- 1Departamento de Biodiversidad y Biología Experimental, Facultad de Ciencias Exactas y Naturales, Universidad de Buenos Aires, Buenos Aires, Argentina
- 2Instituto de Biodiversidad y Biología Experimental y Aplicada (IBBEA), CONICET-Universidad de Buenos Aires, Buenos Aires, Argentina
- 3Department of Biology, Faculty of Marine and Environmental Sciences, University of Cádiz, Puerto Real, Spain
- 4Marine Research Institute (INMAR), Marine Campus of International Excellence (CEIMAR) and Agrifood Campus of International Excellence (ceiA3), Puerto Real, Spain
- 5Instituto Tecnológico de Chascomús (CONICET-UNSAM), Chascomús, Argentina
- 6Department of Biology and Center for Medical Life Science, Waseda University, Tokyo, Japan
Gonadotropin-inhibitory hormone, GnIH, is named because of its function in birds and mammals; however, in other vertebrates this function is not yet clearly established. More than half of the vertebrate species are teleosts. This group is characterized by the 3R whole genome duplication, a fact that could have been responsible for the great phenotypic complexity and great variability in reproductive strategies and sexual behavior. In this context, we revise GnIH cell bodies and fibers distribution in adult brains of teleosts, discuss its relationship with GnRH variants and summarize the few reports available about the ontogeny of the GnIH system. Considering all the information presented in this review, we propose that in teleosts, GnIH could have other functions beyond reproduction or act as an integrative signal in the reproductive process. However, further studies are required in order to clarify the role of GnIH in this group including its involvement in development, a key stage that strongly impacts on adult life.
Introduction
In 2000, Tsutsui's group isolated, for the first time, a novel hypothalamic neuropeptide from the brain of the Japanese quail, Coturnix japonica, which inhibited luteinizing hormone (LH) release from the anterior pituitary and named it gonadotropin-inhibitory hormone (GnIH) (1). This finding had the novelty that, at that moment, it was known that gonadotropin secretion was mainly under the stimulatory effect of gonadotropin-releasing hormone (GnRH), but an inhibitory neuropeptide of gonadotropin secretion had been not discovered. The discovery of GnIH opened a new research field in reproductive neuroendocrinology from a novel standpoint. Since then, GnIH orthologs were described in protochordates (2) and many vertebrate taxa including agnathans, teleosts, amphibians, reptiles, birds, and mammals [for review see (3, 4)]. In some of these groups, it is clear that GnIH is involved in the regulation of reproduction, inhibition of pituitary gonadotropins, and sexual behavior [for reviews see (5–9)]; however, up to this moment this is far to be a common feature. After Tsutsui's first finding (1), Satake et al. (10) characterized in quail a cDNA encoding GnIH and two GnIH-related peptides. Later, in most vertebrate species, these peptides were deduced from the cDNA sequences of their precursors, but the GnIH peptide was isolated and identified only in a few species: quail (1), starlings (11), zebra finches (12), chicken (13), rats (14), Siberian hamsters (15), bullfrog (16), turtles (17), primates (18), humans (19), and goldfish (20). All these GnIH orthologs have an Arg-Phe-amide as a C-terminal sequence and thus, they are part of the RF-amide family. Particularly, GnIH and its related peptides, either putative or identified, possess a common LPXRFamide or MPXRFamide (X: L or Q) C-terminus motif [for reviews see (5–9)].
It is known that more than half of the vertebrate species are teleosts, and teleost-3R genome duplication could have been responsible for the great phenotypic complexity observed in this group of vertebrates (21). They occupy all aquatic environments and present a tremendous variability of reproductive strategies and sexual behavior (22). In this context, fish GnIH and their related peptides could have undertaken new and maybe unexpected functions.
Early-branching lineages of ray-finned fishes (such as gars) and teleosts already present a GnIH precursor suggesting that this peptide emerged before to the teleost whole genome duplication. Early-branching lineages of teleosts, such as anguiliforms and otophysans (Cypriniformes, Characiformes, and Siluriformes), and salmoniforms, ovalentarians (some Cichliformes, Cyprinodontiformes, Atheriniformes, and Beloniformes) (23) and some Pleuronectiformes (24), exhibit a GnIH precursor encoding three peptides. However, in late-branching evolved species belonging to ovalentarians (some Cichliformes, Perciformes), Pleuronectiformes and Tetraodontiformes, two peptides were described (3) (Figure 1). Then, it seems that species belonging to early branching teleost lineages contain GnIH and 2 related peptides, whereas those species belonging to late branching lineages possessed GnIH and 1 or 2 related peptides, suggesting that one was lost in the course of teleost evolution. The meaning of this fact is still unknown, but it represents an interesting matter of study in neuropeptide evolution (3, 25).
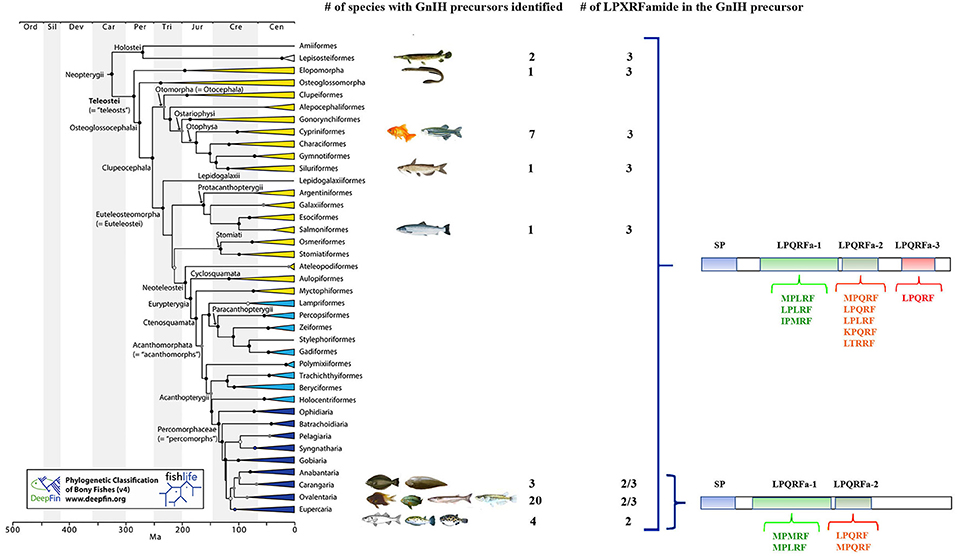
Figure 1. Phylogenetic tree of fish showing the different orders in which GnIH precursor genes have been identified and the number of LPXRFamide peptides present in these GnIH precursors. The amino acid motifs present in the C-terminal region of these LPXRFamide peptides are also presented. SP, signal peptide. The accession numbers of the identified and possible GnIH precursor sequences obtained from US National Center for Biotechnology Information database are: Lepisosteiformes: Atractosteus tropicus (Álvarez-González, C.A., personal communication; transcriptome sequencing: PRJNA395289), Lepisosteus oculatus (XP_015213317.1); Elopomorpha: Anguila japonica (XP_013998456.1); Cypriniformes: Carassius auratus (BAC06473.1), Catla catla (AUO16369.1), Cyprinus carpio (AML83913.1), Danio rerio (NP_001076418.1), Pygocentrus natteri (XP_017549097.1), Sinocyclocheilus grahami (XP_016150344.1), Sinocyclocheilus rhinocerous (XP_016370559.1); Siluriformes: Ictalurus punctatus (XP_017336524.1); Salmoniformes: Salmo salar (XP_013998456.1); Carangaria: Cynoglossus semilaevis (AMB48604.1), Paralichthys orbignyanus (Mechaly A.S., personal communication), Solea senegalensis (24); Ovalentaria: Austrofundulus limnaeus (XP_013866639.1), C. dimerus (25), Cyprinodon variegates (XP_015229614.1), Fundulus heteroclitus (XP_012729657.1), Iconisemion striatum (SBP35361.1), Kryptolebias marmoratus (XP_017278134.1), Neolamprologus brichardi (XM_006788075.1), Nothobranchius furzeri (XP_015811406.1), Nothobranchius kuhntae (SBQ91527.1), Nothobranchius pienaari (SBR89569.1), Odontesthes bonariensis (Somoza G.M., personal communication), Oreochromis niloticus (NP_001298256.1), Oryzias latipes (XP_004073896.1), Poecilia formosa (XP_007562706.1), Poecilia latipinna (XP_014884496.1), Poecilia mexicana (XP_014852162.1), Poecilia reticulata (XP_008419875.1), Pundamilia nyererei (XP_013765199.1), Stegastes partitus (XP_008290012.1), Xiphophorus maculatus (XP_005802819.1); Eupercaria: Dicentrarchus labrax (CEK03537.1), Takifugu rubripes (NP_001092115.1), Tetraodon nigroviridis (BAF34880.1), Thalassomabi fasciatum (ANV28067.1). The phylogenetic tree was taken from Figure 1 of Betancur et al. (23).
The GnIH System in Fish
Neuroanatomical Distribution of GnIH Cell Bodies and Fibers
In order to gain understanding about the GnIH system in fishes, several studies have investigated the precise localization of GnIH-producing cells in the brain and peripheral organs of teleosts by using PCR, in situ hybridization and immunohistochemical techniques [for review see (3)]. Although these studies have reported important consistencies in the brain GnIH innervation pattern, the localization of GnIH cell bodies showed considerable dissimilarities in many of the analyzed species. For instance, in sockeye salmon, Oncorhynchus nerka, (26) and tilapia, Oreochromis niloticus (27), immunohistochemistry revealed the presence of GnIH-immunoreactive (GnIH-ir) cells only in the diencephalic posterior periventricular nucleus (NPPv), whereas studies developed in other species reported the presence of GnIH-ir cell populations also in other brain regions (3). In this sense, increasing evidence obtained in the last years also suggest that GnIH neurons in teleosts are not only restricted to the caudal preoptic area/hypothalamus (24, 25, 28–31), as it occurs in birds and mammals (32) (Figure 2).
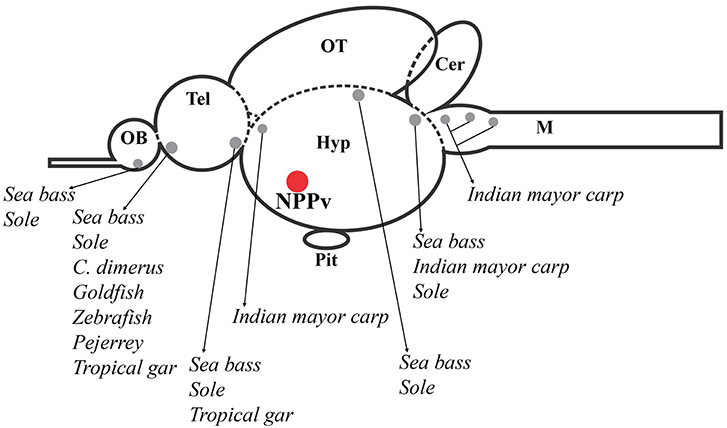
Figure 2. Schematic representation of a sagittal section of the fish brain showing GnIH cell populations described in several brain areas of different fish species, which are represented by gray circles. The red circle indicates the presence of GnIH cells in the nucleus posterioris periventricularis (NPPv) that has been reported in all fish species studied until now. OB, olfactory bulb; Tel, telencephalon; Hyp, hypothalamus; OT, optic tectum; Pit, pituitary; Cer, cerebellum; M, medulla.
Analyzing the cell clusters reported in fish species from the forebrain to the hindbrain, the most rostral GnIH-cell population described is the one present in the olfactory bulb or terminal nerve ganglion cells (TNgc)/the nucleus olfacto retinalis (NOR) of goldfish, Carassius auratus (20); developing Indian major carp, Labeo rohita (33); sea bass, Dicentrarchus labrax (29); cichlid fish, Cichlasoma dimerus (25); zebrafish, Danio rerio (30); sole, Solea senegalesis (24), and pejerrey, Odontesthes bonariensis (31). This immunostaining was consistent with gnih expression detected in these areas of sea bass brain by RT-PCR. Moreover, these results were confirmed by sensitive laser-capture microdissection followed by quantitative real-time PCR (29). Similar analysis and/or in situ hybridization studies in this brain region appear necessary to confirm the presence of GnIH in these cells in other teleost species. More recently, immunohistochemical studies also showed that GnIH neurons are located in this transitional region between the olfactory bulbs and telencephalic hemispheres of pejerrey, Odontesthes bonariensis, an atheriniform species (31), and the tropical gar, Atractosteus tropicus, an ancient lepisosteiform fish (28). More caudally, in the ventral telencephalic area, another GnIH-cell population was described, for the first time, in sea bass (29). Similarly, Aliaga-Guerrero et al. (24) reported the presence of GnIH-ir neurons in the central and lateral subdivision of the ventral telencephalon using specific antibodies developed against sole GnIH.
In the diencephalon, GnIH-ir neurons were detected in the suprachiasmatic nucleus in the tropical gar (28); while, in the India major carp, GnIH-cell masses were observed in the magnocellular preoptic nucleus (NOPm) (33). Among the diencephalic GnIH cell masses identified in different species, the one present in the posterior periventricular nucleus (NPPv) of the caudal preoptic area is the most conserved in all fish species studied so far, including goldfish (20), sockeye salmon (26), Indian major carp (33), orange-spotted grouper, Epinephelus coioides (34), sea bass (29), tilapia (27), Cichlasoma dimerus (25), pejerrey (31), zebrafish (35), sole (24), and tropical gar (28). Moreover, in agnathans, the most ancient lineage of vertebrates, a lamprey gnih precursor mRNA was only expressed in the rostral and caudal regions of the bed nucleus of the tract of the postoptic commissure (nTPOC) in the hypothalamus (36). The presence of other, but more posterior, hypothalamic GnIH-ir cell population was also seen in tropical gar, within the tuberal hypothalamus (28). In addition, GnIH-ir cells were also present in the dorsal mesencephalic tegmentum, as well as the rostral rhombencephalon of Indian major carp, sea bass and sole (24, 29, 33). The analysis of gnih expression in the mesencephalic tegmentum confirmed these neurons as genuine GnIH-expressing cells by using sensitive laser-capture microdissection followed by quantitative real-time PCR in sea bass (29).
The profuse innervation of GnIH cells in the brain is a common feature of all birds and mammals studied so far, as well as in fishes (20, 24–29, 31, 33). This pattern of the distribution of GnIH projections strongly suggests that GnIH acts in many brain sites and then its function can be not only related to reproduction.
GnIH Fiber Projections to the Pituitary Gland
In fish, GnIH-ir fibers were found running along the ventral hypothalamus, reaching the infundibulum to project into the pituitary [for review see (3)]. It is important to highlight to the readers who are not specialized in fish neuroendocrinology, that nerve terminals of hypophysiotropic neuroendocrine cells from fish can establish direct contacts (like a “synaptic terminal”) or end close to pituitary cells to release their neurohormones (37), and they do not exhibit neither a median eminence nor the portal vasculature reported in tetrapods (38). These fibers were found in the proximal pars distalis (PPD) of goldfish (20), sockeye salmon (26), sea bass (29), tilapia (27), zebrafish (35), pejerrey (31), and sole (24) and, more recently, they were also found in the neurohypophysis of the tropical gar (28). The presence of GnIH-ir fibers in the PPD reinforces the role of this neuropeptide in the regulation of pituitary hormone secretion also in fish. Furthermore, GnIH-ir fibers were observed in close proximity to FSH, LH, and GH cells in the pituitary of sea bass (29), and FSH, LH, POMC, and α-MSH cells in the pituitary of tilapia (27). Nevertheless, GnIH-pituitary innervation has not been demonstrated in adult specimens of other fish species, such as the Indian mayor carp and C. dimerus (25, 33). However, we cannot discard that a sexual-stage-dependent plasticity in the GnIH-pituitary innervation, or a neurovascular supply to the pituitary, exists as it was reported in zebrafish (39).
GnIH and Photoperiodic Control of Reproduction
Much has been written about the role of the GnIH system in transducing and/or mediating photoperiodic effects on reproduction through its interactions with the pineal organ and retina in vertebrates (40–43). The pineal organ of fish is a light-sensitive structure responsible for the nocturnal production of melatonin, playing a central role in the transduction of daily and seasonal information (44). To date in fish, only a few studies have investigated the links between GnIH and melatonin (45–49). In cinnamon clownfish, Amphipirion melanopus, it was shown that GnIH and the melatonin receptor MT1 co-localized in diencephalic cells (46). Moreover, it has been reported the existence of day-night differences in the expression of gnih in sea bass, suggesting a role of melatonin in the modulation of the GnIH system in this species (50). Accordingly, GnIH fibers were localized in the pineal organ of sea bass (29), sole (24), pejerrey (31), and tropical gar (28), suggesting the existence of bidirectional connections between the pineal organ and GnIH cells. Besides GnIH-ir fibers were found in the vascular sac of sea bass (29), sole (24) and C. dimerus (51), an organ that represents a sensor of daily and seasonal changes in day length and has been involved in the photoperiodic control of reproduction and other rhythmic processes in some teleost species (52). The interactions between GnIH and both sensor systems (pineal organ and vascular sac) could imply a role of GnIH in the relay between environment and seasonal reproduction in this group of vertebrates. Other studies observed that gnih was expressed in the retina of different fish species, such as sea bass (29), zebrafish (30), sole (24), and C. dimerus (51). However, only one report relating retinal GnIH with the reproductive cycle has been published so far, showing a decrease in retinal gnih expression in late-vitellogenic zebrafish females (30). The fact that GnIH is expressed in the retina could indicate its modulatory role in this photosensory organ, but further studies appear necessary to clarify the physiological significance of this GnIH action.
GnIH Receptors
The study of distribution of GnIH receptors (GnIH-R) has provided relevant information to recognize the neural targets of GnIH cells, helping to identify new putative roles of this neuropeptide in the brain and peripheral organs. Unfortunately, the precise identification of GnIH-R containing cells is still scarce and only a few studies have used molecular tools and antibodies to address its detailed localization in the fish brain. Both GnIH-ir fibers and GnIH-R were widely distributed in the tilapia brain but they were particularly evident in cells bodies of the preoptic area, hypothalamus, optic tectum, semicircular torus, and caudal midbrain tegmentum. They also coexist in the olfactory bulbs, ventral/dorsal telencephalon and in the rhombencephalon (27). In addition, GnIH-R immunoreactivity was found in LH, ACTH, and α-MSH cells of tilapia pituitary (27). Moreover, three different GnIH-R subtypes have been identified in goldfish (53) and zebrafish (54), but their presence in other fish is still not reported. In goldfish, three subtypes of GnIH-Rs were localized in neuroendocrine regions as the preoptic area and the NPPv, the preoptic nucleus (NPO), and the lateral tuberal nucleus (NLT), whereas only two GnIH-R subtypes (GnIH-R1 and GnIH-R2) were observed in the pars intermedia of the pituitary gland. Surprisingly, no signals of GnIH-R were observed in the proximal and rostral pars distalis of the goldfish pituitary (54). On the other hand, the presence of gnih-r transcripts was also revealed in the brain and pituitary of zebrafish (53), grass puffer, Takifugu niphobles (45), and tongue sole, Cynoglossus semilaevis (55), by using RT-PCR. In addition, RT-PCR and in situ hybridization studies have reported the expression of gnih and gnih-r in some peripheral fish organs, including the gonads (24, 25, 29, 30, 34, 45, 46, 53, 54, 56–58), which could indicate an autocrine/paracrine role of GnIH in gonadal function.
GnIH and GnRH Relationships
It is well-established that in vertebrates, multiple GnRH variants are expressed by different neurons in the brain of a single species. These variants are currently classified into three different types, according to their amino-acid sequence, neuroanatomical localization, embryological origin, and synteny: GnRH1, GnRH2, and GnRH3 [for review see (59)]. In the case of teleost fish, GnRH1, the most variable GnRH type according to its amino-acid sequence, is expressed in neurons originated from the olfactory placode during embryogenesis (59–62) and plays the classical hypophysiotropic function in most species. GnRH2 is mainly produced by midbrain tegmental neurons and it has been proposed that plays a key role in reproductive behavior [for review see (63)]. Finally, GnRH3 is expressed in ventral forebrain neurons, from the olfactory bulbs to the hypothalamus, and seems to act as a neuromodulator of olfactory and visual information related to reproduction (64, 65). This variant also plays hypophysiotropic functions especially in those teleost species expressing two GnRH variants: GnRH2 and GnRH3 as most of Cypriniformes and Salmoniformes (38, 66).
In birds and mammals, GnIH regulates gonadotrophs' function either directly or indirectly via GnRH neurons (11, 67–69). Considering that GnRH is the key neuropeptide in the control of gonadotropin synthesis and secretion, it could be the candidate through which GnIH acts in fish. In this conceptual frame, several studies have analyzed the relationship between both systems, although most of the mare focused on physiological approaches. Even though it could be considered that morphological associations may allow us to infer physiological interactions, there is scarce information on the relationship between GnIH and GnRH neurons in fishes.
GnIH-GnRH1 Neuroanatomical Interactions
As it was previously mentioned, GnRH1 is the main hypophisiotropic variant in most fishes, but neither in tilapia (27) nor in C. dimerus (70), axo-somatic or fiber-fiber contacts were observed between GnRH1 and GnIH neurons; although, in C. dimerus GnIH axons were detected in close proximity to GnRH1 fibers. Additionally, in sea bass (71) and zebrafish (35), GnIH terminals contacted GnRH1 cells or GnRH3 in the preoptic area, respectively. Taking into consideration these results, it is possible that either there are interspecific differences in this interaction, or it shows plasticity depending on the sexual stage, as it was suggested in sea bass (71) and C. dimerus (70). Another possibility is that GnIH can modulate other neurons, as those producing kisspeptin, dopamine or neuropeptide Y, to control gonadotropin secretion. For instance, in zebrafish, GnIH-immunoreactive fibers were observed interacting with kisspeptin receptor-1a-expressing neurons in the preoptic area (35), and a GnIH innervation on Kiss2 cells of the nucleus of the lateral recess has been reported in sea bass (71), although in tilapia, GnIH cells do not seem to be connected with either GnRH1, GnRH3 or kisspeptin neurons (27).
GnIH-GnRH2 Neuroanatomical Interactions
In Indian major carp (33), sea bass (29), and sole (24) a cluster of GnIH somas were localized in the midbrain; however, this is not a common feature of all analyzed species. Only one study reported the GnRH2 and GnIH relationship and demonstrated fiber-to-fiber contacts in the nucleus lateralis tuberis and the midbrain tegmentum, suggesting a possible regulation between them (70). This GnIH-GnRH2 association could represent the morphological substrate of a network mediating the transduction of environmental information to the reproductive axis. This is further supported by the interactions among GnRH2, GnIH and melatonin reported in several fish species (24, 29, 46–48, 72, 73).
GnIH-GnRH3 Neuroanatomical Interactions
Finally, GnRH3 and GnIH neurons were observed in the TNgc of most fish species [for review see, (20, 24, 25, 29, 30, 53, 62)]. The co-localization of both peptides in the same neurons was observed in C. dimerus since early developmental stages (51) and in adults of this species (70), pejerrey (31), and sea bass (Figures 3A,B). Moreover, a deeper study on co-localization showed, for the first time, that GnIH and GnRH3 peptides are localized in different neurosecretory vesicles (Figure 3C), suggesting that both peptides can be independently regulated and secreted. It is also interesting to notice that in most of the brain regions analyzed in C. dimerus, some fibers co-expressed GnRH3 and GnIH; whereas some other fibers only expressed GnRH3 or GnIH, suggesting that these fibers correspond to neurons located in other brain areas as the NPPv (for GnIH) or from the OB, ventral TEL and POA (for GnRH3) (Figure 4). However, no contacts between GnRH3 fibers and GnIH neurons were observed (70).
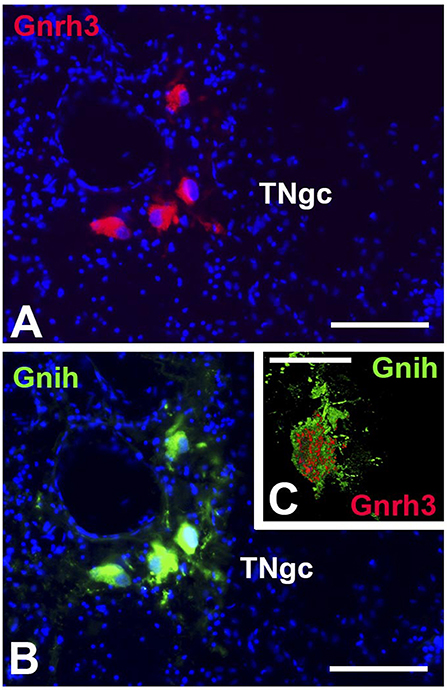
Figure 3. Coexpression of GnIH and GnRH3 in the terminal nerve area of sea bass. GnRH3 positive immunolabeling (red, A) and GnIH positive immunolabeling (green, B) in the same cell bodies of the terminal nerve region, presenting evidence of co-expression of these two neurohormones. Images (A,B) were captured on a conventional fluorescence photomicroscope. (C) High resolution confocal image presenting evidence for the co-localization of GnIH (green) and GnRH3 (red) in the same cell bodies, but packaged in separate neurosecretory vesicles. In A and B, the nuclei of cells are stained with DAPI (blue). TNgc: terminal nerve ganglion cells. Scale bars = 100 μm in (A,B), and 50 μm in (C).
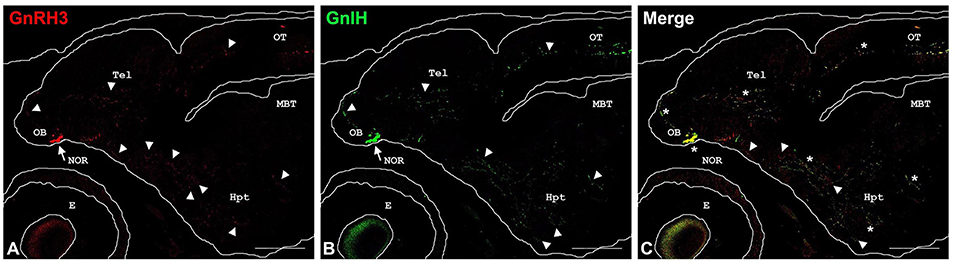
Figure 4. Double-labeling immunofluorescence in parasagittal brain sections of 27 days post-hatching C. dimerus larvae using GnIH and GnRH3-GAP antisera. Microphotographs present GnRH3-GAP-immunoreactive (GnRH3) neurons (red, A) and GnIH-immunoreactive (GnIH) neurons (green, B). In (C), a merge image of (A,B) is presented. Immunoreactive cell somata are indicated by arrows and some representative fibers appear marked by arrowheads. Co-localization is indicated by asterisks. Scale bar: 200 μm. OB, olfactory bulb; NOR, nucleus olfacto retinalis; Tel, telencephalon; Hyp, hypothalamus; OT, optic tectum; MBT, midbrain tegmentum; E, eye.
Physiological Interactions Between GnIH and GnRHs
The physiological action of GnIH on GnRH synthesis and its modulating effects over GnRH-stimulatory action on the synthesis and release of gonadotropins or GH are summarized in Table 1. From these data, GnIH can prevent the GnRH-stimulatory action on the synthesis and/or release of FSH, LH, and GH. For example, Moussavi et al. (74, 75) showed that when GnRH2 or GnRH3 are co-administrated with goldfish GnIH-III (gGnIH-III), the stimulatory action of GnRH2/GnRH3 on LH secretion was attenuated by goldfish GnIH-III, together with lhβ or fshβ synthesis, especially during mid and late recrudescence (74); while gGnIH-III prevented GnRH2/GnRH3 stimulation of gh transcript levels and GH secretion (75). Moreover, gGnIH-III differentially affected GnRH2 and GnRH3 actions depending on the sexual stage. Similar effects of GnIH peptides were demonstrated in Amphiprion melanopus (46) and in Astyanax altiparanae (78). This preventive-GnRH-stimulatory effect has been proposed to depend on the action of estradiol or neuroestrogen levels; the abundance of GnIH-R, GnRH and estrogen receptors, and the inhibition of cAMP pathways or the hyperpolarization of gonadotropes by activating K+, both exerted by the activation of GnIH-R. Other possibility is that GnIH-R and GnRH-R could form heterodimers modifying the action of their ligands on gonadotrophs [for review see (79)]. Although these statements have been mostly established in birds and mammals (80, 81), there is evidence that a similar mechanism could be operating in teleosts (34, 55, 82, 83).
On the other hand, GnIH can stimulate, inhibit, or even have no effect on the synthesis of GnRH variants (Table 1). These discrepancies could be due to differences in sexual stage, route of administration, sampling times and brain regions analyzed. Also, based on studies performed in birds and mammals, it has been proposed that GnIH could indirectly modulate the expression and activity of brain aromatase regulating estradiol levels locally [for review see (79)].
Nowadays, further studies appear necessary to clarify GnIH actions over gonadotropin secretion and/or synthesis in fish. According to anatomical and physiological results presented in this section, it must be emphasized that GnIH interaction with GnRH occurs in fish, and either directly or indirectly GnIH can stimulate or inhibits hypothalamic-pituitary axis depending on the reproductive state of individuals.
Ontogeny of GnIH System
As Sandvik et al. (84) referred, although RFamide peptides are poorly studied during development, the few reports available in the field show interesting results indicating that many of these peptides have different roles in early stages and in adults. The few studies addressing GnIH ontogeny show that this peptide is not an exception. To date, there are only four studies analyzing the GnIH expression pattern during fish development, showing that this peptide is detected from early developmental stages (33, 50, 51, 53). In zebrafish gnih and gnih-r transcripts were detected from 1-day post-fertilization (dpf) (prime-5 stage) or blastula stage, respectively; however, in this study, no temporal variations in the expression were evaluated (53). In sea bass, gnih and gnih-r transcripts were detected from 5 dpf, and although the authors did not quantify the expression in stages prior to hatching, two temporal increases in the gnih messengers were observed: one from 5 days post-hatching (dph) to 25 dph, when the larva starts exogenous feeding and the gonad is still undifferentiated, and the other by 150 dph during the onset of gonadal differentiation (50). Studies performed in C. dimerus showed that gnih was first detected at 1 dph, increased from 12 dph and reached a peak at 20 dph, when the development of gonadal primordia occurred (51). As we previously mentioned, in different species one or more GnIH cell clusters were observed apart from that of NPPv. The spatial-temporal expression pattern of these nuclei could suggest different origins or functions during development. For example, in the Indian major carp, GnIH cells were observed in the NPPv and in the olfactory system (epithelium and bulb) at hatching. This mentioned area showed no GnIH-ir in adults, suggesting a role of these cells during development (33). By contrast, in C. dimerus GnIH neurons in the NOR was detected by 3 dph, while NPPv cells by 14 dph (51). The cells in the NOR increase in number from 5 dph, coinciding with the time when larvae start to feed exogenously, and continue to increase in number during the development and differentiation of gonadal primordia. In the same direction, it was observed an increase of NPPv cell number during the development and differentiation of the gonadal primordia. Based on these results, it is suggested that GnIH could be involved in the onset of feeding and gonadal development or sex differentiation in teleosts. This new concept is supported by the variations of gnih and gnih-r levels in sea bass and C.dimerus during these critical periods of early gonadal development (50, 51).
On the other hand, during development GnIH fibers innervate different brain regions (33, 51). Particularly in C. dimerus, the presence of GnIH fibers was observed reaching the pituitary from 14 dph to 85 dph, but they clearly diminished from 37 dph on (51). Considering that GnRH1 fibers were detected at 30 dph (85), we could speculate a shift in the neuroendocrine control of pituitary function occurring before gonadal differentiation. The fact that no GnIH fibers innervating the pituitary gland were detected in adults of this species (25), could imply that this neuropeptide would act differently in larvae and adults.
Biotic and abiotic factors, especially temperature and photoperiod, are critical features that could irreversibly affect different biological aspects during development. Even though the neuroendocrine system integrates environmental information, little is known about its development and how it is altered by these factors. For example, low or high incubation temperatures during early developmental stages determine different sex ratio of pejerrey and sea bass larvae (86, 87) indicating that the reproductive axis, at some point, has been altered. To our knowledge, only one study reported the effect of temperature and photoperiod on GnIH system during development (50). In this study, sea bass reared at high temperature, showed a decrease in the expression of gnih and gnih-r, suggesting that this neuropeptide could be involved in the reported effect of temperature on sex differentiation. Moreover, a seasonal shift in a daily variation of GnIH system related to the reproductive season was demonstrated, indicating the influence of the photoperiod on this system (50). In summary, GnIH in fish development is an almost unexplored area, so more studies are needed in order to further elucidate its role at this particular stage.
Expanding GnIH Functions Beyond Reproduction
Since the discovery of GnIH, most studies have analyzed the effect of this peptide on the reproductive axis, leaving aside their possible role in the regulation of other functions. In this sense, neuroanatomical localization studies showed in all fish species analyzed that GnIH fibers are broadly distributed along the nervous system, not only in the preoptic-hypothalamic area but also in the retina-optic tract and midbrain, suggesting a potential role of GnIH as neuromodulator or neurotransmitter. In sea bass, GnIH seems to participate in the regulation of fish behavior, as their administration affected the diurnal/nocturnal ratio of locomotors activity during the reproductive cycle (48, 77). It is important to highlight that in this species a cluster of GnIH cells was observed in the midbrain innervating sensory-motor areas (29). On the other hand, there is increasing evidence regarding the effect of GnIH on the synthesis and release of GH (25, 26, 57, 75, 76, 88). Usually, after GnIH administration different responses on the GH synthesis and release were observed depending on the species or the experimental approach. For example, in vitro administration of GnIH stimulated GH release in sockeye salmon and C. dimerus (25, 26) while in grass puffer, GnIH can increase the abundance of gh messengers (88). However, icv administration of GnIH decreased gh in sea bass (76), whereas intraperitoneal administration of GnIH did not affect GH release in tilapia (57). However, also concerning to GH regulation, a clear dependence on the reproductive status and on the experimental approach was observed in goldfish (75). In conclusion, these results indicate that GnIH exerts complex effects on basal and GnRH-stimulated somatotrope function in a seasonal-reproductive manner, and thus, this peptide could be involved in the regulation of somatic growth and/or in the interaction between growth and reproduction.
Finally, the ventral telencephalon and the NPPv also exhibit neuropeptide Y (NPY) producing cells in several fish species (89–91). NPY has been implicated in the modulation of gonadotropin release, but also in the regulation of feeding and growth (38, 92). Since GnIH cells are observed in these regions, it is suggested that this neuropeptide could establish a crosstalk among growth, feeding, and reproductive axes. Whether GnIH and NPY are interacting to modulate reproduction, feeding and growth in fish remains to be elucidated.
In conclusion, although the effects of GnIH on reproduction are very clear in birds and mammals, there are still some inconsistencies in fishes that should be addressed soon. Because of our attempt to generalize GnIH function, it is possible that different modes of action or other roles beyond reproduction are leaving aside in fishes. Moreover, as reproduction is a complex event that involves the integration of internal and external cues, it is possible that GnIH acts as a link among them. Interestingly, recent mutation studies for reproductive neuroendocrine factors have shown that, contrary to mammals, kisspeptin, and GnRH null fish can reproduce normally, suggesting a compensatory multifactorial neuroendocrine control of reproduction (93–97). Notably, they found an up-regulation of different neuropeptides involved in the control of reproduction in zebrafish including GnIH (97). According Marvel et al. (97), the up-regulation of GnIH messengers could be related to the action of GnIH as a stimulator of pituitary gonadotropins, as it was already demonstrated in some teleost fish species (25, 74). Further studies are still required in order to clarify the role of GnIH in teleosts including its involvement in development, a key stage that strongly impacts on adult biology.
Author Contributions
MD, JM-C, JP-S, GS, KT, and PV contributed equally to the manuscript and approved it for publication.
Funding
This work was supported by Agencia Nacional de Promoción Científica y Tecnológica (PICT 2015-2783 to GS), Universidad de Buenos Aires (20020160100110BA to PV), Consejo Nacional de Investigaciones Científicas y Técnicas (CONICET) (11220130100501CO to PV), Junta de Andalucía (P10-AGR-05916 to JM-C), and the European Union's Seventh Framework Programme (FP7/2007-2013, grant no. 331964 to JM-C).
Conflict of Interest Statement
The authors declare that the research was conducted in the absence of any commercial or financial relationships that could be construed as a potential conflict of interest.
Acknowledgments
We thank Dr. Guillermo Ortí (The George Washington University) for his advice in fish systematic and Dr. Daniela I. Pérez Sirkin and Mr. Federico Obertello for their valuable contributions.
References
1. Tsutsui K, Saigoh E, Ukena K, Teranishi H, Fujisawa Y, Kikuchi M, et al. A novel avian hypothalamic peptide inhibiting gonadotropin release. Biochem Biophys Res Commun. (2000) 275:6612–7. doi: 10.1006/bbrc.2000.3350
2. Osugi T, Okamura T, Son YL, Ohkubo M, Ubuka T, Henmi Y, et al. Evolutionary origin of GnIH and NPFF in chordates: insights from novel amphioxus RFamide peptides. PLoS ONE (2014) 9:e100962. doi: 10.1371/journal.pone.0100962
3. Muñoz-Cueto JA, Paullada-Salmerón JA, Aliaga-Guerrero M, Cowan ME, Parhar IS, Ubuka T. A journey through the gonadotropin-inhibitory hormone system of fish. Front Endocrinol. (2017) 8:285. doi: 10.3389/fendo.2017.00285
4. Tsutsui K, Osugi T, Son YL, Ubuka T. Review: structure, function and evolution of GnIH. Gen Comp Endocrinol. (2018) 264:48–57. doi: 10.1016/j.ygcen.2017.07.024
5. Tsutsui K. Review: a new key neurohormone controlling reproduction, gonadotropin-inhibitory hormone (GnIH): biosynthesis, mode of action and functional significance. Prog Neurobiol. (2009) 88:76–88. doi: 10.1016/j.pneurobio.2009.02.003
6. Tsutsui K. How to contribute to the progress of neuroendocrinology: new insights from discovering novel neuropeptides and neurosteroids regulating pituitary and brain functions. Gen Comp Endocrinol. (2016) 227:3–15. doi: 10.1016/j.ygcen.2015.05.019
7. Tsutsui K, Bentley GE, Bedecarrats G, Osugi T, Ubuka T, Kriegsfeld LJ. Review: gonadotropin-inhibitory hormone (GnIH) and its control of central and peripheral reproductive function. Front Neuroendocrinol. (2010) 31:284–95. doi: 10.1016/j.yfrne.2010.03.001
8. Tsutsui K, Bentley GE, Kriegsfeld LJ, Osugi T, Seong JY, Vaudry H. Review: discovery and evolutionary history of gonadotrophin-inhibitory hormone and kisspeptin: new key neuropeptides controlling reproduction. J Neuroendocrinol. (2010) 22:716–27. doi: 10.1111/j.1365-2826.2010.02018.x
9. Tsutsui K, Ubuka T, Son YL, Bentley GE, Kriegsfeld LJ. Contribution of GnIH research to the progress of reproductive neuroendocrinology. Front Endocrinol. (2015) 6:179. doi: 10.3389/fendo.2015.00179
10. Satake H, Hisada M, Kawada T, Minakata H, Ukena K, Tsutsui K. Characterization of a cDNA encoding a novel avian hypothalamic neuropeptide exerting an inhibitory effect on gonadotropin release. Biochem J. (2001) 354:379–85. doi: 10.1042/bj3540379
11. Ubuka T, Kim S, Huang YC, Reid J, Jiang J, Osugi T, et al. Gonadotropin-inhibitory hormone neurons interact directly with gonadotropin-releasing hormone-I and -II neurons in European starling brain. Endocrinol (2008) 149:268–78. doi: 10.1210/en.2007-0983
12. Tobari Y, Iijima N, Tsunekawa K, Osugi T, Okanoya K, Tsutsui K, et al. Identification of gonadotropin-inhibitory hormone in the zebra finch (Taeniopygia guttata): peptide isolation, cDNA cloning and brain distribution. Peptides (2010) 31:816–26. doi: 10.1016/j.peptides.2010.01.015
13. McConn B, Wang G, Yi J, Gilbert ER, Osugi T, Ubuka T, et al. Gonadotropin-inhibitory hormone-stimulation of food intake is mediated by hypothalamic effects in chicks. Neuropeptides (2014) 48:327–34. doi: 10.1016/j.npep.2014.09.001
14. Ukena K, Iwakoshi E, Minakata H, Tsutsui K. A novel rat hypothalamic RFamide-related peptide identified by immunoaffinity chromatography and mass spectrometry. FEBS Lett. (2002) 512:255–8. doi: 10.1016/S0014-5793(02)02275-5
15. Ubuka T, Inoue K, Fukuda Y, Mizuno T, Ukena K, Kriegsfeld LJ, et al. (2012). Identification, expression, and physiological functions of Siberian hamster gonadotropin-inhibitory hormone. Endocrinol 153, 373–385. doi: 10.1210/en.2011-1110
16. Ukena K, Koda A, Yamamoto K, Kobayashi T, Iwakoshi-Ukena E, Minakata H, et al. Novel neuropeptides related to frog growth hormone-releasing peptide: isolation, sequence, and functional analysis. Endocrinol (2003) 144:3879–84. doi: 10.1210/en.2003-0359
17. Ukena K, Iwakoshi-Ukena E, Osugi T, Tsutsui K. Identification and localization of gonadotropin-inhibitory hormone (GnIH) orthologs in the hypothalamus of the red-eared slider turtle, Trachemysscripta elegans. Gen Comp Endocrinol. (2016) 227:69–76. doi: 10.1016/j.ygcen.2015.06.009
18. Ubuka T, Lai H, Kitani M, Suzuuchi A, Pham V, Cadigan PA, et al. Gonadotropin-inhibitory hormone identification, cDNA cloning, and distribution in rhesus macaque brain. J Comp Neurol. (2009) 517:841–55. doi: 10.1002/cne.22191
19. Ubuka T, Morgan K, Pawson AJ, Osugi T, Chowdhury VS, Minakata H, et al. Identification of human GnIH homologs, RFRP-1 and RFRP-3, and the cognate receptor, GPR147 in the human hypothalamic pituitary axis. PLoS ONE (2009) 4:e8400. doi: 10.1371/journal.pone.0008400
20. Sawada K, Ukena K, Satake H, Iwakoshi E, Minakata H, Tsutsui K. Novel fish hypothalamic neuropeptide: cloning of a cDNA encoding the precursor polypeptide and identification and localization of the mature peptide. Eur J Biochem. (2002) 269:6000–8. doi: 10.1046/j.1432-1033.2002.03351.x
21. Meyer A, Schartl M. Gene and genome duplications in vertebrates: the one-to-four (-to-eight in fish) rule and the evolution of novel gene functions. Curr Opin Cell Biol. (1999) 11:699–704.
22. Juntti SA, Fernald RD. Timing reproduction in teleost fish: cues and mechanisms. Curr. Opin. Neurobiol. (2016) 38:57–62. doi: 10.1016/j.conb.2016.02.006
23. Betancur RR, Wiley EO, Arratia G, Acero A, Bailly N, Miya M, et al. Phylogenetic classification of bony fishes. BMC Evol. Biol. (2017) 17:162. doi: 10.1186/s12862-017-0958-3
24. Aliaga-Guerrero M, Paullada-Salmerón JA, Piquer V, Mañanós EL, Muñoz-Cueto JA. Gonadotropin-inhibitory hormone in the flatfish, Solea senegalensis: molecular cloning, brain localization and physiological effects. J Comp Neurol. (2018) 526:349–70. doi: 10.1002/cne.24339
25. Di Yorio MP, Pérez Sirkin DI, Delgadin TH, Shimizu A, Tsutsui K, Somoza GM, et al. Gonadotropin-inhibitory hormone in the cichlid fish Cichlasoma dimerus: structure, brain distribution and differential effects on the secretion of gonadotropins and growth hormone. J Neuroendocrinol. (2017) 28:5. doi: 10.1111/jne.12377
26. Amano M, Moriyama S, Iigo M, Kitamura S, Amiya N, Yamamori K, et al. Novel fish hypothalamic neuropeptides stimulate the release of gonadotrophins and growth hormone from the pituitary of sockeye salmon. J Endocrinol. (2006) 188:417–23. doi: 10.1677/joe.1.06494
27. Ogawa S, Sivalingam M, Biran J, Golan M, Anthonysamy RS, Levavi-Sivan B, et al. Distribution of LPXRFa, a gonadotropin-inhibitory hormone ortholog peptide, and LPXRFa receptor in the brain and pituitary of the tilapia. J Comp Neurol. (2016) 524:2753–75. doi: 10.1002/cne.23990
28. Di Yorio MP, Perez Sirkin DI, Battista A, Álvarez-González CA, Tsutsui K, Vissio PG. Gonadotropin inhibitory hormone (gnih) distribution in the brain of the ancient fish, Atractosteus tropicus (Lepisosteiformes: lepisosteidae). In: 11th International Symposium on Reproductive Physiology of Fish. Manaus (2018).
29. Paullada-Salmerón JA, Cowan M, Aliaga-Guerrero M, Gómez A, Zanuy S, Mañanos E, et al. LPXRFa peptide system in the European sea bass: a molecular and immunohistochemical approach. J Comp Neurol. (2016) 524:176–98. doi: 10.1002/cne.23833
30. Corchuelo S, Martinez ERM, Butzge AJ, Doretto LB, Ricci JMB, Valentin FN, et al. Characterization of Gnrh/Gnih elements in the olfacto-retinal system and ovary during zebrafish ovarian maturation. Mol Cell Endocrinol. (2017) 450:1–13. doi: 10.1016/j.mce.2017.04.002
31. Pahí-Rosero AM, Pérez MR, López GC, Vissio PG, Somoza GM. Brain distribution of immunoreactive neurons and fibers expressing gnih in pejerrey, Odontesthes bonariensis. In: 11th International Symposium on Reproductive Physiology of Fish. Manaus (2018).
32. Tsutsui K, Ubuka T. How to contribute to the progress of neuroendocrinology: discovery of GnIH and progress of GnIH research. Front Endocrinol. (2018) 9:662. doi: 10.3389/fendo.2018.00662
33. Biswas S, Jadhao AG, Pinelli C, Palande NV, Tsutsui K. GnIH and GnRH expressions in the central nervous system and pituitary of Indian major carp, Labeo rohita during ontogeny: an immunocytochemical study. Gen Comp Endocrinol. (2015) 220:88–92. doi: 10.1016/j.ygcen.2014.06.005
34. Wang Q, Qi X, Guo Y, Li S, Zhang Y, Liu X, et al. Molecular identification of GnIH/GnIHR signal and its reproductive function in protogynous hermaphroditic orange-spotted grouper (Epinephelus coioides). Gen Comp Endocrinol. (2015) 216:9–23. doi: 10.1016/j.ygcen.2015.04.016
35. Spicer OS, Zmora N, Wong TT, Golan M, Levavi-Sivan B, Gothilf Y, et al. The gonadotropin-inhibitory hormone (Lpxrfa) system's regulation of reproduction in the brain-pituitary axis of the zebrafish (Danio rerio). Biol Reprod. (2017) 96:1031–42. doi: 10.1093/biolre/iox032
36. Osugi T, Daukss D, Gazda K, Ubuka T, Kosugi T, Nozaki M, et al. Evolutionary origin of the structure and function of gonadotropin-inhibitory hormone: insights from lampreys. Endocrinol (2012) 153:2362–74. doi: 10.1210/en.2011-2046
37. Cerdá-Reverter JM, Canosa LF. Neuroendocrine systems of the fish brain. In: Bernier, NJ, Van Der Kraak, G, Farrell, AP, Brauner, CJ. Editors. Fish Neuroendocrinology, Vol. 28. Amsterdan: Academic Press (2009). p. 3–74.
38. Zohar Y, Muñoz-Cueto JA, Elizur A, Kah O. Neuroendocrinology of reproduction in teleost fish. Gen Comp Endocrinol. (2010) 165:438–55. doi: 10.1016/j.ygcen.2009.04.017
39. Golan M, Zelinger E, Zohar Y, Levavi-Sivan B. Architecture of GnRH-gonadotrope-vasculature reveals a dual mode of gonadotropin regulation in fish. Endocrinol (2015) 156:4163–73. doi: 10.1210/en.2015-1150
40. Ubuka T, Bentley GE, Ukena K, Wingfield JC, Tsutsui K. Melatonin induces the expression of gonadotropin-inhibitory hormone in the avian brain. Proc Natl Acad Sci USA. (2005) 102:3052–7. doi: 10.1073/pnas.0403840102
41. Chowdhury VS, Yamamoto K, Ubuka T, Bentley GE, Hattori A, Tsutsui K. Melatonin stimulates the release of gonadotropin-inhibitory hormone by the avian hypothalamus. Endocrinol (2010) 151:271–80. doi: 10.1210/en.2009-0908
42. Tsutsui K, Ubuka T, Bentley GE, Kriegsfeld LJ. Review: regulatory mechanisms of gonadotropin-inhibitory hormone (GnIH) synthesis and release in photoperiodic animals. Front Neurosci. (2013) 7:60. doi: 10.3389/fnins.2013.00060
43. Piekarski DJ, Jarjisian SG, Perez L, Ahmad H, Dhawan N, Zucker I, et al. Effects of pinealectomy and short day lengths on reproduction and neuronal RFRP-3, kisspeptin, and GnRH in female turkish hamsters. J Biol Rhythm. (2014) 29:181–91. doi: 10.1177/0748730414532423
44. Falcón J, Migaud H, Muñoz-Cueto JA, Carrillo M. Current knowledge on the melatonin system in teleost fish. Gen. Comp. Endocrinol. (2010) 165:469–82. doi: 10.1016/j.ygcen.2009.04.026
45. Shahjahan M, Ikegami T, Osugi T, Ukena K, Doi H, Hattori A, et al. Synchronised expressions of LPXRFamide peptide and its receptor genes: seasonal, diurnal and circadian changes during spawning period in grass puffer. J Neuroendocrinol. (2011) 23:39–51. doi: 10.1111/j.1365-2826.2010.02081.x
46. Choi YJ, Kim NN, Habibi HR, Choi CY. Effects of gonadotropin inhibitory hormone or gonadotropin-releasing hormone on reproduction-related genes in the protandrous cinnamon clownfish, Amphiprion melanopus. Gen Comp Endocrinol. (2016) 235:89–99. doi: 10.1016/j.ygcen.2016.06.010
47. Choi YJ, Habibi HR, Choi CY. Profiles of gonadotropin-inhibitory hormone and melatonin during the sex change and maturation of cinnamon clownfish, Amphiprion melanopus. Biochem Biophys Res Commun. (2016) 475:189–93. doi: 10.1016/j.bbrc.2016.05.073
48. Cowan M, Paullada-Salmerón JA, López-Olmeda JF, Sánchez-Vázquez FJ, Muñoz-Cueto JA. Effects of pinealectomy on the neuroendocrine reproductive system and locomotor activity in male European sea bass, Dicentrarchus labrax. Comp Biochem Physiol A Mol Integr Physiol. (2017) 207:1–12. doi: 10.1016/j.cbpa.2017.02.008
49. Yumnamcha T, Khan ZA, Rajiv C, Devi SD, Mondal G, Sanjita Devi H, et al. Interaction of melatonin and gonadotropin-inhibitory hormone on the zebrafish brain-pituitary-reproductive axis. Mol Reprod Dev. (2017) 84:389–400. doi: 10.1002/mrd.22795
50. Paullada-Salmerón JA, Loentgen GH, Cowan M, Aliaga-Guerrero M, Rendón-Unceta MC, Muñoz-Cueto JA. Developmental changes and day-night expression of the gonadotropin-inhibitory hormone system in the European sea bass: effects of rearing temperature. Comp Biochem Physiol A Mol Integr Physiol. (2017) 206:54–62. doi: 10.1016/j.cbpa.2017.01.009
51. Di Yorio MP, Sallemi JE, Toledo Solís FJ, Pérez Sirkin DI, Delgadin TH, Tsutsui K, et al. Ontogeny of gonadotropin-inhibitory hormone (GnIH) in the cichlid fish Cichlasoma dimerus. J Neuroendocrinol. (2018) 30:e12608. doi: 10.1111/jne.12608
52. Nakane Y, Ikegami K, Iigo M, Ono H, Takeda K, Takahashi D, et al. The saccus vasculosus of fish is a sensor of seasonal changes in day lenght. Nat Commun. (2013) 4:2108. doi: 10.1038/ncomms3108
53. Zhang Y, Li S, Liu Y, Lu D, Chen H, Huang X, et al. Structural diversity of the GnIH/GnIH receptor system in teleost: its involvement in early development and the negative control of LH release. Peptides (2010) 31:1034–43. doi: 10.1016/j.peptides.2010.03.003
54. Qi X, Zhou W, Li S, Lu D, Yi S, Xie R, et al. Evidences for the regulation of GnRH and GTH expression by GnIH in the goldfish, Carassius auratus. Mol Cell Endocrinol. (2013) 366:9–20. doi: 10.1016/j.mce.2012.11.001
55. Wang B, Yang G, Liu Q, Qin J, Xu Y, Li W, et al. Characterization of LPXRFa receptor in the half-smooth tongue sole (Cynoglossus semilaevis): molecular cloning, expression profiles, and differential activation of signaling pathways by LPXRFa peptides. Comp Biochem Physiol A Mol Integr Physiol. (2018) 223:23–32. doi: 10.1016/j.cbpa.2018.05.008
56. Qi X, Zhou W, Lu D, Wang Q, Zhang H, Li S, et al. Sexual dimorphism of steroidogenesis regulated by GnIH in the goldfish, Carassius auratus. Biol Reprod. (2013) 88:8177. doi: 10.1095/biolreprod.112.105114
57. Biran J, Golan M, Mizrahi N, Ogawa S, Parhar IS, Levavi-Sivan B. LPXRFa, the piscine ortholog of GnIH, and LPXRF receptor positively regulate gonadotropin secretion in tilapia (Oreochromis niloticus). Endocrinol (2014) 155:4391–401. doi: 10.1210/en.2013-2047
58. Wang B, Liu Q, Liu X, Xu Y, Shi B. Molecular characterization and expression profiles of LPXRFa at the brain-pituitary-gonad axis of half-smooth tongue sole (Cynoglossus semilaevis) during ovarian maturation. Comp Biochem Physiol B Biochem Mol Biol. (2018) 216:59–68. doi: 10.1016/j.cbpb.2017.11.016
59. Roch GJ, Busby ER, Sherwood NM. GnRH receptors and peptides: skating backward. Gen Comp Endocrinol. (2014) 209:118–34. doi: 10.1016/j.ygcen.2014.07.025
60. González-Martínez D, Zmora N, Zanuy S, Sarasquete C, Elizur A, Kah O, et al. Developmental expression of three different prepro-GnRH (gonadotrophin-releasing hormone) messengers in the brain of the European sea bass (Dicentrarchus labrax). J Chem Neuroanat. (2002) 23:255–67. doi: 10.1016/S0891-0618(02)00004-2
61. González-Martínez D, Zmora N, Saligaut D, Zanuy S, Elizur A, Kah O, et al. New insights in developmental origins of different GnRH (gonadotrophin-releasing hormone) systems in perciform fish: an immunohistochemical study in the European sea bass (Dicentrarchus labrax). J Chem Neuroanat. (2004) 28:1–15. doi: 10.1016/j.jchemneu.2004.05.001
62. Kah O, Lethimonier C, Somoza G, Guilgur LG, Vaillant C, Lareyre JJ. GnRH and GnRH receptors in metazoa: a historical, comparative, and evolutive perspective. Gen Comp Endocrinol. (2007) 153:346–64. doi: 10.1016/j.ygcen.2007.01.030
63. Parhar IS, Ogawa S, Ubuka T. Reproductive neuroendocrine pathways of social behavior. Front Endocrinol. (2016) 7:28. doi: 10.3389/fendo.2016.00028.
64. Kawai T, Oka Y, Eisthen H. The role of the terminal nerve and GnRH in olfactory system neuromodulation. Zoolog Sci. (2009) 26:669–80. doi: 10.2108/zsj.26.669
65. Umatani C, Misu R, Oishi S, Yamaguchi K, Abe H, Oka Y. GnRH suppresses excitability of visual processing neurons in the optic tectum. J Neurophysiol. (2015) 114:2775–84. doi: 10.1152/jn.00710.2015
66. Guilgur LG, Moncaut NP, Canário AV, Somoza GM. Evolution of GnRH ligands and receptors in gnathostomata. Comp Biochem Physiol. (2006) 144:272–83. doi: 10.1016/j.cbpa.2006.02.016
67. Kriegsfeld LJ, Mei DF, Bentley GE, Ubuka T, Mason AO, Inoue K, et al. Identifcation and characterization of a gonadotropin-inhibitory system in the brains of mammals. Proc Natl Acad Sci USA. (2006) 103:2410–5. doi: 10.1073/pnas.0511003103
68. Tsutsui K, Ubuka T. GnIH Control of Feeding and Reproductive Behaviors. Front Endocrinol. (2016) 7:170. doi: 10.3389/fendo.2016.00170
69. Peragine DE, Pokarowski M, Mendoza-Viveros L, Swift-Gallant A, Cheng HM, Bentley GE, et al. RFamide-related peptide-3 (RFRP-3) suppresses sexual maturation in a eusocial mammal. Proc Natl Acad Sci USA. (2017) 114:1207–12. doi: 10.1073/pnas.1616913114
70. Di Yorio MP, Pérez Sirkin DI, Muñoz-Cueto JA, Delgadin TH, Tsutsui K, Somoza GM, et al. Morphological relationship between GnIH and GnRH neurons in the brain of the neotropical cichlid fish Cichlasoma dimerus. Gen Comp Endocrinol. (2018). doi: 10.1016/j.ygcen.2018.06.010. [Epub ahead of print].
71. Muñoz-Cueto JA, Cowan ME, Loentgen GH, Aliaga-Guerrero M, Fuentès M, Besseau L, et al. The gonadotropin-inhibitory hormone system of fish: the case of sea bass (Dicentrarchus labrax. In: 11th International Symposium on Reproductive Physiology of Fish. Manaus (2018).
72. Servili A, Lethimonier C, Lareyre JJ, López-Olmeda JF, Sánchez-Vázquez FJ, Kah O, et al. The highly conserved gonadotropin-releasing hormone-2 form acts as a melatonin-releasing factor in the pineal of a teleost fish, the European sea bass Dicentrarchus labrax. Endocrinol (2010) 151:2265–75. doi: 10.1210/en.2009-1207
73. Servili A, Herrera-Pérez P, Yáñez J, Muñoz-Cueto JA. Afferent and efferent connections of the pineal organ in the European sea bass Dicentrarchus labrax: a carbocyanine dye tract-tracing study. Brain Behav Evol. (2011) 78, 272–85. doi: 10.1159/000330824
74. Moussavi M, Wlasichuk M, Chang JP, Habibi HR. Seasonal effect of gonadotrophin inhibitory hormone on gonadotrophin-releasing hormone-induced gonadotroph functions in the goldfish pituitary. J Neuroendocrinol. (2013) 25:506–516. doi: 10.1111/jne.12024
75. Moussavi M, Wlasichuk M, Chang JP, Habibi HR. Seasonal effects of GnIH on basal and GnRH-induced goldfish somatotrope functions. J Endocrinol. (2014) 223:191–202. doi: 10.1530/JOE-14-0441
76. Paullada-Salmerón JA, Cowan M, Aliaga-Guerrero M, Morano F, Zanuy S, Muñoz-Cueto JA. Gonadotropin inhibitory hormone down-regulates the brain-pituitary reproductive axis of male European sea bass (Dicentrarchus labrax). Biol Reprod. (2016) 94:1–11. doi: 10.1095/biolreprod.116.139022
77. Paullada-Salmerón JA, Cowan M, Aliaga-Guerrero M, López-Olmeda JF, Mañanós EL, Zanuy S, et al. Testicular steroidogenesis and locomotor activity are regulated by gonadotropin-inhibitory hormone in male European sea bass. PLoS ONE (2016) 11:e0165494. doi: 10.1371/journal.pone.0165494
78. Branco GS, Melo AG, Ricci JMB, Digmayer M, de Jesus LWO, Habibi HR, et al. Effects of GnRH and the dual regulatory actions of GnIH in the pituitary explants and brain slices of Astyanax altiparanae males. Gen. Comp. Endocrinol. (2018). doi: 10.1016/j.ygcen.2018.08.006. [Epub ahead of print].
79. Ubuka T, Parhar I. Dual actions of mammalian and piscine gonadotropin-inhibitory hormones, RFamide-related peptides and LPXRFamide peptides, in the hypothalamic-pituitary-gonadal axis. Front Endocrinol. (2018) 8:377. doi: 10.3389/fendo.2017.00377
80. Son YL, Ubuka T, Millar RP, Kanasaki H, Tsutsui K. Gonadotropin-inhibitory hormone inhibits GnRH-induced gonadotropin subunit gene transcriptions by inhibiting AC/cAMP/PKA-dependent ERK pathway in LβT2 cells. Endocrinol (2012) 153:2332–43. doi: 10.1210/en.2011-1904
81. Wu M, Dumalska I, Morozova E, van den Pol AN, Alreja M. Gonadotropin inhibitory hormone inhibits basal forebrain vGluT2-gonadotropin-releasing hormone neurons via a direct postsynaptic mechanism. J. Physiol. (2009) 587:1401–11. doi: 10.1113/jphysiol.2008.16644748
82. Wang B, Yang G, Liu Q, Qin J, Xu Y, Li W, et al. Inhibitory action of tongue sole LPXRFa, the piscine ortholog of gonadotropin-inhibitory hormone, on the signaling pathway induced by tongue sole kisspeptin in COS-7 cells transfected with their cognate receptors. Peptides (2017) 95:62–7. doi: 10.1016/j.peptides.2017.07.014
83. Wang B, Yang G, Xu Y, Li W, Liu X. Recent studies of LPXRFa receptor signaling in fish and other vertebrates. Gen. Comp. Endocrinol. (2018). doi: 10.1016/j.ygcen.2018.11.011. [Epub ahead of print].
84. Sandvik GK, Hodne K, Haug TM, Okubo K, Weltzien FA. Review: RFamide peptides in early vertebrate development. Front Endocrinol. (2014) 5:203. doi: 10.3389/fendo.2014.00203
85. Pandolfi M, Parhar IS, Ravaglia MA, Meijide FJ, Maggese MC, Paz DA. Ontogeny and distribution of gonadotropin-releasing hormone (GnRH) neuronal systems in the brain of the cichlid fish Cichlasoma dimerus. Anat Embryol. (2002) 205:271–81. doi: 10.1007/s00429-002-0253-x
86. Strüssmann CA, Saito T, Usui M, Yamada H, Takashima F. Thermal thresholds and critical period of thermolabile sex determination in two atherinid fishes, Odontesthes bonariensis and Patagonina hatcheri. J Experiment Zool. (1997) 278:167–77.
87. Ospina-Alvarez N, Piferrer F. Temperature-dependent sex determination in fish revisited: prevalence, a single sex ratio response pattern, and possible effects of climate change. PLoS ONE (2008) 3:e2837. doi: 10.1371/journal.pone.0002837
88. Shahjahan M, Doi H, Ando H. LPXRFamide peptide stimulates growth hormone and prolactin gene expression during the spawning period in the grass puffer, a semi-lunar synchronized spawner. Gen Comp Endocrinol. (2016) 227:77–83. doi: 10.1016/j.ygcen.2015.09.008
89. Peng C, Gallin W, Peter RE, Blomqvist AG, Larhammar D. Neuropeptide-Y gene expression in the goldfish brain: distribution and regulation by ovarian steroids. Endocrinol (1994) 134:1095 e1103. doi: 10.1210/endo.134.3.8119148
90. Cerda-Reverter JM, Anglade I, Martínez-Rodríguez G, Mazurais D, Muñoz-Cueto JA, Carrillo M, et al. Characterization of neuropeptide Y expression in the brain of a perciform fish, the sea bass (Dicentrarchus labrax). J Chem Neuroanat. (2000) 19:197–210. doi: 10.1016/S0891-0618(00)00063-6
91. Rodríguez-Gómez FJ, Rendón-Unceta MC, Sarasquete C, Muñoz-Cueto JA. Distribution of neuropeptide Y-like immunoreactivity in the brain of the Senegalese sole (Solea senegalensis). Anatomical. Record. (2001) 262: 227–37. doi: 10.1002/1097-0185(20010301)262:3%3C227::AID-AR1027%3E3.0.CO;2-X
92. Shahjahan M, Kitahashi T, Parhar IS. Central pathways integrating metabolism and reproduction in teleosts. Front Endocrinol. (2014) 5:36. doi: 10.3389/fendo.2014.00036
93. Tang H, Liu Y, Luo D, Ogawa S, Yin Y, Li S, et al. The kiss/kissr systems are dispensable for zebrafish reproduction: evidence from gene knockout studies. Endocrinology (2015) 156:589–99. doi: 10.1210/en.2014-1204
94. Spicer OS, Wong TT, Zmora N, Zohar Y. Targeted mutagenesis of the hypophysiotropic Gnrh3 in zebrafish (Danio rerio) reveals no effects on reproductive performance. PLoS ONE (2016) 11:e0158141. doi: 10.1371/journal.pone.0158141
95. Liu Y, Tang H, Xie R, Li S, Liu X, Lin H, et al. Genetic evidence for multifactorial control of the reproductive axis in zebrafish. Endocrinology (2017) 158:604–11. doi: 10.1210/en.2016-1540
96. Trudeau VL. Facing the challenges of neuropeptide gene knockouts: why do they not inhibit reproduction in adult teleost fish? Front Neurosci. (2018) 12:302. doi: 10.3389/fnins.2018.00302
Keywords: GnIH, fish, brain, ontogeny, GnRH, growth, reproduction
Citation: Di Yorio MP, Muñoz-Cueto JA, Paullada-Salmerón JA, Somoza GM, Tsutsui K and Vissio PG (2019) The Gonadotropin-Inhibitory Hormone: What We Know and What We Still Have to Learn From Fish. Front. Endocrinol. 10:78. doi: 10.3389/fendo.2019.00078
Received: 03 December 2018; Accepted: 28 January 2019;
Published: 19 February 2019.
Edited by:
Honoo Satake, Suntory Foundation for Life Sciences, JapanReviewed by:
Hironori Ando, Niigata University, JapanSatoshi Ogawa, Monash University Malaysia, Malaysia
Copyright © 2019 Di Yorio, Muñoz-Cueto, Paullada-Salmerón, Somoza, Tsutsui and Vissio. This is an open-access article distributed under the terms of the Creative Commons Attribution License (CC BY). The use, distribution or reproduction in other forums is permitted, provided the original author(s) and the copyright owner(s) are credited and that the original publication in this journal is cited, in accordance with accepted academic practice. No use, distribution or reproduction is permitted which does not comply with these terms.
*Correspondence: Paula G. Vissio, cHZpc3Npb0BnbWFpbC5jb20=