- 1Peking University People's Hospital & Peking University Institute of Hematology, Beijing Key Laboratory of Hematopoietic Stem Cell Transplantation, Beijing, China
- 2Peking-Tsinghua Center for Life Sciences, Beijing, China
Allogeneic stem cell transplantation (allo-SCT) is a curable method for the treatment of hematological malignancies. In the past two decades, the establishment of haploidentical transplant modalities make “everyone has a donor” become a reality. However, graft-versus-host disease (GVHD) and relapse remain the major two causes of death either in the human leukocyte antigen (HLA)-matched transplant or haploidentical transplant settings, both of which restrict the improvement of transplant outcomes. Preclinical mice model showed that both donor-derived T cells and natural killer (NK) cells play important role in the pathogenesis of GVHD and the effects of graft-versus-leukemia (GVL). Hence, understanding the immune mechanisms of GVHD and GVL would provide potential strategies for the control of leukemia relapse without aggravating GVHD. The purpose of the current review is to summarize the biology of GVHD and GVL responses in preclinical models and to discuss potential novel therapeutic strategies to reduce the relapse rate after allo-SCT. We will also review the approaches, including optimal donor selection and, conditioning regimens, donor lymphocyte infusion, BCR/ABL-specific CTL, and chimeric antigen receptor-modified T cells, which have been successfully used in the clinic to enhance and preserve anti-leukemia activity, especially GVL effects, without aggravating GVHD or alleviate GVHD.
Introduction
Allogeneic hematopoietic stem cell transplantation (Allo-HSCT) remains a potentially curative therapeutic strategy for hematological malignancies (1–4). Currently, for patients who require transplantation, but have no related or unrelated donors with matching human-leukocyte antigen (HLA), haploidentical HSCT is an alternative modality, allowing everyone to have a donor (5, 6). Allo-HSCT benefits these malignancies due to a graft-versus-leukemia (GVL) effect that is mainly mediated by donor-derived alloreactive T cells and/or natural killer (NK) cells (7–12). However, T cells are also responsible for acute and/or chronic graft versus-host disease (GVHD), which leads to significant morbidity and mortality (13–15). Although the depletion of T cells from allografts alleviates GVHD either in human leukocyte antigen (HLA)-matched transplant settings or in HLA-haploidentical transplant modalities, removal of these cells results in increased graft failure and increased rates of leukemia relapse (16). Unfortunately, the immunosuppressive agents used for the prophylaxis and treatment of GVHD can also reduce the beneficial GVL effects. Therefore, the separation of GVL effects from GVHD remains the “holy grail” of allo-HSCT (17–20), which is urgently needed to allow a more effective therapy for hematological malignancies.
The challenge for the separation of GVL effects from GVHD is attributed to the underlying similarity of the alloreactive T responses between the two processes (4, 21, 22). In the past 20 years, great efforts have been made by researchers to elucidate specific distinguishing immune mechanisms of GVL vs. GVHD (23–25). In addition, a number of preclinical experiments have been performed to identify approaches that could be successfully used to separate GVL effects from GVHD (26–41). Clinically, a series of strategies, including donor selection, allograft engineering, adoptive immune cell infusion, and pharmacological agents have been established (42–44). More recently, the use of chimeric antigen receptor T (CAR-T) cells that target tumor cells with a limited capacity for GVHD induction, have been identified for enhancing GVL effects without aggravating GVHD (45–47). Previously, several reviews have been published related to the separation of GVL from GVHD (48–51) The present review briefly summarizes the underlying mechanisms related to GVHD and GVL effects, mainly focusing on recent advances in strategies for enhancing and preserving anti-leukemia activity without aggravating GVHD, especially approaches aimed at the separation of GVL effects from GVHD in preclinical mouse models and in the clinic.
Mechanisms Relevant to GVHD and GVL Effects
The pathophysiology of acute GVHD had been reviewed by several researchers (13–15), beginning with the activation of host antigen-presenting cells (APCs) by damage-associated molecular patterns and/or pathogen-associated molecular patterns expressed on damaged tissues. Activated host APCs then present host antigens to donor T cells, leading to alloactivation and inflammatory cytokine release, for example Interferon-γ (IFN-γ) and lipopolysaccharides (LPS). These inflammatory cytokines then recruit and induce the proliferation of additional immune effector cells, including Th1, Th2, Th17, neutrophils, and macrophages, which cause tissue injury and inflammation in a reaction that overwhelms any tolerance-promoting response from immune suppressor cells, such as regulatory T cells (Treg) (52), regulatory B cells (Breg) (22), mesenchymal stem cells (MSC) (25), and myeloid-derived suppressor cells (MDSC) (53).
The mechanisms underlying GVL are of interest (8, 54), as both T cells, natural killer (NK) cells, and cytokines, such as IFN-γ and tumor necrosis factor-α, possess anti-leukemia activity. Two molecular path ways, including perforin and Fas, are mainly used by T cell to mediate cytotoxicity. CD3+CD4+ T cells utilize the Fas pathway and CD3+CD8+ T cells use both, while NK cells employ the perforin pathway. Interestingly, all of these cells also play an antileukemia role via cytokine release. Recently, more attention has been focused on the role of γδT cells (55), and iNKT cells in GVL effects. The target antigens for alloreactive T cells include major histocompatibility complex (MHC) and multiplex immunohistochemistry (miHC), or leukemia-associated antigens (56). The importance of MHC and miHC antigens in GVL is underlined by the close association between GVHD and GVL, although selective miHC antigens are considered to be attractive targets for anti-leukemia immunotherapy. More recently, a number of studies have demonstrated that the overall balance between regulatory cells, including Treg, MDSC, and effector cells might be related to the extent of organ damage in GVHD settings and the effects of GVL in anti-leukemia settings (Figures 1–3) (21, 22, 25, 52, 53, 57).
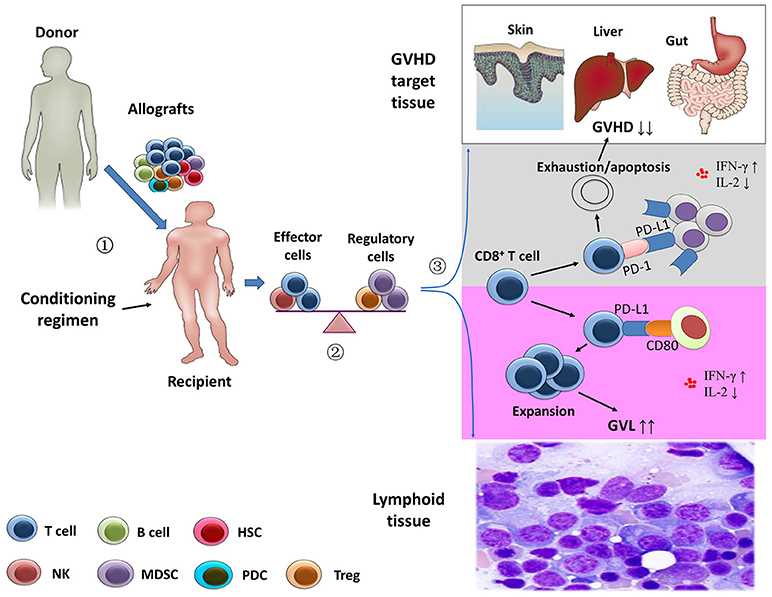
Figure 1. Separation of the graft-versus-leukemia effects from graft-versus-host disease. Stem cell harvests obtained from healthy donor were infused in the recipients after the conditioning regimen.
After transplantation, the balance between effector immune cells and regulatory immune cells might contribute to the prevention of graft-versus-host disease (GVHD) and the anti-leukemia activity.
Using animal models, Ni et al. (58) showed that the depletion of CD4+ T cells following allogeneic stem cell transplantation significantly increased systemic levels of interferon-γ and decreased interleukin-2. In GVHD-targeted tissues, CD4+ T cell depletion enhanced the interaction of PD-L1/PD-1 interactions between CD8+ T cells and cells of GVHD-targeted tissues, leading to exhaustion and apoptosis of host-attacking CD8+ T cells (gray area). However, the profiles of the cytokines might promote expansion of CD8+ T cells via PD-L1/CD80 interactions in lymphoid tissue, resulting in an enhanced anti-leukemia capacity (purple area) (21).
Strategies For Enhancing and Preserving Anti-Leukemia Effects Without Aggravating GVHD in Preclinical Models
Several strategies, such as the use of cytokines (59), selectively depletion of alloreactive T cells, regulatory immune cells (60, 61), and pharmacological agents, such as bortezomib and azacitidine (AZA), have been investigated to enhance and preserve the anti-leukemia effects without aggravating GVHD after allo-HSCT (Table 1) (31, 72, 73).
Cytokines
Granulocyte colony-stimulating factor (G-CSF) is widely used during transplantation to mobilize hemopoietic stem cells, which is also a mediator of T cell tolerance (74, 75). Using a murine leukemia model, several researchers have demonstrated that G-CSF mobilization of peripheral blood stem cell transplantation could maintain GVL effects through T cells via a perforin-dependent pathway and/or NKTs and prevent GVHD by reducing systemic levels of LPS and TNF-α as well as inducing a type 2 cytokine profile, CD34+ monocyte, and tolerogenic APCs (19, 76). Subsequent studies have shown that allografts mobilized by G-CSF analogs, such as pegylated G-CSF and progenipoietins (engineered chimeric G-CSF and Flt-3L protein), have marked tolerogenic properties that reside in the T cell and APC compartments. Additionally, mobilization with G-CSF analogs allows the concurrent enhancement of NKT cell numbers and activities, promoting host DC activation and subsequent CD8-dependent GVL effects while promoting the generation of Tregs to prevent CD4-dependent GVHD.
Except for G-CSF and its anlogs, other cytokines (77), including KGF, IL-11, IL-18 (28), IL-35 (62), and interleukin-12/23p40 (78), can also be used to separate GVL effects from GVHD in animal models. Moreover, the roles in GVHD of IL-21 and IL-22, two proinflammatory cytokines produced by Th17 cells, have been assessed in several studies (79–81). Couturier et al. (59) and Hanash et al. (79), respectively, demonstrated that IL-22 deficiency in donor T cells and abrogation of donor T-cell IL-21 signaling, could alleviate murine acute GVHD mortality while sparing the GVL effects. Hartung et al. (82) indicated that allografts mobilized by G-CSF plus stem cell factor exerted significantly enhanced antileukemic activity compared with those harvested after treatment with G-CSF alone, suggesting that a combination of different cytokines may be a better strategy for the separation of GVL effects from GVHD.
Depletion of Alloreactive Cells
To investigate the subsets of T cells that were effector cells with anti-leukemia effects without causing GVHD, a murine transplant model of chronic phase chronic myelogenous leukemia was generated. Zheng et al. (63) found that CD4+CD62L−CD44+CD25− effector memory T cells (CD4+ TEMs), but not naïve T cells (TN), unprimed to recipient cells mediated GVL without causing GVHD, because they retained key cytolytic functions but lacked other features that are pivotal for initiating GVHD. In another study, Chen et al. (83) reported that sorted CD45RB+CD62L+CD44+ central memory T cells (TCM, a mix of CD4+ and CD8+ cells) did not cause GVHD in a fully MHC-mismatched transplant mouse model. However, using the same model as Chen et al. (83), Zheng et al. (64) demonstrated that highly purified CD8+ TCM induced GVHD, albeit less severe than that induced by TN. However, CD8+ TCM also contribute to GVL.
More recently, using multiple GVHD models (two murine allogeneic HCT models and a human → mouse xenogeneic HCT model), Ni et al. (58) showed that CD4+ T cell depletion increased serum IFN-γ levels, leading to an upregulation of PD-L1 in recipient tissues and donor CD8+ T cells. In GVHD target tissues, they also found that increased PD-L1/PD-1 interactions between recipient tissues and donor CD8+ T cells led to T cell exhaustion and apoptosis, thereby preventing GVHD. In lymphoid tissues, enhanced PD-L1/CD80 interactions between CD8+ T cells augmented T cell survival and expansion and preserved the GVL response. In summary, the data reported by Ni et al. (58) suggested that the separation of GVL effects from GVHD could be ascribed to the PD-L1–mediated effect on CD8+ T cells depending on whether CD4+ T cells were present, the nature of the interacting partner expressed by CD8+ T cells, and the tissue microenvironment (Figure 1) (19, 21).
In the clinic, depletion of TN from stem cell allografts has been successfully used to reduce the incidence of chronic GVHD, while preserving the transfer of functional T cell memory (84). Overall, these results suggest that depletion of alloreactive T cells may represent a promising method to preserve GVL effects with decreasing or without causing GVHD.
Adoptive Transfer of Effective Immune Cells
Adoptive transfer of effective cells represents another strategy for the separation of GVL effects from GVHD. Olson et al. (85) demonstrated that donor T cells exhibited reduced proliferation, CD25 expression, and IFN-γ production in the presence of NK cells. In addition, activated NK cells mediated direct lysis of reisolated GVHD-inducing T cells in vitro, both of which lead to the alleviation of GVHD. In addition, the GVL effects were maintained in the presence of NK cells. Using mismatched hematopoietic transplant models, Ruggeri et al. (86) demonstrated, for the first time, that donor-versus-recipient NK cell alloreactivity could eliminate leukemia relapse by killing host lymphohematopoietic cells, and protect patients against GVHD by eliminating recipient-type APCs. The effects of NK cells in enhancing anti-leukemia activity and mitigating GVHD has also confirmed by other researchers. Ghosh et al. (87) reported that adoptively transferred donor-type unsorted TRAIL+ T cells could potentially enhance the curative potential of allo-HSCT by increasing the GVT response via fratricide of alloactivated T cells and suppressing GVHD through limiting alloreactive T cell expansion.
Recently, CAR T-cells have been shown to possess a novel adoptive immune therapy (40, 88). Both allogeneic and syngeneic CAR T cells show initial expansion as effector T cells. Jacoby et al. (88) found that, in a mouse model, CAR-mediated acute GVHD was only observed in the presence of leukemia, suggesting that CAR-target interactions induced GVHD. Additionally, Ghosh et al. (40) demonstrated that allogeneic donor CD19-specific CD28z CAR T cells could promote anti-lymphoma activity by non-alloreactive cells, which retained activity against CD19+ targets, with minimal GVHD by exhaustion and eventual deletion of the alloreactive CAR-T cells. They also reported that first-generation and 4-1BB-costimulated CARs increased GVHD. Overall, the data obtained from the mouse models suggest that CAR T cells could be used to enhance the anti-leukemia response, although its' effects on GVHD remain controversial.
Regulatory Immune Cells
Regulatory cell subsets, including Tregs, Bregs, MDSCs, and MSCs, may not only control immune homeostasis, but they also reduce detrimental T cell responses to foreign antigens. In 2003, Edinger et al. (52) observed that, in a mouse model, CD4+CD25+ Tregs could suppress the early expansion of alloreactive donor T cells, their IL-2-receptor alpha-chain expression and their capacity to induce GVHD without compomising their GVT effects, mediated primarily by the perforin lysis pathway of T conv cells. Interestingly, recipient-type specific Tregs could also control GVHD while favoring immune reconstitution and maintaining GVL effects. (89) In addition, Zheng et al. (90) reported that ex vivo-induced CD8hi Tregs controlled GVHD in an allospecific manner by reducing alloreactive T cell proliferation as well as decreasing inflammatory cytokine and chemokine secretion within target organs through a CTLA-4-dependent mechanism in humanized mice. Currently reported data in the literatures suggest that Tregs might be the most important regulatory cells in preventing GVHD (4) through a series of approaches, including aurora A/JAK2 inhibition (91, 92), selective TNFR2 activation (93), DR3 signaling modulation (94), activated protein C signals (95), and IL-2 (96), which can be used to alleviate GVHD through a Tregs-dependent mechanism.
MDSCs are a heterogeneous group of immature immunosuppressive cells of the myeloid lineage, which can induce immunosuppressive cells such as Tregs and skew macrophages toward a proinflammatory type 2 phenotype via IL-10 production. MDSCs can also suppress T-cells via arginase-1, NO, reactive oxygen species, heme oxygenase-1, TFG-β and IL-10, as well as promote Tregs. Highfill et al. (53) found that MDSCs generated in the presence of IL-13 could inhibit GVHD, migrate to sites of allopriming, and limit the activation and proliferation of donor T cells as well as induce a type 2 T cell response that was indispensable for GVHD prevention, but they did not diminish the GVL effect of donor T cells.
Another type of regulatory immune cells is the MSCs, which can inhibit the activation, proliferation, and function of T cells via arginase-1, NO, reactive oxygen species, chemokines, TGF-β, and IL-10. Interestingly, in vivo experiment have shown that MSCs are actively induced to undergo perforin-dependent apoptosis by recipient phagocytes that produced indoleamine 2,3-dioxygenase, which was essential to initiate MSC-induced immunosuppression (97). Directing the migration of MSCs by CCR7 from their broad battle field (inflammatory organs) to the modulatory center of the immune response could attenuate GVHD by exerting immunosuppressive effects on T cells, while preserving GVL effects by sparing the NK cell activity that contributes to GVL effects (25, 98).
Bregs can suppress immunopathology by prohibiting the expansion of pathogenic T cells and other pro-inflammatory lymphocytes through the production of IL-10, IL-35, and TGF-β (99). Our group showed that, in the acute GVHD mouse model, cotransplantation of Bregs prevented onset by inhibiting Th1 and Th17 differentiation and expanding regulatory T cells. In the GVL mouse model, Bregs contributed to the suppression of acute GVHD but had no adverse effects on GVL activity (22).
Excluding the abovementioned regulatory cells, group 2 innate lymphoid cells (ILC2) make up a large portion of the ILC population, which can polarize T cells to Th2 cells by secreting IL-4, and macrophages or DCs to an macrophage 2 or type 2 chemokine-secreting phenotype by secreting IL-13, respectively (100). ILC2 can alleviate GVHD by reducing donor Th1 and Th17 cells as well as accumulating MDSCs mediated by IL-13. Moreover, ILC2 do not inhibit the GVL response (101).
In summary, these preclinical studies suggest that cotransplantation or adoptive transfer of regulatory cells could be successfully used to alleviate GVHD without compromising the GVL effects. Therefore, pilot studies are warranted to evaluate the safety and feasibility of these regulatory cells in preventing and/or treating GVHD as well as preserving GVL effects in clinic.
Signaling Pathways
Several signaling pathways have been demonstrated to be correlated with T cell function. Janus kinases (JAKs) are intracellular signaling components of many type I/II cytokines (102, 103). There are 4 members of the JAK family that regulate the development and function of immune cells, including DCs, macrophages, T cells, B cells, and neutrophils, of which JAK1, JAK2, and JAK3 may be most relevant for the pathophysiology of GVHD (51). In murine models of GVHD and leukemia or lymphoma relapse, treatment with ruxolitinib reduced GVHD in the skin, liver, and gastrointestinal organs while preserving GVL activity, leading to improved survival (44, 104, 105). Betts et al. (91) found that the transfer of JAK2−−/−− donor T cells to allogeneic recipients led to attenuate GVHD by inhibiting Th1 differentiation, promoting Th2 polarization, and increasing and/or stabilizing CD8+ iTreg, yet it maintained GVL effects (106). In addition, pacritinib, a multikinase inhibitor with potent activity against JAK2, could significantly reduce GVHD and xenogeneic skin graft rejection in distinct rodent models and maintain donor anti-tumor immunity. Overall, these data suggest that JAK inhibition or other compounds, such as TG101348 (92), represents a new and potentially clinically relevant approach to separate GVL effects from GVHD.
Excluding JAKs, increasing data have demonstrated that targeting signaling pathways, such as the PKCα and PKCθ (66), MEK (68), NFAT (65), and IRE-1a/XBP-1 pathway (67), ikaros (107), toll-like receptor/myeloid differentiation factor 88 (108), DR3 signaling (94), and activated protein C signals (95), might provide strategies for alleviating GVHD, while enhancing or without compromising the GVL effects.
Pharmacological Agents
The roles played by biological agents in the separation of GVL effects from GVHD have been investigated in animal models (38, 71). Sun et al. (38) demonstrated that bortezomib might rapidly induce the preferential deletion of very high-affinity alloreactive T cells, thus allowing for expansion of the remaining T cells to maintain GVT responses yet with a reduced potential for promoting GVHD. Ehx et al. (71) found that AZA significantly decreased human T-cell proliferation as well as IFN-γ and TNF-α serum levels, and it reduced the expression of GRANZYME B and PERFORIN 1 by cytotoxic T cells, leading to the prevention of GVHD. AZA could also induce the expression of tumor antigens by AML cells, leading to the generation of donor-derived tumor specific cytotoxic T cells, which have been demonstrated to prevent AML relapse (70). In addition, Stokes et al. (69) reported that bendamustine could alleviate GVHD by enhancing MDSC suppressive function without compromising GVL effects.
Caballero-Velázquez et al. (30) showed that the combination of sirolimus and bortezomib synergistically inhibited both the activation and proliferation of stimulated T cells. Additionally, the production of Th1 cytokines (IFN γ, IL-2, and TNF-α) was significantly inhibited. This effect was due, at least in part, to the inhibition of Erk and Akt phosphorylation. In vivo, the combination reduced the risk of GVHD without hampering GVL effects, as shown in mice that received GVHD prophylaxis with sirolimus plus bortezomib infused with tumor WEHI cells plus C57BL/6 donor BM and splenocytes. Overall, this study suggests a synergistic effect of the combination different pharmacological agents to prevent GVHD while maintaining the GVL effect.
In summary, experiment results from mouse models suggest that effective and regulatory immune cells play a key role in separation of GVL effects from GVHD. The approaches explored in preclinical settings have demonstrated, for example, that cytokines or inhibitors targeting signaling pathways of T cells might enhance and/or preserve ant-leukemia effects without compromising GVHD through regulating the functions of effective and regulatory immune cells (Figures 2, 3).
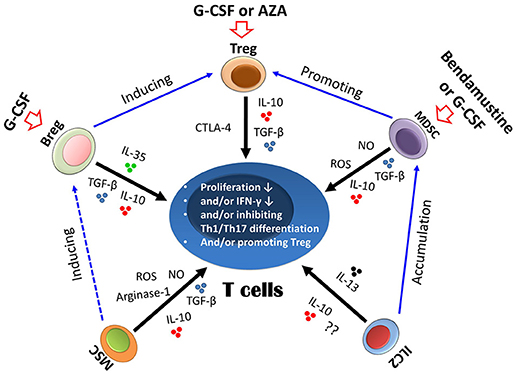
Figure 2. Suppressive mechanism of regulatory immune cells on T cells. Different regulatory cells could suppress T cells either via cytokines, such as IL-10 and TGF-β, or via other molecules, such as arginase-1 and reactive oxygen species (ROS) (indicated by black arrows). The biological interactions between different regulatory cells are indicated by blue arrows. Regulatory immune cells could also be induced by a number of approaches, such as granulocyte colony-stimulating factor (G-CSF), azacitidine (AZA), and bendamustine (indicated by red arrows). Treg, regulatory T cells; Bregs, regulatory B cells; MSCs, mesenchymal stem cells; MDSCs, myeloid-derived suppressor cells; ILC2, group 2 innate lymphoid cells.
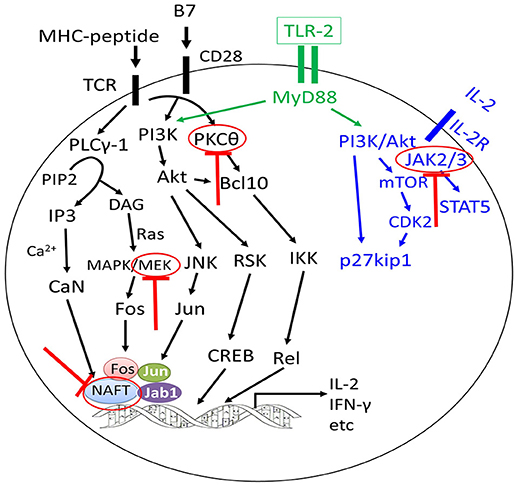
Figure 3. Approaches to separate GVL effects from GVHD using inhibitors targeting different signaling pathways of T cells. The graft-versus-leukemia effects could be enhanced or preserved by targeting different signaling pathways of T cells without aggravating graft-versus-host disease (GVHD) or with alleviation of GVHD (highlighted by red colors).
Strategies For Enhancing and Preserving Anti-Leukemia Effects Without Aggravating GVHD in the Clinic
Several approaches, including donor selection, conditioning regimens, graft engineering and adoptive transfusion of immune cells, have been successfully used in the clinic to separate GVL effects from GVHD before and after allo-HSCT (Figure 4).
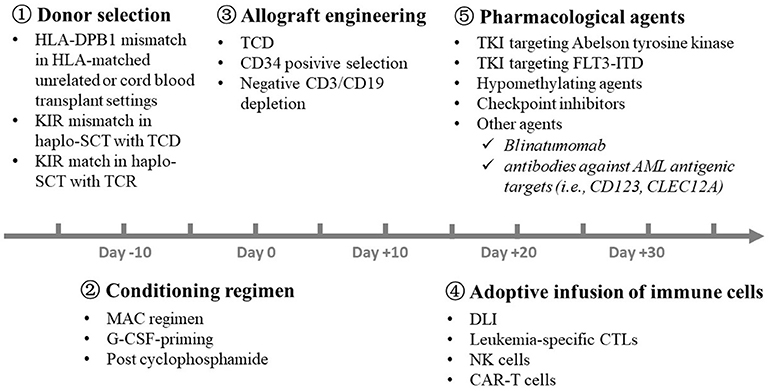
Figure 4. Strategies for the separation of GVL effects from GVHD in the clinic. A number of approaches, including donor selection,
conditioning regimen,
graft engineering,
adoptive transfusion of immune cells, and
pharmacological agents, have been successfully used in the clinic to separate GVL effects from GVHD at different time point before and after allo-HSCT. GVL, graft-versus-leukemia; GVHD, graft-versus-host disease; HLA, human leukocyte antigen; KIR, killer immunoglobulin-like receptor; SCT, stem cell transplantation; TCD, T cell depletion; TCR, T cell replete; MAC, myeloablative regimen; G-CSF, granulocyte colony-stimulating factor; DLI, donor lymphocyte infusion; NK, natural killer; CAR-T, chimeric antigen receptor T; TKI, Tyrosine kinase inhibitor; AML, acute myeloid leukemia.
Donor Selection
In unrelated donor transplantation settings, Kawase et al. (109) suggested that donor selection made in consideration of these results might allow the separation of GVL from acute GVHD in patients with AML, ALL, or those with chronic myeloid leukemia (CML), especially in HLA-DPB1 mismatch combinations. Fleischhauer et al. (110) further demonstrated that avoidance of an unrelated donor with a non-permissive T-cell-epitope mismatch at HLA-DPB1 might contribute to a lower risk of mortality. In cord blood transplant modality, HLA-DPB1 mismatch was also associated with a significant reduction of leukemia relapse (HR 0.61, P = 0.001), and no significant effect of HLA-DPB1 mismatch was observed on the risk of acute GVHD, engraftment or mortality (111). Laghmouchi et al. (112) suggested that the allo-HLA-DP-specific T cell repertoire contained T cells with restricted recognition of hematopoietic cells, which might contribute to specific GVL effector reactivity without coincident GVHD (112).
In T cell depleted haplo-SCT settings, Ruggeri et al. (86) showed that increased NK cell alloreactivity in humans, based on the “missing self” model, was associated with a decreased CIR and improved survival in patients with AML but not in patients with ALL. In contrast, Huang et al. (113) following the Beijing Protocol, demonstrated that host MHC class I could determine NK cell responses. The functional recovery of donor-derived NK cells was higher in recipients that expressed ligands for donor inhibitory KIRs, and a high functional NK recovery correlated with better relapse control (114). In haplo-SCT with PT/Cy settings, Shimoni et al. (115) also demonstrated a trend toward higher relapse rates in patients with KIR ligand mismatching (HR 1.36, P = 0.09) in a total group of 444 acute leukemia patients. This trend was observed in patients with AML (HR 1.48, P = 0.07) but not in those with ALL (HR 0.95, P = 0.88).
In summary, these data suggest that donor selection according to HLA-DPB1 mismatch, NK cell alloreactivity, and other variables (116–119), could represent a strategy for the separation of GVL effects and GVHD, although further studies are still needed.
Conditioning Regimen
More recently retrospective registry studies and some, but not all, prospective randomized trials have demonstrated increased relapse rates in recipients of an RIC compared with an MAC regimen in patients with AML and MDS who underwent allo-HSCT (120, 121). However, these finding remain controversial (3). In a multicenter randomized controlled trial (122), 178 HR-AML patients received haplo-HSCT with conditioning regimens involving recombinant human G-CSF or non-rhG-CSF. The cumulative incidences of acute GVHD, chronic GVHD, transplantation-related toxicity, and infectious complications appeared to be equivalent. The 2-year probabilities of LFS and OS in the G-CSF-priming and non-rhG-CSF-priming groups were 55.1 vs. 32.6% (P < 0.01) and 59.6 vs. 34.8% (P < 0.01), respectively. This study suggests that the G-CSF-priming conditioning regimen is an acceptable choice for HR-AML patients, which may lead to partially separation of GVL effects from GVHD.
Overall, considering the central importance of regimen in determinng leukemia relapse risk based on the biological characteristics of disease and pretransplantation minimal residual disease (MRD), there remains an urgent need for randomized comparisons of different conditioning regimens to separate GVL from GVHD.
Allograft Engineering
In a single-arm clinical trial, 35 cases patients with high-risk leukemia received naïve T cell-depleted G-PBSCs following a myeloablative conditioning regimen. GVHD prevention includes tacrolimus immunosuppression alone. Bleakley et al. (42) reported that all the cases engrafted. GVHD in these patients was universally corticosteroid responsive, although the incidence of aGVHD was not reduced. Chronic GVHD was remarkably infrequent (9%) compared with historical rates of ~50% with T cell-replete grafts. Memory T cells in the graft resulted in rapid T cell recovery and the transfer of protective virus-specific immunity. No excessive rates of infection or relapse occurred, and the OS was 78% at 2 years. These results suggest that the depletion of naïve T cells from allografts not only reduces the incidence of cGVHD but also preserves the transfer of functional T cell memory.
To decrease the incidence of GVHD in haploidentical allograft settings, the Perugia group established a protocol that includes TCD and a graft containing a mega-dose of highly purified CD34+ cells (average 10 × 106/kg body weight), which is administered following a myeloablative conditioning regimen (86). This protocol ensures a high engraftment rate, despite the HLA barrier, without triggering GVHD. However, the benefit (the absence of GVHD) from this CD34 selected haplotype transplant approach is offset by a very slow immune recovery due to the small number of T cells infused and the ATG application, which result in high rates of opportunistic infections, such as viral and fungal infections, leading to a high TRM. To accelerate immune recovery, the Perugia group demonstrated, for the first time, that the adoptive transfer of Tregs promotes lymphoid reconstitution and improves immunity to opportunistic pathogens without weakening the GVL effects in the TCD haploidentical setting (16). This finding suggests that the adoptive transfer of gene modified T cells and/or pathogen-specific T cells may be needed to improve clinical outcomes. In a phase II study, researchers from Germany found that haplo-SCT with a negative CD3/CD19 depletion and reduced intensity conditioning allowed for a successful transplantation in an older, heavily pretreated patient population (16). The estimated 2-event free survival was 25%. The incidence of grade II-IV aGVHD was 46%, and the incidence of cGVHD was 18%. Therefore, new strategies are needed to further establish novel strategies for the separation of GVL effects and GVHD.
Luznik et al. (123) summarized that in vivo cyclophosphamide posttransplantation (PT/Cy) could induce the destruction of peripheral, alloantigen-reactive T cells, while a relative resistance of donor Teff/memory T cells to PT/Cy, as demonstrated in mice, might contribute to the overall reconstitution of peripheral T-cell pools and immune competence over the long term. These results suggest that in vivo allograft engineering with PT/Cy represents a novel method for GVL and GVHD separation, and it has been widely used in haploidentical and HLA-matched sibling donor transplant settings (6, 124).
Adoptive Transfusion of Immune Cells
Currently, adoptive transfusion of immune cells, such as donor lymphocyte infusion (DLI), cytotoxic T lymphocyte (CTLs), NK cells, and CAR-T, had been successfully used to separate GVL effects from GVHD.
DLI
In 1990, Kolb et al. (125) first reported sustained remission after DLI in patients with CML who relapsed after allo-HSCT. Since then, DLI had become the mainstay allogeneic cellular therapy. The NCI recommendations list DLI as the routinely considered method for patients who relapsed after allo-HSCT and do not have GVHD (126). Based on immune tolerance induced by G-CSF, such as the ability to polarize T cells from the Th1 to the Th2 phenotype and the hyporesponsiveness of T cells, Huang's group established a modified DLI protocol (127) that includes the following: (i) the use of G-CSF mobilized peripheral blood stem cell harvests (G-PBSCs) instead of a steady lymphocyte infusion; (ii) the introduction of short-term immune suppressive agents, including cyclosporine A (CSA) or methotrexate (MTX), to further decrease the incidence of GVHD. Impressively, the feasibility and efficacy of the modified DLI were confirmed either for treatment or prevention of relapse after haploidentical HSCT (127). Our group also demonstrated that MRD-directed DLI could significantly decreased the relapse rate without aggravating GVHD (128). The use of DLI was also demonstrated in patients who underwent haploidentical HSCT with PT/Cy (129).
Recently, Nikiforow et al. (130) undertook a phase I study of DLI depleted of CD25+ T cells in 21 patients with hematologic malignancies who had relapsed after allo-HSCT. Two dose levels were administered: 1 × 107 (n = 6) and 3 × 107 CD3+ cells/kg (n = 15). A median 2.3 log-depletion of Tregs was achieved. Seven subjects (33%) developed clinically significant GVHD by 1 year, including one patient who died. At dose level 1, five subjects had progressive disease and one had stable disease. At dose level 2, nine subjects (60%) achieved or maintained responses (8 CR, 1 PR), including seven with active disease at the time of infusion. A shorter period between relapse and infusion was associated with the response at dose level 2 (P = 0.016). The 1-year survival rate was 53% among patients treated with dose level 2. Four of eight subjects with AML remained in remission at 1 year. When compared to unmodified DLI in 14 contemporaneous patients meeting study eligibility, CD25/Treg depletion was associated with a better response rate and improved EFS.
Overall, the available data suggest that DLI represents a widely used approach in prophylactic, pre-emptive therapy and therapy for relapse either in HLA-matched HSCT or in haploidentical transplant settings. Furthermore, CD25/Treg-depleted DLI appears to be feasible and capable of inducing GVL effects without excessive GVHD (131), although multicenter, prospective study are warranted to confirm the results.
Leukemia Specific CTLs
Researchers from Italy have investigated the feasibility of expanding/priming p190BCR-ABL–specific T cells in vitro by stimulation with DCs pulsed with p190BCR-ABL peptides derived from the BCR-ABL junctional region and alternative splicing, and of adoptively administering them to patients with relapsed disease (132). Three patients were enrolled in this study. Patient 1 was a 61-year-old man experiencing a second molecular recurrence after matched unrelated donor (MUD) alloHSCT and unmanipulated DLIs. Patient 2 was a 30-year-old man diagnosed with Ph+ ALLwith hyperleukocytosis and central nervous system (CNS) involvement, experiencing his third hematologic relapse (BM blast 66%, F317L mutation) after MUD-HSCT, DLI, and rescue therapy with nilotinib. Patient 3 was a 62-year-old woman diagnosed with Ph+ ALL with CNS involvement, showing persistent molecular disease (last MRD before T-cell therapy 0.1% BCR-ABL/ABL) after induction, maintenance chemotherapy, and prolonged TKI treatment. She was not eligible for alloHSCT due to comorbidities. The results showed no postinfusion toxicity, except for a grade II skin GVHD in the patient who was treated for hematologic relapse. All patients achieved a molecular or hematologic CR after T-cell therapy, upon emergence of p190BCR-ABL-specific T cells in the BM. These results demonstrate that p190BCR-ABL-specific CTLs are capable of controlling treatment-refractory Ph+ ALL in vivo, and they support the development of adoptive immunotherapeutic approaches with BCR-ABL CTLs in Ph+ ALL. Therefore, further studies including large sample sizes are needed to confirm the abovementioned results.
Excuding BCR-ABL CTLs, the anti-leukemia effects of WT1 specific CTL were also observed in 11 relapsed or high-risk leukemia patients who underwent allo-HSCT (133). Chapuis et al. (133) found that CD8+ transferred T cells with a memory phenotype could be detected after long-term follow-up. An approach to generate multi-TAA-specific CTLs using peptide libraries of 15-mer peptides overlapping by 11 amino acids spanning the whole amino acid sequence of a target antigen was developed by Weber et al. (134) They also showed that TAAmix-specific CTLs could inhibit the colony formation of leukemia blasts. In summary, leukemia-specific CTLs might be a promising method for enhancing anti-leukemia activity.
NK Cells
The role played by NK cells in anti-leukemia activity had been fully investigated in allo-HSCT settings. In a dose-escalation study, Choi et al. (43) showed that, when given 2–3 weeks after haploidentical HSCT, donor-derived NK cells were well-tolerated at a median total dose of 2.0 × 108 cells/kg. In a phase I study, the safety of haploidentical third-party NK cell infusion was further confirmed in 21 patients with high-risk myeloid malignancies who received a preparative regimen with busulfan and fludarabine followed by infusion of IL-2-activated NK cells with a dose ranging from 0.02 to 8.32 × 106/kg. Lee et al. (135) demonstrated that five patients were alive, and 5 and 11 cases had died from transplant-related causes and relapse, respectively. Among the total patients, only 5 cases developed a maximum acute GVHD of grade 2, and 2 cases grade 3 GVHD. These results indicated that the infusion of third-party NK cells was well-tolerated and did not increase the rate of GVHD after allo-HSCT. Ciurea et al. (136) initiated a phase 1 dose-escalation study of membrane-bound interleukin 21-expanded donor NK cells infused before and after haploidentical HSCT for high-risk myeloid malignancies. NK cells were infused on days −2, +7, and +28 posttransplant. All NK expansions achieved the required cell number, and 11 of 13 patients enrolled received all 3 planned NK-cell doses (1 × 105/kg to 1 × 108/kg per dose). No infusional reactions or dose-limiting toxicities occurred. All patients were engrafted with donor cells. Seven patients (54%) developed grade I-II acute GVHD (aGVHD), and no patients developed grade III-IV aGVHD or chronic GVHD. All other patients were alive and in remission at the last follow-up (median, 14.7 months). Overall, this trial demonstrated the production feasibility and safety of infusing high doses of ex vivo-expanded NK cells after haploidentical HSCT without adverse effects, increased GVHD, or higher mortality, which was associated with significantly improved NK-cell numbers and function, fewer viral infections, and a low relapse rate posttransplant. Further study deomonstrated that CD56+ donor cell infusion after PT/Cy and short-course cyclosporine were feasible with prompt engraftment, rapid reconstitution of CD4+ T, Tregs and NK cells and a reduced incidence of relapse and acute GVHD (137).
CAR-T Cells
Researchers from Peking University described six ALL patients with no response to modified DLI who received one and two infusions of CAR T cells from haplo-HSCT donors. Five patients (83.33%) achieved MRD-negative remission; one patient was discharged without evaluation after developing severe thrombotic microangiopathies (46, 47, 138). More recently, Anwer et al. (45) performed a systemic review, including 72 patients from seven studies who were treated with donor-derived CAR T cells. The authors reported that the use of donor-derived CAR T cell for relapse prophylaxis, MRD clearance or salvage from relapse is therefore highly effective, and the risk of GVHD flare is very low.
In summary, donor-derived CAR T-cell infusion seems to be an effective and safe alternative method for relapsed B-ALL after haplo-HSCT (47). Therefore, with the definition of multiple antigen targets, such as CD7, CD38. CD138, FLT-3, and B-cell maturation antigen, CAR-T cell could be increasingly used for anti-hematological malignancies.
Pharmacological Agents
Tyrosine Kinase Inhibitor (TKI) Targeting Abelson Tyrosine Kinase
Currently, few patients with CML will receive allo-HSCT. Therefore, the use of TKI after transplantation mainly focuses on cases with Ph-positive ALL (139–141). Chen et al. (142) reported that 14 patients who were positive for BCR-ABL1 expression, received imatinib therapy after allo-HSCT. Eight patients became BCR-ABL1-negative at 1 month after imatinib therapy, and only two patients died from hematological relapse. In the nonimatinib-treated group, six of 20 patients relapsed, and five of these patients died from hematological relapse. Here, recommendations for the use of TKIs according to the pre- and post-transplant MRD status by the Acute Leukemia Working Party of the European Society for Blood and Marrow Transplantation are provided as follows (143).
First, for cases with positive pre-MRD, but negative posttransplantation MRD (post-MRD), prophylactic TKI should be administered according to the pretransplantation mutation status, or observation only. If positive post-MRD is detected, imatinib or another TKI can be administered according to the mutation status. If MRD reoccurs within 3 months after transplantation or at a high level, a 2nd generation TKI should be given.
Second, for cases with positive post-MRD not considering the status of pre-MRD, TKI is administered according to mutation status or using 2nd generation TKI.
Third, for cases with both negative pre-MRD and negative post-MRD, prophylactic TKI or observation, if positive post-MRD is detected, imatinib or another TKI can be administered according to the mutation status. If MRD reoccurrs within 3 months after transplantation or at a high level, a 2nd generation TKI should be given.
TKIs Targeting FLT3-ITD
A number of FLT3 TKIs have been or are being investigated in allo-HSCT settings for FLT3-ITD AML, including sorafenib (144, 145), midostaurin, quizartinib, crenolanib, and gilteritinib (144–149). The mechanism of action of TKIs targeting FLT3 may not only involved in direct tumor cell killing, but also in increased interleukin-15, leadings to an increase in CD8+CD107a+IFN-γ+ T cells with features of longevity (high levels of Bcl-2 and reduced PD-1 levels), which could eradicate leukemia in secondary recipients (146). More recently, Xuan et al. (147) performed a study that enrolled a total of 144 patients with FLT3-ITD AML undergoing allo-HSCT. Depending on whether they were receiving sorafenib before transplantation or sorafenib maintenance after transplantation, patients were divided into 4 groups: patients receiving sorafenib before transplantation (group A; n = 36), patients receiving sorafenib after transplantation (group B; n = 32), patients receiving sorafenib both before and after transplantation (group C; n = 26), and patients receiving sorafenib neither before nor after transplantation (group D; n = 50). Xuan et al. (147) showed that the 3-year relapse rates were 22.2, 18.8, 15.8, and 46.1% for groups A, B, C, and D, respectively (P = 0.006). The 3-year LFS rates were 69.4, 78.1, 80.4, and 34.8%, respectively (P < 0.001). A multivariate analysis revealed that sorafenib before transplantation, sorafenib maintenance after transplantation, and their combined application were protective factors for a lower relapse rate and longer LFS, respectively.
More recent studies have shown that targeting the FLT3-ITD driver mutation with a highly potent and selective FLT3 inhibitor, such as quizartinib, is a promising clinical strategy to help improve clinical outcomes in patients with relapsed or refractory AML (148, 149). Therefore, further studies are needed to investigate the effectiveness of these agents in allo-HSCT settings, especially for the separation of GVL from GVHD.
Hypomethylating Agents
Hypomethylating agents are used as treatments for relapse and may also be used in pre-emptive interventions after allo-HSCT (150–154). In a phase 1 study enrolling 27 patients with AML post allo-HSCT. Goodyear et al. (155) showed that azacitidine (AZA) both augmented the expansion of regulatory T cells and induced cytotoxic CD8+ T-cell responses to several tumor antigens, and leading to hopes that it might facilitate successful cultivation of the GVL response without inducing significant GVHD. In a multicenter retrospective study, Craddock et al. (156) investigated the tolerability and activity of AZA in 181 patients who relapsed after an allograft for AML (n = 116) or MDS (n = 65). Sixty-nine patients received additional DLI. Forty-six of 157 (25%) assessable patients responded to AZA therapy: 24 (15%) achieved a CR and 22 a PR. In patients who achieved a CR, the 2-year overall survival was 48 vs. 12% for the whole population. The authors suggested that AZA represents an important new therapy in select patients with AML/MDS who relapse after allo-HSCT, thus warranting prospective studies. Moreover, the combination of sorafenib, AZA, and DLI represent a novel direction for the treatment or prevention of relapse without aggravating GVHD after allo-HSCT (151, 157).
Recently, Schroeder et al. (158) retrospectively analyzed data obtained for 36 patients with hematological (n = 35) or molecular relapse (n = 1) of AML (n = 29), or MDS (n = 7). Decitabine (DAC) was the first salvage therapy in 16 patients (44%), whereas 20 patients (56%) had previously received 1–5 lines of salvage therapy, including 16 cases who had been treated with AZA. In 22 patients (61%), a median of 2 DLI per patient (range, 1–5) was administered in addition to DAC. As a result, the overall response rate was 25%, including 6 CR (17%) and 3 PR (8%). Three patients within the first-line group achieved CR, while 3 patients receiving DAC as second-line treatment reached CR, including 2 patients with previous AZA failure. The median duration of CR was 10 months (range, 2–33), and none of the patients have relapsed to date. The incidence of acute and chronic GVHD was 19 and 5% (158). These data suggest that DAC may be an alternative to AZA or even a second choice after AZA failure. In summary, hypomethylating agents used alone or in combination with DLI might represent promising approaches for the separation of GVL from GVHD in the clinic.
Checkpoint Inhibitors
The relapse of hematological malignancies after allo-HSCT can be mediated by high levels of checkpoint receptors, including PD-1 and CTLA-4, on donor derived effective T cells and high expression of cognate ligands on residual leukemia cells (159, 160). In a phase 1 study, Bashey et al. (161). showed that a single dose of ipilimumab (between 0.1 and 3.0 mg/kg) for patients with malignancies who relapsed after allo-HSCT did not seem to cause clinically significant GVHD and achieved responses in 3 patients with lymphoid malignancies. In a subsequent phase 1/2 study, ipilimumab was started at 3 mg/kg but could be escalated to 10 mg/kg (162). Although no objective responses were observed in six patients who received ipilimumab treatment at 3 mg/kg, a total of 13 patients presented a decrease in tumor burden among 22 patients treated at a dose of 10 mg/kg, with four responses persisting for >1 year. Impressively, four patients with extramedullary AML and one patient with smoldering MDS that developed into AML had a complete response. These data suggest a particular sensitivity of AML to ipilimumab treatment after allo-HSCT. Davids et al. (162) also observed that responders showed a reduction of CD4+ regulatory T cells with an increase in conventional T cells in peripheral blood as well as an increase in CD62L− effector memory T cells.
A phase 2 investigator-initiated trial enrolled patients with lymphoid malignancies who relapsed after allogeneic HSCT (n = 10) and high-risk patients after autologous HSCT (n = 7) (163). Both cases received 10 mg of oral lenalidomide daily for 21 days followed by intravenous ipilimumab at 3 mg/kg body weight. The regimen was repeated 4 weeks later for a total of 4 treatments. Khouri et al. (163) demonstrated that 4 of 10 patients in the allogeneic group had complete responses and 3 partial responses. The disease in 6 of 7 patients in the autologous group remains in remission. The authors suggested that the responses might be related to a 2- to 3 -fold increases in inducible ICOS+CD4+FoxP3− T cells number.
In summary, checkpoint inhibitor used alone or in combination with other methods, such as immunomodulatory agents (163) and CAR-T cells (164), could be promising approaches for the treatment or prevention of relapse after transplantation without aggravating GVHD, although further studies are warranted for confirmation.
Other Agents
Several other novel agents (165), including histone deacetylase inhibitor (panobinostat), and monoclonal antibodies, such as blinatumomab (a novel bispecific CD19-directed CD3 T-cell engager), as well as antibodies against AML antigenic targets (i.e., CD123, CLEC12A), have been or are currently being investigated for the prevention and treatment of relapse in patients with hematological malignancies who have undergone allo-HSCT. Therefore, further prospective studies are warranted to select optimal methods that are currently available for killing leukemia cells without leading to GVHD.
Future Directions
In the past two decades, increasing evidence supports the notion that GVL effects could be, at least partially, separated from GVHD both in animal models and in the clinic. Recently, Fanning et al. (18) have demonstrated that Vβ spectratyping can identify T cells involved in antihost and antitumor reactivity and that tumor presensitization can aid in the separation of GVHD and GVL responses. However, no studies have demonstrated the successful use of this technique for separating GVL effects from GVHD in patients who have undergone allo-HSCT. In addition, several other questions remain to be answered in the future. First, although preclinical experiments have demonstrated the feasibility of a number of strategies for enhancing or preserving anti-leukemia activity without compromising GVHD, planned prospective studies are required to evaluate the clinical efficacy and to move these approaches from preclinical research to the standard-of care. Second, it remains uncertain whether the available methods for inducing anti-leukemia activity without causing GVHD can be successfully used in different transplant modalities, especially haploidentical allografts. Third, little is known about the immunological mechanisms underlying the separation of GVL effects from GVHD. Therefore, further studies are imperative.
In summary, with the elucidation of the immune mechanisms of both GVL effects and GVHD, the advances in the establishment of novel approaches for the prevention and/or treatment of leukemia relapse and GVHD, as well as the evaluation of these new methods based on prospective clinical trials, an increasing number of patients will benefit from the successful separation of GVL effects from GVHD, ultimately leading to superior survival.
Author Contributions
X-JH designed the study. Y-JC and X-YZ collected data and drafted the manuscript. All authors contributed to data interpretation, manuscript preparation, and approval of the final version.
Funding
This work was supported by the National Key Research and Development Program of China (no. 2017YFA0104500), the Foundation for Innovative Research Groups of the National Natural Science Foundation of China (81621001), and the Beijing Natural Science Foundation (7162196).
Conflict of Interest Statement
The authors declare that the research was conducted in the absence of any commercial or financial relationships that could be construed as a potential conflict of interest.
Acknowledgments
We would also like to thank American journal experts (https://www.aje.com/) for assistance in editing this manuscript.
References
1. Copelan EA. Hematopoietic stem-cell transplantation. N Engl J Med. (2006) 354:1813–26. doi: 10.1056/NEJMra052638
2. Wang Y, Liu QF, Xu LP, Liu KY, Zhang XH, Ma X, et al. Haploidentical vs identical-sibling transplant for AML in remission: a multicenter, prospective study. Blood (2015) 125:3956–62. doi: 10.1182/blood-2015-02-627786
3. Fasslrinner F, Schetelig J, Burchert A, Kramer M, Trenschel R, Hegenbart U, et al. Long-term efficacy of reduced-intensity versus myeloablative conditioning before allogeneic haemopoietic cell transplantation in patients with acute myeloid leukaemia in first complete remission: retrospective follow-up of an open-label, randomised phase 3 trial. Lancet Haematol. (2018) 5:e161–9. doi: 10.1016/S2352-3026(18)30022-X
4. Blazar BR, MacDonald KPA, Hill GR. Immune regulatory cell infusion for graft-versus-host disease prevention and therapy. Blood (2018) 131:2651–60. doi: 10.1182/blood-2017-11-785865
5. Chang YJ, Huang XJ. Haploidentical stem cell transplantation: anti-thymocyte globulin-based experience. Semin Hematol. (2016) 53:82–9. doi: 10.1053/j.seminhematol.2016.01.004
6. Kanakry CG, Fuchs EJ, Luznik L. Modern approaches to HLA-haploidentical blood or marrow transplantation. Nat Rev Clin Oncol. (2016) 13:10–24. doi: 10.1038/nrclinonc.2015.128
7. Negrin RS. Graft-versus-host disease versus graft-versus-leukemia. Hematology Am Soc Hematol Educ Program (2015) 2015:225–30. doi: 10.1182/asheducation-2015.1.225
8. Bleakley M, Riddell SR. Molecules and mechanisms of the graft-versus-leukaemia effect. Nat Rev Cancer (2004) 4:371–80. doi: 10.1038/nrc1365
9. Dickinson AM, Norden J, Li S, Hromadnikova I, Schmid C, Schmetzer H, et al. Graft-versus-Leukemia effect following hematopoietic stem cell transplantation for leukemia. Front Immunol. (2017) 8:496. doi: 10.3389/fimmu.2017.00496
10. Falkenburg JHF, Jedema I. Graft versus tumor effects and why people relapse. Hematology Am Soc Hematol Educ Program (2017) 2017:693–8. doi: 10.1182/asheducation-2017.1.693
11. Locatelli F, Pende D, Falco M, Della Chiesa M, Moretta A, Moretta L. NK cells mediate a crucial graft-versus-leukemia effect in Haploidentical-HSCT to cure high-risk acute leukemia. Trends Immunol. (2018) 39:577–90. doi: 10.1016/j.it.2018.04.009
12. Cooley S, Parham P, Miller JS. Strategies to activate NK cells to prevent relapse and induce remission following hematopoietic stem cell transplantation. Blood (2018) 131:1053–62. doi: 10.1182/blood-2017-08-752170
13. Ferrara JL, Levine JE, Reddy P, Holler E. Graft-versus-host disease. Lancet (2009) 373:1550–61. doi: 10.1016/S0140-6736(09)60237-3
14. Zeiser R, Blazar BR. Acute graft-versus-host disease - biologic process, prevention, and therapy. N Engl J Med. (2017) 377:2167–79. doi: 10.1056/NEJMra1609337
15. Holtan SG, Pasquini M, Weisdorf DJ. Acute graft-versus-host disease: a bench-to-bedside update. Blood (2014) 124:363–73. doi: 10.1182/blood-2014-01-514786
16. Martelli MF, Di Ianni M, Ruggeri L, Pierini A, Falzetti F, Carotti A, et al. “Designed” grafts for HLA-haploidentical stem cell transplantation. Blood (2014) 123:967–73. doi: 10.1182/blood-2013-10-531764
17. Li J, Heinrichs J, Haarberg K, Semple K, Veerapathran A, Liu C, et al. HY-specific induced regulatory T cells display high specificity and efficacy in the prevention of acute graft-versus-host disease. J Immunol. (2015) 195:717–25. doi: 10.4049/jimmunol.1401250
18. Fanning SL, Zilberberg J, Stein J, Vazzana K, Berger SA, Korngold R, et al. Unraveling graft-versus-host disease and graft-versus-leukemia responses using TCR Vβ spectratype analysis in a murine bone marrow transplantation model. J Immunol. (2013) 190:447–57. doi: 10.4049/jimmunol.1201641
19. Morris ES, MacDonald KP, Rowe V, Banovic T, Kuns RD, Don AL, et al. NKT cell-dependent leukemia eradication following stem cell mobilization with potent G-CSF analogs. J Clin Invest. (2005) 115:3093–103. doi: 10.1172/JCI25249
20. Song Y, Hu B, Liu Y, Jin Z, Zhang Y, Lin D, et al. IL-12/IL-18-preactivated donor NK cells enhance GVL effects and mitigate GvHD after allogeneic hematopoietic stem cell transplantation. Eur J Immunol. (2018) 48:670–82. doi: 10.1002/eji.201747177
21. Brennan TV, Yang Y. PD-L1 serves as a double agent in separating GVL from GVHD. J Clin Invest. (2017) 127:1627–30. doi: 10.1172/JCI94196
22. Hu Y, He GL, Zhao XY, Zhao XS, Wang Y, Xu LP, et al. Regulatory B cells promote graft-versus-host disease prevention and maintain graft-versus-leukemia activity following allogeneic bone marrow transplantation. Oncoimmunology (2017) 6:e1284721. doi: 10.1080/2162402X.2017.1284721
23. Okiyama N, Furumoto Y, Villarroel VA, Linton JT, Tsai WL, Gutermuth J, et al. Reversal of CD8 T-cell-mediated mucocutaneous graft-versus-host-like disease by the JAK inhibitor tofacitinib. J Invest Dermatol. (2014) 134:992–1000. doi: 10.1038/jid.2013.476
24. Banovic T, MacDonald KP, Markey KA, Morris ES, Kuns RD, Varelias A, et al. Donor treatment with a multipegylated G-CSF maximizes graft-versus-leukemia effects. Biol Blood Marrow Transplant. (2009) 15:126–30. doi: 10.1016/j.bbmt.2008.11.019
25. Li H, Jiang Y, Jiang X, Guo X, Ning H, Li Y, et al. CCR7 guides migration of mesenchymal stem cell to secondary lymphoid organs: a novel approach to separate GvHD from GvL effect. Stem Cells (2014) 32:1890–903. doi: 10.1002/stem.1656
26. Montagna D, Daudt L, Locatelli F, Montini E, Turin I, Lisini D, et al. Single-cell cloning of human, donor-derived antileukemia T-cell lines for in vitro separation of graft-versus-leukemia effect from graft-versus-host reaction. Cancer Res (2006) 66:7310–6. doi: 10.1158/0008-5472.CAN-06-0591
27. Coghill JM, Carlson MJ, Panoskaltsis-Mortari A, West ML, Burgents JE, Blazar BR, et al. Separation of graft-versus-host disease from graft-versus-leukemia responses by targeting CC-chemokine receptor 7 on donor T cells. Blood (2010) 115:4914–22. doi: 10.1182/blood-2009-08-239848
28. Reddy P, Teshima T, Hildebrandt G, Williams DL, Liu C, Cooke KR, et al. Pretreatment of donors with interleukin-18 attenuates acute graft-versus-host disease via STAT6 and preserves graft-versus-leukemia effects. Blood (2003) 101:2877–85. doi: 10.1182/blood-2002-08-2566
29. Long J, Chang L, Shen Y, Gao WH, Wu YN, Dou HB, et al. Valproic acid ameliorates graft-versus-host disease by downregulating Th1 and Th17 cells. J Immunol. (2015) 195:1849–57. doi: 10.4049/jimmunol.1500578
30. Caballero-Velázquez T, Sánchez-Abarca LI, Gutierrez-Cosio S, Blanco B, Calderon C, Herrero C, et al. The novel combination of sirolimus and bortezomib prevents graft-versus-host disease but maintains the graft-versus-leukemia effect after allogeneic transplantation. Haematologica (2012) 97:1329–37. doi: 10.3324/haematol.2011.058677
31. Wu Y, Heinrichs J, Bastian D, Fu J, Nguyen H, Schutt S, et al. MicroRNA-17-92 controls T-cell responses in graft-versus-host disease and leukemia relapse in mice. Blood (2015) 126:1314–23. doi: 10.1182/blood-2015-02-627356
32. Krijanovski OI, Hill GR, Cooke KR, Teshima T, Crawford JM, Brinson YS, et al. Keratinocyte growth factor separates graft-versus-leukemia effects from graft-versus-host disease. Blood (1999) 94:825–31.
33. Zeng Y, Stokes J, Hahn S, Hoffman E, Katsanis E. Activated MHC-mismatched T helper-1 lymphocyte infusion enhances GvL with limited GvHD. Bone Marrow Transplant. (2014) 49:1076–83. doi: 10.1038/bmt.2014.91
34. Fricke S, Hilger N, Fricke C, Schönfelder U, Behre G, Ruschpler P, et al. Prevention of graft-versus-host-disease with preserved graft-versus-leukemia-effect by ex vivo and In vivo modulation of CD4(+) T-cells. Cell Mol Life Sci. (2014) 71:2135–48. doi: 10.1007/s00018-013-1476-0
35. Marcondes AM, Karoopongse E, Lesnikova M, Margineantu D, Welte T, Dinarello CA, et al. α-1-Antitrypsin (AAT)-modified donor cells suppress GVHD but enhance the GVL effect: a role for mitochondrial bioenergetics. Blood (2014) 124:2881–91. doi: 10.1182/blood-2014-04-570440
36. Lu Y, Giver CR, Sharma A, Li JM, Darlak KA, Owens LM, et al. IFN-γ and indoleamine 2,3-dioxygenase signaling between donor dendritic cells and T cells regulates graft versus host and graft versus leukemia activity. Blood (2012) 119:1075–85. doi: 10.1182/blood-2010-12-322891
37. Wang H, Asavaroengchai W, Yeap BY, Wang MG, Wang S, Sykes M, et al. Paradoxical effects of IFN-gamma in graft-versus-host disease reflect promotion of lymphohematopoietic graft-versus-host reactions and inhibition of epithelial tissue injury. Blood (2009) 113:3612–9. doi: 10.1182/blood-2008-07-168419
38. Sun K, Welniak LA, Panoskaltsis-Mortari A, O'Shaughnessy MJ, Liu H, Barao I, et al. Inhibition of acute graft-versus-host disease with retention of graft-versus-tumor effects by the proteasome inhibitor bortezomib. Proc Natl Acad Sci USA. (2004) 101:8120–5. doi: 10.1073/pnas.0401563101
39. Karimi MA, Bryson JL, Richman LP, Fesnak AD, Leichner TM, Satake A, et al. NKG2D expression by CD8+ T cells contributes to GVHD and GVT effects in a murine model of allogeneic HSCT. Blood (2015) 125:3655–63. doi: 10.1182/blood-2015-02-629006
40. Ghosh A, Smith M, James SE, Davila ML, Velardi E, Argyropoulos KV, et al. Donor CD19 CAR T cells exert potent graft-versus-lymphoma activity with diminished graft-versus-host activity. Nat Med. (2017) 23:242–9. doi: 10.1038/nm.4258
41. Teshima T, Hill GR, Pan L, Brinson YS, van den Brink MR, Cooke KR, et al. IL-11 separates graft-versus-leukemia effects from graft-versus-host disease after bone marrow transplantation. J Clin Invest. (1999) 104:317–25. doi: 10.1172/JCI7111
42. Bleakley M, Heimfeld S, Loeb KR, Jones LA, Chaney C, Seropian S, et al. Outcomes of acute leukemia patients transplanted with naive T cell-depleted stem cell grafts. J Clin Invest. (2015) 125:2677–89. doi: 10.1172/JCI81229
43. Choi I, Yoon SR, Park SY, Kim H, Jung SJ, Jang YJ, et al. Donor-derived natural killer cells infused after human leukocyte antigen-haploidentical hematopoietic cell transplantation: a dose-escalation study. Biol Blood Marrow Transplant. (2014) 20:696–704. doi: 10.1016/j.bbmt.2014.01.031
44. Choi J, Cooper ML, Staser K, Ashami K, Vij KR, Wang B, et al. Baricitinib-induced blockade of interferon gamma receptor and interleukin-6 receptor for the prevention and treatment of graft-versus-host disease. Leukemia (2018) 32:2483–94. doi: 10.1038/s41375-018-0123-z
45. Anwer F, Shaukat AA, Zahid U, Husnain M, McBride A, Persky D, et al. Donor origin CAR T cells: graft versus malignancy effect without GVHD, a systematic review. Immunotherapy (2017) 9:123–30. doi: 10.2217/imt-2016-0127
46. Kochenderfer JN, Dudley ME, Carpenter RO, Kassim SH, Rose JJ, Telford WG, et al. Donor-derived CD19-targeted T cells cause regression of malignancy persisting after allogeneic hematopoietic stem cell transplantation. Blood (2013) 122:4129–39. doi: 10.1182/blood-2013-08-519413
47. Park JH, Rivière I, Gonen M, Wang X, Sénéchal B, Curran KJ, et al. Long-term follow-up of CD19 CAR therapy in acute lymphoblastic leukemia. N Engl J Med. (2018) 378:449–59. doi: 10.1056/NEJMoa1709919
48. Morris ES, MacDonald KP, Hill GR. Stem cell mobilization with G-CSF analogs: a rational approach to separate GVHD and GVL? Blood (2006) 107:3430–5. doi: 10.1182/blood-2005-10-4299
49. Stenger EO, Turnquist HR, Mapara MY, Thomson AW. Dendritic cells and regulation of graft-versus-host disease and graft-versus-leukemia activity. Blood (2012) 119:5088–103. doi: 10.1182/blood-2011-11-364091
50. Warren EH, Deeg HJ. Dissecting graft-versus-leukemia from graft-versus-host-disease using novel strategies. Tissue Antigens (2013) 81:183–93. doi: 10.1111/tan.12090
51. Schroeder MA, Choi J, Staser K, DiPersio JF. The role of janus kinase signaling in graft-versus-host disease and graft versus leukemia. Biol Blood Marrow Transplant. (2018) 24:1125–34. doi: 10.1016/j.bbmt.2017.12.797
52. Edinger M, Hoffmann P, Ermann J, Drago K, Fathman CG, Strober S, et al. CD4+CD25+ regulatory T cells preserve graft-versus-tumor activity while inhibiting graft-versus-host disease after bone marrow transplantation. Nat Med. (2003) 9:1144–50. doi: 10.1038/nm915
53. Highfill SL, Rodriguez PC, Zhou Q, Goetz CA, Koehn BH, Veenstra R, et al. Bone marrow myeloid-derived suppressor cells (MDSCs) inhibit graft-versus-host disease (GVHD) via an arginase-1-dependent mechanism that is up-regulated by interleukin-13. Blood (2010) 116:5738–47. doi: 10.1182/blood-2010-06-287839
54. Mavroudis D, Barrett J. The graft-versus-leukemia effect. Curr Opin Hematol. (1996) 3:423–9. doi: 10.1097/00062752-199603060-00005
55. Handgretinger R, Schilbach K. The potential role of gammadelta T cells after allogeneic HCT for leukemia. Blood (2018) 131:1063–72. doi: 10.1182/blood-2017-08-752162
56. Zilberberg J, Feinman R, Korngold R. Strategies for the identification of T cell-recognized tumor antigens in hematological malignancies for improved graft-versus-tumor responses after allogeneic blood and marrow transplantation. Biol Blood Marrow Transplant. (2015) 21:1000–7. doi: 10.1016/j.bbmt.2014.11.001
57. Heinrichs J, Li J, Nguyen H, Wu Y, Bastian D, Daethanasanmak A, et al. CD8(+) Tregs promote GVHD prevention and overcome the impaired GVL effect mediated by CD4(+) Tregs in mice. Oncoimmunology (2016) 5:e1146842. doi: 10.1080/2162402X.2016.1146842
58. Ni X, Song Q, Cassady K, Deng R, Jin H, Zhang M, et al. PD-L1 interacts with CD80 to regulate graft-versus-leukemia activity of donor CD8+ T cells. J Clin Invest. (2017) 127:1960–77. doi: 10.1172/JCI91138
59. Couturier M, Lamarthée B, Arbez J, Renauld JC, Bossard C, Malard F, et al. IL-22 deficiency in donor T cells attenuates murine acute graft-versus-host disease mortality while sparing the graft-versus-leukemia effect. Leukemia (2013) 27:1527–37. doi: 10.1038/leu.2013.39
60. Darlak KA, Wang Y, Li JM, Harris WA, Owens LM, Waller EK. Enrichment of IL-12-producing plasmacytoid dendritic cells in donor bone marrow grafts enhances graft-versus-leukemia activity in allogeneic hematopoietic stem cell transplantation. Biol Blood Marrow Transplant. (2013) 19:1331–9. doi: 10.1016/j.bbmt.2013.06.016
61. Sato K, Yamashita N, Yamashita N, Baba M, Matsuyama T. Regulatory dendritic cells protect mice from murine acute graft-versus-host disease and leukemia relapse. Immunity (2003) 18:367–79. doi: 10.1016/S1074-7613(03)00055-4
62. Liu Y, Wu Y, Wang Y, Cai Y, Hu B, Bao G, et al. IL-35 mitigates murine acute graft-versus-host disease with retention of graft-versus-leukemia effects. Leukemia (2015) 29:939–46. doi: 10.1038/leu.2014.310
63. Zheng H, Matte-Martone C, Li H, Anderson BE, Venketesan S, Sheng Tan H, et al. Effector memory CD4+ T cells mediate graft-versus-leukemia without inducing graft-versus-host disease. Blood (2008) 111:2476–84. doi: 10.1182/blood-2007-08-109678
64. Zheng H, Matte-Martone C, Jain D, McNiff J, Shlomchik WD. Central memory CD8+ T cells induce graft-versus-host disease and mediate graft-versus-leukemia. J Immunol. (2009) 182:5938–48. doi: 10.4049/jimmunol.0802212
65. Vaeth M, Bäuerlein CA, Pusch T, Findeis J, Chopra M, Mottok A, et al. Selective NFAT targeting in T cells ameliorates GvHD while maintaining antitumor activity. Proc Natl Acad Sci USA. (2015) 112:1125–30. doi: 10.1073/pnas.1409290112
66. Haarberg KM, Li J, Heinrichs J, Wang D, Liu C, Bronk CC, et al. Pharmacologic inhibition of PKCalpha and PKCtheta prevents GVHD while preserving GVL activity in mice. Blood (2013) 122:2500–11. doi: 10.1182/blood-2012-12-471938
67. Schutt SD, Wu Y, Tang CH, Bastian D, Nguyen H, Sofi MH, et al. Inhibition of the IRE-1α/XBP-1 pathway prevents chronic GVHD and preserves the GVL effect in mice. Blood Adv. (2018) 2:414–27. doi: 10.1182/bloodadvances.2017009068
68. Itamura H, Shindo T, Tawara I, Kubota Y, Kariya R, Okada S, et al. The MEK inhibitor trametinib separates murine graft-versus-host disease from graft-versus-tumor effects. JCI Insight (2016) 1:e86331. doi: 10.1172/jci.insight.86331
69. Stokes J, Hoffman EA, Zeng Y, Larmonier N, Katsanis E. Post-transplant bendamustine reduces GvHD while preserving GvL in experimental haploidentical bone marrow transplantation. Br J Haematol. (2016) 174:102–16. doi: 10.1111/bjh.14034
70. Choi J, Ritchey J, Prior JL, Holt M, Shannon WD, Deych E, et al. In vivo administration of hypomethylating agents mitigate graft-versus-host disease without sacrificing graft-versus-leukemia. Blood (2010) 116:129–39. doi: 10.1182/blood-2009-12-257253
71. Ehx G, Fransolet G, de Leval L, D'Hondt S, Lucas S, Hannon M, et al. Azacytidine prevents experimental xenogeneic graft-versus-host disease without abrogating graft-versus-leukemia effects. Oncoimmunology (2017) 6:e1314425. doi: 10.1080/2162402X.2017.1314425
72. Ghosh A, Holland AM, Dogan Y, Yim NL, Rao UK, Young LF, et al. PLZF confers effector functions to donor T cells that preserve graft-versus-tumor effects while attenuating GVHD. Cancer Res. (2013) 73:4687–96. doi: 10.1158/0008-5472.CAN-12-4699
73. Wu Y, Schutt S, Paz K, Zhang M, Flynn RP, Bastian D, et al. MicroRNA-17-92 is required for T-cell and B-cell pathogenicity in chronic graft-versus-host disease in mice. Blood (2018) 131:1974–86. doi: 10.1182/blood-2017-06-789321
74. Rutella S, Zavala F, Danese S, Kared H, Leone G. Granulocyte colony-stimulating factor: a novel mediator of T cell tolerance. J Immunol. (2005) 175:7085–91. doi: 10.4049/jimmunol.175.11.7085
75. Perobelli SM, Mercadante AC, Galvani RG, Gonçalves-Silva T, Alves AP, Pereira-Neves A, et al. G-CSF-induced suppressor IL-10+ neutrophils promote regulatory T cells that inhibit graft-versus-host disease in a long-lasting and specific way. J Immunol. (2016) 197:3725–34. doi: 10.4049/jimmunol.1502023
76. Pan L, Teshima T, Hill GR, Bungard D, Brinson YS, Reddy VS, et al. Granulocyte colony-stimulating factor-mobilized allogeneic stem cell transplantation maintains graft-versus-leukemia effects through a perforin-dependent pathway while preventing graft-versus-host disease. Blood (1999) 93:4071–8.
77. Wang H, Yang YG. The complex and central role of interferon-γ in graft-versus-host disease and graft-versus-tumor activity. Immunol Rev. (2014) 258:30–44. doi: 10.1111/imr.12151
78. Wu Y, Bastian D, Schutt S, Nguyen H, Fu J, Heinrichs J, et al. Essential role of interleukin-12/23p40 in the development of graft-versus-host disease in mice. Biol Blood Marrow Transplant. (2015) 21:1195–204. doi: 10.1016/j.bbmt.2015.03.016
79. Hanash AM, Kappel LW, Yim NL, Nejat RA, Goldberg GL, Smith OM, et al. Abrogation of donor T-cell IL-21 signaling leads to tissue-specific modulation of immunity and separation of GVHD from GVL. Blood (2011) 118:446–55. doi: 10.1182/blood-2010-07-294785
80. Pan B, Xia F, Wu Y, Zhang F, Lu Z, Fu R, et al. Recipient-derived IL-22 alleviates murine acute graft-versus-host disease in association with reduced activation of antigen presenting cells. Cytokine (2018) 111:33–40. doi: 10.1016/j.cyto.2018.08.010
81. Hanash AM, Dudakov JA, Hua G, O'Connor MH, Young LF, Singer NV, et al. Interleukin-22 protects intestinal stem cells from immune-mediated tissue damage and regulates sensitivity to graft versus host disease. Immunity (2012) 37:339–50. doi: 10.1016/j.immuni.2012.05.028
82. Hartung G, Uharek L, Zeis M, Glass B, Steinmann J, Dreger P, et al. Superior antileukemic activity of murine peripheral blood progenitor cell (PBPC) grafts mobilized by G-CSF and stem cell factor (SCF) as compared to G-CSF alone. Bone Marrow Transplant. (1998) 21(Suppl. 3):S16–20.
83. Chen BJ, Deoliveira D, Cui X, Le NT, Son J, Whitesides JF, et al. Inability of memory T cells to induce graft-versus-host disease is a result of an abortive alloresponse. Blood (2007) 109:3115–23. doi: 10.1182/blood-2006-04-016410
84. Bleakley M, Turtle CJ, Riddell SR. Augmentation of anti-tumor immunity by adoptive T-cell transfer after allogeneic hematopoietic stem cell transplantation. Expert Rev Hematol. (2014) 5:409–25. doi: 10.1586/ehm.12.28
85. Olson JA, Leveson-Gower DB, Gill S, Baker J, Beilhack A, Negrin RS. NK cells mediate reduction of GVHD by inhibiting activated, alloreactive T cells while retaining GVT effects. Blood (2010) 115:4293–301. doi: 10.1182/blood-2009-05-222190
86. Ruggeri L, Capanni M, Urbani E, Perruccio K, Shlomchik WD, Tosti A, et al. Effectiveness of donor natural killer cell alloreactivity in mismatched hematopoietic transplants. Science (2002) 295:2097–100. doi: 10.1126/science.1068440
87. Ghosh A, Dogan Y, Moroz M, Holland AM, Yim NL, Rao UK, et al. Adoptively transferred TRAIL+ T cells suppress GVHD and augment antitumor activity. J Clin Invest. (2013) 123:2654–62. doi: 10.1172/JCI66301
88. Jacoby E, Yang Y, Qin H, Chien CD, Kochenderfer JN, Fry TJ. Murine allogeneic CD19 CAR T cells harbor potent antileukemic activity but have the potential to mediate lethal GVHD. Blood (2016) 127:1361–70. doi: 10.1182/blood-2015-08-664250
89. Trenado A, Charlotte F, Fisson S, Yagello M, Klatzmann D, Salomon BL, et al. Recipient-type specific CD4+CD25+ regulatory T cells favor immune reconstitution and control graft-versus-host disease while maintaining graft-versus-leukemia. J Clin Invest. (2003) 112:1688–96. doi: 10.1172/JCI17702
90. Zheng J, Liu Y, Liu Y, Liu M, Xiang Z, Lam KT, et al. Human CD8+ regulatory T cells inhibit GVHD and preserve general immunity in humanized mice. Sci Transl Med. (2013) 5:168ra9. doi: 10.1126/scitranslmed.3004943
91. Betts BC, Bastian D, Iamsawat S, Nguyen H, Heinrichs JL, Wu Y, et al. Targeting JAK2 reduces GVHD and xenograft rejection through regulation of T cell differentiation. Proc Natl Acad Sci USA. (2018) 115:1582–7. doi: 10.1073/pnas.1712452115
92. Betts BC, Veerapathran A, Pidala J, Yang H, Horna P, Walton K, et al. Targeting Aurora kinase A and JAK2 prevents GVHD while maintaining Treg and antitumor CTL function. Sci Transl Med. (2017) 9:eaai8269. doi: 10.1126/scitranslmed.aai8269
93. Chopra M, Biehl M, Steinfatt T, Brandl A, Kums J, Amich J, et al. Exogenous TNFR2 activation protects from acute GvHD via host T reg cell expansion. J Exp Med. (2016) 213:1881–900. doi: 10.1084/jem.20151563
94. Nishikii H, Kim BS, Yokoyama Y, Chen Y, Baker J, Pierini A, et al. DR3 signaling modulates the function of Foxp3+ regulatory T cells and the severity of acute graft-versus-host disease. Blood (2016) 128:2846–58. doi: 10.1182/blood-2016-06-723783
95. Ranjan S, Goihl A, Kohli S, Gadi I, Pierau M, Shahzad K, et al. Activated protein C protects from GvHD via PAR2/PAR3 signalling in regulatory T-cells. Nat Commun. (2017) 8:311. doi: 10.1038/s41467-017-00169-4
96. Koreth J, Matsuoka K, Kim HT, McDonough SM, Bindra B, Alyea EP, et al. Interleukin-2 and regulatory T cells in graft-versus-host disease. N Engl J Med. (2011) 365:2055–66. doi: 10.1056/NEJMoa1108188
97. Galleu A, Riffo-Vasquez Y, Trento C, Lomas C, Dolcetti L, Cheung TS, et al. Apoptosis in mesenchymal stromal cells induces In vivo recipient-mediated immunomodulation. Sci Transl Med. (2017) 9:eaam7828. doi: 10.1126/scitranslmed.aam7828
98. Auletta JJ, Eid SK, Wuttisarnwattana P, Silva I, Metheny L, Keller MD, et al. Human mesenchymal stromal cells attenuate graft-versus-host disease and maintain graft-versus-leukemia activity following experimental allogeneic bone marrow transplantation. Stem Cells (2015) 33:601–14. doi: 10.1002/stem.1867
99. Rosser EC, Mauri C. Regulatory B cells: origin, phenotype, and function. Immunity (2015) 42:607–12. doi: 10.1016/j.immuni.2015.04.005
100. Colonna M. Innate lymphoid cells: diversity, plasticity, and unique functions in immunity. Immunity (2018) 48:1104–17. doi: 10.1016/j.immuni.2018.05.013
101. Bruce DW, Stefanski HE, Vincent BG, Dant TA, Reisdorf S, Bommiasamy H, et al. Type 2 innate lymphoid cells treat and prevent acute gastrointestinal graft-versus-host disease. J Clin Invest. (2017) 127:1813–25. doi: 10.1172/JCI91816
102. MacDonald KP, Blazar BR, Hill GR. Cytokine mediators of chronic graft-versus-host disease. J Clin Invest. (2017) 127:2452–63. doi: 10.1172/JCI90593
103. Schwartz DM, Bonelli M, Gadina M, O'Shea JJ. Type I/II cytokines, JAKs, and new strategies for treating autoimmune diseases. Nat Rev Rheumatol. (2016) 12:25–36. doi: 10.1038/nrrheum.2015.167
104. Carniti C, Gimondi S, Vendramin A, Recordati C, Confalonieri D, Bermema A, et al. Pharmacologic inhibition of JAK1/JAK2 signaling reduces experimental murine acute GVHD while preserving GVT effects. Clin Cancer Res. (2015) 21:3740–9. doi: 10.1158/1078-0432.CCR-14-2758
105. Choi J, Cooper ML, Alahmari B, Ritchey J, Collins L, Holt M, et al. Pharmacologic blockade of JAK1/JAK2 reduces GvHD and preserves the graft-versus-leukemia effect. PLoS ONE (2014) 9:e109799. doi: 10.1371/journal.pone.0109799
106. Iamsawat S, Daenthanasanmak A, Voss JH, Nguyen H, Bastian D, Liu C, et al. Stabilization of Foxp3 by targeting JAK2 enhances efficacy of CD8 induced regulatory T cells in the prevention of graft-versus-host disease. J Immunol. (2018) 201:2812–23. doi: 10.4049/jimmunol.1800793
107. Toubai T, Guoqing H, Rossi C, Mathewson N, Oravecz-Wilson K, Cummings E, et al. Ikaros deficiency in host hematopoietic cells separates GVL from GVHD after experimental allogeneic hematopoietic cell transplantation. Oncoimmunology (2015) 4:e1016699. doi: 10.1080/2162402X.2015.1016699
108. Lim JY, Ryu DB, Lee SE, Park G, Choi EY, Min CK. Differential effect of MyD88 signal in donor T cells on graft-versus-leukemia effect and graft-versus-host disease after experimental allogeneic stem cell transplantation. Mol Cells (2015) 38:966–74. doi: 10.14348/molcells.2015.0158
109. Kawase T, Matsuo K, Kashiwase K, Inoko H, Saji H, Ogawa S, et al. HLA mismatch combinations associated with decreased risk of relapse: implications for the molecular mechanism. Blood (2009) 113:2851–8. doi: 10.1182/blood-2008-08-171934
110. Fleischhauer K, Shaw BE, Gooley T, Malkki M, Bardy P, Bignon JD, et al. Effect of T-cell-epitope matching at HLA-DPB1 in recipients of unrelated-donor haemopoietic-cell transplantation: a retrospective study. Lancet Oncol. (2012) 13:366–74. doi: 10.1016/S1470-2045(12)70004-9
111. Yabe T, Azuma F, Kashiwase K, Matsumoto K, Orihara T, Yabe H, et al. HLA-DPB1 mismatch induces a graft-versus-leukemia effect without severe acute GVHD after single-unit umbilical cord blood transplantation. Leukemia (2018) 32:168–75. doi: 10.1038/leu.2017.202
112. Laghmouchi A, Hoogstraten C, Van Balen P, Falkenburg JHF, Jedema I. The allo-HLA-DP restricted T cell repertoire provoked by allogeneic dendritic cells contains T cells that show restricted recognition of hematopoietic cells including primary malignant cells. Haematologica (2018). doi: 10.3324/haematol.2018.193680. [Epub ahead of print].
113. Huang XJ, Zhao XY, Liu DH, Liu KY, Xu LP. Deleterious effects of KIR ligand incompatibility on clinical outcomes in haploidentical hematopoietic stem cell transplantation without in vitro T-cell depletion. Leukemia (2007) 21:848–51. doi: 10.1038/sj.leu.2404566
114. Zhao XY, Chang YJ, Zhao XS, Xu LP, Zhang XH, Liu KY, et al. Recipient expression of ligands for donor inhibitory KIRs enhances NK-cell function to control leukemic relapse after haploidentical transplantation. Eur J Immunol. (2015) 45:2396–408. doi: 10.1002/eji.201445057
115. Shimoni A, Labopin M, Lorentino F, Van Lint MT, Koc Y, Gülbas Z, et al. Killer cell immunoglobulin-like receptor ligand mismatching and outcome after haploidentical transplantation with post-transplant cyclophosphamide. Leukemia (2018). doi: 10.1038/s41375-018-0170-5. [Epub ahead of print].
116. Chang YJ, Luznik L, Fuchs EJ, Huang XJ. How do we choose the best donor for T-cell-replete, HLA-haploidentical transplantation? J Hematol Oncol. (2016) 9:35. doi: 10.1186/s13045-016-0265-2
117. Escudero A, Martínez-Romera I, Fernández L, Valentín J, González-Vicent M, Vicario JL, et al. Donor KIR genotype impacts on clinical outcome after T cell-depleted HLA matched related allogeneic transplantation for high-risk pediatric leukemia patients. Biol Blood Marrow Transplant. (2018) 24:2493–500. doi: 10.1016/j.bbmt.2018.08.009
118. Fleischhauer K, Hsu KC, Shaw BE. Prevention of relapse after allogeneic hematopoietic cell transplantation by donor and cell source selection. Bone Marrow Transplant. (2018) 53:1498–507. doi: 10.1038/s41409-018-0218-1
119. Widman A, Reshef R. Precision in donor selection: identifying ideal stem-cell donors through their T cells. Exp Hematol. (2016) 44:1020–3. doi: 10.1016/j.exphem.2016.07.013
120. Kröger N, Iacobelli S, Franke GN, Platzbecker U, Uddin R, Hübel K, et al. Dose-reduced versus standard conditioning followed by allogeneic stem-cell transplantation for patients with myelodysplastic syndrome: a prospective randomized phase III study of the EBMT (RICMAC Trial). J Clin Oncol. (2017) 35:2157–64. doi: 10.1200/JCO.2016.70.7349
121. Scott BL, Pasquini MC, Logan BR, Wu J, Devine SM, Porter DL, et al. Myeloablative versus reduced-intensity hematopoietic cell transplantation for acute myeloid leukemia and myelodysplastic syndromes. J Clin Oncol. (2017) 35:1154–61. doi: 10.1200/JCO.2016.70.7091
122. Gao L, Wen Q, Chen X, Liu Y, Zhang C, Gao L, et al. Effects of priming with recombinant human granulocyte colony-stimulating factor on conditioning regimen for high-risk acute myeloid leukemia patients undergoing human leukocyte antigen-haploidentical hematopoietic stem cell transplantation: a multicenter randomized controlled study in southwest China. Biol Blood Marrow Transplant. (2014) 20:1932–9. doi: 10.1016/j.bbmt.2014.08.001
123. Luznik L, O'Donnell PV, Fuchs EJ. Post-transplantation cyclophosphamide for tolerance induction in HLA-haploidentical bone marrow transplantation. Semin Oncol. (2012) 39:683–93. doi: 10.1053/j.seminoncol.2012.09.005
124. Romee R, Cooley S, Berrien-Elliott MM, Westervelt P, Verneris MR, Wagner JE, et al. First-in-human phase 1 clinical study of the IL-15 superagonist complex ALT-803 to treat relapse after transplantation. Blood (2018) 131:2515–27. doi: 10.1182/blood-2017-12-823757
125. Kolb HJ, Mittermüller J, Clemm C, Holler E, Ledderose G, Brehm G, et al. Donor leukocyte transfusions for treatment of recurrent chronic myelogenous leukemia in marrow transplant patients. Blood (1990) 76:2462–5.
126. de Lima M, Porter DL, Battiwalla M, Bishop MR, Giralt SA, Hardy NM, et al. Proceedings from the National Cancer Institute's Second International Workshop on the Biology, Prevention, and Treatment of Relapse After Hematopoietic Stem Cell Transplantation: part III. Prevention and treatment of relapse after allogeneic transplantation. Biol Blood Marrow Transplant. (2014) 20:4–13. doi: 10.1016/j.bbmt.2013.08.012
127. Chang YJ, Huang XJ. Donor lymphocyte infusions for relapse after allogeneic transplantation: when, if and for whom? Blood Rev. (2013) 27:55–62. doi: 10.1016/j.blre.2012.11.002
128. Yan CH, Liu DH, Liu KY, Xu LP, Liu YR, Chen H, et al. Risk stratification-directed donor lymphocyte infusion could reduce relapse of standard-risk acute leukemia patients after allogeneic hematopoietic stem cell transplantation. Blood (2012) 119:3256–62. doi: 10.1182/blood-2011-09-380386
129. Goldsmith SR, Slade M, DiPersio JF, Westervelt P, Schroeder MA, Gao F, et al. Donor-lymphocyte infusion following haploidentical hematopoietic cell transplantation with peripheral blood stem cell grafts and PTCy. Bone Marrow Transplant. (2017) 52:1623–8. doi: 10.1038/bmt.2017.193
130. Nikiforow S, Kim HT, Daley H, Reynolds C, Jones KT, Armand P, et al. A phase I study of CD25/regulatory T-cell-depleted donor lymphocyte infusion for relapse after allogeneic stem cell transplantation. Haematologica (2016) 101:1251–9. doi: 10.3324/haematol.2015.141176
131. Di Ianni M, Olioso P, Giancola R, Santarone S, Natale A, Papalinetti G, et al. Treg-protected donor lymphocyte infusions: a new tool to address the graft-versus-leukemia effect in the absence of graft-versus-host disease in patients relapsed after HSCT. Int J Hematol. (2017) 106:860–4. doi: 10.1007/s12185-017-2292-3
132. Comoli P, Basso S, Riva G, Barozzi P, Guido I, Gurrado A, et al. BCR-ABL-specific T-cell therapy in Ph+ ALL patients on tyrosine-kinase inhibitors. Blood (2017) 129:582–6. doi: 10.1182/blood-2016-07-731091
133. Chapuis AG, Ragnarsson GB, Nguyen HN, Chaney CN, Pufnock JS, Schmitt TM, et al. Transferred WT1-reactive CD8+ T cells can mediate antileukemic activity and persist in post-transplant patients. Sci Transl Med. (2013) 5:174ra27. doi: 10.1126/scitranslmed.3004916
134. Weber G, Gerdemann U, Caruana I, Savoldo B, Hensel NF, Rabin KR, et al. Generation of multi-leukemia antigen-specific T cells to enhance the graft-versus-leukemia effect after allogeneic stem cell transplant. Leukemia (2013) 27:1538–47. doi: 10.1038/leu.2013.66
135. Lee DA, Denman CJ, Rondon G, Woodworth G, Chen J, Fisher T, et al. Haploidentical natural killer cells infused before allogeneic stem cell transplantation for myeloid malignancies: a phase I trial. Biol Blood Marrow Transplant. (2016) 22:1290–8. doi: 10.1016/j.bbmt.2016.04.009
136. Ciurea SO, Schafer JR, Bassett R, Denman CJ, Cao K, Willis D, et al. Phase 1 clinical trial using mbIL21 ex vivo-expanded donor-derived NK cells after haploidentical transplantation. Blood (2017) 130:1857–68. doi: 10.1182/blood-2017-05-785659
137. Jaiswal SR, Zaman S, Nedunchezhian M, Chakrabarti A, Bhakuni P, Ahmed M, et al. CD56-enriched donor cell infusion after post-transplantation cyclophosphamide for haploidentical transplantation of advanced myeloid malignancies is associated with prompt reconstitution of mature natural killer cells and regulatory T cells with reduced incidence of acute graft versus host disease: a pilot study. Cytotherapy (2017) 19:531–42. doi: 10.1016/j.jcyt.2016.12.006
138. Chen Y, Cheng Y, Suo P, Yan C, Wang Y, Chen Y, et al. Donor-derived CD19-targeted T cell infusion induces minimal residual disease-negative remission in relapsed B-cell acute lymphoblastic leukaemia with no response to donor lymphocyte infusions after haploidentical haematopoietic stem cell transplantation. Br J Haematol. (2017) 179:598–605. doi: 10.1111/bjh.14923
139. Carpenter PA, Snyder DS, Flowers ME, Sanders JE, Gooley TA, Martin PJ, et al. Prophylactic administration of imatinib after hematopoietic cell transplantation for high-risk Philadelphia chromosome-positive leukemia. Blood (2007) 109:2791–3. doi: 10.1182/blood-2006-04-019836
140. Brissot E, Labopin M, Beckers MM, Socié G, Rambaldi A, Volin L, et al. Tyrosine kinase inhibitors improve long-term outcome of allogeneic hematopoietic stem cell transplantation for adult patients with Philadelphia chromosome positive acute lymphoblastic leukemia. Haematologica (2015) 100:392–9. doi: 10.3324/haematol.2014.116954
141. Pfeifer H, Wassmann B, Bethge W, Dengler J, Bornhäuser M, Stadler M, et al. Randomized comparison of prophylactic and minimal residual disease-triggered imatinib after allogeneic stem cell transplantation for BCR-ABL1-positive acute lymphoblastic leukemia. Leukemia (2013) 27:1254–62. doi: 10.1038/leu.2012.352
142. Chen H, Liu KY, Xu LP, Liu DH, Chen YH, Zhao XY, et al. Administration of imatinib after allogeneic hematopoietic stem cell transplantation may improve disease-free survival for patients with Philadelphia chromosome-positive acute lymphobla stic leukemia. J Hematol Oncol. (2012) 5:29. doi: 10.1186/1756-8722-5-29
143. Giebel S, Czyz A, Ottmann O, Baron F, Brissot E, Ciceri F, et al. Use of tyrosine kinase inhibitors to prevent relapse after allogeneic hematopoietic stem cell transplantation for patients with Philadelphia chromosome-positive acute lymphoblastic leukemia: a position statement of the Acute Leukemia Working Party of the European Society for Blood and Marrow Transplantation. Cancer (2016) 122:2941–51. doi: 10.1002/cncr.30130
144. Brunner AM, Li S, Fathi AT, Wadleigh M, Ho VT, Collier K, et al. Haematopoietic cell transplantation with and without sorafenib maintenance for patients with FLT3-ITD acute myeloid leukaemia in first complete remission. Br J Haematol. (2016) 175:496–504. doi: 10.1111/bjh.14260
145. Battipaglia G, Ruggeri A, Massoud R, El Cheikh J, Jestin M, Antar A, et al. Efficacy and feasibility of sorafenib as a maintenance agent after allogeneic hematopoietic stem cell transplantation for Fms-like tyrosine kinase 3-mutated acute myeloid leukemia. Cancer (2017) 123:2867–74. doi: 10.1002/cncr.30680
146. Mathew NR, Baumgartner F, Braun L, O'Sullivan D, Thomas S, Waterhouse M, et al. Sorafenib promotes graft-versus-leukemia activity in mice and humans through IL-15 production in FLT3-ITD-mutant leukemia cells. Nat Med. (2018) 24:282–91. doi: 10.1038/nm.4484
147. Xuan L, Wang Y, Huang F, Jiang E, Deng L, Wu B, et al. Effect of sorafenib on the outcomes of patients with FLT3-ITD acute myeloid leukemia undergoing allogeneic hematopoietic stem cell transplantation. Cancer (2018) 124:1954–63. doi: 10.1002/cncr.31295
148. Cortes JE, Tallman MS, Schiller GJ, Trone D, Gammon G, Goldberg SL, et al. Phase 2b study of two dosing regimens of quizartinib monotherapy in FLT3-ITD mutated, relapsed or refractory AML. Blood (2018) 132:598–607. doi: 10.1182/blood-2018-01-821629
149. Cortes J, Perl AE, Döhner H, Kantarjian H, Martinelli G, Kovacsovics T, et al. Quizartinib, an FLT3 inhibitor, as monotherapy in patients with relapsed or refractory acute myeloid leukaemia: an open-label, multicentre, single-arm, phase 2 trial. Lancet Oncol. (2018) 19:889–903. doi: 10.1016/S1470-2045(18)30240-7
150. Waespe N, Van Den Akker M, Klaassen RJ, Lieberman L, Irwin MS, Ali SS, et al. Response to treatment with azacitidine in children with advanced myelodysplastic syndrome prior to hematopoietic stem cell transplantation. Haematologica (2016) 101:1508–15. doi: 10.3324/haematol.2016.145821
151. Ishikawa T, Fujii N, Imada M, Aoe M, Meguri Y, Inomata T, et al. Graft-versus-leukemia effect with a WT1-specific T-cell response induced by azacitidine and donor lymphocyte infusions after allogeneic hematopoietic stem cell transplantation. Cytotherapy (2017) 19:514–20. doi: 10.1016/j.jcyt.2016.12.007
152. Cooper ML, Choi J, Karpova D, Vij K, Ritchey J, Schroeder MA, et al. Azacitidine mitigates graft-versus-host disease via differential effects on the proliferation of T effectors and natural regulatory T cells in vivo. J Immunol. (2017) 198:3746–54. doi: 10.4049/jimmunol.1502399
153. El-Cheikh J, Massoud R, Fares E, Kreidieh N, Mahfouz R, Charafeddine M, et al. Low-dose 5-azacytidine as preventive therapy for relapse of AML and MDS following allogeneic HCT. Bone Marrow Transplant. (2017) 52:918–21. doi: 10.1038/bmt.2017.31
154. de Lima M, Oran B, Champlin RE, Papadopoulos EB, Giralt SA, Scott BL, et al. CC-486 maintenance after stem cell transplantation in patients with acute myeloid leukemia or myelodysplastic syndromes. Biol Blood Marrow Transplant. (2018) 24:2017–24. doi: 10.1016/j.bbmt.2018.06.016
155. Goodyear OC, Dennis M, Jilani NY, Loke J, Siddique S, Ryan G, et al. Azacitidine augments expansion of regulatory T cells after allogeneic stem cell transplantation in patients with acute myeloid leukemia (AML). Blood (2012) 119:3361–9. doi: 10.1182/blood-2011-09-377044
156. Craddock C, Labopin M, Robin M, Finke J, Chevallier P, Yakoub-Agha I, et al. Clinical activity of azacitidine in patients who relapse after allogeneic stem cell transplantation for acute myeloid leukemia. Haematologica (2016) 101:879–83. doi: 10.3324/haematol.2015.140996
157. Rautenberg C, Nachtkamp K, Dienst A, Schmidt PV, Heyn C, Kondakci M, et al. Sorafenib and azacitidine as salvage therapy for relapse of FLT3-ITD mutated AML after allo-SCT. Eur J Haematol. (2017) 98:348–54. doi: 10.1111/ejh.12832
158. Schroeder T, Rautenberg C, Krüger W, Platzbecker U, Bug G, Steinmann J, et al. Treatment of relapsed AML and MDS after allogeneic stem cell transplantation with decitabine and DLI-a retrospective multicenter analysis on behalf of the German Cooperative Transplant Study Group. Ann Hematol. (2018) 97:335–42. doi: 10.1007/s00277-017-3185-5
159. Liu L, Chang YJ, Xu LP, Zhang XH, Wang Y, Liu KY, et al. T cell exhaustion characterized by compromised MHC class I and II restricted cytotoxic activity associates with acute B lymphoblastic leukemia relapse after allogeneic hematopoietic stem cell transplantation. Clin Immunol. (2018) 190:32–40. doi: 10.1016/j.clim.2018.02.009
160. Liu L, Chang YJ, Xu LP, Zhang XH, Wang Y, Liu KY, et al. Reversal of T cell exhaustion by the first donor lymphocyte infusion is associated with the persistently effective antileukemic responses in patients with relapsed AML after Allo-HSCT. Biol Blood Marrow Transplant. (2018) 24:1350–9. doi: 10.1016/j.bbmt.2018.03.030
161. Bashey A, Medina B, Corringham S, Pasek M, Carrier E, Vrooman L, et al. CTLA4 blockade with ipilimumab to treat relapse of malignancy after allogeneic hematopoietic cell transplantation. Blood (2009) 113:1581–8. doi: 10.1182/blood-2008-07-168468
162. Davids MS, Kim HT, Bachireddy P, Costello c, Liguori R, Savel A, et al. Ipilimumab for patients with relapse after allogeneic transplantation. N Engl J Med. (2016) 375:143–53. doi: 10.1056/NEJMoa1601202
163. Khouri IF, Fernandez Curbelo I, Turturro F, Jabbour EJ, Milton DR, Bassett RL, et al. Ipilimumab plus Lenalidomide after allogeneic and autologous stem cell transplantation for patients with lymphoid malignancies. Clin Cancer Res. (2018) 24:1011–8. doi: 10.1158/1078-0432.CCR-17-2777
164. Rafiq S, Yeku OO, Jackson HJ, Purdon TJ, van Leeuwen DG, Drakes DJ, et al. Targeted delivery of a PD-1-blocking scFv by CAR-T cells enhances anti-tumor efficacy in vivo. Nat Biotechnol. (2018) 36:847–56. doi: 10.1038/nbt.4195
Keywords: allogeneic stem cell transplantation, graft-versus-leukemia, graft-versus-host disease, relapse, G-CSF
Citation: Chang Y-J, Zhao X-Y and Huang X-J (2018) Strategies for Enhancing and Preserving Anti-leukemia Effects Without Aggravating Graft-Versus-Host Disease. Front. Immunol. 9:3041. doi: 10.3389/fimmu.2018.03041
Received: 01 October 2018; Accepted: 10 December 2018;
Published: 21 December 2018.
Edited by:
Brian Christopher Betts, University of Minnesota Twin Cities, United StatesReviewed by:
Sergio Querol, Banc de Sang i Teixits, SpainLuisa Sisinni, Hospital de la Santa Creu i Sant Pau, Spain
Copyright © 2018 Chang, Zhao and Huang. This is an open-access article distributed under the terms of the Creative Commons Attribution License (CC BY). The use, distribution or reproduction in other forums is permitted, provided the original author(s) and the copyright owner(s) are credited and that the original publication in this journal is cited, in accordance with accepted academic practice. No use, distribution or reproduction is permitted which does not comply with these terms.
*Correspondence: Xiao-Jun Huang, aHVhbmd4aWFvanVuQGJqbXUuZWR1LmNu