- 1Institute of Anatomy, University Medical Center of the Johannes Gutenberg-University Mainz, Mainz, Germany
- 2Focus Program Translational Neurosciences, University Medical Center of the Johannes Gutenberg-University, Mainz, Germany
- 3Institute of Anatomy, Brandenburg Medical School, Neuruppin, Germany
Background: The abducens nerve (AN), our sixth cranial nerve, is responsible for the innervation of the lateral rectus muscle of the eye. The abducens nerve is a vulnerable structure at the skull base with its long intracranial course and complex topographic relationships. The AN anatomy in the petroclival region, where the nerve passes from the posterior to the middle cranial fossa, is of great interest for neurosurgical procedures. Despite detailed studies of its anatomy from the past 150 years, there is a need for more recent data on macroscopical and microscopical aspects of the AN in well defined populations.
Methods: We investigated macroscopical variations and the number of nerve fibers of the AN in the petroclival region in German body donors.
Results: In our histological samples (n = 24) we counted 4688 (+/−1,041) nerve fibers per AN. There was no correlation between sex, age and body side regarding the number of nerve fibers. In our macroscopic examination (n = 76 skull base sides), we found six duplications (four left-sided, two right-sided; 7.9%) and one triplication (right-sided; 1.3%) of the AN in the petroclival region. The AN triplication was further examined: Three nerve bundles pierce the dura mater separately and united before passing under the petrosphenoidal ligament (of Gruber).
Conclusion: Variations of the AN in the petroclival region are not a rare phenomenon but occur very frequently. Consequently, we have developed a new classification system for AN variations. This knowledge might help neurosurgeons, as it prepares them to be aware of such variations and adapt their surgical approaches accordingly.
1 Introduction
Abducens nerve (AN) palsy is a disease of the sixth cranial nerve that causes the inability to contract the lateral rectus muscle (LRM) due to insufficient innervation. Consequently, the other extraocular muscles become more dominant, resulting in a lack of gaze control. Patients report diplopia and often compensate by rotating their heads to the affected side (1).
AN palsy is the most prevalent cranial nerve palsy in neurological emergency rooms with incidences between 4.66 and 11.3/100.000 inhabitants (2, 3). The dissection of the internal carotid artery in the cavernous sinus is the most common pathology leading to AN palsy due to the artery's close topographic relationship to the AN. Additionally, skull base fractures involving trauma settings, in particular the petrosal bone fractures (both longitudinal and transverse), can affect the AN in its course which then contributes to neurologic deficits (4, 5). Furthermore, systemic diseases, such as chronic hyperglycemia and chronic, degenerative neurological diseases like multiple sclerosis, have been shown to correlate to the development of AN palsy (3). Petroclival meningiomas and other tumors of the skull base, such as the facial schwannoma can infiltrate the structural integrity of the AN and its course. The AN and its surrounding structures, like the posterior clinoid process, are important landmarks for surgical orientation (6). For example, the AN's dural entrance at the clivus is one of the landmarks for the inferomedial paraclival triangle, which serves as a roadmap for surgical interventions at the skull base (7). The trans-petrosal approach of petroclival tumors (meningiomas or facial schwannomas) holds the risk of cranial nerve affection in this region (8). The AN is particularly vulnerable during those procedures because of its exposed position in both the posterior and middle cranial fossa and shows statistically significant postoperative complications (9). In order to perform these surgical interventions safely, it is crucial to know about the macroscopic anatomy and its topographic relations and possible variations, in particular the localizations of aberrant nerve branches, very well. The differences in the prevalence of occurrence among different ethnicities should additionally be considered.
In its ordinary course, the AN exits the brainstem at the pontomedullary junction and travels in its subarachnoid course through the prepontine cistern, piercing the dura at the clivus region. From the dural porus, meningeal layers accompany the nerve and form a pocket filled with cerebrospinal fluid around the nerve (10–12). The petroclival region is of particular interest regarding the AN vulnerability as the meningeal sheet of the AN is attached to the periosteal dura (13) and the moveability of the AN is restricted by fixations at the dural entrance (14) and the petrosphenoidal ligament (15). The nerve is running between the clival (inner) and periosteal (outer) dural layer in a meningeal sheet. These two layers are separated by the so-called petroclival venous confluence by Destrieux et al. (13). Ozveren et al. (16) revealed that the dural layer extends after the porus, following the nerve as it travels cranially up to the Dorello's canal. The arachnoid layer also extends in a similar manner. Joo et al. (17) found similar dural relations regarding the AN with the dural sleeve ending at the LRM.The AN usually travels below the petrosphenoidal ligament of Gruber in Dorello's canal (18, 19) and continues its course through the cavernous sinus before entering the orbit. The petrosphenoidal ligament of Gruber can be partly ossified, the so-called Foramen sphenopetrosum osseum anomalum (20).
Given the lack of recent studies on AN anatomy in a German population (both in terms of macroscopical variations and microscopical aspects), we investigated the number of AN fibers and its dural entrance. This information could be interesting for future surgical procedures at the skull base.
2 Materials and methods
This study was performed on adult human skull bases from 12 formalin-fixed body donors from the dissection course (five males and seven females) and 52 skull base specimens (26 right and 26 left sides) from the anatomical collection of the Institute of Anatomy, University Medical Center of the Johannes Gutenberg-University Mainz. The age of the 12 donors ranged between 65 and 95 years (mean age: 83.36). Skull base specimens were of unknown sex, age, and medical history. All body donors donated their bodies willingly for research and education. This study was approved by the ethical committee of Rhineland-Palatinate (2023-17245).
2.1 Histological examination
Semithin sectioning was used for the histological analysis. The tissue samples from the subarachnoid part of the abducens nerve were taken after opening the skull and removing the brain. All samples were taken from the last 0.5 cm of the AN before the dural porus. After fixation in glutaraldehyde, samples were placed in a phosphate buffer (3 × 15 min). Fixation was continued with 2% osmium tetroxide for two hours, followed by phosphate buffer. Dehydration was carried out with an ethanol series in ascending ethanol concentrations (50%–70%–90%–100%). The samples were then treated with a mixture of propylene oxide (PO) and epoxide resin (EPON). The propylene oxide was used as a transition solvent in this process. After three PO/EPON mixtures (1 h each) with an ascending portion of the epoxide resin, samples were placed in pure epoxy resin overnight. After pouring, polymerization followed at 65°C in an incubator for 2 days. Using the Metvacut E microtome (Reichert-Jung, Germany), the blocks were cut into 1 µm thick slices. Slices were stained with 1% azure II and 1% methylene blue (21). After the staining process was finished, samples were mounted using Cytoseal xyl.
The histological sections were imaged with a Leica MS 5 tripod (Leica Microsystems, Germany) and a JVC KY-F75U C mount digital camera (JVC, Yokohama, Japan) and digitally processed with the histology software Diskus (Version 4.807713).
2.2 Analyzed area
Using the Diskus software described above, an area of 225 × 175 µm2 was randomly selected over which a grid was placed. The grid consisted of 9 × 7 square boxes of 25 × 25 µm2 each. The total area of the mapped nerve cross-section was calculated using the software by outlining the nerve cross-section. A total of three different histological sections were evaluated for each nerve. An average value for the number of fibers per grid area and its total area was calculated from the three values per nerve. In addition, the simple standard deviation was determined in each case.
2.3 Counting method and calculations
The number of nerve fibers was counted manually using a mechanical hand counter. The “Forbidden Rule of Gunderson” method was used for manual counting. Nerve fibers on the square's left and bottom lines were not counted. However, those on an upper or right line are included (22). The number of nerve fibers of a grid box (225 × 175 µm2) was extrapolated to the previously determined total area of the nerve. Our calculations neglected the tissue shrinkage due to histological processing, as previous studies showed no significant difference when this factor was taken into account (23).
2.4 Statistical analysis
The data acquired by the study were expressed as mean values with standard deviation (+/−SD). T-test was used as a statistical tool. A p-value of <0.05 was considered to be statistically significant.
2.5 Macroscopical examination
52 skull base sides (26 right, 26 left) were examined macroscopically. The course of the AN was assessed by further anatomical dissection. The dural layer was carefully removed, so the course of the AN could be seen before passing under Gruber's ligament. Further investigation of its course in the cavernous sinus was done until the nerve passed the superior orbital fissure. The long storage time of the samples examined did not allow further histological analysis.
3 Results
We focused our investigations on the number of nerve fibers, a comparison between left and right side and macroscopic variations of the AN in the petroclival region, where the AN pierces the dura and switches from the subarachnoid part to the gulfar or petroclival segment in its further course (14).
3.1 Light microscopic observations
We analyzed the number of fibers per nerve in the petroclival region to see if there are differences in number of nerve fibers between the left and right side, taking into account the role of sex and age.
Most of the cross-sections appeared to be oval or irregular shaped with small bundles held together by thin connective tissue layers. The nerve fibers appeared as empty spaces bordered by a ring representing the myelin sheath.
3.1.1 Quantitative observations
The details of the cross-section area of the nerves, as well as general data about our donors, are described in Table 1.
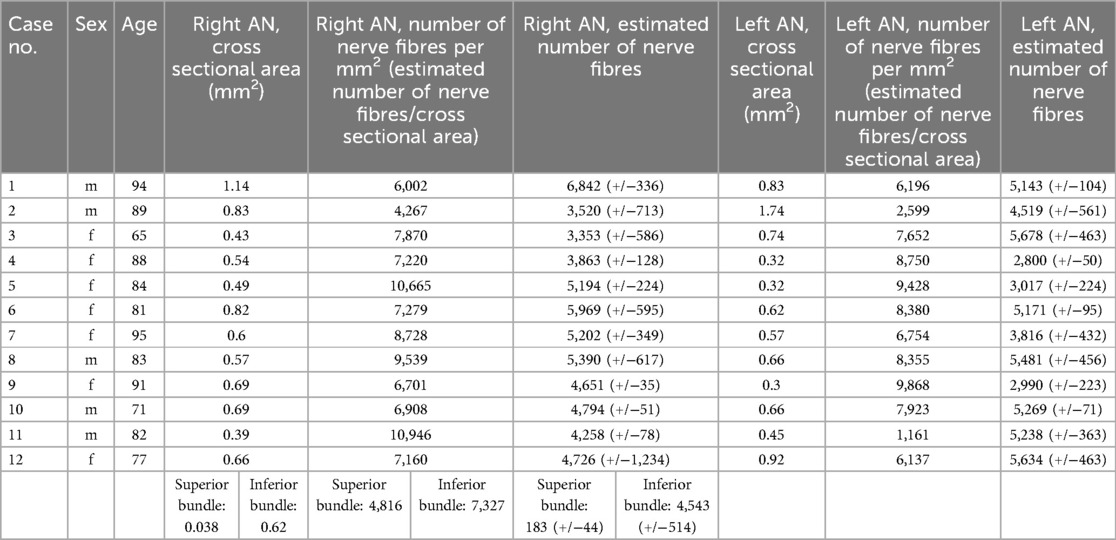
Table 1. Number of AN fibres (mean value of three regions and standard deviation) on the right and left side. The superior and inferior bundle of the duplication were handled as one nerve in our statistical analysis.
We did count a mean of 4,688 (+/−1,041) nerve fibers per nerve. On average, we found 4,563 (+/−1,547) in the left and 4,814 (+/−1,778) in the right AN. On the left side, the numbers ranged between 2,800 and 5,876, and on the right side, from 3,353 to 6,842 (Figure 1). There is no lateralization in the number of nerve fibers (p = 0.27).
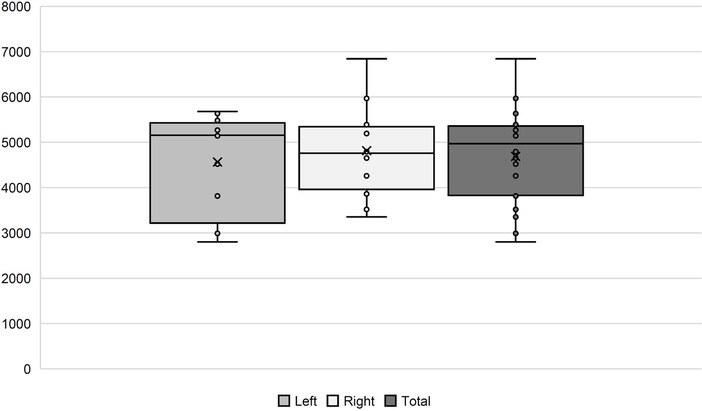
Figure 1. Number of AN fibers in our histological samples. In the boxplots, comparison between the left and right side, as well as the total amount, is shown.
The estimated mean of the male donors was 5,045 (+/−877) nerve fibers, while the female donors showed 4,433 (+/−1,103). There was no significant correlation between number of nerve fibers and sex or age.
3.1.2 Histological analysis of the duplication
We found a macroscopical duplication of the right AN in a 77-year-old female body donor (Table 1: no. 12). We further examined this duplication histologically (Figure 2). There was one main AN branch (inferior bundle) with a cross-sectional area of 0.62 mm2 and an accessory branch (superior bundle) with a cross-sectional area of 0.038 mm2.
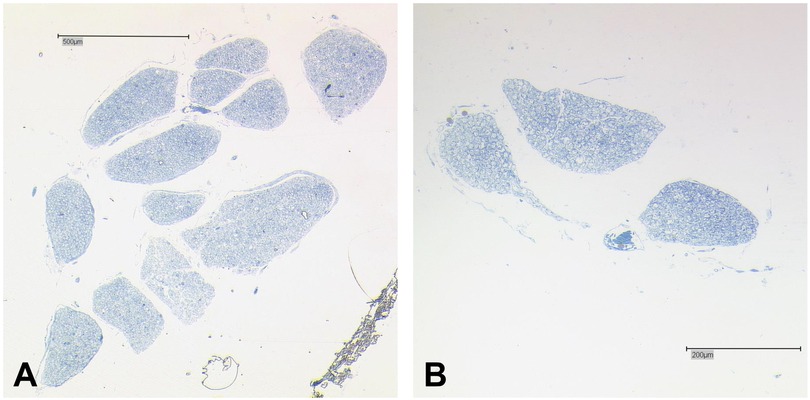
Figure 2. Histology of the duplicated AN. (A) Main fiber bundle (inferior bundle) of the AN. The main trunk is organized in twelve bundles that are separated by thin layers of connective tissue. (B) Accessory AN branch organized in three small bundles. Bar = 500 μm.
In total, both bundles combined contained an estimated number of 4,726 fibers with the inferior bundle contributing 4,543, and the superior bundle 183 fibers.
3.2 Macroscopic examination
Seven of the 76 skull base sides showed variations in the petroclival region regarding the AN. Figure 3 shows the percentages of duplications and triplications we found.
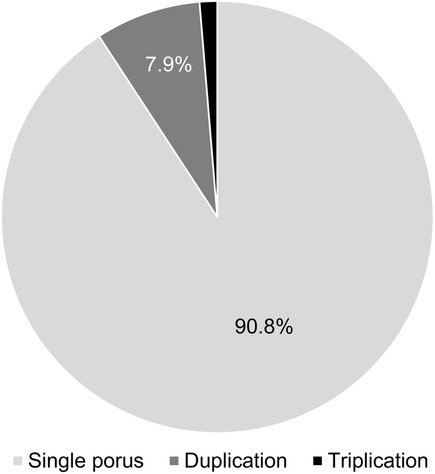
Figure 3. Number of AN dural pori. In most cases (90.8%), only one dural porus was present. Dural porus duplication was observed in 7.9%, and dural porus triplication in 1.3%.
In most cases, only one AN and one single dural porus were observed (90.8%). There were six duplications (four left, two right-sided) (7.9%) and one triplication (right-sided) (1.3%), each with separate dural entrances. The dural pori were, in most cases, slot-shaped, but we also found round pori. Figure 4 shows two examples of our macroscopical findings.
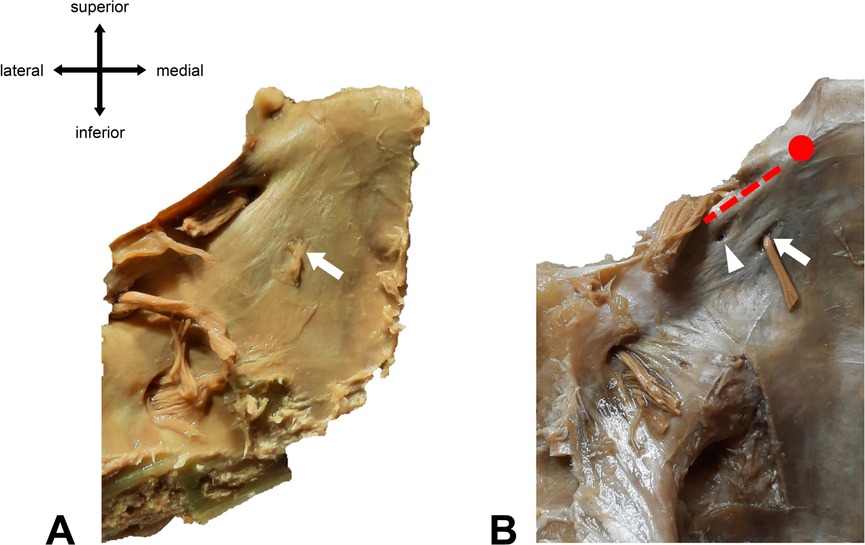
Figure 4. Different types of abducens dural entrances. (A) Single left dural porus (white arrow) on the clivus at its textbook position (lateral to the midline of the clivus). (B) Duplicated left dural porus. The main dural entrance is marked with a white arrow. The white arrowhead points to the accessory porus, which is located superiorly and laterally to the main porus. Red dotted line = location of Gruber’s ligament. Red dot = location of the posterior clinoid process.
Most of the accessory branches are located superiorly and laterally to the dural entrance of the main trunk. The average distance between the main and the accessory porus was 2.5 mm (+/−1 mm).
3.3 Triplication of the AN
One case of an AN triplication was found at a right skull base side. After carefully removing the dural layer, we could see that all three branches connected closely after piercing the dura mater (Figure 5). The petrosphenoidal ligament (of Gruber) was partly ossified in this case (incomplete Foramen petrosphenoideum osseum anomalum).
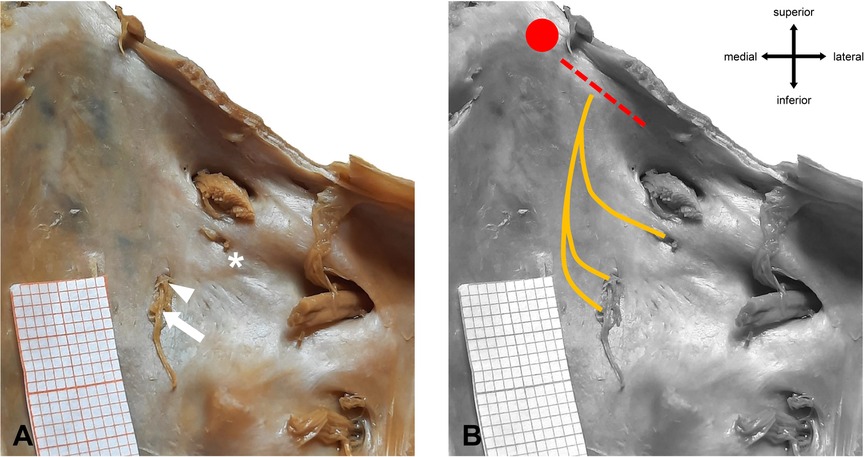
Figure 5. Macroscopic anatomy of AN trifurcation. (A) Right side of the clivus with main dural porus (arrow) and two accessory dural pori (arrowhead, asterisk). (B) Subdural course of the AN fibers. All three fiber bundles merge before entering Dorello’s canal. Red dotted line = location of Gruber’s ligament. Red dot = location of the posterior clinoid process.
4 Discussion
The AN in humans has been investigated in multiple previous studies during the last two centuries (24). Here, we discuss our findings focusing on the following topics:
I. Amount of AN fibers
II. L-R-Asymmetry and sex related differences of the AN
III. Macroscopic variations of the AN
IV. Suggestion for a new branching scheme
4.1 Different amounts of AN fibers in the literature
We noticed a broad discrepancy in the number of nerve fibers for the AN in literature. The mean estimated number of nerve fibers ranged between 1,997 (25) and 7,220 (26) per AN. The first study that investigated this topic in humans was published in 1845 by Rosenthal. He found an estimated mean of 2,000–2,500 fibers per AN in one human. Almost 40 years later, Bors counted 4,698 fibers (27) in an AN of an adult man.
While several studies in the following years were performed (28, 29), all of them had very limited sample sizes. Interestingly, the numbers of nerve fibers steadily increased. Harley was the first to perform a bigger study regarding the sample size with twenty-four donors (30). Harley investigated the left-right-asymmetry in the amount of nerve fibers and found no significant difference (30). This was confirmed by later studies (31).
Table 2 shows the studies that were previously done on this topic, along with the estimated number of nerve fibers.
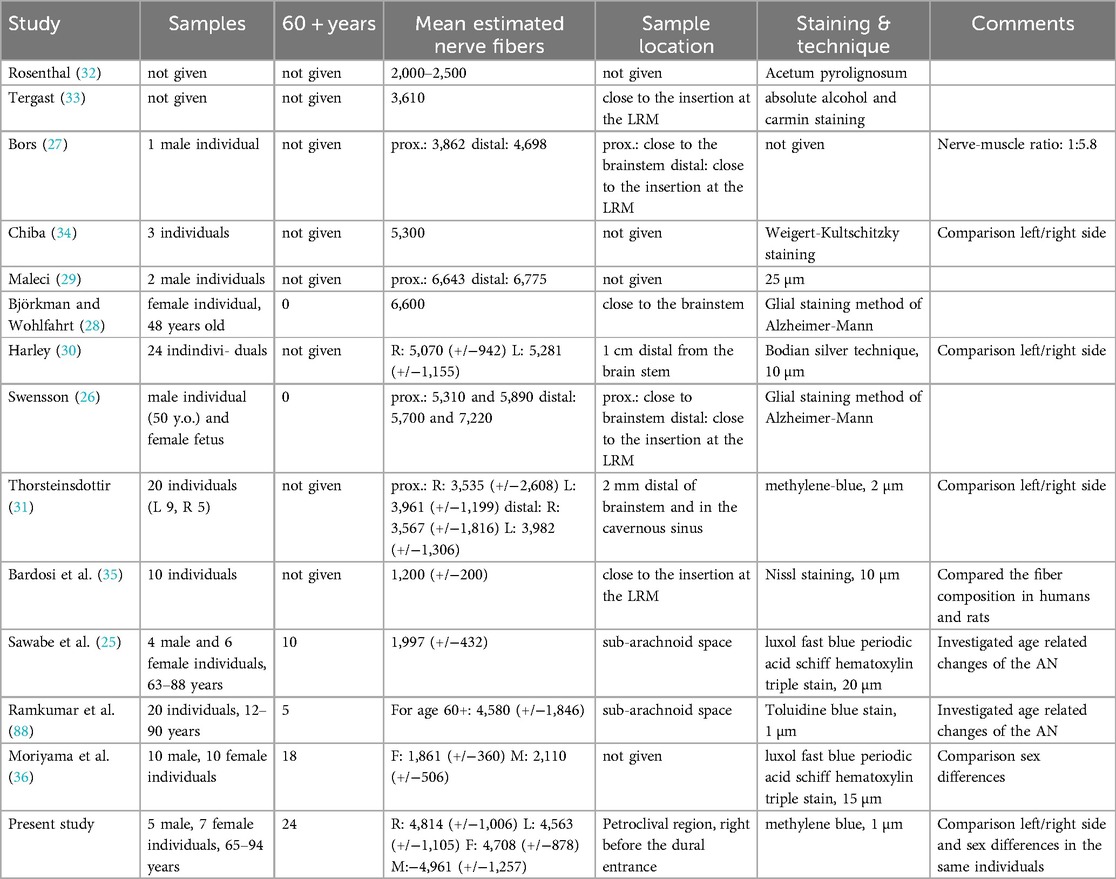
Table 2. Comparison of different histological studies on the AN. M stands for male; F for female while R stands for right side and L for left side. Data that was not available was indicated by “not given”.
4.1.1 Impact of sample location
Generally, nerve fibers increase from proximal to distal sample locations. Bors counted 3,862 in the proximal part of the AN and 4,698 in a more distal location (27). While his study only included one male donor of unknown age and ethnicity, this trend was confirmed by other studies with larger sample sizes and also for both sides of the individual (30). It is generally known that the myelinated nerve fibers branch into multiple fibers when traveling from the brainstem to the orbit (26). Sunderland and Hughes added that with its course through the cavernous sinus, the AN is joined mainly by sympathetic bundles of the internal carotid plexus (37). This phenomenon was confirmed with the use of Sihler's stain in a study done by Wysiadecki et al. (7). Some authors suggest that the sample location can be too close to the brainstem; in this case the amount of myelinated nerve fibers is underestimated (26).
4.1.2 Impact of staining technique and slice thickness
Thorsteinsdottir (31) explained the variability of the amount of nerve fibers in literature with different staining techniques. She used methylene blue staining in her work and argued that she might not detect nerve fibers under 2–3 µm in diameter with her methods. According to Tomasch and Schwarzacher (38) most fibers fall in the 2–3 µm category. Furthermore, Agdhur (39) stated that low magnification combined with intense staining could lead to a too-low calculation of nerve fibers and that the differentiation between small nerve fibers and glial cells in the central nervous system is complicated, which could have an influence on why the number counted in samples taken close to the brain stem might be hard to assess.
4.1.3 Impact of ethnicity
Studies of Japanese origin reveal a significantly lower number of AN fibers compared to other populations. Sawabe et al. (25) performed a study with Japanese body donors and reported a mean of 1,997 nerve fibers (10 donors). Moriyama et al. (36) found similar numbers in the Japanese population (for females: 1,861 +/−360, for males: 2,110 +/−506). They used samples from 10 male and 10 female body donors with an age of 54–90 years.
4.2 Left-right-asymmetry of the AN
In this study we did not find a L-R-Asymmetry of the number of AN fibers.
Studies of asymmetries in other cranial nerves have been published in the past. The morphometry of the recurrent laryngeal nerve differs in humans and rats, with the right nerve generally having thicker fibers (40). Nevertheless, other studies on human cranial nerves showed that in most cases there is no significant lateralization (41). Regarding the abducens nerve, two previous studies compared numbers of left and right nerve fibers (30, 31). Both could not find significant differences in fiber number, but according to Thorsteinsdottir (31), the left AN has more fibers with a diameter of >5 µm measured in the prepontine cistern and the cavernous sinus.
4.2.1 Sex-related differences in AN nerve fibers
We could not show sex-related differences in the number of AN fibers. Moriyama et al. (36) investigated the association between sex and the number of nerve fibers in the AN. They found that there is no sex-related difference in the AN but only used the right AN of the donors (36). In contrast, the vestibulocochlear nerve is sexually dimorphic: female body donors tend to have fewer nerve fibers than male donors (36, 42).
4.3 Macroscopic variations of the AN
The following section provides an overview of established theories, as well as recent findings about the branching of the AN.
4.3.1 Literature overview
The prevalence of AN supernumerary variations ranges from 0% to 80% (43, 44) (Table 3).
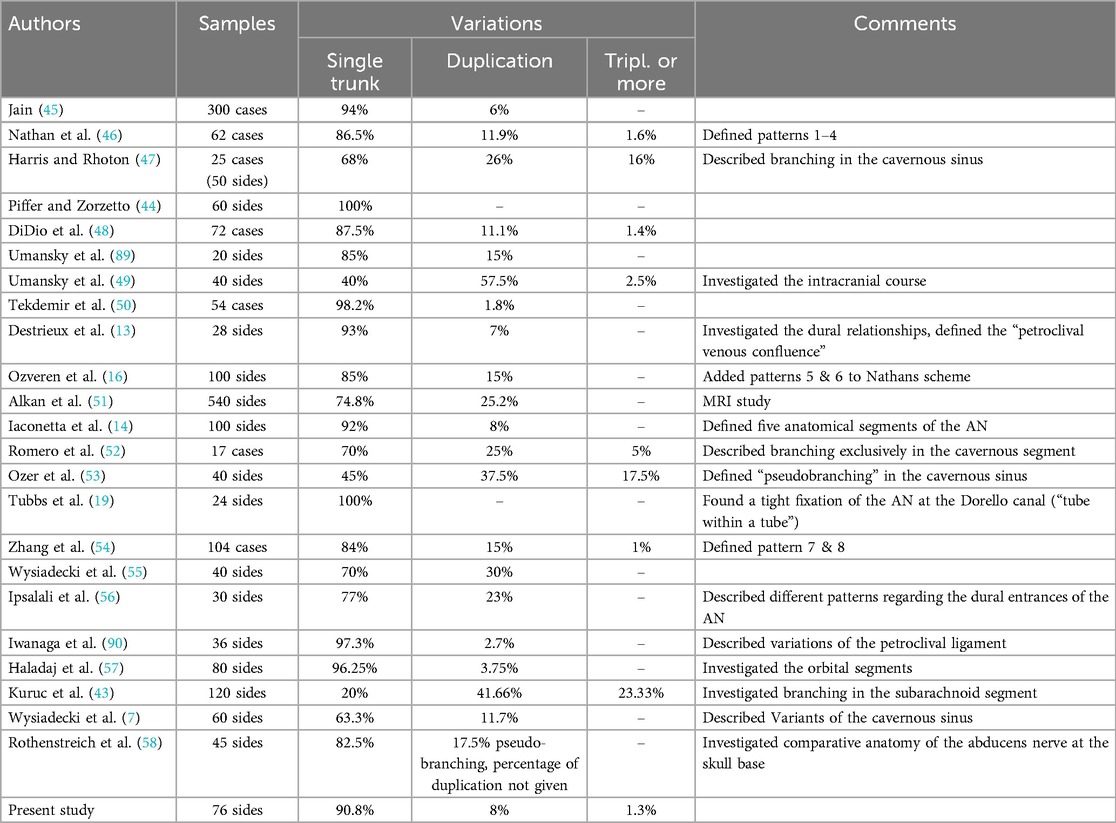
Table 3. Comparison of studies on macroscopic AN variations regarding duplications and triplications.
Other literature reviews found the percentage of duplication for the AN around 7.6% (24) and 5%–28.6% (59). Kuruc et al. (43) described the prevalence of AN variations in the prepontine cistern to be up to 80% in an Eastern European population, which is much higher than any study published before (43).
4.3.2 Embryological development of the AN and variations in development
The somatomotor AN develops early in the hindbrain (rhombomeres r5 and r6). Transcription factors of the myogenic progenitor cells stimulate the extension of the axons out of their respective columns (60). Gilbert stated that the target of the AN, the lateral rectus muscle, originates from two myotomes (61). Different abducens nerve strands could be a result of multiple AN branches that did not fuse together correctly. This theory was brought up first by Bremer, who stated that supernumerary branches of the AN could be the result of failure to resorb aberrant nerve fibers during development (62). Wahl et al. (63) postulated that the innervation of different somitomeres in chick embryos is facilitated by multiple AN branches.
Sato et al. described a change in the AN course during development and proposed that many anatomical structures can alter or modify the course of the AN at various time points of development (64). The developing veins in the parasellar region, around the hypophyseal gland, can alter the course of the AN through the cavernous sinus and separate the oculomotor nerve from the AN. It is shown, however, that the AN has close relations to the ophthalmic nerve, the first branch of the trigeminal nerve. These two nerves are usually separated by an arterial branch of the inferolateral trunk of the internal carotid artery (64). Furthermore, there are case reports about a persistent trigeminal artery (PTA) (65). This artery usually regresses after the manifestation of the posterior communicating artery. In those individuals where it persists or even during development, the PTA has a close relation to the dural entrance of the AN at the clivus and might be able to alter the anatomy of the AN (65). These relations were already described in previous studies as possible cause for a loop-alteration of the AN course (66, 67).
4.3.3 The anterior inferior cerebellar artery
The AN has a close relation to the anterior inferior cerebellar artery (AICA). AN palsy might be associated with compression by the AICA (68) but the close relation could also explain branching of the AN. Descriptions of two abducens roots separated by the AICA which was running between them (14, 45, 49, 69) are found in literature. In a case report, Borg et al. described a 76-year-old woman with an aberrant course of the AN due to compression by an ectatic AICA (70). Additionally, a penetration of the AN by the AICA has been reported (67). In most cases the AICA passes under the AN, so called basal course (67, 71).
4.3.4 Interactions in the cavernous sinus
Sunderland and Hughes (37) found that the AN, after entering the cavernous sinus, splits into multiple bundles, which are separated “through thick dural septa from the fibrous dural prolongation that accompany the AN in its course through the sinus.” Other studies showed similar arrangements with the AN branching in the cavernous sinus close to the posterior aspect of the ICA (10, 47). Ozer first introduced the term pseudobranching for this phenomenon (53). The prevalance of pseudobranching ranges from 17.5% to 37.5% (55, 58). Pseudobranching was also analyzed histologically (7).
4.3.5 The lateral rectus muscle (LRM)
Haladaj found that the lateral rectus muscle has a “dual-headed origin” (72). Nam showed that in most cases a single trunk of the AN branches into two nerves right before the LRM (73). Peng et al. studied the LRM's innervation in primates and humans and could show that the LRM in both species has a compartmentalized innervation with a superior and an inferior zone (74). Branching of the AN might be related to functional units of the RLM (48).
4.3.6 External oculomotor muscles in comparative anatomy
The function of the retractor bulbi muscle (RBM) is to pull the eye further back into the orbit, in response to corneal/trigeminal stimulation. The RBM and the LRM originate from the same blastema. In birds this muscle is divided into two separate portions, the quadratus and pyramidalis muscles (75). Lateral parts of the RBM are innervated by the AN. In most species there are two different cell populations of the AN nucleus found: the dorsal (main or principal) nucleus and the ventral (accessory) nucleus. In frogs (Rana ridibunda) AN fibers that innervate this muscle originate from both the main and accessory abducens nucleus (76). In cats, the sub-nucleus of the AN nucleus innervates the RBM (77). In monkeys the equivalent of the RBM is called the accessory lateral rectus muscle (ALR). Spencer and Porter (78) could show that in some cases both the LRM and the ALR are innervated by the principal abducens nucleus. The ventral abducens nucleus has closer relations to the sensory neurons with trigeminal input, in correlation with the RBM-reflex. Even though this sub-nucleus is not found in humans, these conditions may explain the possibility of two roots of the AN.
4.4 Relations to and differentiation from the trigeminal nerve (TN)
Ogut et al. performed a study on 19 formalin-fixed heads and found different shapes of the TN porus (elliptical, oval, and slit-like). They found no variations in the subarachnoid portion of the TN. The estimated distance between the AN and the trigeminal dural entrance was found to be 5.7–9.03 mm for the right and 4.64–8.81 mm for the left side (79). Another study in the petroclival region also showed no branching variations of the TN (80). Arslan stated that the mean estimate distance between the trigeminal ganglion and the AN is 1.87 mm (81).
In a case report, Dupont et al. showed a duplicated AN with a dural porus situated at a similar position like the topmost dural porus in our triplication (82). In this particular case, the ipsilateral roof of the trigeminal porus was ossified. This ossification may be interpreted as a trigeminal bridge, as it has been described by Wegner (83) and Lang (84).
4.5 Triplications of the AN are a rare phenomenon
DiDio et al. described a triplication in a male (48). In this case, the AN pierced the dura as a single strand, then three nerve branches emerged in the petroclival segment and rejoined before entering the cavernous sinus. Nathan et al. published a case with an AN branching into three trunks in the subarachnoid region, piercing the dura at the clivus separately before rejoining in the cavernous sinus (46). A similar branching was observed by Zhang et al. (54), but all nerve strands passed below the petrosphenoid ligament of Gruber. In our case, the three branches emerging in the subarachnoid space were piercing the dura separately, rejoined to one single strand before passing Dorello's canal. Kuruc et al. described intradural triplications in the prepontine cistern (subarachnoid segment) without investigating their further course (43). Branching in the cavernous sinus are a form of the aforementioned pseudobranching but can also be seen as triplications. Figure 6 illustrates the different types of triplications.
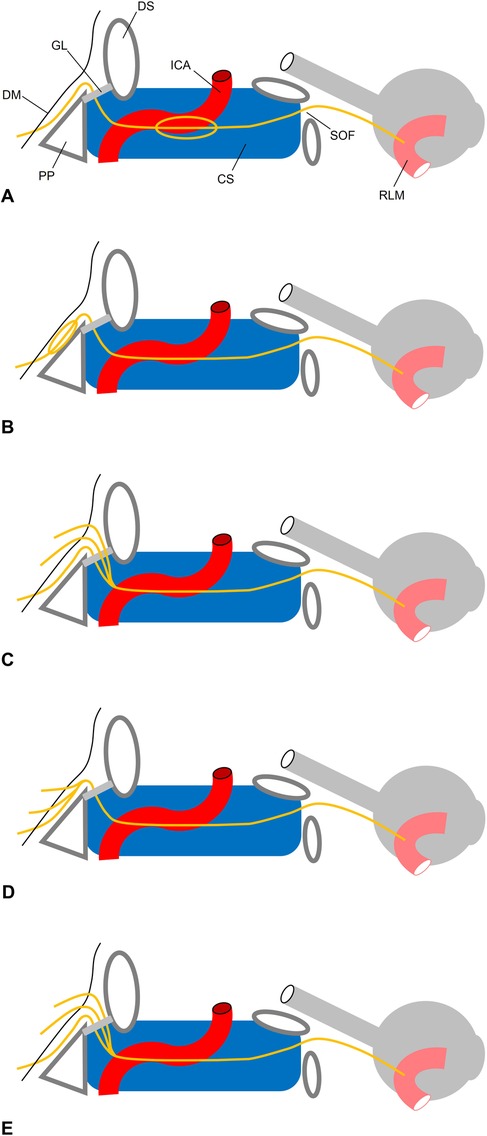
Figure 6. Topography of AN triplication. (A) Pseudobranching in the cavernous sinus (CS). (B) Pseudobranching under the clival dura mater (DM) as described by (48). The AN pierces the DM through a single porus (C) trifurcation as described by (46). Three branches pierce the DM through three dural pori. While the most inferior fiber bunde lies below Gruber’s (GL), the two other bundle lie above the GL. All three bundles merge in the CS. (D) Present case. Three branches pierce the DM through three dural pori. All three fiber bundles merge before entering Dorello’s canal. (E) Trifurcation as described by (54). Three branches pierce the DM through three dural pori. All fibers lie below GL and merge within the CS at the posterior aspect of the internal carotid artery (ACI). PP, petrous pyramid; DS, dorsum sellae; SOF, superior orbital fissure; RLM, rectus lateral muscle.
4.6 Suggestion for a new classification system
In literature there are various approaches to categorize variations of the AN. The most prominent ones are the morphological categorization by Nathan (46) and later Ovzeren (16) in six distinct branching patterns, and the approach of branching in specific segments by Wysiadecki (55) and Ozer (53). Nathan et al. published the first branching scheme in 1976. They described four different patterns of the AN (Pattern 1). Ozveren et al. added Patterns 5 and 6 to this scheme. Those patterns are based primarily on cases by Tillack and Winer (85) (Pattern 5), Nathan et al. (46) and Jain (45). Zhang added patterns VII (duplication with piercing the dura separately with multiple branching in the cavernous sinus and merging as one trunk before entering the orbit) and VIII (duplication as a loop exclusively in the cavernous segment). Wysiadecki et al. published another scheme regarding the cavernous segment: Type I is a single nerve strand. Type II and III are both duplications of the AN, with type II being reserved for duplications only in the cavernous part of the nerve's course (55).
Iaconetta et al. proposed a five-segment classification for the AN: cisternal, gulfar, cavernous, fissural and intraconal (14). Other authors specified the petroclival region as the AN segment starting at the dural entrance and ending in the Dorello's canal (53, 56). Studies show that this region is of considerable interest when investigating variations (53). More recently, Ipsilali published a variation scheme that investigated the petroclival region (Ipsilali et al., 2019) and focused on the dural entry pores but did not further investigate the AN course.
To describe the branching in a systematic way we suggest using four different main categories A-D (Figure 7). Each category stands for a different branching pattern: Category A is a loop shaped branching, starting as a single trunk branching into two or more roots before merging to one trunk again. This variation can be mostly seen in the petroclival segment of the AN (12, 46, 48–50, 53–55, 69). Category B are two or more roots exiting the brainstem merging to one nerve strand during their course at the skull base. Most of the merging is observed at the petroclival segment and at the start of the cavernous segment (49). Category C is a Y-shaped pattern with the AN starting as a single trunk branching into two or more roots innervating the LRM separately. These variations are observed in the orbital segments and the cavernous segments and can be seen as early branching (7, 53, 57). Category D shows reports of two trunks of the AN travelling from the brain stem to the orbit separately. This variation was only described by Jain and Testut (45, 86). Wysiadecki et al. published a somewhat similar case with the difference of the two trunks merging in the orbit before rebranching again (87).
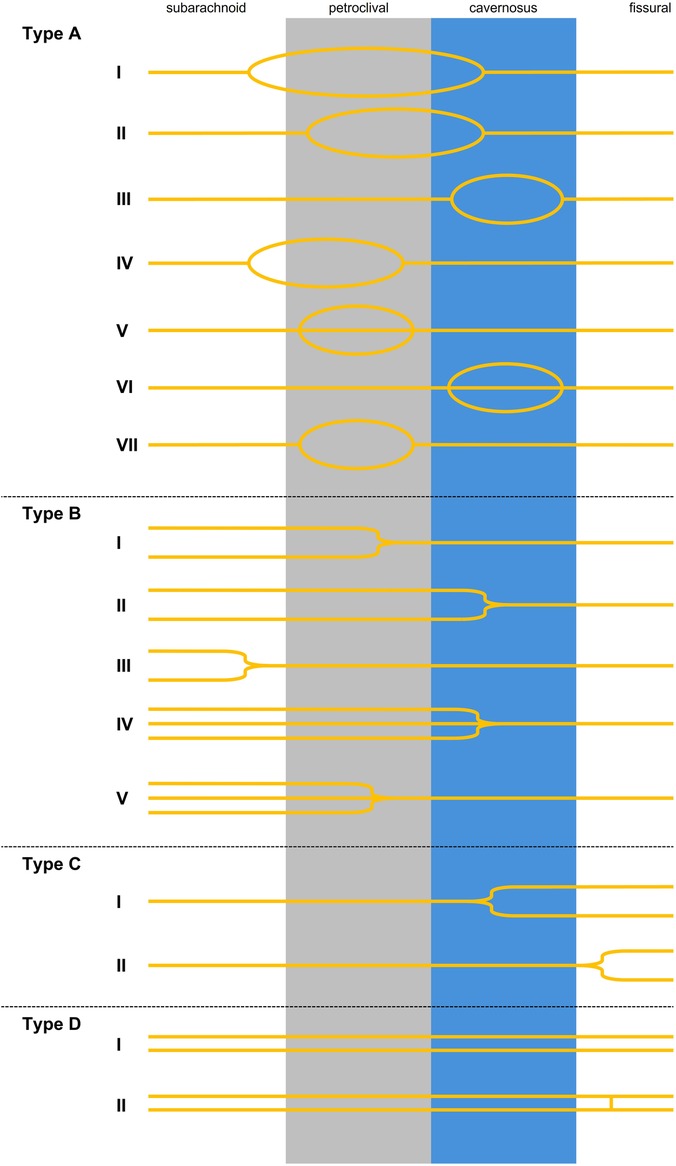
Figure 7. Categories of the AN branching: A = loop shaped AN. AI: loop starts in the subarachnoid segment and the branches merge in the cavernous sinus (12, 46, 50, 54, 55, 69, 91). AII: looping starts in the petroclival segment and merging in the cavernous sinus (92). AIII: looping exclusively in the cavernous sinus (17, 49, 53–55). AIV: looping starts in the subarachnoid region and merging in the petroclival segment (55). AV: Tripliation exclusively in the petroclival region DiDio et al. (48). AVI: pseudobranching into three or more rootlets exclusively in the cavernous segment (17, 47, 52, 53, 55). AVII: looping exclusively in the petroclival region (49). Type B: multiple trunks merging. BI: two trunks merging in the petroclival segment (49). BII: two trunks merging in the cavernous sinus (7, 12, 45, 46, 49, 53–55, 69), BIII: two trunks merging in the prepontine cistern (49, 54). BIV: three trunks merging in the cavernous sinus (46, 54). BV: three trunks merging in the petroclival segment (present study). C = one strand splitting into multiple trunks. CI: one strand splitting into two trunks in the cavernous sinus (7, 53). CII: one strand splitting into two trunks in the fissural segment (57). D = two separate trunks travelling from the brainstem to the orbit. DI: two nerve strands travelling from brainstem to orbit (45, 86). DII: Variation in which the two branches travel separately and have a short connection at the fissural segment (87).
Our branching scheme shows that the most variability of the AN is displayed in the petroclival region, mostly in the morphological form of a loop shape (Category A) or multiple roots merging to a single trunk (Category B).
4.7 Limitations of this study
As our study was limited to already formalin-fixated body donors who underwent the fixation process in some cases multiple years ago, histological analysis was not possible in all cases. Especially, we were not able to perform immunohistochemical analysis on our material.
4.8 Future investigations
In this study, we focused on the petroclival region, because of its importance in skull base surgery. In the future, it could be interesting to study the AN and its variations in other anatomical areas. Furthermore, it would be advantageous to analyze the branching of the AN with immunohistochemical methods that allow to differentiate fiber qualities. Additionally, systematic analysis of different populations would be advantageous.
5 Conclusion
This study aims to provide recent morphometric data for the abducens nerve in body donors of the University of Mainz, Germany. With over 8% of macroscopic variations in the petroclival region, our results might be relevant for neurosurgery in this location.
Data availability statement
The original contributions presented in the study are included in the article/Supplementary Material, further inquiries can be directed to the corresponding author.
Ethics statement
The studies involving humans were approved by Ethical committee of Rhineland-Palatinate. The studies were conducted in accordance with the local legislation and institutional requirements. The participants provided their written informed consent to participate in this study.
Author contributions
MB: Formal analysis, Investigation, Visualization, Writing – original draft, Writing – review & editing. MS: Funding acquisition, Resources, Writing – original draft, Writing – review & editing. SS: Conceptualization, Methodology, Project administration, Supervision, Validation, Visualization, Writing – original draft, Writing – review & editing.
Funding
The author(s) declare that no financial support was received for the research and/or publication of this article.
Acknowledgments
The authors thank Kerstin Bahr for excellent technical assistance. We thank Prof. Andreas Winkelmann, Neuruppin, and Dr. Michael Wolf-Vollenbröker, Düsseldorf, for proofreading the manuscript. We sincerely thank those who donated their bodies to science so that anatomical research could be performed. Results from such research can potentially increase mankind's overall knowledge that can then improve patient care. Therefore, these donors and their families deserve our highest gratitude.
Conflict of interest
The author(s) declared that they were an editorial board member of Frontiers, at the time of submission. This had no impact on the peer review process and the final decision.
The authors declare that the research was conducted in the absence of any commercial or financial relationships that could be construed as a potential conflict of interest.
Generative AI statement
The author(s) declare that no Generative AI was used in the creation of this manuscript.
Publisher's note
All claims expressed in this article are solely those of the authors and do not necessarily represent those of their affiliated organizations, or those of the publisher, the editors and the reviewers. Any product that may be evaluated in this article, or claim that may be made by its manufacturer, is not guaranteed or endorsed by the publisher.
References
1. Limmroth V. Erkrankungen der hirnnerven. In: Limmroth V, Diener HC, editors. Neurologie für Praktiker. Darmstadt: Steinkopff-Verlag (2006). p. 401–17. doi: 10.1007/3-7985-1621-9_14
2. Jung EH, Kim S-J, Lee JY, Cho B-J. The incidence and etiology of sixth cranial nerve palsy in Koreans: a 10-year nationwide cohort study. Sci Rep. (2019) 9:18419. doi: 10.1038/s41598-019-54975-5
3. Patel SV, Mutyala S, Leske DA, Hodge DO, Holmes JM. Incidence, associations, and evaluation of sixth nerve palsy using a population-based method. Ophthalmology. (2004) 111:369–75. doi: 10.1016/j.ophtha.2003.05.024
4. Katsuno M, Yokota H, Yamamoto Y, Teramoto A. Bilateral traumatic abducens nerve palsy associated with skull base fracture–case report. Neurol Med Chir (Tokyo). (2007) 47:307–9. doi: 10.2176/nmc.47.307
5. Sbai AA, Es-Sahli F, Lachgar A, Elayoubi F. Concomitant abducens and facial nerve palsies following bone temporal fracture: case report. Ann Med Surg (Lond). (2022) 80:104318. doi: 10.1016/j.amsu.2022.104318
6. Drazin D, Wang JM, Alonso F, Patel DM, Granger A, Shoja MM, et al. Intracranial anatomical triangles: a comprehensive illustrated review. Cureus. (2017) 9(10):e1741. doi: 10.7759/cureus.1741
7. Wysiadecki G, Radek M, Tubbs RS, Iwanaga J, Walocha J, Brzeziński P, et al. Microsurgical anatomy of the inferomedial paraclival triangle: contents, topographical relationships and anatomical variations. Brain Sci. (2021) 11:596. doi: 10.3390/brainsci11050596
8. Tomio R, Horiguchi T, Shibao S, Tamura R, Yoshida K, Kawase T. Anterior transpetrosal approach and the tumor removal rate, postoperative neurological changes, and complications: experience in 274 cases over 33 years. J Neurosurg. (2024) 141(1):108–16. doi: 10.3171/2023.11.JNS231532
9. Jung I-H, Yoo J, Park HH, Hong C-K. Differences in surgical outcome between petroclival meningioma and anterior petrous meningioma. Acta Neurochir. (2021) 163:1697–704. doi: 10.1007/s00701-021-04753-y
10. Lang J. Eintritt und Verlauf der Hirnnerven (III, IV, VI) im Sinus Cavernosus. Z Anat Entwickl Gesch. (1974) 145:87–99. doi: 10.1007/BF00519129
11. Özveren MF, Erol FS, Alkan A, Kocak A, Önal C, Türe U. Microanatomical architecture of Dorello’s canal and its clinical implications. Oper Neurosurg. (2007) 60:1–8. doi: 10.1227/01.NEU.0000249229.89988.4D
12. Ozveren MF, Uchida K, Tekdemir I, Cobanoglu B, Akdemir I, Kawase T, et al. Dural and arachnoid membraneous protection of the abducens nerve at the petroclival region. Skull Base. (2002) 12:181–8. doi: 10.1055/s-2002-35749-1
13. Destrieux C, Velut S, Kakou MK, Lefrancq T, Arbeille B, Santini J-J. A new concept in Dorello’s canal microanatomy: the petroclival venous confluence. J Neurosurg. (1997) 87:67–72. doi: 10.3171/jns.1997.87.1.0067
14. Iaconetta G, Fusco M, Cavallo LM, Cappabianca P, Samii M, Tschabitscher M. The abducens nerve: microanatomic and endoscopic study. Oper Neurosurg. (2007) 61:7–14. doi: 10.1227/01.neu.0000289706.42061.19
15. Gruber W. Beitrage zur Anatomie des Keilbeines und Schlafenbeines. Imperatorskaja Akademija Nauk (St. Petersbourg) 7. ser., Tome. (1859) 1(3):3–l3.
16. Ozveren MF, Sam B, Akdemir I, Alkan A, Tekdemir I, Deda H. Duplication of the abducens nerve at the petroclival region: an anatomic study. Neurosurgery. (2003) 52:645–52; discussion 651–2. doi: 10.1227/01.neu.0000048186.18741.3c
17. Joo W, Yoshioka F, Funaki T, Rhoton AL. Microsurgical anatomy of the abducens nerve. Clin Anat. (2012) 25:1030–42. doi: 10.1002/ca.22047
18. Dorello P. Considerazioni sopra la causa della paralisi transitoria dell’ adducente nelIe flogosi dell’ orecchio medio. Atti Clin Otorinolaringoiatrica Univ Roma. (1905) 3:207–17.
19. Tubbs RS, Radcliff V, Shoja MM, Naftel RP, Mortazavi MM, Zurada A, et al. Dorello canal revisited: an observation that potentially explains the frequency of abducens nerve injury after head injury. World Neurosurg. (2012) 77:119–21. doi: 10.1016/j.wneu.2011.03.046
20. Prescher A, Brors D, Schick B. Topographische anatomie der felsenbeinspitze und des dorello-kanals. In: Bootz F, Strauss G, editors. Die Chirurgie der lateralen Schädelbasis. Berlin, Heidelberg: Springer (2002). p. 31–5. doi: 10.1007/978-3-642-56058-3_8
21. Richardson KC, Jarett L, Finke EH. Embedding in epoxy resins for ultrathin sectioning in electron microscopy. Stain Technol. (1960) 35:313–23. doi: 10.3109/10520296009114754
22. Gundersen HJG. Notes on the estimation of the numerical density of arbitrary profiles: the edge effect. J Microsc. (1977) 111:219–23. doi: 10.1111/j.1365-2818.1977.tb00062.x
23. Schüz A, Palm G. Density of neurons and synapses in the cerebral cortex of the mouse. J Comp Neurol. (1989) 286:442–55. doi: 10.1002/cne.902860404
24. Kshettry VR, Lee JH, Ammirati M. The Dorello canal: historical development, controversies in microsurgical anatomy, and clinical implications. Neurosurg Focus. (2013) 34:E4. doi: 10.3171/2012.11.FOCUS12344
25. Sawabe Y, Matsumoto K, Goto N, Otsuka N, Kobayashi N. Morphometric nerve fiber analysis and aging process of the human abducent nerve. Okajimas Folia Anat Jpn. (1998) 74:337–43. doi: 10.2535/ofaj1936.74.6_337
26. Swensson A. Fiber analysis of the trochlear nerve and abducens nerve. Acta Anat (Basel). (1949) 7:154–72. doi: 10.1159/000140379
28. Björkman A, Wohlfart G. Faseranalyse der Nn. oculomotorius, trochlearis und abducens des Menschen und des N. abducens verschiedener Tiere. Z Mikr-Anat Forsch. (1936) 39:631–47.
29. Maleci O. Contributo alla conoscenza delle variazioni quantitative delle cellule nervose nella sensenze. Arch Ital Anat Embryol. (1934) 33:883–901.
30. Harley RD. A quantitative study of the cells and fibers in the nucleus: nerve complexes of the fourth and sixth cranial nerves. Am J Ophthalmol. (1942) 25:1029–42. doi: 10.1016/S0002-9394(42)91316-3
31. Thorsteinsdottir K. Über Faserzahlen des N [ervus] Oculomotorius, N [ervus] Trochlearis, N [ervus] abducens, N [ervus] ophthalmicus, N [ervus] Maxillaris und N [ervus] Mandibularis Sowie die Faszikelanzahl des N [ervus] Maxillaris. [Doctoral Dissertation]. Würzburg, Germany: University of Würzburg (1982).
32. Rosenthal D. De numero atque mensura microscopica fibrillarum elementarium systematis cerebro-spinalis symbolae (1845).
33. Tergast P. Ueber das Verhältniss von Nerve und Muskel. Archiv f Mikrosk Anatomie. (1873) 9:36–46. doi: 10.1007/BF02956155
34. Chiba M. Die Abstufbarkeit der Seitwärtswendung des Auges und die Zahl der Fasern im N. abducens. Pflüger’s Archiv für die Gesamte Physiologie des Menschen und der Tiere. (1926) 212:150–7. doi: 10.1007/BF01723126
35. Bardosi A, Shallo J, Schäfer C, Mühlendyck H. Morphometric comparison between human and rat abducens and oculomotor nerves. Acta Anat (Basel). (1990) 138:24–31. doi: 10.1159/000146916
36. Moriyama H, Hayashi S, Inoue Y, Itoh M, Otsuka N. Sex differences in morphometric aspects of the peripheral nerves and related diseases. NeuroRehabilitation. (2016) 39:413–22. doi: 10.3233/NRE-161372
37. Sunderland S, Hughes ESR. The pupillo-constrictor pathway and the nerves to the ocular muscles in man. Brain. (1946) 69:301–9. doi: 10.1093/brain/69.4.301
38. Tomasch J, Schwarzacher HG. Die innere Struktur peripherer menschlicher Nerven im Lichte faseranalytischer Untersuchungen. Cells Tissues Organs. (1952) 16:315–54. doi: 10.1159/000140785
39. Agduhr E. A contribution to the technique of determining the number of nerve cells per volume unit of tissue. Anat Rec. (1941) 80:191–202. doi: 10.1002/ar.1090800205
40. Jotz GP, De Campos D, Rodrigues MF, Xavier LL. Histological asymmetry of the human recurrent laryngeal nerve. J Voice. (2011) 25:8–14. doi: 10.1016/j.jvoice.2009.06.007
41. Miyauchi Y, Moriyama H, Goto N, Goto J, Ezure H. Morphometric nerve fiber analysis of the human Inferior alveolar nerve: lateral asymmetry. Okajimas Folia Anat Jpn. (2002) 79:11–4. doi: 10.2535/ofaj.79.11
42. Moriyama H, Itoh M, Shimada K, Otsuka N. Morphometric analysis of fibers of the human vestibular nerve: sex differences. Eur Arch Otorhinolaryngol. (2007) 264:471–5. doi: 10.1007/s00405-006-0197-5
43. Kuruc R, Haviarova Z, Halgas F, Matejcik V. Intracranial intradural variations of nerve roots. Bratisl Lek Listy. (2021) 122:242–7. doi: 10.4149/BLL_2021_039
44. Piffer CR, Zorzetto NL. Course and relations of the abducens nerve. Anat Anz. (1980) 147:42–6.7396225
45. Jain KK. Aberrant roots of the abducent nerve. J Neurosurg. (1964) 21:349–51. doi: 10.3171/jns.1964.21.5.0349
46. Nathan H, Ouaknine G, Kosary IZ. The abducens nerve. Anatomical variations in its course. J Neurosurg. (1974) 41:561–6. doi: 10.3171/jns.1974.41.5.0561
47. Harris FS, Rhoton AL. Anatomy of the cavernous sinus: a microsurgical study. J Neurosurg. (1976) 45:169–80. doi: 10.3171/jns.1976.45.2.0169
48. DiDio LJ, Baptista CA, Teofilovski-Parapid G. Anatomical variations of the abducent nerve in humans. Arch Ital Anat Embriol. (1990) 95:167–72.2102068
49. Umansky F, Valarezo A, Elidan J. The microsurgical anatomy of the abducens nerve in its intracranial course. Laryngoscope. (1992) 102:1285–92. doi: 10.1288/00005537-199211000-00016
50. Tekdemir İ, Deda H, Karahan ST, Arıncı K. The intracranial course of the abducens nerve. Turk Neurosurg. (1996) 6:96–102.
51. Alkan A, Sigirci A, Ozveren MF, Kutlu R, Altinok T, Onal C, et al. The cisternal segment of the abducens nerve in man: three-dimensional MR imaging. Eur J Radiol. (2004) 51:218–22. doi: 10.1016/j.ejrad.2003.10.004
52. Romero FR, Ramos JG, Chaddad-Neto F, Bethencourt JMA, de Oliveira E. Microsurgical anatomy and injuries of the abducens nerve. Arq Neuro-Psiquiatr. (2009) 67:96–101. doi: 10.1590/S0004-282X2009000100022
53. Ozer E, Icke C, Arda N. Microanatomical study of the intracranial abducens nerve: clinical interest and surgical perspective. Turk Neurosurg. (2010) 20:449–56. doi: 10.5137/1019-5149.JTN.3303-10.1
54. Zhang Y, Yu H, Shen B-Y, Zhong C-J, Liu E-Z, Lin Y-Z, et al. Microsurgical anatomy of the abducens nerve. Surg Radiol Anat. (2012) 34:3–14. doi: 10.1007/s00276-011-0850-6
55. Wysiadecki G, Orkisz S, Gałązkiewicz-Stolarczyk M, Brzeziński P, Polguj M, Topol M. The abducens nerve: its topography and anatomical variations in intracranial course with clinical commentary. Folia Morphol (Warsz). (2015) 74:236–44. doi: 10.5603/FM.2015.0037
56. Ipsalali HO, Ciftci AC, Kilic D, Sendemir G, Seyhan S, Kaya I, et al. Variations of the 6th cranial nerve (nervus abducens) in the petroclival region: a microsurgical study. Morphologie. (2019) 103:103–9. doi: 10.1016/j.morpho.2019.01.001
57. Haładaj R. Comparison of lateral and medial rectus muscle in human: an anatomical study with particular emphasis on morphology, intramuscular innervation pattern variations and discussion on clinical significance. Surg Radiol Anat. (2020) 42:607–16. doi: 10.1007/s00276-019-02400-x
58. Rotenstreich L, Eran A, Siegler Y, Grossman R, Edery N, Cohen R, et al. Unveiling the vulnerability of the human abducens nerve: insights from comparative cranial base anatomy in mammals and primates. Front Neuroanat. (2024) 18:1383126. doi: 10.3389/fnana.2024.1383126
59. Wang JMH, Edwards BA, Loukas M, Oskouian RJ, Tubbs RS. Supernumerary abducens nerves: a comprehensive review. World Neurosurg. (2018) 112:39–45. doi: 10.1016/j.wneu.2017.11.052
60. Guthrie S. Patterning and axon guidance of cranial motor neurons. Nat Rev Neurosci. (2007) 8:859–71. doi: 10.1038/nrn2254
61. Gilbert PW. The origin and development of the extrinsic ocular muscles in the domestic cat. J Morphol. (1947) 81(2):151–93. doi: 10.1002/jmor.1050810203
62. Bremer JL. Aberrant roots and branches of the abducent and hypoglossal nerves. J Comp Neurol Psychol. (1908) 18:619–39. doi: 10.1002/cne.920180605
63. Wahl CM, Noden DM, Baker R. Developmental relations between sixth nerve motor neurons and their targets in the chick embryo. Dev Dyn. (1994) 201:191–202. doi: 10.1002/aja.1002010209
64. Sato M, Cho KH, Yamamoto M, Hirouchi H, Murakami G, Abe H, et al. Cavernous sinus and abducens nerve in human fetuses near term. Surg Radiol Anat. (2020) 42:761–70. doi: 10.1007/s00276-020-02443-5
65. Salas E, Ziyal IM, Sekhar LN, Wright DC. Persistent trigeminal artery: an anatomic study. Neurosurgery. (1998) 43:557–61. doi: 10.1097/00006123-199809000-00082
66. Lang J, Reiter U. Course of the abducens nerve before the exit zone from the central nervous system to the lateral rectus muscle. Neurochirurgia (Stuttg). (1985) 28:1–5. doi: 10.1055/s-2008-1054170
67. Sunderland S. Neurovascular relations and anomalies at the base of the brain. J Neurol Neurosurg Psychiatry. (1948) 11:243–57. doi: 10.1136/jnnp.11.4.243
68. Hara R, Mano T, Yano S, Toda T. Isolated Abducens nerve palsy caused by anterior Inferior cerebellar artery compression. Intern Med. (2022) 61:2991–2. doi: 10.2169/internalmedicine.9294-21
69. Iaconetta G, Tessitore E, Samii M. Duplicated abducent nerve and its course: microanatomical study and surgery-related considerations. J Neurosurg. (2001) 95:853–8. doi: 10.3171/jns.2001.95.5.0853
70. Borg A, Zrinzo L. Aberrant abducent nerve during microvascular decompression for trigeminal neuralgia. World Neurosurg. (2020) 138:454–6. doi: 10.1016/j.wneu.2020.03.115
71. Stopford JS. The arteries of the pons and medulla Oblongata. J Anat Physiol. (1916) 50:131–64.17233055
72. Haładaj R. Normal anatomy and anomalies of the rectus extraocular muscles in human: a review of the recent data and findings. BioMed Res Int. (2019) 2019:1–9. doi: 10.1155/2019/8909162
73. Nam YS, Kim I, Shin SY. Detailed anatomy of the abducens nerve in the lateral rectus muscle. Clin Anat. (2017) 30:873–7. doi: 10.1002/ca.22918
74. Peng M, Poukens V, Da Silva Costa RM, Yoo L, Tychsen L, Demer JL. Compartmentalized innervation of primate lateral rectus muscle. Invest Ophthalmol Vis Sci. (2010) 51:4612. doi: 10.1167/iovs.10-5330
75. Székely G, Matesz C. The protection of the eye: the accessory abducens nucleus. In: Székely G, Matesz C, editors. The Efferent System of Cranial Nerve Nuclei: A Comparative Neuromorphological Study. Berlin, Heidelberg: Springer (1993). p. 19–23. doi: 10.1007/978-3-642-77938-1_5
76. Gonzalez A, Munoz M. Distribution and morphology of abducens motoneurons innervating the lateral rectus and retractor bulbi muscles in the frog rana ridibunda. Neurosci Lett. (1987) 79:29–34. doi: 10.1016/0304-3940(87)90667-7
77. Spencer RF, Baker R, McCrea RA. Localization and morphology of cat retractor bulbi motoneurons. J Neurophysiol. (1980) 43:754–70. doi: 10.1152/jn.1980.43.3.754
78. Spencer RF, Porter JD. Innervation and structure of extraocular muscles in the monkey in comparison to those of the cat. J Comp Neurol. (1981) 198:649–65. doi: 10.1002/cne.901980407
79. Ogut E, Armagan K, Barut C. Reappraisal of the types of trigeminal porus and importance in surgical applications. Surg Radiol Anat. (2021) 43:1169–78. doi: 10.1007/s00276-020-02651-z
80. Ciołkowski M, Sharifi M, Krajewski P, Ciszek B. Topography and morphometry of the porus trigeminus. Neurol Neurochir Pol. (2006) 40:173–8.16794955
81. Arslan M, Deda H, Avci E, Elhan A, Tekdemir I, Tubbs S, et al. Anatomy of Meckel’s cave and the trigeminal ganglion: anatomical landmarks for a safer approach to them. Turk Neurosurg. (2011) 22(3):317–23. doi: 10.5137/1019-5149.JTN.5213-11.1
82. Dupont G, Altafulla J, Iwanaga J, Watanabe K, Tubbs RS. Ossification of the roof of the porus trigeminus with duplicated abducens nerve. Anat Cell Biol. (2019) 52:211. doi: 10.5115/acb.2019.52.2.211
83. Wegner RN. Das ligamentum sphenopetrosum gruber=abducensbrücke und homologe gebilde. Anat Anz. (1920) 53:161–75.
84. Lang J. Structure and postnatal organization of heretofore uninvestigated and infrequent ossifications of the sella turcica region. Acta Anat (Basel). (1977) 99:121–39. doi: 10.1159/000144840
87. Wysiadecki G, Polguj M, Topol M. An unusual variant of the abducens nerve duplication with two nerve trunks merging within the orbit: a case report with comments on developmental background. Surg Radiol Anat. (2016) 38:625–9. doi: 10.1007/s00276-015-1573-x
88. Ramkumar M, Sharma S, Jacob TG, Bhardwaj DN, Nag TC, Roy TS. The human trochlear and abducens nerves at different ages - a morphometric study. Aging Dis. (2015) 6:6. doi: 10.14336/AD.2014.0310
89. Umansky F, Elidan J, Valarezo A. Dorello’s canal: a microanatomical study. J Neurosurg. (1991) 75:294–8. doi: 10.3171/jns.1991.75.2.0294
90. Iwanaga J, Altafulla JJ, Gutierrez S, Dupont G, Watanabe K, Litvack Z, et al. The petroclinoid ligament: its morphometrics, relationships, variations, and suggestion for new terminology. J Neurol Surg B Skull Base. (2020) 81:603–9. doi: 10.1055/s-0039-1692699
91. Choi C-Y, Han S-R, Yee G-T, Lee C-H. A case of aberrant abducens nerve in a cadaver and review of its clinical significance. J Korean Neurosurg Soc. (2010) 47:377. doi: 10.3340/jkns.2010.47.5.377
Keywords: cranial nerve, dura mater, skull base, anatomical variation, neurosurgery
Citation: Bach M, Schmeisser MJ and Schumann S (2025) Morphometric analysis of the abducens nerve in the petroclival region. Front. Surg. 12:1574047. doi: 10.3389/fsurg.2025.1574047
Received: 10 February 2025; Accepted: 9 April 2025;
Published: 24 April 2025.
Edited by:
Aristotelis Kalyvas, University of Toronto, CanadaReviewed by:
Francesco Carbone, Städtisches Klinikum Karlsruhe, GermanyFrancesco Corrivetti, Azienda Sanitaria Locale Salerno, Italy
Copyright: © 2025 Bach, Schmeisser and Schumann. This is an open-access article distributed under the terms of the Creative Commons Attribution License (CC BY). The use, distribution or reproduction in other forums is permitted, provided the original author(s) and the copyright owner(s) are credited and that the original publication in this journal is cited, in accordance with accepted academic practice. No use, distribution or reproduction is permitted which does not comply with these terms.
*Correspondence: S. Schumann, c3Zlbi5zY2h1bWFubkBtaGItZm9udGFuZS5kZQ==