- 1Institute of Marine Research (IIM), National Research Council (CSIC), Vigo, Spain
- 2Department of Cell Biology, Physiology and Immunology, Universidad Autónoma de Barcelona, Bellaterra, Spain
In recent years, the innate immune response has gained importance since evidence indicates that after an adequate priming protocol, it is possible to obtain some prolonged and enhanced immune responses. Nevertheless, several factors, such as the timing and method of administration of the immunostimulants, must be carefully considered. An inappropriate protocol can transform the treatments into a double-edged sword for the teleost immune system, resulting in a stressful and immunosuppressive state. In this work, we analyzed the long-term effects of different stimuli (β-glucans, lipopolysaccharide, and polyinosinic:polycytidylic acid) on the transcriptome modulation induced by Spring Viremia Carp Virus (SVCV) in adult zebrafish (Danio rerio) and on the mortality caused by this infection. At 35 days post-immunostimulation, the transcriptome was found to be highly altered compared to that of the control fish, and these stimuli also conditioned the response to SVCV challenge, especially in the case of β-glucans. No protection against SVCV was found with any of the stimuli, and non-significant higher mortalities were even observed, especially with β-glucans. However, in the short term (pre-stimulation with β-glucan and infection after 7 days), slight protection was observed after infection. The transcriptome response in the zebrafish kidney at 35 days posttreatment with β-glucans revealed a significant response associated with stress and immunosuppression. The identification of genes that were differentially expressed before and after the infection seemed to indicate a high energy cost of the immunostimulation that was prolonged over time and could explain the lack of protection against SVCV. Differential responses to stress and alterations in lipid metabolism, the tryptophan–kynurenine pathway, and interferon-gamma signaling seem to be some of the mechanisms involved in this response, which represents the end of trained immunity and the beginning of a stressful state characterized by immunosuppression.
Introduction
Studies on the effect of immunostimulants in fish aquaculture have been conducted in past decades (1). These immunostimulants include different pathogen-associated molecular patterns (PAMPs), such as polyinosinic:polycytidylic acid [poly(I:C)], lipopolysaccharide (LPS), and β-glucans (2). PAMPs are recognized by host cells through specific pattern-recognition receptors (PRRs), which are germline-encoded host sensors with a key role in innate immunity (3). These PRRs can be proteins expressed mainly in the cell membrane or endosomes of innate immune cells, such as toll-like receptors, cytoplasmic receptors, such as RIG-I-like receptors or NOD-like receptors, or secreted receptors, such as mannose-binding lectin (MBL) of the complement system (3, 4). Nevertheless, the PRRs that sense LPS and β-glucans have not been completely identified in teleosts (5, 6).
Many PRRs can share several signaling pathways, and they have the ability to influence the type, intensity, and duration of the immune response (7). Signaling through PRRs after a first immune stimulus may result in cell reprogramming, which changes and conditions the immune response triggered by a second stimulus. This reprogramming is a host adaptation that coordinates multiple receptors and reflects the plasticity of innate immunity (8).
The term “trained immunity” was coined by Netea et al. in 2011 (9) to refer to cross-protection between infections that occurs independently of T and B cells or to the enhanced non-specific innate immune protection elicited by microbial components or PAMPs. This innate memory is mainly provided by NK cells and macrophages. It has been shown that the priming (or training) of mice with several PAMPs, such as β-glucans, peptidoglycans, or flagellin, can protect against a subsequent lethal infection (10). In contrast, LPS-induced tolerance has been described as the transformation of macrophages to a state that is much less responsive to subsequent challenges with LPS. Such a response should protect organisms from hyperinflammation or sepsis (11). Nevertheless, this suppression can become pernicious when the organism has to fight against a pathogen. Both tolerance and training involve long-term epigenetic reprogramming of the innate immune system after the first contact with a particular pathogen or PAMP (12–14).
It has been demonstrated that β-glucans, which are probably the most commonly used immunostimulant in fish aquaculture, are able to induce metabolic changes that reflect a shift from oxidative phosphorylation to aerobic glycolysis (Warburg effect) during the establishment of trained immunity (15). Because increasing evidence indicates a close relation between metabolism and epigenetic reprogramming (16, 17), the effect of β-glucans on metabolism could mediate changes in the epigenetic pattern. Other metabolic pathways that are independent of glucose metabolism can play a role in the induction of trained immunity by β-glucans (18). Although the identity of the PRR responsible for recognizing β-glucans in fish remains unknown (19), mammals sense this PAMP via dectin-1, a C-type lectin receptor that is absent in fish genomes (20). In mammals, training immunity occurs in a dectin-1-dependent manner in macrophages (21).
The outcome of β-glucan stimulation depends on several factors, such as the administration route and fish species, and its protective effect depends on the infectious agent (19). It is also important to keep in mind that only a very small fraction of orally administered β-glucans pass through the intestine to the circulation (22). Therefore, the intraperitoneal administration of β-glucans can provide higher levels of protection, even after a single injection (19). There are many works based on studying the effect of β-glucan pretreatment on the resolution of bacterial infections in fish after either oral or intraperitoneal administration (23–27). However, investigations of resistance against viral infections after β-glucan stimulation in teleosts (28–32), or in vertebrates in general (33–35), are scarce.
The main premise that must be taken into account when considering a substance as a good immunostimulant is the absence of pathogenic, toxic, or other undesirable effects after administration to aquatic organisms. Nevertheless, the administration of a certain immunostimulant can be a stressful stimulus and produce indirect pernicious effects at the immune level. In non-mammalian vertebrates, such as fish, the relation between stress and the immune system is more evident. Neuropeptides and cytokines play roles in the immune and neuroendocrine systems and belong to the same family of molecules (36, 37). A prompt but intense stimulus can result in acute stress; therefore, long-lasting effects on several stress- and immune-related parameters could be observed. Because the head kidney of fish is a unique structure in vertebrates that performs important functions for the endocrine, nervous, and the immune systems (36), it is an ideal tissue for studying the potential effects of immunostimulants.
In this work, we analyzed the long-lived response induced by three PAMPs [LPS, poly(I:C), and β-glucans] in zebrafish at 35 days posttreatment and investigated their effects on survival after Spring Viremia Carp Virus (SVCV) infection. None of the treatments induced protection against viral challenge, and non-significant higher mortalities were even found in the PAMP-treated groups. Kidney samples were collected from non-challenged and SVCV-challenged fish after 24 h, and microarray analyses were conducted. Special attention was paid to β-glucans, which induced a stronger and more specific transcriptome modulation in both uninfected and infected zebrafish. The transcriptome response at 35 days posttreatment with β-glucans revealed interesting changes that could be related to the stress response, immunosuppression, or tolerance. This response to β-glucans seems to be mediated by the interplay among different metabolic and immune processes, such as the alteration of lipid metabolism, the tryptophan–kynurenine pathway and interferon-gamma signaling.
Materials and Methods
Fish, Viruses, and Bacteria
Zebrafish were obtained from our experimental facility, where the fish were cultured using established protocols (38, 39) (see http://zfin.org/zf_info/zfbook/zfbk.html). In this work, adult wild-type zebrafish were used for transcriptome and mortality analyses. Double-transgenic Tg(mpeg:mCherry/mpx:GFP) zebrafish embryos, in which macrophages are labeled red and neutrophils are labeled green, and wild-type embryos were used in this work for microinjection experiments. Zebrafish were euthanized using a tricaine methanesulfonate (MS-222) overdose (500 mg/l−1). For microinjection experiments, larvae were anesthetized by adding two drops of a 0.05% MS-222 solution to a Petri plate with 10 ml of water. Fish care and the challenge experiments were conducted according to the guidelines of the CSIC National Committee on Bioethics under approval number ES360570202001/16/FUN01/PAT.05/tipoE/BNG.
Spring Viremia Carp Virus isolate 56/70 was propagated on epithelioma papulosum cyprini (EPC) carp cells (ATCC CRL-2872) containing MEM (Gibco) supplemented with 2% FBS (Gibco) and 100 µg/ml Primocin (InvivoGen) and titrated in 96-well plates. The TCID50/ml was calculated according to the Reed and Muench method (40).
Plasmid Construction
The zebrafish interferon gamma 1-2 (ifng1-2) gene was amplified by PCR (primers presented in Table 1 in Data Sheet S1 in Supplementary Material), and the PCR product was cloned using the pcDNA 3.1/V5-His TOPO TA Expression Kit (Invitrogen). One Shot TOP10F′ competent cells (Invitrogen) were transformed to generate the plasmid construct (pcDNA 3.1-ifng1-2). Plasmid purifications were conducted using the PureLink HiPure Plasmid Midiprep Kit (Invitrogen) following the manufacturer’s instructions.
Reagents
Lipopolysaccharide from Escherichia coli O111:B4, poly(I:C), a TDO-inhibitor [C80C91 or 6-fluoro-3-((1E)-2-(3-pyridinyl)ethenyl)-1H-indole], and an IDO-inhibitor (1-MT or 1-methyl-d-tryptophan) were purchased from Sigma-Aldrich (reference numbers L2630, P1530, SML0287, and 452483, respectively). β-glucans extracted from Saccharomyces cerevisiae were obtained from Biotec-Mackzymal. The zebrafish IFN-gamma 1-2 recombinant protein was acquired from Kingfisher Biotech (reference number RP1045Z).
Stimulation With PAMPs and Challenge With SVCV
Four groups composed of 92 adult zebrafish each were intraperitoneally (i.p.) injected with 20 µl of one of the following treatments: β-glucans (1 mg/ml), LPS (0.75 mg/ml), poly(I:C) (1 mg/ml), and control treatment with phosphate-buffered saline (PBS). After 35 days, half of the individuals were i.p. infected with 20 µl of a SVCV suspension (3 × 102 TCID50/ml), and the remaining fish, which served as uninfected controls, were inoculated with viral medium (MEM + 2% FBS + Primocin). For microarray hybridization, a total of 16 fish from each treatment (β-glucans-control, LPS-control, poly(I:C)-control, β-glucans-SVCV, LPS-SVCV and poly(I:C)-SVCV) were sacrificed at 24 h postinfection, and the kidney was removed, yielding four pooled biological replicates (four fish/replicate). Total RNA was extracted as described below, and RNA quality was assessed with the Agilent 2100 Bioanalyzer (RIN ≥ 7).
To determine the long-term effects of the different PAMPs on mortality after challenge with SVCV, the remaining 30 fish from each group were divided into 3 replicates (10 fish per replicate), and mortality was recorded for a period of 3 weeks.
To analyze potential short-term priming with β-glucans, a total of 48 zebrafish were i.p. inoculated with 20 µl of β-glucans (1 mg/ml) or PBS. After a rest period of 7 days, half of the fish were i.p. infected with 20 µl of an SVCV suspension (1.5 × 102 TCID50/ml), and the remaining individuals were inoculated with viral medium (control fish). The fish from each group were distributed in three biological replicates (eight individuals/replicate). Mortality was recorded for a period of 3 weeks. In parallel, the same experiment was conducted to analyze gene expression by qPCR at 24 h postinfection in kidney samples (five individual fish by treatment).
RNA Isolation and cDNA Transcription
Total RNA was isolated using the Maxwell 16 LEV Simply RNA Tissue Kit (Promega) according to the manufacturer’s instructions. cDNA synthesis was conducted with an NZY First-Strand cDNA Synthesis kit (NZYTech) using 0.3 µg of total RNA.
Microarray Analysis
The 4 × 44K Zebrafish Gene Expression Microarray (V3, AMADID 026437) from Agilent Technologies (Madrid, Spain) was used to analyze gene expression in the different samples (Glucans, LPS, poly(I:C), Glucans-SVCV, LPS-SVCV, and poly(I:C)-SVCV).
The labeling of 1 µg of RNA and hybridizations were carried out at the Universidad Autónoma de Barcelona microarray facility, in compliance with the Minimum Information about a Microarray Experiment (MIAME) standards (41). The signal was captured, processed, and segmented using an Agilent G2565B scanner (Agilent Technologies, Madrid, Spain) with Agilent Feature Extraction Software (v9.5) protocol GE1-v5_95 using an extended dynamic range and preprocessing by Agilent Feature Extraction v9.5.5.1.
The results for the fluorescence intensity data and quality annotations were imported into GeneSpring GX version 14.9 (Agilent Technologies). All of the control features were excluded from the subsequent analyses. After grouping the biological replicates (four replicates per treatment), entities with an expression level between the 20th and 95th percentiles in the raw data were retained and used in the subsequent analyses. The gene-level experiment was carried out by normalizing the data via a percentile shift at the 75th percentile and using the median of all samples as the baseline transformation. Differentially expressed genes were identified through volcano plot filtering. An unpaired t-test was conducted without correction, and data were considered significant at p ≤ 0.01. The fold-change cut-off was set at 1.5. Raw and normalized data were deposited in the NCBI’s Gene Expression Omnibus (GEO, http://www.ncbi.nlm.nih.gov/geo/) and are available under the accession number GSE113241. The heatmaps included in this work were also constructed with GeneSpring GX version 14.9.
Microarray Validation and qPCR
Microarray results were validated through qPCR by analyzing the expression of nine different genes in the same samples used to hybridize the microarray, specifically, in the samples Glucans-SVCV and PBS-SVCV. Those genes were nk-lysin a (nkla), nk-lysin d (nkld), interferon-gamma 1-2 (ifng1-2), tryptophan 2,3-dioxygenase a (tdo2a), cyclin y (ccny), membrane-bound O-acyltransferase domain containing 4 (mboat4), pyruvate dehydrogenase kinase isozyme 3a (pdk3a), nuclear receptor subfamily 1 group D member 2a (nr1d2a), and adrenomedullin 2a (adm2a). The correlation between microarray results and qPCR fold-change values was analyzed using the Pearson correlation coefficient. Specific qPCR primers were designed using the Primer3 software (42), and their amplification efficiency was calculated using seven serial twofold dilutions of cDNA with the threshold cycle (CT) slope method (43). Primer sequences are listed in Table 1 in Data Sheet S1 in Supplementary Material. Individual qPCR reactions were carried out in a 25 µl reaction volume using 12.5 µl of SYBR GREEN PCR Master Mix (Applied Biosystems), 10.5 µl of ultrapure water (Sigma-Aldrich), 0.5 µl of each specific primer (10 µM), and 1 µl of fivefold diluted cDNA template in MicroAmp optical 96-well reaction plates (Applied Biosystems). All reactions were performed using technical triplicates in a 7300 Real-Time PCR System thermocycler (Applied Biosystems), with an initial denaturation (95°C, 10 min) followed by 40 cycles of a denaturation step (95°C, 15 s) and one hybridization-elongation step (60°C, 1 min). The relative expression levels of the genes were normalized using 18S ribosomal RNA (18 s) as a reference gene following the Pfaffl method (43).
Panther and Blast2GO Transcriptome Analysis
Lists of genes that were significantly up- and downregulated relative to the corresponding control were imported into the Protein ANalysis THrough Evolutionary Relationships (PANTHER) Classification System Version 13.0 (44) to classify the modulated genes into Gene Ontology (GO)-Slim Biological Processes.
Enrichment analysis was carried out using the Blast2GO software (45, 46) to detect the major GO terms that were overrepresented in relation to the entire microarray chip (p-values ≤0.01 for biological process and ≤0.005 for molecular function).
Overexpression of ifng1-2 in Zebrafish Larvae
The expression plasmid pcDNA3.1-ifng1-2 and the corresponding control plasmid (pcDNA3.1) were microinjected into one-cell stage zebrafish embryos using a glass microneedle incorporated into the Narishige MN-151 micromanipulator and the Narishige IM-30 microinjector. Wild-type zebrafish embryos were microinjected with 200 pg/egg (final volume of 2 nl, diluted in PBS) of the recombinant plasmid or the empty plasmid (60 embryos per treatment). Three days after plasmid injection [3 days postfertilization (dpf)], half of the larvae from each treatment were infected through microinjection into the duct of Cuvier (47) with 2 nl of an SVCV suspension (1.5 × 105 TCID50/ml) diluted in PBS and 0.1% phenol red. The remaining larvae were microinjected with the same volume of PBS + 0.1% phenol red (uninfected controls). The infections were carried out at 23°C. Larvae from each group (pcDNA3.1-Control, pcDNA3.1-ifng1-2-Control, pcDNA3.1-SVCV, and pcDNA3.1-ifng1-2–SVCV) were distributed in three biological replicates (10 larvae/replicate) into 6-well plates. Mortality was assessed during the next 5 days postinfection (dpi). This experiment was replicated three times. Samples were also collected to assess by qPCR the correct replication of the expression plasmid pcDNA3.1-ifng1-2 in non-infected larvae (3 biological replicates, 10 larvae/replicate).
To analyze the effect of ifng1-2 overexpression in macrophages and neutrophils, double-transgenic Tg(mpeg:mCherry/mpx:GFP) embryos were also microinjected with 200 pg/egg of pcDNA3.1-ifng1-2 and pcDNA3. At 3 dpf, half of the larvae from each treatment were challenged with SVCV (103 TCID50/ml). The remaining larvae were microinjected with the same volume of PBS + 0.1% phenol red (uninfected controls). The cell morphology was analyzed in 4 dpf larvae using a TSC SPE confocal microscope (Leica), and images were processed with the LAS AF software (Leica).
Effect of Ifng1-2 and TDO and IDO Inhibitors on Mortality After an SVCV Challenge in Adult Zebrafish
Six groups of 24 adult zebrafish were i.p. inoculated with a 10 µl volume of one of the following treatments: recombinant zebrafish Ifng1-2 (0.003 µg/µl) co-administered with SVCV, a TDO inhibitor (680C91; 1.5 µg/µl) in combination with an IDO inhibitor (1-MT; 1.5 µg/µl) and SVCV, SVCV alone, Ifng1-2 without SVCV, a TDO-inhibitor and an IDO-inhibitor without SVCV, or viral medium alone (MEM + 2% FBS + Primocin + 0.08% DMSO). The SVCV concentration was 3 × 102 TCID50/ml, and all treatments were diluted in viral medium. The fish from each group were distributed in three biological replicates (eight individuals/replicate). Mortality was recorded during the next 3 weeks.
Statistical Analyses
For the survival experiments, Kaplan–Meier survival curves were constructed and analyzed with the log-rank (Mantel–Cox) test. The Pearson correlation coefficient was calculated using the SPSS software version 23.0.
Results
Effect of Different PAMPs on the Zebrafish Transcriptome After 35 Days
The number and intensity of modulated genes in the zebrafish kidney 35 days after the administration of three different PAMPs are shown as stacked column charts in Figure 1A. Additionally, the genes that were significantly affected by the long-term administration of β-glucans, poly(I:C), and LPS and their corresponding fold-changes are represented in Data Sheet S2 in Supplementary Material.
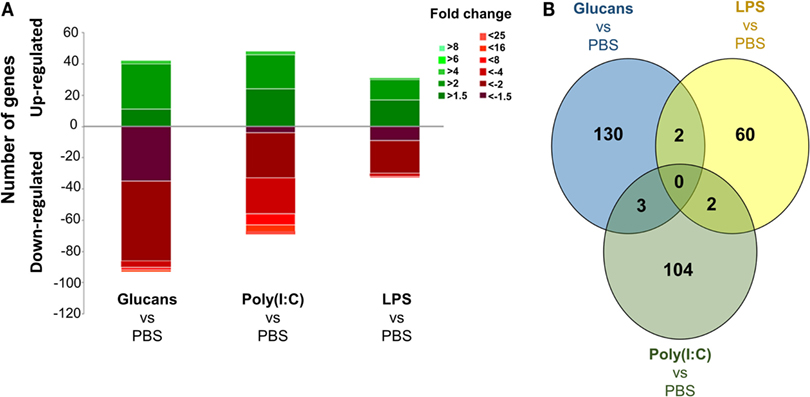
Figure 1. Analysis of gene modulation in the zebrafish kidney at 35 days poststimulation with the three pathogen-associated molecular patterns (PAMPs) compared to individuals inoculated with phosphate-buffered saline. (A) Stacked column chart reflecting the distribution of the DEGs. Statistically significant differentially expressed genes are subdivided according to intensity (fold change) and sense (up- and downregulation). (B) Venn diagram showing the number of shared and exclusive modulated genes in the three treated groups. Even after 35 days, the response was found to be very exclusive depending on the PAMP.
The number of differentially expressed genes (DEGs) in the PAMP-treated fish compared to the control (PBS-injected) fish indicates that the effect of the immunostimulation endured over time. The response to LPS administration was the most equilibrated, with a lower number of DEGs, 64 genes in total, and similar quantities of up- and downregulated genes, 31 and 33 genes, respectively. Stimulation with β-glucans and poly(I:C) induced similar responses in terms of the number of modulated genes, with 135 and 109 DEGs, respectively, and a predominance of downregulated genes was observed (Figure 1A). Nevertheless, a Venn diagram comparing the number of shared and exclusive DEGs among the three treatments revealed strikingly different gene modulation patterns (Figure 1B). No DEGs were common to the three PAMPs. In addition, only two genes were shared by glucans and LPS or LPS and poly(I:C), and three DEGs were common to glucans and poly(I:C). These results reflect an exclusive reprograming of the transcriptome for each PAMP.
Differential Responses to SVCV Challenge After Previous Administration of Different PAMPs and Effects on Survival
When the transcriptome response to SVCV was analyzed at 24 h postinfection in fish that were previously stimulated with PAMPs or not (Data Sheet S3 in Supplementary Material), the number of DEGs relative to PBS-inoculated zebrafish was found to be higher when PAMP stimulation and viral infection were combined than when the fish received PAMP stimulation or SVCV infection alone (Figure 2A). This multiplied response was drastic in the case of the individuals pretreated with β-glucans and then infected with SVCV after 35 days. In these individuals (Glucans-SVCV), the number of DEGs relative to the PBS group was 2,486, whereas only 135 and 113 genes were modulated in the Glucans and PBS-SVCV groups, respectively. This difference implies that the response to the virus was 21 times higher in the Glucans-SVCV group than in the PBS-SVCV group and 18 times higher in the Glucan-SVCV group than in the Glucans group. The additive effect of the two stimuli was much lower in the case of poly(I:C) [946 DEGs in poly(I:C)-SVCV] and especially LPS (250 DEGs in LPS-SVCV) (Figure 2A).
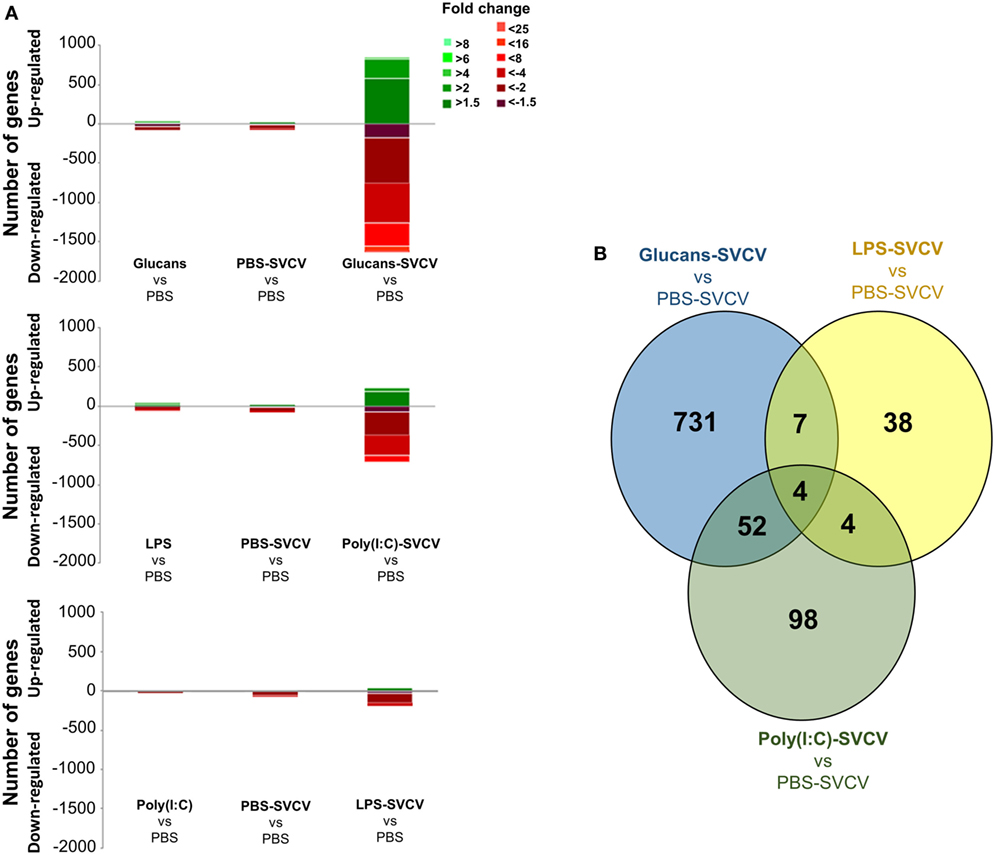
Figure 2. Analysis of gene modulation in the zebrafish kidney at 24 h postinfection with Spring Viremia Carp Virus (SVCV) in individuals that were previously stimulated with pathogen-associated molecular patterns (PAMPs). (A) Stacked column chart reflecting the distribution of the DEGs compared to individuals inoculated with phosphate-buffered saline (PBS). Statistically significant differentially expressed genes are subdivided according to intensity (fold change) and sense (up- and downregulation). (B) Venn diagram showing the number of shared and exclusive modulated genes after SVCV challenge in the three treated groups compared to PBS-SVCV fish. As observed in the absence of infection, the response was very exclusive depending on the PAMP.
When the transcriptome response to SVCV challenge in fish that were previously treated with different stimuli was compared with the response to infection alone (PBS-SVCV group) (Data Sheet S4 in Supplementary Material) through a Venn diagram, the number of exclusive DEGs was also high, representing 92.1% in the Glucans-SVCV group, 62% in the poly(I:C)-SVCV group, and 71.7% in the LPS-SVCV group (Figure 2B).
A dendrogram and a heatmap of the overall microarray data revealed two main clusters of similarity (Figure 3A). In the first cluster, zebrafish groups that were inoculated with PAMPs or PBS but uninfected were placed together. Interestingly, in this cluster, the PBS-SVCV group was also included, which was closely related to the poly(I:C)-injected fish. The expression pattern of the Glucans group was the most different within this cluster and formed an independent branch. Therefore, the treatment with β-glucans induced a stronger long-lasting effect on the transcriptome. In the other cluster, the groups that were stimulated with the three PAMPs and then infected with SVCV were included, with the Glucans-SVCV group being the most different.
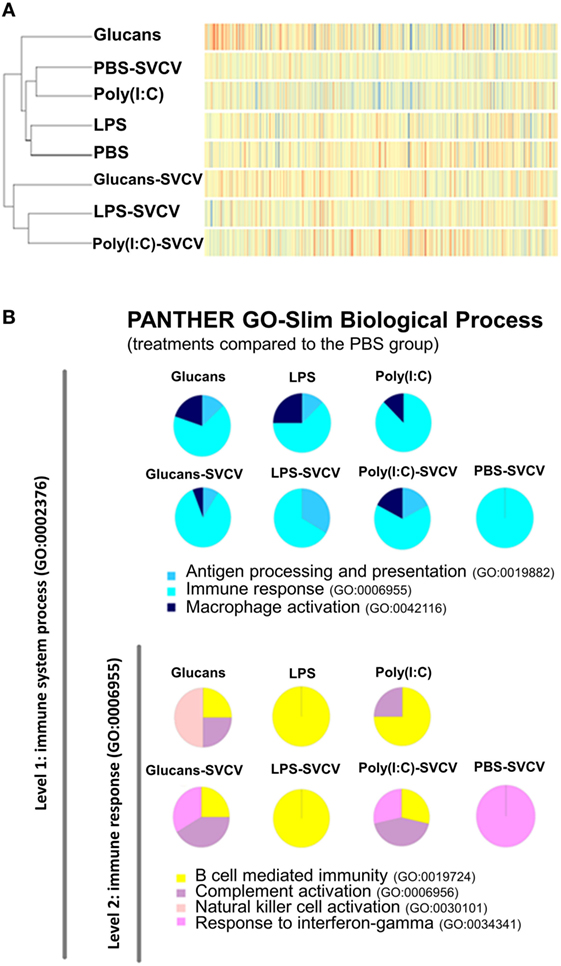
Figure 3. Similarity analysis of the transcriptome response at 35 days post-immunostimulation in the presence or absence of Spring Viremia Carp Virus (SVCV) infection. (A) Dendrogram and heatmap representing the overall microarray results. The samples were divided into two clusters, one containing the pathogen-associated molecular patterns (PAMP)-stimulated groups and the individuals infected in the absence of pre-stimulation, and the other including the groups that were pre-stimulated with PAMPs and then infected with SVCV. In both clusters, the β-glucans formed a separate branch, indicating that this stimulus induced the most differential response. (B) Functional classification of DEGs in the zebrafish kidney between the different treatments and the phosphate-buffered saline-treated group according to Slim Biological Process Gene Ontology Terms. The level 1 category “Immune System Process” was selected, and within that category, the level 2 category “Immune Response.” After SVCV challenge, the gene categorization was conditioned by previous stimulation with a PAMP.
The DEGs in all treatment groups, infected and uninfected, relative to the PBS uninfected group, were categorized according to their biological processes using the PANTHER software. In the “Immune response” category, the three PAMP-treated groups presented genes in “B cell mediated immunity”; Glucans and poly(I:C) also presented genes in “complement activation,” whereas only Glucans presented genes belonging to the “natural killer cell activation” category (Figure 3B). After infection in the absence of previous immunostimulation (PBS-SVCV), the response seemed to be dominated by “response to interferon-gamma,” but individuals that were previously treated with PAMPs maintained certain mechanisms triggered by the PAMP even after SVCV infection (Figure 3B), presenting a completely different response than the non-immunostimulated group. This finding could indicate a longer conditioning effect after the first stimulus (PAMP) than after the second stimulus (SVCV).
Despite these amplified responses to the virus in individuals that were previously stimulated with PAMPs, a reduction of mortality was not observed (Figure 4A). In contrast, although the differences were not found to be statistically significant, higher mortality after SVCV infection was observed in the three groups stimulated with PAMPs.
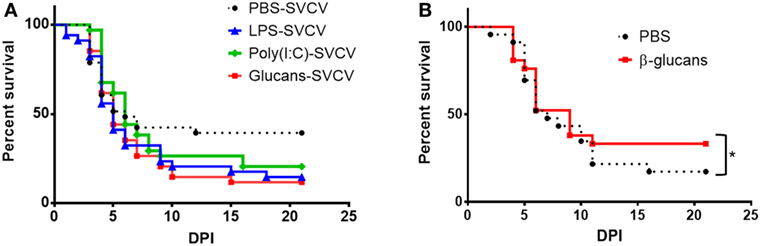
Figure 4. Effect of pathogen-associated molecular patterns (PAMPs) on the survival of adult zebrafish after a Spring Viremia Carp Virus (SVCV) challenge. (A) Kaplan–Meier survival curves after an SVCV i.p. challenge. Fish were stimulated with PAMPs or inoculated with phosphate-buffered saline (PBS), and 35 days after a single-dose administration, they were i.p. challenged with SVCV. No significant differences were observed among the different groups, although the PAMP-treated zebrafish showed a lower survival rate. (B) Kaplan–Meier survival curves after SVCV i.p. challenge. Fish were previously inoculated with β-glucans or PBS and i.p. challenged with SVCV 7 days after a single-dose administration. A slight but significant increase in the survival rate was observed in fish that were previously treated with β-glucans. Significant differences are represented by asterisks (*p < 0.05).
The Modulated Transcriptome of β-Glucan-Treated Fish at 35 Days Poststimulation Suggests an Immunosupressive State
Due to the larger/stronger response of the individuals treated with β-glucans, we focused our attention on this immunostimulant. Because the SVCV infections conducted in this work were performed after a long resting period (35 days after stimulation with the different PAMPs), we wanted to determine if β-glucans were able to provide protection against SVCV at 7 days poststimulation. In this case, β-glucan administration slightly but significantly increased the survival rate from 17.4 to 33.3% (Figure 4B), indicating that the effect of this stimulus after 7 days benefits the resolution of the disease.
To determine why β-glucans were not able to protect after a long rest period but had a profound effect on gene expression, we analyzed in more detail the modulation of the transcriptome in treated fish 35 days after i.p. injection (Data Sheet S5 in Supplementary Material). When the complete set of DEGs was taken into account, interesting clues about the effects of long-term stimulation with β-glucans were revealed (Table 2 in Data Sheet S1 in Supplementary Material). In this case, it is interesting to highlight the enriched GO terms related to steroid hormones in the comparison Glucans-SVCV vs. Glucans, such as “intracellular steroid hormone receptor signaling pathway” and “response to epinephrine,” which do not appear in the comparison PBS-SVCV vs. PBS. These terms are related to the activation of stress response pathways. Indeed, “response to epinephrine” is already represented in the comparison Glucans vs. PBS. It is also worth noting that terms related to the antiviral responses that were observed after SVCV infection in the comparison PBS-SVCV vs. PBS (such as chemotaxis and inflammation) were not observed after infection in the comparison Glucans-SVCV vs. Glucans (Table 2 in Data Sheet S1 in Supplementary Material).
If we focus on the GO terms associated with the top 25 most up- and downmodulated genes (Table 1) in the β-glucan-treated fish after infection relative to non-stimulated but infected fish (Table 1), it is interesting to note that the GO terms enriched in the upregulated genes were related to lipid transport (“medium-chain fatty acid transport”), the catabolism of amino acids, especially tryptophan (“tryptophan catabolic process to acetyl-CoA,” “tryptophan catabolic process to kynurenine,” “tryptophan 2,3-dioxigenase activity,” “tyrosine metabolic process,” and “l-phenylalanine catabolic process”), and interferon-gamma activity (“interferon-gamma receptor binding”). Interestingly, an immunosuppressive profile seems to be present in individuals that previously received β-glucans, represented by GO categories enriched in the most downmodulated genes that were directly related to pathogen recognition (“detection of diacyl bacterial lipopeptide,” “toll-like receptor 6 signaling pathway,” and “MyD88-dependent toll-like receptor signaling pathway”) and inflammation (“positive regulation of interleukin-6 biosynthetic process,” “T-helper 1 type immune response,” and “regulation of cytokine secretion”) (Table 2).
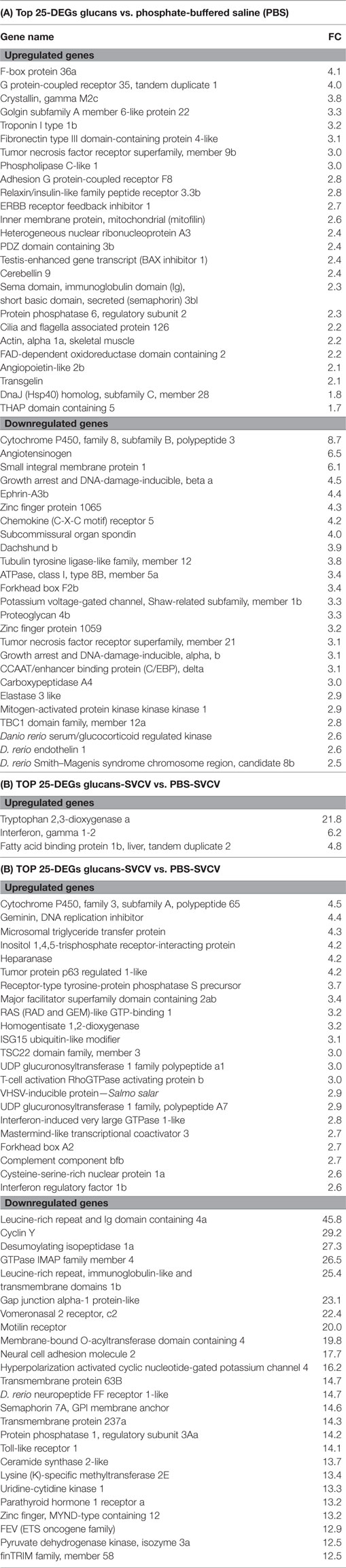
Table 1. Top 25 most up- and downregulated genes in β-glucan-treated zebrafish compared to unstimulated fish in the absence (A) or presence (B) of Spring Viremia Carp Virus (SVCV) infection.
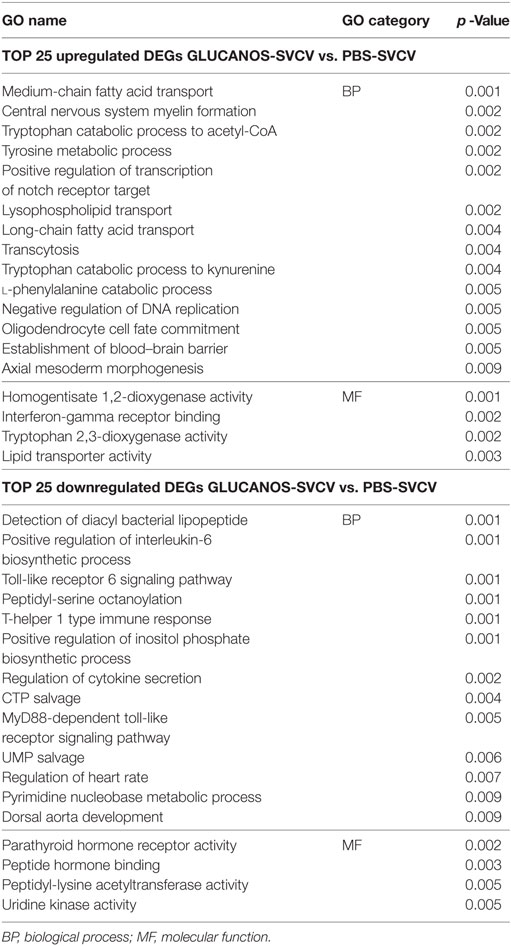
Table 2. Gene ontology (GO) enrichment analysis of the top 25 most modulated genes in fish that previously received β-glucans and were then infected [Glucans-Spring Viremia Carp Virus (SVCV)] compared to untreated and infected fish (PBS-SVCV).
β-Glucans Modify Lipid Metabolism by Inhibiting the Synthesis of Fatty Acids and Cholesterol Before and After Viral Infection
Lipid transport seemed to characterize the response to SVCV in individuals that were previously treated with β-glucans. This finding was consistent with the modulation of genes that were overexpressed, such as fatty acid-binding protein 1b, liver, tandem duplicate 2 (fabp1b.2), microsomal triglyceride transfer protein (mtp), and major facilitator superfamily domain containing 2ab (mfsd2ab) (Table 1). It is known that β-glucans induce changes in lipid metabolism, and these metabolic changes could condition the immune status of the host. To determine the differential expression profiles of genes encoding key proteins in different lipid metabolic pathways [fatty acid oxidation (FAO), ketolysis, lipogenesis, and cholesterol biosynthesis], specific heatmaps for each pathway were constructed (Figure 5).
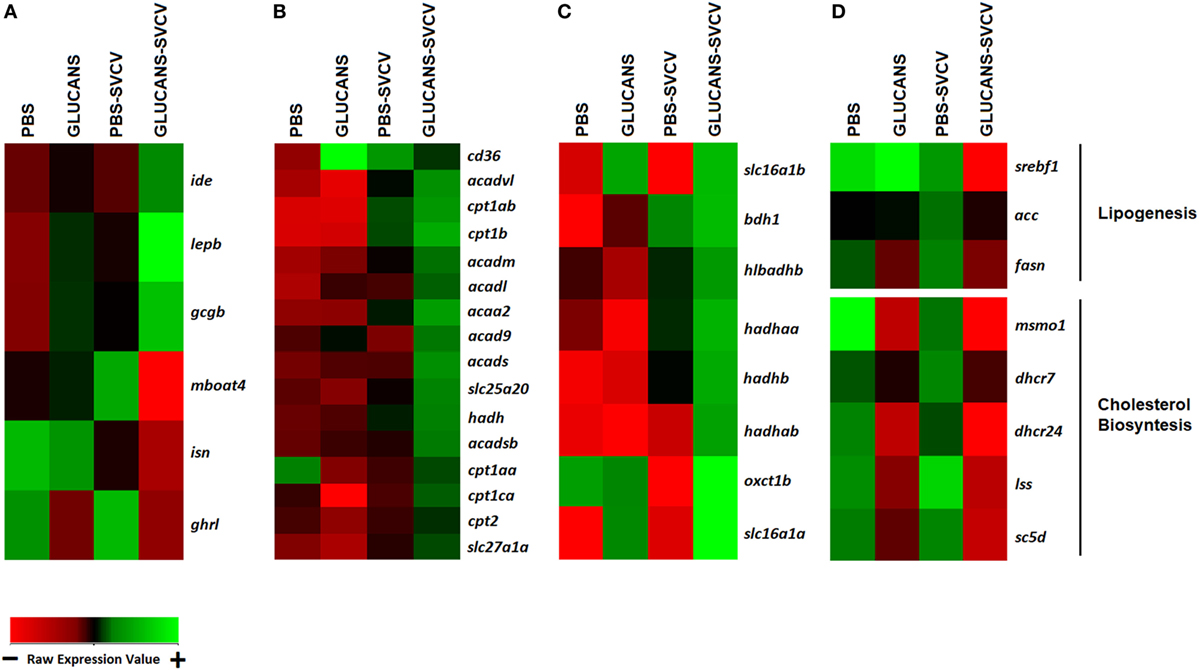
Figure 5. Heatmaps representing different components related to lipid metabolism and transport. (A) Hormone genes involved in lipid metabolism. (B) Genes encoding key components of fatty acid oxidation. (C) Ketolysis. (D) Lipogenesis and cholesterol biosynthesis. A color gradient scheme representing gene modulation (red: lower expression; green: higher expression) is shown on the bottom.
Hormone genes that could underlie the modulation of these pathways are shown in Figure 5A. The genes leptin (lepb), insulin-degrading enzyme (ide), and glucagon (gcgb), whose expression is potentiated by double stimulation with β-glucans and SVCV, are inhibitors of the synthesis of lipids and favor lipolysis and ketolysis (48, 49). On the other hand, an opposite effect was observed for insulin (isn), ghrelin (ghrl) and membrane-bound o-acyltransferase domain containing 4 (mboat4), which endode proteins that activate lipogenesis (50, 51). In accordance with these observations, the heatmaps associated with lipid metabolism showed that Glucans-SVCV zebrafish present a clear induction of FAO (Figure 5B) and ketolysis (Figure 5C) and a significant inhibition of fatty acid syntesis (Figure 5D), which is reflected in the downregulation of key components of lipid synthesis, such as sterol regulatory element binding transcription factor 1 (srebf1), fatty acid synthase (fasn), and acetyl-coA carboxylase (acc), and genes of the cholesterol synthesis pathway (Figure 5D). The effect on lipid metabolism was also observed in fish that were stimulated with β-glucans alone, in which the inhibition of cholesterol synthesis, the downregulation of ghrl, and the upregulation of the fatty acid translocase scavenger receptor class B, member 3 (cd36), which is essential for fatty acid import into cells and efficient oxidation, were observed.
Effect of Ifng1-2 on Macrophage Activation and Survival After an SVCV Challenge
The transcriptome of infected fish that were previously treated with β-glucans was enriched in the immune term “interferon-gamma receptor binding,” as a result of the high expression of the ifng1-2 gene, among others (Table 1). IFN-γ is an important activator of macrophages in mammals, with immunostimulatory and immunomodulatory properties. Nevertheless, in this case, Ifng1-2 seeems to be associated to an immunosuppressive state. For that reason, we wondered if this molecule has a pernicious effect in zebrafish during an infection with SVCV or if its association with the immunosuppression depends on other factors.
An expression plasmid encoding ifng1-2 (pcDNA3.1-ifng1-2) was constructed, and its correct replication was confirmed in 3 dpf larvae (Figure 1 in Data Sheet S1 in Supplementary Material). The plasmid pcDNA3.1-ifng1-2 and the corresponding empty plasmid (pcDNA3.1) were microinjected into double-transgenic Tg(mpeg:mCherry/mpx:GFP) zebrafish embryos alone or in combination with SVCV. When we compared the morphology of macrophages and neutrophils in 4 dpf larvae, it was evident that larvae overexpressing ifng1-2 presented a different morphology than the controls for both cell types (Figure 6A). Both neutrophils and macrophages changed from a spherical morphology to a dendritic morphology, which was especially evident in infected and pcDNA3.1-ifng1-2-treated fish; in the case of macrophages, these changes probably reflect the activation of these cells.
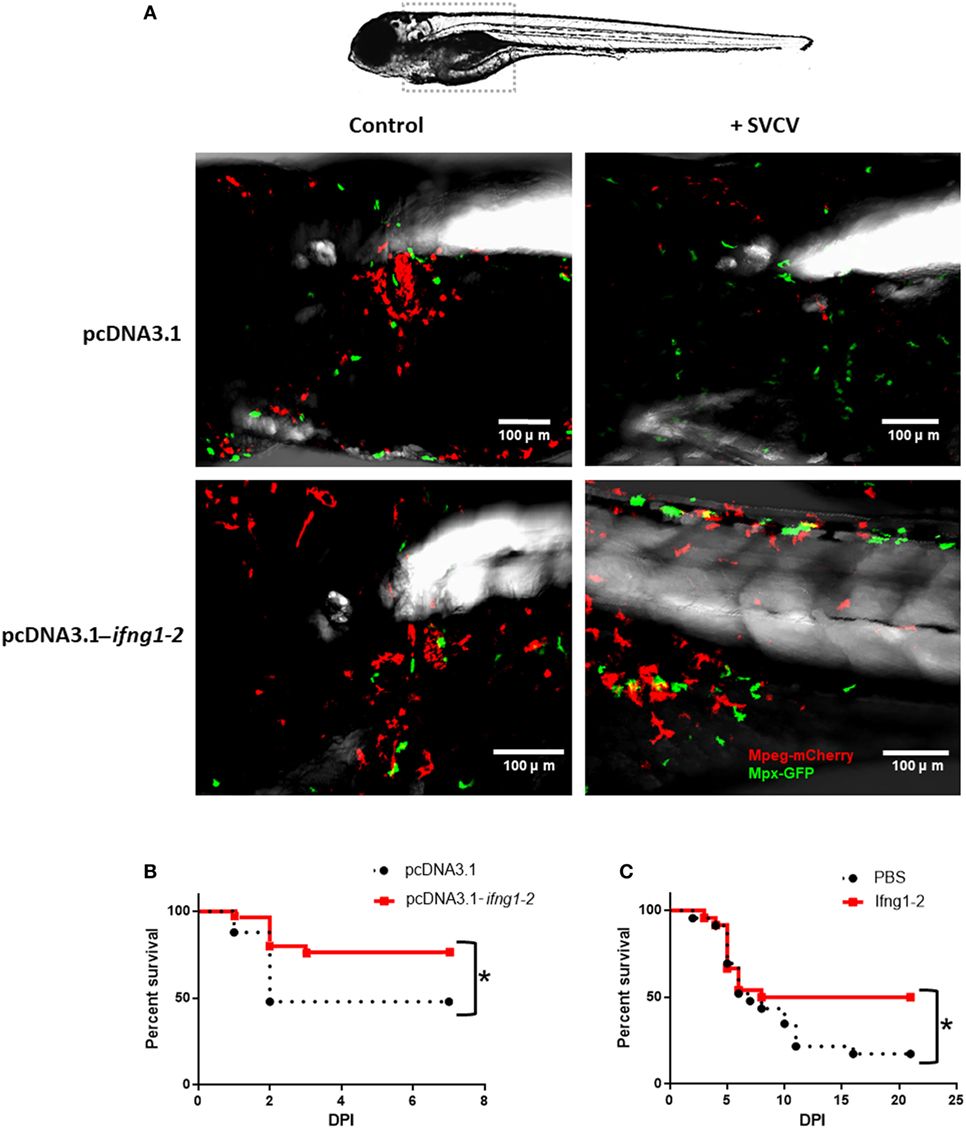
Figure 6. Effect of Ifng1-2 on the activation of immune cells and on survival against Spring Viremia Carp Virus (SVCV). (A) Zebrafish larvae overexpressing ifng1-2 show the activation of macrophages and neutrophils, with an evident change in morphology from a spherical morphology to a dendritic morphology. (B) Kaplan–Meier survival curves after SVCV challenge in zebrafish larvae overexpressing or not overexpressing ifng1-2 (C) Kaplan–Meier survival curves after SVCV challenge in adult zebrafish co-inoculated or not co-inoculated with the Ifng1-2 recombinant protein. Significant differences are represented by asterisks (*p < 0.05).
The overexpression of ifng1-2 in wild-type zebrafish larvae also revealed the ability to significantly increase survival after an SVCV challenge (Figure 6B). The survival of the empty plasmid-treated fish was 48%, whereas this rate increased to 76.7% in individuals that were previously inoculated with the expression plasmid pcDNA3.1-ifng1-2. This protective capability against SVCV was also tested in adult zebrafish using the recombinant protein (Figure 6C). In this case, the untreated fish exhibited a survival rate of 17.4%, but individuals that were inoculated with Ifng1-2 significantly increased their survival to 50%.
The Alteration of the Tryptophan–Kynurenine Pathway Influences the Survival Rate After SVCV Infection
As observed in the GO enrichment analysis, the kynurenine pathway of tryptophan catabolism was highly altered in the Glucan-SVCV individuals compared to the PBS-SVCV fish (Figure 7A). Interestingly, this pathway has a close relation with the activity of IFN-γ. A heatmap including the genes that encode some of the main proteins involved in tryptophan catabolism, such as ifng1-2, tryptophan 2,3-dioxygenase a (tdo2a), tryptophan hydroxylase 2 (tph2), and kynurenine aminotransferase 2 (kyat2), revealed that previous stimulation with β-glucans determines the response of these genes to an SVCV challenge (Figure 7B). The genes ifng1-2 and tdo2a were two of the most overexpressed genes in Glucans-SVCV vs. PBS-SVCV fish (Table 1). However, after short-term glucan stimulation, only ifng1-2 was slightly overexpressed in individuals treated with glucans and then infected and tdo2a was not modulated (Figure 2 in Data Sheet S1 in Supplementary Material). Although it was not included in this heatmap, it is also interesting to highlight the second most upregulated gene in the Glucans group relative to the PBS group, the G protein-coupled receptor 35 (gpr35), because intermediates of the kynurenine pathway are endogenous agonists of this receptor.
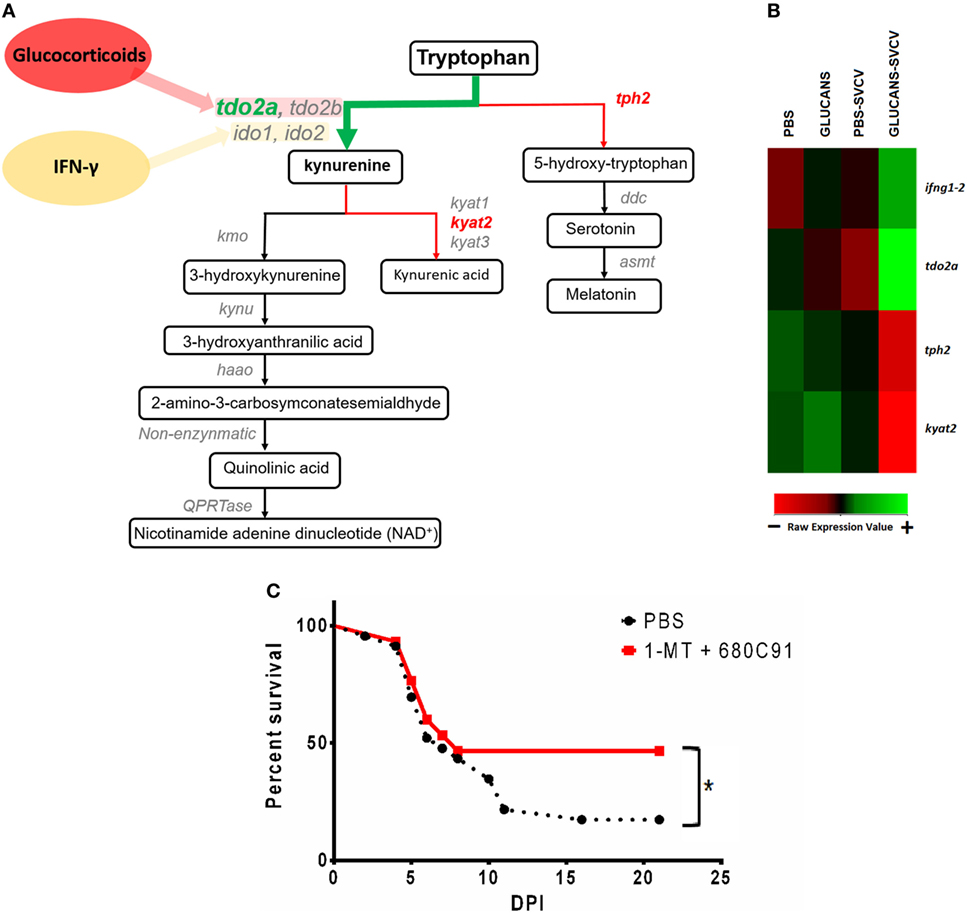
Figure 7. Analysis of the kynurenine pathway of tryptophan catabolism in zebrafish inoculated with β-glucans. (A) Schematic representation of the kynurenine pathway and the DEGs in the comparison Glucans-Spring Viremia Carp Virus (SVCV) vs. Glucans-PBS (tdo2a, kyat2) and the comparison Glucans-SVCV vs. PBS (tph2). (B) Heatmap representing some of the most affected genes after β-glucan treatment. Whereas ifng1-2 and tdo2a are highly expressed in the Glucans-SVCV group, tph2 and kyat2 have lower expression levels in these fish. A color gradient scheme representing gene modulation (red: lower expression; green: higher expression) is shown on the bottom. (C) Kaplan–Meier survival curves representing the effect of a TDO-inhibitor (C80C91) and an IDO-inhibitor (1-MT) during a challenge with SVCV in adult zebrafish. A significant increase in the survival rate was observed in individuals inoculated with the inhibitors. Significant differences are represented by asterisks (*p < 0.05).
To investigate the importance of this pathway in mortality after SVCV, a TDO-inhibitor (C80C91) and an IDO-inhibitor (1-MT) were inoculated together with SVCV. The blockage of the kynurenine pathway with these inhibitors significantly increased the survival rate after infection (Figure 7C), with values that changed from 17.4% in untreated fish to 46.7% in individuals that received C80C91 + 1-MT. Therefore, the blockage of the kynurenine pathway significantly reduces the mortality caused by SVCV.
Validation of the Microarray Data by qPCR
Correlation of the fold-change values for the comparison Glucans-SVCV vs. PBS-SVCV between microarray and qPCR showed a Pearson correlation coefficient (r) of 0.943 (p < 0.001). The expression data obtained for the selected genes are plotted in Figure 3 in Data Sheet S1 in Supplementary Material.
Discussion
Typically, the use of immunostimulants, especially β-glucans, in aquaculture has been conducted with the objective of increasing the non-specific defense mechanisms of the cultured fish (2). Nevertheless, several factors, such as the timing and method of administration, must be carefully considered (2). An inappropriate treatment protocol can transform the immunostimulants into a double-edged sword for the teleost immune system, resulting in a stressful and immunosuppressive state. The long-term effects of immunostimulants, even after a single administration, can produce distress situation, with a negative impact on immune competence. Chronic stress involves metabolic changes that allow the organism to face the demand for resources that is associated with stress. A prolonged immune stimulus consumes energy resources due to the cost required to generate an appropriate immune response. When this demand is prolonged over time, these resources are exhausted, leading to immunosuppression (52).
The trained immunity induced by β-glucans seems to be limited to a period of approximately 20 days. This effect was observed after in vitro stimulation of murine spleen-derived monocytes with this substance (53) and after in vivo stimulation in zebrafish (32). Moreover, a work based on long-term protection against the virus IHNV after an i.p. injection of β-glucans in trout revealed that beyond 36 days poststimulation, a higher mortality rate occurred in pre-immunized individuals (28). The genes encoding proinflammatory cytokines, such as TNF-α, IL-1β, and IL-6, which are usually found to be upregulated during cell training (15, 32, 53) were not differentially modulated in our microarray results. These findings, together with the absence of protection against SVCV, led us to consider that in this work, we are describing long-term mechanisms that could persist beyond the end of training immunity.
In this work, we wanted to analyze the long-term effects of a punctual administration of three different PAMPs [LPS, poly(I:C), and β-glucans] on the immune system of zebrafish and their consequences during a viral infection. At 35 days posttreatment, none of the PAMPs was able to provide protection after infection, and higher mortalities were even observed for the three treatments, especially for β-glucans. Moreover, the transcriptome of fish that were previously treated with PAMPs was found to be highly altered, even after this long period of time, and this effect was more pronounced with β-glucans.
This is not the first time that a negative effect of β-glucans was observed in teleosts. The in vitro administration of β-glucans to turbot (Scophthalmus maximus) and gilthead seabream (Sparus aurata) phagocytes revealed that high doses of this molecule directly induced the respiratory burst and rapidly led to cell exhaustion (52), which may increase disease susceptibility. In vivo studies in large yellow croaker (Larimichthys crocea) showed that individuals fed high doses of β-glucans were less resistant to a challenge with Vibrio harveyi, probably because high doses resulted in immunosuppression or feedback regulation (54). A recent study in rainbow trout (Oncorhynchus mykiss) demonstrated that overdoses of β-glucans can lead to poor immune responses, probably due to the activation of stress response mechanisms (55).
The response triggered by a stressful stimulus is mediated by the release of stress hormones, such as glucocorticoids (GCs), which can increase or suppress certain pathways of the immune response depending on the intensity and duration of the stressor (36). While an acute stress is more closely associated with eustress (“good stress”), which involves short-term challenges that result in immunoenhancement, distress (“bad stress”) is caused by repeated or prolonged stress over time, which can cause immune suppression (56). In our case, in the short term (pre-stimulation with β-glucan and infection after 7 days), eustress could be responsible of the mechanisms that underlie training immunity and the slight protection observed after infection. In the long term (pre-stimulation with β-glucan and infection after 35 days), the distress generated by the high energy cost of prolonged training immunity could explain the lack of protection against SVCV and even the tendency toward an increase in mortality.
Several factors, mainly involved in cell metabolism, could explain the lack of protection against the viral challenge. In mammals, processes such as glycolysis, the pentose phosphate pathway, glutaminolysis, and cholesterol and fatty acid synthesis, are usually upregulated after β-glucan training, and the blockade of some of these pathways (such as glycolysis, glutaminolysis, and cholesterol synthesis) inhibits training immunity (57). The synthesis of lipids in immune cells is associated with cell proliferation and the generation of inflammatory cytokines; however, FAO is more closely associated with tolerance, the suppression of the immune response, memory, and a long cellular lifespan (58). The microarray revealed that genes related to fatty acid and cholesterol synthesis are inhibited in individuals treated with β-glucans, especially after viral infection, and genes related to FAO were overexpressed. In our results, fasn, acc, and srebp1 mRNA levels decreased in immunostimulated fish. However, it is known that mammalian target of rapamycin (mTOR), the key player in training immunity activation (15), promotes the synthesis of fatty acids through the induction of these three genes (59). These results suggest a shift of training immunity to a more immunotolerant profile in our experiments.
Another remarkable aspect of the altered transcriptome of glucan-treated fish was amino acid depletion, which is another tolerance indicator. Among the most DEGs in the Glucans-SVCV group relative to the PBS-SVCV group are the TDO gene tdo2a and homogentisate 1,2-dioxygenase (hgm), which encode enzymes involved in amino acid catabolism. Phenylalanine and tryptophan are essential amino acids that are necessary for mTORC1 activation (60–62). TDO and indoleamine 2,3-dioxygenase (IDO), which catalyze the first step of tryptophan catabolism, are the rate-limiting enzymes of the kynurenine pathway (63). TDO is mainly produced in the liver but is expressed at immune-privileged sites and in some tumors and is associated with tolerance and immune evasion (17). Therefore, because tryptophan is degraded by the action of IDO and TDO in the kynurenine pathway, these enzymes act as inhibitors of mTOR activation. Indeed, the use of inhibitors of IDO activity, such as D-1MT, reversed the inhibitory effects generated by IDO on mTOR activation (64).
The intermediates of the kynurenine pathway, due to their toxic potential, may cause tissue damage and, as a consequence, generate higher stress, which can in turn interfere with the immune response to minimize tissue damage. It was recently observed that feed supplementation with kynurenic acid (KYNA) in rainbow trout had a toxic/stress-inducing effect, and this deleterious effect was related to the dose of KYNA (65). This pathological status was manifested during a subsequent infection with Yersinia ruckeri, with a higher mortality rate in animals that were administered a higher concentration of KYNA (65). On the other hand, kynurenic acid is an endogenous agonist of gpr35 (which was the second most upregulated gene in β-glucan-stimulated zebrafish in the absence of infection), and its activation increases FAO and induces an anti-inflammatory state (66, 67). Additionally, kynurenic acid and kynurenine are endogenous agonists of the aryl hydrocarbon receptor (AhR) (68), which mediates immunosuppressive effects on the immune system (69), leading to inhibition of the promoter activity of IL-6 (70). Consistent with our results, the depletion of tryptophan from the medium impairs the antiviral response in mammals by inducing the formation of regulatory T cells and inhibiting the formation of effector T cells (71). These effects are observed in our transcriptome results, with a downregulation of the “T-helper 1 type immune response” and “positive regulation of interleukin-6 biosynthetic process” in the comparison Glucans-SVCV vs. PBS-SVCV. The regulation of the innate responses by the adaptive immune system is known in mammals (72) and also it has been reported in zebrafish (73).
The overexpression of the ifng1-2 gene after viral infection in fish that previously received β-glucans initially contradicted the impaired response of these individuals. In fact, in our experiments, the overexpression of ifng1-2 in zebrafish induced the activation of immune cells and was able to increase survival after an SVCV challenge. Nevertheless, a previous work reported an unexpected in vivo failure of Ifng1-2 to increase the resistance of zebrafish to bacterial and viral infections (74). IFN-γ is a pleiotropic cytokine, and it has been classically associated with a proinflammatory immune response (75). However, IFN-γ induces the expression of IDO, which catalyzes the same step as TDO in the kynurenine pathway (63). Therefore, the activation of the kynurenine pathway results from the induction of TDO through the action of GCs and from the induction of IDO through the overexpression of IFN-γ. This process not only depletes tryptophan from the medium but also probably interferes with the antiviral response of IFN-γ. The lack of induction of the tdo2a and the slight overexpression of ifng1-2 compared to the infected controls in the short-term immunostimulation experiment would explain the increased survival of the individuals treated with glucans. Moreover, the higher viral resistance that was observed in this work when we applied the inhibitors of TDO and IDO together supports this hypothesis.
The depletion of tryptophan from the medium seems to be harmful for fish dealing with SVCV infection, as has been described for other viruses (76–78). However, it has been reported that toxic intermediates of the kynurenine pathway present bactericidal effects (76). Indeed, i.p. injection of β-glucans in Salmo salar was able to increase survival against Gram-negative bacteria, even after a long resting period (23). Interestingly, tryptophan depletion (by the action of TDO or IDO) and IFN-γ signaling can have opposite effects in the context of infection, depending on the type of pathogen (virus or bacteria) (76, 79).
In summary, long-term immunostimulation with β-glucans does not protect against SVCV infection. This pre-stimulation seems to lead the zebrafish immune system toward a strategy of immunosuppression and tolerization, which results in pernicious effects for responding to the virus. The interplay among GCs, lipid metabolism, the kynurenine pathway, IFN-γ, and mTOR activation seems to be the mechanism behind this response.
Ethics Statement
Fish care and the challenge experiments were conducted according to the guidelines of the CSIC National Committee on Bioethics under approval number ES360570202001/16/FUN01/PAT.05/tipoE/BNG.
Author Contributions
BN and AF conceived and designed the study. MA-R and PP performed the experimental procedures and data analyses. FR-L and LT conducted the microarray hybridizations. MA-R, PP, AF, and BN wrote the manuscript. All authors reviewed the manuscript.
Conflict of Interest Statement
The authors declare that the research was conducted in the absence of any commercial or financial relationships that could be construed as a potential conflict of interest.
Acknowledgments
We want to thank Judit Castro and the aquarium staff for their technical assistance.
Funding
This work was funded by the projects AGL2014-51773-C3 and BIO2017-82851-C3-1-R from the Spanish Ministerio de Economía y Competitividad, Proyecto Intramural Especial, PIE 201230E057 from Agencia Estatal Consejo Superior de Investigaciones Científicas (CSIC), and IN607B 2016/12 from Consellería de Economía, Emprego e Industria (GAIN), Xunta de Galicia. MA-R was the recipient of an FPU fellowship from the Ministerio de Educación (FPU014/05517).
Supplementary Material
The Supplementary Material for this article can be found online at https://www.frontiersin.org/articles/10.3389/fimmu.2018.01575/full#supplementary-material.
References
1. Vallejos-Vidal E, Reyes-López F, Teles M, MacKenzie S. The response of fish to immunostimulant diets. Fish Shellfish Immunol (2016) 56:34–69. doi:10.1016/j.fsi.2016.06.028
2. Anderson DP. Immunostimulants, adjuvants, and vaccine carriers in fish: applications to aquaculture. Annu Rev Fish Dis (1992) 2:281–307. doi:10.1016/0959-8030(92)90067-8
3. Mogensen TH. Pathogen recognition and inflammatory signaling in innate immune defenses. Clin Microbiol Rev (2009) 22:240–73. doi:10.1128/CMR.00046-08
4. Degn SE, Thiel S. Humoral pattern recognition and the complement system. Scand J Immunol (2013) 78:181–93. doi:10.1111/sji.12070
5. Dalmo RA, Bogwald J. Beta-glucans as conductors of immune symphonies. Fish Shellfish Immunol (2008) 25:384–96. doi:10.1016/j.fsi.2008.04.008
6. Sepulcre MP, Alcaraz-Pérez F, López-Muñoz A, Roca FJ, Meseguer J, Cayuela ML, et al. Evolution of lipopolysaccharide (LPS) recognition and signaling: fish TLR4 does not recognize LPS and negatively regulates NF-kappaB activation. J Immunol (2009) 182:1836–45. doi:10.4049/jimmunol.0801755
7. Lee MS, Kim Y-J. Signaling pathways downstream of pattern-recognition receptors and their cross talk. Annu Rev Biochem (2007) 76:447–80. doi:10.1146/annurev.biochem.76.060605.122847
8. Hotz C, Roetzer LC, Huber T, Sailer A, Oberson A, Treinies M, et al. TLR and RLR signaling are reprogrammed in opposite directions after detection of viral infection. J Immunol (2015) 195:4387–95. doi:10.4049/jimmunol.1500079
9. Netea MG, Quintin J, Van Der Meer JWM. Trained immunity: a memory for innate host defense. Cell Host Microbe (2011) 9:355–61. doi:10.1016/j.chom.2011.04.006
10. Netea MG, Joosten LAB, Latz E, Mills KHG, Natoli G, Stunnenberg HG, et al. Trained immunity: a program of innate immune memory in health and disease. Science (2016) 352:aaf1098. doi:10.1126/science.aaf1098
11. Fan H, Cook JA. Molecular mechanisms of endotoxin tolerance. J Endotoxin Res (2004) 10:71–84. doi:10.1177/09680519040100020301
12. Foster SL, Hargreaves DC, Medzhitov R. Gene-specific control of inflammation by TLR-induced chromatin modifications. Nature (2007) 447:972–8. doi:10.1038/nature05836
13. Quintin J, Saeed S, Martens JHA, Giamarellos-Bourboulis EJ, Ifrim DC, Logie C, et al. Candida albicans infection affords protection against reinfection via functional reprogramming of monocytes. Cell Host Microbe (2012) 12:223–32. doi:10.1016/j.chom.2012.06.006
14. Saeed S, Quintin J, Kerstens HHD, Rao NA, Aghajanirefah A, Matarese F, et al. Epigenetic programming of monocyte-to-macrophage differentiation and trained innate immunity. Science (2014) 345:1251086. doi:10.1126/science.1251086
15. Cheng SC, Quintin J, Cramer RA, Shepardson KM, Saeed S, Kumar V, et al. mTOR/HIF1 alpha-mediated aerobic glycolysis as metabolic basis for trained immunity. Science (2014) 345:1250684. doi:10.1126/science.1250684
16. Kelly B, O’Neill LAJ. Metabolic reprogramming in macrophages and dendritic cells in innate immunity. Cell Res (2015) 25:771–84. doi:10.1038/cr.2015.68
17. Wong CC, Qian Y, Yu J. Interplay between epigenetics and metabolism in oncogenesis: mechanisms and therapeutic approaches. Oncogene (2017) 36:3359–74. doi:10.1038/onc.2016.485
18. Arts RJW, Joosten LAB, Netea MG. Immunometabolic circuits in trained immunity. Semin Immunol (2016) 28:425–30. doi:10.1016/j.smim.2016.09.002
19. Petit J, Wiegertjes GF. Long-lived effects of administering β-glucans: indications for trained immunity in fish. Dev Comp Immunol (2016) 64:93–102. doi:10.1016/j.dci.2016.03.003
20. Sattler S, Ghadially H, Hofer E. Evolution of the C-type lectin-like receptor genes of the DECTIN-1 cluster in the NK gene complex. ScientificWorldJournal (2012) 2012:1–11. doi:10.1100/2012/931386
21. Walachowski S, Tabouret G, Fabre M, Foucras G. Molecular analysis of a short-term model of β-glucans-trained immunity highlights the accessory contribution of GM-CSF in priming mouse macrophages response. Front Immunol (2017) 8:1089. doi:10.3389/fimmu.2017.01089
22. Sandvik A, Wang YY, Morton HC, Aasen AO, Wang JE, Johansen FE. Oral and systemic administration of β-glucan protects against lipopolysaccharide-induced shock and organ injury in rats. Clin Exp Immunol (2007) 148:168–77. doi:10.1111/j.1365-2249.2006.03320.x
23. Robertsen B, Rorstad G, Engstad R, Raa J. Enhancement of non-specific disease resistance in Atlantic salmon, Salmo salar L., by a glucan from Saccharomyces cerevisiae cell walls. J Fish Dis (1990) 13:391–400. doi:10.1111/j.1365-2761.1990.tb00798.x
24. Selvaraj V, Sampath K, Sekar V. Administration of yeast glucan enhances survival and some non-specific and specific immune parameters in carp (Cyprinus carpio) infected with Aeromonas hydrophila. Fish Shellfish Immunol (2005) 19:293–306. doi:10.1016/j.fsi.2005.01.001
25. Rodríguez I, Chamorro R, Novoa B, Figueras A. β-Glucan administration enhances disease resistance and some innate immune responses in zebrafish (Danio rerio). Fish Shellfish Immunol (2009) 27:369–73. doi:10.1016/j.fsi.2009.02.007
26. El-Boshy ME, El-Ashram AM, Abdelhamid FM, Gadalla HA. Immunomodulatory effect of dietary Saccharomyces cerevisiae, beta-glucan and laminaran in mercuric chloride treated Nile tilapia (Oreochromis niloticus) and experimentally infected with Aeromonas hydrophila. Fish Shellfish Immunol (2010) 28:802–8. doi:10.1016/j.fsi.2010.01.017
27. Chang C-S, Huang S-L, Chen S, Chen S-N. Innate immune responses and efficacy of using mushroom beta-glucan mixture (MBG) on orange-spotted grouper, Epinephelus coioides, aquaculture. Fish Shellfish Immunol (2013) 35:115–25. doi:10.1016/j.fsi.2013.04.004
28. LaPatra SE, Lauda KA, Jones GR, Shewmaker WS, Bayne CJ. Resistance to IHN virus infection in rainbow trout is increased by glucan while subsequent production of serum neutralizing activity is decreased. Fish Shellfish Immunol (1998) 8:435–46. doi:10.1006/fsim.1998.0151
29. Sealey WM, Barrows FT, Hang A, Johansen KA, Overturf K, LaPatra SE, et al. Evaluation of the ability of barley genotypes containing different amounts of β-glucan to alter growth and disease resistance of rainbow trout Oncorhynchus mykiss. Anim Feed Sci Technol (2008) 141:115–28. doi:10.1016/j.anifeedsci.2007.05.022
30. Kim Y, Ke F, Zhang QY. Effect of β-glucan on activity of antioxidant enzymes and Mx gene expression in virus infected grass carp. Fish Shellfish Immunol (2009) 27:336–40. doi:10.1016/j.fsi.2009.06.006
31. Beaulaurier J, Bickford N, Gregg JL, Grady CA, Gannam AL, Winton JR, et al. Susceptibility of pacific herring to viral hemorrhagic septicemia is influenced by diet. J Aquat Anim Health (2012) 24:43–8. doi:10.1080/08997659.2012.668511
32. M Medina-Gali R, Ortega-Villaizan MDM, Mercado L, Novoa B, Coll J, Perez L. Beta-glucan enhances the response to SVCV infection in zebrafish. Dev Comp Immunol (2018) 84:307–14. doi:10.1016/j.dci.2018.02.019
33. Reynolds JA, Kastello MD, Harrington DG, Crabbs CL, Peters CJ, Jemski JV, et al. Glucan-induced enhancement of host resistance to selected infectious diseases. Infect Immun (1980) 30:51–7.
34. Jung K, Ha Y, Ha S-K, Han DU, Kim D-W, Moon WK, et al. Antiviral effect of Saccharomyces cerevisiae beta-glucan to swine influenza virus by increased production of interferon-gamma and nitric oxide. J Vet Med B Infect Dis Vet Public Health (2004) 51:72–6. doi:10.1111/j.1439-0450.2004.00732.x
35. Muramatsu D, Iwai A, Aoki S, Uchiyama H, Kawata K, Nakayama Y, et al. β-Glucan Derived from Aureobasidium pullulans is effective for the prevention of influenza in mice. PLoS One (2012) 7:e41399. doi:10.1371/journal.pone.0041399
36. Tort L. Stress and immune modulation in fish. Dev Comp Immunol (2011) 35:1366–75. doi:10.1016/j.dci.2011.07.002
37. Nistico G. Communications among central nervous system, neuroendocrine and immune systems: interleukin-2. Prog Neurobiol (1993) 40:463–75. doi:10.1016/0301-0082(93)90018-N
38. Westerfield M. The Zebrafish Book. A Guide for the Laboratory Use of Zebrafish (Danio rerio). 4th ed. Eugene: University of Oregon Press (2000).
39. Nüsslein-Volhard C, Dahm R. Zebrafish: A Practical Approach. New York: Oxford University Press (2002).
40. Reed LJ, Muench H. A simple method of estimating fifty percent endpoints. Am J Epidemiol (1938) 27:493–7. doi:10.1093/oxfordjournals.aje.a118408
41. Brazma A, Hingamp P, Quackenbush J, Sherlock G, Spellman P, Stoeckert C, et al. Minimum information about a microarray experiment (MIAME)-toward standards for microarray data. Nat Genet (2001) 29:365–71. doi:10.1038/ng1201-365
42. Rozen S, Skaletsky H. Primer3 on the WWW for general users and for biologist programmers. In: Misener S, Krawetz SA, editors. Bioinformatics Methods and Protocols. Totowa, NJ: Humana Press (2000). p. 365–86.
43. Pfaffl MW, Hageleit M. Validities of mRNA quantification using recombinant RNA and recombinant DNA external calibration curves in real-time RT-PCR. Biotechnol Lett (2001) 23:275–82. doi:10.1023/A:1005658330108
44. Thomas PD, Campbell MJ, Kejariwal A, Mi H, Karlak B, Daverman R, et al. PANTHER: a library of protein families and subfamilies indexed by function. Genome Res (2003) 13:2129–41. doi:10.1101/gr.772403
45. Conesa A, Gotz S, Garcia-Gomez JM, Terol J, Talon M, Robles M. Blast2GO: a universal tool for annotation, visualization and analysis in functional genomics research. Bioinformatics (2005) 21:3674–6. doi:10.1093/bioinformatics/bti610
46. Gotz S, Garcia-Gomez JM, Terol J, Williams TD, Nagaraj SH, Nueda MJ, et al. High-throughput functional annotation and data mining with the Blast2GO suite. Nucleic Acids Res (2008) 36:3420–35. doi:10.1093/nar/gkn176
47. Benard EL, van der Sar AM, Ellett F, Lieschke GJ, Spaink HP, Meijer AH. Infection of zebrafish embryos with intracellular bacterial pathogens. J Vis Exp (2012) 61:e3781. doi:10.3791/3781
48. Habegger KM, Heppner KM, Geary N, Bartness TJ, DiMarchi R, Tschöp MH. The metabolic actions of glucagon revisited. Nat Rev Endocrinol (2010) 6:689–97. doi:10.1038/nrendo.2010.187
49. Deck CA, Honeycutt JL, Cheung E, Reynolds HM, Borski RJ. Assessing the functional role of leptin in energy homeostasis and the stress response in vertebrates. Front Endocrinol (2017) 8:63. doi:10.3389/fendo.2017.00063
50. Foretz M, Guichard C, Ferre P, Foufelle F. Sterol regulatory element binding protein-1c is a major mediator of insulin action on the hepatic expression of glucokinase and lipogenesis-related genes. Proc Natl Acad Sci U S A (1999) 96:12737–42. doi:10.1073/pnas.96.22.12737
51. Li Z, Xu G, Qin Y, Zhang C, Tang H, Yin Y, et al. Ghrelin promotes hepatic lipogenesis by activation of mTOR-PPARγ signaling pathway. Proc Natl Acad Sci U S A (2014) 111:13163–8. doi:10.1073/pnas.1411571111
52. Castro R, Couso N, Obach A, Lamas J. Effect of different B-glucans on the respiratory burst of turbot (Psetta maxima) and gilthead seabream (Sparus aurata) phagocytes. Fish Shellfish Immunol (1999) 9:529–41. doi:10.1006/fsim.1999.0210
53. Garcia-Valtanen P, Guzman-Genuino RM, Williams DL, Hayball JD, Diener KR. Evaluation of trained immunity by β-1, 3 (d)-glucan on murine monocytes in vitro and duration of response in vivo. Immunol Cell Biol (2017) 95:601–10. doi:10.1038/icb.2017.13
54. Ai Q, Mai K, Zhang L, Tan B, Zhang W, Xu W, et al. Effects of dietary β-1, 3 glucan on innate immune response of large yellow croaker, Pseudosciaena crocea. Fish Shellfish Immunol (2007) 22:394–402. doi:10.1016/j.fsi.2006.06.011
55. Douxfils J, Fierro-Castro C, Mandiki SNM, Emile W, Tort L, Kestemont P. Dietary β-glucans differentially modulate immune and stress-related gene expression in lymphoid organs from healthy and Aeromonas hydrophila-infected rainbow trout (Oncorhynchus mykiss). Fish Shellfish Immunol (2017) 63:285–96. doi:10.1016/j.fsi.2017.02.027
56. Dhabhar FS. Enhancing versus suppressive effects of stress on immune function: implications for immunoprotection versus. Allergy Asthma Clin Immunol (2008) 4:2–11. doi:10.1186/1710-1492-4-1-2
57. Arts RJW, Novakovic B, ter Horst R, Carvalho A, Bekkering S, Lachmandas E, et al. Glutaminolysis and fumarate accumulation integrate immunometabolic and epigenetic programs in trained immunity. Cell Metab (2016) 24:807–19. doi:10.1016/j.cmet.2016.10.008
58. O’Neill LAJ, Kishton RJ, Rathmell J. A guide to immunometabolism for immunologists. Nat Rev Immunol (2016) 16:553–65. doi:10.1038/nri.2016.70
59. Jones RG, Pearce EJ. MenTORing immunity: mTOR signaling in the development and function of tissue-resident immune cells. Immunity (2017) 46:730–42. doi:10.1016/j.immuni.2017.04.028
60. Blommaart EFC, Luiken JJFP, Blommaart PJE, Van Woerkom GM, Meijer AJ. Phosphorylation of ribosomal protein S6 is inhibitory for autophagy in isolated rat hepatocytes. J Biol Chem (1995) 270:2320–6. doi:10.1074/jbc.270.5.2320
61. Hara K, Yonezawa K, Weng Q-P, Kozlowski MT, Belham C, Avruch J. Amino acid sufficiency and mTOR regulate p70 S6 kinase and eIF-4E BP1 through a common effector mechanism. J Biol Chem (1998) 273:14484–94. doi:10.1074/jbc.273.23.14484
62. Wang X, Campbell LE, Miller CM, Proud CG. Amino acid availability regulates p70 S6 kinase and multiple translation factors. Biochem J (1998) 334(Pt 1):261–7. doi:10.1042/bj3340261
63. Badawy AAB. Kynurenine pathway of tryptophan metabolism: regulatory and functional aspects. Int J Tryptophan Res (2017) 10:1–20. doi:10.1177/1178646917691938
64. Hou DY, Muller AJ, Sharma MD, DuHadaway J, Banerjee T, Johnson M, et al. Inhibition of indoleamine 2,3-dioxygenase in dendritic cells by stereoisomers of 1-methyl-tryptophan correlates with antitumor responses. Cancer Res (2007) 67:792–801. doi:10.1158/0008-5472.CAN-06-2925
65. Kaczorek E, Szarek J, Mikiewicz M, Terech-Majewska E, Schulz P, Małaczewska J, et al. Effect of feed supplementation with kynurenic acid on the morphology of the liver, kidney and gills in rainbow trout (Oncorhynchus mykiss Walbaum, 1792), healthy and experimentally infected with Yersinia ruckeri. J Fish Dis (2017) 40:873–84. doi:10.1111/jfd.12567
66. Fallarini S, Magliulo L, Paoletti T, de Lalla C, Lombardi G. Expression of functional GPR35 in human iNKT cells. Biochem Biophys Res Commun (2010) 398:420–5. doi:10.1016/j.bbrc.2010.06.091
67. Agudelo LZ, Ferreira DMS, Cervenka I, Bryzgalova G, Dadvar S, Jannig PR, et al. Kynurenic acid and Gpr35 regulate adipose tissue energy homeostasis and inflammation. Cell Metab (2018) 27:378–92.e5. doi:10.1016/j.cmet.2018.01.004
68. Seok S-H, Ma Z-X, Feltenberger JB, Chen H, Chen H, Scarlett C, et al. Trace derivatives of kynurenine potently activate the aryl hydrocarbon receptor (AHR). J Biol Chem (2017) 4. doi:10.1074/jbc.RA117.000631
69. Mezrich JD, Fechner JH, Zhang X, Johnson BP, William J. An interaction between kynurenine and the aryl hydrocarbon receptor can generate regulatory T cells. J Immunol (2010) 185:3190–8. doi:10.4049/jimmunol.0903670
70. Kimura A, Naka T, Nakahama T, Chinen I, Masuda K, Nohara K, et al. Aryl hydrocarbon receptor in combination with Stat1 regulates LPS-induced inflammatory responses. J Exp Med (2009) 206:2027–35. doi:10.1084/jem.20090560
71. Routy JP, Routy B, Graziani GM, Mehraj V. The kynurenine pathway is a double-edged sword in immune-privileged sites and in cancer: Implications for immunotherapy. Int J Tryptophan Res (2016) 9:67–77. doi:10.4137/IJTR.S38355
72. Kim KD, Zhao J, Auh S, Yang X, Du P, Tang H, et al. Adaptive immune cells temper initial innate responses. Nat Med (2007) 13:1248–52. doi:10.1038/nm1633
73. García-Valtanen P, Martínez-López A, López-Muñoz A, Bello-Perez M, Medina-Gali RM, Ortega-Villaizán MD, et al. Zebra fish lacking adaptive immunity acquire an antiviral alert state characterized by upregulated gene expression of apoptosis, multigene families, and interferon-related genes. Front Immunol (2017) 8:121. doi:10.3389/fimmu.2017.00121
74. López-Muñoz A, Roca FJ, Meseguer J, Mulero V. New insights into the evolution of IFNs: zebrafish group II IFNs induce a rapid and transient expression of IFN-dependent genes and display powerful antiviral activities. J Immunol (2009) 182:3440–9. doi:10.4049/jimmunol.0802528
75. Zhang J. Yin and yang interplay of IFN-gamma in inflammation and autoimmune disease. J Clin Invest (2007) 117:871–3. doi:10.1172/JCI31860
76. Schmidt SV, Schultze JL. New insights into IDO biology in bacterial and viral infections. Front Immunol (2014) 5:384. doi:10.3389/fimmu.2014.00384
77. Guillonneau C, Mintern JD, Hubert F-X, Hurt AC, Besra GS, Porcelli S, et al. Combined NKT cell activation and influenza virus vaccination boosts memory CTL generation and protective immunity. Proc Natl Acad Sci U S A (2009) 106:3330–5. doi:10.1073/pnas.0813309106
78. Fallarini S, Paoletti T, Panza L, Lombardi G. α-Galactosylceramide modulates the induction of indoleamine 2,3-dioxygenase in antigen presenting cells. Biochem Pharmacol (2008) 76:738–50. doi:10.1016/j.bcp.2008.07.001
Keywords: zebrafish, immunostimulants, β-glucans, stress, tolerance, IFN-γ, kynurenine, TDO
Citation: Álvarez-Rodríguez M, Pereiro P, Reyes-López FE, Tort L, Figueras A and Novoa B (2018) Analysis of the Long-Lived Responses Induced by Immunostimulants and Their Effects on a Viral Infection in Zebrafish (Danio rerio). Front. Immunol. 9:1575. doi: 10.3389/fimmu.2018.01575
Received: 23 April 2018; Accepted: 25 June 2018;
Published: 09 July 2018
Edited by:
Brian Dixon, University of Waterloo, CanadaReviewed by:
Jorge Galindo-Villegas, Universidad de Murcia, SpainFrancesco Buonocore, Università degli Studi della Tuscia, Italy
Copyright: © 2018 Álvarez-Rodríguez, Pereiro, Reyes-López, Tort, Figueras and Novoa. This is an open-access article distributed under the terms of the Creative Commons Attribution License (CC BY). The use, distribution or reproduction in other forums is permitted, provided the original author(s) and the copyright owner(s) are credited and that the original publication in this journal is cited, in accordance with accepted academic practice. No use, distribution or reproduction is permitted which does not comply with these terms.
*Correspondence: Beatriz Novoa, YmVhdHJpem5vdm9hQGlpbS5jc2ljLmVz