- Centre for Inflammation and Tissue Repair, UCL Respiratory, London, United Kingdom
In this review we give an update on the mechanisms of naturally acquired immunity against Streptococcus pneumoniae, one of the major human bacterial pathogens that is a common cause of pneumonia, septicaemia, and meningitis. A clear understanding of the natural mechanisms of immunity to S. pneumoniae is necessary to help define why the very young and elderly are at high risk of disease, and for devising new prevention strategies. Recent data has shown that nasopharynx colonization by S. pneumoniae induces antibody responses to protein and capsular antigens in both mice and humans, and also induces Th17 CD4+ cellular immune responses in mice and increases pre-existing responses in humans. These responses are protective, demonstrating that colonization is an immunizing event. We discuss the data from animal models and humans on the relative importance of naturally acquired antibody and Th17 cells on immunity to S. pneumoniae at three different anatomical sites of infection, the nasopharynx (the site of natural asymptomatic carriage), the lung (site of pneumonia), and the blood (site of sepsis). Mouse data suggest that CD4+ Th17 cells prevent both primary and secondary nasopharyngeal carriage with no role for antibody induced by previous colonization. In contrast, antibody is necessary for prevention of sepsis but CD4+ cellular responses are not. Protection against pneumonia requires a combination of both antibody and Th17 cells, in both cases targeting protein rather than capsular antigen. Proof of which immune component prevents human infection is less easily available, but two recent papers demonstrate that human IgG targeting S. pneumoniae protein antigens is highly protective against septicaemia. The role of CD4+ responses to prior nasopharyngeal colonization for protective immunity in humans is unclear. The evidence that there is significant naturally-acquired immunity to S. pneumoniae independent of anti-capsular polysaccharide has clinical implications for the detection of subjects at risk of S. pneumoniae infections, and the data showing the importance of protein antigens as targets for antibody and Th17 mediated immunity should aid the development of new vaccine strategies.
Introduction
Streptococcus pneumoniae is a major cause of acute otitis media, community-acquired pneumonia, bacterial sepsis, and meningitis and is estimated to be responsible for over 800,000 deaths annually in children (1). S. pneumoniae strains causing invasive infections are surrounded by a polysaccharide capsule layer that inhibits innate and adaptive immune responses to infection (2). The capsule has a varied biochemical composition and antigenic structure between S. pneumoniae strains, resulting in over 90 different capsular serotypes (3). Serotype specific S. pneumoniae vaccines have been developed using capsular antigen linked to carrier protein, termed pneumococcal conjugated vaccines (PCVs), and these are highly effective against the serotypes included in the vaccine (4–6). However, there is still a high level of mortality and morbidity due to S. pneumoniae infections due to the restricted serotype coverage of PCVs resulting in serotype-replacement disease, and their high cost leading to incomplete use of PCVs in low and middle-income countries. In addition, PCVs are less effective at preventing pneumonia compared to septicaemia and meningitis, despite pneumonia making the largest contribution to the burden of disease (1, 7, 8). Novel approaches are needed to overcome the limitations of the present PCV. One way of identifying new preventative strategies could be to define the mechanisms of natural adaptive immunity to S. pneumoniae, which could then be targeted to enhance immunity against infection in high-risk groups such as children and older adults. In this review we will discuss the evidence for naturally acquired adaptive immunity to S. pneumoniae and the mechanisms by which this maybe mediated.
Clinical and Epidemiological Evidence for Adaptive Immunity to S. pneumoniae
S. pneumoniae Nasopharyngeal Colonization
Unlike some major bacterial respiratory pathogens such as Mycobacterium tuberculosis, S. pneumoniae is also a ubiquitous human commensal of the upper respiratory tract, specifically of the nasopharynx. Colonization of the nasopharynx occurs in the first years of life and repeatedly thereafter (9–11). Successive episodes of colonization with different pneumococcal strains occur in children up to 2 years of age (9, 10), with the point prevalence of nasopharyngeal carriage estimated at 27–65% among infants. This colonization prevalence decreases with age to < 10% during adult life (12–14), and even lower rates in the elderly (15). This decline in colonization seems to have a multifactorial nature, one element of could be maturation of the immune system with age. The main nasopharyngeal reservoir for the spread of S. pneumoniae is in children, and consequently vaccination of children with PCV (which prevents nasopharyngeal colonization) has resulted in significant herd immunity against adult S. pneumoniae pneumonia (12).
Epidemiological Evidence for Naturally Acquired Adaptive Immunity to S. pneumoniae
Subjects with defects of their adaptive immune response are more susceptible to S. pneumoniae infections. These groups include people with genetic or acquired defects in immunoglobulin production, and those who have severe defects of adaptive immunity due to stem cell transplantation or HIV infection (16–18). In addition, subjects with deficiencies in the classical pathway of complement activation have a massively increased incidence of pneumococcal septicaemia, meningitis, and bacterial pneumonia (19). Although this will reflect weakened complement-mediated innate immunity to S. pneumoniae (20), the classical complement pathway is also vital for antibody mediated killing of S. pneumoniae (2), and hence the susceptibility of subjects with classical pathway defects will partially reflect weakened antibody mediated immunity. Furthermore, the incidence of S. pneumoniae infection with age has a pronounced U shaped curve with the highest incidence in infants and the elderly (21), a pattern that suggests there is an adaptive immune response to S. pneumoniae that improves in children with maturity then perhaps wanes due to immunosenescence in the elderly. The frequency of S. pneumoniae septicaemia associated with pneumonia is also higher in infants than adults, indicating that in adults the immune system is able to prevent spread to the blood from the lungs. Overall, these epidemiological data indicate that there is significant naturally acquired adaptive immunity that helps prevent S. pneumoniae infections. Importantly, the incidence of infection with all serotypes falls at the same time (22), an epidemiological observation that suggests naturally acquired resistance to S. pneumoniae infections is mediated by serotype independent mechanisms rather than anticapsular antibody.
This evidence of naturally acquired adaptive immunity to S. pneumoniae leads to the question of how this has developed? Only a small proportion of humans have been exposed to S. pneumoniae during invasive diseases such as septicaemia and meningitis, and although many more humans will have had an episode of S. pneumoniae pneumonia this would have been with a single strain and capsular serotype. In contrast, almost all humans have been exposed to S. pneumoniae via nasopharyngeal colonization events on multiple occasions and by many different strains. Hence, if most (if not all) humans have a naturally acquired serotype-independent adaptive immunity to S. pneumoniae then this must have occurred because of colonization rather than active infectious disease.
Evidence That S. pneumoniae Nasopharyngeal Colonization Is an Immunizing Event
Data obtained during natural and experimental human infection and from mouse models have now shown conclusively that colonization of the nasopharynx by S. pneumoniae is indeed an immunizing event. Multiple longitudinal studies of serum antibody responses in infants show the development of antibody responses to S. pneumoniae capsular and protein antigens in serum and saliva after human colonization events (11, 14, 23, 24). In murine models, several authors have shown both antibody and T-cell mediated immune responses develop after a colonization event with S. pneumoniae or exposure to S. pneumoniae antigens in the nasopharynx (25–32). In addition, after successful experimental human colonization with S. pneumoniae pre-existing antibody responses (33, 34) and S. pneumoniae-specific blood and lung IL-17 producing CD4+ memory cells are increased (35), providing enhanced humoral and cellular immunity. These data confirm that colonization is an immunizing event, and this is supported by data from infants showing gradual increases in anti-protein antigen IgG responses with age and correlations between colonization events and increases in anti-capsular antibody for several serotypes (11).
Mechanisms of Naturally Acquired Adaptive Immune Protection Against S. pneumoniae Infection
The data discussed above demonstrate that humans do develop naturally acquired adaptive immune responses against S. pneumoniae in response to nasopharyngeal colonization and that these responses are likely to be protective. The adaptive response to nasopharyngeal colonization by S. pneumoniae includes acquisition of anti-capsular (14, 36, 37) and anti-protein antibodies (38–40), as well as CD4+ cellular immune responses targeting protein antigens alone (39, 41). The observation of CD4+ cellular immune responses is important, as Th17 CD4+ responses are a common mechanism for pathogen clearance at mucosal surfaces via rapid recruitment of neutrophils to the site of infection, improved epithelial barrier function, and increased secretion of antibacterial peptides (42, 43). However, to effectively utilize these findings to design preventative strategies against S. pneumoniae it is necessary to go one step further and identify which of these responses actually protect against S. pneumoniae infections.
All currently licensed pneumococcal vaccines use polysaccharide capsular antigen, and capsular antibodies against the capsule are highly protective against infection caused by that serotype (5, 44, 45). As a consequence, historically the literature has also emphasized the role of anti-capsule antibodies as the mechanism for naturally acquired immunity against S. pneumoniae (46). This is supported by the early data showing that sera from survivors of severe S. pneumoniae provided serotype-specific protection when used as a passive antibody treatment (47–51). However, as mentioned above the epidemiological evidence suggests that naturally acquired immunity has a significant serotype-independent component. Analysis of datasets from several countries (e.g., USA, Finland and Israel), demonstrated a decrease in incidence of invasive disease with age independent of increases in serotype-specific antibody levels, suggesting a different mechanism of protection such as acquired immunity to non-capsular antigens or maturation of non-specific immune responses (22). Theoretical modeling also suggested that only 30–60% of the reduction in recolonization is led by anti-capsular immunity generated during previous colonization events (52). Animal model data has shown that antibody and more recently cell mediated immune responses targeting protein rather than capsular antigens can protect against S. pneumoniae infections, providing proof of principle that these capsular-antigen independent mechanisms could mediate naturally acquired immunity to S. pneumoniae in humans (25, 26, 38, 53). Which proteins dominant the human antibody response to S. pneumoniae protein antigens was recently defined by Croucher using a proteome microarray that displays more than 2,000 potential S. pneumoniae antigens. Of these, 208 had significant IgG responses in sera obtained from 35 healthy US adults. About half of these antigens were allelic variants of the highly variable surface proteins PspA, PspC, ZmpA, and ZmpB expressed by different S. pneumoniae strains (54). The remaining proteins recognized by human sera were more conserved between strains and were enriched in motifs for adhesion or degradation, cell wall metabolism, or solute binding for transport. These results are consistent with a previous screen reverse vaccinology approach that identified proteins recognized by antibody in human sera which identified the proteins PspC and PspA, along with PcsB and StkP (55). There are only limited data on the identification of S. pneumoniae antigens that induce Th17 CD4+ cell responses as this is technically difficult. The available data was obtained in mice by screening of an expression library containing >96% of predicted pneumococcal protein and identified several proteins (including ABC transporter lipoproteins) that induce Th17 responses after exposure to whole pneumococci and as purified protein antigens, with little overlap between with antibody-inducing protein antigens (56).
Overall, the data from human and animal studies show colonization induces both anti-capsule and anti-protein antibody responses, and in addition cell mediated immunity to protein antigens. The anatomy, associated immunological tissues, and interactions with soluble immune effectors in mucosal lining fluid or in the plasma all vary between the common anatomical sites of S. pneumoniae infection, creating potential variations in the relative efficacy of different immune effectors for controlling S. pneumoniae infection between anatomical sites. Hence the following discussion of which mechanisms of naturally acquired adaptive immunity protect against S. pneumoniae is divided into three representative sites of infection; (i) the nasopharynx as a mucosal site colonized by S. pneumoniae; (ii) the blood as a site of systemic invasive infection; and (iii) the lungs as a deep site of mucosal infection intimately associated with the systemic circulation, and also the site responsible for the biggest burden of severe infections globally. In general, data from animal studies (mainly in mice) can clearly define protective mechanisms but is of more limited utility due to potential differences in immune function between species. However, after early childhood all humans have had multiple episodes of S. pneumoniae colonization each of which will stimulate some degree of immune response, and this background situation of considerable prior exposure to S. pneumoniae over many years is difficult to replicate in mouse models. Human data is directly relevant but it is usually only possible to provide potential correlations rather than direct proof between a specific immune effector and protection against disease.
Prevention of Subsequent Colonization
Mouse Data
Whether antibodies have an important role in controlling a primary episode of nasopharyngeal colonization by S. pneumoniae was investigated by McCool and Weiser using murine models of infection and mice knock out strains affecting specific aspects of antibody mediated immunity (38). They established a murine model of colonization in xid mice, which have a poor antibody response to polysaccharide capsule. S. pneumoniae clearance from the nasopharynx was similar in xid mice compared to wild-type mice. These results were corroborated using uMT mice, which lack B cell and antibody responses, which were also still able to clear S. pneumoniae nasopharyngeal colonization. These results demonstrate that antibody responses do not aid clearance of primary colonization events, and have been confirmed by other studies (57). Instead, protection against recolonization was lost in mice deficient in CD4+ cells, highlighting the requirement of cellular immunity rather than antibody in protection against recolonization (58). Similar data were obtained using administration of the S. pneumoniae antigen cell wall polysaccharide intranasally, which inhibited subsequent colonization independent of antibody but dependent on CD4+ cells (29). In addition, these authors confirmed for the first time an important role for IL-17 in preventing colonization, although the exact mechanism by which this cytokine provided protection was not determined. Vaccination with killed unencapsulated S. pneumoniae also induced Th17 CD4+ immunity which prevented nasopharyngeal colonization with a heterologous S. pneumoniae serotype (27, 59).
The data obtained from these studies indicated an important role of CD4+ cells rather than antibodies for prevention of a second episode of S. pneumoniae colonization, and this has been confirmed by more detailed investigation in mice. Zhang et al. demonstrated that the clearance of both the initial and second episode of S. pneumoniae colonization in adult mice was dependent on cellular responses rather than humoral immunity (58, 60). Clearance of the initial episode of colonization involved recruitment of monocytes/macrophages into the upper airway lumen via a TLR2-dependent mechanism which required an IL-17 secreting CD4+ cell population (57, 60). Prevention of a second episode of colonization also required IL-17 and CD4+ cells, and was mediated by rapid neutrophil recruitment to the nasopharynx. These findings are supported by other data showing CD4+ cells and IL-17 are required for protection against colonization and mediate the protective effect of immunization with whole cell vaccines against colonization[28, 29, 31].
Overall, the murine data demonstrate that Th17 responses to protein antigens are critical for controlling S. pneumoniae colonization after a previous episode of colonization; subsequent work has identified several protein antigens that are able to induce Th17 mediated immunity but whether these antigens are the same for different S. pneumoniae strains is not known (56). These data do not preclude a potential role for vaccine induced antibody for prevention of colonization after vaccination, and in mice vaccination with protein antigens elicits protection against S. pneumoniae colonization (61, 62) and passive immunization with anticapsular antibodies prevents both colonization and transmission between littermates (63, 64).
Human Data
As discussed above longitudinal studies have demonstrated that antibodies to both protein and capsule antigens develop after episodes of S. pneumoniae colonization. As a consequence adult sera contains antibodies to multiple capsular S. pneumoniae serotypes and one hundred or so protein antigens (11, 54). In addition, different studies have identified Th17 CD4+ responses to S. pneumoniae in adenoid tissue (65, 66), bronchoalveolar lavage (35), and blood (31, 35, 67). Evidence for which of these naturally acquired immune mechanisms can prevent colonization in humans is less readily obtained. The impressive reduction in S. pneumoniae vaccine serotype prevalence as nasopharyngeal commensals in populations vaccinated with PCV demonstrates that high levels of anti-capsular antibody do prevent colonization (5–7). Recent data obtained using the experimental human pneumococcal carriage model (EHPC) and murine models suggest anticapsular antibody inhibits the establishment of colonization by serotype-specific agglutination of S. pneumoniae (63, 68). Whether the lower levels of anti-capsular antibody induced by natural colonization rather than vaccination with a PCV is enough to prevent colonization is not clear.
The most important data on immune mechanisms that prevent colonization in humans has been obtained using the EHPC model. In this experimental human infection model, human volunteers are inoculated intranasally with a serotype 6B strain leading to successful nasopharyngeal colonization in approximately half of subjects (34). Previous colonization prevented recolonization with the same strain (34). In this model, all recruited subjects had detectable IgG levels to S. pneumoniae antigens prior to nasopharyngeal administration of S. pneumoniae, but anti-pneumococcal IgG levels did not correlate with whether colonization was successful or not (34). In contrast, a previous EHPC model suggested that antibodies to the S. pneumoniae surface protein PspA correlated with prevention of successful colonization, providing some evidence that anti-protein antibody may prevent colonization (33). Exposure to serotype 6B or 23F strains increased antibody responses to multiple S. pneumoniae proteins (e.g., PspA, PspC, PsaA, and PdB), with the highest increases in carriage positive subjects (33, 34, 69). Systemic anti-capsule antibodies were only detected in subjects that developed carriage after challenge (34). S. pneumoniae specific IL-17 secreting CD4+ cells were found in the lung prior to exposure to the 6B strain but were increased in bronchoalveolar fluid 17-fold and in blood 8-fold in volunteers in which colonization was successful (35, 69). Overall these data confirm that pre-existing antibody and local CD4+ cellular immunity to S. pneumoniae are significantly boosted by colonization events, and that this seems to prevent recolonization for at least a period of several months. However, the data are unable to define which of these immune mechanisms was important for the prevention of recolonization.
Prevention of S. pneumoniae Septicaemia and Meningitis
Mouse Data
Mouse data also clearly demonstrates that colonization of the nasopharynx promotes protection against invasive disease, but in contrast to prevention of colonization this is dependent on antibody mediated immunity, not CD4+ cells. Cohen et al. showed that a previous episode of colonization with the S. pneumoniae D39 strain was highly protective against subsequent lethal invasive pneumonia, with the major effect being prevention of septicaemia (70). CD4+ depleted mice were still protected against septicaemia, whereas no protection was seen in antibody deficient mice, and passive transfer of serum from colonized mice into immune naïve mice also protected against S. pneumoniae septicaemia. Similarly, Bou Ghanem et al found that previous colonization protected against S. pneumoniae pneumonia with septicaemia caused by the TIGR4 strain, with the most profound effect seen on blood colony forming units (CFU) (71). Repeated episodes of nasopharynx colonization in antibody deficient mice did not induced any protection whereas protection persisted after CD4+ cell depletion, and the authors proposed long-lived antibody secreting CD138+ cells were responsible for the protective effect of prior colonization. These data demonstrating the important role of antibody are perhaps not surprising as protection against septicaemia in mice is dependent on soluble mediators such as complement and naturally and induced antibody working in concert with the reticuloendothelial system, especially the spleen (72–74), rather than CD4+ Th17 mediated mechanisms.
Human Data
It was commonly accepted for many years that the major mechanism of natural immunity against invasive pneumococcal disease (IPD) was anti-capsular antibodies generated by previous episodes of either colonization or infection (44, 48, 50). However, this assumption has been progressively threatened by epidemiological data as discussed above. More recently experimental data by Wilson et al. has demonstrated that antibody to protein rather than capsular antigen is a major mechanism of naturally acquired immunity to S. pneumoniae septicaemia and IPD (75). To do so the authors used Intravenous Immunoglobulin (IVIG) which is used to prevent infections in individuals with primary antibody deficiency (76, 77). IVIG contains the pooled IgG from the plasma of approximately a thousand donors who are unlikely to have been vaccinated against S. pneumoniae due to their lack of risk factors, and therefore contains the naturally acquired antibody repertoire for the population from which the donors are obtained. Antibodies to both S. pneumoniae capsule and multiple proteins were detected in IVIG. Immunoblots against lysates from different pneumococcal strains demonstrated a highly conserved pattern of protein targets recognized by IVIG, including major pneumococcal surface proteins such as choline binding proteins and lipoproteins. Importantly, opsonophagocytosis of S. pneumoniae was improved against unencapsulated bacteria but reduced when anticapsular antibody was depleted from the IVIG preparation. IVIG was highly protective against S. pneumoniae septicaemia in mouse models, even after depletion of anticapsular antibody. These data demonstrate there is a high level of naturally acquired antibody to S. pneumoniae protein antigens in adult sera that promotes bacterial clearance during sepsis. In addition, passive vaccination of mice with sera obtained from human EHPC subjects colonized with the serotype 6B strain conferred protection against the D39 serotype 2 strain in a pneumonia model in which lethality is driven by septicaemia (34). The data from these two studies provide direct proof that antiprotein antibody is an important mechanism of naturally acquired immunity to S. pneumoniae systemic infection.
Prevention of S. pneumoniae Pneumonia
The nasopharynx represents a mucosal site of infection, and septicaemia a systemic site, whereas the commonest site of serious infection, the lungs, represents a combination of the two. In the early stages of pneumonia infection is limited to interactions with the mucosal surface, which are similar to those found in the nasopharynx. As the infection develops there is a breakdown in the epithelial/endothelial barrier function and cellular recruitment to the alveoli, recruiting systemic soluble and white cell-mediated immune mechanisms to the alveoli. Hence, both mucosal and systemic mechanisms of adaptive immunity could potentially have roles in protection against S. pneumoniae pneumonia.
Mouse Data
Several studies have examined the role of colonization-induced immunity for protection against pneumonia in murine models. In a murine model of asymptomatic carriage with the D39 strain the number of CFU recovered from lung was reduced compared to control mice after a lethal pneumonia invasive challenge suggesting that colonization does improve local lung immunity against S. pneumoniae pneumonia (26). The authors also showed increased levels of IL-17A and CD4+ cells in lungs of previously colonized mice, indicating a potential role for T cell mediated immunity for prevention of pneumonia. These data were developed by Wilson et al. using a non-invasive S. pneumoniae serotype 19F strain that only causes lung infection without sepsis to allow lung immunity to be assessed separate to protection against systemic infection (25). Prior colonization protected against subsequent pneumonia, with a marked reduction in lung CFU. Additional experiments demonstrated that B cells, CD4+ and IL-17 were each necessary for protection against S. pneumoniae pneumonia caused by the 19F strain. These results suggested that naturally acquired protective immunity to S. pneumoniae pneumonia required a combination of both humoral immunity and Th17 CD4+ cells. Protection also required neutrophils, presumably as the main mechanism of bacterial killing, and previously colonized mice seemed to have a more rapid influx of neutrophils into the lungs during S. pneumoniae pneumonia compatible with improved local Th17 immunity (58). There was no detectable anti-capsular IgG responses after colonization. This suggested the humoral component of protection against pneumonia required anti-protein antibodies instead, and in agreement with previous mouse and human colonization models (28, 31, 69) the authors detected antibody responses to the important surface protein antigens PsaA, PhtD, and PpmA.
Two additional papers have also demonstrated an important role for Th17 CD4+ cells induced by prior exposure to S. pneumoniae for protection against subsequent severe S. pneumoniae pneumonia (78, 79). In both these papers protection was seen against pneumonia caused by a different capsular serotype, demonstrating that it was dependent on recognition of protein antigens. Smith et al. used prior exposure to S. pneumoniae through self-limiting lung infection rather than colonization, and demonstrated that protection was specific to S. pneumoniae and required administration of live rather than dead bacteria, suggesting bacterial replication within the respiratory tract was necessary. Importantly, they identified a population of tissue resident memory T cells within the lungs that mediated the antigen specific Th17 immunity to S. pneumoniae and that was restricted to the lobe that had been previously exposed to S. pneumoniae. It remains to be seen whether the low quantities of bacteria that reach the lung due to microaspiration from “pure” nasopharyngeal colonization are also sufficient to induce this population of lung tissue resident memory T cells.
Human Data
The increased incidence of lung infection in patients with immunoglobulin deficiencies (16, 80), HIV infection (81), or inherited disorders affecting IL-17 pathways [e.g., hyperIgE syndrome (82)], do indicate roles for both antibody and Th17 CD4+ cells for protection against S. pneumoniae pneumonia in humans as well as mice. However, these data are confounded by the presence of additional immune effects for each condition, with even immunoglobulin deficiencies resulting in functional impairment of S. pneumoniae-specific CD4+ cells (83). As previously mentioned, data obtained using bronchoalveolar lavage fluid has confirmed there are pre-existing CD4+ cells present in the human lung that produce IL-17 (and often TNFalpha) when stimulated with S. pneumoniae (36). The authors demonstrated that IL-17 improved S. pneumoniae killing mediated by either macrophages or neutrophils (31), providing an additional mechanism by which IL-17 can assist immunity to extracellular bacteria. BALF also contains antibody to S. pneumoniae protein and capsular antigens (33, 34, 69). Hence the human data has identified local pulmonary Th17 CD4+ and antibody specific for S. pneumoniae that are boosted by nasopharyngeal colonization which could potentially assist lung immunity to pneumonia as has been described in mouse models. However, the additional step of identifying which of these immune responses is actually protective against S. pneumoniae lung infection in humans is not easily achieved.
Implications of Data on Naturally Acquired Immunity to S. pneumoniae
As described above murine studies have defined how naturally acquired immunity due to prior colonization can protect against S. pneumoniae infections, and much of the data has been reinforced by epidemiological and experimental data obtained from humans. These mechanisms are summarized in Figure 1. In this last section, we will discuss how the data on naturally acquired infection may effect vaccination strategies and our understanding of the mechanisms underpinning susceptibility to S. pneumoniae in high risk groups.
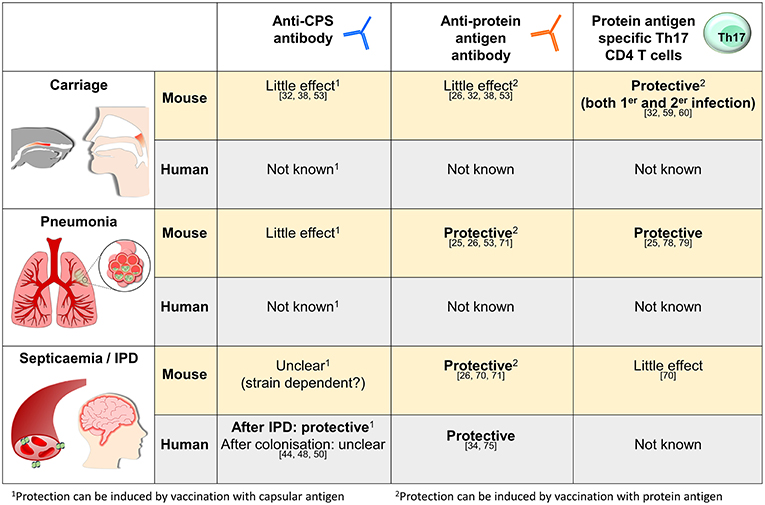
Figure 1. Summary of what is known about the mechanisms of naturally acquired (as opposed to vaccine-induced) adaptive immunity to S. pneumoniae in mice and humans for three representative sites of infection, the nasopharynx, the lungs, and in the blood. The references relevant for each statement are as shown.
In children, pneumococcal colonization events occur frequently and as discussed above this is necessary for the development of immunity against S. pneumoniae. During adulthood, colonization rates hugely decrease (15, 36, 84), but the data from the EHPC model clearly show that a colonization event still stimulates a boost to the pre-existing immune responses (34, 35, 69). Hence colonization has significant immune benefits to the host that could be affected if the ecology of S. pneumoniae colonization is altered. Multiple studies have indeed shown that routine PCV vaccination in children has profound effects on S. pneumoniae colonization, with hugely decreased carriage rates of the serotypes present in the vaccine (5, 6). However, there has been a parallel increase in colonization with non-vaccine serotypes reported to have a lower invasive disease potential (85, 86), and this alteration of carriage serotype ecology in favor or less invasive serotypes should also maintain the beneficial effects of colonization on natural mechanisms of immunity. If more aggressive preventative strategies reduce overall S. pneumoniae carriage rates then there might be a reduction in the strength of naturally immunity, and potentially a paradoxical increase risk of infection in adults.
The data on naturally acquired immunity should also help identify why certain risk groups such as the elderly, patients with chronic lung disease, or HIV infection are highly susceptible to S. pneumoniae infections. Studies performed both in mice and patients have highlighted the phenomenon of immunosenescence (87, 88), including an increase in baseline inflammation that seems to impair the development of immunity against S. pneumoniae after colonization (88, 89). In the elderly the reduced frequency of colonization events (15, 90) and the decreased levels and activity of IgM memory B cells (91) could also have an impact on colonization-induced immunity to S. pneumoniae. Streptococcus pneumoniae specific CD4 responses are dysregulated by HIV infection (92), and these may underpin the greatly increased susceptibility of HIV positive subjects to S. pneumoniae infections. How age and comorbidity affects naturally acquired adaptive responses needs to be clearly defined so that there is a much clearer understanding why these groups are highly susceptible to S. pneumoniae. Effective methods of measuring the strength of antiprotein and CD4+ cellular immune response to S. pneumoniae could also considerable improve our ability to measure susceptibility to this pathogen, which at present depends on measuring anticapsular antibody levels alone.
The recognition that there is a significant element on natural adaptive immunity to S. pneumoniae should also provide opportunities for new strategies of vaccination for prevention of S. pneumoniae infections. Firstly, strategies could be developed that boost existing naturally acquired immunity, especially in high risk subjects. Secondly, by knowing the mechanisms required for preventing specific diseases such as pneumonia these could be targeted by novel vaccines, for example by aiming to induce CD4+ cellular (mainly Th17) mediated immunity. Furthermore, the recognition that protein antigens in humans can provide significant immunity should boost research into vaccines that induce immune responses to protein antigen.
Summary
This review has discussed the induction and mechanisms of the naturally acquired immune response to S. pneumoniae. Multiple lines of evidence from animal experiments and in humans have convincingly demonstrated that nasopharyngeal colonization stimulates significant levels of naturally acquired immunity to S. pneumoniae that helps prevent subsequent colonization events as well as systemic and pulmonary infections. The mechanisms involved in preventing infection depends on anatomical site, with an emphasis on antibody during systemic infection and on Th17 CD4+ cells for mucosal infection, with pneumonia seemingly a combination of the two. In contrast to previous assumptions, antibody to protein as well as capsular antigens has an important role. Future work is required to delineate which protein antigens are the most important for protective immunity and whether there are important roles for other cellular immune mechanisms [e.g., Tregs, which have been shown to be important for innate immunity (93)] as well as Th17 CD4+ cells. Overall, these findings may lead to a much better understanding of why certain patient groups are at high risk of S. pneumoniae infection and should help improve future vaccine strategies.
Author Contributions
ER-S, GE, and JB contributed to draft the manuscript. ER-S and JB contributed to revising it critically and wrote the final version.
Conflict of Interest Statement
The authors declare that the research was conducted in the absence of any commercial or financial relationships that could be construed as a potential conflict of interest.
References
1. O'Brien KL, Wolfson LJ, Watt JP, Henkle E, Deloria-Knoll M, McCall N, et al. Burden of disease caused by Streptococcus pneumoniae in children younger than 5 years: global estimates. Lancet. (2009) 374:893–902. doi: 10.1016/S0140-6736(09)61204-6
2. Hyams C, Camberlein E, Cohen JM, Bax K, Brown JS, Hyams C, et al. The Streptococcus pneumoniae capsule inhibits complement activity and neutrophil phagocytosis by multiple mechanisms. Infect Immun. (2010) 78:704–15. doi: 10.1128/IAI.00881-09
3. Bentley SD, Aanensen DM, Mavroidi A, Saunders D, Rabbinowitsch E, Collins M, et al. Genetic analysis of the capsular biosynthetic locus from all 90 pneumococcal serotypes. PLoS Genet. (2006) 2:e31. doi: 10.1371/journal.pgen.0020031
4. Smit P, Oberholzer D, Hayden-Smith S, Koornhof HJ, Hilleman MR, Smit P, et al. Protective efficacy of pneumococcal polysaccharide vaccines. JAMA. (1977) 238:2613–6. doi: 10.1001/jama.1977.03280250039019
5. Shinefield HR, Black S. Efficacy of pneumococcal conjugate vaccines in large scale field trials. Pediatr Infect Dis J. (2000) 19:394–7. doi: 10.1097/00006454-200004000-00036
6. Whitney CG, Farley MM, Hadler J, Harrison LH, Bennett NM, Lynfield R, et al. Decline in invasive pneumococcal disease after the introduction of protein-polysaccharide conjugate vaccine. N Engl J Med. (2003) 348:1737–46. doi: 10.1056/NEJMoa022823
7. Bonten MJ, Huijts SM, Bolkenbaas M, Webber C, Patterson S, Gault S, et al. Polysaccharide conjugate vaccine against pneumococcal pneumonia in adults. N Engl J Med. (2015) 372:1114–25. doi: 10.1056/NEJMoa1408544
8. Jose RJ, Brown JS. Adult pneumococcal vaccination:advances, impact, and unmet needs. Curr Opin Pulm Med. (2017) 23:225–30. doi: 10.1097/MCP.0000000000000369
9. Hussain M, Melegaro A, Pebody RG, George R, Edmunds WJ, Talukdar R, et al. A longitudinal household study of Streptococcus pneumoniae nasopharyngeal carriage in a UK setting. Epidemiol Infect. (2005) 133:891–8. doi: 10.1017/S0950268805004012
10. Hill PC, Cheung YB, Akisanya A, Sankareh K, Lahai G, Greenwood BM, et al. Nasopharyngeal carriage of Streptococcus pneumoniae in Gambian infants:a longitudinal study. Clin Infect Dis. (2008) 46:807–14. doi: 10.1086/528688
11. Turner P, Turner C, Green N, Ashton L, Lwe E, Jankhot A, et al. Serum antibody responses to pneumococcal colonization in the first 2 years of life:results from an SE Asian longitudinal cohort study. Clin Microbiol Infect. (2013) 19:E551–8. doi: 10.1111/1469-0691.12431
12. Abdullahi O, Karani A, Tigoi CC, Mugo D, Kungu S, Wanjiru E, et al. The prevalence and risk factors for pneumococcal colonization of the nasopharynx among children in Kilifi District, Kenya. PLoS ONE. (2012) 7:e30787. doi: 10.1371/journal.pone.0030787
13. Yahiaoui RY, den Heijer CDj, van Bijnen EM, Paget WJ, Pringle M, Goossens H, et al. Prevalence and antibiotic resistance of commensal Streptococcus pneumoniae in nine European countries. Future Microbiol. (2016) 11:737–44. doi: 10.2217/fmb-2015-0011
14. Goldblatt D, Hussain M, Andrews N, Ashton L, Virta C, Melegaro A, et al. Antibody responses to nasopharyngeal carriage of Streptococcus pneumoniae in adults:a longitudinal household study. J Infect Dis. (2005) 192:387–93. doi: 10.1086/431524
15. Ridda I, Macintyre CR, Lindley R, McIntyre PB, Brown M, Oftadeh S, et al. Lack of pneumococcal carriage in the hospitalised elderly. Vaccine. (2010) 28:3902–4. doi: 10.1016/j.vaccine.2010.03.073
16. Oksenhendler E, Gérard L, Fieschi C, Malphettes M, Mouillot G, Jaussaud R, et al. Infections in 252 patients with common variable immunodeficiency. Clin Infect Dis. (2008) 46:1547–54. doi: 10.1086/587669
17. Kumar D, Humar A, Plevneshi A, Siegal D, Franke N, Green K, et al. Invasive pneumococcal disease in adult hematopoietic stem cell transplant recipients:a decade of prospective population-based surveillance. Bone Marrow Transplant. (2008) 41:743–7. doi: 10.1038/sj.bmt.1705964
18. Janoff EN, Breiman RF, Daley CL, Hopewell PC, Janoff EN, et al. Pneumococcal disease during HIV infection. Epidemiologic, clinical, and immunologic perspectives. Ann Intern Med. (1992) 117:314–24. doi: 10.7326/0003-4819-117-4-314
19. Jönsson G, Truedsson L, Sturfelt G, Oxelius VA, Braconier JH, Sjöholm AG, Jonsson G, et al. Hereditary C2 deficiency in Sweden:frequent occurrence of invasive infection, atherosclerosis, and rheumatic disease. Medicine. (2005) 84:23–34. doi: 10.1097/01.md.0000152371.22747.1e
20. Yuste J, Sen A, Truedsson L, Jönsson G, Tay LS, Hyams C, et al. Impaired opsonization with C3b and phagocytosis of Streptococcus pneumoniae in sera from subjects with defects in the classical complement pathway. Infect Immun. (2008) 76:3761–70. doi: 10.1128/IAI.00291-08
21. Melegaro A, Edmunds WJ, Pebody R, Miller E, George R, Melegaro A, et al. The current burden of pneumococcal disease in England and Wales. J Infect. (2006) 52:37–48. doi: 10.1016/j.jinf.2005.02.008
22. Lipsitch M, Whitney CG, Zell E, Kaijalainen T, Dagan R, Malley R. Are anticapsular antibodies the primary mechanism of protection against invasive pneumococcal disease? PLoS Med. (2005) 2:e15. doi: 10.1371/journal.pmed.0020015
23. Prevaes SM, van Wamel WJ, de Vogel CP, Veenhoven RH, van Gils EJ, van Belkum A, et al. Nasopharyngeal colonization elicits antibody responses to staphylococcal and pneumococcal proteins that are not associated with a reduced risk of subsequent carriage. Infect Immun. (2012) 80:2186–93. doi: 10.1128/IAI.00037-12
24. Simell B, Kilpi TM. Kayhty H. Pneumococcal carriage and otitis media induce salivary antibodies to pneumococcal capsular polysaccharides in children. J Infect Dis. (2002) 186:1106–14. doi: 10.1086/344235
25. Wilson R, Cohen JM, Jose RJ, de Vogel C, Baxendale H, Brown JS, Wilson R, et al. Protection against Streptococcus pneumoniae lung infection after nasopharyngeal colonization requires both humoral and cellular immune responses. Mucosal Immunol. (2015) 8:627–39. doi: 10.1038/mi.2014.95
26. Richards L, Ferreira DM, Miyaji EN, Andrew PW, Kadioglu A, Richards L, et al. The immunising effect of pneumococcal nasopharyngeal colonisation; protection against future colonisation and fatal invasive disease. Immunobiology. (2010) 215:251–63. doi: 10.1016/j.imbio.2009.12.004
27. Malley R, Lipsitch M, Stack A, Saladino R, Fleisher G, Pelton S, et al. Intranasal immunization with killed unencapsulated whole cells prevents colonization and invasive disease by capsulated pneumococci. Infect Immun. (2001) 69:4870–3. doi: 10.1128/IAI.69.8.4870-4873.2001
28. Malley R, Trzcinski K, Srivastava A, Thompson CM, Anderson PW, Lipsitch M, Malley R, et al. CD4+ T cells mediate antibody-independent acquired immunity to pneumococcal colonization. Proc Natl Acad Sci USA. (2005) 102:4848–53. doi: 10.1073/pnas.0501254102
29. Malley R, Srivastava A, Lipsitch M, Thompson CM, Watkins C, Tzianabos A, et al. Antibody-independent, interleukin−17A-mediated, cross-serotype immunity to pneumococci in mice immunized intranasally with the cell wall polysaccharide. Infect Immun. (2006) 74:2187–95. doi: 10.1128/IAI.74.4.2187-2195.2006
30. Basset A, Thompson CM, Hollingshead SK, Briles DE, Ades EW, Lipsitch M, et al. Antibody-independent, CD4+ T-cell-dependent protection against pneumococcal colonization elicited by intranasal immunization with purified pneumococcal proteins. Infect Immun. (2007) 75:5460–4. doi: 10.1128/IAI.00773-07
31. Lu YJ, Gross J, Bogaert D, Finn A, Bagrade L, Zhang Q, et al. Interleukin−17A mediates acquired immunity to pneumococcal colonization. PLoS Pathog. (2008) 4:e1000159. doi: 10.1371/journal.ppat.1000159
32. Bogaert D, Weinberger D, Thompson C, Lipsitch M, Malley R, Bogaert D, et al. Impaired innate and adaptive immunity to Streptococcus pneumoniae and its effect on colonization in an infant mouse model. Infect Immun. (2009) 77:1613–22. doi: 10.1128/IAI.00871-08
33. McCool TL, Cate TR, Moy G, Weiser JN, McCool TL, et al. The immune response to pneumococcal proteins during experimental human carriage. J Exp Med. (2002) 195:359–65. doi: 10.1084/jem.20011576
34. Ferreira DM, Neill DR, Bangert M, Gritzfeld JF, Green N, Wright AK, et al. Controlled human infection and rechallenge with Streptococcus pneumoniae reveals the protective efficacy of carriage in healthy adults. Am J Respir Crit Care Med. (2013) 187:855–64. doi: 10.1164/rccm.201212-2277OC
35. Wright AK, Bangert M, Gritzfeld JF, Ferreira DM, Jambo KC, Wright AD, et al. Experimental human pneumococcal carriage augments IL−17A-dependent T-cell defence of the lung. PLoS Pathog. (2013) 9:e1003274. doi: 10.1371/journal.ppat.1003274
36. Zhang Q, Bernatoniene J, Bagrade L, Pollard AJ, Mitchell TJ, Paton JC, et al. Serum and mucosal antibody responses to pneumococcal protein antigens in children:relationships with carriage status. Eur J Immunol. (2006) 36:46–57. doi: 10.1002/eji.200535101
37. Weinberger DM, Dagan R, Givon-Lavi N, Regev-Yochay G, Malley R, Lipsitch M, Weinberger DM, et al. Epidemiologic evidence for serotype-specific acquired immunity to pneumococcal carriage. J Infect Dis. (2008) 197:1511–8. doi: 10.1086/587941
38. McCool TL, Weiser JN. Limited role of antibody in clearance of Streptococcus pneumoniae in a murine model of colonization. Infect Immun. (2004) 72:5807–13. doi: 10.1128/IAI.72.10.5807-5813.2004
39. Zhang Q, Bernatoniene J, Bagrade L, Paton JC, Mitchell TJ, Hammerschmidt S, et al. Regulation of production of mucosal antibody to pneumococcal protein antigens by T-cell-derived gamma interferon and interleukin−10 in children. Infect Immun. (2006) 74:4735–43. doi: 10.1128/IAI.00165-06
40. Lebon A, Verkaik NJ, Labout JA, de Vogel CP, Hooijkaas H, Verbrugh HA, et al. Natural antibodies against several pneumococcal virulence proteins in children during the pre-pneumococcal-vaccine era:the generation R study. Infect Immun. (2011) 79:1680–7. doi: 10.1128/IAI.01379-10
41. Mureithi MW, Finn A, Ota MO, Zhang Q, Davenport V, Mitchell TJ, et al. T cell memory response to pneumococcal protein antigens in an area of high pneumococcal carriage and disease. J Infect Dis. (2009) 200:783–93. doi: 10.1086/605023
42. McAleer JP, Kolls JK. Directing traffic:IL−17 and IL−22 coordinate pulmonary immune defense. Immunol Rev. (2014) 260:129–44. doi: 10.1111/imr.12183
43. Curtis MM, Way SS. Interleukin−17 in host defence against bacterial, mycobacterial and fungal pathogens. Immunology. (2009) 126:177–85. doi: 10.1111/j.1365-2567.2008.03017.x
44. Macleod CM, Hodges RG. Prevention of pneumococcal pneumonia by immunization with specific capsular polysaccharides. J Exp Med. (1945) 82:445–65. doi: 10.1084/jem.82.6.445
45. Whitney CG. Preventing pneumococcal disease. ACIP recommends pneumococcal polysaccharide vaccine for all adults age > or = 65. Geriatrics. (2003) 58:20–2, 25.
46. Janoff EN, Musher DM. Streptococcus pneumoniae, in Mandell, Douglas, and Bennett's Principles and Practice of Infectious Diseases. Philadelphia: Saunders (2015).
47. Casadevall A. Antibody-based therapies for emerging infectious diseases. Emerg Infect Dis. (1996) 2:200–8. doi: 10.3201/eid0203.960306
48. Bull CG. The mechanism of the curative action of antipneumococcus serum. J Exp Med. (1915) 22:457–64. doi: 10.1084/jem.22.4.457
49. Casadevall A, Scharff MD. Serum therapy revisited:animal models of infection and development of passive antibody therapy. Antimicrob Agents Chemother. (1994) 38:1695–702. doi: 10.1128/AAC.38.8.1695
50. Bullowa JG, Wilcox C. Therapeutic serum for pneumococcus type V (Cooper) pneumonia. J Clin Invest. (1936) 15:711–4. doi: 10.1172/JCI100824
52. Cobey S, Lipsitch M. Niche and neutral effects of acquired immunity permit coexistence of pneumococcal serotypes. Science. (2012) 335:1376–80. doi: 10.1126/science.1215947
53. Roche AM, King SJ. Weiser JN. Live attenuated Streptococcus pneumoniae strains induce serotype-independent mucosal and systemic protection in mice. Infect Immun. (2007) 75:2469–75. doi: 10.1128/IAI.01972-06
54. Croucher NJ, Campo JJ, Le TQ, Liang X, Bentley SD, Hanage WP, et al. Diverse evolutionary patterns of pneumococcal antigens identified by pangenome-wide immunological screening. Proc Natl Acad Sci USA. (2017) 114:E357–66. doi: 10.1073/pnas.1613937114
55. Giefing C, Meinke AL, Hanner M, Henics T, Bui MD, Gelbmann D, et al. Discovery of a novel class of highly conserved vaccine antigens using genomic scale antigenic fingerprinting of pneumococcus with human antibodies. J Exp Med. (2008) 205:117–31. doi: 10.1084/jem.20071168
56. Moffitt KL, Gierahn TM, Lu YJ, Gouveia P, Alderson M, Flechtner JB, et al. T(H)17-based vaccine design for prevention of Streptococcus pneumoniae colonization. Cell Host Microbe. (2011) 9:158–65. doi: 10.1016/j.chom.2011.01.007
57. Trzcinski K, Thompson C, Malley R, Lipsitch M. Antibodies to conserved pneumococcal antigens correlate with, but are not required for, protection against pneumococcal colonization induced by prior exposure in a mouse model. Infect Immun. (2005) 73:7043–6. doi: 10.1128/IAI.73.10.7043-7046.2005
58. Zhang Z, Clarke TB, Weiser JN. Cellular effectors mediating Th17-dependent clearance of pneumococcal colonization in mice. J Clin Invest. (2009) 119:1899–909. doi: 10.1172/JCI36731
59. Malley R, Morse SC, Leite LC, Areas AP, Ho PL, Kubrusly FS, et al. Multiserotype protection of mice against pneumococcal colonization of the nasopharynx and middle ear by killed nonencapsulated cells given intranasally with a nontoxic adjuvant. Infect Immun. (2004) 72:4290–2. doi: 10.1128/IAI.72.7.4290-4292.2004
60. van Rossum AM, Lysenko ES. Weiser JN. Host and bacterial factors contributing to the clearance of colonization by Streptococcus pneumoniae in a murine model. Infect Immun. (2005) 73:7718–26. doi: 10.1128/IAI.73.11.7718-7726.2005
61. Briles DE, Hollingshead SK, Paton JC, Ades EW, Novak L, van Ginkel FW, et al. Immunizations with pneumococcal surface protein A and pneumolysin are protective against pneumonia in a murine model of pulmonary infection with Streptococcus pneumoniae. J Infect Dis. (2003) 188:339–48. doi: 10.1086/376571
62. Balachandran P, Brooks-Walter A, Virolainen-Julkunen A, Hollingshead SK, Briles DE, Balachandran P, et al. Role of pneumococcal surface protein C in nasopharyngeal carriage and pneumonia and its ability to elicit protection against carriage of Streptococcus pneumoniae. Infect Immun. (2002) 70:2526–34. doi: 10.1128/IAI.70.5.2526-2534.2002
63. Roche AM, Richard AL, Rahkola JT, Janoff EN, Weiser JN, Roche AM, et al. Antibody blocks acquisition of bacterial colonization through agglutination. Mucosal Immunol. (2015) 8:176–85. doi: 10.1038/mi.2014.55
64. Zangari T, Wang Y, Weiser JN. Streptococcus pneumoniae Transmission is blocked by type-specific immunity in an infant mouse model. MBio. (2017) 8:e00188–17. doi: 10.1128/mBio.00188-17
65. Pido-Lopez J, Kwok WW, Mitchell TJ, Heyderman RS, Williams NA, Pido-Lopez J, et al. Acquisition of pneumococci specific effector and regulatory Cd4+ T cells localising within human upper respiratory-tract mucosal lymphoid tissue. PLoS Pathog. (2011) 7:e1002396. doi: 10.1371/journal.ppat.1002396
66. Zhang Q, Leong SC, McNamara PS, Mubarak A, Malley R, Finn A, Zhang Q, et al. Characterisation of regulatory T cells in nasal associated lymphoid tissue in children:relationships with pneumococcal colonization. PLoS Pathog. (2011) 7:e1002175. doi: 10.1371/journal.ppat.1002175
67. Lundgren A, Bhuiyan TR, Novak D, Kaim J, Reske A, Lu YJ, et al. Characterization of Th17 responses to Streptococcus pneumoniae in humans:comparisons between adults and children in a developed and a developing country. Vaccine. (2012) 30:3897–907. doi: 10.1016/j.vaccine.2012.03.082
68. Mitsi E, Roche AM, Reiné J, Zangari T, Owugha JT, Pennington SH, et al. Agglutination by anti-capsular polysaccharide antibody is associated with protection against experimental human pneumococcal carriage. Mucosal Immunol. (2017) 10:385–94. doi: 10.1038/mi.2016.71
69. Wright AK, Ferreira DM, Gritzfeld JF, Wright AD, Armitage K, Jambo KC, et al. Human nasal challenge with Streptococcus pneumoniae is immunising in the absence of carriage. PLoS Pathog. (2012) 8:e1002622. doi: 10.1371/journal.ppat.1002622
70. Cohen JM, Khandavilli S, Camberlein E, Hyams C, Baxendale HE, Brown JS, Cohen JM, et al. Protective contributions against invasive Streptococcus pneumoniae pneumonia of antibody and Th17-cell responses to nasopharyngeal colonisation. PLoS ONE. (2011) 6:e25558. doi: 10.1371/journal.pone.0025558
71. Bou Ghanem EN, Maung NHT, Siwapornchai N, Goodwin AE, Clark S, Muñoz-Elías EJ, et al. Nasopharyngeal exposure to Streptococcus pneumoniae induces extended age-dependent protection against pulmonary infection mediated by antibodies and CD138(+) cells. J Immunol. (2018) 200:3739–51. doi: 10.4049/jimmunol.1701065
72. Brown JS, Hussell T, Gilliland SM, Holden DW, Paton JC, Ehrenstein MR, et al. The classical pathway is the dominant complement pathway required for innate immunity to Streptococcus pneumoniae infection in mice. Proc Natl Acad Sci USA. (2002) 99:16969–74. doi: 10.1073/pnas.012669199
73. Kang YS, Do Y, Lee HK, Park SH, Cheong C, Lynch RM, et al. A dominant complement fixation pathway for pneumococcal polysaccharides initiated by SIGN-R1 interacting with C1q. Cell. (2006) 125:47–58. doi: 10.1016/j.cell.2006.01.046
74. Gerlini A, Colomba L, Furi L, Braccini T, Manso AS, Pammolli A, et al. The role of host and microbial factors in the pathogenesis of pneumococcal bacteraemia arising from a single bacterial cell bottleneck. PLoS Pathog. (2014) 10:e1004026. doi: 10.1371/journal.ppat.1004026
75. Wilson R, et al. Naturally acquired human immunity to pneumococcus is dependent on antibody to protein antigens. PLoS Pathog. (2017) 13:e1006137. doi: 10.1371/journal.ppat.1006137
76. Quinti I, Soresina A, Guerra A, Rondelli R, Spadaro G, Agostini C, et al. Effectiveness of immunoglobulin replacement therapy on clinical outcome in patients with primary antibody deficiencies:results from a multicenter prospective cohort study. J Clin Immunol. (2011) 31:315–22. doi: 10.1007/s10875-011-9511-0
77. Pourpak Z, Aghamohammadi A, Sedighipour L, Farhoudi A, Movahedi M, Gharagozlou M, et al. Effect of regular intravenous immunoglobulin therapy on prevention of pneumonia in patients with common variable immunodeficiency. J Microbiol Immunol Infect. (2006) 39:114–20.
78. Wang Y, Jiang B, Guo Y, Li W, Tian Y, Sonnenberg GF, et al. Cross-protective mucosal immunity mediated by memory Th17 cells against Streptococcus pneumoniae lung infection. Mucosal Immunol. (2017) 10:250–9. doi: 10.1038/mi.2016.41
79. Smith NM, Wasserman GA, Coleman FT, Hilliard KL, Yamamoto K, Lipsitz E, et al. Regionally compartmentalized resident memory T cells mediate naturally acquired protection against pneumococcal pneumonia. Mucosal Immunol. (2018) 11:220–35. doi: 10.1038/mi.2017.43
80. Rosen FS, Cooper MD, Wedgwood RJ. The primary immunodeficiencies. N Engl J Med. (1995) 333:431–40. doi: 10.1056/NEJM199508173330707
81. Dworkin MS, Ward JW, Hanson DL, Jones JL, Kaplan JE, Dworkin MS, et al. Pneumococcal disease among human immunodeficiency virus-infected persons:incidence, risk factors, and impact of vaccination. Clin Infect Dis. (2001) 32:794–800. doi: 10.1086/319218
82. Milner JD, Brenchley JM, Laurence A, Freeman AF, Hill BJ, Elias KM, et al. Impaired T(H)17 cell differentiation in subjects with autosomal dominant hyper-IgE syndrome. Nature. (2008) 452:773–6. doi: 10.1038/nature06764
83. Perreau M, Vigano S, Bellanger F, Pellaton C, Buss G, Comte D, et al. Exhaustion of bacteria-specific CD4 T cells and microbial translocation in common variable immunodeficiency disorders. J Exp Med. (2014) 211:2033–45. doi: 10.1084/jem.20140039
84. Esposito S, Mari D, Bergamaschini L, Orenti A, Terranova L, Ruggiero L, et al. Pneumococcal colonization in older adults. Immun Ageing. (2016) 13:2. doi: 10.1186/s12979-016-0057-0
85. O'Brien KL, Millar EV, Zell ER, Bronsdon M, Weatherholtz R, Reid R, et al. Effect of pneumococcal conjugate vaccine on nasopharyngeal colonization among immunized and unimmunized children in a community-randomized trial. J Infect Dis. (2007) 196:1211–20. doi: 10.1086/521833
86. Lindstrand A, Galanis I, Darenberg J, Morfeldt E, Naucler P, Blennow M, et al. Unaltered pneumococcal carriage prevalence due to expansion of non-vaccine types of low invasive potential 8 years after vaccine introduction in Stockholm, Sweden. Vaccine. (2016) 34:4565–71. doi: 10.1016/j.vaccine.2016.07.031
87. Puchta A, Naidoo A, Verschoor CP, Loukov D, Thevaranjan N, Mandur TS, et al. TNF drives monocyte dysfunction with age and results in impaired anti-pneumococcal immunity. PLoS Pathog. (2016) 12:e1005368. doi: 10.1371/journal.ppat.1005368
88. Boyd AR, Orihuela CJ. Dysregulated inflammation as a risk factor for pneumonia in the elderly. Aging Dis. (2011) 2:487–500.
89. Krone CL, Trzcinski K, Zborowski T, Sanders EA, Bogaert D, Krone CL, et al. Impaired innate mucosal immunity in aged mice permits prolonged Streptococcus pneumoniae colonization. Infect Immun. (2013) 81:4615–25. doi: 10.1128/IAI.00618-13
90. Adler H, Ferreira DM, Gordon SB, Rylance J, Adler H, et al. Pneumococcal capsular polysaccharide immunity in the elderly. Clin Vaccine Immunol. (2017) 24:e00004–17. doi: 10.1128/CVI.00004-17
91. Shi Y, Yamazaki T, Okubo Y, Uehara Y, Sugane K, Agematsu K, Shi Y, et al. Regulation of aged humoral immune defense against pneumococcal bacteria by IgM memory B cell. J Immunol. (2005) 175:3262–7. doi: 10.4049/jimmunol.175.5.3262
92. Glennie SJ, Sepako E, Mzinza D, Harawa V, Miles DJ, Jambo KC, et al. Impaired CD4 T cell memory response to Streptococcus pneumoniae precedes CD4 T cell depletion in HIV-infected Malawian adults. PLoS ONE. (2011) 6:e25610. doi: 10.1371/journal.pone.0025610
Keywords: immunity, pneumococcus (Streptococcus pneumoniae), natural acquired immunity, pneumonia, protection
Citation: Ramos-Sevillano E, Ercoli G and Brown JS (2019) Mechanisms of Naturally Acquired Immunity to Streptococcus pneumoniae. Front. Immunol. 10:358. doi: 10.3389/fimmu.2019.00358
Received: 15 August 2018; Accepted: 12 February 2019;
Published: 01 March 2019.
Edited by:
Jesús Gonzalo-Asensio, University of Zaragoza, SpainReviewed by:
Yukihiro Akeda, Osaka University, JapanJason W. Rosch, St. Jude Children's Research Hospital, United States
Norbert W. Suttorp, Charité Medical University of Berlin, Germany
Copyright © 2019 Ramos-Sevillano, Ercoli and Brown. This is an open-access article distributed under the terms of the Creative Commons Attribution License (CC BY). The use, distribution or reproduction in other forums is permitted, provided the original author(s) and the copyright owner(s) are credited and that the original publication in this journal is cited, in accordance with accepted academic practice. No use, distribution or reproduction is permitted which does not comply with these terms.
*Correspondence: Jeremy S. Brown, amVyZW15LmJyb3duQHVjbC5hYy51aw==