- 1IRMB, Univ Montpellier, INSERM, CHU Montpellier, Montpellier, France
- 2Department of Internal Medicine, Multiorganic Diseases, Saint-Eloi Hospital, Montpellier, France
- 3Clinical Immunology and Osteoarticular Diseases Therapeutic Unit, Lapeyronie University Hospital, Montpellier, France
Systemic sclerosis (SSc) is a rare autoimmune disease, which is potentially lethal. The physiopathology of the disease is still incompletely elucidated although the role of fibroblasts, endothelial cells (ECs), immune cells. and the environment (i.e., oxidative stress) has been demonstrated. This is an intractable disease with an urgent need to provide better therapeutic options to patients. Mesenchymal stem cells (MSCs) represent a promising therapeutic approach thanks to the number of trophic and pleiotropic properties they exert. Among these, MSCs display anti-fibrotic, angiogenic, and immunomodulatory capacities that might be of interest in the treatment of SSc by acting on different processes that are dysregulated in the disease. In the recent years, the therapeutic effectiveness of MSCs has been demonstrated in different preclinical animal models and is being investigated in phase I clinical trials. Both allogenic and autologous transplantation of MSCs isolated from bone marrow or adipose tissue is being evaluated. The rationale for using allogenic MSCs in SSc, as well as in other autoimmune diseases, is based on the possibility that autologous MSCs might be altered in these diseases. In SSc, reports from the literature are controversial. Nevertheless, the role of the oxidative environment and of the crosstalk with neighboring cells (fibroblasts and ECs) on the functional properties of MSCs has been reported. Here, we review the preclinical and clinical data reporting the interest of MSC-based treatment in SSc and question the use of autologous or allogeneic MSCs in perspective of clinical applications.
Introduction
Systemic sclerosis (SSc) is a rare autoimmune disease, which affects most frequently middle-age patients with a prevalence ranging from 100 to 300 per million depending on the country. The pathophysiology of SSc is still not completely understood even though three main axes of dysfunction are reported: fibrosis, vascular activation and immune abnormalities. The disease is characterized by vascular damage and diffuse fibrosis, which mainly affects skin and lung tissues but heart and digestive tract could also be involved (1). SSc is typically classified as limited or diffuse according to the extent and distribution of skin fibrosis (2). One of the earliest and most frequent symptom is the Raynaud's Phenomenon but vasculopathy is also responsible of other clinical signs including digital ulcers, pulmonary arterial hypertension, and telangiectasia (3). All of these symptoms are responsible for increased morbidity and lead to functional disability (reduced mouth opening and loss of hand function, for example), pain, and psychological consequences. This impacts not only the patient's quality of life but also reduces his life expectancy. In at least half of the cases, patients will die from SSc-related disorders and the other half from higher incidence of malignancies and cardiovascular diseases compared to the general population (4–6).
There is no curative treatment to date. Only symptomatic treatments are commonly proposed to patients to alleviate pain and improve function. Novel therapeutic strategies are being envisaged among which mesenchymal stem cells (MSCs)-based therapy, which is currently under evaluation in the clinics. The first clinical trial has evaluated the safety of MSCs-containing autologous stromal vascular fraction (SVF) (7) but the option of using allogeneic MSCs is also under evaluation since publications have reported potential alterations of MSCs from SSc patients. Here, we review the available literature on MSC-based cell therapies in SSc from pre-clinical models to clinical applications and discuss the interest of using allogeneic or autologous MSCs for future clinical trials.
Generalities on Mesenchymal Stem Cells
MSCs are adult multipotent progenitor cells, which have been first identified in the bone marrow (BM) (8). In addition to bone marrow-derived MSCs (BM-MSCs), they have been described in different niches and isolated from several tissues, including adipose tissue (ASCs), umbilical cord (UC-MSCs), placenta, or dental pulp (9). Actually, MSCs have been proposed to be present in virtually all organs as pericytes, which show identical differentiation capacities in vitro. However, recent lineage-tracing experiments indicate that in vivo, pericytes do not contribute to other cell lineages (10). These results therefore challenge the concept that MSCs, or at least part of them, are pericytes behaving as multipotent tissue progenitors.
MSCs are defined by 3 criteria, as proposed by the International Society for Cell Therapy: (1) plastic adherence, (2) expression of the cell surface markers CD73, CD90, CD105, and lack of expression of the hematopoietic markers CD11b or CD14, CD19 or CD79α, CD34, CD45, HLA-DR, and (3) capacity of differentiation into adipocytes, chondrocytes, and osteoblasts (11). Along with their potential of multilineage differentiation, MSCs exert a number of paracrine functions: they support survival and differentiation of hematopoietic stem cells, induce cell proliferation and have anti-fibrotic, anti-apoptotic, pro-angiogenic, anti-bacterial, and anti-inflammatory effects (12). These effects are mediated primarily through the secretion of soluble mediators but can be enhanced upon contact of MSCs with the target cells (13). The soluble factors are released in the extracellular milieu or within extracellular vesicles that protect them from degradation and allow their transfer throughout the organism [for review, see (12, 14)]. Although, MSCs from different tissue sources share similar properties, they may display some differences in their differentiation potential or immunomodulatory capacity (15, 16).
Thanks to this pleiotropic activity, the therapeutic efficacy of MSCs has been investigated in different pathological conditions, from inflammatory diseases to acute or degenerative diseases. So many different applications as rheumatic diseases (17), stroke (18), lupus and scleroderma (19), heart diseases (20), or bone defects (21) have been evaluated. To date, thousands of patients have been enrolled in hundreds of clinical trials and safety of MSC injection has been proved. In 2016, a meta-analysis has reported no acute toxicity, death, infection, organ systemic failure or risk malignancy after MSC implantation (22). Only transient fever was frequently observed. Efficacy of MSC-based treatments as compared to standards of care is still however under evaluation but promising results have been achieved mostly in phase II trials. For SSc, very few trials have been initiated and results from a couple of registered phase I/II clinical trials are still pending.
Therapeutic Effect of MSCs in Preclinical Models of SSc
Before the initiation of clinical trials, the interest of using MSCs in the treatment of SSc has been evaluated in different preclinical models. Three models have been used: the model of bleomycin-induced pulmonary fibrosis, the model of hypochlorous acid (HOCl)-induced SSc and the tight skin (Tsk1/+) mouse model of SSc.
The Model of Bleomycin-Induced Fibrosis
The model of bleomycin-induced fibrosis is the most widely used model to replicate scleroderma, or dermal or pulmonary fibrosis in mice or rats. According to the mode of administration, fibrosis can be induced in skin when bleomycin is injected daily sub-dermally or in lung after a single intra-tracheal instillation. Fibrosis in lung, skin, and internal organs may be induced via the use of osmotic pumps that deliver a constant amount of bleomycin over a number of days (23, 24). It is believed that bleomycin causes breaks in DNA, resulting in overproduction of reactive oxygen species (ROS) and inflammatory response that activate fibroblasts and subsequently fibrosis formation. Importantly, the bleomycin models replicate some of the earliest patterns of SSc but do not present with the typical clinical signs and autoantibody patterns of SSc.
The therapeutic potential of MSCs has been first demonstrated in the mouse model of lung fibrosis. One intravenous injection of 500,000 allogenic BM-MSCs on the day of bleomycin instillation was shown to reduce collagen content and inflammation in the lungs (25). At day 14 after injection, the authors could detect engraftment of BM-MSCs in areas of bleomycin-induced injury where they adopted an epithelium-like phenotype. Another study confirmed that systemic injection of allogenic BM-MSCs after intra-tracheal instillation of bleomycin protected mice from injury and fibrosis through the suppression of inflammation (26). They also reported that BM-MSCs differentiated into distinct lung cell phenotypes and secreted chemokines that might have attracted endogenous cells. Due to the relatively low numbers of transplanted MSCs and persistence of some injury in BM-suppressed animals, the authors suggest that both endogenous and exogenous MSCs likely contribute to the repair process. Another study comparing minimally cultured (2 h) to conventionally cultured (9 days) BM-MSCs indicated that both types of cell preparations ameliorated as efficiently inflammatory and progressive fibrotic lung injury (27). Minimally cultured BM-MSCs had a higher proliferative capacity, expressed higher levels of stem cell markers, and chemokine receptors but lower levels of type I procollagen, α-smooth muscle actin (α-sma) and transforming growth factor-β (TGFβ) that might be more advantageous for cell-based therapy.
The therapeutic effect of BM-MSCs has been also confirmed in rat models of bleomycin-induced lung fibrosis. One intravenous injection of five millions of BM-MSCs resulted in decreased levels of TGFβ1, platelet-derived growth factor-A (PDGF-A), PDGF-B, insulin growth factor-1 (IGF1), and in lower collagen content in lungs (28). Another report has shown that systemic injection of syngeneic BM-MSCs ameliorated fibrosis and decreased inflammatory and angiogenic markers as well as nitric oxide metabolites (29).
In a dermal fibrosis model induced by daily subcutaneous injection of bleomycin for 4 weeks, syngeneic BM-MSCs were injected subcutaneously into the lesion skin every day (30). Treatment with BM-MSCs resulted in a basket-weave organization of collagen arrangement similar to normal skin, with few inflammatory cells and α-sma-positive myofibroblasts as well as down-regulation of TGFβ, type I collagen, and heat-shock protein 47 (HSP47) expression in skin.
The impact of MSCs from different species or tissues on treatment efficacy has been evaluated in the mouse model of bleomycin-induced lung fibrosis. Human UC-MSCs injected systemically 24 h after bleomycin instillation in SCID mice were reported to reduce inflammation markers, collagen content, and inhibit expression of TGFβ, interferon-γ (IFNγ), tumor necrosis factor-α (TNFα) (31). In this study, improvement of tissue remodeling was shown with increased levels of matrix metalloproteinase-2 (MMP-2) and decreased levels of their endogenous inhibitors, tissue inhibitor of MMP (TIMP). Of interest, another report demonstrated that both human amnion- and chorion-derived fetal MSCs displayed similar reduction in the severity of bleomycin-induced lung fibrosis as allogeneic murine amnion- and chorion-derived fetal MSCs (32). Amniotic fluid MSCs were shown to inhibit collagen deposition and to preserve pulmonary function, which could be related to transient increase of MMP-2 (33). Cells were observed to localize within fibrotic lesions with a preferential targeting to the area of fibrosis. Similarly, compared to BM-MSCs, human amnion-derived MSCs reduced collagen deposition and increased MMP-9 activity (34). Finally, ASCs attenuated lung fibrosis induced by repetitive intra-tracheal administrations of bleomycin, namely hyperplasia of Club cells (Clara cells) and cuboidal alveolar epithelial cells, infiltration of the perialveolar ducts by inflammatory cells, septal thickening, enlarged alveoli, and extensive fibrosis (35). It also led to suppression of epithelial cell apoptosis and expression of TGFβ suggesting that irrespective of the tissue or species origin, MSCs are potent inhibitors of lung fibrosis.
The time of cell injection after disease induction may be one important factor to control for better efficacy of cell therapy. Most frequently, MSCs have been implanted at the time or within the few hours after bleomycin instillation. Interestingly, early treatment (day 0) with murine or rat BM-MSCs on the day of bleomycin instillation resulted in a significant reduction of fibrotic changes that was not seen when BM-MSCs-based treatment was delayed (day 7) (25, 36). Similarly, two injections of low (2.8 × 106cells/kg) or high (5.6 × 106cells/kg) doses of human BM-MSCs at day 7 and 15 after fibrosis induction did not lead to improvement of lung function or rescue of damage tissue (37). Injection of autologous ASCs via the trachea at day 15 of the disease did not improve the severity of lung injury but prevented further aggravation of lung damage (38). In this report, the majority of ASCs did not penetrate inside the lung region at week 3 but some cells had sprouted deep into the distorted architecture of the lung at week 6 after disease induction. By contrast, injections of murine amniotic fluid-derived MSCs at either day 0 or day 14 were both efficient to inhibit fibrosis indicating improvement of the disease in both acute and chronic remodeling events (33). Injections of amniotic MSCs or BM-MSCs at day 3 or 6 were even more efficient to reduce lung inflammation than the treatment at day 1 (39). In addition, in a model of repeated injections of bleomycin at day 0 and 7, BM-MSCs injected at day 10 were beneficial in terms of collagen deposition reduction and MMP-9 down-regulation (34).
The age of the donors may be another parameter that might change the therapeutic potential of MSCs. One study compared ASCs from aged (>22 months) and young (4 months) mice (40). At day 21 after bleomycin instillation, mice receiving young ASCs exhibited decreased fibrosis, MMP-2 activity, oxidative stress, and markers of apoptosis vs. untreated controls. Improved treatment with young-donor ASCs was associated with decreased mRNA expression of MMP-2, IGF receptor, and protein kinase B (AKT) activation.
For more a decade since 2003, the skin or lung bleomycin-induced model of local fibrosis was the single model used to evaluate the therapeutic effect of MSCs. However, it does not reproduce the main characteristics of the diffuse human SSc. With respect to the systemic nature of the disease, relevant models of systemic fibrosis have to be used to better evaluate the efficacy of MSCs in models closer to human SSc.
The Model of Bleomycin-Induced Lung and Skin Fibrosis in Aged Mice
A study relied on the use of an established aged (18–22-month-old) mouse model of bleomycin-induced lung fibrosis to test the hypothesis that fibrosis may develop simultaneously in multiple organs and notably affects lung, skin and wound healing. Mice developed irreversible lung and skin fibrosis as well as delayed wound closure at day 21 (41). In this model, intravenous single injection of allogeneic ASCs attenuated lung fibrosis as evaluated by semi-quantitative Ashcroft score on Masson's-trichrome stained histological sections. ASCs also accelerated wound healing as shown by increased total wound size and wound gaps. This effect was associated with higher levels of caveolin-1 and lower level of αvintegrin, TNFα, and miR-199-3p in lung and skin wounds. These results therefore support the hypothesis that ASCs may prevent systemic fibrosis and enhance wound healing but rely on the use of aged mice that are expensive and difficult to get.
The Murine Model of HOCl-Induced SSc
In order to evaluate the therapeutic effect of MSCs in a systemic model of scleroderma, the murine model of HOCl-induced SSc is relevant, cheap and reproducible. After daily intradermal injections of HOCl for 6 weeks, mice develop a systemic disease with the main features of the human form, including skin and lung fibrosis, vascular abnormalities and the production of Anti-Scl-70 autoantibodies, which are anti-nuclear topoisomerase antibodies mainly detected in diffuse systemic scleroderma (42). Our group demonstrated the therapeutic effect of a single intravenous injection of syngeneic BM-MSCs in preventive (day 0) but also curative (day 21) approaches (43). Interestingly, the dose escalation study revealed that the best improvement of both skin and lung fibrosis was obtained with the lowest dose of 2.5 × 105 BM-MSCs. We also compared different sources of cells, i.e., allogenic, xenogeneic, and syngeneic BM-MSCs as well as xenogeneic ASCs, which seemed to be the most potent as compared to BM-MSCs (44). The therapeutic effect was observed on all features of SSc, including reduction of fibrotic, inflammatory. and oxidative markers, but also reduction of autoantibodies Scl-70 while matrix remodeling markers were increased. Finally, infused GFP-expressing allogeneic BM-MSCs were entrapped in the lungs where they were detected at days 1 and 2 but not at day 7. Interestingly, either murine allogenic or human xenogeneic BM-MSCs did not migrate to the skin although skin fibrosis was reduced, indicating a systemic effect of BM-MSCs (44, 45).
The Model of Tight Skin (Tsk1/+) Mouse
The tight skin 1 (Tsk1/+) mouse occurred as a spontaneous mutation in fibrillin-1, which controls the biologically active levels of TGFβ through binding to the latent TGFβ binding proteins (LTBP) 1 and 4 (46). It has therefore been suggested that activation of the TGFβ signaling axis is involved in the development of the phenotype. This genetic model of SSc is characterized by hyperplasia of the sub-cutaneous loose connective tissue with abnormal dermis, osteopenia and deregulation of the interleukin-4 (IL4)/IL4 receptor (IL4R) signaling pathway. Interestingly in this model, MSCs have been shown to ameliorate osteopenia by rescuing impaired lineage differentiation of the recipient BM-MSCs (47). In this interesting study, the authors further demonstrated that MSC-derived extracellular vesicles containing miR-151-5p rescued the disease phenotypes via regulation of the IL4R pathway in recipient BM-MSCs.
Altogether, the beneficial effect of MSCs-based treatment has been proved in the different models of local or systemic scleroderma (Table 1). Some clues of their mechanism of action have also been investigated as discussed below.
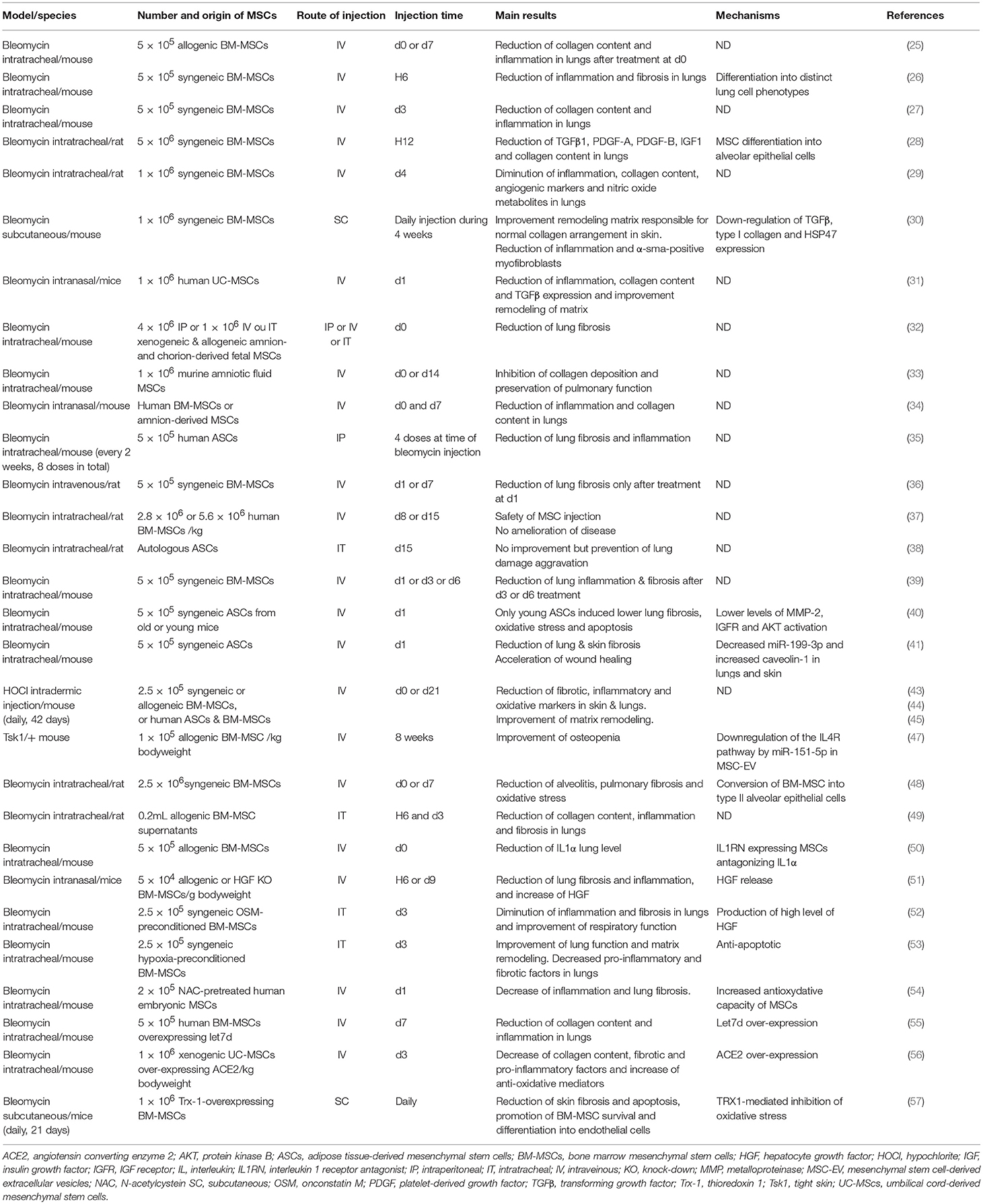
Table 1. Summary of studies on the role of mesenchymal stem cells in preclinical models of systemic sclerosis.
Mechanisms of Action of MSC-Based Therapy in SSc
The capacity of MSCs to differentiate into several cell types of musculoskeletal tissues was the first property that has attracted the attention of researchers and clinicians (58). Since then, several mechanisms of action have been proposed to play important roles in various disorders and diseases via the secretion of many soluble mediators as summarized in (12). Besides anti-apoptotic and anti-bacterial functions, the support of hematopoietic stem cells, the chemoattracting effect, the proliferative, and protective role of MSCs has been largely exemplified. In SSc, the main functions of MSCs are proposed to be anti-inflammatory to counteract the dysregulation of the immune system, anti-fibrotic to down-regulate the excessive production of collagen associated to thickening of skin and internal organs but also pro-angiogenic to counteract the widespread vasculopathy. A number of reviews have discussed the interest of using MSCs for the treatment of SSc (19, 59, 60). In the next paragraph, we focused our attention on the mechanisms that have been deciphered specifically in preclinical models of SSc.
Systemic administration of MSCs results in their homing in lungs and cell engraftment is increased in lungs from bleomycin-induced mice, in particular in areas of injury where they adopt an epithelial cell morphology and display anti-oxidative role (25, 48). Of interest, in the HOCl-induced systemic model of SSc, both human or green fluorescent protein (GFP)-positive murine BM-MSCs were shown to be retained in the lungs of mice after intravenous administration while no cells were detected in the skin suggesting a systemic effect of infused BM-MSCs (43, 45). In addition, tracheal instillation of BM-MSC supernatants decreased the number of apoptotic cells, collagen content, inflammation and fibrotic scores in lungs in the model of bleomycin-induced lung fibrosis (49). These results suggest that BM-MSCs preferentially act through the secretion of soluble mediators that are distributed throughout the body but they can also act directly in contact with damaged lungs where they preferentially reside.
Some secreted mediators involved in their therapeutic effect including IL1-RA, hepatocyte growth factor (HGF) have been identified in murine models of lung fibrosis (50, 51). However, novel strategies of MSC-based treatments have been tested in order to improve their efficacy by enhancing survival after in vivo administration or increasing their trophic action. Indeed, preconditioning of BM-MSCs before administration was shown to enhance their therapeutic efficacy. Namely, oncostatin M- or hypoxia-preconditioned BM-MSCs improved pulmonary respiratory function thanks to decreased inflammatory and fibrotic markers as compared to unconditioned BM-MSCs and this effect was mediated at least through the upregulation of HGF (52, 53). Pre-treatment of BM-MSCs with N-acetylcysteine was able to increase both treatment efficacy in a murine model of bleomycin-induced lung injury and BM-MSC survival through the production of anti-oxidant factors (54). In order to improve their therapeutic function, strategies to over-express factors have also been tested. Overexpression of let7d, which is a microRNA known to have anti-fibrotic effects, in BM-MSCs resulted in slight decrease of collagen content in lungs and improved mouse survival when injected at day 7 in bleomycin-induced mice while naïve BM-MSCs did not have any beneficial effect (55). Similarly, human UC-MSCs over-expressing angiotensin-converting enzyme 2 (ACE2) were more efficient than naïve UC-MSCs to decrease collagen content, fibrotic, and pro-inflammatory factors while increasing anti-oxidative and anti-inflammatory mediators (56). Thioredoxin 1 (Trx-1)-overexpressing BM-MSCs were also shown to inhibit apoptosis and fibrosis under hypoxic conditions and to promote the formation of tubular-like structures by endothelial cells in bleomycin-induced lung injury (57). Indeed, strategies for enhancing MSCs survival and/or efficiency have been demonstrated to be of interest in preclinical models of SSc. A summary of the potential (or proven) mechanisms of action of MSCs in the treatment of SSc is provided in Figure 1.
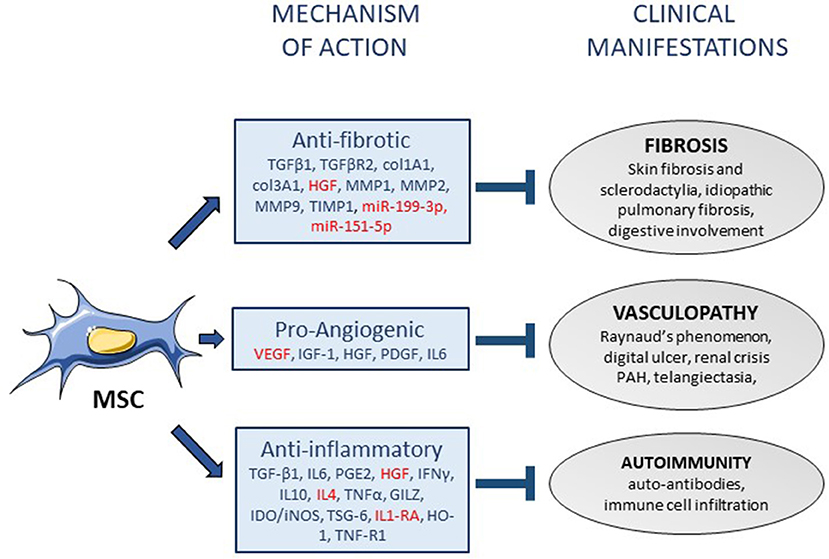
Figure 1. Mechanisms of action of mesenchymal stem cells in systemic sclerosis. Mesenchymal stem cells (MSC) display various functions that might participate to their mechanisms of action in the treatment of systemic sclerosis (SSc), through the release of soluble mediators (arrows). The mediators that have been associated with anti-fibrotic, pro-angiogenic or anti-inflammatory functions of MSCs are listed in the squares (in red: mediators specifically identified in SSc). Three mechanisms of action of MSCs can be associated with the improvement of the three main clinical manifestations of SSc (blind-ended arrows). PAH, pulmonary arterial hypertension.
Characterization of MSCs from SSc Patients
Early studies evaluating the phenotypic and functional characteristics of MSCs from SSc patients reported no major difference as compared with MSCs from healthy donors. Indeed, BM-MSCs from two patients with SSc have been compared to those of healthy donors 10 years ago (61). No major differences in their proliferative rate, adipogenic or osteogenic differentiation potential, expression of surface markers and immunosuppressive properties were reported between the two sources of BM-MSCs. Some years later, similar data were obtained using BM-MSCs from 12 scleroderma patients in comparison with 13 healthy controls (62). SSc and healthy BM-MSCs displayed the same phenotype, identical capacities to proliferate and form CFU-F, similar capacities to differentiate into adipogenic and osteogenic lineages, equal capacities to support long-term hematopoiesis, and similar anti-proliferative properties. SSc ASCs were also shown to have comparable phenotypic markers and functional characteristics (proliferative and differentiation potential) as healthy donor ASCs (63). However, this cohort of six SSc patients was characterized by cutaneous local forms of scleroderma (generalized morphea, linear and/or plaque scleroderma) and not diffuse systemic sclerosis. More recently, no difference in proliferative capacities and potential to support endothelial cell tube formation was noticed between ASCs from ten patients with diffuse SSc or eight healthy donors (64).
By contrast, other studies have reported some differences. As an example, MSCs isolated from the skin of scleroderma patients expressed more PDGF-R than those from healthy donors, resulting in the production of higher levels of ROS (65). Nevertheless, SSc-MSCs were still able to improve the antioxidant defenses by counterbalancing ROS accumulation. More recently, the impact of the pro-oxidant environment exerted by SSc sera on BM-MSCs has been investigated. Exposure of BM-MSCs to SSc sera enhanced their antioxidant capacities as well as their osteogenic and adipogenic potentials while their immunosuppressive functions were reduced (66). With the objective to evaluate the consequence of TGFβ activation in SSc, analysis of several members of the TGFβ family has been undertaken in BM-MSCs from nine patients with diffuse SSc (67). A highly significant increase in mRNA and protein levels of TGFβ receptor II (TβRII) was detected in SSc BM-MSCs as compared to healthy controls. Moreover, in response to TGFβ activation, the production of type I collagen and Smad-3 phosphorylation was up-regulated in SSc BM-MSCs and also in SSc fibroblasts. An earlier study reported that nerve growth factor receptor (NGFR)-positive BM-MSCs, a sub-population of MSCs with high proliferative and clonogenic potentials, were less numerous in BM from SSc patients than from healthy controls (68). They displayed lower clonogenic potential (10-fold less), had a reduced fold expansion rate (105-fold less), showed signs of rapid aging and stress and, never differentiated into adipocytes or osteoblasts. In line with these data, Cipriani and coauthors have described that BM-MSCs from SSc subjects expressed up-regulated α-sma and transgelin (TAGLN or SM22α) genes and displayed reduced proliferative activity and migration potential (69). An increase in senescence markers (senescence-associated β-galactosidase (SA-βGal), p21, IL6, TGFβ) was also observed while their immunosuppressive activity on lymphocyte proliferation and regulatory phenotype induction was retained (70). Interestingly, the same team showed an impaired crosstalk between endothelial cells (ECs) and BM-MSCs (71). In coculture conditions, SSc ECs induced increased levels of VEGF-A in SSc MSCs and of TGFβ, PDGF-R, α-sma, type I collagen in both SSc and healthy MSCs. Despite the production of high levels of VEFG-A by SSc MSCs, they had lower angiogenic performance. The authors also detected increased expression of TGFβ and PDGF-BB in SSc ECs when co-cultured either with MSCs from healthy or SSc individuals. This impaired crosstalk between ECs and MSCs suggested that ECs may be involved in the early steps leading to fibrosis by producing factors that induce a phenotype switch of MSCs toward myofibroblast cells. In another study, SSc BM-MSCs exhibited altered differentiation into contractile and synthetic vascular smooth muscle cells when stimulated by connective tissue growth factor (CTGF) or b-FGF. Moreover, stimulation with TGFβ1 induced a myofibroblast-like phenotype with high production of α-sma and collagens, higher migration capacity but less proliferative capacity (72). Accordingly, SSc BM-MSCs expressed up-regulated levels of proangiogenic factors including VEGF, stromal derived factor-1 (SDF-1) and C-X-C chemokine receptor (CXCR4) (73). However, they noticed that SSc BM-MSCs promoted angiogenesis and improved capillary morphogenesis as compared to healthy MSCs.
A unique study has identified altered functions in SSc ASCs (74). No alteration in their phenotype or differentiation potential was noticed but their proliferative rate, metabolic activity and migration capacity were reduced as compared to healthy matched control ASCs. However, no data on the expression of TβRII or other fibrotic markers as well as on their immunosuppressive and angiogenic functions were available. Further functional analysis is therefore required to fully decipher the therapeutic potential of ASCs isolated from SSc patients.
Clinical Data on MSC-based Treatment in SSc
Case Reports Using Fat Injection
In recent decades, fat tissue grafting has been used to treat skin atrophy or fibrosis thanks to its biocompatibility and property of filling but also to its high content in multipotent stem or progenitor cells with regenerative potential. The first report on the implantation of fat in hands of patients who presented Raynaud's phenomenon was published in 2014 (75). Among the thirteen treated patients, nine patients suffered from scleroderma. There were no complications and the treatment showed some evidence of perfusion. In 2015, another study reported a significant improvement of digital ulcerations, hand grasping, and pain after injection of adipose tissue-derived cells into fingers of 15 SSc patients suffering from digital ulcers (76). More recently, the effect of one local injection of autologous SVF associated with platelet-rich plasma into malar and perioral areas in six patients showed improvement of skin elasticity and vascularization (77). Some indication of efficacy was suggested by these case reports but the role of ASCs contained within the SVF could not be drawn.
Case Reports Using MSCs
The first patient who received BM-MSCs in the treatment of progressive diffuse SSc has been reported in 2008 (78). A young female patient had severe disease, refractory to all immunosuppressive drugs. At time of implantation, she presented with six painful ulcerations and received 6 × 107 intravenous administration of allogeneic haploidentical-related donor BM-MSCs. No adverse events were reported and 3 months after treatment, a significant decrease in the patient's painful ulcerations was measured. Vascular improvement in the blood circulation of hands and fingers was noticed but level of Scl-70 autoantibodies was not decreased. In 2011, the same team reported four supplementary cases of allogeneic BM-MSC systemic injection (0.22 to 1.8 × 106 BM-MSCs/kg bodyweight) (79). Here again, improvement of vasculopathy and skin fibrosis was observed but the study underscored the need to set phase II clinical trials for efficacy assessment.
Local injection of autologous ASCs in combination with hyaluronic acid (HA) solution has been first tested in 2013 (63). Infiltration of 8 × 105 ASCs/mL HA was done in a single area for each patient, either the face or the arm chosen with the patient consent. The procedure improved considerably skin fibrosis for four out of six patients and moderately for one patient. All patients showed arrest of local disease progression (100%), four of them presented regression of dyschromia (67%), five patients increased skin softening (83%), four patients showed better sensitivity (67%), and one patient reported erythema reduction (17%). The study demonstrated that ASCs could be successfully implanted locally in patients with severe disease, thus representing a good, feasible, and efficient cell-based soft-tissue augmentation strategy. Of interest, the same team thereafter compared autologous fat and ASC transplantation to evaluate clinical improvement of mouth opening in two groups of five patients (80). Both procedures obtained significant results but neither one emerged as a first-choice technique.
Clinical Trials With MSCs
The results of the first open phase I clinical trial evaluating the interest of MSCs, and more precisely of MSC-containing SVF, on 12 SSc patients with severe functional hand handicap were published in 2015 (7). Injection of autologous SVF into fingers was safe and a significant improvement in pain, grasping capacity, finger edema, Raynaud's phenomenon, and quality of life was recorded at 6 months. At 12 months follow-up, a significant improvement in skin sclerosis, motion, edema and strength of the hand was observed. SVF was therefore claimed as a promising therapy whose effect persisted at least 1 year after injection (81). Finally, at 22 and 30 months after treatment, safety, tolerability and efficacy were very encouraging (82). The same team is now including 40 patients in a randomized double blind phase II trial to evaluate efficacy of the approach.
There are only 4 clinical trials recorded in the United State National library of Medicine (www.clinicaltrials.gov). One trial has been completed but no result is available. Only one of those is recruiting in France since 2014. This is a phase I/II trial which assesses the effect of one intravenous injection of intrafamilial allogenic MSCs on 20 patients. The trial is still enrolling patients and data are not yet available. Another phase I/II clinical trial has started in November 2017. It evaluates the therapeutic effect of allogeneic BM-MSCs after injection in intramuscular areas of affected limbs in 20 scleroderma patients with digital ulcers and is recruiting (NCT03211793) (83).
In summary, data from case reports and clinical trials are scare and need to be taken with cautious in terms of efficacy (Table 2). One question still pending is whether autologous or allogeneic ASCs or BM-MSCs have to be used in the clinics since some studies have reported phenotypic or functional alterations of MSCs from SSc patients.
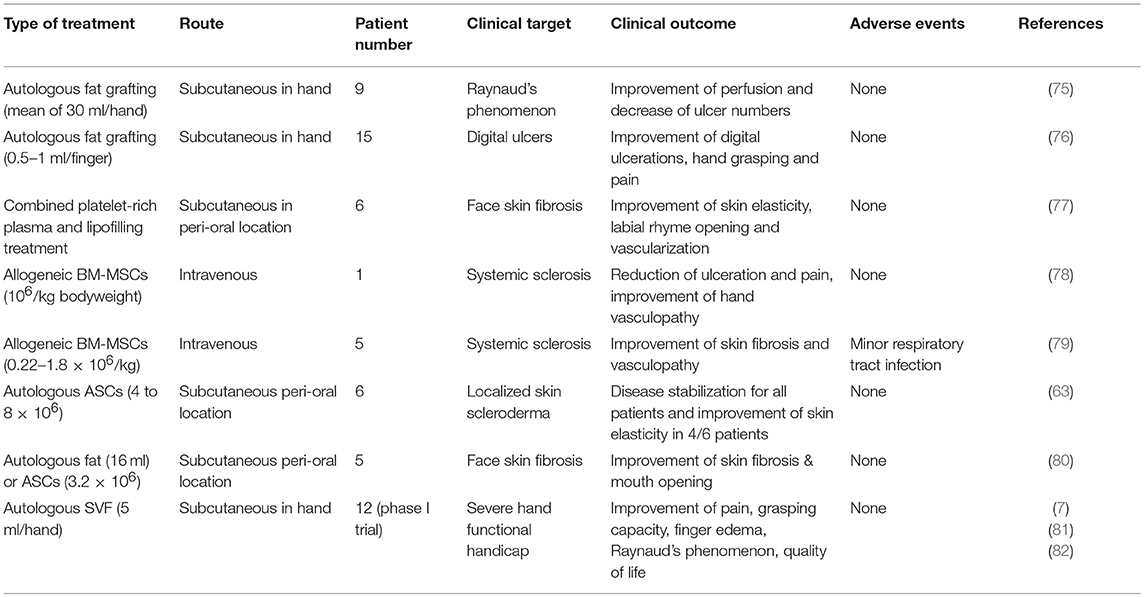
Table 2. Summary of clinical trials using mesenchymal stem cells for the treatment of systemic sclerosis.
Conclusion
Many studies have now established the beneficial effect of the administration of BM-MSCs, ASCs or MSCs from other tissue sources in different preclinical models characterized by local or systemic fibrosis. Some evidence of safety and efficacy of MSC-containing SVF or culture expanded MSCs has been described from the clinics but efficacy needs to be further proved in phase II clinical trials that are ongoing. Both autologous and allogeneic MSCs from BM or adipose tissue are being assessed but the risk that the functional properties of MSCs isolated from SSc patients are altered is under debate. Contradictory results are reported in the literature but a number of reports discuss the reduction of the number of clonogenic cells, proliferative rate, differentiation, and angiogenic potentials. MSCs from SSc patients display a more mature and myofibroblast-like phenotype, probably related to microenvironmental signals dysregulated during the disease. They express higher levels of TβRII and TGFβ, which is released into the extracellular medium where it can act in an autocrine or paracrine manner. Moreover, the crosstalk between MSCs and ECs contribute to the altered expression of different molecules involved in angiogenesis, inducing a switch of perivascular MSCs toward a myofibroblast population, further supporting the fibrotic process. The finding that MSCs from SSc patients constitutively overexpress mediators involved in the fibrotic and angiogenic processes might indicate that MSCs are altered by the environment secondary to the onset of the disease or, that they might participate to the physiopathology of the disease. With respect to the use of autologous MSCs for clinical applications, further investigation on their functional properties is likely needed.
Author Contributions
All authors listed have made a substantial, direct and intellectual contribution to the work, and approved it for publication.
Conflict of Interest Statement
The authors declare that the research was conducted in the absence of any commercial or financial relationships that could be construed as a potential conflict of interest.
Acknowledgments
Work in the laboratory Inserm U1183 was supported by the Inserm Institute and the University of Montpellier. Study was also supported by the Montpellier-Nîmes University Hospital and Association des Sclérodermiques de France (ASF) for funding. We thank the Agence Nationale pour la Recherche for support of the national infrastructure: ECELLFRANCE: development of a national adult mesenchymal stem cell based therapy platform (ANR-11-INSB-005).
References
1. Chighizola C, Shoenfeld Y, Meroni PL. Systemic sclerosis. Introduction. Autoimmun Rev. (2011) 10:239–40. doi: 10.1016/j.autrev.2011.02.005
2. LeRoy EC, Medsger TA Jr. Criteria for the classification of early systemic sclerosis. J Rheumatol. (2001) 28:1573–6.
3. Desbois AC, Cacoub P. Systemic sclerosis: an update in 2016. Autoimmun Rev. (2016) 15:417–26. doi: 10.1016/j.autrev.2016.01.007
4. Elhai M, Meune C, Boubaya M, Avouac J, Hachulla E, Balbir-Gurman A, et al. Mapping and predicting mortality from systemic sclerosis. Ann Rheum Dis. (2017) 76:1897–905. doi: 10.1136/annrheumdis-2017-211448
5. Poudel DR, Jayakumar D, Danve A, Sehra ST, Derk CT. Determinants of mortality in systemic sclerosis: a focused review. Rheumatol Int. (2017) 38:1847–58. doi: 10.1007/s00296-017-3826-y
6. Zeineddine N, Khoury LE, Mosak J. Systemic sclerosis and malignancy: a review of current data. J Clin Med Res. (2016) 8:625–32. doi: 10.14740/jocmr2606w
7. Granel B, Daumas A, Jouve E, Harle JR, Nguyen PS, Chabannon C, et al. Safety, tolerability and potential efficacy of injection of autologous adipose-derived stromal vascular fraction in the fingers of patients with systemic sclerosis: an open-label phase I trial. Ann Rheum Dis. (2015) 74:2175–82. doi: 10.1136/annrheumdis-2014-205681
8. Friedenstein AJ, Gorskaja JF, Kulagina NN. Fibroblast precursors in normal and irradiated mouse hematopoietic organs. Exp Hematol. (1976) 4:267–74.
9. da Silva Meirelles L, Chagastelles PC, Nardi NB. Mesenchymal stem cells reside in virtually all post-natal organs and tissues. J Cell Sci. (2006) 119:2204–13. doi: 10.1242/jcs.02932
10. Guimaraes-Camboa N, Cattaneo P, Sun Y, Moore-Morris T, Gu Y, Dalton ND, et al. Pericytes of multiple organs do not behave as mesenchymal stem cells in vivo. Cell Stem Cell (2017) 20:345–59 e5. doi: 10.1016/j.stem.2016.12.006
11. Dominici M, Le Blanc K, Mueller I, Slaper-Cortenbach I, Marini F, Krause D, et al. Minimal criteria for defining multipotent mesenchymal stromal cells. The international society for cellular therapy position statement. Cytotherapy (2006) 8:315–7. doi: 10.1080/14653240600855905
12. Maumus M, Jorgensen C, Noel D. Mesenchymal stem cells in regenerative medicine applied to rheumatic diseases: role of secretome and exosomes. Biochimie (2013) 95:2229–34. doi: 10.1016/j.biochi.2013.04.017
13. Luz-Crawford P, Noel D, Fernandez X, Khoury M, Figueroa F, Carrion F, et al. Mesenchymal stem cells repress Th17 molecular program through the PD-1 pathway. PLoS ONE (2012) 7:e45272. doi: 10.1371/journal.pone.0045272
14. Abbasi-Malati Z, Roushandeh AM, Kuwahara Y, Roudkenar MH. Mesenchymal stem cells on horizon: a new arsenal of therapeutic agents. Stem Cell Rev. (2018) 14:484–99. doi: 10.1007/s12015-018-9817-x
15. Kern S, Eichler H, Stoeve J, Kluter H, Bieback K. Comparative analysis of mesenchymal stem cells from bone marrow, umbilical cord blood, or adipose tissue. Stem Cells (2006) 24:1294–301. doi: 10.1634/stemcells.2005-0342
16. Keyser KA, Beagles KE, Kiem HP. Comparison of mesenchymal stem cells from different tissues to suppress T-cell activation. Cell Transplant. (2007) 16:555–62. doi: 10.3727/000000007783464939
17. Ruiz M, Cosenza S, Maumus M, Jorgensen C, Noel D. Therapeutic application of mesenchymal stem cells in osteoarthritis. Expert Opin Biol Ther. (2015) 16:33–42. doi: 10.1517/14712598.2016.1093108
18. Toyoshima A, Yasuhara T, Date I. mesenchymal stem cell therapy for ischemic stroke. Acta Med Okayama (2017) 71:263–8. doi: 10.18926/AMO/55302
19. Cras A, Farge D, Carmoi T, Lataillade JJ, Wang DD, Sun L. Update on mesenchymal stem cell-based therapy in lupus and scleroderma. Arthritis Res Ther. (2015) 17:301. doi: 10.1186/s13075-015-0819-7
20. Yu H, Lu K, Zhu J, Wang J. Stem cell therapy for ischemic heart diseases. Br Med Bull. (2017) 121:135–54. doi: 10.1093/bmb/ldw059
21. Paduano F, Marrelli M, Amantea M, Rengo C, Rengo S, Goldberg M, et al. Adipose tissue as a strategic source of mesenchymal stem cells in bone regeneration: a topical review on the most promising craniomaxillofacial applications. Int J Mol Sci. (2017) 18:2140. doi: 10.3390/ijms18102140
22. Lalu MM, McIntyre L, Pugliese C, Fergusson D, Winston BW, Marshall JC, et al. Safety of cell therapy with mesenchymal stromal cells (SafeCell): a systematic review and meta-analysis of clinical trials. PLoS ONE (2012) 7:e47559. doi: 10.1371/journal.pone.0047559
23. Artlett CM. Animal models of systemic sclerosis: their utility and limitations. Open Access Rheumatol. (2014) 6:65–81. doi: 10.2147/OARRR.S50009
24. Moeller A, Ask K, Warburton D, Gauldie J, Kolb M. The bleomycin animal model: a useful tool to investigate treatment options for idiopathic pulmonary fibrosis? Int J Biochem Cell Biol. (2008) 40:362–82. doi: 10.1016/j.biocel.2007.08.011
25. Ortiz LA, Gambelli F, McBride C, Gaupp D, Baddoo M, Kaminski N, et al. Mesenchymal stem cell engraftment in lung is enhanced in response to bleomycin exposure and ameliorates its fibrotic effects. Proc Natl Acad Sci USA. (2003) 100:8407–11. doi: 10.1073/pnas.1432929100
26. Rojas M, Xu J, Woods CR, Mora AL, Spears W, Roman J, et al. Bone marrow-derived mesenchymal stem cells in repair of the injured lung. Am J Respir Cell Mol Biol. (2005) 33:145–52. doi: 10.1165/rcmb.2004-0330OC
27. Kumamoto M, Nishiwaki T, Matsuo N, Kimura H, Matsushima K. Minimally cultured bone marrow mesenchymal stem cells ameliorate fibrotic lung injury. Eur Respir J. (2009) 34:740–8. doi: 10.1183/09031936.00128508
28. Zhao F, Zhang YF, Liu YG, Zhou JJ, Li ZK, Wu CG, et al. Therapeutic effects of bone marrow-derived mesenchymal stem cells engraftment on bleomycin-induced lung injury in rats. Transplant Proc. (2008) 40:1700–5. doi: 10.1016/j.transproceed.2008.01.080
29. Lee SH, Jang AS, Kim YE, Cha JY, Kim TH, Jung S, et al. Modulation of cytokine and nitric oxide by mesenchymal stem cell transfer in lung injury/fibrosis. Respir Res. (2010) 11:16. doi: 10.1186/1465-9921-11-16
30. Wu Y, Huang S, Enhe J, Ma K, Yang S, Sun T, et al. Bone marrow-derived mesenchymal stem cell attenuates skin fibrosis development in mice. Int Wound J. (2014) 11:701–10. doi: 10.1111/iwj.12034
31. Moodley Y, Atienza D, Manuelpillai U, Samuel CS, Tchongue J, Ilancheran S, et al. Human umbilical cord mesenchymal stem cells reduce fibrosis of bleomycin-induced lung injury. Am J Pathol. (2009) 175:303–13. doi: 10.2353/ajpath.2009.080629
32. Cargnoni A, Gibelli L, Tosini A, Signoroni PB, Nassuato C, Arienti D, et al. Transplantation of allogeneic and xenogeneic placenta-derived cells reduces bleomycin-induced lung fibrosis. Cell Transplant (2009) 18:405–22. doi: 10.3727/096368909788809857
33. Garcia O, Carraro G, Turcatel G, Hall M, Sedrakyan S, Roche T, et al. Amniotic fluid stem cells inhibit the progression of bleomycin-induced pulmonary fibrosis via CCL2 modulation in bronchoalveolar lavage. PLoS ONE (2013) 8:e71679. doi: 10.1371/journal.pone.0071679
34. Moodley Y, Vaghjiani V, Chan J, Baltic S, Ryan M, Tchongue J, et al. Anti-inflammatory effects of adult stem cells in sustained lung injury: a comparative study. PLoS ONE (2013) 8:e69299. doi: 10.1371/journal.pone.0069299
35. Lee SH, Lee EJ, Lee SY, Kim JH, Shim JJ, Shin C, et al. The effect of adipose stem cell therapy on pulmonary fibrosis induced by repetitive intratracheal bleomycin in mice. Exp Lung Res. (2014) 40:117–25. doi: 10.3109/01902148.2014.881930
36. Sabry MM, Elkalawy SA, Abo-Elnour RK, Abd-El-Maksod DF. Histolgical and immunohistochemical study on the effect of stem cell therapy on bleomycin induced pulmonary fibrosis in albino rat. Int J Stem Cells (2014) 7:33–42. doi: 10.15283/ijsc.2014.7.1.33
37. Braun RK, Koch JM, Hacker TA, Pegelow D, Kim J, Raval AN, et al. Cardiopulmonary and histological characterization of an acute rat lung injury model demonstrating safety of mesenchymal stromal cell infusion. Cytotherapy (2016) 18:536–45. doi: 10.1016/j.jcyt.2016.01.010
38. Uji M, Nakada A, Nakamura T, Hirata K. Effect of intratracheal administration of adipose-derived stromal cells on bleomycin-induced lung injury in a rat model. Osaka City Med J. (2015) 61:81–91. doi: 10.1183/13993003.congress-2015.PA943
39. Yu SH, Liu LJ, Lv B, Che CL, Fan DP, Wang LF, et al. Inhibition of bleomycin-induced pulmonary fibrosis by bone marrow-derived mesenchymal stem cells might be mediated by decreasing MMP9, TIMP-1, INF-gamma, and TGF-beta. Cell Biochem Funct. (2015) 33:356–66. doi: 10.1002/cbf.3118
40. Tashiro J, Elliot SJ, Gerth DJ, Xia X, Pereira-Simon S, Choi R, et al. Therapeutic benefits of young, but not old, adipose-derived mesenchymal stem cells in a chronic mouse model of bleomycin-induced pulmonary fibrosis. Transl Res. (2015) 166:554–67. doi: 10.1016/j.trsl.2015.09.004
41. Rubio GA, Elliot SJ, Wikramanayake TC, Xia X, Pereira-Simon S, Thaller SR, et al. Mesenchymal stromal cells prevent bleomycin-induced lung and skin fibrosis in aged mice and restore wound healing. J Cell Physiol. (2018) 233:5503–12. doi: 10.1002/jcp.26418
42. Servettaz A, Goulvestre C, Kavian N, Nicco C, Guilpain P, Chereau C, et al. Selective oxidation of DNA topoisomerase 1 induces systemic sclerosis in the mouse. J Immunol. (2009) 182:5855–64. doi: 10.4049/jimmunol.0803705
43. Maria AT, Toupet K, Bony C, Pirot N, Vozenin MC, Petit B, et al. Anti-fibrotic, anti-oxidant and immunomodulatory effects of mesenchymal stem cells in HOCl-induced systemic sclerosis. Arthritis Rheumatol. (2016) 68:1013–25. doi: 10.1002/art.39477
44. Maria AT, Toupet K, Maumus M, Fonteneau G, Le Quellec A, Jorgensen C, et al. Human adipose mesenchymal stem cells as potent anti-fibrosis therapy for systemic sclerosis. J Autoimmun. (2016) 70:31–9. doi: 10.1016/j.jaut.2016.03.013
45. Maria AT, Jorgensen C, Le Quellec A, Noel D, Guilpain P. Reply. Arthritis Rheumatol. (2016) 68:2348–50. doi: 10.1002/art.39756
46. Baxter RM, Crowell TP, McCrann ME, Frew EM, Gardner H. Analysis of the tight skin (Tsk1/+) mouse as a model for testing antifibrotic agents. Lab Invest. (2005) 85:1199–209. doi: 10.1038/labinvest.3700331
47. Chen C, Wang D, Moshaverinia A, Liu D, Kou X, Yu W, et al. Mesenchymal stem cell transplantation in tight-skin mice identifies miR-151-5p as a therapeutic target for systemic sclerosis. Cell Res. (2017) 27:559–77. doi: 10.1038/cr.2017.11
48. Huang K, Kang X, Wang X, Wu S, Xiao J, Li Z, et al. Conversion of bone marrow mesenchymal stem cells into type II alveolar epithelial cells reduces pulmonary fibrosis by decreasing oxidative stress in rats. Mol Med Rep. (2015) 11:1685–92. doi: 10.3892/mmr.2014.2981
49. Shen Q, Chen B, Xiao Z, Zhao L, Xu X, Wan X, et al. Paracrine factors from mesenchymal stem cells attenuate epithelial injury and lung fibrosis. Mol Med Rep. (2015) 11:2831–7. doi: 10.3892/mmr.2014.3092
50. Ortiz LA, Dutreil M, Fattman C, Pandey AC, Torres G, Go K, et al. Interleukin 1 receptor antagonist mediates the antiinflammatory and antifibrotic effect of mesenchymal stem cells during lung injury. Proc Natl Acad Sci USA. (2007) 104:11002–7. doi: 10.1073/pnas.0704421104
51. Cahill EF, Kennelly H, Carty F, Mahon BP, English K. Hepatocyte growth factor is required for mesenchymal stromal cell protection against bleomycin-induced pulmonary fibrosis. Stem Cells Transl Med. (2016) 5:1307–18. doi: 10.5966/sctm.2015-0337
52. Lan YW, Theng SM, Huang TT, Choo KB, Chen CM, Kuo HP, et al. Oncostatin M-preconditioned mesenchymal stem cells alleviate bleomycin-induced pulmonary fibrosis through paracrine effects of the hepatocyte growth factor. Stem Cells Transl Med. (2017) 6:1006–17. doi: 10.5966/sctm.2016-0054
53. Lan YW, Choo KB, Chen CM, Hung TH, Chen YB, Hsieh CH, et al. Hypoxia-preconditioned mesenchymal stem cells attenuate bleomycin-induced pulmonary fibrosis. Stem Cell Res Ther. (2015) 6:97. doi: 10.1186/s13287-015-0081-6
54. Wang Q, Zhu H, Zhou WG, Guo XC, Wu MJ, Xu ZY, et al. N-acetylcysteine-pretreated human embryonic mesenchymal stem cell administration protects against bleomycin-induced lung injury. Am J Med Sci. (2013) 346:113–22. doi: 10.1097/MAJ.0b013e318266e8d8
55. Huleihel L, Sellares J, Cardenes N, Alvarez D, Faner R, Sakamoto K, et al. Modified mesenchymal stem cells using miRNA transduction alter lung injury in a bleomycin model. Am J Physiol Lung Cell Mol Physiol. (2017) 313:L92–103. doi: 10.1152/ajplung.00323.2016
56. Min F, Gao F, Li Q, Liu Z. Therapeutic effect of human umbilical cord mesenchymal stem cells modified by angiotensin-converting enzyme 2 gene on bleomycin-induced lung fibrosis injury. Mol Med Rep. (2015) 11:2387–96. doi: 10.3892/mmr.2014.3025
57. Jiang M, Yu Y, Luo J, Gao Q, Zhang L, Wang Q, et al. Bone Marrow-derived mesenchymal stem cells expressing thioredoxin 1 attenuate bleomycin-induced skin fibrosis and oxidative stress in scleroderma. J Invest Dermatol. (2017) 137:1223–33. doi: 10.1016/j.jid.2017.01.011
58. Pittenger MF, Mackay AM, Beck SC, Jaiswal RK, Douglas R, Mosca JD, et al. Multilineage potential of adult human mesenchymal stem cells. Science (1999) 284:143–7. doi: 10.1126/science.284.5411.143
59. Maria AT, Maumus M, Le Quellec A, Jorgensen C, Noel D, Guilpain P. Adipose-derived mesenchymal stem cells in autoimmune disorders: state of the art and perspectives for systemic sclerosis. Clin Rev Allergy Immunol. (2017) 52:234–59. doi: 10.1007/s12016-016-8552-9
60. Peltzer J, Aletti M, Frescaline N, Busson E, Lataillade JJ, Martinaud C. Mesenchymal stromal cells based therapy in systemic sclerosis: rational and challenges. Front Immunol. (2018) 9:2013. doi: 10.3389/fimmu.2018.02013
61. Bocelli-Tyndall C, Bracci L, Spagnoli G, Braccini A, Bouchenaki M, Ceredig R, et al. Bone marrow mesenchymal stromal cells (BM-MSCs) from healthy donors and auto-immune disease patients reduce the proliferation of autologous- and allogeneic-stimulated lymphocytes in vitro. Rheumatology (2007) 46:403–8. doi: 10.1093/rheumatology/kel267
62. Larghero J, Farge D, Braccini A, Lecourt S, Scherberich A, Fois E, et al. Phenotypical and functional characteristics of in vitro expanded bone marrow mesenchymal stem cells from patients with systemic sclerosis. Ann Rheum Dis. (2008) 67:443–9. doi: 10.1136/ard.2007.071233
63. Scuderi N, Ceccarelli S, Onesti MG, Fioramonti P, Guidi C, Romano F, et al. Human adipose-derived stromal cells for cell-based therapies in the treatment of systemic sclerosis. Cell Transplant. (2013) 22:779–95. doi: 10.3727/096368912X639017
64. Capelli C, Zaccara E, Cipriani P, Di Benedetto P, Maglione W, Andracco R, et al. Phenotypical and functional characteristics of “in vitro” expanded adipose-derived mesenchymal stromal cells from patients with systemic sclerosis. Cell Transplant. (2017) 26:841–54.doi: 10.3727/096368917X694822
65. Orciani M, Svegliati S, Gorbi S, Spadoni T, Lazzarini R, Regoli F, et al. Alterations of ROS pathways in scleroderma begin at stem cell level. J Biol Regul Homeost Agents (2013) 27:211–24.
66. Fonteneau G, Bony C, Goulabchand R, Maria ATJ, Le Quellec A, Riviere S, et al. Serum-mediated oxidative stress from systemic sclerosis patients affects mesenchymal stem cell function. Front Immunol. (2017) 8:988. doi: 10.3389/fimmu.2017.00988
67. Vanneaux V, Farge-Bancel D, Lecourt S, Baraut J, Cras A, Jean-Louis F, et al. Expression of transforming growth factor beta receptor II in mesenchymal stem cells from systemic sclerosis patients. BMJ Open (2013) 3:e001890. doi: 10.1136/bmjopen-2012-001890
68. Del Papa N, Quirici N, Soligo D, Scavullo C, Cortiana M, Borsotti C, et al. Bone marrow endothelial progenitors are defective in systemic sclerosis. Arthritis Rheum. (2006) 54:2605–15. doi: 10.1002/art.22035
69. Cipriani P, Marrelli A, Benedetto PD, Liakouli V, Carubbi F, Ruscitti P, et al. Scleroderma mesenchymal stem cells display a different phenotype from healthy controls; implications for regenerative medicine. Angiogenesis (2013) 16:595–607. doi: 10.1007/s10456-013-9338-9
70. Cipriani P, Di Benedetto P, Liakouli V, Del Papa B, Di Padova M, Di Ianni M, et al. Mesenchymal stem cells (MSCs) from scleroderma patients (SSc) preserve their immunomodulatory properties although senescent and normally induce T regulatory cells (Tregs) with a functional phenotype: implications for cellular-based therapy. Clin Exp Immunol. (2013) 173:195–206. doi: 10.1111/cei.12111
71. Cipriani P, Di Benedetto P, Ruscitti P, Campese AF, Liakouli V, Carubbi F, et al. Impaired endothelium-mesenchymal stem cells cross-talk in systemic sclerosis: a link between vascular and fibrotic features. Arthritis Res Ther. (2014) 16:442. doi: 10.1186/s13075-014-0442-z
72. Hegner B, Schaub T, Catar R, Kusch A, Wagner P, Essin K, et al. Intrinsic deregulation of vascular smooth muscle and myofibroblast differentiation in mesenchymal stromal cells from patients with systemic sclerosis. PLoS ONE (2016) 11:e0153101. doi: 10.1371/journal.pone.0153101
73. Guiducci S, Manetti M, Romano E, Mazzanti B, Ceccarelli C, Dal Pozzo S, et al. Bone marrow-derived mesenchymal stem cells from early diffuse systemic sclerosis exhibit a paracrine machinery and stimulate angiogenesis in vitro. Ann Rheum Dis. (2011) 70:2011–21. doi: 10.1136/ard.2011.150607
74. Griffin M, Ryan CM, Pathan O, Abraham D, Denton CP, Butler PE. Characteristics of human adipose derived stem cells in scleroderma in comparison to sex and age matched normal controls: implications for regenerative medicine. Stem Cell Res Ther. (2017) 8:23. doi: 10.1186/s13287-016-0444-7
75. Bank J, Fuller SM, Henry GI, Zachary LS. Fat grafting to the hand in patients with Raynaud phenomenon: a novel therapeutic modality. Plast Reconstr Surg. (2014) 133:1109–18. doi: 10.1097/PRS.0000000000000104
76. Del Papa N, Di Luca G, Sambataro D, Zaccara E, Maglione W, Gabrielli A, et al. Regional implantation of autologous adipose tissue-derived cells induces a prompt healing of long-lasting indolent digital ulcers in patients with systemic sclerosis. Cell Transplant. (2015) 24:2297–305. doi: 10.3727/096368914X685636
77. Virzi F, Bianca P, Giammona A, Apuzzo T, Di Franco S, Mangiapane LR, et al. Combined platelet-rich plasma and lipofilling treatment provides great improvement in facial skin-induced lesion regeneration for scleroderma patients. Stem Cell Res Ther. (2017) 8:236. doi: 10.1186/s13287-017-0690-3
78. M Schendel M, Foll J, Muller LP, Keysser G, Behre G. Marked improvement of severe progressive systemic sclerosis after transplantation of mesenchymal stem cells from an allogeneic haploidentical-related donor mediated by ligation of CD137L. Leukemia (2008) 22:1062–4. doi: 10.1038/sj.leu.2404996
79. Keyszer G, Christopeit M, Fick S, Schendel M, Taute BM, Behre G, et al. Treatment of severe progressive systemic sclerosis with transplantation of mesenchymal stromal cells from allogeneic related donors: report of five cases. Arthritis Rheum. (2011) 63:2540–2. doi: 10.1002/art.30431
80. Onesti MG, Fioramonti P, Carella S, Fino P, Marchese C, Scuderi N. Improvement of mouth functional disability in systemic sclerosis patients over one year in a trial of fat transplantation versus adipose-derived stromal cells. Stem Cells Int. (2016) 2016:2416192. doi: 10.1155/2016/2416192
81. Guillaume-Jugnot P, Daumas A, Magalon J, Sautereau N, Veran J, Magalon G, et al. State of the art. Autologous fat graft and adipose tissue-derived stromal vascular fraction injection for hand therapy in systemic sclerosis patients. Curr Res Transl Med. (2016) 64:35–42. doi: 10.1016/j.retram.2016.01.006
82. Daumas A, Magalon J, Jouve E, Truillet R, Casanova D, Giraudo L, et al. Long-term follow-up after autologous adipose-derived stromal vascular fraction injection into fingers in systemic sclerosis patients. Curr Res Transl Med. (2017) 65:40–3. doi: 10.1016/j.retram.2016.10.006
83. van Rhijn-Brouwer FCC, Gremmels H, Fledderus JO, Schuurman AH, Bonte-Mineur F, Vonk MC, et al. A randomised placebo-controlled double-blind trial to assess the safety of intramuscular administration of allogeneic mesenchymal stromal cells for digital ulcers in systemic sclerosis: the MANUS Trial protocol. BMJ Open (2018) 8:e020479. doi: 10.1136/bmjopen-2017-020479
Keywords: mesenchymal stem cell, systemic sclerosis, allogenic, cell therapy, bleomycin, HOCl
Citation: Rozier P, Maria A, Goulabchand R, Jorgensen C, Guilpain P and Noël D (2018) Mesenchymal Stem Cells in Systemic Sclerosis: Allogenic or Autologous Approaches for Therapeutic Use? Front. Immunol. 9:2938. doi: 10.3389/fimmu.2018.02938
Received: 02 August 2018; Accepted: 29 November 2018;
Published: 14 December 2018.
Edited by:
John Isaacs, Newcastle University, United KingdomReviewed by:
Xinhua Yu, Forschungszentrum Borstel (LG), GermanyMasahide Tone, Cedars-Sinai Medical Center, United States
Copyright © 2018 Rozier, Maria, Goulabchand, Jorgensen, Guilpain and Noël. This is an open-access article distributed under the terms of the Creative Commons Attribution License (CC BY). The use, distribution or reproduction in other forums is permitted, provided the original author(s) and the copyright owner(s) are credited and that the original publication in this journal is cited, in accordance with accepted academic practice. No use, distribution or reproduction is permitted which does not comply with these terms.
*Correspondence: Danièle Noël, ZGFuaWVsZS5ub2VsQGluc2VybS5mcg==
†These authors have contributed equally to this work