- 1Open Innovation Laboratory in Health Technologies (LIO), Ecole de Technologie Supérieure (ETS), Montreal, QC, Canada
- 2CHUM Research Center, Université de Montréal, Montreal, QC, Canada
- 3Laboratoire de Pathokinésiologie de Montréal, Institut Universitaire sur la Réadaptation en Déficience Physique de Montréal-Centre de Recherche Interdisciplinaire en Réadaptation du Montréal Métropolitain, Université de Montréal, Montreal, QC, Canada
In immersive VR, a self-avatar that replicates the user’s movements and is viewed from a first-person perspective can substitute the real body. If the avatar’s movements are sufficiently synchronized with the user’s actual movements, the user can experience a sense of embodiment over the avatar. Recent studies have shown that discrepancies between the movements of the avatar and those of the user can be well tolerated while maintaining high levels of embodiment. The point at which a distortion is perceived (detection threshold) and its impact on the level of embodiment has not been studied in lower limb tasks such as gait. This study aimed to identify a detection threshold of gait asymmetry by unilaterally manipulating the step length of a self-avatar, and the effect of this detection on perceived embodiment. A real-time step length distortion model was developed, and a detection threshold between actual and avatar’s gait movement was assessed on thirty healthy participants. The step length was manipulated to introduce gait asymmetry (ascending condition) or start from a large asymmetry that was gradually decreased (descending). The results showed that, on average, the avatar’s step length could be increased by up to 12% before the participants detected the distortion. Furthermore, in the descending condition, they detected increases that were above 9%. The point of detection had no effect on the sense of embodiment as participants still reported being embodied in their avatars, even when they consciously detected the step length distortion. The sense of embodiment was closely correlated with the level of distortion; as distortion increased, embodiment decreased, and vice versa. For a given distortion level, embodiment was similar whether in the ascending or descending condition. This suggests that embodiment can be achieved even when the avatar’s spatial alignment initially differs from the participants’, provided that alignment is gradually restored. These results provide valuable insights into participants’ ability to tolerate movement discrepancies in embodied avatar experiences during gait in virtual environments, with potential applications in motor training and gait rehabilitation.
1 Introduction
In immersive virtual reality (VR), a self-avatar that mimics the user’s movements and is viewed from a first-person perspective (1 PP) can act as a substitute for the user’s real body, which is not visible from within the virtual environment (VE). When the avatar’s movements are accurately synchronized with the user’s own movements, it can create a sense of embodiment (SoE) over the avatar (Spanlang et al., 2014). This SoE is commonly recognized to consist of three primary components: body ownership, agency, and self-location (Kilteni and Slater, 2012). Body ownership refers to the attribution of the virtual body to oneself. The sense of agency pertains to the feeling of having global motor control, where one can initiate and control the movements of the virtual body. Self-location refers to the perception of being located within the virtual body.
Extensive research has demonstrated that embodiment in VR is robust and can produce a particularly convincing illusion (Slater et al., 2008; Banakou et al., 2013; Kokkinara et al., 2016). The congruence between the user’s movements and their avatar’s movements is considered a critical factor in generating and sustaining the SoE (Kilteni et al., 2015). Congruent visuotactile stimulations (where users simultaneously experience tactile sensations and observe their avatar’s corresponding body parts being touched) can also induce embodiment, but their contribution to the overall illusion is lesser in comparison to visuomotor stimulations (Kokkinara and Slater, 2014). Conversely, visuomotor and visuotactile stimuli that are incongruent, at any point during the experience, have both been shown to equally lead to a loss of the illusion, in what Kokkinara and Slater (2014) called a break in the SoE.
Nevertheless, it has been shown that self-avatar movements that are spatially distorted with respect to those performed by the user can still be accepted and not break the SoE (Bourdin et al., 2019; Burin et al., 2019; Porssut et al., 2019; Cohn et al., 2020; Gonzalez-Franco et al., 2020). For instance, Kokkinara et al., 2015 found that participants accepted a spatial distortion in the form of 11° and 22° angular offsets, applied to the shoulder, during a reaching movement with the arm fully extended. Notably, this distortion did not impact body ownership or agency, as perceived by the participants. However, when spatiotemporal distortions were applied (increased velocities of 2x and 4x), they were consciously detected, which diminished the reported level of agency but not the level of ownership.
Porssut et al. (2019) conducted a study exploring various levels of spatial distortions between real and avatar hand positions while participants were instructed to follow a target with their hand. They observed that ownership and agency scores decreased as the magnitude of distortion increased. Despite the decrease in the level of embodiment, participants still reported feeling a SoE in their avatars, even when consciously aware of the spatial mismatch. They employed an approach that differed from that of Kokkinara and Slater (2014) to compute embodiment scores which were based on the frequency of reported breaks in the SoE. They identified a critical threshold of 60%, beyond which a significant distortion ultimately broke the SoE. Notably, this threshold can be extended if the distortion is intentionally designed to assist in achieving a particularly challenging task (Galvan Debarba et al., 2018; Porssut et al., 2019; 2022; Delahaye et al., 2023). Conversely, factors such as incongruent haptic feedback in the form of a spatial mismatch during self-contact significantly decreases body ownership and agency (Bovet et al., 2018). Additionally, a discrepancy between internal proprioceptive feedback and the feedback that would be expected given the avatar’s position (such as the realization of a mismatch between one’s own arm reaching full extension before the avatar’s arm does) can also disrupt embodiment (Porssut et al., 2022).
In addition, the manner in which the distortion is applied has an impact on its level of acceptance. Instantaneous distortions are generally poorly tolerated and diminish embodiment due to the abrupt introduction of sensory conflicts (Porssut et al., 2019; Cohn et al., 2020; Gonzalez-Franco et al., 2020) Conversely, gradually applied distortions can increase the detection threshold and enhance acceptance (Porssut et al., 2019). Instantaneous distortions cause a significant change in the avatar’s movements, negatively affecting embodiment, yet they do not result in a complete break in embodiment as long as visuomotor synchronization between the avatar and real movements is maintained. In fact, when these abrupt distortions are suddenly removed, thereby restoring visuomotor congruency, embodiment begins to rebuild even in the case of semantic violations (Padrao et al., 2016; Cohn et al., 2020; Gonzalez-Franco et al., 2020). It remains unclear whether, once the SoE has been broken, it can also be regained by gradually decreasing the magnitude of distortion to bring it back below the threshold rather than reverting to perfect congruence (no distortion) and having to gradually re-increase the distortion. Previous studies have identified a detection threshold through adaptive staircase methods for spatiotemporal distortions during hand-reaching movements, gradually reducing the magnitude until this threshold was reached (Bovet et al., 2018; Galvan Debarba et al., 2018). Although these studies demonstrated that a large distortion exceeding the detection threshold can go unnoticed again when its intensity is decreased, they did not evaluate the impact of the adaptive staircase method on the SoE.
When users perceive the movements of their self-avatar as being their own and are not aware of applied distortions, this allows experimenters or clinicians to act upon the perception-action loop by modifying the visual feedback observed in response to motor commands. This could be exploited by intentionally amplifying errors made by individuals during their motor tasks or exercises. By emphasizing and accentuating errors, individuals become more aware of their movement discrepancies and are more likely to engage in cognitive processing to correct those errors (Reisman et al., 2013; Janeh et al., 2019). Conversely, the self-avatar can also be programmed to perform a distorted movement that is closer to the intended movement than the one that a patient produces. Indeed, when users are embodied in their self-avatars onto which spatial distortions are applied, they tend to follow their avatar’s movements by unconsciously adjusting their movements to match those of their avatar in order to reduce the discrepancy between what is seen (visual information) and what is felt (proprioceptive feedback) (Bourdin et al., 2019; Burin et al., 2019; Cohn et al., 2020; Gonzalez-Franco et al., 2020; Berger et al., 2022). This phenomenon, recently coined by Gonzalez-Franco et al. (2020), as the “self-avatar follower effect,” has possible implications for motor rehabilitation.
One potential application of applying such manipulations to the movements of an embodied self-avatar is for the rehabilitation of patients who suffer from gait asymmetries, which are commonly observed in a neurological population (Patterson et al., 2008). Spatiotemporal distortions could be applied to alter the asymmetry of the avatar’s gait in order to drive unconscious corrections (Willaert et al., 2020). If embodiment is maintained, patients may perceive the distorted avatar movements as their own, which could implicitly affect their movements to alter their gait and potentially help reduce gait asymmetries. For such applications of embodied self-avatars, it is important to have a good understanding of the extent to which an avatar’s movements can be distorted without conscious detection by its user and without causing a break in embodiment.
The relationship between movement distortions and embodiment has primarily been explored in the context of upper limb movements, such as reaching tasks. Research involving lower limb movements is relatively limited, though functional demands and sensory properties differ between the upper and lower limbs (Hajnal et al., 2007). For instance, upper limb movements are involved in executing voluntary movements and exhibit fine motor control, while the lower limbs have a smaller representation in the somatosensory cortex and are mostly involved in locomotion. The detection of discrepancies between apparent (avatar) and actual performed movements (participants) may therefore differ between the upper and lower limbs. This is particularly true in the case of gait, which is a complex rhythmic motion driven by the nervous system’s ability to coordinate movement with minimal conscious effort (Paul et al., 2005; Malone and Bastian, 2010) and is usually less visually guided, unlike reaching movements that require more deliberate motor planning and execution (Miall and Wolpert, 1996).
This study aims to better understand the relationship between the amplitude of spatial distortions applied to a self-avatar’s gait, their conscious detection, and the ensuing SoE. This understanding is necessary for applications in gait rehabilitation seeking to address asymmetry by manipulating one limb of the self-avatar. We employed a virtual self-avatar whose step length was unilaterally increased, thereby producing an asymmetrical gait pattern. The step length distortions were gradually applied and the direction of the distortion was systematically altered over time. The first objective was to establish the threshold for detecting distortions (specifically, step length asymmetry) below which users do not perceive the disparities between the gait of their avatar and their actual gait. The second objective sought to characterize the relation between this detection threshold and users’ individual levels of subjective SoE. Lastly, a tertiary objective was to investigate whether diminishing the amplitude of a detected step length distortion could restore the sense of embodiment.
We applied step length distortion to both the dominant and non-dominant legs, as prior research has indicated that limb differences exist during able-bodied walking, which may be attributed to limb dominance (Sadeghi et al., 2000). Moreover, proprioceptive acuity has been found to vary between the dominant and non-dominant lower limbs (Han et al., 2013). Furthermore, there’s evidence suggesting that proprioceptive drift, where one’s sense of the location of their body parts shifts towards an external stimulus, is less pronounced in the dominant hand compared to the non-dominant hand. This is likely because proprioceptive information associated with the dominant limb is more stable, leading to a decreased drift (Dempsey-Jones and Kritikos, 2019). These findings suggest that limb dominance can impact both motor control and perception, implying that the dominant and non-dominant lower limbs may respond differently to the step length distortion, which may impact the detection threshold. Therefore, this study also aims to explore how limb dominance influences the ability to detect spatial distortions and its impact on the sense of embodiment.
Our initial hypotheses posited that embodied users can detect unilateral step length distortions of the self-avatar (H1). Given prior findings suggesting that users can eventually detect significant avatar distortion during upper movements (Galvan Debarba et al., 2018; Porssut et al., 2019; 2022), we anticipate that participants will similarly detect the distortions during gait, as these gradually increasing step length distortions eventually present an asymmetry that deviates from their typical symmetrical gait. Moreover, we hypothesize that the detection of these distortions will lead to a disruption in the SoE (H2). As the distortions gradually deviate from participants’ natural gait patterns, the conscious awareness that the avatar no longer replicates their body movements is likely to have a negative impact on their SoE. Lastly, when the step length distortion is reversed and gradually decreases, we hypothesize that the SoE will remain consistent at a given distortion level, regardless of whether the distortion is increasing or decreasing (H3). Since the congruence between the user’s movements and the avatar’s movements enhances the SoE (Kilteni and Slater, 2012), it is plausible that the SoE can be restored as the incongruences diminish. In other words, we expect that the decreasing distortion will positively impact the SoE, indicating that the level of SoE is influenced by the magnitude of the distortion rather than its direction.
The contributions of this paper are threefold: (i) Defining a detection threshold for gait distortions in a self-avatar with unilaterally increased step length during healthy gait; (ii) exploring the impact of the detection threshold on SoE and its interaction with limb dominance; (iii) examining the restoration of the SoE through reduction of large, detected distortions.
2 Methodology
2.1 Participants
Thirty-five healthy participants volunteered to take part in this study. Inclusion criteria were to have no pathological, medical or lower limb conditions that could affect gait. Ethical approval was obtained (CE15.104) through the Research Ethics Boards of Centre hospitalier de l’Université de Montreal (CHUM) and of Ecole de technologie supérieure (ETS). All participants signed written consent and completed a demographic questionnaire before the start of the experiment. Participants were compensated $40 CAD for their participation.
2.2 Virtual environment and avatar
The VE was developed with Unity 3D game engine version 2019.3.13f1 (Unity 3D) and was a replica of our research lab with a large virtual mirror placed 1.0 m in front of the virtual treadmill. The dimensions of the virtual treadmill and the safety railings correspond with that of the real world to ensure safe walking in the VE (Figure 1A). The VE was visualized through a head-mounted display (HMD), HTC VIVE Pro Eye (1,440 × 1,600 pixels per eye, 90 Hz refresh rate, 110° field of view) with a first-person perspective (1 PP) relative to the avatar (Figure 1B). The HMD was connected to a desktop PC with an 8 GB Nvidia GeForce RTX 3070 graphic card, 64 GB of memory and 1 TB SSD. A female and male avatar were designed in MakeHuman 1.20 (MakeHuman) and were individually scaled to match the height and weight of each participant, ensuring an anthropometrically personalized representation. Further customization was performed based on the participants’ gender and skin color to increase avatar resemblance. The female avatar wore a white T-shirt, and the male avatar wore a grey T-shirt. Both avatars wore blue shorts and black sneakers (Figures 1A, B). This standardized clothing choice aimed to maintain consistency across participants and minimize potential biases introduced by varying clothing.
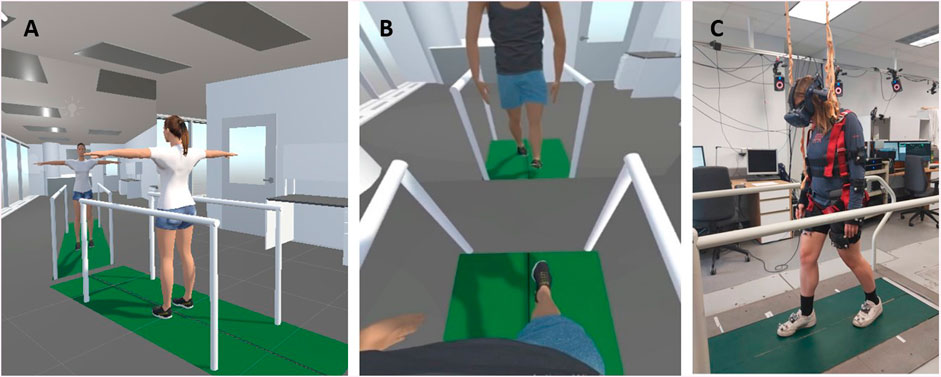
Figure 1. Experimental setup. (A) The VE, female avatar and virtual treadmill and virtual mirror, (B) A male participant’s avatar walking in the VE in front of the virtual mirror, viewed in 1 PP, (C) A participant wearing the mocap clusters, the HMD and a safety harness.
2.3 Equipment and software
The experiment was performed on a split-belt treadmill (Advanced Mechanical Technology Inc., 187 Waterdown, MA, United States), which has two belts that can be controlled separately, allowing for different speeds or directions of movement. In our experiment, the speeds of both belts were identical throughout. During the experiment, participants were fitted with a safety harness to support their weight in the event of a fall due to loss of balance while walking on the treadmill. They were further equipped with 14 rigid body marker clusters placed with Velcro straps on specific body parts (toes, feet, upper legs, pelvis, hands, elbows, upper arms, and between the shoulders) (Figure 1C). The marker clusters were tracked in real-time by 12 Vicon T20-S cameras (Vicon Motion Systems Ltd., Oxford, United Kingdom) running the Vicon Tracker 3.6 software. Vicon Pegasus Advanced 1.2.2 (Vicon Motion Systems Ltd., Oxford, United Kingdom) retargeted the limb positions and orientations onto the virtual avatar and displayed them in the VE in real-time. Vicon software (Tracker and Pegasus) was run on a separate desktop PC equipped with a 4 GB Nvidia Quadro M2000 graphic card and 32 GB of memory, and a 500 GB SSD.
2.4 Real-time step length distortion model
An algorithm was developed to dynamically alter one step length of the avatar in real-time, inducing gait asymmetry. The avatar’s step length is modified by gradually introducing a spatial offset between the avatar’s ankle and the user’s actual ankle position along the anteroposterior (AP) axis of the tibia (Figure 2). The spatial offset linearly increases starting at mid-swing, identified as the moment the ankle is directly under the hip joint, during the forward swing of the gait cycle (Figure 2A). The spatial offset gradually causes the avatar’s ankle to advance along the AP axis of the tibia until heel strike. Conversely, the offset starts decreasing at heel strike (Figure 2B), crossing into a negative offset at midstance that increases until heel off (Figure 2C). At this point, the negative offset is gradually decreased, reaching a null offset at mid-swing. The ratio of the positive to negative offset that is applied is equal to the ratio between the most anterior and most posterior positions of the ankle, relative to the hip joint, for each participant.
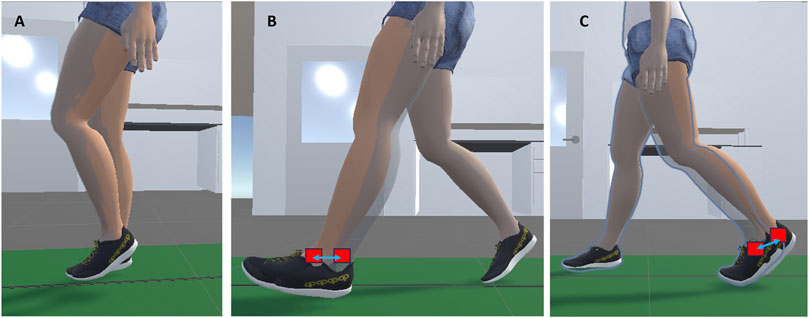
Figure 2. Left step length distortion (lateral view). The translucent avatar represents the participant’s actual leg position, and the opaque avatar illustrates the distorted position of the left lower leg. The blue arrow represents the offset value. Participants were not able to see the translucent avatar or red cubes, and saw their avatars from a 1 PP. (A) The avatar’s left limb reaches mid-swing. The AP offset of the ankle is null and starts increasing linearly along the AP axis of the tibia. (B) The avatar’s left heel contacts the ground (heel-strike). Maximum positive offset of the ankle is reached, and it starts decreasing. (C) The avatar’s left heel loses contact with the ground (heel off). Maximum negative offset of the ankle is reached and it starts decreasing, reaching a null offset at mid-swing (A).
The resulting kinematics of the lower limb of the avatar are computed using inverse kinematics with FinalIK (RootMotion). To preserve the visuotactile synchronization, which has been shown to maintain the SoE (Kokkinara et al., 2015), the avatar’s (distorted) heel strike is always synchronized with the heel strike of the user.
2.5 Experimental setup and protocol
Participants were invited to perform the experiment in one single session, which lasted 3h30. A schematic overview of the protocol can be found in Figure 3. First, dominance was determined by asking participants to kick a foam ball towards the wall. The foot used to kick was deemed the dominant one.
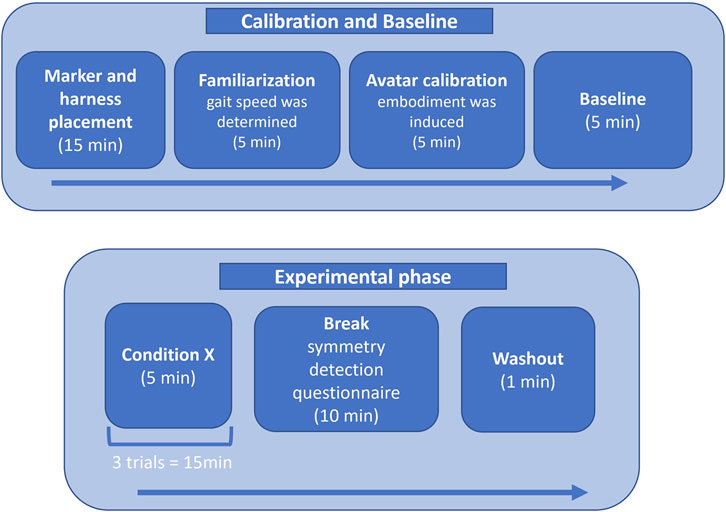
Figure 3. Schematic overview of the protocol. Conditions X represents all four conditions DomAsc, N-DomAsc, DomDesc and N-DomDesc.
2.5.1 Calibration and baseline
The experiment started with a 5-min period of treadmill walking without wearing the HMD or viewing the VE. This was done to familiarize participants with walking on the treadmill, to ensure that the marker clusters had been placed correctly and were not slipping, and to determine their self-selected gait speed. The latter was obtained by slowly increasing the speed of the treadmill until participants indicated that they felt comfortable and were walking at a pace that they considered natural and preferred. Participants were then fitted with the HMD and the avatar was calibrated to collocate with the position of the participants. During this calibration phase, participants were asked to look straight ahead with their feet pointing straight forward and their arm resting along their body. The avatar was not visible, to prevent the participants from observing visual artifacts. Once the calibration phase was completed, participants were asked to move their lower and upper limbs for 1 minute while observing their movements being performed by their avatar, alternating between looking directly down at their virtual body and looking ahead to view their virtual body in the mirror. This was done to induce the feeling of embodiment. This active visuomotor control has been shown to induce the experience of body ownership over a virtual avatar (Kokkinara and Slater, 2014).
A 5-min period of walking while wearing the HMD was then imposed in order to familiarize participants with walking in the VE. For this period and for the entire experiment, participants were instructed to alternate between looking in the mirror and looking down at their virtual body. This was done to ensure that participants saw their avatar’s lower limbs, whose movements were manipulated. They were further instructed not to hold the safety railings while walking and to inform the experimenter immediately if any dizziness, nausea, or any discomfort occurred so that the experiment could be stopped. During the last 2 minutes of this baseline period, the participant’s average step length was calculated.
2.5.2 Experimental phase
The experimental phase of this study was divided into four conditions (Figure 3) during which the detection threshold between the participant’s gait and their avatar’s gait was assessed. The four conditions were randomized across participants and lasted 15 min each. The conditions consisted of two ascending and two descending conditions. In ascending conditions, the step length of the avatar was gradually increased from baseline (no distortion) to a maximum of 30% increase of their baseline step length, in 1% increments at every 10 strides. Conversely, in the descending conditions, the step length of the avatar was initially set to 30% of the baseline and then decreased to baseline in 1% increments every 10 strides. To verify if leg dominance plays a role in the detection, the distortion was applied to both the dominant (DomAsc and DomDesc) and non-dominant legs (N-DomAsc and N-DomDesc).
The selection of the distortion range, ranging from 0% to 30%, was determined by conducting four pilot studies. An increase of to 30% was perceived as a substantial distortion that elicited clearly noticeable changes in the avatar’s gait patterns without inducing an extremely unstable gait that may induce discomfort or instability.
During the initial 10 strides of each condition, no distortion was applied (0%). For every condition, three consecutive trials were executed, which lasted 5 min per trial. The treadmill was not stopped between trials. At the end of each trial, when the maximal distortion level was reached, participants were instructed to keep walking while looking at the avatar’s head in the virtual mirror for 30 s. During this time, the distortion applied to the step length of the avatar was suddenly returned to 0% (ascending conditions) or 30% (descending conditions), and the next trial was initiated.
A 10-min break was given between each condition. Before the start of each condition, a 1-min washout period was imposed to avoid any possible adaptations due to exposure to the step length distortions (Seung-Jae and Hermano Igo, 2012; Lauzière et al., 2014). During the washout period, the step length of the avatar was not distorted and participants were instructed to observe their avatar by looking down at their body and in the mirror while walking.
2.5.2.1 Detection threshold
To evaluate the detection threshold, participants were instructed to pay attention to the gait of their self-avatar and inform the experimenter as soon as it was accurately replicating their own gait (descending conditions) or no longer accurately replicating their gait (ascending conditions). The specific instructions given before the ascending conditions (DomAsc/N-DomAsc) were: “Let us know when you feel that avatar is no longer walking as you are.” This instruction was inverted for the descending series (DomDesc/N-DomDesc): “Let us know when you feel that the avatar is walking as you are.” The detection task was completed while walking, and participants verbally responded with “Now”. The point of detection was recorded during each condition. The step length of the avatar continued to increase or decrease after the reported detection point until the minimum distortion of 0% (DomDesc/N-DomDesc) or the maximum distortion of 30% (DomAsc/N-DomAsc) was reached.
To better understand what visual information participants used to detect the step length distortion, participants were asked to answer the following question in between each condition, during the break (hereinafter referred to as the “Symmetry detection questionnaire”); “What type of information did you use to judge the asymmetry (DomAsc/N-DomAsc)/symmetry (DomDesc/N-DomDesc) between you and your avatar?.” The answers were categorized. Participants could answer from one or more of nine multiple-choice options: trunk flexion, hip flexion, hip extension, heel strike, knee flexion, knee extension, ankle flexion, ankle extension, and other.
2.5.2.2 Sense of embodiment
To assess the subjective feeling of embodiment across different distortion levels in-real time, an additional question was asked at 3% intervals during each condition. This interval was determined to be adequate based on insights gained from four pilot trials. Asking the question at a 1% interval proved too frequent and would potentially divert attention away from observing the avatar’s gait. The participants were asked to rate their agreement with the following statement from 0 to 7: “I feel that the virtual body is my own body,” where 0 indicates strong disagreement and 7 indicates strong agreement. Participants responded to the question verbally while walking. This approach allowed for monitoring of the progression of the SoE throughout the experiment, across different distortions. The embodiment question and detection task were explained prior to the first condition to ensure that participants understood them correctly.
2.6 Data analysis
2.6.1 Detection threshold
The detection threshold of each participant was obtained by averaging the point of detection (distortion %) of the three trials, in each of the four conditions (DomAsc, N-DomAsc, DomDesc, N-DomDesc).
A linear mixed-effects model (LMM) was employed to analyze the data, with dominance (dominant and non-dominant) and direction (ascending and descending) as fixed effects to account for the main effects and interactions. Additionally, these predictors were treated as random intercepts and slopes, accommodating the variability associated with individual observations and accounting for the repeated measures design. The assumption of normality for the residuals was accepted based on the Shapiro-Wilk test (W = 0.98, p = 0.31) and assessing the QQ plots.
In the absence of a significant main or interaction effects for dominance, suggesting that dominance does not significantly affect the detection threshold, the data for the dominant leg and the non-dominant leg conditions were grouped together, resulting in only two conditions: ascending (Asc) and descending (Desc), each comprising six trials in total. To assess if there were significant differences between the detection threshold of both conditions, a paired sample T-test was applied for pairwise comparison analysis.
The coefficient of variation (CV) was calculated to assess inter-subject variability in the detection thresholds. Furthermore, responses from the symmetry detection questionnaire were analyzed by calculating the percentage of participants who selected each possible answer within each category for each condition.
2.6.2 Sense of embodiment
The embodiment scores were calculated by averaging the embodiment scores of the three trials of each condition and for each distortion level (every 3% increment). Then, the group mean for each distortion level was calculated. To evaluate the impact of the point of detection on the sense of embodiment, a separate analysis was conducted around the point of detection of each participant. The mean embodiment was calculated for each participant at five specific points: at the point of detection (D%), detection +3% distortion (D + 3%), detection +6% distortion (D + 6%), detection −3% distortion (D-3%), detection −6% distortion (D-6%).
For both analyses, two distinct linear mixed-effects models (LMMs) were employed, with direction (ascending, descending), dominance, and distortion levels set as fixed effects to investigate their main effects and interactions. To accommodate for individual variability, each model included random intercepts for participants and allowed the influence of direction, dominance, and distortion levels to vary across individuals by incorporating them as random slopes within each subject.
In light of the absence of significant dominance effects and its interactions, data from the dominant and non-dominant leg conditions were then combined. Subsequently, a revised LMM model was then applied, including direction and distortion levels as fixed effects while maintaining these predictors as random effects and slopes within subjects. The Shapiro-Wilk test and QQ plots indicated that the residuals of both models did not meet the assumption of normality. However, LMMs are known for their robustness in such situations (Schielzeth et al., 2020). Pairwise comparisons were conducted using the Tukey HSD method, which corrected the p-values for multiple comparisons.
Lastly, the relationship between the distortion levels and the embodiment score was measured with the Pearson correlation coefficient.
Data processing and analysis were performed using a custom-made program written in MATLAB R2020 (Mathworks, Natick, MA), and statistical analysis was performed in R Core Team (2023) (RStudio, PBC, Boston, MA). The statistical significance level was established at 0.05 for all tests.
3 Results
Thirty-five participants completed the study. Four participants contributed to the pilot testing and were not included in the analysis. One participant’s data was excluded due to technical issues during the experiment. The remaining 30 participants (10 females, 20 males; 26.1 (±4.1) years; 174.6 (±10.2) cm; 71.6 (±12.2) kg; 3 left-footed) were included in the analysis. The average self-selected gait speed of the participants was 1.20 m/s (±0.1).
3.1 Detection threshold
All participants were able to detect the mismatch/match between their own gait and their avatars during all conditions (Figure 4).
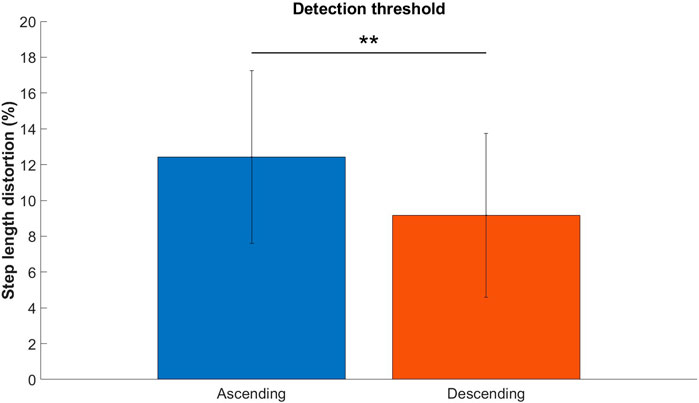
Figure 4. Detection threshold: Mean detection threshold in % (N = 30 participants). Error bars represent the standard deviation. **p< 0.01.
The LMM results revealed that dominance did not have a significant main effect (SE = 0.86, t (87) = 1.43, p = 0.15) while the direction (SE = 1.28, t (87) = −2.067, p = 0.04) did have a significant effect. Furthermore, no significant interaction between dominance and direction was found (SE = 0.98, t (87) = −1.23, p = 0.22). Consequently, trials with the dominant and non-dominant legs were combined. In the ascending condition (Asc), the mean detection threshold across 30 participants was found to be 12.4% (standard deviation (SD) = 4.8%), while in the descending condition, the threshold was 9.2% (SD = 4.6%). The paired sample T-test revealed that the detection threshold during the ascending condition was significantly higher than the descending threshold (t (29) = 2.75 p = 0.01).
The CV revealed that the inter-subject variability of the detection threshold was higher for the descending conditions (50.1%) compared to the ascending conditions (38.7%).
Figure 5 presents the results of the symmetry detection questionnaire for the ascending and descending conditions. Participants used hip flexion (Asc: 88%, Desc 81%) as the most prominent information to judge their symmetry/asymmetry during the ascending and descending condition, followed by hip extension (Asc: 40%, Desc 38%), trunk flexion (Asc: 38%, Desc 33%), knee extension (Asc: 35%, Desc 36%) and heel strike (Asc: 28%, Desc 35%). Eleven participants indicated relying on another type of information than provided (Other option) to judge their asymmetry/symmetry with the avatar during either the ascending or descending condition.
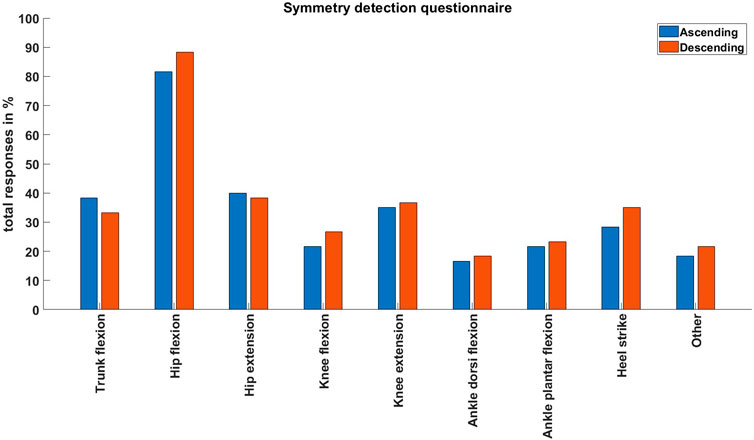
Figure 5. Symmetry detection questionnaire: Total of all answer per category in % (N = 30 participants).
3.2 Sense of embodiment
The mean embodiment scores are presented in Figure 6.
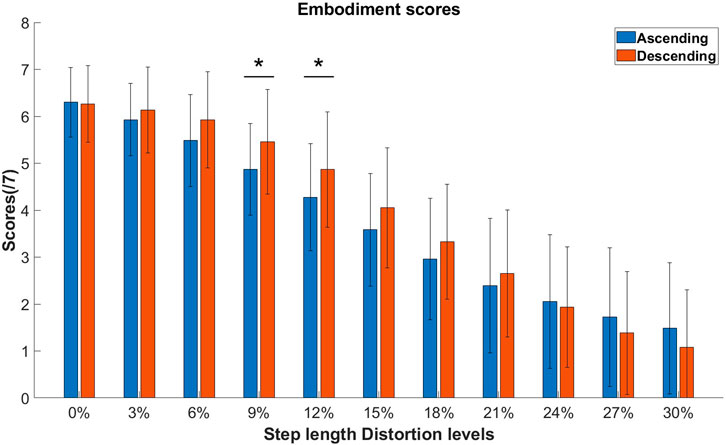
Figure 6. Mean embodiment scores at every 3% distortion level. Error bars represent the standard deviation. Note that in the descending condition, the distortions went from 30% to 0%, so the figure is read from right to left for this condition. The significant difference between conditions at the same distortion level is displayed atop the bars. Furthermore, significance was observed between each adjacent distortion level within the same direction (asc or desc). Comparisons between distortion levels that were more distant from each other were not carried out.
Initially, the linear model revealed a significant main effect of the distortion levels on the sense of embodiment (SE = 0.01, t(1,283) = −14.35, p < 0.001). However, dominance (SE = 0.23, t(1,283) = 0.25, p = 0.80) and direction (SE = 0.23, t(1,283) = 0.61, p = 0.55) did not have a significant main affect. As described in the methodology (2.5.2 The sense of embodiment), the embodiment scores were aggregated between each leg, resulting in two conditions (Asc and Desc).
Within the ascending condition, an increase in distortion levels led to a gradual decrease in the feeling of embodiment from 0% [Mean (M) = 6.3/7, SD = 0.7] to 30% (M = 1.5/7, SD = 1.4). In contrast, the descending conditions exhibited an inverse pattern: as the distortion levels decreased, the feeling of embodiment increased from 30% (M = 1.1/7, SD = 0.6) to 0% (6.3/7, SD = 0.8).
The subsequent LMM analysis demonstrated a significant main effect of distortion levels on the sense of embodiment [SE = 0.01, t(627) = −14.90, p < 0.001]. In contrast, the main effect of direction was not significant [SE = 0.21, t(627) = 0.12, p = 0.91], and the interaction effect between distortion levels and direction also did not reach statistical significance [SE = 0.01, t(627) = −1.29, p = 0.20]. Pairwise comparisons revealed significant differences between all adjacent distortion levels in the ascending conditions. Similar findings were observed in the descending conditions. Comparisons between levels that were further apart (e.g., 0% and 6%) were not performed.
The Pearson correlation coefficient revealed a significant negative relationship between the distortion levels and the embodiment scores, for both the ascending (r = −0.82, p < 0.0002) and the descending (r = −0.84, p < 0.0002) conditions.
Figure 7 shows the mean embodiment scores for the five specific time points around the point of detection.
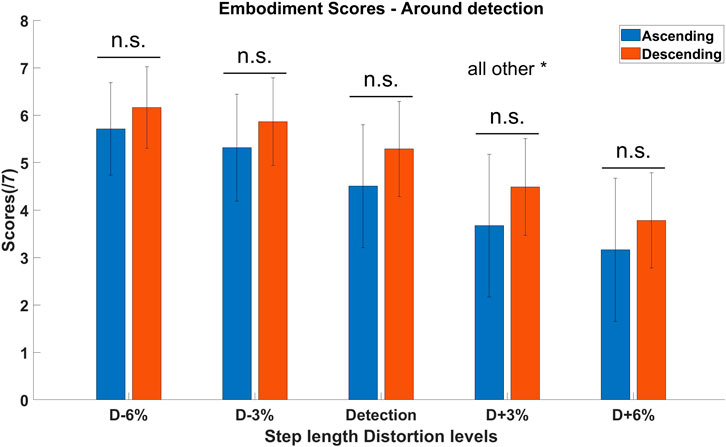
Figure 7. Mean embodiment scores around detection, with distortion levels relative to the point of detection. The results for the descending condition were inverted to compare the results with the ascending condition at each distortion level. n.s indicates the absence of significance. All other comparisons were significant. Ascending and descending conditions were only compared at the same level of distortion.
The LMM did not reveal any significant main effect of dominance, which aligns with the earlier analysis. Consequently, we combined the embodiment scores for the dominant and non-dominant legs for the embodiment scores around the point of detection. The results of the subsequent LMM analysis showed that distortion level [SE = 0.02, t (267) = −11.92, p < 0.001] and direction [SE = 0.18, t (267) = 2.96, p = 0.003] both had significant main effects on embodiment scores. In contrast, the interaction effect between distortion level and direction was not significant [SE = 0.01, t (267) = 0.76, p = 0.44].
The pairwise comparisons further revealed significant differences in embodiment scores between all adjacent distortion levels within the ascending and descending conditions. However, when comparing the embodiment scores at the same distortion levels between ascending and descending conditions, no significant differences were found. These comparisons were specifically conducted at identical levels of distortion to assess the effect of direction (Ascending/Descending) on embodiment.
4 Discussion
4.1 Detection threshold
The first objective of this study was to identify a detection threshold between a participant’s actual gait movements and that of their self-avatar, when their step length is unilaterally distorted. The results show that on average, a unilateral increase in the step length of their self-avatar that is below 12% (ascending) and 9% (descending) goes unnoticed by healthy participants. This supports our first hypothesis (H1) that participants would indeed perceive the unilateral distortion in step length as increases that were above those thresholds were eventually perceived.
The limb to which the step length increase was applied did not have a significant effect on the perception threshold. The role of limb dominance within embodiment experiments has primarily been explored in tasks involving the upper limbs. In the well-studied rubber hand illusion (Botvinick and Cohen, 1998), ownership can be induced with similar strength for both the dominant and non-dominant hand. However, larger proprioceptive drifts are observed in the non-dominant hand (Dempsey-Jones and Kritikos, 2019). This is likely attributed to the frequent use of the dominant hand in fine motor tasks, which tends to rely more on proprioceptive information. Consequently, it may exhibit greater resistance to the false visual information provided by the rubber hand, leading to a reduced drift in the dominant hand. Conversely, relatively little has been studied with regards to the effect of dominance or embodiment on detecting visuomotor discrepancies. The results of this study suggest that limb dominance does not significantly affect the ability to detect gait differences within embodied settings. However, in this study, participants had the contralateral limb available for comparison to detect gait differences in their avatar, unlike in upper body movement contexts where participants could only compare their real arm with the avatar’s arm Therefore, it is difficult to establish if the detection was based on the visuo-proprioceptive incongruency or the comparison of the movements of both virtual limbs or a combination of both.
Significantly higher detection thresholds were found during the ascending conditions, indicating that participants were less sensitive to the distortion when it gradually increased than when it gradually decreased. Moreover, it is possible that participants were more accurate in the task of identifying the moment where their avatar’s movements first matched their own (descending), as opposed to identifying the moment where it no longer matched their own (ascending). However, a study measuring the detection threshold of asymmetry during gait, using a split-belt treadmill, reported higher detection thresholds during the descending conditions (Lauzière et al., 2014). This contrasts with the results found in the current study. In their study, participants walked on a split-belt treadmill with each leg on a separate belt. The speed of one belt was gradually increased until participants perceived a difference in belt speed (ascending threshold), while the descending threshold was obtained by initially setting one belt faster than the other and then gradually decreasing the speed until participants perceived the belt speeds as equal. Nevertheless, a direct comparison of the threshold and symmetry ratios may not be possible due to the different characteristics of the distortions between these two approaches.
In the current study, participants were specifically instructed to detect differences between their avatar’s gait and their own, which could have influenced the detection threshold. Therefore, larger distortion may go unnoticed if participants are not instructed to detect or pay attention to potential mismatches between their self-avatars and themselves. Burns et al. (2006)found that individuals who were explicitly made aware that a misalignment between their actual and virtual hands would occur, were able to detect a discrepancy of 20°. In contrast, participants who were not provided with this information only became consciously aware of the discrepancy when it reached 40°. Additionally, a study by Galvan Debarba et al. (2018) further emphasizes the importance of informing participants about potential mismatches. Their research demonstrated that participants tend to perform poorly in detecting discrepancies during tasks involving reaching for objects in VR when they are not informed about the possibility of a mismatch.
The detection threshold for step length distortion shows considerable variability among participants in both ascending and descending conditions, indicating that individuals have different sensitivities in perceiving these discrepancies. This study obtained the detection threshold by explicitly asking participants to report whether they detected the discrepancies. Previous studies using similar methods within an embodiment distortion paradigm also reported that their detection threshold suffers from a large variability between participants (Galvan Debarba et al., 2018; Porssut et al., 2019; Feick et al., 2022). Feick et al. (2022) suggested that this detection variability may be linked to the participant’s previous experience in VR. They found higher thresholds for participants with less experience than those with prior VR experience. A trend toward a higher detection threshold for participants who often play video games has also been reported (Porssut et al., 2022).
In the subjective detection question, participants reported using similar information to detect the mismatch/match during the ascending and descending conditions. Hip flexion was the information that was most reported to be used to perceive the step length distortion. Participants might have relied on their proprioceptive awareness of hip movement and the visual differences between with the avatar’s hip flexion while walking. Indeed, an increase in step length leads to an increase in hip flexion, hip extension, and knee flexion in able-bodied gait (Sawicki and Ferris, 2009; Lim et al., 2017). Therefore, it is plausible that participants noticed the differences between their own actual hip flexion and that of their avatars due to the noticeable increase in hip flexion resulting from the manipulated step lengths.
4.2 Sense of embodiment
A second objective of this study was to assess how the different distortion levels and the point of detection impacted the SoE. The results revealed a gradual decrease in embodiment as the distortion levels increased and a gradual increase in embodiment as the distortion levels decreased. The results found during the ascending conditions are consistent with previous research by Porssut et al. (2019), who also reported a decrease in embodiment when they gradually introduced a discrepancy between the real and the avatar’s arm during a reaching task.
The point of detection did not cause a break in embodiment, nor was there a rapid decline seen after the detection. Rather, we observe a steady decline in embodiment as the distortion increases. The level of distortion strongly correlated with the level of embodiment, but the conscious detection of the distortion had no impact on the sense of embodiment. Despite being consciously aware of the distortion, participants still reported feeling embodied in their avatars. This is in contradiction with our second hypothesis (H2), which suggested that the detection of distortion would lead to a break in embodiment.
This finding is consistent with research conducted by Porssut et al. (2019) on the upper limb, who similarly found that the detection of a distortion did not disrupt embodiment during an arm movement. However, in their study, a distortion that was gradually introduced and continually increased after the point of detection eventually led to a break in embodiment. Subsequently, the same group of authors found that embodiment remains after a discrepancy is consciously detected, as long as it does not create a visuo-proprioceptive conflict during an arm-reaching task (Porssut et al., 2022). These conflicts arose when the elbows of the participants reached maximum extension before their avatars did. In our study, similar conflicts did not occur as the lower limb joints did not reach the limits of their ranges of motion during gait. The knee joint comes closest to its limit, and typically reaches a minimum flexion of approximately 5° (Goldberg et al., 2006).
Kannape and Blanke (2013) have previously measured the detection threshold between a users’ gait and that of their avatar. Participants were presented with a full-body avatar mimicking their movements in real-time, viewed from a 3rd-person perspective on a large screen that was positioned in front of a treadmill. A temporal delay between the participants’ movements and those of the avatar was then gradually introduced. Participants were asked whether they felt their movements were accurately replicated by the avatar. They reported that the sense of agency progressively decreased as the temporal distortion increased, without a clear break in agency at the point of detection. These results are analogous to our results, although they only relate to agency. Embodiment was not measured in that study as the 3rd-person perspective is not conducive to strong feelings of embodiment (Spanlang et al., 2014).
Results from the descending conditions also showed no effect of detection on embodiment. Instead, the level of embodiment shows an inverse relationship with the level of the distortions, and it continued to increase gradually even after the point of detection, where participants reported that the avatar was replicating their gait movements again. When comparing the levels of embodiments at different distortion levels, the directions of the distortions (increasing or decreasing) did not have a significant effect. This indicates that for a given step length distortion, the embodiment level is similar whether that distortion is the result of a large distortion that was gradually decreased or null distortion that was gradually increased.
When a large distortion is initially highly noticeable, it disrupts embodiment, but as the distortion diminishes gradually, participants seem to have adapted to the changes, resulting in the observed trend of higher levels of embodiment. Another factor at play is that, in the descending condition, participants may be evaluating their embodiment relative to the previous distortion level, which was comparatively worse. This comparison might slightly bias the perception of embodiment positively. In contrast, in the ascending condition, the point of comparison is comparatively better, introducing a negative bias to the SoE.
These findings confirm our third hypothesis (H3), which suggests that high levels of embodiment can be established when the avatar’s body is initially not spatially aligned with that of the participants, a deviation from the conventional approach to inducing embodiment (Spanlang et al., 2014). Instead, the results of this study demonstrate that certain levels of embodiment can be regained when a very large and clearly noticeable step length distortion is gradually diminished which initially disrupted embodiment. In other words, the direction of the distortion did not appear to play a significant role in the establishment of embodiment. Indeed, previous research indicated that embodiment could be re-established after an abrupt distortion is removed, as such distortions have a negative impact on embodiment (Padrao et al., 2016; Cohn et al., 2020; Gonzalez-Franco et al., 2020). However, the outcomes of our study suggest that embodiment can also be restored by gradually reducing the magnitude of a substantial distortion while still maintaining visuomotor synchronization rather than solely and abruptly restoring the spatial alignment between the avatar and the participant’s movements.
4.3 Limitations
A limitation of this study arises from the participants’ prior awareness that a distortion towards the gait of the avatar would occur. Although participants were not explicitly informed about the exact nature of the distortion (distortion of their step length), their visual attention was steered to perceive the gait differences of the avatar throughout the experiment. As discussed, this has been shown to lower the detection threshold. Therefore, the thresholds identified in this study may underestimate the size of distortions that would go undetected when users are not aware of potential manipulation of their avatar’s movements. Additionally, participants’ prior VR experience was not assessed, which previous research has suggested might influence sensitivity to spatial distortions (Feick et al., 2022; Porssut et al., 2022). Given the reported variability in detection thresholds, it is plausible that prior exposure to VR could have affected our results. Participants with different levels of VR familiarity might exhibit varied thresholds for detecting step length distortions due to differing baseline sensitivities or adaptation skills.
Another limitation of this is that participants’ visual attention was directed to the avatar’s movements. Healthy individuals typically do not focus on their own feet during gait, which may have affected their natural gait. However, this instruction was necessary to ensure that participants were looking at the gait distortions. Moreover, in a rehabilitation setting, similar instructions would be necessary to ensure that patients accurately observe the avatar’s gait distortions. Furthermore, it’s possible that participants may have compared the movements of one leg with the other rather than solely comparing their real leg’s movements with those of the avatar. This uncertainty presents a limitation since the visibility of both limbs made it difficult to isolate the specific information used to detect the gait asymmetry. Nevertheless, the results remain relevant as they reflect detection thresholds in the context of unilateral step length distortion in applications where these type of manipulations of an avatar’s gait would be applied.
Another limitation lies in the methodology for assessing the subjective feeling of embodiment during different distortion levels. An interval of 3% was deemed to be adequate to monitor the progression of the embodiment feeling across the different distortion levels, after conducting four pilot studies. This limitation arose from the practical consideration that requiring participants to continuously respond to the embodiment question at 1% intervals could have been potentially distracting throughout the experiment. Furthermore, due to these constrains we were not able to employ the validated embodiment questionnaire recently established by Peck and Gonzalez Franco (2021). Instead, a single question was used to assess the embodiment. This question is only one of 16 questions that comprise the Peck and Gonzalez Franco questionnaire, related to the ownership component of embodiment. We therefore have an incomplete measure of the SoE.
Moreover, the repeated measurement of embodiment could have influenced the detection threshold by diverting the participants’ attention from identifying discrepancies between the avatar’s movements and their own. Individual differences in how participants managed the division of their attention between these discrepancies and the evaluation and reporting of their SoE may partially explain the inter-participant variability in detection thresholds.
4.4 Future work
These findings have potential implications for motor rehabilitation, particularly in the context of designing avatars for therapeutic use. They provide a better understanding of the size distortions that can be applied to a self-avatar’s gait when aiming for the distortions to go undetected. Avatars can be configured to distort their gait subtly, ensuring that any distortion remains below a predefined detection threshold, such as a 12% increase in step length. This can be done while still preserving a strong sense of embodiment. Similarly, the results show how a given distortion size affects the level of embodiment. If users report a level of embodiment that is below what is required, adjustments can be made to reduce the distortion, effectively increasing the level of embodiment. This approach has the potential to help patients perceive the altered movement as their own, which could ultimately enhance their rehabilitation and recovery (Tambone et al., 2021).
To inform whether the detection of distortions must be avoided and what level of embodiment must be maintained, the complex relationship between the detection of distortions, the subjective level of embodiment, and the strength of resulting motor adjustments should be better understood. Our research provides valuable insights into the relation between the first two elements, shedding light on how extensively we can modify an avatar’s gait without it becoming noticeable and how far we can push these distortions while maintaining a relatively high SoE. Further investigation is needed to explore how these factors correlate with changes in proprioception and motor performance, in phenomena like the follower effect (Gonzalez-Franco et al., 2020). Also, when using the paradigm of error exaggeration, the requirements with regard to avoiding detection and maintaining a given level of embodiment may differ.
It is important to note that the detection threshold was obtained with healthy participants. It is possible that the ability to perceive spatial distortion between themselves and their embodied avatar during gait is altered in patients suffering from motor and sensory deficits. Future work should investigate if the detection thresholds for these patients are indeed different from those observed in a healthy population. Although similar detection thresholds were found between healthy participants and mildly ataxic patients with focal lesions in the cerebellum during the split-belt treadmill paradigm (Hoogkamer et al., 2015), the underlying mechanism involved may be distinct from embodied avatar movement distortions protocols.
Lastly, future studies could explore the impact of participants’ prior experience with VR on the detection thresholds for self-avatar movement distortions. As our current study did not evaluate participants’ VR experience, it remains uncertain whether such experience influenced the observed variability in our results. Therefore, future research could investigate the impact of participants’ VR experience, particularly their exposure to VR simulations featuring virtual self-avatars, on the detection thresholds. This exploration could provide additional insights into the factors influencing perceptual thresholds in VR environments.
5 Conclusion
Our results show that healthy participants perceive unilateral step length discrepancies that are above 12% and 9% in the ascending and descending conditions, respectively. There is a strong correlation between the level of distortion and the sense of embodiment. As the distortion levels increase, the sense of embodiment decreases, and vice versa. Contrary to the initial hypothesis, the detection of distortion does not break the SoE; participants continue to feel embodied in their avatars even when aware of the distortion. Furthermore, our results show that embodiment can be induced at high levels by gradually decreasing the distortion applied to a virtual self-avatar, rather than the traditional method that consists of inducing embodiment without distortions and gradually applying them. These results provide insights into participants’ tolerance for discrepancies in embodied avatar distortion paradigms during gait in virtual environments, with a potential application for motor training and gait rehabilitation.
Data availability statement
The raw data supporting the conclusions of this article will be made available by the authors, without undue reservation.
Ethics statement
The studies involving humans were approved by Research Ethics Boards of Centre hospitalier de l’Université de Montreal (CHUM) and of Ecole de technologie supérieure (ETS). The studies were conducted in accordance with the local legislation and institutional requirements. The participants provided their written informed consent to participate in this study.
Author contributions
IW: Conceptualization, Data curation, Formal Analysis, Investigation, Methodology, Software, Validation, Visualization, Writing–original draft, Writing–review and editing. RA: Conceptualization, Investigation, Methodology, Supervision, Writing–review and editing. VV: Conceptualization, Methodology, Software, Writing–review and editing. SN: Conceptualization, Supervision, Writing–review and editing. CD: Conceptualization, Supervision, Writing–review and editing. DL: Conceptualization, Funding acquisition, Methodology, Resources, Software, Supervision, Writing–review and editing.
Funding
The author(s) declare financial support was received for the research, authorship, and/or publication of this article. Funding for this research was provided by a Team Research Grant of the Fonds de recherche du Quebec—Nature et technologies (FRQNT) and its strategic group in Interactive Technologies of Engineering in Rehabilitation (INTER) as well as by Natural Sciences and Engineering Research Council of Canada (NSERC).
Acknowledgments
The authors express gratitude to the participants for volunteering in this research. Additionally, the authors acknowledge the financial support received from FRQNT, NSERC and INTER. The authors also thank Serge Vicente for his valuable insights and contributions to the statistical analysis.
Conflict of interest
The authors declare that the research was conducted in the absence of any commercial or financial relationships that could be construed as a potential conflict of interest.
Publisher’s note
All claims expressed in this article are solely those of the authors and do not necessarily represent those of their affiliated organizations, or those of the publisher, the editors and the reviewers. Any product that may be evaluated in this article, or claim that may be made by its manufacturer, is not guaranteed or endorsed by the publisher.
References
Banakou, D., Groten, R., and Slater, M. (2013). Illusory ownership of a virtual child body causes overestimation of object sizes and implicit attitude changes. Proc. Natl. Acad. Sci. 110, 12846–12851. doi:10.1073/PNAS.1306779110
Berger, C. C., Lin, B., Lenggenhager, B., Lanier, J., and Gonzalez-Franco, M. (2022). Follow your nose: extended arm reach after pinocchio illusion in virtual reality. Front. Virtual Real 3, 1–12. doi:10.3389/frvir.2022.712375
Botvinick, M., and Cohen, J. (1998). Rubber hands ‘feel’ touch that eyes see. Nature 391, 756. doi:10.1038/35784
Bourdin, P., Martini, M., and Sanchez-Vives, M. V. (2019). Altered visual feedback from an embodied avatar unconsciously influences movement amplitude and muscle activity. Sci. Rep. 9, 19747. doi:10.1038/s41598-019-56034-5
Bovet, S., Debarba, H. G., Herbelin, B., Molla, E., and Boulic, R. (2018). The critical role of self-contact for embodiment in virtual reality. IEEE Trans. Vis. Comput. Graph 24, 1428–1436. doi:10.1109/TVCG.2018.2794658
Burin, D., Kilteni, K., Rabuffetti, M., Slater, M., and Pia, L. (2019). Body ownership increases the interference between observed and executed movements. PLoS One 14, 02098999. doi:10.1371/journal.pone.0209899
Burns, E., Razzaque, S., Panter, A. T., Whitton, M. C., McCallus, M. R., and Brooks, F. P. (2006). The hand is more easily fooled than the eye: users are more sensitive to visual interpenetration than to visual-proprioceptive discrepancy. Presence Teleoperators and Virtual Environ. 15, 1–15. doi:10.1162/PRES.2006.15.1.1
Cohn, B. A., Maselli, A., Ofek, E., and Gonzalez-Franco, M. (2020). “SnapMove: movement projection mapping in virtual reality,” in Proceedings - 2020 IEEE International Conference on Artificial Intelligence and Virtual Reality, AIVR 2020 (Utrecht, Netherlands: Institute of Electrical and Electronics Engineers Inc.), 74–81. doi:10.1109/AIVR50618.2020.00024
Delahaye, M., Blanke, O., Boulic, R., and Herbelin, B. (2023). Avatar error in your favor: embodied avatars can fix users’ mistakes without them noticing. PLoS One 18, e0266212. doi:10.1371/JOURNAL.PONE.0266212
Dempsey-Jones, H., and Kritikos, A. (2019). Handedness modulates proprioceptive drift in the rubber hand illusion. Exp. Brain Res. 237, 351–361. doi:10.1007/S00221-018-5391-3
Feick, M., Regitz, K. P., Tang, A., and Krüger, A. (2022). “Designing visuo-haptic illusions with proxies in virtual reality: exploration of grasp, movement trajectory and object mass,” in Proceedings of the 2022 SIGCHI Conference on Human Factors in Computing Systems.
Galvan Debarba, H., Boulic, R., Salomon, R., Blanke, O., and Herbelin, B. (2018). Self-attribution of distorted reaching movements in immersive virtual reality. Comput. Graph 76, 142–152. doi:10.1016/J.CAG.2018.09.001
Goldberg, S. R., Õunpuu, S., Arnold, A. S., Gage, J. R., and Delp, S. L. (2006). Kinematic and kinetic factors that correlate with improved knee flexion following treatment for stiff-knee gait. J. Biomech. 39, 689–698. doi:10.1016/J.JBIOMECH.2005.01.015
Gonzalez-Franco, M., Cohn, B., Ofek, E., Burin, D., and Maselli, A. (2020). “The self-avatar follower effect in virtual reality,” in Proceedings - 2020 IEEE Conference on Virtual Reality and 3D User Interfaces, VR 2020, 18–25. doi:10.1109/VR46266.2020.1580500165557
Hajnal, A., Fonseca, S., Harrison, S., Kinsella-Shaw, J., and Carello, C. (2007). Comparison of dynamic (effortful) touch by hand and foot. J. Mot. Behav. 39, 82–88. doi:10.3200/JMBR.39.2.82-88
Han, J., Anson, J., Waddington, G., and Adams, R. (2013). Proprioceptive performance of bilateral upper and lower limb joints: side-general and site-specific effects. Exp. Brain Res. 226, 313–323. doi:10.1007/s00221-013-3437-0
Hoogkamer, W., Bruijn, S. M., Potocanac, Z., Van Calenbergh, F., Swinnen, S. P., and Duysens, J. (2015). Gait asymmetry during early split-belt walking is related to perception of belt speed difference. J. Neurophysiol. 114, 1705–1712. doi:10.1152/jn.00937.2014
Janeh, O., Fründt, O., Schönwald, B., Gulberti, A., Buhmann, C., Gerloff, C., et al. (2019). Gait training in virtual reality: short-term effects of different virtual manipulation techniques in Parkinson’s disease. Cells 8, 419. doi:10.3390/cells8050419
Kannape, O. A., and Blanke, O. (2013). Self in motion: sensorimotor and cognitive mechanisms in gait agency. J. Neurophysiol. 110, 1837–1847. doi:10.1152/jn.01042.2012
Kilteni, K., Maselli, A., Kording, K. P., and Slater, M. (2015). Over my fake body: body ownership illusions for studying the multisensory basis of own-body perception. Front. Hum. Neurosci. 9, 141. doi:10.3389/fnhum.2015.00141
Kilteni, K., and Slater, M. (2012). The sense of embodiment in virtual reality. Presence 21, 373–387. doi:10.1162/PRES_a_00124
Kokkinara, E., Kilteni, K., Blom, K. J., and Slater, M. (2016). First person perspective of seated participants over a walking virtual body leads to illusory agency over the walking. Sci. Rep. 6, 28879. doi:10.1038/srep28879
Kokkinara, E., and Slater, M. (2014). Measuring the effects through time of the influence of visuomotor and visuotactile synchronous stimulation on a virtual body ownership illusion. Perception 43, 43–58. doi:10.1068/p7545
Kokkinara, E., Slater, M., and López-Moliner, J. (2015). The effects of visuomotor calibration to the perceived space and body, through embodiment in immersive virtual reality. ACM Trans. Appl. Percept. 13, 1–22. doi:10.1145/2818998
Lauzière, S., Miéville, C., Duclos, C., Aissaoui, R., and Nadeau, S. (2014). Perception threshold of locomotor symmetry while walking on a split-belt treadmill in healthy elderly individuals. Percept. Mot. Ski. 118, 475–490. doi:10.2466/25.15.PMS.118k17w6
Lim, Y. P., Lin, Y.-C., and Pandy, M. G. (2017). Effects of step length and step frequency on lower-limb muscle function in human gait. J. Biomech. 57, 1–7. doi:10.1016/j.jbiomech.2017.03.004
Malone, L. A., and Bastian, A. J. (2010). Thinking about walking: effects of conscious correction versus distraction on locomotor adaptation. J. Neurophysiol. 103, 1954–1962. doi:10.1152/JN.00832.2009
Miall, R. C., and Wolpert, D. M. (1996). Forward models for physiological motor control. Neural Netw. 9, 1265–1279. doi:10.1016/S0893-6080(96)00035-4
Padrao, G., Gonzalez-Franco, M., Sanchez-Vives, M. V., Slater, M., and Rodriguez-Fornells, A. (2016). Violating body movement semantics: neural signatures of self-generated and external-generated errors. Neuroimage 124, 147–156. doi:10.1016/j.neuroimage.2015.08.022
Patterson, K. K., Parafianowicz, I., Danells, C. J., Closson, V., Verrier, M. C., Staines, W. R., et al. (2008). Gait asymmetry in community-ambulating stroke survivors. Arch. Phys. Med. Rehabil. 89, 304–310. doi:10.1016/J.APMR.2007.08.142
Paul, S. S., Ada, L., and Canning, C. G. (2005). Automaticity of walking – implications for physiotherapy practice. Phys. Ther. Rev. 10, 15–23. doi:10.1179/108331905X43463
Peck, T. C., and Gonzalez-Franco, M. (2021). Avatar Embodiment. A Standardized Questionnaire. Front. Virtual Real. 1, 44–44. doi:10.3389/frvir.2020.575943
Porssut, T., Blanke, O., Herbelin, B., and Boulic, R. (2022). Reaching articular limits can negatively impact embodiment in virtual reality. PLoS One 17, e0255554. doi:10.1371/journal.pone.0255554
Porssut, T., Herbelin, B., and Boulic, R. (2019). “Reconciling being in-control vs. being helped for the execution of complex movements in VR,” in 26th IEEE Conference on Virtual Reality and 3D User Interfaces, VR 2019 - Proceedings, 529–537. doi:10.1109/VR.2019.8797716
Reisman, D. S., McLean, H., Keller, J., Danks, K. A., and Bastian, A. J. (2013). Repeated split-belt treadmill training improves poststroke step length asymmetry. Neurorehabil Neural Repair 27, 460–468. doi:10.1177/1545968312474118
Sadeghi, H., Allard, P., Prince, F., and Labelle, H. (2000). Symmetry and limb dominance in able-bodied gait: a review. Gait Posture 12, 34–45. doi:10.1016/S0966-6362(00)00070-9
Sawicki, G. S., and Ferris, D. P. (2009). Powered ankle exoskeletons reveal the metabolic cost of plantar flexor mechanical work during walking with longer steps at constant step frequency. J. Exp. Biol. 212 (Pt 1), 21–31. doi:10.1242/JEB.017269
Schielzeth, H., Dingemanse, N. J., Nakagawa, S., Westneat, D. F., Allegue, H., Teplitsky, C., et al. (2020). Robustness of linear mixed-effects models to violations of distributional assumptions. Methods Ecol. Evol. 11, 1141–1152. doi:10.1111/2041-210X.13434
Seung-Jae, K., and Hermano Igo, K. (2012). Effects of implicit visual feedback distortion on human gait. Exp. Brain Res. 218, 495–502. doi:10.1007/s00221-012-3044-5
Slater, M., Pérez Marcos, D., Ehrsson, H., and Sanchez-Vives, M. V. (2008). Towards a digital body: the virtual arm illusion. Front. Hum. Neurosci. 2, 6. doi:10.3389/neuro.09.006.2008
Spanlang, B., Normand, J.-M., Borland, D., Kilteni, K., Giannopoulos, E., Pomés, A. s., et al. (2014). How to build an embodiment lab: achieving body representation illusions in virtual reality. Front. Robot. AI 1. doi:10.3389/frobt.2014.00009
Tambone, R., Giachero, A., Calati, M., Molo, M. T., Burin, D., Pyasik, M., et al. (2021). Using body ownership to modulate the motor system in stroke patients. Psychol. Sci. 32, 655–667. doi:10.1177/0956797620975774
Willaert, I., Aissaoui, R., Nadeau, S., Duclos, C., and Labbe, D. R. (2020). “Modulating the gait of a real-time self-avatar to induce changes in stride length during treadmill walking,” in Proceedings - 2020 IEEE Conference on Virtual Reality and 3D User Interfaces, VRW 2020, 718–719. doi:10.1109/VRW50115.2020.00210
Keywords: virtual reality, embodiment, perception, distortion, gait, step length, gait asymmetry
Citation: Willaert I, Aissaoui R, Vallageas V, Nadeau S, Duclos C and Labbe DR (2024) Detection threshold of distorted self-avatar step length during gait and the effects on the sense of embodiment. Front. Virtual Real. 5:1339296. doi: 10.3389/frvir.2024.1339296
Received: 15 November 2023; Accepted: 30 September 2024;
Published: 10 October 2024.
Edited by:
Akrivi Katifori, Athena Research Center, GreeceReviewed by:
Adélaïde Genay, The University of Melbourne, AustraliaMaria Roussou, National and Kapodistrian University of Athens, Greece
Christos Lougiakis, National and Kapodistrian University of Athens, in collaboration with reviewer MR
Copyright © 2024 Willaert, Aissaoui, Vallageas, Nadeau, Duclos and Labbe. This is an open-access article distributed under the terms of the Creative Commons Attribution License (CC BY). The use, distribution or reproduction in other forums is permitted, provided the original author(s) and the copyright owner(s) are credited and that the original publication in this journal is cited, in accordance with accepted academic practice. No use, distribution or reproduction is permitted which does not comply with these terms.
*Correspondence: Iris Willaert, aXJpcy53aWxsYWVydC4xQGVucy5ldHNtdGwuY2E=; David R. Labbe, ZGF2aWQubGFiYmVAZXRzbXRsLmNh