Mesenchymal Stromal Cells and Exosomes: Progress and Challenges
- 1Department of Pediatrics, School of Medicine and Public Health, University of Wisconsin-Madison, Madison, WI, United States
- 2Department of Medicine, School of Medicine and Public Health, University of Wisconsin-Madison, Madison, WI, United States
- 3Carbone Cancer Center, University of Wisconsin-Madison, Madison, WI, United States
Due to their robust immunomodulatory capabilities, mesenchymal stem/stromal cells (MSCs) have been used as a cellular therapy for a number of human diseases. Part of the mechanism of action of MSCs is the production of extracellular vesicles (EVs) that contain proteins, nucleic acids, and lipids that transmit signals to recipient cells that change their biologic behavior. This review briefly summarizes the development of MSCs as a treatment for human diseases as well as describes our present understanding of exosomes; how they exert their effects on target cells, and how they are differentiated from other EVs. The current treatment paradigm for acute radiation syndrome (ARS) is discussed, and how MSCs and MSC derived exosomes are emerging as treatment options for treating patients after radiation exposure. Other conditions such as graft-versus-host disease and cardiovascular disease/stroke are discussed as examples to highlight the immunomodulatory and regenerative capacity of MSC-exosomes. Finally, a consideration is given to how these cell-based therapies could possibly be deployed in the event of a catastrophic radiation exposure event.
Infusion of MSCS for Treating Inflammatory Diseases
Mesenchymal stem/stromal cells (MSCs) are a potent immunomodulatory cell subset that are readily obtainable and easily expandable in vitro. MSCs can be obtained from many different tissues (bone marrow, adipose tissue, peripheral blood, umbilical cord blood), and are being studied for a number of conditions due to their ability to differentiate into various cell types, to migrate to various tissues, and to function as potent immunomodulators (Hass et al., 2011; Musiał-Wysocka et al., 2019). These cells are already approved in Europe for the treatment of complex perianal fistulas in adults with non-active/mildly active luminal Crohn’s disease (daradstrocel, Alofisel) and in Japan for steroid-refractory acute graft-versus-host-disease (GVHD) (TEMCELL). A Biologics License Application (BLA) has been submitted to the Food and Drug Administration (FDA) in the United States for steroid refractory acute GVHD in children (remestemcel-L, Ryoncil), with approval expected late 2020. Future BLAs may soon follow since MSCs have shown to be safe or exhibit clinical efficacy for the treatment of other highly inflammatory conditions such as chronic GVHD (Gao et al., 2016; Chen et al., 2019), ankylosing spondylitis (Wang P. et al., 2014), atopic dermatitis (Kim et al., 2017), bronchopulmonary dysplasia (Chang et al., 2014; Ahn et al., 2017), pulmonary emphysema (de Oliveira et al., 2017), non-ischemic cardiomyopathy (Chin et al., 2011; Butler et al., 2017), liver allograft rejection (Shi et al., 2017) and cirrhosis (Zhang et al., 2012; Suk et al., 2016; Liang et al., 2017), juvenile idiopathic arthritis (Swart et al., 2019), type 1 and type 2 diabetes (Jiang et al., 2011; Cai et al., 2016; Bhansali et al., 2017), rheumatoid arthritis (Park et al., 2018; Shadmanfar et al., 2018; Ghoryani et al., 2019), multiple sclerosis (Mohyeddin Bonab et al., 2007; Karussis et al., 2010; Bonab et al., 2012; Li J.F. et al., 2014; Harris et al., 2018; Riordan et al., 2018), systemic lupus erythematous (Wang D. et al., 2013, 2014), and osteoarthritis (Davatchi et al., 2011, 2016; Koh and Choi, 2012; Orozco et al., 2013; Wong et al., 2013; Vega et al., 2015; Lamo-Espinosa et al., 2016, 2018; Soler et al., 2016; Emadedin et al., 2018; Khalifeh Soltani et al., 2019; Matas et al., 2019).
Infusion of MSCS for Tissue Repair and Regeneration
In part due to their immunomodulatory properties, MSCs have been observed to promote a regenerative environment that aids in the functional recovery of various damaged tissues (Bernardo et al., 2012). MSCs have proven to be safe or exhibited clinical efficacy in the field of regenerative medicine. Examples include improving neurologic function in amyotrophic lateral sclerosis (Petrou et al., 2016; Sykova et al., 2017; Berry et al., 2019), cerebral palsy (Wang X. et al., 2013; Huang et al., 2018), delayed encephalopathy after carbon monoxide poisoning (Wang H. et al., 2016), epilepsy (Hlebokazov et al., 2017), stroke (Bang et al., 2005; Lee et al., 2010), metachromatic leukodystrophy (Koc et al., 2002), and spinal cord injury (Vaquero et al., 2018); improved sexual function in erectile dysfunction (Al Demour et al., 2018); improved motor activity with frailty disorder (Tompkins et al., 2017), and multiple system atrophy (Lee et al., 2008, 2012; Singer et al., 2019); improved cardiovascular function in heart failure (Hare et al., 2012; Golpanian et al., 2015; Mathiasen et al., 2015; Bartolucci et al., 2017), and myocardial ischemia/angina (Hare et al., 2009; Friis et al., 2011; Haack-Sorensen et al., 2013; Karantalis et al., 2014; Kim et al., 2018), improved bone repair in hypophosphatasia (Taketani et al., 2015), lumbar disc degeneration (Orozco et al., 2011; Noriega et al., 2017), osteogenesis imperfecta (Gotherstrom et al., 2014), and osteonecrosis (Hernigou et al., 2018); improved healing from kidney injury (Tan et al., 2012; Saad et al., 2017); improved healing from liver injury related to acute on chronic hepatitis (Shi et al., 2012; Lin et al., 2017) and ischemic biliary lesions following liver transplantation (Zhang et al., 2017); improved hematopoietic recovery (Xiao et al., 2013; Zhang et al., 2013; Xiong et al., 2014); and accelerated wound healing (Falanga et al., 2007). From these indications, remestemcel-L is already in phase III trials for advanced heart failure and chronic low back pain.
Potential Mechanisms of Action
Mesenchymal stem/stromal cells have been shown to suppress inflammation through direct cell-to-cell contact in inflamed tissues and through production of numerous anti-inflammatory molecules such as indoleamine 2,3 dioxygenase (IDO) (Su et al., 2014), nitric oxide (NO) (Su et al., 2014), prostaglandin E2 (PGE2) (Hsu et al., 2013), transforming growth factor (TGF)-β (de Araujo Farias et al., 2018), heme oxygenase 1 (HO1) (Chabannes et al., 2007), and hepatocyte growth factor (HGF) (Lee et al., 2018), among others. These molecules suppress the effect of immune cells such as macrophages (Nemeth et al., 2009; Eslani et al., 2018), monocytes (Cutler et al., 2010), dendritic cells (Jiang et al., 2005), B-cells (Corcione et al., 2006), NK cells (Sotiropoulou et al., 2006), and T-cells (Engela et al., 2013; Li M. et al., 2014). In addition to immuno-suppressive molecules, MSCs can influence target cell function through the secretion of large amounts of exosomes. MSC-derived exosomes have been investigated in preclinical models as a potential therapeutic for many of the same conditions that MSCs have shown efficacy in treating, such as wound healing (Fang et al., 2016; Samaeekia et al., 2018), angiogenesis (Teng et al., 2015; Huang et al., 2017), bronchopulmonary dysplasia (Braun et al., 2018), and various autoimmune disorders (Riazifar et al., 2019), but have also shown efficacy in facilitating skeletal muscle regeneration (Nakamura et al., 2015), neurogenesis (Reza-Zaldivar et al., 2019), recovery from stroke (Zhang and Chopp, 2016), and tendon repair (Chamberlain et al., 2019).
To date, no clinical trials infusing MSC-exosomes have been published although some studies have been completed (NCT03384433, NCT02138331) or are recruiting/about to open to accrual (Table 1).
This review focuses on recent pre-clinical work on the potential therapeutic uses of MSCs and MSC-exosomes to polarize or “educate” immune cells into anti-inflammatory cells, with treatment of acute radiation syndrome (ARS) and GVHD as models for the systemic effects of the anti-inflammatory properties of MSC-exosomes. The organ-specific regenerative effects of MSC-exosomes are also explored, using cardiovascular disease and stroke as examples. ARS is also used as an example of how MSC derived exosomes could be developed as a cell-based therapeutic, with consideration given to the potential challenges and drawbacks of such an approach. For a thorough review on how MSC exosomes are being used for the treatment of other conditions, a recently published review is highly recommended (Joo et al., 2020).
Exosomes: Formation, Characteristics, and Cargo
Extracellular vesicles (EVs) are lipid bilayer particles that are released from cells. This diverse family of particles includes microvesicles (MVs), apoptotic bodies, and exosomes. EVs are composed of membrane-bound particles that are classified according to size with exosomes generally defined as 30–150 nm in diameter (Helwa et al., 2017; Bebelman et al., 2018). Exosomes are generally considered to be produced through the inward budding of late stage endosomes, forming multi-vesicular bodies (MVBs) which then release these “buds” (exosomes) upon fusion with the plasma membrane (Hessvik and Llorente, 2018). Due to the fact that exosomes are differentiated from EVs based on their relative size, it can be difficult to separate exosomes from smaller EVs. In fact, the formation of a smaller subset of EVs in the size range of what is considered to be exosomes has been observed through the direct budding of the plasma membrane (Casado et al., 2017). Cholesterol, sphingomyelin, ceramide, and various lipid molecules are found in large quantities on the exosomal membrane (Mashouri et al., 2019). Once the exosomes are released into the intercellular space, they can be taken up by recipient cells by endocytosis, receptor–ligand binding, or through direct binding (Kahroba et al., 2019).
Exosomes exert their effects by releasing their contents into the cytosol of recipient cells. Exosome cargo can consist of a number of different molecules such as nucleic acids (DNA, RNA, mRNA miRNA), pro-inflammatory and anti-inflammatory cytokines, enzymes, and various other proteins (Mathivanan et al., 2010; D’Asti et al., 2012). Cytokines can be found not only encapsulated in the exosome, but also imbedded in the exosomal membrane itself (Fitzgerald et al., 2018). The authors of this study hypothesized that exosomes can deliver smaller amounts of cytokines directly to the intended target cell, a more efficient delivery mechanism compared to the traditional cytokine “dump” into the intercellular space (which could be taken up by any cell with a corresponding receptor). Other proteins found in the exosomal membrane such as various heat-shock and signaling proteins have shown to perform immunomodulatory functions as well (Urbanelli et al., 2013; Reddy et al., 2018). A visual representation of exosomes, their formation, and their cargo can be seen in Figure 1.
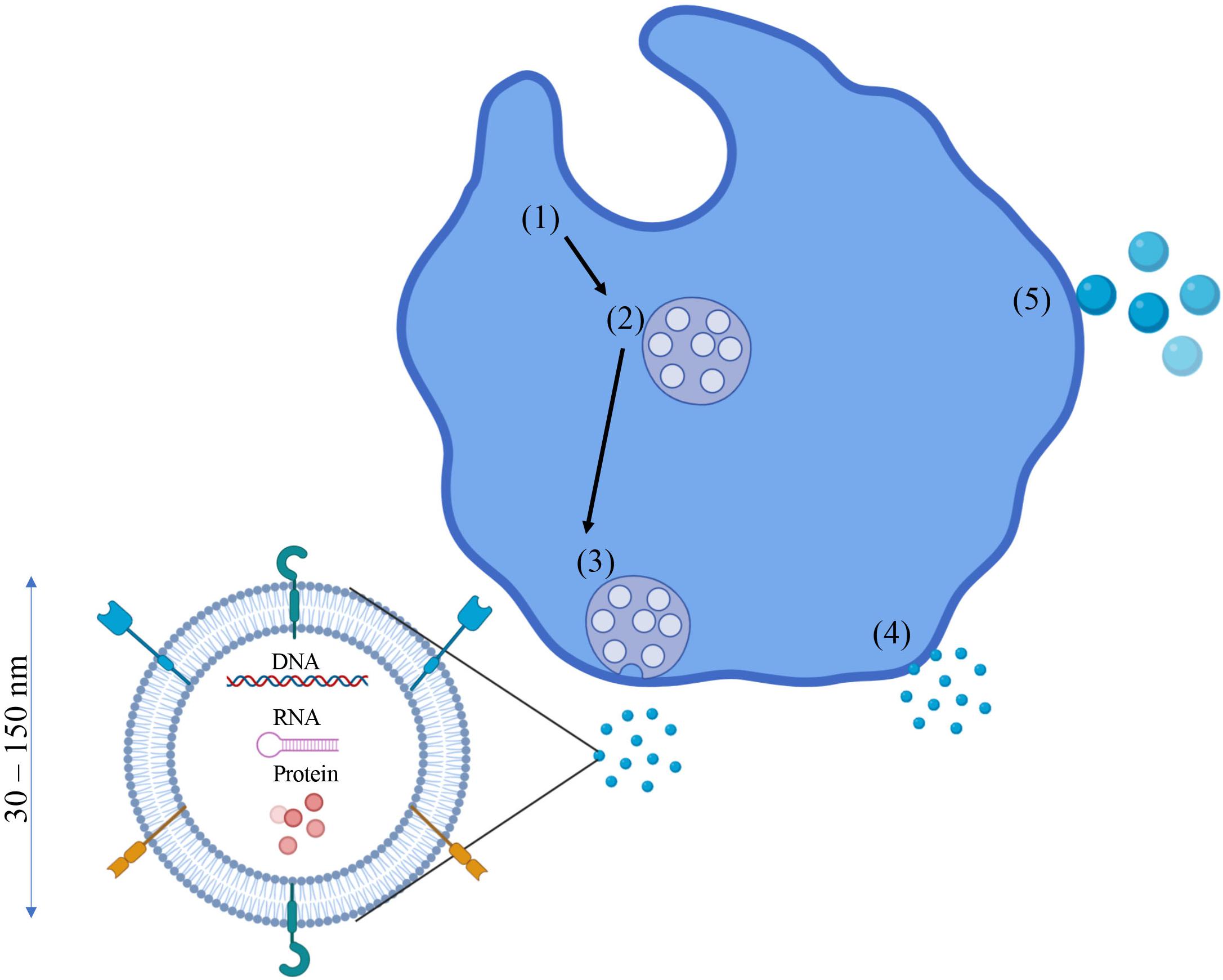
Figure 1. Exosomes: Formation, cargo, and characteristics. Exosome formation typically begins as endosomes (1) begin to bud inward and form multi-vesicular bodies (MVBs) (2). These MVBs then fuse with the plasma membrane (3) and release the exosomes into the intracellular space. However, the plasma membrane can also bleb off small EVs 30–150 nm in diameter which also fall in the same size classification as exosomes, so differentiating the two can be difficult (4). If these plasma membrane blebs are > 150 nm in diameter, they are classified as microvesicles or microparticles (5). Exosomes can contain a number of different molecules as cargo such as proteins/cytokines (free floating and membrane bound), DNA, RNA, and other nucleic acids.
Potential Benefits and Challenges of Using MSC-Exosomes
Utilizing MSC-exosomes as a therapy has a number of advantages compared to using MSCs themselves. One advantage is that viability is not a concern with exosomes, as they are not cells. This makes exosomes potentially much easier to use post thaw. Indeed, there is preliminary evidence that the thawing process may alter exosomal membranes so that they are absorbed more easily by target cells, although more research is needed to confirm this finding (Cheng et al., 2019). In one report, familial patients who received autologous adipose tissue-derived MSCs all had incidences of pulmonary embolisms related to the infusion (Jung et al., 2013). As MSC-exosomes are not a cellular product, there should be no risk to potential patients of developing pulmonary embolisms. Exosomes can also cross the blood–brain barrier, while MSCs cannot, making MSC-exosomes an attractive potential therapy option for various degenerative brain disorders (Chen et al., 2016). Just as with any novel therapy, there are challenges that need to be addressed before MSC-exosomes can be used in the clinic. First and foremost there needs to be a method of selecting suitable donors for the production of the MSCs that are used to produce exosomes, as well as the development of an exosome isolation protocol that meets good manufacturing practice (GMP) standards. Additionally, a consistent release criterion (size, surface marker expression, cargo, etc.) needs to be established for the exosomes themselves before they can be infused into potential patients. Depending on the condition being treated, markers need to be identified that distinguish functional from non-functional exosomes. Also, the optimal dose of MSC-exosomes for humans is unknown, which would need to be determined for each condition being treated. Furthermore, the best route of administration (local versus systemic) is unclear, as is the length of time MSC-exosomes remain in the patient before they are cleared by phagocytic cells. These challenges must be overcome and standards must be defined before patients can be tested for the immunomodulatory and regenerative capabilities of MSC-exosomes.
MSC Derived Exosomes for the Treatment of ARS
Acute radiation syndrome is caused by a high dose of ionizing radiation (>1 GY) over a short period of time (Lopez and Martin, 2011). The most severe side effects of ARS occur due to damage in highly proliferative cells found in the skin, the gastrointestinal tract, and the bone marrow (Heslet et al., 2012). Loss of bone marrow progenitor cells places patients at high risk for infections, as they can no longer produce leukocytes (Dainiak, 2018). The current standard of care for ARS involves the use of supportive care measures such as prophylactic antibiotics, blood and platelet transfusions, and the growth factors granulocyte colony-stimulating factor (G-CSF) and granulocyte-macrophage colony-stimulating factor (GM-CSF) (Waselenko et al., 2004; Gourmelon et al., 2010) which are FDA-approved to treat ARS. These interventions can keep patients alive and provide valuable time to patients who are waiting for an allogeneic hematopoietic stem cell transplant (HSCT) (Weisdorf et al., 2006). However, this process can take several weeks, during which time the patient may die from the initial exposure event. Even if the patient successfully receives a HSCT, this procedure comes with its own set of risks such as engraftment failure and GVHD (Ghimire et al., 2017; Ozdemir and Civriz Bozdag, 2018).
Most cases of ARS are seen in nuclear power plant employees upon accidental exposure resulting from incidents occurring at the plants such as those seen at Chernobyl and Fukushima (Mettler et al., 2007; Cerezo and Macia, 2012). Increasing usage of medical isotopes like iodine-131 to treat cancer has resulted in patients needing to cryopreserve their own (autologous) hematopoietic stem cells prior to treatment so that the bone marrow can be rescued from the cancer treatment. However, due to the current proliferation of nuclear technology worldwide, a mass exposure event from a terrorist attack using an improvised nuclear device or from a nuclear warhead deployed as an act-of-war is also a possibility. ARS could also impact future astronauts as both government agencies like the National Aeronautics and Space Administration (NASA) and private companies push for human voyages back to the moon as well as to Mars. An event such as a solar flare could expose astronauts to a high dose of cosmic radiation (Chancellor et al., 2014). Possible sources of radiation exposure, as well as current treatment options are summarized in Figure 2. Due to the current standard of care for ARS as well as the increased risk of exposure events due to accidents at power plants, cancer treatments, political instability, or through the colonization of the inner solar system; a priority has been placed on the development of “off-the-shelf” cell-based therapies that seek to mitigate or even reverse the deleterious effects of ARS.
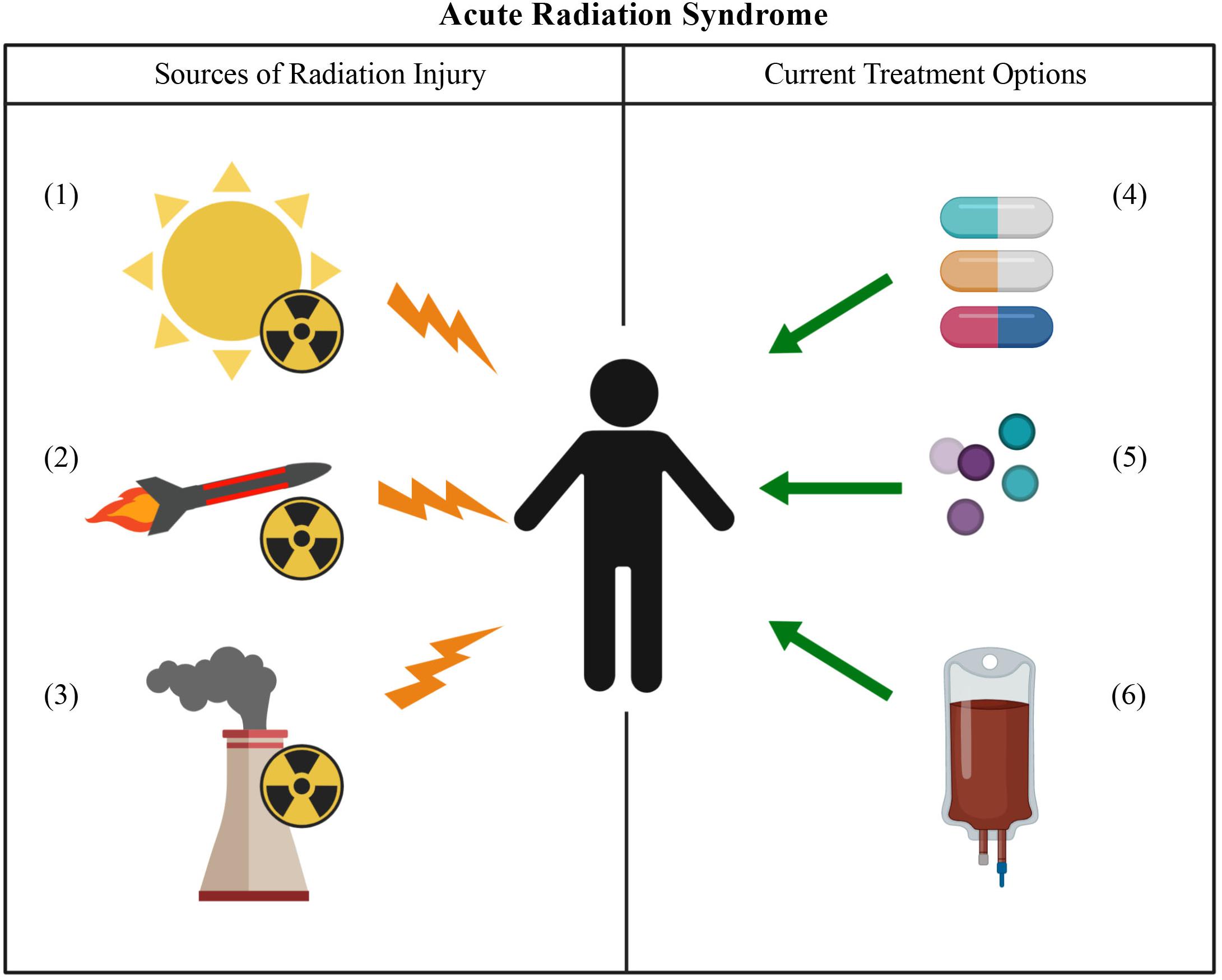
Figure 2. Acute radiation syndrome: sources of injury and current treatment options. Sources of radiation injury can include solar flares (1), nuclear weapons (2), or an accident at a nuclear power plant (3). The current treatment options for ARS involve the use of antibiotics (4) and the growth factors G-CSF and GM-CSF (5). In the more severe cases of ARS, an HSCT may need to be performed (6).
A number of preclinical studies have shown that MSCs can be used to reverse radiation damage seen in a variety of tissues, including the bone marrow (Fukumoto, 2016). The majority of preclinical work has been done utilizing bone marrow-derived MSCs for treatment of ARS (Lange et al., 2011; Yang et al., 2012). However, adipose tissue derived MSCs have also shown to prolong survival in irradiated mice, as well as enhance the reconstitution of hematopoietic cells (Cousin et al., 2003). Intramuscularly injected human placenta-derived MSCs have also shown the ability to enhance hematopoietic regeneration, reverse severe weight loss, and increase survival in lethally irradiated mice (Gaberman et al., 2013; Pinzur et al., 2018). Interestingly, in a study that exposed C57/BL6 mice to lethal irradiation followed by subsequent treatment with bone marrow-derived syngeneic MSCs within 24 h after exposure, the infused MSCs were cleared from the recipient mice within 3 days (Yang et al., 2012). This finding suggests that the protective effect seen in these mice was not due to the infused MSCs themselves, but rather through endogenous cells that were “educated” by the MSCs. One way in which these target cells could have been educated was by paracrine factors such as EVs produced from MSCs. Indeed, sublethally irradiated C57/BL6 lineage negative bone marrow cells (isolated 7 days after irradiation) showed an increased capacity to engraft in syngeneic recipient mice after being cultured with murine or human MSC-EVs (Wen et al., 2016).
Presently, only one clinical trial using allogeneic ex vivo expanded placental MSCs (PLX-R18, Pluristem Ltd.) is available for ARS, but is not yet recruiting (NCT03797040). However as stated above, it is possible that the benefits of MSCs in ARS may not be from MSCs themselves. Rather, the MSCs may be educating other immune cell subsets such as macrophages to mediate their radioprotective effects (Davies et al., 2017). Human MSC-educated macrophages, or “MEMs,” are a high interleukin (IL)-6 and IL-10 producing macrophage subset (Kim and Hematti, 2009) that are more effective than human MSCs alone in treating ARS in an immunocompromised xenogeneic model (Bouchlaka et al., 2017). In this study, MEMs expressed higher levels of inhibitory molecules such as PD-L1 and PD-L2, as well as molecules CD73 and arginase-1, when compared to untreated macrophages. Furthermore, MEMs were found to secrete higher levels of IL-6, while exhibiting a greater capacity to inhibit the proliferation of T-cells while promoting fibroblast proliferation as compared to untreated macrophages. IL-6 is typically considered to be a pro-inflammatory cytokine (Tanaka et al., 2014), although it has also been found in some instances to have anti-inflammatory effects as well as playing a role in tissue regeneration (Scheller et al., 2011; Galun and Rose-John, 2013). In fact, the presence of IL-6 has been associated with reduced inflammation caused by radiation induced injury (Bell et al., 2019). This increased secretion of IL-6 was enhanced when MEMs were “primed” with the TLR-4 ligand lipopolysaccharide (LPS) (Bouchlaka et al., 2017). The role of LPS in promoting the radio-protective effect of MEMs was investigated in a recent study, where LPS induced generation of MSC derived exosomes in a dose-dependent manner (Kink et al., 2019). In this study, human bone marrow-derived MSCs were primed with LPS for 24 h, after which time exosomes were harvested (Kink et al., 2019). The LPS-MSC exosomes (and unprimed MSC exosomes) were then cultured with human macrophages for 3 days, followed by infusion into immunocompromised mice with ARS. Results from this study show that the LPS-primed MSC exosome educated macrophages (LPS EEMs) significantly prolonged survival from ARS, enhanced hematopoietic recovery (by complete blood count and histologic analysis of bone marrow and spleen), and increased phagocytic capacity compared to unprimed MSC exosome educated macrophages (EEMs). The mechanism behind this protective effect is currently unknown, although there are a few intriguing avenues to investigate. The protection may be due to the effects of the increased levels of IL-6 or enhanced expression of PD-L1 found on the surface of the LPS EEMs. This immunomodulatory environment could possibly facilitate the reconstitution of the host mouse bone marrow. In order to investigate these possibilities, researchers could perform a similar study to those mentioned above, except for the inclusion of the administration of IL-6 and/or PD-L1 blocking monoclonal antibodies and observe how the radio-protective effect of the LPS-EEMs is altered. Furthermore, the use of LPS causes some concerns in a clinical setting, as LPS is toxic to humans (Stewart et al., 2006). Therefore, it would be prudent to perform similar experiments using synthetic LPS analogs in place of LPS.
MSC Derived Exosomes for the Treatment of GVHD and COVID-19
Due to their immunomodulatory capabilities, MSC-exosomes are an intriguing potential therapy for GVHD. Similar to ARS, GVHD is characterized by damaging proinflammatory responses that affect multiple organs. Immunosuppression with corticosteroids remains the most common treatment for both acute and chronic GVHD, but with sustained response rates around 40–50%, more effective approaches are needed (Garnett et al., 2013). Recent preclinical studies have investigated the use of human MSC-exosomes in mice with acute GVHD. Both umbilical cord derived MSC-exosomes and BM derived MSC exosomes prolonged survival of mice with GVHD compared to controls (Wang L. et al., 2016; Fujii et al., 2018). Furthermore, these studies showed a lower number of T cells, impaired T cell proliferation, and lower levels of proinflammatory cytokines IL-2, TNF-α, and IFN-γ in MSC-exosome treated mice (Wang L. et al., 2016; Fujii et al., 2018). Human BM derived MSC-exosomes have also shown efficacy in mouse models of chronic GVHD. In this study, treated mice showed improved survival, diminished clinical scores, reduced fibrosis in the skin, lung and liver, inhibition of Th17 cells, and induction of IL-10 expressing regulatory cells (Lai et al., 2018). Interestingly, BM derived MSC-exosomes have been given to a human patient with severe therapy-resistant GVHD as an individualized compassionate use treatment (Kordelas et al., 2014). The patient responded well to the therapy, as patient PBMCs showed decreased production of IL-1β, TNF-α, and IFN-γ after the third infusion (Kordelas et al., 2014). Additionally, clinical GVHD symptoms improved significantly, which allowed for a reduced dosage of steroids (Kordelas et al., 2014). The patient remained stable for months before eventually dying 7 months later due to pneumonia (Kordelas et al., 2014). A more recent finding, although not GVHD related, also shows the impact that MSC-exosomes have on reducing inflammatory responses; 24 severe COVID-19 patients were given a dose of ExoFloTM (a BM derived MSC-exosome product) at a single hospital center (Sengupta et al., 2020). Of these 24 patients, 17 fully recovered, three died, and three remain in intensive care at the time of publication (Sengupta et al., 2020). There were no adverse reactions to ExoFlowTM seen in any of the patients (Sengupta et al., 2020). Patients showed a significant decrease in neutrophil count, an increase in T lymphocyte count, reversal of hypoxia, and downregulation of cytokine storm (Sengupta et al., 2020). More clinical work is needed to determine if MSC-exosomes constitute an effective therapy for inflammatory diseases, but initial results are promising.
MSC Derived Exosomes for the Treatment of Cardiovascular Disease and Stroke
Cardiovascular diseases are the leading cause of morbidity and mortality worldwide. One major cause/result of these conditions is the death of cardiomyocytes and the subsequent loss of tissue remodeling capabilities (Olivetti et al., 1996). Cell-based therapies, including MSCs, have been investigated as a potential therapeutic option to help replace the lost cardiomyocytes, and improve heart function (Golpanian et al., 2016; Majka et al., 2017). MSC-exosomes have also shown promise in promoting cardioprotection in a mouse model of myocardial ischemia/reperfusion (I/R) injury, evidenced by reduced infarct size in mice treated with purified MSC-exosomes (Lai et al., 2010). Similarly, in a rat model of myocardial ischemic injury, human umbilical cord MSC-exosomes were found to reduce cardiac cell fibrosis, suppress apoptosis, and promote proliferation (Zhao et al., 2015). In a different study, researchers found that this protective effect of MSC-exosomes was in part due to their ability to deliver miRNAs, specifically miR-19a (Yu et al., 2015). Enhanced myocardial viability has also been observed in an I/R injury mouse model, resulting from increased ATP levels and decreased oxidative stress seen in the heart tissue of mice treated with MSC-exosomes (Arslan et al., 2013). Like cardiovascular disease, stroke is one of the leading causes of death and disability. MSC therapy has shown preclinical and clinical success in promoting recovery from stroke (Zhang and Chopp, 2016), and MSC-exosomes have shown promise in various preclinical models of stroke recovery. In rat models of traumatic brain injury (TBI), administration of MSC-exosomes has been shown to enhance neurogenesis and angiogenesis, and improve spatial learning and sensorimotor functional recovery (Xin et al., 2013a; Zhang et al., 2015). One potential mechanism of the enhanced recovery from TBI is the delivery of the miRNA miR-133b, as MSC-exosomes with elevated miR-133b led to improved axonal remodeling and neurological function when compared to standard MSC-exosomes (Xin et al., 2013b). MSC-exosomes promote tissue repair/remodeling in a number of preclinical disease models in addition to cardiovascular disease/stroke, and provide an exciting potential therapy for patients who suffer from these debilitating conditions.
Implementation of Cell-Based Therapies for ARS
Considerations on how MSCs/MSC-exosomes can be best utilized need to be taken into account on a condition by condition basis. Here, ARS is used as an example to explore what hurdles need to be cleared before MSCs/MSC-exosomes can be used as a treatment for this condition. Cell-based treatment strategies for ARS need to be safe and efficacious in the event of a potentially lethal radiation exposure. To achieve this goal, a number of logistical challenges need to be taken into account. In order to outline the nature of these challenges, and develop strategies to overcome them, the National Institute of Allergy and Infectious Diseases (NIAID) co-sponsored an international workshop in July 2015 in Paris, France, with the Institut de Radioprotection et de Sûreté Nucléaire. A report on this workshop was published in Radiation Research (DiCarlo et al., 2017). In this report, the authors summarize the numerous regulatory hurdles that need to be cleared in order for these cell-based therapies to be approved for human use. Of particular interest was the potential of MSC produced paracrine factors, particularly exosomes, to treat the effects of radiation exposure (Tran et al., 2013). However, the report stated that both cell-based therapies as well as exosomes alone could be used in a mass exposure event (DiCarlo et al., 2017). Patients who were exposed to higher levels of radiation could receive MSCs or MSC exosome educated cells, while the larger number of patients exposed to lower radiation levels (who still have surviving hematopoietic stem cells) could receive MSC exosomes alone to help boost the capacity of their own bone marrow to replenish itself. Importantly, in both of these scenarios, the patient would be receiving an “off-the-shelf” thawed product, so the development of a consistent and effective post-thaw procedure prior to infusion is of upmost importance. Cell-based therapies would likely be stored in a cryopreserved state at a few centralized locations. Therefore, therapeutic cells would need to show efficacy up to 24 or 48 h after the initial radiation exposure in order to account for the time it would take for the product to reach the patient.
Conclusion
Mesenchymal stem/stromal cell-exosomes are an emerging treatment for a variety of inflammatory and degenerative conditions, and are beginning to be translated from preclinical models into early phase clinical trials. For ARS, not only will therapies like MSCs, MSC-exosomes, and MSC-educated/MSC exosome-educated macrophages need to be tested for safety and efficacy, but they will also need to retain their function after cryopreservation/thawing so that supplies could be added to a National Stockpile or be transported on a space shuttle. Testing these cell-based options will either require clinical trials in patients with cancer receiving molecularly targeted radioactive therapies that show hematopoietic toxicity requiring growth factors, transfusions or HSCT, or become approved through mechanisms that bypass testing in patients like the FDA two-animal rule (Singh and Olabisi, 2017). Likewise, biomanufacturing standards need to be defined and standardized release criteria need to be developed for MSC-exosomes as they are used to treat other inflammatory conditions such as GVHD or COVID-19, as well as degenerative conditions such as cardiovascular disease and stroke. Depending on the indication, different potency assays may need to be used to verify anti-inflammatory versus tissue regenerative properties of MSC-exosomes. For these milestones to be met, increasing support from government agencies like NIAID, Department of Defense, NASA, and FDA will be needed to insure successful biomanufacturing of MSC-exosomes.
Author Contributions
MF drafted the manuscript. JK, PH, and CC revised the manuscript. All authors approved the final version of the manuscript.
Funding
This work was supported in part by the Don Anderson GVHD fund and Crystal Carney Fund for Leukemia Research (PH), St. Baldrick’s-Stand Up To Cancer Pediatric Dream Team Translational Research Grant SU2C-AACR-DT-27-17, NIH/NCATS UL1TR000427 to the UW ICTR and NIH/NCI P30 CA014520 to the UWCCC (PH and CC), and NIH/NCI R01 CA215461 (CC). Stand Up To Cancer is a division of the Entertainment Industry Foundation. Research grants are administered by the American Association for Cancer Research, the scientific partner of SU2C. The contents of this article do not necessarily reflect the views or policies of the Department of Health and Human Services, nor does mention of trade names, commercial products, or organizations imply endorsement by the US Government. None of these funding sources had any input in the study design, analysis, manuscript preparation, or decision to submit for publication.
Conflict of Interest
PH and CC are inventors on patents related to this publication. CC reports honorarium from Nektar Therapeutics, who had no input in the study design, analysis, manuscript preparation, or decision to submit for publication.
The remaining authors declare that the research was conducted in the absence of any commercial or financial relationships that could be construed as a potential conflict of interest.
References
Ahn, S. Y., Chang, Y. S., Kim, J. H., Sung, S. I., and Park, W. S. (2017). Two-year follow-up outcomes of premature infants enrolled in the phase I trial of mesenchymal stem cells transplantation for bronchopulmonary dysplasia. J. Pediatr. 185, 49–54.e2. doi: 10.1016/j.jpeds.2017.02.061
Al Demour, S., Jafar, H., Adwan, S., Alsharif, A., Alhawari, H., Alrabadi, A., et al. (2018). Safety and potential therapeutic effect of two intracavernous autologous bone marrow derived mesenchymal stem cells injections in diabetic patients with erectile dysfunction: an open label phase I clinical trial. Urol. Int. 101, 358–365. doi: 10.1159/000492120
Arslan, F., Lai, R. C., Smeets, M. B., Akeroyd, L., Choo, A., Aguor, E. N., et al. (2013). Mesenchymal stem cell-derived exosomes increase ATP levels, decrease oxidative stress and activate PI3K/Akt pathway to enhance myocardial viability and prevent adverse remodeling after myocardial ischemia/reperfusion injury. Stem Cell Res. 10, 301–312. doi: 10.1016/j.scr.2013.01.002
Bang, O. Y., Lee, J. S., Lee, P. H., and Lee, G. (2005). Autologous mesenchymal stem cell transplantation in stroke patients. Ann. Neurol. 57, 874–882. doi: 10.1002/ana.20501
Bartolucci, J., Verdugo, F. J., Gonzalez, P. L., Larrea, R. E., Abarzua, E., Goset, C., et al. (2017). Safety and efficacy of the intravenous infusion of umbilical cord mesenchymal stem cells in patients with heart failure: a phase 1/2 randomized controlled trial (RIMECARD trial [Randomized Clinical Trial of Intravenous Infusion Umbilical Cord Mesenchymal Stem Cells on Cardiopathy]). Circ. Res. 121, 1192–1204. doi: 10.1161/circresaha.117.310712
Bebelman, M. P., Smit, M. J., Pegtel, D. M., and Baglio, S. R. (2018). Biogenesis and function of extracellular vesicles in cancer. Pharmacol. Ther. 188, 1–11. doi: 10.1016/j.pharmthera.2018.02.013
Bell, B. I., Koduri, S., Salas Salinas, C., Monslow, J., Puré, E., Ben-Josef, E., et al. (2019). Interleukin 6 signaling blockade exacerbates acute and late injury from focal intestinal irradiation. Int J. Radiat. Oncol. Biol. Phys. 103, 719–727. doi: 10.1016/j.ijrobp.2018.10.007
Bernardo, M. E., Pagliara, D., and Locatelli, F. (2012). Mesenchymal stromal cell therapy: a revolution in Regenerative Medicine? Bone Marrow Transplant. 47, 164–171. doi: 10.1038/bmt.2011.81
Berry, J. D., Cudkowicz, M. E., Windebank, A. J., Staff, N. P., Owegi, M., Nicholson, K., et al. (2019). NurOwn, phase 2, randomized, clinical trial in patients with ALS: safety, clinical, and biomarker results. Neurology 93, e2294–e2305. doi: 10.1212/wnl.0000000000008620
Bhansali, S., Dutta, P., Kumar, V., Yadav, M. K., Jain, A., Mudaliar, S., et al. (2017). Efficacy of autologous bone marrow-derived mesenchymal stem cell and mononuclear cell transplantation in type 2 diabetes mellitus: a randomized. Placebo-Controlled Comparative Study. Stem Cells Dev. 26, 471–481. doi: 10.1089/scd.2016.0275
Bonab, M. M., Sahraian, M. A., Aghsaie, A., Karvigh, S. A., Hosseinian, S. M., Nikbin, B., et al. (2012). Autologous mesenchymal stem cell therapy in progressive multiple sclerosis: an open label study. Curr. Stem Cell Res. Ther. 7, 407–414. doi: 10.2174/157488812804484648
Bouchlaka, M. N., Moffitt, A. B., Kim, J., Kink, J. A., Bloom, D. D., Love, C., et al. (2017). Human mesenchymal stem cell-educated macrophages are a distinct high IL-6-producing subset that confer protection in graft-versus-host-disease and radiation injury models. Biol. Blood Marrow Transplant. 23, 897–905. doi: 10.1016/j.bbmt.2017.02.018
Braun, R. K., Chetty, C., Balasubramaniam, V., Centanni, R., Haraldsdottir, K., Hematti, P., et al. (2018). Intraperitoneal injection of MSC-derived exosomes prevent experimental bronchopulmonary dysplasia. Biochem. Biophys. Res. Commun. 503, 2653–2658. doi: 10.1016/j.bbrc.2018.08.019
Butler, J., Epstein, S. E., Greene, S. J., Quyyumi, A. A., Sikora, S., Kim, R. J., et al. (2017). Intravenous allogeneic mesenchymal stem cells for nonischemic cardiomyopathy: safety and efficacy results of a phase II-A randomized trial. Circ. Res. 120, 332–340. doi: 10.1161/circresaha.116.309717
Cai, J., Wu, Z., Xu, X., Liao, L., Chen, J., Huang, L., et al. (2016). Umbilical cord mesenchymal stromal cell with autologous bone marrow cell transplantation in established type 1 diabetes: a pilot randomized controlled open-label clinical study to assess safety and impact on insulin secretion. Diabetes Care 39, 149–157. doi: 10.2337/dc15-0171
Casado, S., Lobo, M. D. V. T., and Paíno, C. L. (2017). Dynamics of plasma membrane surface related to the release of extracellular vesicles by mesenchymal stem cells in culture. Sci. Rep. 7:6767.
Cerezo, L., and Macia, I. G. M. (2012). Acute radiation syndrome and Fukushima: A watershed moment? Rep. Pract. Oncol. Radiother. 17, 1–3. doi: 10.1016/j.rpor.2012.01.001
Chabannes, D., Hill, M., Merieau, E., Rossignol, J., Brion, R., Soulillou, J. P., et al. (2007). A role for heme oxygenase-1 in the immunosuppressive effect of adult rat and human mesenchymal stem cells. Blood 110, 3691–3694. doi: 10.1182/blood-2007-02-075481
Chamberlain, C. S., Clements, A. E. B., Kink, J. A., Choi, U., Baer, G. S., Halanski, M. A., et al. (2019). Extracellular vesicle-educated macrophages promote early Achilles tendon healing. Stem Cells 37, 652–662. doi: 10.1002/stem.2988
Chancellor, J. C., Scott, G. B., and Sutton, J. P. (2014). Space radiation: the number one risk to astronaut health beyond low earth orbit. Life 4, 491–510. doi: 10.3390/life4030491
Chang, Y. S., Ahn, S. Y., Yoo, H. S., Sung, S. I., Choi, S. J., Oh, W. I., et al. (2014). Mesenchymal stem cells for bronchopulmonary dysplasia: phase 1 dose-escalation clinical trial. J. Pediatr. 164, 966–972.e6. doi: 10.1016/j.jpeds.2013.12.011
Chen, C. C., Liu, L., Ma, F., Wong, C. W., Guo, X. E., Chacko, J. V., et al. (2016). Elucidation of exosome migration across the blood-brain barrier model in vitro. Cell. Mol. Bioeng. 9, 509–529. doi: 10.1007/s12195-016-0458-3
Chen, S., Zhao, K., Lin, R., Wang, S., Fan, Z., Huang, F., et al. (2019). The efficacy of mesenchymal stem cells in bronchiolitis obliterans syndrome after allogeneic HSCT: a multicenter prospective cohort study. EBioMedicine 49, 213–222. doi: 10.1016/j.ebiom.2019.09.039
Cheng, Y., Zeng, Q., Han, Q., and Xia, W. (2019). Effect of pH, temperature and freezing-thawing on quantity changes and cellular uptake of exosomes. Protein Cell 10, 295–299. doi: 10.1007/s13238-018-0529-4
Chin, S. P., Poey, A. C., Wong, C. Y., Chang, S. K., Tan, C. S., Ng, M. T., et al. (2011). Intramyocardial and intracoronary autologous bone marrow-derived mesenchymal stromal cell treatment in chronic severe dilated cardiomyopathy. Cytotherapy 13, 814–821. doi: 10.3109/14653249.2011.574118
Corcione, A., Benvenuto, F., Ferretti, E., Giunti, D., Cappiello, V., Cazzanti, F., et al. (2006). Human mesenchymal stem cells modulate B-cell functions. Blood 107, 367–372.
Cousin, B., Andre, M., Arnaud, E., Penicaud, L., and Casteilla, L. (2003). Reconstitution of lethally irradiated mice by cells isolated from adipose tissue. Biochem. Biophys. Res. Commun. 301, 1016–1022. doi: 10.1016/s0006-291x(03)00061-5
Cutler, A. J., Limbani, V., Girdlestone, J., and Navarrete, C. V. (2010). Umbilical cord-derived mesenchymal stromal cells modulate monocyte function to suppress T cell proliferation. J. Immunol. 185, 6617–6623. doi: 10.4049/jimmunol.1002239
Dainiak, N. (2018). Medical management of acute radiation syndrome and associated infections in a high-casualty incident. J. Radiat. Res. 59, ii54–ii64. doi: 10.1093/jrr/rry004
D’Asti, E., Garnier, D., Lee, T. H., Montermini, L., Meehan, B., and Rak, J. (2012). Oncogenic extracellular vesicles in brain tumor progression. Front. Physiol. 3:294. doi: 10.3389/fphys.2012.00294
Davatchi, F., Abdollahi, B. S., Mohyeddin, M., Shahram, F., and Nikbin, B. (2011). Mesenchymal stem cell therapy for knee osteoarthritis. Preliminary report of four patients. Int. J. Rheum. Dis. 14, 211–215. doi: 10.1111/j.1756-185x.2011.01599.x
Davatchi, F., Sadeghi Abdollahi, B., Mohyeddin, M., and Nikbin, B. (2016). Mesenchymal stem cell therapy for knee osteoarthritis: 5 years follow-up of three patients. Int. J. Rheum. Dis. 19, 219–225. doi: 10.1111/1756-185x.12670
Davies, L. C., Boberg, E., and Le Blanc, K. (2017). Commentary: role of mesenchymal stromal cell-mediated crosstalk with macrophages in graft-versus-host disease and tissue repair. Biol. Blood Marrow Transplant. 23, 861–862. doi: 10.1016/j.bbmt.2017.04.006
de Araujo Farias, V., Carrillo-Galvez, A. B., Martin, F., and Anderson, P. (2018). TGF-beta and mesenchymal stromal cells in regenerative medicine, autoimmunity and cancer. Cytokine Growth Factor Rev. 43, 25–37. doi: 10.1016/j.cytogfr.2018.06.002
de Oliveira, H. G., Cruz, F. F., Antunes, M. A., De Macedo Neto, A. V., Oliveira, G. A., Svartman, F. M., et al. (2017). Combined bone marrow-derived mesenchymal stromal cell therapy and one-way endobronchial valve placement in patients with pulmonary emphysema: a phase i clinical trial. Stem Cells Transl. Med. 6, 962–969. doi: 10.1002/sctm.16-0315
DiCarlo, A. L., Tamarat, R., Rios, C. I., Benderitter, M., Czarniecki, C. W., Allio, T. C., et al. (2017). Cellular therapies for treatment of radiation injury: report from a NIH/NIAID and IRSN workshop. Radiat. Res. 188, e54–e75. doi: 10.1667/rr14810.1
Emadedin, M., Labibzadeh, N., Liastani, M. G., Karimi, A., Jaroughi, N., Bolurieh, T., et al. (2018). Intra-articular implantation of autologous bone marrow-derived mesenchymal stromal cells to treat knee osteoarthritis: a randomized, triple-blind, placebo-controlled phase 1/2 clinical trial. Cytotherapy 20, 1238–1246. doi: 10.1016/j.jcyt.2018.08.005
Engela, A. U., Baan, C. C., Litjens, N. H., Franquesa, M., Betjes, M. G., Weimar, W., et al. (2013). Mesenchymal stem cells control alloreactive CD8(+) CD28(-) T cells. Clin. Exp. Immunol. 174, 449–458. doi: 10.1111/cei.12199
Eslani, M., Putra, I., Shen, X., Hamouie, J., Tadepalli, A., Anwar, K. N., et al. (2018). Cornea-derived mesenchymal stromal cells therapeutically modulate macrophage immunophenotype and angiogenic function. Stem Cells 36, 775–784. doi: 10.1002/stem.2781
Falanga, V., Iwamoto, S., Chartier, M., Yufit, T., Butmarc, J., Kouttab, N., et al. (2007). Autologous bone marrow-derived cultured mesenchymal stem cells delivered in a fibrin spray accelerate healing in murine and human cutaneous wounds. Tissue Eng. 13, 1299–1312. doi: 10.1089/ten.2006.0278
Fang, S., Xu, C., Zhang, Y., Xue, C., Yang, C., Bi, H., et al. (2016). Umbilical cord-derived mesenchymal stem cell-derived exosomal microRNAs suppress myofibroblast differentiation by inhibiting the transforming growth factor-beta/SMAD2 pathway during wound healing. Stem Cells Transl. Med. 5, 1425–1439. doi: 10.5966/sctm.2015-0367
Fitzgerald, W., Freeman, M. L., Lederman, M. M., Vasilieva, E., Romero, R., and Margolis, L. (2018). A system of cytokines encapsulated in ExtraCellular vesicles. Sci. Rep. 8:8973.
Friis, T., Haack-Sorensen, M., Mathiasen, A. B., Ripa, R. S., Kristoffersen, U. S., Jorgensen, E., et al. (2011). Mesenchymal stromal cell derived endothelial progenitor treatment in patients with refractory angina. Scand. Cardiovasc. J. 45, 161–168. doi: 10.3109/14017431.2011.569571
Fujii, S., Miura, Y., Fujishiro, A., Shindo, T., Shimazu, Y., Hirai, H., et al. (2018). Graft-versus-host disease amelioration by human bone marrow mesenchymal stromal/stem cell-derived extracellular vesicles is associated with peripheral preservation of naive T cell populations. Stem Cells 36, 434–445. doi: 10.1002/stem.2759
Fukumoto, R. (2016). Mesenchymal stem cell therapy for acute radiation syndrome. Mil. Med. Res. 3:17.
Gaberman, E., Pinzur, L., Levdansky, L., Tsirlin, M., Netzer, N., Aberman, Z., et al. (2013). Mitigation of lethal radiation syndrome in mice by intramuscular injection of 3D cultured adherent human placental stromal cells. PLoS One 8:e66549. doi: 10.1371/journal.pone.0066549
Galun, E., and Rose-John, S. (2013). The regenerative activity of interleukin-6. Methods Mol. Biol. 982, 59–77. doi: 10.1007/978-1-62703-308-4_4
Gao, L., Zhang, Y., Hu, B., Liu, J., Kong, P., Lou, S., et al. (2016). Phase II multicenter, randomized, double-blind controlled study of efficacy and safety of umbilical cord-derived mesenchymal stromal cells in the prophylaxis of chronic graft-versus-host disease after HLA-Haploidentical stem-cell transplantation. J. Clin. Oncol. 34, 2843–2850. doi: 10.1200/jco.2015.65.3642
Garnett, C., Apperley, J. F., and Pavlù, J. (2013). Treatment and management of graft-versus-host disease: improving response and survival. Ther. Adv. Hematol. 4, 366–378. doi: 10.1177/2040620713489842
Ghimire, S., Weber, D., Mavin, E., Wang, X. N., Dickinson, A. M., and Holler, E. (2017). Pathophysiology of GvHD and other HSCT-related major complications. Front. Immunol. 8:79. doi: 10.3389/fimmu.2017.00079
Ghoryani, M., Shariati-Sarabi, Z., Tavakkol-Afshari, J., Ghasemi, A., Poursamimi, J., and Mohammadi, M. (2019). Amelioration of clinical symptoms of patients with refractory rheumatoid arthritis following treatment with autologous bone marrow-derived mesenchymal stem cells: a successful clinical trial in Iran. Biomed. Pharmacother. 109, 1834–1840. doi: 10.1016/j.biopha.2018.11.056
Golpanian, S., El-Khorazaty, J., Mendizabal, A., Difede, D. L., Suncion, V. Y., Karantalis, V., et al. (2015). Effect of aging on human mesenchymal stem cell therapy in ischemic cardiomyopathy patients. J. Am. Coll. Cardiol. 65, 125–132. doi: 10.1016/j.jacc.2014.10.040
Golpanian, S., Wolf, A., Hatzistergos, K. E., and Hare, J. M. (2016). Rebuilding the damaged heart: mesenchymal stem cells, cell-based therapy, and engineered heart tissue. Physiol. Rev. 96, 1127–1168. doi: 10.1152/physrev.00019.2015
Gotherstrom, C., Westgren, M., Shaw, S. W., Astrom, E., Biswas, A., Byers, P. H., et al. (2014). Pre- and postnatal transplantation of fetal mesenchymal stem cells in osteogenesis imperfecta: a two-center experience. Stem Cells Transl. Med. 3, 255–264. doi: 10.5966/sctm.2013-0090
Gourmelon, P., Benderitter, M., Bertho, J. M., Huet, C., Gorin, N. C., and De Revel, P. (2010). European consensus on the medical management of acute radiation syndrome and analysis of the radiation accidents in Belgium and Senegal. Health Phys. 98, 825–832. doi: 10.1097/hp.0b013e3181ce64d4
Haack-Sorensen, M., Friis, T., Mathiasen, A. B., Jorgensen, E., Hansen, L., Dickmeiss, E., et al. (2013). Direct intramyocardial mesenchymal stromal cell injections in patients with severe refractory angina: one-year follow-up. Cell Transplant. 22, 521–528. doi: 10.3727/096368912x636830
Hare, J. M., Fishman, J. E., Gerstenblith, G., Difede Velazquez, D. L., Zambrano, J. P., Suncion, V. Y., et al. (2012). Comparison of allogeneic vs autologous bone marrow-derived mesenchymal stem cells delivered by transendocardial injection in patients with ischemic cardiomyopathy: the POSEIDON randomized trial. JAMA 308, 2369–2379.
Hare, J. M., Traverse, J. H., Henry, T. D., Dib, N., Strumpf, R. K., Schulman, S. P., et al. (2009). A randomized, double-blind, placebo-controlled, dose-escalation study of intravenous adult human mesenchymal stem cells (prochymal) after acute myocardial infarction. J. Am. Coll. Cardiol. 54, 2277–2286. doi: 10.1016/j.jacc.2009.06.055
Harris, V. K., Stark, J., Vyshkina, T., Blackshear, L., Joo, G., Stefanova, V., et al. (2018). Phase I trial of intrathecal mesenchymal stem cell-derived neural progenitors in progressive multiple sclerosis. EBioMedicine 29, 23–30. doi: 10.1016/j.ebiom.2018.02.002
Hass, R., Kasper, C., Böhm, S., and Jacobs, R. (2011). Different populations and sources of human mesenchymal stem cells (MSC): a comparison of adult and neonatal tissue-derived MSC. Cell Commun. Signal. 9:12.
Helwa, I., Cai, J., Drewry, M. D., Zimmerman, A., Dinkins, M. B., Khaled, M. L., et al. (2017). A comparative study of serum exosome isolation using differential ultracentrifugation and three commercial reagents. PLoS One 12:e0170628. doi: 10.1371/journal.pone.0170628
Hernigou, P., Dubory, A., Homma, Y., Guissou, I., Flouzat Lachaniette, C. H., Chevallier, N., et al. (2018). Cell therapy versus simultaneous contralateral decompression in symptomatic corticosteroid osteonecrosis: a thirty year follow-up prospective randomized study of one hundred and twenty five adult patients. Int. Orthop. 42, 1639–1649. doi: 10.1007/s00264-018-3941-8
Heslet, L., Bay, C., and Nepper-Christensen, S. (2012). Acute radiation syndrome (ARS) - treatment of the reduced host defense. Int. J. Gen. Med. 5, 105–115.
Hessvik, N. P., and Llorente, A. (2018). Current knowledge on exosome biogenesis and release. Cell. Mol. Life Sci. 75, 193–208. doi: 10.1007/s00018-017-2595-9
Hlebokazov, F., Dakukina, T., Ihnatsenko, S., Kosmacheva, S., Potapnev, M., Shakhbazau, A., et al. (2017). Treatment of refractory epilepsy patients with autologous mesenchymal stem cells reduces seizure frequency: an open label study. Adv. Med. Sci. 62, 273–279. doi: 10.1016/j.advms.2016.12.004
Hsu, W. T., Lin, C. H., Chiang, B. L., Jui, H. Y., Wu, K. K., and Lee, C. M. (2013). Prostaglandin E2 potentiates mesenchymal stem cell-induced IL-10+IFN-gamma+CD4+ regulatory T cells to control transplant arteriosclerosis. J. Immunol. 190, 2372–2380. doi: 10.4049/jimmunol.1202996
Huang, J. H., Yin, X. M., Xu, Y., Xu, C. C., Lin, X., Ye, F. B., et al. (2017). Systemic administration of exosomes released from mesenchymal stromal cells attenuates apoptosis, inflammation, and promotes angiogenesis after spinal cord injury in rats. J. Neurotrauma 34, 3388–3396. doi: 10.1089/neu.2017.5063
Huang, L., Zhang, C., Gu, J., Wu, W., Shen, Z., Zhou, X., et al. (2018). A randomized, placebo-controlled trial of human umbilical cord blood mesenchymal stem cell infusion for children with cerebral palsy. Cell Transplant. 27, 325–334. doi: 10.1177/0963689717729379
Jiang, R., Han, Z., Zhuo, G., Qu, X., Li, X., Wang, X., et al. (2011). Transplantation of placenta-derived mesenchymal stem cells in type 2 diabetes: a pilot study. Front. Med. 5, 94–100. doi: 10.1007/s11684-011-0116-z
Jiang, X. X., Zhang, Y., Liu, B., Zhang, S. X., Wu, Y., Yu, X. D., et al. (2005). Human mesenchymal stem cells inhibit differentiation and function of monocyte-derived dendritic cells. Blood 105, 4120–4126. doi: 10.1182/blood-2004-02-0586
Joo, H. S., Suh, J. H., Lee, H. J., Bang, E. S., and Lee, J. M. (2020). Current knowledge and future perspectives on mesenchymal stem cell-derived exosomes as a new therapeutic agent. Int. J. Mol. Sci. 21:727. doi: 10.3390/ijms21030727
Jung, J. W., Kwon, M., Choi, J. C., Shin, J. W., Park, I. W., Choi, B. W., et al. (2013). Familial occurrence of pulmonary embolism after intravenous, adipose tissue-derived stem cell therapy. Yonsei Med. J. 54, 1293–1296.
Kahroba, H., Hejazi, M. S., and Samadi, N. (2019). Exosomes: from carcinogenesis and metastasis to diagnosis and treatment of gastric cancer. Cell. Mol. Life Sci. 76, 1747–1758. doi: 10.1007/s00018-019-03035-2
Karantalis, V., Difede, D. L., Gerstenblith, G., Pham, S., Symes, J., Zambrano, J. P., et al. (2014). Autologous mesenchymal stem cells produce concordant improvements in regional function, tissue perfusion, and fibrotic burden when administered to patients undergoing coronary artery bypass grafting: the Prospective Randomized Study of Mesenchymal Stem Cell Therapy in Patients Undergoing Cardiac Surgery (PROMETHEUS) trial. Circ. Res. 114, 1302–1310. doi: 10.1161/circresaha.114.303180
Karussis, D., Karageorgiou, C., Vaknin-Dembinsky, A., Gowda-Kurkalli, B., Gomori, J. M., Kassis, I., et al. (2010). Safety and immunological effects of mesenchymal stem cell transplantation in patients with multiple sclerosis and amyotrophic lateral sclerosis. Arch. Neurol. 67, 1187–1194.
Khalifeh Soltani, S., Forogh, B., Ahmadbeigi, N., Hadizadeh Kharazi, H., Fallahzadeh, K., Kashani, L., et al. (2019). Safety and efficacy of allogenic placental mesenchymal stem cells for treating knee osteoarthritis: a pilot study. Cytotherapy 21, 54–63. doi: 10.1016/j.jcyt.2018.11.003
Kim, H. S., Lee, J. H., Roh, K. H., Jun, H. J., Kang, K. S., and Kim, T. Y. (2017). Clinical trial of human umbilical cord blood-derived stem cells for the treatment of moderate-to-severe Atopic dermatitis: phase I/IIa studies. Stem Cells 35, 248–255. doi: 10.1002/stem.2401
Kim, J., and Hematti, P. (2009). Mesenchymal stem cell-educated macrophages: a novel type of alternatively activated macrophages. Exp. Hematol. 37, 1445–1453. doi: 10.1016/j.exphem.2009.09.004
Kim, S. H., Cho, J. H., Lee, Y. H., Lee, J. H., Kim, S. S., Kim, M. Y., et al. (2018). Improvement in left ventricular function with intracoronary mesenchymal stem cell therapy in a patient with anterior wall ST-segment elevation myocardial infarction. Cardiovasc. Drugs Ther. 32, 329–338. doi: 10.1007/s10557-018-6804-z
Kink, J. A., Forsberg, M. H., Reshetylo, S., Besharat, S., Childs, C. J., Pederson, J. D., et al. (2019). Macrophages educated with exosomes from primed mesenchymal stem cells treat acute radiation syndrome by promoting hematopoietic recovery. Biol. Blood Marrow Transplant. 25, 2124–2133. doi: 10.1016/j.bbmt.2019.07.026
Koc, O. N., Day, J., Nieder, M., Gerson, S. L., Lazarus, H. M., and Krivit, W. (2002). Allogeneic mesenchymal stem cell infusion for treatment of metachromatic leukodystrophy (MLD) and Hurler syndrome (MPS-IH). Bone Marrow Transplant. 30, 215–222. doi: 10.1038/sj.bmt.1703650
Koh, Y. G., and Choi, Y. J. (2012). Infrapatellar fat pad-derived mesenchymal stem cell therapy for knee osteoarthritis. Knee 19, 902–907. doi: 10.1016/j.knee.2012.04.001
Kordelas, L., Rebmann, V., Ludwig, A. K., Radtke, S., Ruesing, J., Doeppner, T. R., et al. (2014). MSC-derived exosomes: a novel tool to treat therapy-refractory graft-versus-host disease. Leukemia 28, 970–973. doi: 10.1038/leu.2014.41
Lai, P., Chen, X., Guo, L., Wang, Y., Liu, X., Liu, Y., et al. (2018). A potent immunomodulatory role of exosomes derived from mesenchymal stromal cells in preventing cGVHD. J. Hematol. Oncol. 11, 135–135.
Lai, R. C., Arslan, F., Lee, M. M., Sze, N. S., Choo, A., Chen, T. S., et al. (2010). Exosome secreted by MSC reduces myocardial ischemia/reperfusion injury. Stem Cell Res. 4, 214–222. doi: 10.1016/j.scr.2009.12.003
Lamo-Espinosa, J. M., Mora, G., Blanco, J. F., Granero-Molto, F., Nunez-Cordoba, J. M., Lopez-Elio, S., et al. (2018). Intra-articular injection of two different doses of autologous bone marrow mesenchymal stem cells versus hyaluronic acid in the treatment of knee osteoarthritis: long-term follow up of a multicenter randomized controlled clinical trial (phase I/II). J. Transl. Med. 16:213.
Lamo-Espinosa, J. M., Mora, G., Blanco, J. F., Granero-Molto, F., Nunez-Cordoba, J. M., Sanchez-Echenique, C., et al. (2016). Intra-articular injection of two different doses of autologous bone marrow mesenchymal stem cells versus hyaluronic acid in the treatment of knee osteoarthritis: multicenter randomized controlled clinical trial (phase I/II). J. Transl. Med. 14:246.
Lange, C., Brunswig-Spickenheier, B., Cappallo-Obermann, H., Eggert, K., Gehling, U. M., Rudolph, C., et al. (2011). Radiation rescue: mesenchymal stromal cells protect from lethal irradiation. PLoS One 6:e14486. doi: 10.1371/journal.pone.0014486
Lee, E. J., Hwang, I., Lee, J. Y., Park, J. N., Kim, K. C., Kim, G. H., et al. (2018). Hepatocyte growth factor improves the therapeutic efficacy of human bone marrow mesenchymal stem cells via RAD51. Mol. Ther. 26, 845–859. doi: 10.1016/j.ymthe.2017.12.015
Lee, J. S., Hong, J. M., Moon, G. J., Lee, P. H., Ahn, Y. H., Bang, O. Y., et al. (2010). A long-term follow-up study of intravenous autologous mesenchymal stem cell transplantation in patients with ischemic stroke. Stem Cells 28, 1099–1106. doi: 10.1002/stem.430
Lee, P. H., Kim, J. W., Bang, O. Y., Ahn, Y. H., Joo, I. S., and Huh, K. (2008). Autologous mesenchymal stem cell therapy delays the progression of neurological deficits in patients with multiple system atrophy. Clin. Pharmacol. Ther. 83, 723–730. doi: 10.1038/sj.clpt.6100386
Lee, P. H., Lee, J. E., Kim, H. S., Song, S. K., Lee, H. S., Nam, H. S., et al. (2012). A randomized trial of mesenchymal stem cells in multiple system atrophy. Ann. Neurol. 72, 32–40.
Li, J. F., Zhang, D. J., Geng, T., Chen, L., Huang, H., Yin, H. L., et al. (2014). The potential of human umbilical cord-derived mesenchymal stem cells as a novel cellular therapy for multiple sclerosis. Cell Transplant. 23(Suppl. 1), S113–S122.
Li, M., Sun, X., Kuang, X., Liao, Y., Li, H., and Luo, D. (2014). Mesenchymal stem cells suppress CD8+ T cell-mediated activation by suppressing natural killer group 2, member D protein receptor expression and secretion of prostaglandin E2, indoleamine 2, 3-dioxygenase and transforming growth factor-beta. Clin. Exp. Immunol. 178, 516–524. doi: 10.1111/cei.12423
Liang, J., Zhang, H., Zhao, C., Wang, D., Ma, X., Zhao, S., et al. (2017). Effects of allogeneic mesenchymal stem cell transplantation in the treatment of liver cirrhosis caused by autoimmune diseases. Int. J. Rheum. Dis. 20, 1219–1226. doi: 10.1111/1756-185x.13015
Lin, B. L., Chen, J. F., Qiu, W. H., Wang, K. W., Xie, D. Y., Chen, X. Y., et al. (2017). Allogeneic bone marrow-derived mesenchymal stromal cells for hepatitis B virus-related acute-on-chronic liver failure: a randomized controlled trial. Hepatology 66, 209–219. doi: 10.1002/hep.29189
Lopez, M., and Martin, M. (2011). Medical management of the acute radiation syndrome. Rep. Pract. Oncol. Radiother. 16, 138–146.
Majka, M., Sułkowski, M., Badyra, B., and Musiałek, P. (2017). Concise review: mesenchymal stem cells in cardiovascular regeneration: emerging research directions and clinical applications. Stem Cells Transl. Med. 6, 1859–1867. doi: 10.1002/sctm.16-0484
Mashouri, L., Yousefi, H., Aref, A. R., Ahadi, A. M., Molaei, F., and Alahari, S. K. (2019). Exosomes: composition, biogenesis, and mechanisms in cancer metastasis and drug resistance. Mol. Cancer 18:75.
Matas, J., Orrego, M., Amenabar, D., Infante, C., Tapia-Limonchi, R., Cadiz, M. I., et al. (2019). Umbilical cord-derived mesenchymal stromal cells (MSCs) for knee osteoarthritis: repeated MSC dosing is superior to a single MSC dose and to Hyaluronic acid in a controlled randomized phase I/II trial. Stem Cells Transl. Med. 8, 215–224. doi: 10.1002/sctm.18-0053
Mathiasen, A. B., Qayyum, A. A., Jorgensen, E., Helqvist, S., Fischer-Nielsen, A., Kofoed, K. F., et al. (2015). Bone marrow-derived mesenchymal stromal cell treatment in patients with severe ischaemic heart failure: a randomized placebo-controlled trial (MSC-HF trial). Eur. Heart J. 36, 1744–1753. doi: 10.1093/eurheartj/ehv136
Mathivanan, S., Ji, H., and Simpson, R. J. (2010). Exosomes: extracellular organelles important in intercellular communication. J. Proteomics 73, 1907–1920. doi: 10.1016/j.jprot.2010.06.006
Mettler, F. A. Jr., Gus’kova, A. K., and Gusev, I. (2007). Health effects in those with acute radiation sickness from the Chernobyl accident. Health Phys. 93, 462–469. doi: 10.1097/01.hp.0000278843.27969.74
Mohyeddin Bonab, M., Yazdanbakhsh, S., Lotfi, J., Alimoghaddom, K., Talebian, F., Hooshmand, F., et al. (2007). Does mesenchymal stem cell therapy help multiple sclerosis patients? Report of a pilot study. Iran. J. Immunol. 4, 50–57.
Musiał-Wysocka, A., Kot, M., and Majka, M. (2019). The pros and cons of mesenchymal stem cell-based therapies. Cell Transplant. 28, 801–812. doi: 10.1177/0963689719837897
Nakamura, Y., Miyaki, S., Ishitobi, H., Matsuyama, S., Nakasa, T., Kamei, N., et al. (2015). Mesenchymal-stem-cell-derived exosomes accelerate skeletal muscle regeneration. FEBS Lett. 589, 1257–1265. doi: 10.1016/j.febslet.2015.03.031
Nemeth, K., Leelahavanichkul, A., Yuen, P. S., Mayer, B., Parmelee, A., Doi, K., et al. (2009). Bone marrow stromal cells attenuate sepsis via prostaglandin E(2)-dependent reprogramming of host macrophages to increase their interleukin-10 production. Nat. Med. 15, 42–49. doi: 10.1038/nm.1905
Noriega, D. C., Ardura, F., Hernandez-Ramajo, R., Martin-Ferrero, M. A., Sanchez-Lite, I., Toribio, B., et al. (2017). Intervertebral disc repair by allogeneic mesenchymal bone marrow cells: a randomized controlled trial. Transplantation 101, 1945–1951. doi: 10.1097/tp.0000000000001484
Olivetti, G., Quaini, F., Sala, R., Lagrasta, C., Corradi, D., Bonacina, E., et al. (1996). Acute myocardial infarction in humans is associated with activation of programmed myocyte cell death in the surviving portion of the heart. J. Mol. Cell Cardiol. 28, 2005–2016. doi: 10.1006/jmcc.1996.0193
Orozco, L., Munar, A., Soler, R., Alberca, M., Soler, F., Huguet, M., et al. (2013). Treatment of knee osteoarthritis with autologous mesenchymal stem cells: a pilot study. Transplantation 95, 1535–1541. doi: 10.1097/tp.0b013e318291a2da
Orozco, L., Soler, R., Morera, C., Alberca, M., Sanchez, A., and Garcia-Sancho, J. (2011). Intervertebral disc repair by autologous mesenchymal bone marrow cells: a pilot study. Transplantation 92, 822–828. doi: 10.1097/tp.0b013e3182298a15
Ozdemir, Z. N., and Civriz Bozdag, S. (2018). Graft failure after allogeneic hematopoietic stem cell transplantation. Transfus. Apher. Sci. 57, 163–167.
Park, E. H., Lim, H. S., Lee, S., Roh, K., Seo, K. W., Kang, K. S., et al. (2018). intravenous infusion of umbilical cord blood-derived mesenchymal stem cells in rheumatoid arthritis: a phase Ia clinical trial. Stem Cells Transl. Med. 7, 636–642. doi: 10.1002/sctm.18-0031
Petrou, P., Gothelf, Y., Argov, Z., Gotkine, M., Levy, Y. S., Kassis, I., et al. (2016). Safety and clinical effects of mesenchymal stem cells secreting neurotrophic factor transplantation in patients with amyotrophic lateral sclerosis: results of phase 1/2 and 2a clinical trials. JAMA Neurol. 73, 337–344.
Pinzur, L., Akyuez, L., Levdansky, L., Blumenfeld, M., Volinsky, E., Aberman, Z., et al. (2018). Rescue from lethal acute radiation syndrome (ARS) with severe weight loss by secretome of intramuscularly injected human placental stromal cells. J. Cachexia Sarcopenia Muscle 9, 1079–1092. doi: 10.1002/jcsm.12342
Reddy, V. S., Madala, S. K., Trinath, J., and Reddy, G. B. (2018). Extracellular small heat shock proteins: exosomal biogenesis and function. Cell Stress Chaperones 23, 441–454. doi: 10.1007/s12192-017-0856-z
Reza-Zaldivar, E. E., Hernandez-Sapiens, M. A., Gutierrez-Mercado, Y. K., Sandoval-Avila, S., Gomez-Pinedo, U., Marquez-Aguirre, A. L., et al. (2019). Mesenchymal stem cell-derived exosomes promote neurogenesis and cognitive function recovery in a mouse model of Alzheimer’s disease. Neural Regen. Res. 14, 1626–1634.
Riazifar, M., Mohammadi, M. R., Pone, E. J., Yeri, A., Lasser, C., Segaliny, A. I., et al. (2019). Stem cell-derived exosomes as nanotherapeutics for autoimmune and neurodegenerative disorders. ACS Nano 13, 6670–6688. doi: 10.1021/acsnano.9b01004
Riordan, N. H., Morales, I., Fernandez, G., Allen, N., Fearnot, N. E., Leckrone, M. E., et al. (2018). Clinical feasibility of umbilical cord tissue-derived mesenchymal stem cells in the treatment of multiple sclerosis. J. Transl. Med. 16:57.
Saad, A., Dietz, A. B., Herrmann, S. M. S., Hickson, L. J., Glockner, J. F., Mckusick, M. A., et al. (2017). Autologous mesenchymal stem cells increase cortical perfusion in Renovascular disease. J. Am. Soc. Nephrol. 28, 2777–2785. doi: 10.1681/asn.2017020151
Samaeekia, R., Rabiee, B., Putra, I., Shen, X., Park, Y. J., Hematti, P., et al. (2018). Effect of human corneal mesenchymal stromal cell-derived exosomes on corneal epithelial wound healing. Invest. Ophthalmol. Vis. Sci. 59, 5194–5200.
Scheller, J., Chalaris, A., Schmidt-Arras, D., and Rose-John, S. (2011). The pro- and anti-inflammatory properties of the cytokine interleukin-6. Biochim. Biophys. Acta 1813, 878–888.
Sengupta, V., Sengupta, S., Lazo, A., Woods, P., Nolan, A., and Bremer, N. (2020). Exosomes derived from bone marrow mesenchymal stem cells as treatment for severe COVID-19. Stem Cells Dev. 29, 747–754. doi: 10.1089/scd.2020.0080
Shadmanfar, S., Labibzadeh, N., Emadedin, M., Jaroughi, N., Azimian, V., Mardpour, S., et al. (2018). Intra-articular knee implantation of autologous bone marrow-derived mesenchymal stromal cells in rheumatoid arthritis patients with knee involvement: results of a randomized, triple-blind, placebo-controlled phase 1/2 clinical trial. Cytotherapy 20, 499–506. doi: 10.1016/j.jcyt.2017.12.009
Shi, M., Liu, Z., Wang, Y., Xu, R., Sun, Y., Zhang, M., et al. (2017). A pilot study of mesenchymal stem cell therapy for acute liver allograft rejection. Stem Cells Transl. Med. 6, 2053–2061. doi: 10.1002/sctm.17-0134
Shi, M., Zhang, Z., Xu, R., Lin, H., Fu, J., Zou, Z., et al. (2012). Human mesenchymal stem cell transfusion is safe and improves liver function in acute-on-chronic liver failure patients. Stem Cells Transl. Med. 1, 725–731. doi: 10.5966/sctm.2012-0034
Singer, W., Dietz, A. B., Zeller, A. D., Gehrking, T. L., Schmelzer, J. D., Schmeichel, A. M., et al. (2019). Intrathecal administration of autologous mesenchymal stem cells in multiple system atrophy. Neurology 93, e77–e87. doi: 10.1212/wnl.0000000000007720
Singh, V. K., and Olabisi, A. O. (2017). Nonhuman primates as models for the discovery and development of radiation countermeasures. Expert Opin. Drug Discov. 12, 695–709. doi: 10.1080/17460441.2017.1323863
Soler, R., Orozco, L., Munar, A., Huguet, M., Lopez, R., Vives, J., et al. (2016). Final results of a phase I-II trial using ex vivo expanded autologous Mesenchymal Stromal Cells for the treatment of osteoarthritis of the knee confirming safety and suggesting cartilage regeneration. Knee 23, 647–654. doi: 10.1016/j.knee.2015.08.013
Sotiropoulou, P. A., Perez, S. A., Gritzapis, A. D., Baxevanis, C. N., and Papamichail, M. (2006). Interactions between human mesenchymal stem cells and natural killer cells. Stem Cells 24, 74–85. doi: 10.1634/stemcells.2004-0359
Stewart, I., Schluter, P. J., and Shaw, G. R. (2006). Cyanobacterial lipopolysaccharides and human health - a review. Environ. Health 5:7.
Su, J., Chen, X., Huang, Y., Li, W., Li, J., Cao, K., et al. (2014). Phylogenetic distinction of iNOS and IDO function in mesenchymal stem cell-mediated immunosuppression in mammalian species. Cell Death Differ. 21, 388–396. doi: 10.1038/cdd.2013.149
Suk, K. T., Yoon, J. H., Kim, M. Y., Kim, C. W., Kim, J. K., Park, H., et al. (2016). Transplantation with autologous bone marrow-derived mesenchymal stem cells for alcoholic cirrhosis: phase 2 trial. Hepatology 64, 2185–2197.
Swart, J. F., De Roock, S., Nievelstein, R. A. J., Slaper-Cortenbach, I. C. M., Boelens, J. J., and Wulffraat, N. M. (2019). Bone-marrow derived mesenchymal stromal cells infusion in therapy refractory juvenile idiopathic arthritis patients. Rheumatology 58, 1812–1817. doi: 10.1093/rheumatology/kez157
Sykova, E., Rychmach, P., Drahoradova, I., Konradova, S., Ruzickova, K., Vorisek, I., et al. (2017). Transplantation of mesenchymal stromal cells in patients with amyotrophic lateral sclerosis: results of phase I/IIa clinical trial. Cell Transplant. 26, 647–658. doi: 10.3727/096368916x693716
Taketani, T., Oyama, C., Mihara, A., Tanabe, Y., Abe, M., Hirade, T., et al. (2015). Ex vivo expanded allogeneic mesenchymal stem cells with bone marrow transplantation improved osteogenesis in infants with severe hypophosphatasia. Cell Transplant. 24, 1931–1943. doi: 10.3727/096368914x685410
Tan, J., Wu, W., Xu, X., Liao, L., Zheng, F., Messinger, S., et al. (2012). Induction therapy with autologous mesenchymal stem cells in living-related kidney transplants: a randomized controlled trial. JAMA 307, 1169–1177.
Tanaka, T., Narazaki, M., and Kishimoto, T. (2014). IL-6 in inflammation, immunity, and disease. Cold Spring Harb. Perspect. Biol. 6:a016295.
Teng, X., Chen, L., Chen, W., Yang, J., Yang, Z., and Shen, Z. (2015). Mesenchymal stem cell-derived exosomes improve the microenvironment of infarcted myocardium contributing to angiogenesis and anti-inflammation. Cell. Physiol. Biochem. 37, 2415–2424. doi: 10.1159/000438594
Tompkins, B. A., Difede, D. L., Khan, A., Landin, A. M., Schulman, I. H., Pujol, M. V., et al. (2017). Allogeneic mesenchymal stem cells ameliorate aging frailty: a phase II randomized, double-blind, placebo-controlled clinical trial. J. Gerontol. A Biol. Sci. Med. Sci. 72, 1513–1522. doi: 10.1093/gerona/glx137
Tran, S. D., Liu, Y., Xia, D., Maria, O. M., Khalili, S., Wang, R. W., et al. (2013). Paracrine effects of bone marrow soup restore organ function, regeneration, and repair in salivary glands damaged by irradiation. PLoS One 8:e61632. doi: 10.1371/journal.pone.0061632
Urbanelli, L., Magini, A., Buratta, S., Brozzi, A., Sagini, K., Polchi, A., et al. (2013). Signaling pathways in exosomes biogenesis, secretion and fate. Genes 4, 152–170. doi: 10.3390/genes4020152
Vaquero, J., Zurita, M., Rico, M. A., Aguayo, C., Bonilla, C., Marin, E., et al. (2018). Intrathecal administration of autologous mesenchymal stromal cells for spinal cord injury: safety and efficacy of the 100/3 guideline. Cytotherapy 20, 806–819.
Vega, A., Martin-Ferrero, M. A., Del Canto, F., Alberca, M., Garcia, V., Munar, A., et al. (2015). Treatment of knee osteoarthritis with allogeneic bone marrow mesenchymal stem cells: a randomized controlled trial. Transplantation 99, 1681–1690. doi: 10.1097/tp.0000000000000678
Wang, D., Li, J., Zhang, Y., Zhang, M., Chen, J., Li, X., et al. (2014). Umbilical cord mesenchymal stem cell transplantation in active and refractory systemic lupus erythematosus: a multicenter clinical study. Arthritis Res. Ther. 16:R79.
Wang, D., Zhang, H., Liang, J., Li, X., Feng, X., Wang, H., et al. (2013). Allogeneic mesenchymal stem cell transplantation in severe and refractory systemic lupus erythematosus: 4 years of experience. Cell Transplant. 22, 2267–2277. doi: 10.3727/096368911x582769c
Wang, P., Li, Y., Huang, L., Yang, J., Yang, R., Deng, W., et al. (2014). Effects and safety of allogenic mesenchymal stem cell intravenous infusion in active ankylosing spondylitis patients who failed NSAIDs: a 20-week clinical trial. Cell Transplant. 23, 1293–1303. doi: 10.3727/096368913x667727
Wang, X., Cheng, H., Hua, R., Yang, J., Dai, G., Zhang, Z., et al. (2013). Effects of bone marrow mesenchymal stromal cells on gross motor function measure scores of children with cerebral palsy: a preliminary clinical study. Cytotherapy 15, 1549–1562. doi: 10.1016/j.jcyt.2013.06.001
Wang, H., Li, Y., Wu, Q., Xu, C., and Liu, Q. (2016). Combination of butylphthalide with umbilical mesenchymal stem cells for the treatment of delayed encephalopathy after carbon monoxide poisoning. Medicine 95:e5412. doi: 10.1097/md.0000000000005412
Wang, L., Gu, Z., Zhao, X., Yang, N., Wang, F., Deng, A., et al. (2016). Extracellular vesicles released from human umbilical cord-derived mesenchymal stromal cells prevent life-threatening acute graft-versus-host disease in a mouse model of allogeneic hematopoietic stem cell transplantation. Stem Cells Dev. 25, 1874–1883. doi: 10.1089/scd.2016.0107
Waselenko, J. K., Macvittie, T. J., Blakely, W. F., Pesik, N., Wiley, A. L., Dickerson, W. E., et al. (2004). Medical management of the acute radiation syndrome: recommendations of the Strategic National Stockpile Radiation Working Group. Ann. Intern. Med. 140, 1037–1051.
Weisdorf, D., Chao, N., Waselenko, J. K., Dainiak, N., Armitage, J. O., Mcniece, I., et al. (2006). Acute radiation injury: contingency planning for triage, supportive care, and transplantation. Biol. Blood Marrow Transplant. 12, 672–682. doi: 10.1016/j.bbmt.2006.02.006
Wen, S., Dooner, M., Cheng, Y., Papa, E., Del Tatto, M., Pereira, M., et al. (2016). Mesenchymal stromal cell-derived extracellular vesicles rescue radiation damage to murine marrow hematopoietic cells. Leukemia 30, 2221–2231. doi: 10.1038/leu.2016.107
Wong, K. L., Lee, K. B., Tai, B. C., Law, P., Lee, E. H., and Hui, J. H. (2013). Injectable cultured bone marrow-derived mesenchymal stem cells in varus knees with cartilage defects undergoing high tibial osteotomy: a prospective, randomized controlled clinical trial with 2 years’ follow-up. Arthroscopy 29, 2020–2028. doi: 10.1016/j.arthro.2013.09.074
Xiao, Y., Jiang, Z. J., Pang, Y., Li, L., Gao, Y., Xiao, H. W., et al. (2013). Efficacy and safety of mesenchymal stromal cell treatment from related donors for patients with refractory aplastic anemia. Cytotherapy 15, 760–766. doi: 10.1016/j.jcyt.2013.03.007
Xin, H., Li, Y., Cui, Y., Yang, J. J., Zhang, Z. G., and Chopp, M. (2013a). Systemic administration of exosomes released from mesenchymal stromal cells promote functional recovery and neurovascular plasticity after stroke in rats. J. Cereb. Blood Flow Metab. 33, 1711–1715. doi: 10.1038/jcbfm.2013.152
Xin, H., Li, Y., Liu, Z., Wang, X., Shang, X., Cui, Y., et al. (2013b). MiR-133b promotes neural plasticity and functional recovery after treatment of stroke with multipotent mesenchymal stromal cells in rats via transfer of exosome-enriched extracellular particles. Stem Cells 31, 2737–2746. doi: 10.1002/stem.1409
Xiong, Y. Y., Fan, Q., Huang, F., Zhang, Y., Wang, Y., Chen, X. Y., et al. (2014). Mesenchymal stem cells versus mesenchymal stem cells combined with cord blood for engraftment failure after autologous hematopoietic stem cell transplantation: a pilot prospective, open-label, randomized trial. Biol. Blood Marrow Transplant. 20, 236–242. doi: 10.1016/j.bbmt.2013.11.002
Yang, X., Balakrishnan, I., Torok-Storb, B., and Pillai, M. M. (2012). Marrow stromal cell infusion rescues hematopoiesis in lethally irradiated mice despite rapid clearance after infusion. Adv. Hematol. 2012:142530.
Yu, B., Kim, H. W., Gong, M., Wang, J., Millard, R. W., Wang, Y., et al. (2015). Exosomes secreted from GATA-4 overexpressing mesenchymal stem cells serve as a reservoir of anti-apoptotic microRNAs for cardioprotection. Int. J. Cardiol. 182, 349–360. doi: 10.1016/j.ijcard.2014.12.043
Zhang, Y., Chopp, M., Meng, Y., Katakowski, M., Xin, H., Mahmood, A., et al. (2015). Effect of exosomes derived from multipluripotent mesenchymal stromal cells on functional recovery and neurovascular plasticity in rats after traumatic brain injury. J. Neurosurg. 122, 856–867. doi: 10.3171/2014.11.jns14770
Zhang, Y. C., Liu, W., Fu, B. S., Wang, G. Y., Li, H. B., Yi, H. M., et al. (2017). Therapeutic potentials of umbilical cord-derived mesenchymal stromal cells for ischemic-type biliary lesions following liver transplantation. Cytotherapy 19, 194–199. doi: 10.1016/j.jcyt.2016.11.005
Zhang, Z., Fu, J., Xu, X., Wang, S., Xu, R., Zhao, M., et al. (2013). Safety and immunological responses to human mesenchymal stem cell therapy in difficult-to-treat HIV-1-infected patients. AIDS 27, 1283–1293. doi: 10.1097/qad.0b013e32835fab77
Zhang, Z., Lin, H., Shi, M., Xu, R., Fu, J., Lv, J., et al. (2012). Human umbilical cord mesenchymal stem cells improve liver function and ascites in decompensated liver cirrhosis patients. J. Gastroenterol. Hepatol. 27(Suppl. 2), 112–120. doi: 10.1111/j.1440-1746.2011.07024.x
Zhang, Z. G., and Chopp, M. (2016). Exosomes in stroke pathogenesis and therapy. J. Clin. Invest. 126, 1190–1197. doi: 10.1172/jci81133
Keywords: MSCs, extracellular vesicles, exosomes, acute radiation syndrome, macrophages
Citation: Forsberg MH, Kink JA, Hematti P and Capitini CM (2020) Mesenchymal Stromal Cells and Exosomes: Progress and Challenges. Front. Cell Dev. Biol. 8:665. doi: 10.3389/fcell.2020.00665
Received: 14 April 2020; Accepted: 01 July 2020;
Published: 17 July 2020.
Edited by:
Lindolfo da Silva Meirelles, Universidade Luterana do Brazil, BrazilReviewed by:
Jeroen J. J. P. van den Beucken, Radboud University Nijmegen Medical Centre, NetherlandsNicola Baldini, University of Bologna, Italy
Copyright © 2020 Forsberg, Kink, Hematti and Capitini. This is an open-access article distributed under the terms of the Creative Commons Attribution License (CC BY). The use, distribution or reproduction in other forums is permitted, provided the original author(s) and the copyright owner(s) are credited and that the original publication in this journal is cited, in accordance with accepted academic practice. No use, distribution or reproduction is permitted which does not comply with these terms.
*Correspondence: Christian M. Capitini, ccapitini@pediatrics.wisc.edu