- 1International Research Center–CIPE, A. C. Camargo Cancer Center, São Paulo, Brazil
- 2Department of Pathology, A. C. Camargo Cancer Center, São Paulo, Brazil
- 3Department of Pathology, São Rafael Hospital, Salvador, Brazil
- 4Gonçalo Moniz Institute, Fiocruz, Salvador, Brazil
- 5Health Technology Institute, SENAI CIMATEC, Salvador, Brazil
- 6Department of Head and Neck Surgery and Otorhinolaryngology, A. C. Camargo Cancer Center, São Paulo, Brazil
- 7Department of Clinical Genetics, Vejle University Hospital, Institute of Regional Health Research, University of Southern Denmark, Odense, Denmark
Currently, there is a lack of efficient recurrence prediction methods for papillary thyroid carcinoma (PTC). In this study, we enrolled 202 PTC patients submitted to total thyroidectomy and radioiodine therapy with long-term follow-up (median = 10.7 years). The patients were classified as having favorable clinical outcome (PTC-FCO, no disease in the follow-up) or recurrence (PTC-RE). Alterations in BRAF, RAS, RET, and TERT were investigated (n = 202) and the transcriptome of 48 PTC (>10 years of follow-up) samples was profiled. Although no mutation was associated with the recurrence risk, 68 genes were found as differentially expressed in PTC-RE compared to PTC-FCO. Pathway analysis highlighted a potential role of cancer-related pathways, including signal transduction and FoxO signaling. Among the eight selected genes evaluated by RT-qPCR, SLC2A4 and GADD45B showed down-expression exclusively in the PTC-FCO group compared to non-neoplastic tissues (NT). Increased expression of GADD45B was an independent marker of shorter disease-free survival [hazard ratio (HR) 2.9; 95% confidence interval (CI95) 1.2–7.0] in our cohort and with overall survival in the TCGA dataset (HR = 4.38, CI95 1.2–15.5). In conclusion, GADD45B transcript was identified as a novel prognostic marker candidate in PTC patients treated with total thyroidectomy and radioiodine therapy.
Introduction
The incidence of thyroid carcinoma has tripled over the last 35 years, affecting more than 560,000 people worldwide in 2018 (1). Papillary thyroid carcinoma (PTC) represents 80–85% of all thyroid cancer, presenting a high cure rate and a 5-years overall survival of 98% (2). However, recurrence is a frequent event (10–25%) related to patient morbidity and may occur over 20 years after the initial treatment (3, 4). Clinical-pathological features, such as distant and lymph node metastasis, extrathyroidal extension, and tall cell histologic variant, are associated with more aggressive PTC (5). Nonetheless, the discovery of reliable biomarkers to determine the risk of relapse could be of great value in clinical practice. Low-risk PTC may be eligible for minimalistic surgical approaches (such as thyroid lobectomy) or active surveillance. On the other hand, a more aggressive intervention (such as total thyroidectomy, prophylactic neck dissection, or radioiodine therapy) could be reserved for high risk PTC (5).
The most common genetic driver alterations found in PTC are BRAF (60%) and RAS (13%) point mutations, TERT promoter mutation (9%), and RET/PTC fusion (6%) (6). BRAF and TERT mutations have been frequently associated with more aggressive thyroid carcinomas (7–10). Although the coexistence of both alterations has a synergic effect (11), their role in the prognosis of PTC is still controversial (12, 13).
Gene expression profiling has been widely evaluated in thyroid cancer for biomarker discovery, especially for diagnostic purposes (14–17). Transcriptomic-based studies have revealed predictors candidates of prognosis, including overexpression of MUC1 (18), MEDAG (19), and SPHK1 (20), and down-expression of FMO1 (21), and FOXF1 (22). Signatures of multigene classifiers were also reported (23, 24). Although many prognostic candidates have been suggested, most of them were not confirmed in distinct cohorts (25). The inclusion of a limited number of patients treated with or without radioiodine and followed by short periods could explain the lack of reproducibility.
In this study, we evaluated a cohort of 202 PTC patients (69% of them with more than 10 years of follow-up) with standardized treatment (total thyroidectomy and radioiodine therapy). We reported that the most common genomic alterations (BRAF, RAS, RET, and TERT) found in PTC were not related to the recurrence risk in the long-term follow-up. The transcriptomic profiling (microarray) revealed potential recurrence biomarkers, of which a higher expression of GADD45B (Growth arrest and DNA-damage-inducible, beta) was an independent marker of shorter disease-free survival (confirmed by RT-qPCR).
Materials and Methods
Patient Selection Criteria
Patients with pathological confirmation of PTC treated from July 2001 to December 2010 at A. C. Camargo Cancer Center, São Paulo, Brazil, were retrospectively included in this study. The samples were selected according to the availability of fresh-frozen tissues at our BioBank. The Ethics Committee in Human Research for the Institution approved this study (Protocol n° 1410/10), which was conducted according to the Helsinki Declaration. The tumor specimens obtained from the thyroidectomy were reviewed by an experienced pathologist (CP) using blinded interpretation.
In order to standardize the treatment strategy used in our cohort, only patients submitted to total thyroidectomy followed by radioiodine therapy were enrolled. Patients with other cancer types prior to the thyroid cancer diagnosis were excluded to avoid bias in the prognostic analysis. We also excluded samples with low RNA quality (RNA integrative number < 5). Patients with no evidence of active disease at the follow-up, defined as negative image test by ultrasonography and serum thyroglobulin (< 1 ng/mL with suppressed TSH), were classified as having favorable clinical outcome (FCO). Recurrence (RE) was defined as persistent or recurrent PTC after the definitive treatment with pathologic confirmation (fine-needle aspiration biopsy or surgery) or combined imaging (Computed Tomography or Positron Emission Tomography with Computed Tomography) and strong biochemical evidence (persistent serum thyroglobulin> 2 ng/ml with suppressed TSH< 0.1 mIU/L or thyroglobulin> 5 ng/ml with induced TSH> 30 mIU/L). Due to the presence of late recurrence during the natural history of PTC (3), we have included only patients followed up for more than 5 years in the FCO group. Based on the fact that the microarray assays were used as a “discovery set,” we adopted a minimum follow-up of 10 years.
Following these criteria, a total of 202 patients were included (Table S1). The sample distribution according to the molecular approaches is summarized in Figure S1. We also included 15 non-neoplastic thyroid (NT) tissues in the RT-qPCR analysis. The NT tissues were obtained from surrounding PTC samples showing no histological alterations, hyperplastic, or inflammatory changes in the remaining thyroid parenchyma.
Detection of Genomic Alterations
Nucleic acids (DNA and RNA) were isolated, as previously described (26). Adequate quantity and quality for 202 DNA and 178 RNA PTC specimens were obtained. Point mutations in BRAF (codon 600), KRAS (codon 12/13), HRAS (codon 61), and KRAS (codon 61) were evaluated by pyrosequencing and RET rearrangements (RET/PTC1 and RET/PTC3) by RT-qPCR, as previously described (27). TERT promoter mutations (C228T and C250T hotspots) were investigated by direct Sanger sequencing, as described elsewhere (28).
Gene Expression Profiling
Gene expression microarray experiments were performed in 48 PTC using the SurePrint G3 8x60K platform (Agilent Technologies Inc., Santa Clara, CA, USA), co-hybridized with a pool of nine non-neoplastic thyroid tissues, as previously described (15). This data was generated in a previous study (15) and is available in the GEO database (accession number GSE50901). The probes representing protein-coding genes were selected and quantile-normalized using BRB ArrayTools software (v. 4.4.0). Groups were compared using the limma package (P < 0.01) (29), adopting a fold change (FC) ≥ 1.5 to define differential expression. Since male patients usually present a worse prognosis (30), representing a potential bias in our study, genes found as more or less expressed according to gender and mapped in X or Y chromosomes were excluded. Hierarchical clustering analysis was performed with Euclidean distance and complete linkage using ComplexHeatmap package (31) available for R program.
In silico Molecular Analysis
Genes differentially expressed identified in the microarray analysis were subjected to an in silico exploration, employing two pathway-enrichment tools, KOBAS (v.3.0; kobas.cbi.pku.edu.cn/) and pathDIP (http://ophid.utoronto.ca/pathdip/), using KEGG, Reactome and PANTHER databases. Experimentally detected and computational predicted protein-protein interactions (minimum confidence level for predicted associations of 99%) were used in the pathDIP tool, while literature curated known pathway memberships were used in KOBAS. Pathways highlighted by both tools were designated as putatively disrupted (hypergeometric test with Benjamini and Hochberg correction P < 0.05).
Reverse Transcription Quantitative PCR (RT-qPCR) Analysis
Eight genes (ELMO1, F2RL2, FOXP2, GADD45B, HGD, JUND, S1PR1, and SLC2A4) were selected for RT-qPCR investigation using TaqMan Low Density Arrays® (TLDA; Applied Biosystems, Foster City, CA, USA) in 72 PTC, including 38 samples tested prior by microarray, 34 independent cases, and 15 additional non-neoplastic thyroid samples (histological normal pattern tissue surrounding tumor). The gene selection considered the P-value (FOXP2, GADD45B, HGD, JUND, and SLC2A4 were among the top 15 lowest P-values), fold change (F2RL2 had the highest FC) and pathway analysis (ELMO, GADD45B, S1PR1, and SLC2A4 were members of FoxO signaling or Signal Transduction pathways). Two references (EIF2B1 and PUM1) were selected among five transcripts (18S, EIF2B1, PUM1, TBP, and YWHAZ) using geNorm (32) to obtain the normalized target gene relative expression. GADD45B (target) and reference genes (EIF2B1 and PUM1) were further evaluated in 106 PTCs using individual Taqman assays (Applied Biosystems, Hs04188837_g1, Hs00426752_m1 and Hs00472881_m1, respectively). The reactions were assembled in duplicates (10 ng of cDNA) according to the manufacturer instructions, using automatic pipetting (QIAgility, QIAGEN, Courtaboeuf, France). The amplifications were carried out with 7900HT Real Time PCR System (Applied Biosystems). Normalization was implemented following the Pfaffl method (33).
TCGA Database
Disease-free survival, overall survival, GADD45B expression (RNA sequencing, log2 transformed RSEM+1), and BRAF mutation (exome sequencing) data from PTC patients were retrieved from the UCSC Xena Browser (https://xenabrowser.net/datapages/, accessed in October 2019). In total, 490 PTC subjects had both follow-up and gene expression information available for the analysis.
Statistical Analysis
Statistical analysis and illustrations were performed with BRB ArrayTools (v. 4.4.0), SPSS (v. 21.0; SPSS, Chicago, IL, USA) and Graphpad Prism (v. 5.0; GraphPad Software Inc., La Jolla, CA, USA) software. Genomic alterations were confronted with clinical-pathological features using Fisher exact test with multiple hypothesis correction (Bonferroni test). Relative expression obtained by RT-qPCR was compared among biological groups with Student t-test and ANOVA (Tukey post-hoc test). A two-tailed P < 0.05 value was adopted as significant. Gene expression values were dichotomized in bellow and above the median (RT-qPCR from our cohort and RNA sequencing from TCGA) to perform the survival analyses. The Kaplan–Meier method was used to plot the disease-free and overall survival. Cox proportional-hazards regression was used in the univariate and multivariate survival analysis to estimate the hazard ratio (HR) and 95% confidence intervals (CI95). Variables significantly associated (P < 0.05) in the univariate were included in the multivariate model (conditional backward elimination) (SPSS (v. 21.0; SPSS, Chicago, IL, USA).
Results
PTC Relapse Risk Was Not Associated With BRAF, RAS, RET, and TERT Alterations
We detected BRAFV600E mutation in 62.4% (126/202) of our cases. RAS mutation was found in 2.5% (5/198) (all in NRAS), TERT promoter mutations in 2.6% (5/193; 1 C228T and 4 C250T), and RET rearrangement in 9.2% (16/174; 11 RET/PTC1 and 5 RET/PTC3) of the tumors. Only two cases presented concurrent alterations in BRAF, RAS, and RET, one classical variant (BRAF and RET/PTC), and one follicular variant (BRAF with NRAS). Four of five TERT positive cases also presented BRAF mutations. These four BRAF/TERT concurrent mutations were from patients older than 55 years, tumors larger than 1 cm with extrathyroidal extension. Three were classic variants, and the patients had a favorable clinical outcome. One patient presented a diffuse sclerosing variant of PTC that progressed with distant metastasis and died due to the disease. Tumors harboring BRAF mutations were correlated with the classical variant of PTC (P = 0.007) and the presence of extra-thyroidal extension (P = 0.025). However, no significant association was found after a multiple-comparison correction (Table 1). RAS mutations were prevalent in the follicular variant, TERT in older patients, and RET/PTC in patients with lymph node metastasis (all significant after the multiple-comparison correction). No significant difference was observed between the assessed alterations with the risk of relapse (Table 1).
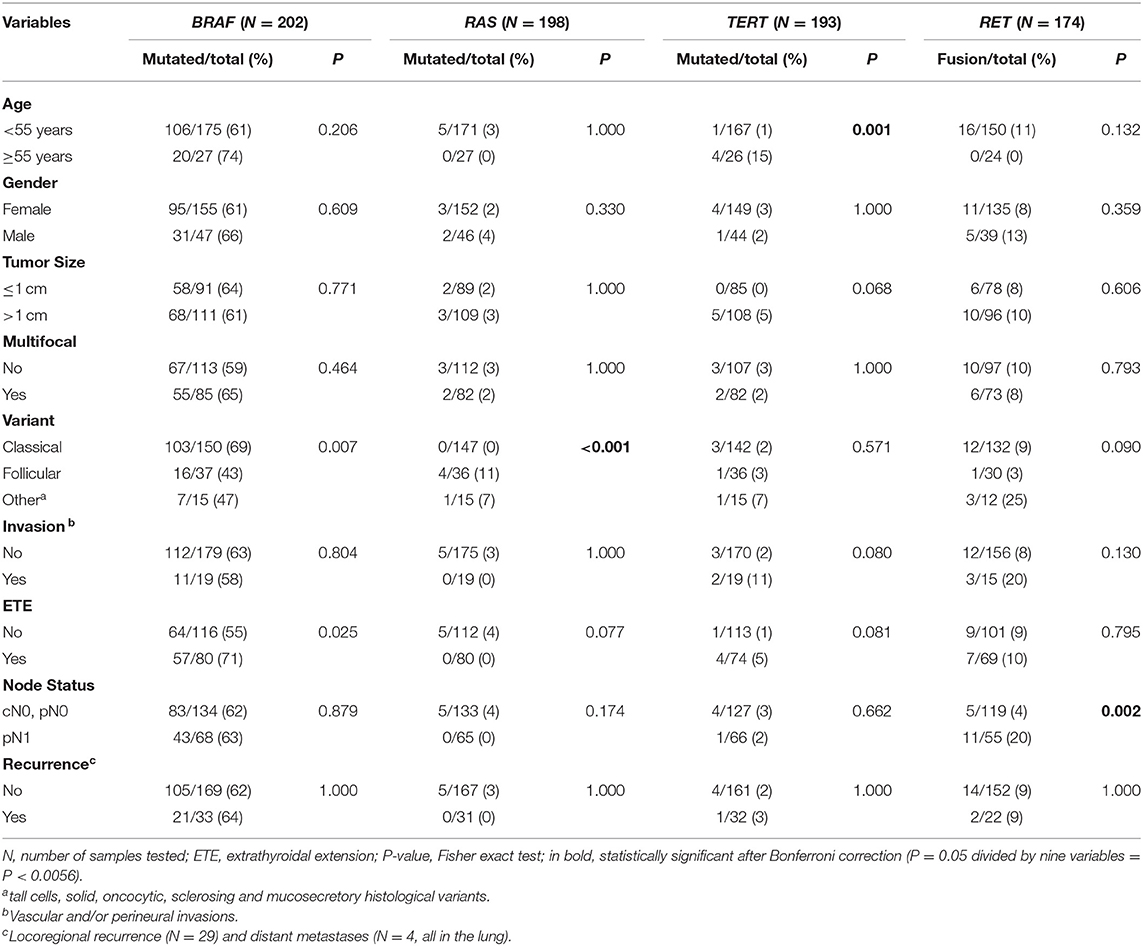
Table 1. Clinical-pathological characteristics according to the status of BRAF, RAS, and TERT mutations and RET rearrangements in papillary thyroid carcinomas.
Gene Expression Profile as a Predictor of PTC Recurrence
Gene expression profile of PTC from patients with recurrence (PTC-RE) was compared with PTC from patients with favorable clinical outcome (PTC-FCO). The microarray analysis unveiled 61 differentially expressed genes (17 less and 44 more expressed in PTC-RE compared to PTC-FCO) (Table S2). A supervised hierarchical clustering analysis including the differentially expressed genes revealed a “high risk” group comprising eight of 13 PTC-RE, and a “low risk” group containing 34 of 35 PTC-FCO (Figure 1).
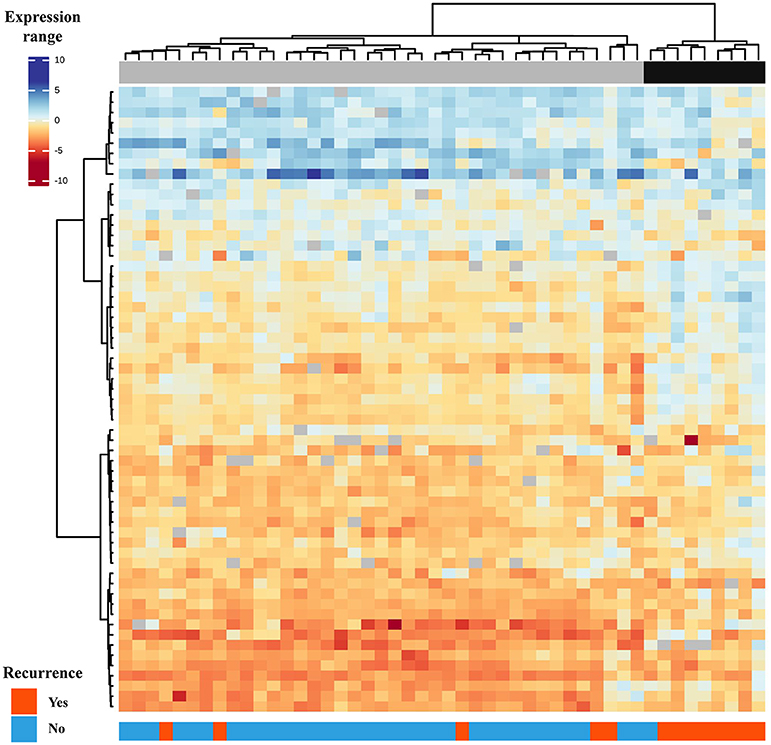
Figure 1. Supervised hierarchical clustering analysis comprising 61 genes differentially expressed in primary PTC-RE compared to PTC-FCO (samples in columns and genes in rows). Two major groups are shown: the first (gray) is enriched by patients with favorable clinical outcomes and the second (black) by patients who relapsed in the follow-up.
Potentially Disrupted Pathways Associated With Recurrence in PTC
To better understand the gene list obtained in the microarray analysis, we performed an in silico molecular analysis using pathDIP (http://ophid.utoronto.ca/pathdip/) and KOBAS (v.3.0; kobas.cbi.pku.edu.cn/) tools. We found an enrichment of signal transduction, peptide ligand-binding receptors, FoxO signaling, and platelet activation, signaling and aggregation pathways (P adjusted < 0.05) (Table 2).
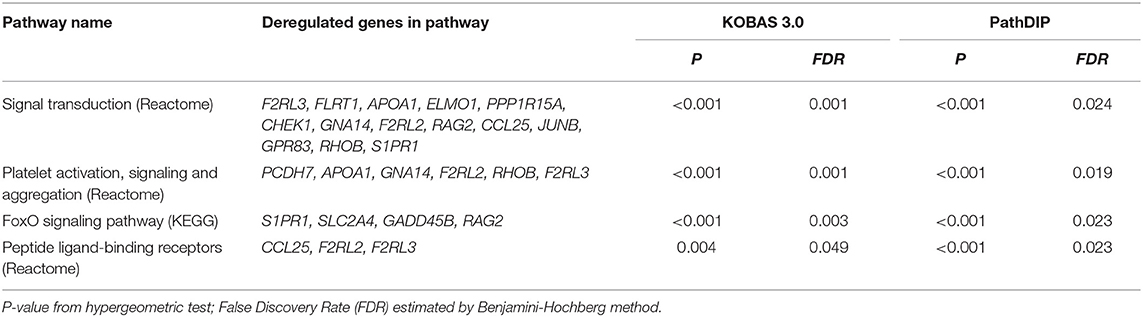
Table 2. Biological pathways potentially altered in PTC from relapsed patients using KOBAS 3.0 and PathDip tools.
Confirmation of Genes Differentially Expressed in PTC by RT-qPCR and Their Association With Clinical Outcome
Eight targets (ELMO1, F2RL2, FOXP2, GADD45B, HGD, JUND, S1PR1, and SLC2A4) and two reference genes (EIF2B1 and PUM1) were assayed by RT-qPCR (N = 72; TLDA method). ELMO1, FOXP2, HGD, and JUND were less expressed, and F2RL2 and S1PR1 more expressed in both PTCs groups compared to non-neoplastic thyroid tissues. GADD45B and SLC2A4 were less expressed only in the PTC-FCO group compared to NT. However, only GADD45B showed a significant difference between the PTC-RE and PTC-FCO (more expressed in PTC-RE) (Figure 2).
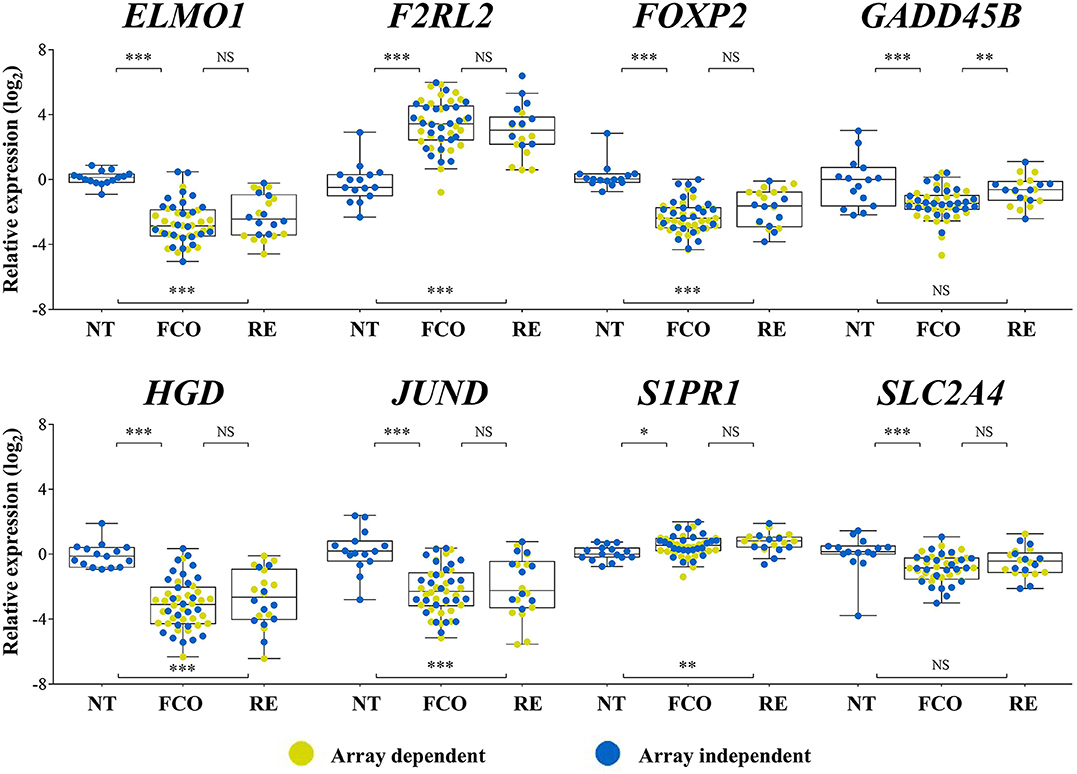
Figure 2. Expression levels of eight genes evaluated by RT-qPCR (TLDA custom assay) in PTC samples previously investigated by microarray analysis (yellow dots) and in an array-independent set of samples (blue dots). GADD45B showed statistically significant higher expression levels in PTC-RE compared to PTC-FCO cases. FCO, favorable clinical outcome; RE, recurrence; ***P < 0.001; **P < 0.01; *P < 0.05; NS, not significant (Tukey post-hoc test).
GADD45B Expression as a Prognostic Marker
A total of 106 PTC samples with available RNA was used to evaluate the GADD45B expression level by RT-qPCR assays using EIF2B1 and PUM1 as references. Combining both PCR sets (TLDA and Taqman individual assay), a cohort with 178 PTC samples was established. A higher expression of GADD45B (median expression as the threshold) was confirmed as a factor related to shorter disease-free survival (HR = 3.6, CI95 1.5–8.4; P = 0.003) (Figure 3A). Multivariate analysis revealed that GADD45B and cervical lymph node metastasis are independent predictors markers of relapse (P = 0.015, HR = 2.9, and P = 0.009, HR = 3.0, respectively) (Table 3).
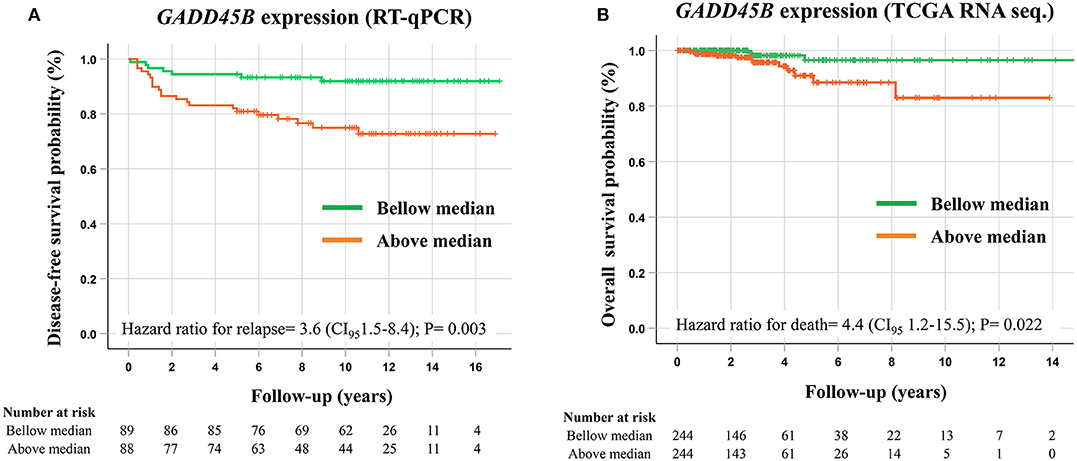
Figure 3. Survival analysis performed according to the GADD45B expression levels. (A) Kaplan–Meier plot demonstrating shorter disease-free survival in patients presenting higher GADD45B expression levels (above median) evaluated by RT-qPCR. (B) Kaplan–Meier plot showing a shorter overall survival in cases presenting higher GADD45B expression in PTC patients from the TCGA database (RNA sequencing). P-values were obtained by Cox proportional-hazards regression, and median expression was used as a cut-off.
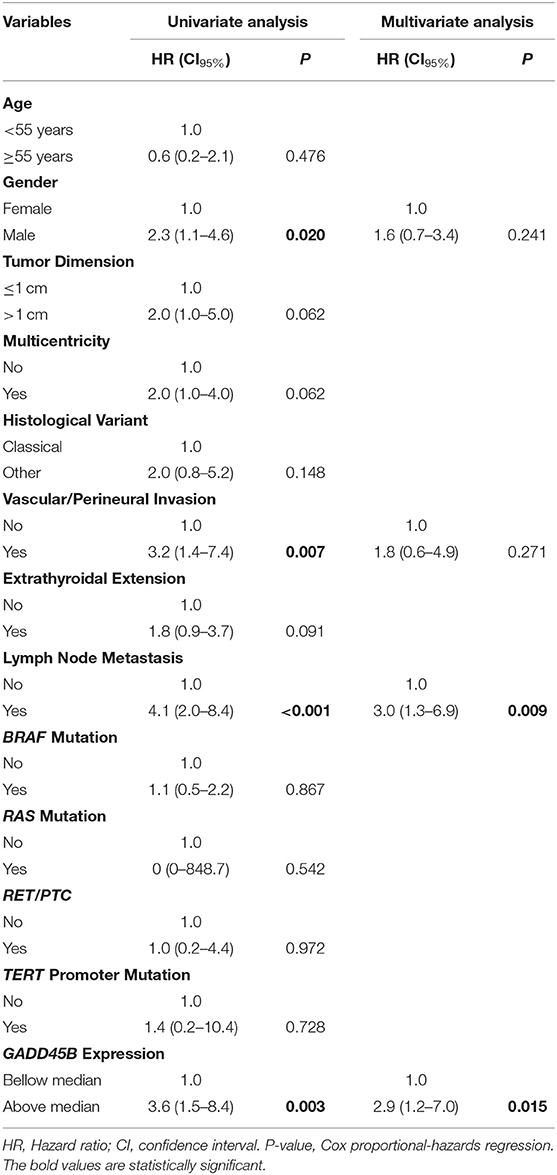
Table 3. Univariate and multivariate analysis, contrasting the risk of relapse of PTC patients with clinical, pathological and molecular features.
Using the RNA sequencing data of PTC (N = 490) from the TCGA database (no standardized treatment), GADD45B also exhibited a prognostic role, being more expressed in patients with shorter overall survival (overall survival analysis: HR = 4.38, CI95 1.2–15.5; P = 0.022) (Figure 3B). However, no association with the recurrence risk was observed (disease-free survival analysis: HR = 0.69, CI95 0.35–1.4; P = 0.279) (Figure S2).
Discussion
In general, PTC is an indolent disease and recurrence can appear long periods after surgery, making the identification of molecular prognostic markers a challenge (34). Herein, we investigated the most common gene alterations described in PTC, as well as transcriptomic data, to identify markers able to anticipate the outcome of patients treated with total thyroidectomy followed by radioiodine therapy. Only patients with a minimum follow-up of 10 years were included in the large-scale gene expression analysis, while specific mutations/rearrangements and mRNAs levels were evaluated in patients followed for at least 5 years.
Among the 202 PTC cases evaluated in this study, BRAF mutation was detected with a high frequency (62.4%), while RAS mutation (2.5%), RET fusions (9.2%), and TERT promoter mutation (2.6%) were uncommon. These frequencies are comparable to the ones available in the TCGA database (BRAF: 59.7%, RAS: 13% RET: 6.3% TERT: 9.4%) (6). Although reports in the literature show that BRAF and TERT mutations are related to aggressive thyroid tumors (8, 9, 35, 36), no association was found with the recurrence risk in our set of cases. This result may be explained by the inclusion of patients treated exclusively by total thyroidectomy and radioiodine therapy. This criterion was adopted to avoid treatment-related bias, which resulted in the exclusion of very low-risk cases and enriched our sample set with a more aggressive phenotype.
Our results were consistent with the association amongst BRAF mutation with extra-thyroidal extension and the classical histological variant (6, 35, 37). Albeit rare in our cohort, RAS mutation was associated with the follicular variant, as previously described (6, 38, 39). Five of 16 RET/PTC cases were RET/PTC3 (NCOA4-RET translocation), an alteration frequently associated with ionizing radiation (40). Nonetheless, those patients have not declared any known prior exposure to radiation. Similar to previous reports, RET/PTC inversion was associated with lymph node involvement (39, 41). However, RET fusions are often observed in young patients, who have a higher frequency of lymph node metastases (39, 42). We found that 44% (7/16) of our RET/PTC positive cases were from patients younger than 30 years, compared to only 16% (25/158) of the RET/PTC negative cases. Conversely, TERT promoter mutation was previously described as being predominantly found in older patients (6, 43), as we observed in our dataset (range of 54–66 years). Telomerase activation is essential to cancer development by keeping the telomere length and overcoming senescence (44). Thyroid follicle cells from old individuals are TERT-deficient and present short-length telomeres (43). In older patients, TERT promoter mutation is suggested to be a consequence of the constant proliferation and activation of the telomerase, due to telomere crisis (43). Despite the small number of cases (N = 4) harboring both BRAFV600E and TERT promoter mutation, their clinical-pathological profile suggests a more aggressive phenotype (older patients with larger tumors and extrathyroidal extension). Even though only two patients from our whole cohort died due to the disease, one of them presented a BRAF/TERT concurrent mutation (diffuse sclerosing variant of PTC).
To our knowledge, no previous study has used high-throughput gene expression analysis to evaluate PTC cases with standardized treatment and long-term follow-up. Although the analysis of a homogenous cohort can eliminate the influence of some confounding factors, different histological types are frequently compared (18, 23, 45). The molecular basis of the thyroid tumor de-differentiation was studied by gene expression microarray in tumors with different degrees of aggressiveness (31 well-differentiated and 13 poorly/undifferentiated thyroid carcinomas) (23). A signature of 29 genes correctly separates 96% of tumors (42/44) according to prognosis, by grouping well-differentiated carcinomas that relapsed together with poorly/undifferentiated carcinomas (23). However, the authors included patients followed for almost 19 years in the unfavorable prognosis group and cases accompanied for <1 year categorized in the good prognosis group. A panel of 63 proteins (tissue microarray) was assessed in 12 anaplastic thyroid cancer associated with a well-differentiated component (45). The authors reported that the expression pattern of eight proteins (β-catenin, E-cadherin, thyroglobulin, topoisomerase IIα, VEGF, p53, BCL-2, and MIB-1) was able to separate anaplastic tumors from their differentiated components with 96% accuracy. Similarly, the signature of 61 differentially expressed genes found in our study was able to correctly classify PTC-RE from PTC-FCO with 87.5% accuracy (61.5% sensitivity and 97.5% specificity). No overlap between the markers found in our study with those aforementioned was found.
Among the pathways enriched by the differentially expressed genes found in our study was the FoxO signaling. This pathway is mainly activated by extracellular pro-apoptotic signals via membrane receptors, promoting downstream activation of forkhead box O3 (FOXO3), and inducing the expression of pro-apoptotic genes in the nucleus (46, 47). BRAFV600E directly inhibits the pro-apoptotic signals from the FoxO pathway (46). Therefore, this pathway is fundamental for the molecular pathogenesis of PTC (48). SLC2A4 (also known as GLUT4), GADD45B, S1PR1, and RAG1 are downstream factors induced by the FoxO pathway (49–52). These transcripts are more expressed in PTC-RE compared to PTC-FCO. However, only GADD45B was confirmed by RT-qPCR with the inclusion of a new group of samples (SLC2A4 and S1PR1 were also tested). Curiously, lower GADD45B expression was also associated with BRAF mutation in our dataset (by RT-qPCR) and confirmed in the TCGA dataset (RNA sequencing for GADD45B analysis and exome sequencing for BRAF genotyping) (Figure S3).
GADD45B is a member of the GADD45 family (Growth arrest and DNA-damage-inducible), which regulates cell proliferation through the participation of DNA replication and repair mechanisms (53), G2/M checkpoint control (54), and apoptosis (55). GADD45 family genes are rapidly induced in response to a variety of stress signals, such as ionizing radiation, pro-apoptotic inflammatory cytokines, mitogen stimulation, and xenobiotics (56). In contrast to the pro-apoptotic effect of GADD45A and GADD45G (57), GADD45B presents dual pro and anti-apoptotic roles (58). The mechanism responsible for inhibiting apoptosis has already been shown to attenuate JNK activation (c-Jun N-terminal kinase) (59) and induce p53 degradation (58). Decreased GADD45B gene expression levels have been described in several human tumors, such as lymphoma, thyroid, breast, cervical, lung, and esophageal cancers, often by epigenetic regulation (60–64). Conversely, increased GADD45B expression levels were associated with shorter recurrence-free and overall survival in the most prevalent and aggressive human cancer types (65). Since we have included non-neoplastic thyroid samples in the RT-qPCR analysis, it was possible to note that GADD45B was underexpressed exclusively in the PTC-FCO. GADD45B showed high expression variability in NT samples, in agreement with the TCGA dataset (Figure S3). It has been proposed that genetic and epigenetic alterations can occur in the earliest carcinogenesis steps, which can also be detected in histological “normal” tissues surrounding tumors (66, 67). GADD45B deficient cells have been reported to be more sensitive to ultraviolet light-induced apoptosis (68). On the other hand, increased GADD45B expression has been related to chemotherapy resistance (69) and survival of tumor cells resistant to ultraviolet light and gamma radiation in medium with low nutrient availability (70). Hence, it is possible that GADD45B deficient PTCs are more susceptible to radioiodine therapy (all patients included in our study received radioiodine therapy after surgery).
Higher expression of GADD45B was an independent factor for shorter disease-free survival in our internal dataset and shorter overall survival in the TCGA cohort. Likewise, high GADD45B protein expression was an independent marker of poorer prognosis in stage II colorectal cancer and a potential marker to indicate post-operative chemotherapy (71). In thyroid cancer, an accurate recurrence predictive biomarker could aid in the de-intensification of the treatment in low risk patients (72). Even though it is widely used and proven to be effective, radioiodine therapy enhances the risk for second primary tumors (mainly hematological malignancies) and alterations in salivary glands (73, 74). The evaluation of GADD45B expression could be incorporated in combination with clinical-pathological information in the routine to aid in the risk stratification of PTC patients. This analysis could be performed using RT-qPCR assay in post-surgical tumor samples, which has the potential to improve the indication and the intensity of radioiodine therapy and TSH suppression, and the medical surveillance frequency. Although a protein analysis using immunohistochemistry could improve even more the applicability of the test in the clinical setting, the RT-qPCR is more sensitive and has wider dynamic range quantification.
In conclusion, we showed that increased expression of GADD45B was an independent marker of poor prognosis of PTC, whereas genomic alterations in BRAF, RAS, RET, and TERT were not associated with the risk of recurrence in our cohort of PTC patients treated with total thyroidectomy and radioiodine therapy in a long-term follow-up.
Data Availability Statement
All large scale data analyzed in this study are publicly available: GEO database (accession number GSE50901) and TCGA consortium (UCSC Xena Browser; https://xenabrowser.net/datapages/).
Ethics Statement
The studies involving human participants were reviewed and approved by Ethics Committee in Human Research of A. C. Camargo Cancer Center Protocol n° 1410/10. The patients/participants provided their written informed consent to participate in this study.
Author Contributions
MB-F, SR, and LK conceived and designed the study. MB-F and JM conducted the experiments. MB-F and FM performed bioinformatics analyses. MB-F, PD, and IS performed statistical analyses. CP performed the histopathological evaluation. JM, PD, IS, CP, and MS contributed to data interpretation. All authors participated in the preparation and approved the final version of the manuscript.
Funding
This researched was funded by grants from the Fundação de Amparo à Pesquisa do Estado de São Paulo (FAPESP) (2015/17707-5 and 2015/20748-5).
Conflict of Interest
The authors declare that the research was conducted in the absence of any commercial or financial relationships that could be construed as a potential conflict of interest.
Supplementary Material
The Supplementary Material for this article can be found online at: https://www.frontiersin.org/articles/10.3389/fendo.2020.00269/full#supplementary-material
Supplementary Figure 1. Flowchart summarizing the number of cases evaluated according to each methodology applied in the study. We excluded: °two samples due to poor quality of the Sanger sequencing; and #four samples without conclusive results for KRAS, HRAS and NRAS mutation. *Four samples were not tested for RET/PTC (RNA was used for expression assays); +TaqMan Low Density Arrays® (TLDA); aTaqman individual assay.
Supplementary Figure 2. Kaplan–Meier plot comparing the disease-free survival of PTC patients from TCGA according to the GADD45B expression (RNA sequencing). P values were obtained by Cox proportional-hazards regression and median expression was used as cut-off.
Supplementary Figure 3. GADD45B expression levels according to the BRAF mutation status. (A) Boxplots illustrating the GADD45B transcript evaluated by RT-qPCR in our internal sample set (NT = 15; PTC BRAF−= 61; PTC BRAF+= 118). (B) GADD45B transcript evaluated by RNA sequencing in the TCGA dataset (NT = 59; PTC BRAF−= 196; PTC BRAF+= 273). NT: non-neoplastic thyroid tissue; PTC: papillary thyroid carcinoma; BRAF−: Negative for BRAF mutation; BRAF+: Positive for BRAF mutation; ****P < 0.001; *P < 0.05; NS: not significant (Tukey post-hoc test).
References
1. Ferlay J, Colombet M, Soerjomataram I, Mathers C, Parkin DM, Pineros M, et al. Estimating the global cancer incidence and mortality in 2018: GLOBOCAN sources and methods. Int J Cancer. (2019) 144:1941–53. doi: 10.1002/ijc.31937
2. Lim H, Devesa SS, Sosa JA, Check D, Kitahara CM. Trends in thyroid cancer incidence and mortality in the united states, 1974–2013. JAMA. (2017) 317:1338–48. doi: 10.1001/jama.2017.2719
3. Hay ID, Thompson GB, Grant CS, Bergstralh EJ, Dvorak CE, Gorman CA, et al. Papillary thyroid carcinoma managed at the mayo clinic during six decades (1940–1999): temporal trends in initial therapy and long-term outcome in 2,444 consecutively treated patients. World J Surg. (2002) 26:879–85. doi: 10.1007/s00268-002-6612-1
4. Mazzaferri EL, Jhiang SM. Long-term impact of initial surgical and medical therapy on papillary and follicular thyroid cancer. Am J Med. (1994) 97:418–28. doi: 10.1016/0002-9343(94)90321-2
5. Tuttle RM, Alzahrani AS. Risk stratification in differentiated thyroid cancer: from detection to final follow-up. J Clin Endocrinol Metab. (2019) 104:4087-100. doi: 10.1210/jc.2019-00177
6. Cancer Genome Atlas Research Network. Integrated genomic characterization of papillary thyroid carcinoma. Cell. (2014). 159:676–90. doi: 10.1016/j.cell.2014.09.050
7. Liu C, Chen T, Liu Z. Associations between BRAF(V600E) and prognostic factors and poor outcomes in papillary thyroid carcinoma: a meta-analysis. World J Surg Oncol. (2016) 14:241. doi: 10.1186/s12957-016-0979-1
8. Pozdeyev N, Gay LM, Sokol ES, Hartmaier R, Deaver KE, Davis S, et al. Genetic analysis of 779 advanced differentiated and anaplastic thyroid cancers. Clin Cancer Res. (2018) 24:3059–68. doi: 10.1158/1078-0432.ccr-18-0373
9. Krasner JR, Alyouha N, Pusztaszeri M, Forest VI, Hier MP, Avior G, et al. Molecular mutations as a possible factor for determining extent of thyroid surgery. J Otolaryngol Head Neck Surg. (2019) 48:51. doi: 10.1186/s40463-019-0372-5
10. Rusinek D, Pfeifer A, Krajewska J, Oczko-Wojciechowska M, Handkiewicz-Junak D, Pawlaczek A, et al. Coexistence of TERT promoter mutations and the BRAF V600E alteration and its impact on histopathological features of papillary thyroid carcinoma in a selected series of polish patients. Int J Mol Sci. (2018) 19:2647. doi: 10.3390/ijms19092647
11. Xing M, Liu R, Liu X, Murugan AK, Zhu G, Zeiger MA, et al. BRAF V600E and TERT promoter mutations cooperatively identify the most aggressive papillary thyroid cancer with highest recurrence. J Clin Oncol. (2014) 32:2718–26. doi: 10.1200/jco.2014.55.5094
12. Gouveia C, Can NT, Bostrom A, Grenert JP, van Zante A, Orloff LA. Lack of association of BRAF mutation with negative prognostic indicators in papillary thyroid carcinoma: the University of California, San Francisco, experience. JAMA Otolaryngol Head Neck Surg. (2013) 139:1164–70. doi: 10.1001/jamaoto.2013.4501
13. de Biase D, Gandolfi G, Ragazzi M, Eszlinger M, Sancisi V, Gugnoni M, et al. TERT Promoter mutations in papillary thyroid microcarcinomas. Thyroid. (2015) 25:1013–9. doi: 10.1089/thy.2015.0101
14. Alexander EK, Kennedy GC, Baloch ZW, Cibas ES, Chudova D, Diggans J, et al. Preoperative diagnosis of benign thyroid nodules with indeterminate cytology. N Engl J Med. (2012) 367:705–15. doi: 10.1056/NEJMoa1203208
15. Barros-Filho MC, Marchi FA, Pinto CA, Rogatto SR, Kowalski LP. High diagnostic accuracy based on CLDN10, HMGA2, and LAMB3 transcripts in papillary thyroid carcinoma. J Clin Endocrinol Metab. (2015) 100:E890–9. doi: 10.1210/jc.2014-4053
16. Wojtas B, Pfeifer A, Oczko-Wojciechowska M, Krajewska J, Czarniecka A, Kukulska A, et al. Gene expression (mRNA) Markers for differentiating between malignant and benign follicular thyroid tumours. Int J Mol Sci. (2017) 18:e1184. doi: 10.3390/ijms18061184
17. Macerola E, Poma AM, Proietti A, Romani R, Torregrossa L, Ugolini C, et al. Digital gene expression analysis on cytology smears can rule-out malignancy in follicular-patterned thyroid tumors. J Mol Diagn. (2019) 22:179–87. doi: 10.1016/j.jmoldx.2019.09.008
18. Wreesmann VB, Sieczka EM, Socci ND, Hezel M, Belbin TJ, Childs G, et al. Genome-wide profiling of papillary thyroid cancer identifies MUC1 as an independent prognostic marker. Cancer Res. (2004) 64:3780–9. doi: 10.1158/0008-5472.can-03-1460
19. Song Y, Fu LJ, Li HT, Qiu XG. Evaluation of MEDAG gene expression in papillary thyroid microcarcinoma: associations with histological features, regional lymph node metastasis and prognosis. Sci Rep. (2019) 9:5800. doi: 10.1038/s41598-019-41701-4
20. Li J, Zhang B, Bai Y, Liu Y, Jin J. Upregulation of sphingosine kinase 1 is associated with recurrence and poor prognosis in papillary thyroid carcinoma. Oncol Lett. (2019) 18:5374–82. doi: 10.3892/ol.2019.10910
21. Luo J, Zhang B, Cui L, Liu T, Gu Y. FMO1 gene expression independently predicts favorable recurrence-free survival of classical papillary thyroid cancer. Future Oncol. (2019) 15:1303–11. doi: 10.2217/fon-2018-0885
22. Gu Y, Hu C. Bioinformatic analysis of the prognostic value and potential regulatory network of FOXF1 in papillary thyroid cancer. Biofactors. (2019) 45:902–11. doi: 10.1002/biof.1561
23. Montero-Conde C, Martin-Campos JM, Lerma E, Gimenez G, Martinez-Guitarte JL, Combalia N, et al. Molecular profiling related to poor prognosis in thyroid carcinoma. Combining gene expression data and biological information. Oncogene. (2008) 27:1554–61. doi: 10.1038/sj.onc.1210792
24. Londero SC, Jespersen ML, Krogdahl A, Bastholt L, Overgaard J, Schytte S, et al. Gene-expression classifier in papillary thyroid carcinoma: validation and application of a classifier for prognostication. Anticancer Res. (2016) 36:749–56. Available online at: http://ar.iiarjournals.org/content/36/2/749.long
25. Kempf E, de Beyer JA, Cook J, Holmes J, Mohammed S, Nguyen TL, et al. Overinterpretation and misreporting of prognostic factor studies in oncology: a systematic review. Br J Cancer. (2018) 119:1288–96. doi: 10.1038/s41416-018-0305-5
26. Barros-Filho MC, Dos Reis MB, Beltrami CM, de Mello JBH, Marchi FA, Kuasne H, et al. DNA methylation-based method to differentiate malignant from benign thyroid lesions. Thyroid. (2019) 29:1244–54. doi: 10.1089/thy.2018.0458
27. Beltrami CM, Dos Reis MB, Barros-Filho MC, Marchi FA, Kuasne H, Pinto CAL, et al. Integrated data analysis reveals potential drivers and pathways disrupted by DNA methylation in papillary thyroid carcinomas. Clin Epigenetics. (2017) 9:45. doi: 10.1186/s13148-017-0346-2
28. Camargo Barros-Filho M, Barreto Menezes de Lima L, Bisarro Dos Reis M, Bette Homem de Mello J, Moraes Beltrami C, Lopes Pinto CA, et al. PFKFB2 promoter hypomethylation as recurrence predictive marker in well-differentiated thyroid carcinomas. Int J Mol Sci. (2019) 20:e61334. doi: 10.3390/ijms20061334
29. Ritchie ME, Phipson B, Wu D, Hu Y, Law CW, Shi W, et al. limma powers differential expression analyses for RNA-sequencing and microarray studies. Nucleic Acids Res. (2015) 43:e47. doi: 10.1093/nar/gkv007
30. Zahedi A, Bondaz L, Rajaraman M, Leslie WD, Jefford C, Young JE, et al. Risk for Thyroid cancer recurrence is higher in men than in women independent of disease stage at presentation. Thyroid. (2019). doi: 10.1089/thy.2018.0775. [Epub ahead of print].
31. Gu Z, Eils R, Schlesner M. Complex heatmaps reveal patterns and correlations in multidimensional genomic data. Bioinformatics. (2016) 32:2847–9. doi: 10.1093/bioinformatics/btw313
32. Vandesompele J, De Preter K, Pattyn F, Poppe B, Van Roy N, De Paepe A, et al. Accurate normalization of real-time quantitative RT-PCR data by geometric averaging of multiple internal control genes. Genome Biol. (2002) 3:e0034. doi: 10.1186/gb-2002-3-7-research0034
33. Pfaffl MW. A new mathematical model for relative quantification in real-time RT-PCR. Nucleic Acids Res. (2001) 29:e45. doi: 10.1093/nar/29.9.e45
34. Dong W, Horiuchi K, Tokumitsu H, Sakamoto A, Noguchi E, Ueda Y, et al. Time-Varying pattern of mortality and recurrence from papillary thyroid cancer: lessons from a long-term follow-Up. Thyroid. (2019) 29:802–8. doi: 10.1089/thy.2018.0128
35. Kim TH, Park YJ, Lim JA, Ahn HY, Lee EK, Lee YJ, et al. The association of the BRAF(V600E) mutation with prognostic factors and poor clinical outcome in papillary thyroid cancer: a meta-analysis. Cancer. (2012) 118:1764–73. doi: 10.1002/cncr.26500
36. Donati B, Ciarrocchi A. Telomerase and telomeres biology in thyroid cancer. Int J Mol Sci. (2019) 20:e2887. doi: 10.3390/ijms20122887
37. Oler G, Cerutti JM. High prevalence of BRAF mutation in a Brazilian cohort of patients with sporadic papillary thyroid carcinomas: correlation with more aggressive phenotype and decreased expression of iodide-metabolizing genes. Cancer. (2009) 115:972–80. doi: 10.1002/cncr.24118
38. Zhu Z, Gandhi M, Nikiforova MN, Fischer AH, Nikiforov YE. Molecular profile and clinical-pathologic features of the follicular variant of papillary thyroid carcinoma. An unusually high prevalence of ras mutations. Am J Clin Pathol. (2003) 120:71–7. doi: 10.1309/nd8d-9laj-trct-g6qd
39. Adeniran AJ, Zhu Z, Gandhi M, Steward DL, Fidler JP, Giordano TJ, et al. Correlation between genetic alterations and microscopic features, clinical manifestations, and prognostic characteristics of thyroid papillary carcinomas. Am J Surg Pathol. (2006) 30:216–22. doi: 10.1097/01.pas.0000176432.73455.1b
40. Nikiforov YE, Rowland JM, Bove KE, Monforte-Munoz H, Fagin JA. Distinct pattern of ret oncogene rearrangements in morphological variants of radiation-induced and sporadic thyroid papillary carcinomas in children. Cancer Res. (1997) 57:1690–4.
41. Wang YL, Zhang RM, Luo ZW, Wu Y, Du X, Wang ZY, et al. High frequency of level II-V lymph node involvement in RET/PTC positive papillary thyroid carcinoma. Eur J Surg Oncol. (2008) 34:77–81. doi: 10.1016/j.ejso.2007.08.012
42. Collins BJ, Chiappetta G, Schneider AB, Santoro M, Pentimalli F, Fogelfeld L, et al. RET expression in papillary thyroid cancer from patients irradiated in childhood for benign conditions. J Clin Endocrinol Metab. (2002) 87:3941–6. doi: 10.1210/jcem.87.8.8748
43. Liu T, Wang N, Cao J, Sofiadis A, Dinets A, Zedenius J, et al. The age- and shorter telomere-dependent TERT promoter mutation in follicular thyroid cell-derived carcinomas. Oncogene. (2014) 33:4978–84. doi: 10.1038/onc.2013.446
44. Yuan X, Larsson C, Xu D. Mechanisms underlying the activation of TERT transcription and telomerase activity in human cancer: old actors and new players. Oncogene. (2019) 38:6172–83. doi: 10.1038/s41388-019-0872-9
45. Wiseman SM, Griffith OL, Deen S, Rajput A, Masoudi H, Gilks B, et al. Identification of molecular markers altered during transformation of differentiated into anaplastic thyroid carcinoma. Arch Surg. (2007) 142:717–27. doi: 10.1001/archsurg.142.8.717
46. Ikenoue T, Hikiba Y, Kanai F, Tanaka Y, Imamura J, Imamura T, et al. Functional analysis of mutations within the kinase activation segment of B-Raf in human colorectal tumors. Cancer Res. (2003) 63:8132–7. Available online at: https://cancerres.aacrjournals.org/content/63/23/8132
47. Lehtinen MK, Yuan Z, Boag PR, Yang Y, Villen J, Becker EB, et al. A conserved MST-FOXO signaling pathway mediates oxidative-stress responses and extends life span. Cell. (2006) 125:987–1001. doi: 10.1016/j.cell.2006.03.046
48. Xing M. Molecular pathogenesis and mechanisms of thyroid cancer. Nat Rev Cancer. (2013) 13:184–99. doi: 10.1038/nrc3431
49. Nakae J, Biggs WH 3rd, Kitamura T, Cavenee WK, Wright CV, Arden KC, et al. Regulation of insulin action and pancreatic beta-cell function by mutated alleles of the gene encoding forkhead transcription factor Foxo1. Nat Genet. (2002) 32:245–53. doi: 10.1038/ng890
50. Tran H, Brunet A, Grenier JM, Datta SR, Fornace AJ Jr., et al. DNA repair pathway stimulated by the forkhead transcription factor FOXO3a through the Gadd45 protein. Science. (2002) 296:530–4. doi: 10.1126/science.1068712
51. Amin RH, Schlissel MS. Foxo1 directly regulates the transcription of recombination-activating genes during B cell development. Nat Immunol. (2008) 9:613–22. doi: 10.1038/ni.1612
52. Fabre S, Carrette F, Chen J, Lang V, Semichon M, Denoyelle C, et al. FOXO1 regulates L-Selectin and a network of human T cell homing molecules downstream of phosphatidylinositol 3-kinase. J Immunol. (2008) 181:2980–9. doi: 10.4049/jimmunol.181.5.2980
53. Smith ML, Ford JM, Hollander MC, Bortnick RA, Amundson SA, Seo YR, et al. p53-mediated DNA repair responses to UV radiation: studies of mouse cells lacking p53, p21, and/or gadd45 genes. Mol Cell Biol. (2000) 20:3705–14. doi: 10.1128/mcb.20.10.3705-3714.2000
54. Vairapandi M, Azam N, Balliet AG, Hoffman B, Liebermann DA. Characterization of MyD118, Gadd45, and proliferating cell nuclear antigen (PCNA) interacting domains. PCNA impedes MyD118 AND Gadd45-mediated negative growth control. J Biol Chem. (2000) 275:16810–9. doi: 10.1074/jbc.275.22.16810
55. Takekawa M, Saito H. A family of stress-inducible GADD45-like proteins mediate activation of the stress-responsive MTK1/MEKK4 MAPKKK. Cell. (1998) 95:521–30. doi: 10.1016/s0092-8674(00)81619-0
56. Moskalev AA, Smit-McBride Z, Shaposhnikov MV, Plyusnina EN, Zhavoronkov A, Budovsky A, et al. Gadd45 proteins: relevance to aging, longevity and age-related pathologies. Ageing Res Rev. (2012) 11:51–66. doi: 10.1016/j.arr.2011.09.003
57. Song L, Li J, Zhang D, Liu ZG, Ye J, Zhan Q, et al. IKKbeta programs to turn on the GADD45alpha-MKK4-JNK apoptotic cascade specifically via p50 NF-kappaB in arsenite response. J Cell Biol. (2006) 175:607–17. doi: 10.1083/jcb.200602149
58. Yu Y, Huang H, Li J, Zhang J, Gao J, Lu B, et al. GADD45beta mediates p53 protein degradation via Src/PP2A/MDM2 pathway upon arsenite treatment. Cell Death Dis. (2013) 4:e637. doi: 10.1038/cddis.2013.162
59. Papa S, Zazzeroni F, Bubici C, Jayawardena S, Alvarez K, Matsuda S, et al. Gadd45 beta mediates the NF-kappa B suppression of JNK signalling by targeting MKK7/JNKK2. Nat Cell Biol. (2004) 6:146–53. doi: 10.1038/ncb1093
60. Ying J, Srivastava G, Hsieh WS, Gao Z, Murray P, Liao SK, et al. The stress-responsive gene GADD45G is a functional tumor suppressor, with its response to environmental stresses frequently disrupted epigenetically in multiple tumors. Clin Cancer Res. (2005) 11:6442–9. doi: 10.1158/1078-0432.ccr-05-0267
61. Zerbini LF, Libermann TA. GADD45 deregulation in cancer: frequently methylated tumor suppressors and potential therapeutic targets. Clin Cancer Res. (2005) 11:6409–13. doi: 10.1158/1078-0432.ccr-05-1475
62. Guo W, Dong Z, Guo Y, Chen Z, Kuang G, Yang Z. Methylation-mediated repression of GADD45A and GADD45G expression in gastric cardia adenocarcinoma. Int J Cancer. (2013) 133:2043–53. doi: 10.1002/ijc.28223
63. Iacobas DA, Tuli NY, Iacobas S, Rasamny JK, Moscatello A, Geliebter J, et al. Gene master regulators of papillary and anaplastic thyroid cancers. Oncotarget. (2018) 9:2410–24. doi: 10.18632/oncotarget.23417
64. Do H, Kim D, Kang J, Son B, Seo D, Youn H, et al. TFAP2C increases cell proliferation by downregulating GADD45B and PMAIP1 in non-small cell lung cancer cells. Biol Res. (2019) 52:35. doi: 10.1186/s40659-019-0244-5
65. Verzella D, Bennett J, Fischietti M, Thotakura AK, Recordati C, Pasqualini F, et al. GADD45beta loss ablates innate immunosuppression in cancer. Cancer Res. (2018) 78:1275–92. doi: 10.1158/0008-5472.can-17-1833
66. Feinberg AP, Ohlsson R, Henikoff S. The epigenetic progenitor origin of human cancer. Nat Rev Genet. (2006) 7:21–33. doi: 10.1038/nrg1748
67. Yim JH, Choi AH, Li AX, Qin H, Chang S, Tong ST, et al. Identification of Tissue-Specific DNA Methylation signatures for thyroid nodule diagnostics. Clin Cancer Res. (2019) 25:544–51. doi: 10.1158/1078-0432.CCR-18-0841
68. Gupta M, Gupta SK, Hoffman B, Liebermann DA. Gadd45a and Gadd45b protect hematopoietic cells from UV-induced apoptosis via distinct signaling pathways, including p38 activation and JNK inhibition. J Biol Chem. (2006) 281:17552–8. doi: 10.1074/jbc.M600950200
69. Cheng TC, Manorek G, Samimi G, Lin X, Berry CC, Howell SB. Identification of genes whose expression is associated with cisplatin resistance in human ovarian carcinoma cells. Cancer Chemother Pharmacol. (2006) 58:384–95. doi: 10.1007/s00280-005-0171-8
70. Engelmann A, Speidel D, Bornkamm GW, Deppert W, Stocking C. Gadd45 beta is a pro-survival factor associated with stress-resistant tumors. Oncogene. (2008) 27:1429–38. doi: 10.1038/sj.onc.1210772
71. Zhao Z, Gao Y, Guan X, Liu Z, Jiang Z, Liu X, et al. GADD45B as a prognostic and predictive biomarker in stage ii colorectal cancer. (2018) 9:e70361. doi: 10.3390/genes9070361
72. Lowenstein LM, Basourakos SP, Williams MD, Troncoso P, Gregg JR, Thompson TC, et al. Active surveillance for prostate and thyroid cancers: evolution in clinical paradigms and lessons learned. Nat Rev Clin Oncol. (2019) 16:168–84. doi: 10.1038/s41571-018-0116-x
73. Teng CJ, Hu YW, Chen SC, Yeh CM, Chiang HL, Chen TJ, et al. Use of radioactive iodine for thyroid cancer and risk of second primary malignancy: a nationwide population-based study. J Natl Cancer Inst. (2016) 361:108. doi: 10.1093/jnci/djv314
74. Selvakumar T, Nies M, Klein Hesselink MS, Brouwers AH, van der Horst-Schrivers ANA, Klein Hesselink EN, et al. Long-term effects of radioiodine treatment on salivary gland function in adult survivors of pediatric differentiated thyroid carcinoma. J Nucl Med. (2018) 60:2 172–177. doi: 10.2967/jnumed.118.212449
Keywords: papillary thyroid cancer, prognostic markers, BRAF mutation, GADD45B, TERT promoter mutation, transcription profiling
Citation: Barros-Filho MC, de Mello JBH, Marchi FA, Pinto CAL, da Silva IC, Damasceno PKF, Soares MBP, Kowalski LP and Rogatto SR (2020) GADD45B Transcript Is a Prognostic Marker in Papillary Thyroid Carcinoma Patients Treated With Total Thyroidectomy and Radioiodine Therapy. Front. Endocrinol. 11:269. doi: 10.3389/fendo.2020.00269
Received: 19 February 2020; Accepted: 14 April 2020;
Published: 30 April 2020.
Edited by:
Christoph Reiners, University Hospital Würzburg, GermanyReviewed by:
Vasyl Vasko, Uniformed Services University of the Health Sciences, United StatesTrevor Edmund Angell, University of Southern California, United States
Copyright © 2020 Barros-Filho, de Mello, Marchi, Pinto, da Silva, Damasceno, Soares, Kowalski and Rogatto. This is an open-access article distributed under the terms of the Creative Commons Attribution License (CC BY). The use, distribution or reproduction in other forums is permitted, provided the original author(s) and the copyright owner(s) are credited and that the original publication in this journal is cited, in accordance with accepted academic practice. No use, distribution or reproduction is permitted which does not comply with these terms.
*Correspondence: Mateus C. Barros-Filho, mfilho@accamargo.org.br; Silvia R. Rogatto, silvia.regina.rogatto@rsyd.dk