- 1Normandie Univ, UNICAEN, CIREVE, Caen, France
- 2Normandie Univ, UNICAEN, INSERM, COMETE, GIP Cyceron, Caen, France
- 3Laboratoire de Cyberpsychologie, Département de Psychologie, Université du Québec en Outaouais, Gatineau, QC, Canada
- 4Aix Marseille Univ, CNRS, ISM, Marseille, France
- 5Université de Lorraine, DevAH, Nancy, France
Field dependence–independence (FDI) is a psychological construct determining an individual’s approach of the perception–cognition coupling. In virtual reality (VR) context, several studies suggest that an individual’s perceptive style is susceptible to shift toward a more FI mode through down-weighting of conflicting visual cues. The present study proposes to investigate the potential flexible nature of FDI following a virtual immersion and to assess if this flexibility might be associated with the subjective experience of VR. 86 participants explored a real-world–like virtual environment for approximately 10 min. FDI levels were measured before and after the VR exposure using the rod-and-frame test. Their subjective experience of VR was measured a posteriori (cybersickness and sense of presence) and used in order to build two experimental groups via a cluster analysis. The results showed that only participants with a poor subjective experience of VR (i.e., a low level of sense of presence associated with a high level of cybersickness) significantly shifted to a more FI mode, which is discussed as a sensory re-weighting mechanism. Pragmatical applications are discussed, and future studies are outlined, based on the conclusion that FDI might be more flexible than we thought, which could shed light on the psychophysiology of VR.
Introduction
Cognitive styles are defined as relatively stable strategies, preferences, and attitudes that determine the individual’s “typical mode of thinking, perceiving, remembering, and problem solving” (Messick, 1976). The most prevalent and studied factor in the literature on cognitive styles is the field dependence–independence (FDI), which is more often considered as a perceptive style (Zhang, 2004). This unidimensional construct corresponds to an individual’s ability to perform perceptual analytic tasks (Witkin et al., 1971) such as measured by the embedded figures test or the rod-and-frame test (Witkin et al., 1962). Field-dependent (FD) individuals are strongly influenced by the holistic organization of the perceptual field: parts of the field are “fused” (Pithers, 2002). They are less analytical, not attentive to detail, and see the perceptual field as a whole. By contrast, field-independent (FI) individuals are strongly dominated by the analytical organization of the perceptual field: the field is broken down into its component parts. These individuals are typically not influenced by the existing structure and can make choices independently of the perceptual field. It is interesting to note that FD and FI perceptive styles were primarily considered to designate the type of preferential sensory cues used for perception (Witkin and Asch, 1948). Indeed, FD individuals use dominantly visual cues, while FI ones use dominantly nonvisual cues, notably vestibular, tactile, and proprioceptive ones (Witkin and Asch, 1948; Tinajero and Páramo, 1998).
FDI scores typically follow a normal distribution, and Witkin et al. (1962) suggested that being on either side is the illustration of different cognitive strategies but not a reliable indicator for overall performance. However, many authors disagreed with this statement (Pithers, 2002; Evans et al., 2013), suggesting that being on the FI side has more advantages than disadvantages. For example, FI individuals seem to reduce their cognitive load by being able to select and represent relevant information (inhibiting irrelevant information), so they have less information to process overall, thereby enhancing efficiency of cognitive processing (Evans et al., 2013; Nori et al., 2021). In addition, and in line with these results, it has been repeatedly found that being more FD is associated with being more prone to motion sickness (Kennedy, 1975; Cian et al., 2011). Since motion sickness is suggested to be caused by a perceptive mismatch between different sensory modalities (Reason and Brand, 1975), it is arguable that FD individuals experience more symptoms because they have more difficulties inhibiting incongruous information and thus resolving perceptive mismatch. Moreover, the prevalence of visual information over other sensory stimuli in virtual environments has to be underlined as the conflict of vision with other senses is often considered as the origin of cybersickness (Bos et al., 2008; Rebenitsch and Owen, 2016). Following from this, it seems likely that FD individuals, relying dominantly on visual cues, will experience more cybersickness in virtual reality (VR). This assumption is empirically supported by the association found between sensitivity to visual information (notably motion parallax) and cybersickness in VR (Fulvio et al., 2021). These two aspects found among FD individuals (poorer inhibition skills and greater sensitivity to perceptive mismatch) probably interact and reveal FDI as a pertinent construct in order to explore individual differences in the way people experience VR.
Cybersickness is a manifestation of the sensory integration of the layered virtual and physical environments, integration which is at the core of VR phenomenology. Yet, this psychological experience cannot be fully considered without a discussion of the sense of presence. The sense of presence is a famous VR concept often described as the sensation of “being there” in the virtual environment (Heeter, 1992; Sheridan, 1992), and is at the heart of many VR experiences (Slater 2018). Here, and in line with Hecht and Reiner (2007), we consider the idea that FDI can shed light on the emergence and maintenance of the sense of presence. Indeed, inhibition abilities are mandatory in order to build a sense of presence, notably by preventing the processing of VR-irrelevant information from the physical world, or even by inhibiting the gap between the “virtual experience and the real-world experiences” (Hecht and Reiner, 2007). Moreover, Hecht and Reiner (2007) have argued that FD individuals have more difficulty in inhibiting bugs or flaws in the virtual visual field since they rely more on internal (non-visual) cues for the processing of information, amplifying breaks in presence (Slater and Steed, 2000). In addition, FD individuals are suggested as presenting less self-completion of the missing cues, which is mandatory to build a sense a presence based on “imperfect” virtual environments. These authors based this discussion on a positive association found between object presence, a sub-dimension of the sense of presence, and FI (Hecht and Reiner, 2007). They also assumed that this association would be observed with the sense of presence in the broadest sense of the term.
Taken together, these theoretical predispositions suggesting FD individuals to be 1) more likely to experience cybersickness and 2) less prone to the sense of presence make FDI an interesting concept in order to explore the psychophysiology of VR. Indeed, the sense of presence and cybersickness are two negatively correlated psychological constructs which largely determine an individual psychological construction of the virtual environment (Weech et al., 2019). Understanding how and why individuals experience VR differently is crucial because this will affect a wide range of behaviors, from the learning and transfer of specific skills (Piccione et al., 2019; Grassini et al., 2020) to the effectiveness and acceptance of psychological therapies (Gutierrez-Maldonado et al., 2010; Wallach et al., 2011; Rus-Calafell et al., 2013), the immersion analgesic effect (Hoffman et al., 2004), and even the performance on a spatial cognition task (Maneuvrier et al., 2020). However, despite a strong correlation between the sense of presence and cybersickness, Maneuvrier et al. (2020) showed association neither between sense of presence and FDI nor between cybersickness and FDI. It has to be noted that FDI was evaluated prior to the virtual immersion. However, it is probable that it is not the assessment of a stable FDI prior to virtual immersion that should be considered with regard to the subjective experience of VR, but rather its flexibility in response to an immersive environment. There is a controversy surrounding the flexible nature of FDI. Witkin et al. (1971) suggested that the level of FDI is stable and pervasive upon reaching adulthood; yet, more recent studies suggest a probable flexibility of FDI and even the possibility to train people to change their cognitive style (Pithers, 2002). Possible changes in FDI are sometimes suggested as sensory re-weighting mechanisms in order to reduce the relative weights of unreliable (visual) information (Bray et al., 2004; Mahboobin et al., 2005; Pavlou et al., 2011; Scotto Di Cesare et al., 2015). A neurophysiological basis for this sensory re-weighting process has notably been outlined (Andersen and Buneo, 2003; Medendorp et al., 2018), and it is suggested as playing a crucial role in coping with perceptive mismatch through an update of the internal model (Oman, 1990; Oman and Cullen, 2014). In addition, sensory re-weighting has been found to be associated with the particularly visually induced motion sickness, that is, cybersickness (Weech et al., 2018; Weech et al., 2020).
The objective of the present study is to investigate the potential flexible nature of FDI during a spatial exploration in VR and to assess if its flexibility might be associated with the subjective experience of VR. We expected that the FD level will be lower post-immersion versus pre-immersion and that this shift toward FI will be associated with a poor subjective experience of VR (a low level of sense of presence and a high level of cybersickness) as a manifestation of sensory re-weighting compensatory mechanisms.
Materials and Methods
Participants
86 participants were recruited from the Psychology Department of the University of Caen Normandie using a range of strategies, by announcements in classes, email lists, or informal personal conversations. 45 of them were female (mean age and standard deviation: 19.82 ± 2.07 years) and 41 were male (20.34 ± 1.83 years). Exclusion criteria were 1) being younger than 18 or older than 35 years, 2) with current or past presence of neurological or psychiatric disorders, 3) having uncorrected vision or visual impairments that does not allow stereoscopic vision, and 4) having motor impairments that make the use of hand controllers impossible. Approval for the protocol was obtained from the Local Ethics Committee (#CEREP-19-011-PD). All participants signed an informed consent prior to data collection in accordance with the Helsinki Declaration (World Medical Association, 2013). Participants were informed that they could stop the experiment at any time without any justification, but none of them did.
Virtual Environment
The Unity3D engine programmed with the C# language was used to create the virtual environment. The HTC Vive™ head-mounted display (HMD, 1,080 × 1,200 pixels per eye, 90 Hz, 110° field of view) was used to run the virtual environment in real time with the API and SDK of OpenVR and SteamVR. The hardware computer supporting the display incorporated an NVIDIA® GTX-1080 graphics card, 16 GB of RAM, and an Intel® Core i5 processor in order to run the software with a constant frame rate (i.e., constant 70 frames per second). The HTC Vive™ hand controllers were used: participants moved in the environment by pressing a button on the controller to teleport them over a short distance. This way of navigation has been found to reduce cybersickness, contrary to linear motion as if the user was walking (Clifton and Palmisano, 2019). The tracking of hand controllers was used in order to allow the participants to open the virtual doors by virtually touching them. Different assets were added in order to increase the subject’s sense of presence: a small haptic (i.e., controller vibration) and auditory (i.e., sound) feedbacks were associated with the touch and opening of doors. Trying to open a locked door also produced different auditory and haptic feedbacks. In addition, two ambient looping sounds were used in the environment: the sound of the wind blowing and intelligent artificial birds singing and flying around. All sounds were 3D-spatialized with the exception of the ambient sounds. The virtual environment was big enough to make the participants think of an open world that they can fully explore, but it was actually a guided tour with 10 “choke points” where participants had to choose between two directions, represented by doors. The doors from the “right direction” could be opened, while doors on the “wrong direction” could not. Wooden signs along the different pathways indicated the right way. The environment represented Ancient Rome, for the simple reason that 3D high-quality models were developed and available in the laboratory (Figure 1).
FDI Assessment
FD was evaluated pre-immersion (FDpre) and post-immersion (FDpost) using a virtual rod-and-frame test (RVR software, Virtualis®, Figure 2). Positioned in an upright sitting position with the feet not touching the ground, participants had to align, with a joystick, a rod initially roll tilted at 27° with respect to the gravitational vertical (0°) within a static frame tilted at 18°. Sixteen trials were made from combinations of two right and left rod tilts and two right and left frame tilts. The trials were presented in a random order. At each trial, the absolute error relative to the gravitational vertical was measured in degree. The individual FDI level was measured using the mean absolute error. The higher the mean absolute error, the more the participant’s subjective visual vertical is influenced by the tilted frame and thus the higher the level of FD. The FDI evolution (FDev) was calculated using a simple ratio: FDev = (FDpost—FDpre)/FDpre. This latter variable corresponded to the FDI flexibility.
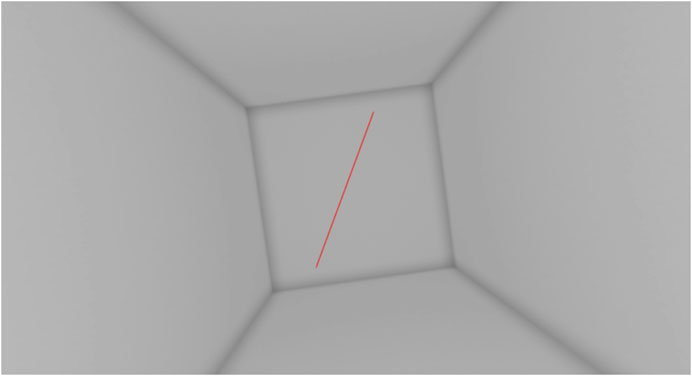
FIGURE 2. First-person view of the virtual Rod and Frame Test (RVR software, Virtualis®) at the beginning of a trial.
Experimental Procedure
Participants were equipped with the head-mounted display and had to pass the first session of the virtual rod-and-frame test (Witkin et al., 1962). Once their FDpre was measured, participants were immersed into a virtual tutorial in order to learn how to interact with the environment (moving around and opening doors). At the end of the tutorial, participants were asked if they wanted to do it again, but none of them chose to. Then, participants were invited to perform a first guided visit in the virtual environment with these instructions: “Follow the signs on the way. There is no time limit, but try to be as fast as possible”. Once the first visit was done, participants were placed back to the beginning of the tour and had to start over, but this time, the signs indicating the way were removed, with the following instructions: “You now have to redo the visit, but without the signs to help you. There is no time limit but try to be as fast as possible”. Once the second visit was completed, participants had to respond to the French validated questionnaires of presence without haptic items (Robillard et al., 2002) and of simulator sickness (Bouchard et al., 2007). Finally, their individual FDI level was measured a second time (FDpost), following exactly the same procedure as before the experiment (FDpre).
Statistical Analysis
All analyses were performed using statistical software JASP, version 0.14.1. First, cybersickness and sense of presence scores were compared (Student t-test) to their validated norms to ensure the validity of the virtual environment. As the whole duration of the virtual exposure was slightly different for each participant, linear regressions were used to control for a possible immersion duration effect on both the sense of presence and cybersickness. Pearson’s r coefficient was used to confirm the association between the sense of presence and cybersickness. Depending on the size of the correlation and its significance, these two constructions were used together to account for the individual VR experience. In addition, Pearson’s r coefficients were used to explore the associations between the sense of presence, cybersickness, FDev, and FDpre. Because our a priori hypotheses were directional, one-tailed tests were used for correlations analyses. In order to build experimental groups based on their individual VR experience as measured by their scores of sense of presence and cybersickness, a K-means clustering unsupervised machine learning algorithm (25 max iterations, 25 random sets, Hartigan–Wong algorithm) was used. The optimal number of clusters k was determined by the silhouette algorithm. In order to assess FDev, both within participants (FDpre and FDpost as repeated measures) and between participants (depending on the VR experience outlined by the number of clusters identified), a mixed model ANOVA was applied. Post hoc Holm’s corrections and Tukey’s tests were used to investigate further the potential differences outlined by the mixed ANOVAs. At last, in order to investigate if FDpre might predict Fdev, a linear regression (enter method) was used with FDev as the dependent variable. Normality was tested using the Shapiro–Wilk test, and collinearity diagnostics using the variance inflation factor. Quantitative data were expressed as the mean ± standard deviations. Size effects were reported using the η2 (Eta-square) for the ANOVAs, R2 for the linear regressions, and Cohen’s d for Tuckey’s post hoc, along with confidence intervals. The significance threshold was set at 0.05.
Results
Sense of Presence and Cybersickness Scores
Compared to the French validated norms of the Presence Questionnaire (Robillard et al., 2002), the participants reported a posteriori significantly higher scores of sense of presence (113.9 ± 11.37) than the norms (91.96 ± 18.99): t (85) = 5.88, p < 0.001, Cohen’s d = 9.89, 95% CI [8.6, 11.27]. Compared to the French validated norms of the Simulator Sickness Questionnaire (Bouchard et al., 2007), the participants reported a posteriori significantly lower scores of cybersickness (5.43 ± 3.63) than the norms (7.12 ± 6.04): t (85) = -4.3, p < 0.001, Cohen’s d = 1.45, 95% CI [1.223, 1.751]. Linear regressions showed that neither the sense of presence scores nor the cybersickness’s ones were significantly associated with the immersion duration (554.47 ± 71.68 s).
Correlation Analyses: VR Experience
Because the Shapiro–Wilk test for multivariate normality was not significant (0.981, p = 0.243), the relationship between presence and cybersickness scores was explored using a Pearson’s r correlation and was found to be moderately negatively correlated, r (86) = -0.365, p = 0.001 95% CI [-0.2, -1], paving the way for a cluster analysis on these two dimensions. In addition, FDpre was correlated neither with cybersickness nor with the sense of presence but significantly correlated with FDev: r (86) = -0.0246, p = 0.011 95% CI [-0.07, -1]. FDev itself was significantly correlated with the sense of presence (r (86) = 0.0215, p = 0.023 95% CI [1, 0.038]) and cybersickness (r (86) = -0.199, p = 0.033 95% CI [-0.021, -1]).
Clustering Analysis and Group Constitution on VR Experience
The k-means clustering, optimized with respect to the silhouette value, found two clusters among the sense of presence and cybersickness dimension (N = 86, R2 = 0.47, AIC = 96.65, BIC = 106.47, silhouette = 0.42, Figure 3). The first cluster was designated as the VRE + group, the one with a good subjective experience of VR, and the second cluster as the VRE group, the one with a poor subjective experience of VR (Table 1).
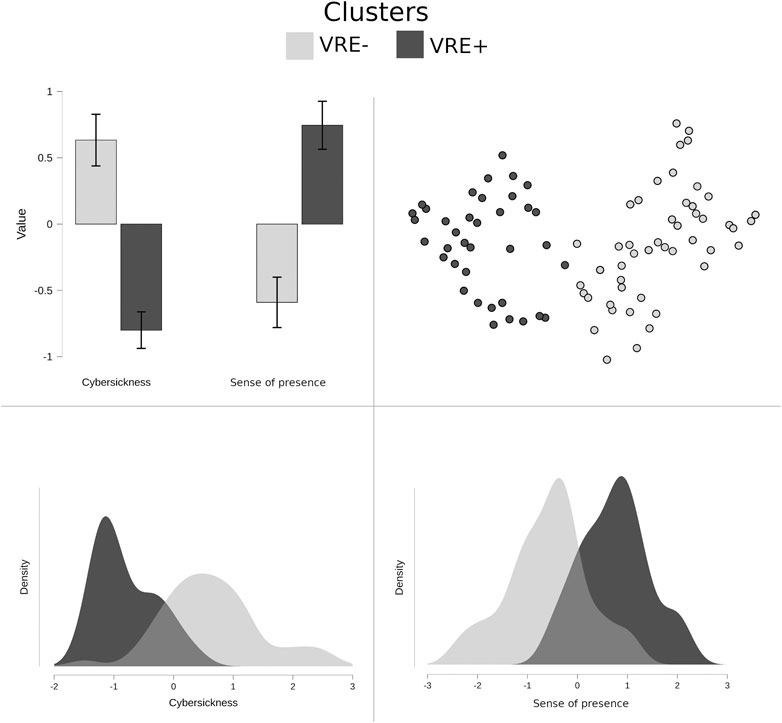
FIGURE 3. Graphical representations of the K-means clustering analysis based on two dimensions (sense of presence, cybersickness), revealing two clusters (VRE for “virtual reality experience -” “ in light gray and VRE + for virtual reality experience +” in dark gray). (A) Cluster mean plots, (B) t-distributed stochastic neighbor embedding (t-SNE) cluster plot. (C) Cluster density plot on cybersickness. (D) Cluster density plot on sense of presence.
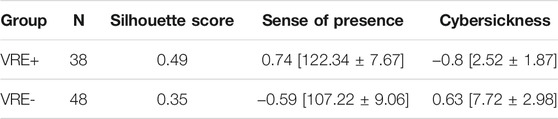
TABLE 1. Means and mean standard deviations of the two experimental groups (VR+, VR-) divided between the clusters found on the analysis of the two dimensions of the VR experience.
ANOVAs on FDI Levels
The whole mixed model ANOVA revealed a significant within-subjects simple effect [F (1.84) = 30.23, p = < 0.001, η2 = 0.013], showing that scores of FDpre (5.67 ± 2.25) were globally significantly higher than scores of FDpost (5.15 ± 2.11): t (86) = 5.66, p < 0.001, Cohen’s d = 0.611, 95% CI [0.33, 0.70]. This represents a global diminution of FD (∼7.7%) post-immersion. No significant between-subjects main effect was found. However, the ANOVA revealed a significant interaction between within-participants (FDpre, FDpost) and between-subjects measures (VRE+, VRE-): F (1.84) = 4.54, p = < 0.036, η2 = 0.002. The post hoc test showed no significant difference on FDpre between groups but revealed a significant simple effect between FDpre and FDpost among the VRE group: t (48) = 5.74, p < 0.001. Based on the FDev ratio measured and the between-groups ANOVA, this represents a mean diminution of FD after the virtual immersion of 3.3% for the VRE + group and 11.2% for the VRE-group, for which the difference is significant: F (1, 84) = 6.25, p = 0.014, η2 = 0.069 (t (84) = 2.5, p = 0.014, Cohen’s d = 0.54, CI 95% [0.016, 0.142]), as illustrated in Figure 4.
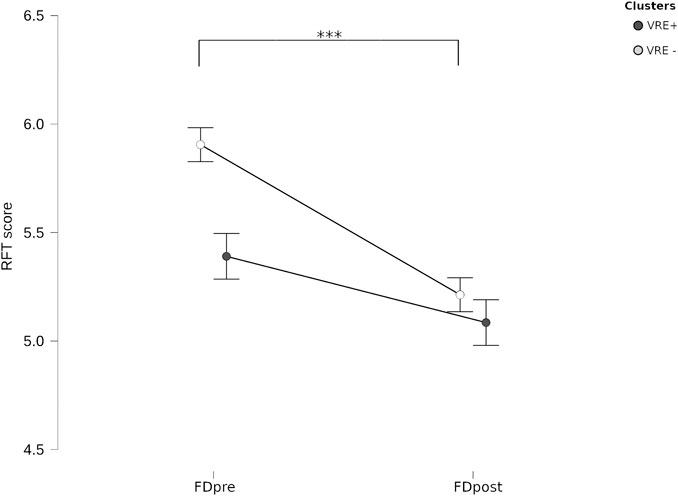
FIGURE 4. Mean RFT (rod-and-frame test) scores and standard errors of FD before (FDpre) and after (FDpost) VR immersion in each VR nexperience cluster (VRE+ and VRE-). ***: p < 0.001.
Linear Regression on FD Evolution
Scores of FDpre significantly predicted FDev (β = -0.24, t (85) = -2.23, p = 0.022, CI 95% [-0.03, -0.002]): F (1,84) = 5,4, R2 = 0.06, p = 0.022.
Discussion
The primary objective of this study was to analyze the possible flexible nature of FDI and assess if its flexibility might be associated with the subjective experience of VR. In agreement with our hypotheses, empirical results of the present study revealed a shift toward FI after the virtual immersion but only among the group of participants reporting a poor subjective experience of VR. First, we will discuss the flexible nature of FDI outlined in this study. Second, we will interpret this flexibility with regard to the sensory re-weighting mechanisms. Finally, we will discuss the role and applications of FDI in the psychophysiology of VR.
The main result of this study is the fact that virtual immersion (in the form of a 10-min spatial exploration task) induced a global diminution of FD, highlighting its flexibility. Previous studies and notably Pavlou et al. (2011) have found a similar phenomenon in participants exposed to strong optokinetic visual stimuli. However, the present study is the first, to our knowledge, to reveal the flexibility of FDI in a real environment–like virtual spatial exploration. The virtual environment used here was not particularly stimulating from a sensory point of view as it did not, for example, incorporate a linear visual flow or any optokinetic visual stimuli. In other words, the virtual environment was not built in order to induce a high state of discomfort. Indeed, the sense of presence and cybersickness scores were, respectively, higher and lower than the norms, thanks to an optimized way of navigation and the quality of interaction (Rebenitsch and Owen, 2016; Clifton and Palmisano, 2019). Despite this, we observed a shift toward FI among the participants manifesting a poor subjective experience of VR but not among those manifesting a good subjective experience of VR. Since the FDI flexibility is not found among every participant, it is unlikely that this shift is a systematic effect of VR exposure, but rather results from the interaction between interindividual factors and immersion. It should be noted that our results do not allow us to draw conclusions about the FDI flexibility of participants with a good subjective experience of VR: it is possible that these individuals manifested less (or no) flexibility because they were already adapted to the immersion not necessary because they are unable to manifest such flexibility. Based on this, we propose that the FDI flexibility observed here results from sensory re-weighting (i.e., adaptative response) in order to cope with a poor subjective experience of VR. Indeed, considering the solid literature discussing the links between perceptive mismatch and motion sickness (Reason and Brand, 1975; Oman, 1990; Rebenitsch and Owen, 2016), between perceptive mismatch and sensory re-weighting (Bray et al., 2004; Oman and Cullen, 2014; Mahboobin et al., 2005; Weech et al., 2018,2020) and between FDI and perceptive mismatch (Kennedy, 1975; Cian et al., 2011), it seems coherent to interpret the shift toward FI as a sensory re-weighting compensatory mechanism. According to this view, internal models, in a situation of perceptive mismatch, might trigger a change of FDI (i.e., perceptive style) in order to cope with sensory incongruence, notably by reducing the processing and weight of problematic cues. In the present study, mismatch between visual cues and other sensory channels may have induced an evolution toward a less visually dependent multisensory integration and thus a shift toward FI. This interpretation is in line with the suggested prevalence of visual incoherence in cybersickness (LaViola, 2000; Bos et al., 2008; Shafer et al., 2017) and with other recent discussions on the role of sensory re-weighting (Mahboobin et al., 2005; Pavlou et al., 2011; Scotto Di Cesare et al., 2015; Weech et al., 2020). It seems important to note that sensory re-weighting is also often associated with postural adjustments (Weech et al., 2020), which is very interesting considering the theory of motion sickness for which postural instability precedes the emergence of symptoms (Stoffregen and Smart, 1998).
If we consider the FDI flexibility as a manifestation of a sensory re-weighting mechanism, then its prevalence among participants with a poor subjective experience of VR is not surprising. While sensory re-weighting is rather well-identified in the regulation of the perceptive conflict leading to cybersickness, their contribution to the sense of presence remains unclear. It can certainly be argued that the sense of presence, and notably the spatial presence, is a complex construction relying on sensory integration and is thus impacted by its quality and its weighting (Biocca et al., 2001; Wirth et al., 2007; Pritchard et al., 2016; Weech et al., 2019). According to this view, participants with a poorly adapted sensory integration strategy will, because of similar bottom-up origins, simultaneously experience more cybersickness and less sense of presence, leading to a shift in their strategies. Moreover, it is possible that the symptoms of cybersickness and the process of sensory re-weighting compensatory mechanisms drain some of the individual’s limited attentional resources (Navon and Gopher, 1979; Gresty and Golding, 2009), known to participate to the emergence and maintenance of the sense of presence in VR (Draper and Blair, 1996; Draper et al., 1998; Bystrom et al., 1999). Of course, it is likely that all these interpretations are interacting, especially when we consider the close relationship between the sense of presence and cybersickness. Indeed, it seems relevant to consider them together since they are both based on similar processes of multisensory integration and are largely intermingled. For example, the negative and distracting effects of cybersickness could reduce attention to the virtual environment, leading to breaks in presence. Due to this, we advocate an integrative approach to the subjective experience of virtual reality as a one-dimensional construct based on the two main aspects of VR psychophysiology: sense of presence and cybersickness. In connection with this, we highlight the use of clustering techniques, which allow us to compare the two groups of participants with different subjective experiences of VR without having to artificially deteriorate the quality of the immersion of one of the experimental groups.
It is particularly interesting to note that while the FDI level pre-immersion is not statistically different between the two groups of participants based on their subjective experience of VR, it is still a significant predictor of the FDI flexibility. In other words, the more FD an individual initially is, the more likely he or she is to reduce his or her FD after a virtual immersion. Notwithstanding, and in accordance with Maneuvrier et al. (2020), there was no association in the present study between FDI pre-immersion and either sense of presence or cybersickness. This seems to corroborate the fact that FDI pre-immersion alone, as assessed by a one-time measure on the RFT, is not a direct and pertinent predictor of the way an individual will experience virtual immersion. The FDI flexibility is outlined here as the real manifestation of the quality of subjective experience of VR, which itself can be partially predicted by FDI pre-immersion. Different interpretations can be proposed on this point. First, FD individuals are said to make greater use of visual cues, usually responsible for sensory incoherence in VR (LaViola, 2000; Bos et al., 2008; Shafer et al., 2017), which would lead to the appearance of cybersickness and the use of sensory re-weighting mechanisms. In addition, these individuals generally have poor inhibition abilities and rely less on internal representations (Pithers, 2002; Hecht and Reiner, 2007; Evans et al., 2013), which might incline them toward a greater susceptibility to cybersickness and a lower susceptibility to the sense of presence. Still, an apparent paradox remains: if FD individuals are more likely to shift toward a more FI perceptive style (because they are more sensitive to cybersickness and less prone to the sense of presence), one may wonder why FDI pre-immersion does not predict the subjective experience of VR. A possible explanation resides in the temporal dimension of these processes. Since the variables constituting the VR experience were measured a posteriori, it is very likely that sensory re-weighting mechanisms had already impacted both of them, enhancing the sense of presence and reducing cybersickness. This could explain why we found no association between FDI pre-immersion and the VR experience: the observable association was likely removed by the impact of sensory re-weighting mechanisms, leaving the FDI flexibility as the only observable manifestation.
Here, one could rightfully argue that if the FDI flexibility is the manifestation of sensory re-weighting mechanisms improving the subjective experience of VR, then the association should, in fine, be positive: the more flexible an individual is, the more their re-weighting mechanisms will be able to positively impact the subjective experience of VR. However, our results clearly indicated the opposite. A simple but rational explanation would be that the absence of sensory re-weighting reveals an a priori adapted sensory integration strategy which does not require to be updated and is most commonly found among FI individuals (Nori et al., 2021). It is particularly complex to investigate these relationships and their temporal dimension when both cybersickness and sense of presence are measured a posteriori. Thus, it is necessary to dynamically assess these variables during (and after) the experimental virtual immersion in order to further explore the relationships between the level of FDI pre-immersion, the FDI flexibility and the subjective experience of VR. In addition, the inertia inherent to motion sickness symptoms should probably be considered: even if a participant manages to quickly shift to a more adapted perceptive style upon the appearance of negative effects, he or she might still be impacted by the pervasiveness of cybersickness which might last for a long time (Rebenitsch and Owen, 2016). In addition, other studies are required to further explore the FDI flexibility: how long does it take to induce the re-weighting, how long does it last and what is its long-term role in the habituation to VR exposure. Recent development using automation and notably machine learning might be of help for future research as the measures of temporal dimensions are facilitated by these tools (Ochs et al., 2018). In the same vein, the use of continuous postural cues (Pavlou et al., 2011; Weech et al., 2020) or standard longitudinal studies could provide insights into the long-term impact of the individual’s flexibility of perceptive style.
Conclusion
The main result of this study is that FDI is not a stable construct and that its flexibility when exposed to virtual immersion is not systematic but differ from one individual to another. Indeed, only the participants reporting a lower subjective experience of VR manifested a significant FD diminution after immersion, which is discussed in this study as a sensory re-weighting compensatory mechanism. This FD diminution has also been showed as associated with the level of FDI pre-immersion, with FD individuals being more prone to the adaptive shift not necessary because they are more flexible, but rather because their visual sensory preference is less adapted. Considering this, we propose to integrate FDI and more precisely the FDI flexibility into the central human factors of VR. Indeed, they are highlighted in this study as playing a decisive role in the psychophysiological understanding of interindividual differences in VR. Moreover, if some markers leading to the FDI flexibility could be outlined, these results and discussions could help predicting the subjective experience of VR. These findings might help advance the discussion in the two fields of interest: on the one hand in the field of perception, by providing a better understanding of the connection between perceptive style and sensory integration, and on the other hand in the field of VR, not only by providing a new rigorous method of investigating the subjective experience of VR but also by giving insights from sensory integration in order to further explore the psychophysiology of VR.
Data Availability Statement
The raw data supporting the conclusions of this article will be made available by the authors, without undue reservation.
Ethics Statement
The studies involving human participants were reviewed and approved by Comité local d'Ethique de la Recherche en Santé (#CEREP-19-011-PD). The patients/participants provided their written informed consent to participate in this study.
Author Contributions
AM and PR designed the experiment. AM reviewed the literature, wrote the manuscript, programmed the virtual environment, did the participants recruitment, data gathering, and preliminary treatment. AM, LMD, and HC discussed the empirical results. AM and HC wrote the first draft. AM, LMD, GC, HC wrote the manuscript. All the authors reviewed the final manuscript.
Funding
This study was not funded by any private or public institution.
Conflict of Interest
The authors declare that the research was conducted in the absence of any commercial or financial relationships that could be construed as a potential conflict of interest.
Publisher’s Note
All claims expressed in this article are solely those of the authors and do not necessarily represent those of their affiliated organizations, or those of the publisher, the editors, and the reviewers. Any product that may be evaluated in this article, or claim that may be made by its manufacturer, is not guaranteed or endorsed by the publisher.
Acknowledgments
The authors would like to thank Hannes Westermann for proofreading the article and the Plan de Rome project for allowing us to use some 3D models in the creation of the virtual environment.
Supplementary Material
The Supplementary Material for this article can be found online at: https://www.frontiersin.org/articles/10.3389/frvir.2021.706712/full#supplementary-material
References
Andersen, R. A., and Buneo, C. A. (2003). Sensorimotor Integration in Posterior Parietal Cortex. Adv. Neurol. 93, 159–177. doi:10.1146/annurev.neuro.25.112701.142922
Biocca, F., Kim, J., and Choi, Y. (2001). Visual Touch in Virtual Environments: An Exploratory Study of Presence, Multimodal Interfaces, and Cross-Modal Sensory Illusions. Presence: Teleoperators Virtual Environ. 10 (3), 247–265. doi:10.1162/105474601300343595
Bos, J. E., Bles, W., and Groen, E. L. (2008). A Theory on Visually Induced Motion Sickness. Displays 29, 47–57. doi:10.1016/j.displa.2007.09.002
Bouchard, S., Robillard, G., and Renaud, P. (2007). Revising the Factor Structure of the Simulator Sickness Questionnaire. Acte de Colloque Du Annu. Rev. CyberTherapy Telemed. 5, 117–122.
Bray, A., Subanandan, A., Isableu, B., Ohlmann, T., Golding, J. F., and Gresty, M. A. (2004). We Are Most Aware of Our Place in the World when about to Fall. Curr. Biol. 14 (15), R609–R610. doi:10.1016/j.cub.2004.07.040
Bystrom, K.-E., Barfield, W., and Hendrix, C. (1999). A Conceptual Model of the Sense of Presence in Virtual Environments. Presence: Teleoperators Virtual Environ. 8 (2), 241–244. doi:10.1162/105474699566107
Cian, C., Ohlmann, T., Ceyte, H., Gresty, M. A., and Golding, J. F. (2011). Off Vertical axis Rotation Motion Sickness and Field Dependence. Aviat Space Environ. Med. 82 (10), 959–963. doi:10.3357/asem.3049.2011
Clifton, J., and Palmisano, S. (2019). Effects of Steering Locomotion and Teleporting on Cybersickness and Presence in HMD-Based Virtual Reality. Virtual Reality 24, 453–468. doi:10.1007/s10055-019-00407-8
Draper, J. V., and Blair, L. M. (1996). “Workload, Flow, and Telepresence during Teleoperation,” in Proceedings of IEEE International Conference on Robotics and Automation, Minneapolis, Minnesota, April 22–28, 1996. doi:10.1109/ROBOT.1996.506844
Draper, J. V., Kaber, D. B., and Usher, J. M. (1998). Telepresence. Hum. Factors 40, 354–375. doi:10.1518/001872098779591386
Evans, C., Richardson, J. T. E., and Waring, M. (2013). Field independence: Reviewing the Evidence. Br. J. Educ. Psychol. 83 (Pt 2), 210–224. doi:10.1111/bjep.12015
Fulvio, J. M., Ji, M., and Rokers, B. (2021). Variations in Visual Sensitivity Predict Motion Sickness in Virtual Reality. Entertain. Comput. 38, 100423. doi:10.1016/j.entcom.2021.100423
Grassini, S., Laumann, K., and Rasmussen Skogstad, M. (2020). The Use of Virtual Reality Alone Does Not Promote Training Performance (But Sense of Presence Does). Front. Psychol. 11, 1743. doi:10.3389/fpsyg.2020.01743
Gresty, M. A., and Golding, J. F. (2009). Impact of Vertigo and Spatial Disorientation on Concurrent Cognitive Tasks. Ann. N.Y Acad. Sci. 1164, 263–267. doi:10.1111/j.1749-6632.2008.03744.x
Gutierrez-Maldonado, J., Gutierrez-Martinez, O., Loreto, D., Penaloza, C., and Nieto, R. (2010). “Presence, Involvement and Efficacy of a Virtual Reality Intervention on Pain,” in Annual Review of Cybertherapy and Telemedicine 2010: Advanced Technologies in Behavioral, Social and Neurosciences. Editors B. K. Wiederhold, G. Riva, and S. I. Kim (Amsterdam: Ios Press), 154, 97–101.
Hecht, D., and Reiner, M. (2007). Field Dependency and the Sense of Object-Presence in Haptic Virtual Environments. CyberPsychology Behav. 10 (2), 243–251. doi:10.1089/cpb.2006.9962
Heeter, C. (1992). Being There: The Subjective Experience of Presence. Presence: Teleoperators Virtual Environ. 1 (2), 262–271. doi:10.1162/pres.1992.1.2.262
Hoffman, H. G., Sharar, S. R., Coda, B., Everett, J. J., Ciol, M., Richards, T., et al. (2004). Manipulating Presence Influences the Magnitude of Virtual Reality Analgesia. Pain 111 (1–2), 162–168. doi:10.1016/j.pain.2004.06.013
Kennedy, R. S. (1975). Motion Sickness Questionnaire and Field independence Scores as Predictors of success in Naval Aviation Training. Aviat Space Environ. Med. 46 (11), 1349–1352.
LaViola, J. J. (2000). A Discussion of Cybersickness in Virtual Environments. SIGCHI Bull. 32 (1), 47–56. doi:10.1145/333329.333344
Mahboobin, A., Loughlin, P. J., Redfern, M. S., and Sparto, P. J. (2005). Sensory Re-weighting in Human Postural Control during Moving-Scene Perturbations. Exp. Brain Res. 167 (2), 260–267. doi:10.1007/s00221-005-0053-7
Maneuvrier, A., Decker, L. M., Ceyte, H., Fleury, P., and Renaud, P. (2020). Presence Promotes Performance on a Virtual Spatial Cognition Task: Impact of Human Factors on Virtual Reality Assessment. Front. Virtual Real. 1. doi:10.3389/frvir.2020.571713
Medendorp, W. P., Alberts, B. B. G. T., Verhagen, W. I. M., Koppen, M., and Selen, L. P. J. (2018). Psychophysical Evaluation of Sensory Reweighting in Bilateral Vestibulopathy. Front. Neurol. 9, 377. doi:10.3389/fneur.2018.00377
Messick, S. (1976). “Individuality in Learning,” in Personality Consistencies in Cognition and Creativity. Editor S. Messick (San Francisco: Jossey-Bass), 4–23.
Navon, D., and Gopher, D. (1979). On the Economy of the Human-Processing System. Psychol. Rev. 86 (3), 214–255. doi:10.1037/0033-295X.86.3.214
Nori, R., Boccia, M., Palmiero, M., and Piccardi, L. (2021). The Contribution of Field independence in Virtual Spatial Updating. Curr. Psychol. doi:10.1007/s12144-021-01788-3
Ochs, M., Jain, S., and Blache, P. (2018). “Toward an Automatic Prediction of the Sense of Presence in Virtual Reality Environment,” in Proceedings of the 6th International Conference on Human-Agent Interaction, Southampton, United Kingdom, December 4, 2018, 161–166. doi:10.1145/3284432.3284452
Oman, C. M., and Cullen, K. E. (2014). Brainstem Processing of Vestibular Sensory Exafference: Implications for Motion Sickness Etiology. Exp. Brain Res. 232 (8), 2483–2492. doi:10.1007/s00221-014-3973-2
Oman, C. M. (1990). Motion Sickness: A Synthesis and Evaluation of the Sensory Conflict Theory. Can. J. Physiol. Pharmacol. 68 (2), 294–303. doi:10.1139/y90-044
Pavlou, M., Quinn, C., Murray, K., Spyridakou, C., Faldon, M., and Bronstein, A. M. (2011). The Effect of Repeated Visual Motion Stimuli on Visual Dependence and Postural Control in normal Subjects. Gait Posture 33 (1), 113–118. doi:10.1016/j.gaitpost.2010.10.085
Piccione, J., Collett, J., and De Foe, A. (2019). Virtual Skills Training: The Role of Presence and agency. Heliyon 5 (11), e02583. doi:10.1016/j.heliyon.2019.e02583
Pithers, R. T. (2002). Cognitive Learning Style: A Review of the Field Dependent-Field Independent Approach. J. Vocational Educ. Train. 54 (1), 117–132. doi:10.1080/13636820200200191
Pritchard, S. C., Zopf, R., Polito, V., Kaplan, D. M., and Williams, M. A. (2016). Non-hierarchical Influence of Visual Form, Touch, and Position Cues on Embodiment, Agency, and Presence in Virtual Reality. Front. Psychol. 7, 1649. doi:10.3389/fpsyg.2016.01649
Rebenitsch, L., and Owen, C. (2016). Review on Cybersickness in Applications and Visual Displays. Virtual Reality 20 (2), 101–125. doi:10.1007/s10055-016-0285-9
Robillard, G., Bouchard, S., Renaud, P., and Cournoyer, L.-G. (2002). “Validation canadienne-française de deux mesures importantes en réalité virtuelle: L’Immersive Tendencies Questionnaire et le Presence Questionnaire,” in Poster presented at the 25ième congrès de la Société Québécoise pour la Recherche en Psychologie (SQRP), Trois Rivères, Québec, Canada, November 1–3, 2002.
Rus-Calafell, M., Gutiérrez-Maldonado, J., and Ribas-Sabaté, J. (2013). Neurocognition, Presence and Acceptance of a VR Programme for Psychotic Patients: A Correlational Study. Stud. Health Technol. Inform. 191, 141–145.
Scotto Di Cesare, C., Macaluso, T., Mestre, D. R., and Bringoux, L. (2015). Slow Changing Postural Cues Cancel Visual Field Dependence on Self-Tilt Detection. Gait Posture 41 (1), 198–202. doi:10.1016/j.gaitpost.2014.09.027
Shafer, D.-M., Carbonara, C.-P., and Kropi, M.-F. (2017). Modern Virtual Reality Technology: Cybersickness, Sense of Presence, and Gender. Media Psychol. Rev. 11 (2).
Sheridan, T. B. (1992). Musings on Telepresence and Virtual Presence. Presence: Teleoperators Virtual Environ. 1 (1), 120–126. doi:10.1162/pres.1992.1.1.120
Slater, M., and Steed, A. (2000). A Virtual Presence Counter. Presence: Teleoperators Virtual Environ. 9 (5), 413–434. doi:10.1162/105474600566925
Slater, M. (2018). Immersion and the Illusion of Presence in Virtual Reality. Br. J. Psychol. 109 (3), 431–433. doi:10.1111/bjop.12305
Stoffregen, T. A., and Smart, L. J. (1998). Postural Instability Precedes Motion Sickness. Brain Res. Bull. 47 (5), 437–448. doi:10.1016/S0361-9230(98)00102-6
Tinajero, C., and Páramo, M. F. (1998). Field Dependence-independence Cognitive Style and Academic Achievement: A Review of Research and Theory. Eur. J. Psychol. Educ. 13 (2), 227–251. doi:10.1007/bf03173091
Wallach, H. S., Safir, M. P., Samana, R., Almog, I., and Horef, R. (2011). “How Can Presence in Psychotherapy Employing VR Be Increased? Chapter for Inclusion in: Systems in Health Care Using Agents and Virtual Reality,” in Advanced Computational Intelligence Paradigms in Healthcare 6. Virtual Reality in Psychotherapy, Rehabilitation, and Assessment. Editors S. Brahnam, and L. C. Jain (Berlin, Heidelberg: Springer Berlin Heidelberg), 129–147. doi:10.1007/978-3-642-17824-5_7
Weech, S., Varghese, J. P., and Barnett-Cowan, M. (2018). Estimating the Sensorimotor Components of Cybersickness. J. Neurophysiol. 120 (5), 2201–2217. doi:10.1152/jn.00477.2018
Weech, S., Kenny, S., and Barnett-Cowan, M. (2019). Presence and Cybersickness in Virtual Reality Are Negatively Related: A Review. Front. Psychol. 10, 158. doi:10.3389/fpsyg.2019.00158
Weech, S., Calderon, C. M., and Barnett-Cowan, M. (2020). Sensory Down-Weighting in Visual-Postural Coupling Is Linked with Lower Cybersickness. Front. Virtual Real. 1. doi:10.3389/frvir.2020.00010
Wirth, W., Hartmann, T., Böcking, S., Vorderer, P., Klimmt, C., Schramm, H., et al. (2007). A Process Model of the Formation of Spatial Presence Experiences. Media Psychol. 9 (3), 493–525. doi:10.1080/15213260701283079
Witkin, H. A., and Asch, S. E. (1948). Studies in Space Orientation. IV. Further Experiments on Perception of the Upright with Displaced Visual fields. J. Exp. Psychol. 38 (6), 762–782. doi:10.1037/h0053671
Witkin, H.-A., Dyk, R. B., Fattuson, H. F., Goodenough, D. R., and Karp, S. A. (1962). Psychological Differentiation: Studies of Development. Menlo Park, CA: Wiley, xii–418.
Witkin, H.-A., Oltman, P.-K., Raskin, E., and Karp, S.-A. (1971). A Manual for the Group Embedded Figures TEst. Oxford, England: Mind Garden, Inc.
World Medical Association (2013). World Medical Association Declaration of Helsinki. JAMA 310 (20), 2191–2194. doi:10.1001/jama.2013.281053
Keywords: sensory re-weighting, virtual reality, cybersickness, sense of presence, field dependence, perceptive style, flexibility, multisensory integration
Citation: Maneuvrier A, Decker LM, Renaud P, Ceyte G and Ceyte H (2021) Field (In)dependence Flexibility Following a Virtual Immersion Is Associated With Cybersickness and Sense of Presence. Front. Virtual Real. 2:706712. doi: 10.3389/frvir.2021.706712
Received: 07 May 2021; Accepted: 12 August 2021;
Published: 28 September 2021.
Edited by:
Regis Kopper, University of North Carolina at Greensboro, United StatesCopyright © 2021 Maneuvrier, Decker, Renaud, Ceyte and Ceyte. This is an open-access article distributed under the terms of the Creative Commons Attribution License (CC BY). The use, distribution or reproduction in other forums is permitted, provided the original author(s) and the copyright owner(s) are credited and that the original publication in this journal is cited, in accordance with accepted academic practice. No use, distribution or reproduction is permitted which does not comply with these terms.
*Correspondence: A. Maneuvrier, YXJ0aHVyLm1hbmV1dnJpZXJAcHJvdG9ubWFpbC5jb20=