salinity. Scientia Hortic. (1998) 78:5–38. doi: 10.1016/S0304-4238(98)00189-7
16. Chhabra R. Classification of salt-affected soils. Arid Land Res Manage. (2004) 19(1):61–79. doi: 10.1080/15324980590887344
17. Bui EN. Soil salinity: a neglected factor in plant ecology and biogeography. J Arid Environ. (2013) 92:14–25. doi: 10.1016/j.jaridenv.2012.12.014
18. Bernstein N. Plants and salt: Plant response and adaptations to salinity. In: Model ecosystems in extreme environments. Amsterdam, The Netherlands: Academic Press (2019). p. 101–12. doi: 10.1016/B978-0-12-812742-1.00005-2
19. Munns R, Passioura JB, Colmer TD, Byrt CS. Osmotic adjustment and energy limitations to plant growth in saline soil. New Phytol. (2020) 225:1091–6. doi: 10.1111/nph.15862
20. Zhao C, Zhang H, Song C, Zhu JK, Shabala S. Mechanisms of plant responses and adaptation to soil salinity. Innovation. (2020) 1.
21. Stavi I, Thevs N, Priori S. Soil salinity and sodicity in drylands: A review of causes, effects, monitoring, and restoration measures. Front Environ Sci. (2021) 9:712831. doi: 10.3389/fenvs.2021.712831
22. Walche A, Haile W, Kiflu A, Tsegaye D. Assessment and characterization of agricultural salt-affected soils around Abaya and Chamo Lakes, South Ethiopia Rift Valley. Appl Environ Soil Sci. (2023) 2023:3946508. doi: 10.1155/2023/3946508
23. Sparks DL, Singh B, Siebecker MG. “Chapter 10 - The Chemistry of Saline and Sodic Soils”. In: Sparks DL, Singh B, Siebecker MGBT-ESC, editors. Environmental Soil Chemistry (Third Edition). Boston: Academic Press. (2024). pp 411–438. doi: 10.1016/B978-0-443-14034-1.00010-1
24. Omar MDM, Massawe BH, Shitindi MJ, Pedersen O, Meliyo JL, Fue KG. Assessment of salt-affected soil in selected rice irrigation schemes in Tanzania: understanding salt types for optimizing management approaches. Front Soil Sci. (2024) 4:1372838/BIBTEX. doi: 10.3389/fsoil.2024.1372838
25. Acosta-Motos JR, Ortuño MF, Bernal-Vicente A, Diaz-Vivancos P, Sanchez-Blanco MJ, Hernandez JA. Plant responses to salt stress: adaptive mechanisms. Agronomy. (2017) 7:18. doi: 10.3390/agronomy7010018
26. Shrivastava P, Kumar R. Soil salinity: A serious environmental issue and plant growth promoting bacteria as one of the tools for its alleviation. Saudi J Biol Sci. (2015) 22:123–31. doi: 10.1016/j.sjbs.2014.12.001
27. Tang H, Du L, Xia C, Luo J. Bridging gaps and seeding futures: A synthesis of soil salinization and the role of plant-soil interactions under climate change. Iscience. (2024) 27(9):110804.
28. Eswar D, Karuppusamy R, Chellamuthu S. Drivers of soil salinity and their correlation with climate change. Curr Opin Environ Sustain. (2021) 50:310–8. doi: 10.1016/j.cosust.2020.10.015
29. Wen W, Timmermans J, Chen Q, van Bodegom PM. A review of remote sensing challenges for food security with respect to salinity and drought threats. Remote Sens. (2020) 13(1):6. doi: 10.3390/rs13010006
30. Hagage M, Hewaidy AGA, Abdulaziz AM. Groundwater quality assessment for drinking, irrigation, aquaculture, and industrial uses in the waterlogged northeastern Nile Delta, Egypt: a multivariate statistical approach and water quality indices. Model Earth Syst Environ. (2025) 11:59. doi: 10.1007/s40808-024-02242-6
31. Anami BS, Malvade NN, Palaiah S. Classification of yield affecting biotic and abiotic paddy crop stresses using field images. Inf Process Agric. (2020) 7:272–85. doi: 10.1016/j.inpa.2019.08.005
32. Tully K, Sullivan C, Weil R, Sanchez P. The state of soil degradation in Sub-Saharan Africa: Baselines, trajectories, and solutions. Sustainability. (2015) 7:6523–52. doi: 10.3390/su7066523
33. Kebede F. Status, drivers, and suggested management scenarios of salt-affected soils in Africa. In: Biosaline Agriculture as a Climate Change Adaptation for Food Security. Springer International Publishing, Cham (2023). p. 259–84. doi: 10.1007/978-3-031-24279-3_13
34. Hamada AM, Hamada YM. Management of abiotic stress and sustainability. In: Plant Life under Changing Environment. Amsterdam, The Netherlands: Academic Press (2020). p. 883–916. doi: 10.1016/B978-0-12-818204-8.00041-2
35. Li CY, He R, Tian CY, Song J. Utilization of halophytes in saline agriculture and restoration of contaminated salinized soils from genes to ecosystem: Suaeda salsa as an example. Marine Pollut Bull. (2023) 197:115728. doi: 10.1016/j.marpolbul.2023.115728
36. Marroquin A, Holmes K, Salazar D. Soil salinization and chemically mediated plant-insect interactions in a changing climate. Curr Opin Insect Sci. (2023) 60:101130. doi: 10.1016/j.cois.2023.101130
37. Navarro-Torre S, Garcia-Caparrós P, Nogales A, Abreu MM, Santos E, Cortinhas AL, et al. Sustainable agricultural management of saline soils in arid and semi-arid Mediterranean regions through halophytes, microbial and soil-based technologies. Environ Exp Bot. (2023) 212:105397. doi: 10.1016/j.envexpbot.2023.105397
38. Page MJ, McKenzie JE, Bossuyt PM, Boutron I, Hoffmann TC, Mulrow CD, et al. The PRISMA 2020 statement: an updated guideline for reporting systematic reviews. Bmj. (2021) 372. doi: 10.1136/bmj.n71
39. Mishra V, Mishra MP. “PRISMA for review of management literature–method, merits, and limitations–an academic review”. In: Advancing Methodologies of Conducting Literature Review in Management Domain. Leeds, England, United Kingdom: Emerald Publishing Limited (2023). p. 125–36. doi: 10.1108/S2754-586520230000002007
41. Ashrafuzzaman M, Artemi C, Santos FD, Schmidt L. Current and future salinity intrusion in the south-western coastal region of Bangladesh. Spanish J Soil Sci. (2022) 12:10017. doi: 10.3389/sjss.2022.10017
42. Ndiaye R. Geographic information science: Contribution to understanding salt and sodium affected soils in the Senegal River Valley. Kansas State University (2009). Available online at: https://www.proquest.com/openview/7b818f8bf4b3a93c0a968331608a1975/1?cbl=18750&pq-origsite=gscholar (Accessed March 15, 2025).
43. FAO. Salt-Affected Soils and their Management (1988). Available online at: https://www.fao.org/4/x5871e/x5871e00.htm (Accessed September 22, 2024).
44. Öztürk M, Dişli E. Hydrochemical and environmental isotopes characteristic of groundwater and controlling factors for waters’ chemical composition in the iron–copper mine area of Elazığ, SE Turkey. Environ Chem. (2022) 19:350–74. doi: 10.1071/EN22070
45. Pérez E, Chebude Y. Chemical analysis of Gaet’ale, a hypersaline pond in Danakil Depression (Ethiopia): New record for the most saline water body on Earth. Aquat Geochem. (2017) 23:109–17. doi: 10.1007/S10498-017-9312-Z
46. Omuto CT, Kome GK, Ramakhanna SJ, Muzira NM, Ruley JA, Jayeoba OJ, et al. Trend of soil salinization in Africa and implications for agro-chemical use in semi-arid croplands. Sci Total Environ. (2024) 951:175503. doi: 10.1016/j.scitotenv.2024.175503
47. Gurmessa SK, MacAllister DJ, White D, Ouedraogo I, Lapworth D, MacDonald A. Assessing groundwater salinity across Africa. Sci Total Environ. (2022) 828:154283. doi: 10.1016/j.scitotenv.2022.154283
48. Borena FR, Hassen JM. Impacts of soil salinity on irrigation potential: In the case of Middle Awash, Ethiopian Review. Open Access Library J. (2022) 9:1–18. doi: 10.4236/oalib.1108123
49. Salih SA, Elsheik MA. The nature and properties of salt affected soil in South Khartoum. Inter Conf Bio Civil Environ Eng. (2014) 3:17–8. doi: 10.15242/IICBE.C0314140?
50. Hailu B, Wogi L, Tamiru S. Salinity Status of Soils of Irrigated Lands and Irrigation Water Quality at Raya Alamata District, Northern Ethiopia. Int J Res Agric Sci. (2020) 7(4):2348–3997. Available online at: https://www.researchgate.net/publication/344189935_Salinity_Status_of_Soils_of_Irrigated_Lands_and_Irrigation_Water_Quality_at_Raya_Alamata_District_Northern_Ethiopia (Accessed November 10, 2024).
51. Diagne A, Amovin-Assagba E, Futakuchi K, Wopereis MC. Estimation of cultivated area, number of farming households and yield for major rice-growing environments in Africa. In: Realizing Africa’s rice promise. CABI, Wallingford UK (2013). p. 35–45. doi: 10.1079/9781845938123.0035
52. FAO. More information on Salt-affected soils | FAO SOILS PORTAL. Food and Agriculture Organization of the United Nations (2018). Available online at: https://www.fao.org/soils-portal/soil-management/management-of-some-problem-soils/salt-affected-soils/more-information-on-salt-affected-soils/en/ (Accessed September 25, 2024).
53. Tessema N, Yadeta D, Kebede A, Ayele GT. Soil and irrigation water salinity, and its consequences for agriculture in Ethiopia: a systematic review. Agriculture. (2022) 13(1):109. doi: 10.3390/agriculture13010109
54. Qureshi AS, Mohammed M, Daba AW, Hailu B, Belay G, Tesfaye A, et al. Improving agricultural productivity on salt-affected soils in Ethiopia: Farmers’ perceptions and proposals. Afr J Agric Res. (2019) 14:897–906. doi: 10.5897/AJAR2019.14077
55. FAO. Land resource potential and constraints at regional and country levels Vol. 90. World Soil Resources Reports (2000). Available online at: ftp://ftp.fao.org/agl/agll/docs/wsr.pdf (Accessed November 24, 2024).
56. Van Oort PAJ. Mapping abiotic stresses for rice in Africa: Drought, cold, iron toxicity, salinity and sodicity. Field Crops Res. (2018) 219:55–75. doi: 10.1016/j.fcr.2018.01.016
57. McCune DC. Effects of airborne saline particles on vegetation in relation to variables of exposure and other factors. Environ Pollut. (1991) 74:176–203. doi: 10.1016/0269-7491(91)90069-9
58. Khaska M, La Salle CLG, Lancelot J, Mohamad A, Verdoux P, Noret A, et al. Origin of groundwater salinity (current seawater vs. saline deep water) in a coastal karst aquifer based on Sr and Cl isotopes. Case study of the La Clape massif (southern France). Appl Geochem. (2013) 37:212–27. doi: 10.1016/j.apgeochem.2013.07.006
59. Leppänen JJ, Luoto TP, Weckström J. Spatio-temporal impact of salinated mine water on Lake Jormasjärvi, Finland. Environ Pollut. (2019) 247:1078–88. doi: 10.1016/j.envpol.2019.01.111
60. Negacz K, Malek Ž, de Vos A, Vellinga P. Saline soils worldwide: Identifying the most promising areas for saline agriculture. J Arid Environ. (2022) 203:104775. doi: 10.1016/j.jaridenv.2022.104775
61. Hammer UT. Saline lake ecosystems of the world Vol. 59. Springer Science & Business Media (1986). Available online at: https://books.google.com/books?hl=en&lr=&id=NOdvPFm6SyoC&oi=fnd&pg=PR9&dq=Hammer:+Saline+lake+ecosystems+of+the+world&ots=E0tTqVhPnn&sig=0nZOOYtr1VQ48UY1KtYt_2CfpbU (Accessed November 17, 2024).
62. Diatta S. Improving pearl millet (Pennisetum glaucum (L.) R. Br.) productivity in salt-affected soils in Senegal: a greenhouse and field investigation. Doctoral dissertation, Virginia Tech (2016). Available online at: https://vtechworks.lib.vt.edu/items/cf528b60-234a-40e8-bc52-59223d40dd01 (Accessed November 10, 2024).
63. Kabore D, Traore M, Segda Z, Sawadogo A, Omuto CT. Statement of soil salinity in Burkina Faso by Kabore. Food and Agriculture Organisation (2021). Available online at: https://www.fao.org/fileadmin/user_upload/GSP/GSAS21/017.pdf (Accessed February 2, 2025).
64. De Villiers MC, Nell JP, Barnard RO, Henning A. Salt-affected soils: South Africa. Food agricultural organization contract No. PR, 26897 (2003). Available online at: https://www.researchgate.net/profile/Anoop-Srivastava/post/I-am-looking-for-a-recent-soil-salinity-map-of-Africa/attachment/59d654de79197b80779ac3f2/AS%3A523166767423489%401501744085582/download/faosodicrza+%283%29.doc (Accessed November 30, 2024).
65. Sackey L, Osei E, Bennoah EO, Tettey A. Assessment of Gypsum as a Soil Ameliorant on Salt-affected Soils in the Ho-Keta Plain of the Volta Region, Ghana. West Bengal, India: International Journal of Plant & Soil Science (2021). doi: 10.9734/ijpss/2022/v34i2131281.
66. Kandinga TEH. Implications of Shallow Groundwater Dynamics on Water and Salinity Management at Kasinthula Sugarcane Scheme, Malawi. University of Pretoria (South Africa (2019). Available online at: https://www.proquest.com/openview/8a48374b453a3737b6c022adfdba0bea/1?pq-origsite=gscholar&cbl=2026366&diss=y (Accessed January 15, 2025).
67. Dubois O. The state of the world’s land and water resources for food and agriculture: managing systems at risk. New York, NY, US: The Food and Agriculture Organization of the United Nations and Earthscan (2011). pp. xxiii+–285. doi: 10.5555/20123051697.
68. Schagerl M, Renaut RW. Dipping into the soda lakes of East Africa. New York, NY, USA: Springer International Publishing (2016) p. 3–24. doi: 10.1007/978-3-319-28622-8_1
69. Araya D, Podgorski J, Berg M. Groundwater salinity in the Horn of Africa: Spatial prediction modeling and estimated people at risk. Environ Int. (2023) 176:107925. doi: 10.1016/j.envint.2023.107925
70. Befus KM, Barnard PL, Hoover DJ, Finzi Hart JA, Voss CI. Increasing threat of coastal groundwater hazards from sea-level rise in California. Nat Climate Change. (2020) 10:946–52. doi: 10.1038/s41558-020-0874-1
71. Magnan AK, Oppenheimer M, Garschagen M, Buchanan MK, Duvat VK, Forbes DL, et al. Sea level rise risks and societal adaptation benefits in low-lying coastal areas. Sci Rep. (2022) 12:10677. doi: 10.1038/s41598-022-14303-w
72. Walche A, Haile W, Kiflu A, Tsegaye D. Spatial analysis and mapping of intensity and types of agricultural salt-affected soils around Abaya and Chamo Lakes, South Ethiopia Rift Valley. Heliyon. (2024) 10:e33410.
73. Zhang TT, Zeng SL, Gao Y, Ouyang ZT, Li B, Fang CM, et al. Assessing impact of land uses on land salinization in the Yellow River Delta, China using an integrated and spatial statistical model. Land Use Policy. (2011) 28:857–66. doi: 10.1016/j.landusepol.2011.03.002
74. Islam SN. Deltaic floodplains development and wetland ecosystems management in the Ganges–Brahmaputra–Meghna Rivers Delta in Bangladesh. Sustain Water Resour Manage. (2016) 2:237–56. doi: 10.1007/s40899-016-0047-6
75. Mastrocicco M, Busico G, Colombani N, Usai A, Ruberti D. Seasonal salinity variations in a Coastal Wetland induced by complex interactions between sea, river and evapoconcentration processes. In: Estuaries and Coastal Zones in Times of Global Change: Proceedings of ICEC-2018. Gateway East, Singapore: Springer Singapore (2020). p. 77–88. doi: 10.1007/978-981-15-2081-5_6
76. Ferreira CS, Kašanin-Grubin M, Solomun MK, Sushkova S, Minkina T, Zhao W, et al. Wetlands as nature-based solutions for water management in different environments. Curr Opin Environ Sci Health. (2023) 33:100476. doi: 10.1016/j.coesh.2023.100476
77. Mohanavelu A, Naganna SR, Al-Ansari N. Irrigation induced salinity and sodicity hazards on soil and groundwater: An overview of its causes, impacts and mitigation strategies. Agriculture. (2021) 11:983. doi: 10.3390/agriculture11100983
78. Haque SA. Salinity problems and crop production in coastal regions of Bangladesh. Pakistan J Bot. (2006) 38:1359–65. https://citeseerx.ist.psu.edu/document?repid=rep1&type=pdf&doi=6f06fdce0c77e0e8bac5543255d288cace3bf2b0 (Accessed December 17, 2024).
79. Bhambure AB, Kerkar S. Traditionally cultivated rice varieties in coastal saline soils of India (2016). Available online at: http://irgu.unigoa.ac.in/drs/handle/unigoa/4410 (Accessed November 28, 2024).
80. Sarangi SK, Maji B. Sustainable rice cultivation in coastal saline soils: a case study Institute, India. In: Achieving sustainable cultivation of rice, vol. 2. Burleigh Dodds Science Publishing (2017). p. 101–36. Available online at: https://www.taylorfrancis.com/chapters/edit/10.4324/9781351114196-7/sustainable-rice-cultivation-coastal-saline-soils-case-study-institute-India-sukanta-sarangi-buddheswar-maji-icar-central-soil-salinity-research (Accessed December 7, 2024).
81. Nguyen MT, Renaud FG, Sebesvari Z, Nguyen DC. Resilience of agricultural systems facing increased salinity intrusion in deltaic coastal areas of Vietnam. Ecol Soc. (2019) 24. doi: 10.5751/ES-11186-240419
82. Abdullah HM, Ahmed SM, Khan BM, Mohana NT, Ahamed T, Islam I. Agriculture and fisheries production in a regional blending and dynamic fresh and saline water systems in the coastal area of Bangladesh. Environ Challenges. (2021) 4:100089. doi: 10.1016/j.envc.2021.100089
83. Suarez DL, Jurinak JJ. The chemistry of the salt-affected soils and waters. In: Agricultural Salinity Assessment and Management. ASCE Manual and Reports on Engineering Practice. Washington DC, US: Agricultural Research Service of the U.S. Department of Agriculture, vol. 71. (2012). p. 57–88. Available online at: https://www.ars.usda.gov/research/publications/publication/?seqNo115=235690 (Accessed October 15, 2024).
84. Lian X, Piao S, Chen A, Huntingford C, Fu B, Li LZ, et al. Multifaceted characteristics of dryland aridity changes in a warming world. Nat Rev Earth Environ. (2021) 2:232–50. doi: 10.1038/s43017-021-00144-0
85. Naorem A, Jayaraman S, Dang YP, Dalal RC, Sinha NK, Rao CS, et al. Soil constraints in an arid environment—challenges, prospects, and implications. Agronomy. (2023) 13:220. doi: 10.3390/agronomy13010220
86. Barreto MB, Mónaco SL, Díaz R, Barreto-Pittol E, López L, Peralba MDCR. Soil organic carbon of mangrove forests (Rhizophora and Avicennia) of the Venezuelan Caribbean coast. Organic Geochem. (2016) 100:51–61. doi: 10.1016/j.orggeochem.2016.08.002
87. Barbiero L, Mohamedou AO, Roger L, Furian S, Aventurier A, Rémy JC, et al. The origin of Vertisols and their relationship to Acid Sulfate Soils in the Senegal valley. Catena. (2005) 59:93–116. doi: 10.1016/j.catena.2004.05.007
88. Sylla M. Soil salinity and acidity: spatial variability and effects on rice production in West Africa’s mangrove zone. Wageningen University and Research (1994). Available online at: https://search.proquest.com/openview/60878439edb4f51e8fdfc814ff6a2f39/1?pq-origsite=gscholar&cbl=18750&diss=y (Accessed November 4, 2024).
89. Balasubramanian V, Sie M, Hijmans RJ, Otsuka K. Increasing rice production in sub-Saharan Africa: challenges and opportunities. Adv Agron. (2007) 94:55–133. doi: 10.1016/S0065-2113(06)94002-4
90. Frenken K ed. Irrigation in Africa in figures: AQUASTAT surve Vol. 29. Food & Agriculture Org (2005). Available online at: https://openknowledge.fao.org/handle/20.500.14283/a0232e (Accessed October 13, 2024).
91. Dewitte O, Jones A, Spaargaren O, Breuning-Madsen H, Brossard M, Dampha A, et al. Harmonisation of the soil map of Africa at the continental scale. Geoderma. (2013) 211:138–53. doi: 10.1016/j.geoderma.2013.07.007
92. Gebremeskel G, Gebremicael TG, Kifle M, Meresa E, Gebremedhin T, Girmay A. Salinization pattern and its spatial distribution in the irrigated agriculture of Northern Ethiopia: An integrated approach of quantitative and spatial analysis. Agric Water Manage. (2018) 206:147–57. doi: 10.1016/j.agwat.2018.05.007
93. Zhang H, Fu X, Zhang Y, Qi Z, Zhang H, Xu Z. Mapping multi-depth soil salinity using remote sensing-enabled machine learning in the yellow river delta, China. Remote Sens. (2023) 15:5640. doi: 10.3390/rs15245640
94. Gorji T, Sertel E, Tanik A. Monitoring soil salinity via remote sensing technology under data scarce conditions: A case study from Turkey. Ecol Indic. (2017) 74:384–91. doi: 10.1016/j.ecolind.2016.11.043
95. Wang N, Xue J, Peng J, Biswas A, He Y, Shi Z. Integrating remote sensing and landscape characteristics to estimate soil salinity using machine learning methods: A case study from Southern Xinjiang, China. Remote Sens. (2020) 12:4118. doi: 10.3390/rs12244118
96. Wang F, Han L, Liu L, Bai C, Ao J, Hu H, et al. Advancements and perspective in the quantitative assessment of soil salinity utilizing remote sensing and machine learning algorithms: A review. Remote Sens. (2024) 16:4812. doi: 10.3390/rs16244812
97. Wang J, Peng J, Li H, Yin C, Liu W, Wang T, et al. Soil salinity mapping using machine learning algorithms with the Sentinel-2 MSI in arid areas, China. Remote Sens. (2021) 13:305. doi: 10.3390/rs13020305
98. Scudiero E, Corwin D, Anderson R, Yemoto K, Clary W, Wang Z, et al. Remote sensing is a viable tool for mapping soil salinity in agricultural lands. California Agric. (2017) 71(4):231–38. doi: 10.3733/ca.2017a0009
99. Abdelwahab MH. A review on reclamation and management practices of wind erosion and salt–affected soils of Sudan. International Journal of. Appl Sci Res. (2022) 5:89–103. doi: 10.56293/IJASR.2022.5436
100. Omar MM, Shitindi MJ, Massawe BJ, Fue KG, Pedersen O, Meliyo JL. Exploring farmers’ perception, knowledge, and management techniques of salt-affected soils to enhance rice production on small land holdings in Tanzania. Cogent Food Agric. (2022) 8:2140470. doi: 10.1080/23311932.2022.2140470
101. Herrmann J, Júnior MS, Luis A, Famba S. Participatory research for agronomic salinity management–experiences from coastal peri-urban vegetable production in Maputo, Mozambique, in: Piloting Strategies to Mitigate Impacts of Salinity in Horticultural Systems of Mozambique. Maputo, Mozambique: Universidade Eduardo Mondlane (2022).
102. Thiam S, Villamor GB, Faye LC, Sène JHB, Diwediga B, Kyei-Baffour N. Monitoring land use and soil salinity changes in coastal landscape: A case study from Senegal. Environ Monit Assess. (2021) 193:1–18. doi: 10.1007/s10661-021-08958-7
103. Connor JD, Ward J, Clifton C, Proctor W, MacDonald DH. Designing, testing and implementing a trial dryland salinity credit trade scheme. Ecol Econ. (2008) 67:574–88. doi: 10.1016/j.ecolecon.2008.01.020
104. Janmaat J. Calculating the cost of irrigation induced soil salinisation in the Tungabhadra project. Agric Econ. (2004) 31:81–96. doi: 10.1111/j.1574-0862.2004.tb00223.x
105. Houk E, Frasier M, Schuck E. The agricultural impacts of irrigation induced waterlogging and soil salinity in the Arkansas Basin. Agric Water Manage. (2006) 85:175–83. doi: 10.1016/j.agwat.2006.04.007
106. Hassan AK, Nangia V, Singh M. Soil salinity management in Iraq using indigenous soil management techniques—analysis of biophysical and socioeconomic data. ASA-CSSA-SSSA Annual International Meeting, November (2013). Available online at: https://scisoc.confex.com/scisoc/2013am/webprogramschedule/Paper81622.html (Accessed March 15, 2025).
107. Aslam M, Prathapar SA. Strategies to mitigate secondary salinization in the Indus Basin of Pakistan: a selective review. Colombo, Sri Lanka: International Water Management Institute (IWMI) (2006), 22p. (IWMI Research Report 097). Available online at: https://htps://hdl.handle.net/10568/39884.
108. Vyshpolsky F, Qadir M, Karimov A, Mukhamedjanov K, Bekbaev U, Paroda R, et al. Enhancing the productivity of high-magnesium soil and water resources in Central Asia through the application of phosphogypsum. Land Degrad Dev. (2008) 19:45–56. doi: 10.1002/ldr.814
109. Winpenny J, Heinz I, Koo-Oshima S. The wealth of waste: The economics of wastewater use in agriculture. Rome: Food and Agriculture Organization of the United Nations (2010).
110. Kushiev H, Noble AD, Abdullaev I, Toshbekov U. Remediation of abandoned saline soils using Glycyrrhiza glabra: A study from the Hungry Steppes of Central Asia. Int J Agric Sustain. (2005) 3:102–13. doi: 10.1080/14735903.2005.9684748
Keywords: sub-Saharan Africa, salt-affected soils, FAO, Solonchaks and Solonetz, arid and semi-arid, irrigation, coastal and delta basins, remote sensing
Citation: Mwesige FF (2025) The extent and distribution of salt-affected soils in sub-Saharan Africa from 1970 to the present: a review of the current state of knowledge. Front. Soil Sci. 5:1571243. doi: 10.3389/fsoil.2025.1571243
Received: 05 February 2025; Accepted: 04 April 2025;
Published: 07 May 2025.
Edited by:
Hassan Ragab El-Ramady, Kafrelsheikh University, EgyptReviewed by:
Azmera Walche Mengesha, Arba Minch University, EthiopiaMohammed Hagage, National Authority for Remote Sensing and Space Sciences, Egypt
Copyright © 2025 Mwesige. This is an open-access article distributed under the terms of the Creative Commons Attribution License (CC BY). The use, distribution or reproduction in other forums is permitted, provided the original author(s) and the copyright owner(s) are credited and that the original publication in this journal is cited, in accordance with accepted academic practice. No use, distribution or reproduction is permitted which does not comply with these terms.
*Correspondence: Finias Fidelis Mwesige, ZmluaWFzZmlkZWxpc0BnbWFpbC5jb20=