- 1Division of Gastroenterology, Departments of Pediatrics, The University of Texas Health Science Center at Houston-McGovern Medical School, Houston, TX, United States
- 2Department of Bioinformatics & Computational Biology, The University of Texas MD Anderson Cancer Center, Houston, TX, United States
- 3Department of Microbiology, Immunology & Parasitology, Louisiana State University, School of Medicine, New Orleans, LA, United States
- 4Pathology and Laboratory Medicine, University of Texas Health Science Center at Houston-McGovern Medical School, Houston, TX, United States
- 5Internal Medicine, Division of Infectious Diseases, The University of Texas Health Science Center at Houston-McGovern Medical School, Houston, TX, United States
- 6Neurology, The University of Texas Health Science Center at Houston-McGovern Medical School, Houston, TX, United States
The gut microbiome plays an important role in immune function and has been implicated in multiple sclerosis (MS). However, how and if the modulation of microbiota can prevent or treat MS remain largely unknown. In this study, we showed that probiotic Lactobacillus reuteri DSM 17938 (L. reuteri) ameliorated the development of murine experimental autoimmune encephalomyelitis (EAE), a widely used animal model of MS, a model which is primarily mediated by TH17 and TH1 cells. We discovered that L. reuteri treatment reduced TH1/TH17 cells and their associated cytokines IFN-γ/IL-17 in EAE mice. We also showed that the loss of diversity of gut microbiota induced by EAE was largely restored by L. reuteri treatment. Taxonomy-based analysis of gut microbiota showed that three “beneficial” genera Bifidobacterium, Prevotella, and Lactobacillus were negatively correlated with EAE clinical severity, whereas the genera Anaeroplasma, Rikenellaceae, and Clostridium were positively correlated with disease severity. Notably, L. reuteri treatment coordinately altered the relative abundance of these EAE-associated taxa. In conclusion, probiotic L. reuteri changed gut microbiota to modulate immune responses in EAE, making it a novel candidate in future studies to modify the severity of MS.
Introduction
Multiple sclerosis (MS) is an autoimmune disease of the central nervous system (CNS) which causes neurological disability in up to two million young adults worldwide (1, 2). The key pathological features of MS include axonal loss, demyelination, gliosis, and a progressive inflammatory reaction involving both the adaptive and the innate immune system (3, 4). During the course of MS, activated autoreactive T cells have been proposed to differentiate into CD4+ T cells characterized by the production of interferon-γ (IFN-γ) by [T helper 1 (TH1) cells] and/or interleukin (IL)-17 by IL-17-producing (TH17) cells. These T cells successively induce inflammatory lesions distributed throughout the CNS (2). Infiltration of TH1 and TH17 CD4+ T cells into the CNS is considered an important contributor to the immunopathogenesis of MS (5).
Interestingly, growing evidence from both rodent and human studies suggests that the gut microbiota contributes to the pathogenesis of MS (6–9). In human with MS, an abnormal profile of gut microbiota (dysbiosis) may be one of the several factors involved in the pathogenesis and progression of MS (7–9). In a rodent model of MS, experimental autoimmune encephalomyelitis (EAE), investigative groups have shown that an alteration of the gut microbiota plays a critical role in the pathogenesis of the disease. Supporting this postulate, two studies showed that oral antibiotic administration reduced the severity of EAE (10, 11). Moreover, two studies revealed that germ-free mice were resistant to the development of EAE (6, 12). However, whether remodeling intestinal microbiota can improve EAE symptoms and markers of brain and systemic inflammation remains unknown.
Our previous studies showed that Lactobacillus reuteri DSM 17938 (L. reuteri) can modulate microbiota and inhibit autoimmunity in an autoimmune disease caused by regulatory T cell (Treg) deficiency (13). In this study, we investigated the effect of L. reuteri on EAE and EAE-associated gut microbiota.
Materials and Methods
Animals
Female wild-type (WT) C57BL/6 (10 weeks-old) mice were purchased from Jackson Laboratories and allowed to acclimatize for 2–3 weeks before experimentation. The mice were housed in groups in polycarbonate cages with free access to a standard diet and water in the specific pathogen free (SPF) animal facility at The University of Texas Health Science Center at Houston. This study was carried out in accordance with the recommendations of the Guide for the Care and Use of Laboratory Animals (NIH) and The Institutional Animal Care and Use Committee (IACUC). The protocol was approved by the IACUC (protocol numbers: AWC-15-0051 and AWC-18-0051).
Induction and Assessment of EAE
EAE was induced in female mice by using the Hooke Kit™ MOG35−55/CFA Emulsion PTX kit (Hooke Laboratories), according to the manufacturer's protocol. In brief, mice were immunized with an emulsion of myelin oligodendrocyte glycoprotein peptide (MOG35−55) in complete Freund's adjuvant (CFA) by subcutaneous injection into two different sites on each hind flank, followed by intraperitoneal administration of pertussis toxin (PTX) in phosphate-buffered saline (PBS) after 2 h MOG35−55 immunization. Then PTX was given again on the following day (14). The mice were examined for clinical signs of EAE in a blinded fashion daily from 7 to 20 days after immunization. EAE was scored on scale 0 to 5 (15). Specifically, we assigned Score 0, no obvious changes in motor function compared to normal control mice; Score 1, limp tail; Score 2, limp tail, and weakness of hind legs; Score 3, limp tail and complete paralysis of hind legs; Score 4, limp tail, complete hind leg and partial front leg paralysis, and Score 5, spontaneously rolling in the cage. We assigned mice “in-between” scores (i.e., 0.5, 1.5, 2.5, 3.5) when the clinical picture best fit between two defined scores.
L. reuteri Treatment of EAE Mice
L. reuteri, originally isolated from human breast milk, was provided by BioGaia AB (Stockholm, Sweden) and cultured anaerobically in De Man, Rogosa Sharpe (MRS, Fisher Scientific, Pittsburgh, PA) media as described previously (16). Each mouse was assigned to either (a) MRS media as a control (EAE), or (b) L. reuteri (EAE+LR), as well as normal control group (Ctrl). On the day 0, mice were given by gavage MRS (100 μl) or L. reuteri [108 colony-forming unit (CFU), 100 μl] followed immediately by the injections of MOG35−55 in CFA, then PTX after 2 h of MOG35−55 injections. On the day 1, EAE mice were given second ip injection of PTX solution. Before the onset of EAE, MRS or L. reuteri were given by gavage, daily, starting from day 0 to day 20 after immunization. We observed the mice daily to evaluate the clinical EAE scores. At the end of the experiments on day 20, blood, spleen, and spinal cord, and colonic contents of each mouse were collected. All samples were analyzed at day 20 post-immunization unless specifically indicated.
Stool Microbial Community Analysis
Colonic contents from Ctrl, EAE, and EAE+LR mice were collected, immediately frozen, and stored at −80°C. The Louisiana State University School of Medicine Microbial Genomics Resource Group (http://metagenomics.lsuhsc.edu) performed sequencing and bioinformatics analysis. Genomic DNA extraction from colonic contents was performed using QIAamp Fast DNA Stool Mini Kit (Qiagen, Germantown, MD) and microbiome 16S rDNA sequencing was performed as previously described (17). The 16S ribosomal DNA hypervariable regions V4 were PCR-amplified using primers V4F GTGCCAGCMGCCGCGGTAA and V4R GGACTACHVGGGTWTCTAAT with Illumina adaptors and molecular barcodes to produce amplicons. Samples were sequenced on Illumina MiSeq (Illumina, San Diego, CA) using V4 sequencing kit. The paired forward and reverse-reads—passed quality control were merged, and then mapped to the SLIVA database to construct OTUs at 97% identity through the UPARSE pipeline (drive5, Tiburon, California) (18). Relative abundance of each OTU was examined at phylum, class, order, family, genus, and species levels. Bacterial alpha and beta diversity metrics, as well as, taxonomic community assessments were produced using QIIME 1.8 (open source, www.qiime.org) (19). The composition of stool microbiota was further analyzed as previously described (20, 21). A systematic search for the genera that correlated with the clinical score was performed by using Spearman's rank correlation coefficient. Random Forest analysis of gut microbiota was performed using the R (http://cran.r-project.org/) package Random Forest (22).
Histopathology
The spinal cord was fixed and stained with hematoxylin and eosin (H&E), CD3 antibody (Agilent, Denmark) and CD68 (Abcam, Cambridge, MA) antibody for histological evaluation by Histology Laboratory of the Department of Pathology and Laboratory Medicine of UT Health Science Center at Houston. Histological quantification was performed by using Image J software (NIH). We measured the area of inflammatory infiltration as indicated in Figure 1C (H&E staining), three areas of lymphocyte infiltration (if presented) from one section slide, with a total of 2–3 sections for one mouse (from one spinal cord); we measured 10 mice in each group. Finally, we calculated the mean of the areas of lymphocyte infiltration (Figure 1D). For CD3 or CD68 expressing cells, we counted CD3 or CD68 staining positive cells in two fields of high power inside the areas of lymphocyte infiltration, with a total of 2–3 sections from each spinal cord. We measured specimens from 10 mice in each group, finally calculating the mean numbers of CD3 or CD68 expressing cells and their standard deviations.
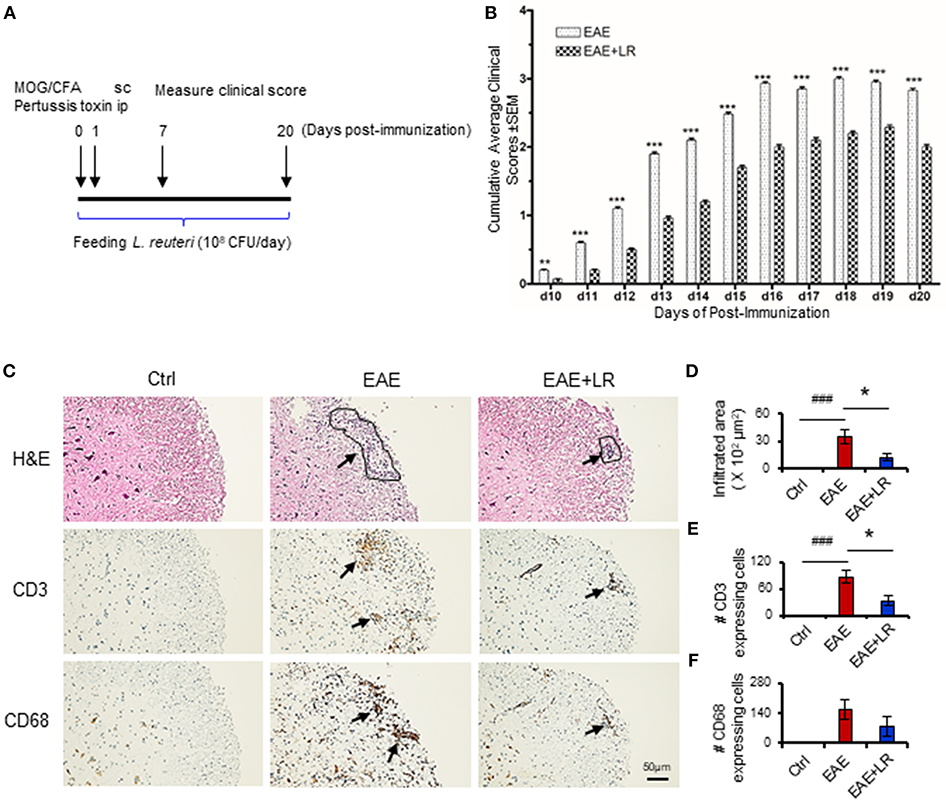
Figure 1. Lactobacillus reuteri ameliorates autoimmune disease in EAE mice. (A) Scheme of the experimental timeline for administering L. reuteri to EAE mice and measuring clinical scores. (B) Clinical scores of C57BL/6J mice with EAE to compare with administered MRS and L. reuteri (n = 37–40 mice per group). (C) The representative images of H&E staining, CD3 staining and CD68 staining of spinal cord slides from Ctrl, EAE, and EAE+LR mice (n = 10 mice per group). Arrows indicate immune cell infiltration, defined as encircled areas in H&E staining of EAE and EAE+LR specimens. (D) The average areas of inflammatory infiltration among group comparisons are shown (see Materials and Methods). (E) The average numbers of CD3+ cell count per defined area of inflammatory infiltration among group comparisons (see Materials and Methods). (F) The average numbers of CD68+ cell count per defined area of inflammatory infiltration among group comparisons (see Materials and Methods). Data are presented as mean ± SEM. *p < 0.05, **p < 0.01, ***p < 0.001. EAE+LR vs. EAE. ###p < 0.001. EAE vs. Ctrl.
In vitro Cell Preparation and Stimulation for Flow Cytometry Analysis
Peripheral blood mononuclear cells (PBMCs) were isolated from whole blood by Ficoll-Paque (GE Healthcare, Chicago, IL). Single-cell suspensions from the spleen were prepared by gently fragmenting and filtering the tissues through 40 μm cell strainers (BD Bioscience, San Jose, CA) into MACS buffer (1x PBS, 0.5% bovine BSA and 2 mM EDTA). For in vitro stimulation, studies of PBMCs and splenic lymphocytes isolated from each mouse were performed in triplicate, with a total of n = 10 mice per group. Briefly, cells were stimulated with 50 ng/mL of phorbol 12-myristate 13-acetate (PMA) and 1 μg/mL of ionomycin in the presence of brefeldin A (5 μ/mL) for 4 h to analyze IFN-γ-producing (TH1) and IL-17-producing (TH17) CD4+ T cells by flow cytometry.
In another set of experiments, splenocytes isolated from mice at day 12 post-immunication were stimulated with 10 μM of MOG35−55 for 2 days to analyze cell proliferation.
In vitro Cell Proliferation Assay
Colorimetric measurement for cell proliferation by using the tetrazolium dye, 2,3-bis (2-methoxy-4-nitro-5-sulfophenyl)-5-[(phenylamino) carbonyl]-2H-tetrazolium hydroxide (XTT) was performed according to the manufacturers protocol (TACSTM XTT Cell Proliferation/Viability Assay, R&D Systems, Inc.,) (23). XTT reduction into formazan, a colored compound, by mitochondrial dehydrogenases is considered proportional to cell number and thus related to cell proliferation after incubation time. After 2 days of 10 μM of MOG35−55 stimulation, 100 μL of cells were incubated with 50 μL of a mixture of XTT and XTT activator followed by incubation of the plates at 37°C in an atmosphere containing 5% CO2 for 4 h. We measured the absorbance at OD450 nm. We calculated the percentage of proliferation as follows: [(OD+MOG-OD−MOG) /OD−MOG] × 100.
Staining Cells for Flow Cytometry Analysis
For evaluation of TH1 and TH17 cells, cells were surface-stained by fluorescein-labeled-CD4. Intracellular staining was performed with a fixation/permeabilization kit, according to the manufacturer's protocol (eBioscience/ThermoFisher, Waltham, MA), and stained with IFN-γ (TH1) and IL-17 (TH17) (BioLegend, San Diego, CA), respectively. The data from all samples were acquired on Gallios flow cytometer (Beckman-Coulter) and analyzed using FlowJo software (FlowJO, LLC) to obtain the percentage of each T cell subsets. The total splenocytes of whole spleen and PBMCs per volume were counted and the absolute numbers of each T cell subsets were calculated, expressed as # of cells per spleen or # of cells/μL in blood.
Plasma Cytokine Assays
Plasma cytokine levels of IFN-γ and IL-17 were assessed using a mouse multi-spot proinflammatory panel kit from Meso Scale Discovery (MSD, Rockville, MD), according to the manufacturer's protocol. Signals were captured and calculated by Imager 2400 (MSD). The levels of cytokines were expressed as picogram/milliliter (pg/mL).
Statistical Analysis
Data are presented as mean ± SEM. Statistical significance was determined by one-way ANOVA corrected for multiple comparisons with Tukey and Dunnett's posttests, or two-way ANOVA for multiple comparisons with a Bonferroni test. We used Fisher's Exact Test to compare the incidence of EAE between groups. The statistical analysis was performed by Prism (GraphPad Software, La Jolla, CA). The correlation between the genera and EAE clinical scores was performed by using Spearman's rank correlation coefficient. Statistical significance was defined as P < 0.05.
Results
L. reuteri Suppresses the Development of EAE in Mice
To determine whether L. reuteri treatment can modulate the progression of EAE, we treated mice with 108 CFU/day of L. reuteri from 0 to 20 days after MOG immunization (Figure 1A). The clinical scores were significantly suppressed in EAE mice with L. reuteri treatment, compared to EAE mice without L. reuteri treatment, starting at day 10 post-immunization and lasting until day 20 (Figure 1B) (Table 1). We calculated the incidence of EAE based on the time point at which the mice developed clinical scores of ≥ 0.5. L. reuteri significantly reduced the incidence of EAE on each day post-immunization (Table 1). In addition, inflammatory cell infiltration was examined in the spinal cord. H&E staining showed that EAE mice had more inflammatory cells in the spinal cord compared with control mice, and L. reuteri treatment consistently reduced inflammatory cell infiltration EAE mice (Figures 1C,D). Immunohistochemical staining indicated that T cells (CD3+) were increased in EAE spinal cord tissues when compared to normal mice, while CD3+ cell staining was significantly decreased in L. reuteri-treated EAE mice (Figures 1C,E). Macrophages (CD68+) were increased in spinal cords of EAE mice when compared to normal mice, but spinal cord CD68+ cells were moderately decreased by L. reuteri treatment (Figures 1C,F). Altogether, these results demonstrate that L. reuteri treatment ameliorates the severity of EAE in mice by reducing inflammatory cell infiltration in the spinal cord.
L. reuteri Decreases TH1/TH17 Cells and Their Associated Cytokines and Reduces MOG35−55-Stimulated Cell Proliferation in EAE Mice
The immunopathology of MS appears to be mediated mainly by TH17 cells but also involves TH1 cells (4). We next examined whether L. reuteri treatment can alter the composition of lymphocytes and their associated cytokines. Flow cytometric analysis demonstrated that the proportions of TH1 and TH17 cells among PBMCs and splenocytes were increased in EAE mice. Both the percentage (Figures 2Aa,c,Ba,c) and absolute numbers (Figures 2Ab,d,Bb,d) of TH1 and TH17 cells were increased in EAE but were reduced after L. reuteri treatment (Figures 2A,B). In addition, L. reuteri treatment significantly reduced the plasma levels of pro-inflammatory cytokines IL-17 and IFN-γ in mice with EAE (Figure 2C). These data indicate that L. reuteri treatment not only decreases the proportion of circulating TH1 and TH17 cells in EAE but also reduces circulating levels of their associated cytokines. In addition, we observed that splenocytes isolated from the mice after 12 day post-immunization with MOG35−55 had increased cell proliferation in vitro, which was decreased by L. reuteri treatment (Figure 2D).
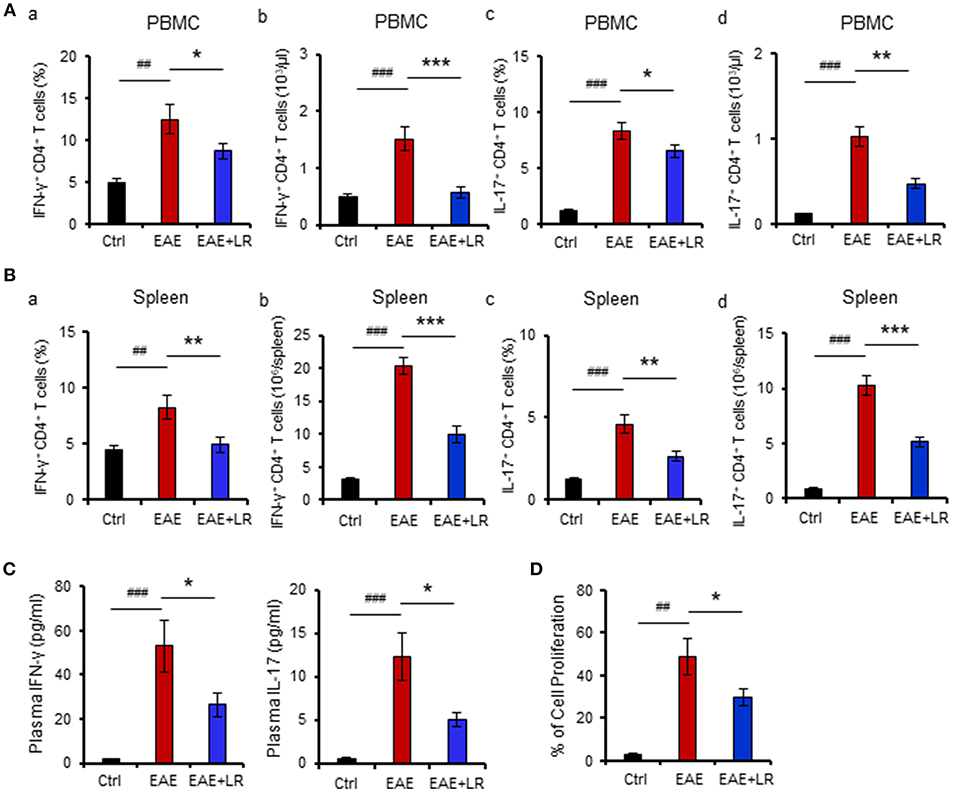
Figure 2. L. reuteri treatment decreases TH1/TH17 cells and their associated cytokines, and reduces MOG35−55-stimulated cell proliferation in EAE mice. (A) PBMCs. (a) the frequency of IFN-γ+ CD4+ T cells (TH1), (b) the absolute number of IFN-γ+ CD4+ T cells, (c) the frequency of IL-17+ CD4+ T cells (TH17), and (d) the absolute number of IL-17+ CD4+ T cells. (B) Spleen. (a) The frequency of IFN-γ+ CD4+ T cells, (b) the absolute number of IFN-γ+ CD4+ T cells, (c) the frequency of IL-17+ CD4+ T cells, and (d) the absolute number of IL-17+ CD4+ T cells. (C) Plasma IL-17 and IFN-γ levels. (D) The percentage of cell proliferation of splenocytes isolated from the mice at d12 post-immunization responded to in vitro MOG35−55 stimulation (see Materials and Methods) in Ctrl, EAE, and EAE+LR mice (n = 10 mice per group). Data are presented as mean ± SEM. In vitro stimulation assays of PBMCs and splenocytes were performed in triplicate. *p < 0.05, **p < 0.01,***p < 0.001. EAE+LR vs. EAE. ##p < 0.01, ###p < 0.001. EAE vs. Ctrl.
L. reuteri Remodels EAE-Associated Intestinal Microbiota in Mice
To determine the microbial populations of colonic contents that exhibited significant differences comparing Ctrl, EAE, and EAE+LR groups, we surveyed fecal bacterial populations by 16s rRNA gene sequencing. The gut microbiota of EAE mice was characterized by less alpha diversity than that of Ctrl mice when measured with PD whole tree; however, other alpha diversity metrics (Chao1, Observed Species, Shannon, and Simpsons) uniformly failed to show any difference in alpha diversity (Figure 3A). Unweighted UniFrac-based 3D principal coordinate analysis (PCoA) revealed a strong effect of L. reuteri on the beta diversity of the gut microbiota in EAE mice (Figure 3B). Remarkably, EAE+LR samples clustered distinctly from Ctrl or EAE samples (Figure 3B), indicating robust differences in the membership of gut bacteria between Ctrl, EAE, and EAE+LR mice.
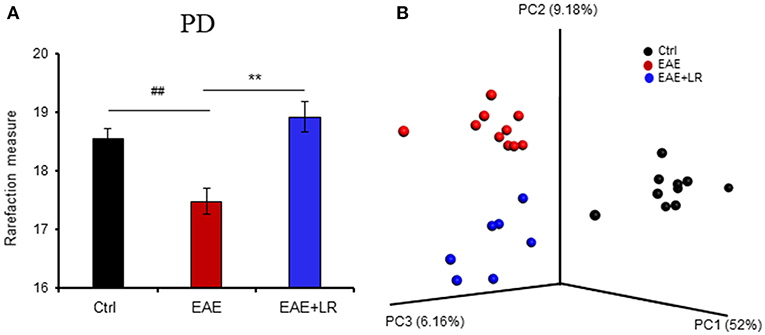
Figure 3. L. reuteri treatment modulates the diversity of the gut microbiota. (A) Gut microbial Phylogenetic Diversity (PD) whole tree analysis, comparing groups of Ctrl, EAE, and EAE+LR mice (n = 7–10 mice per group). (B) Unweighted UniFrac-based 3D PCoA analysis of gut microbiota of Ctrl, EAE, and EAE+LR mice (n = 7–10 mice per group). Data are presented as mean ± SEM. **p < 0.01. EAE+LR vs. EAE. ##p < 0.01. EAE vs. Ctrl.
Taxonomy-based analysis of gut microbiota indicated that the gut microbiota in stool samples from Ctrl, EAE, and EAE+LR mice included four major phyla, Firmicutes, Bacteroidetes, Proteobacteria, and Tenericutes (Figure 4A). The relative abundances of Proteobacteria and Deferribacteres were significantly increased in the colonic contents of EAE mice, while the relative abundance of Bacteroidetes was reduced. L. reuteri treatment reversed the effects of EAE on the relative abundance of these phyla in EAE mice (Figures 4A,B).
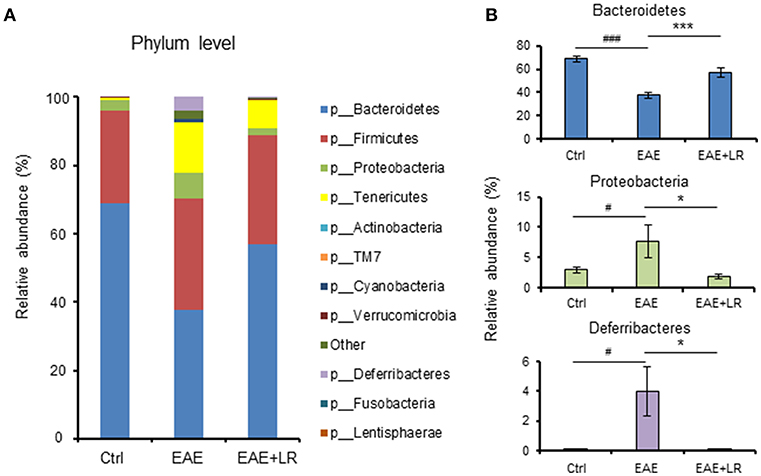
Figure 4. L. reuteri treatment remodels EAE-associated intestinal microbiota at the phylum level. (A) Relative abundance of bacteria at the phylum level for Ctrl, EAE, and EAE+LR mice (n = 7–10 mice per group). (B) Relative abundance of Bacteroidetes, Proteobacteria, and Deferribacteres at the phylum level for Ctrl, EAE, and EAE+LR mice (n = 7–10 mice per group). Data are presented as mean ± SEM. *p < 0.05, ***p < 0.001. EAE+LR vs. EAE. #p < 0.05, ###p < 0.001. EAE vs. Ctrl.
According to our analysis of predominant bacteria (>1% of total bacteria) at the genus level, the relative abundance of Anaeroplasma and Rikenellaceae was increased, while the relative abundance of Prevotella and S24-7 was reduced in EAE mice. After oral feeding of L. reuteri to EAE mice, the percentages of these genera in gut microbiota were improved by L. reuteri treatment (Figures 5A,C).
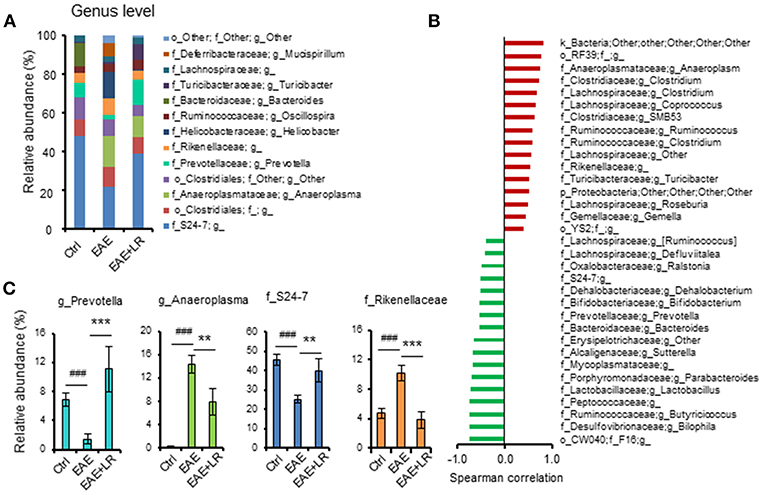
Figure 5. L. reuteri treatment remodels EAE-associated intestinal microbiota at the Genus level. (A) Relative abundance of predominant bacteria (> 1% of total bacteria) at the genus level for Ctrl, EAE, and EAE+LR mice (n = 7–10 mice per group). (B) The Spearman correlation between gut microbiota (o, order; f, family; g, genus) and the EAE clinical scores of all mice. (C) Relative abundance of Prevotella, Anaeroplasma, S24-7, and Rikenellaceae were compared among the mice of Ctrl, EAE, and EAE+LR groups, respectively, (n = 7–10 mice per group). Data are presented as mean ± SEM. **p < 0.01, ***p < 0.001. EAE+LR vs. EAE. ###p < 0.001. EAE vs. Ctrl.
To explore the relationship between gut microbiota and the severity of EAE, we used the Spearman correlation to compare the composition of gut microbiota at the genus level with the clinical scores (Figure 5B). Results showed that 16 genera had a positive correlation (p < 0.05) with clinical scores, while 17 genera had a negative correlation (p < 0.05). Interestingly, Bifidobacterium, Prevotella, Lactobacillus, and S24-7 had a negative correlation, indicating improved clinical scores. Conversely, the genera Clostridium, Anaeroplasma, Ruminococcus, and Rikenellaceae positively correlated with more severe clinical scores. L. reuteri changed the relative abundance of certain microbial taxa relevant to the clinical scores in EAE mice (Figures 5A,C). Altogether, these results indicated that EAE-associated gut microbial dysbiosis could be reprogrammed by oral administration of L. reuteri treatment.
Predictive Model Using Random Forests
We used Random Forests (RF) to build an EAE-predictive model by using the genus-level relative abundance data. The relative importance of each genus in the predictive model was assessed using mean decreasing accuracy. We selected 20 significant genera as the signature gut microbiota to compare with groups Ctrl, EAE, and EAE+LR (Figure 6A). Eight genera came from the phylum Firmicutes and the rest came from the phyla Bacteroidetes, Tenericutes, or Proteobacteria. To test whether these 20 genera had sufficient EAE-predictive power in our sample sets, we performed hierarchical clustering based on the relative abundance of these 20 genera. Results showed that the samples from Ctrl, EAE, or EAE+LR groups clustered together, respectively (Figure 6B). Additionally, we also selected 30 significant genera as the signature gut microbiota to compare with the groups of EAE and EAE+LR treatment, which predicts importance of signature microbiota changed by the disease or by L. reuteri (Figure 6C). Eleven genera came from the phylum Firmicutes, and the rest came mainly from the phyla Proteobacteria and Bacteroidetes. This predictive model confirmed that certain genera had a significant correlation with the clinical scores and were predictive of EAE. For example, Sutterella, Bacteroides, Parabacteroides, Prevotella were all decreased in EAE, whereas Rickenellaceae, Ruminococcus, Anaeroplasma were increased in EAE (Figure 6D). This predictive model additionally confirmed that certain genera were predictive of L. reuteri treatment modifications in EAE, including Prevotella, Rickenellaceae, and Anaeroplasma.
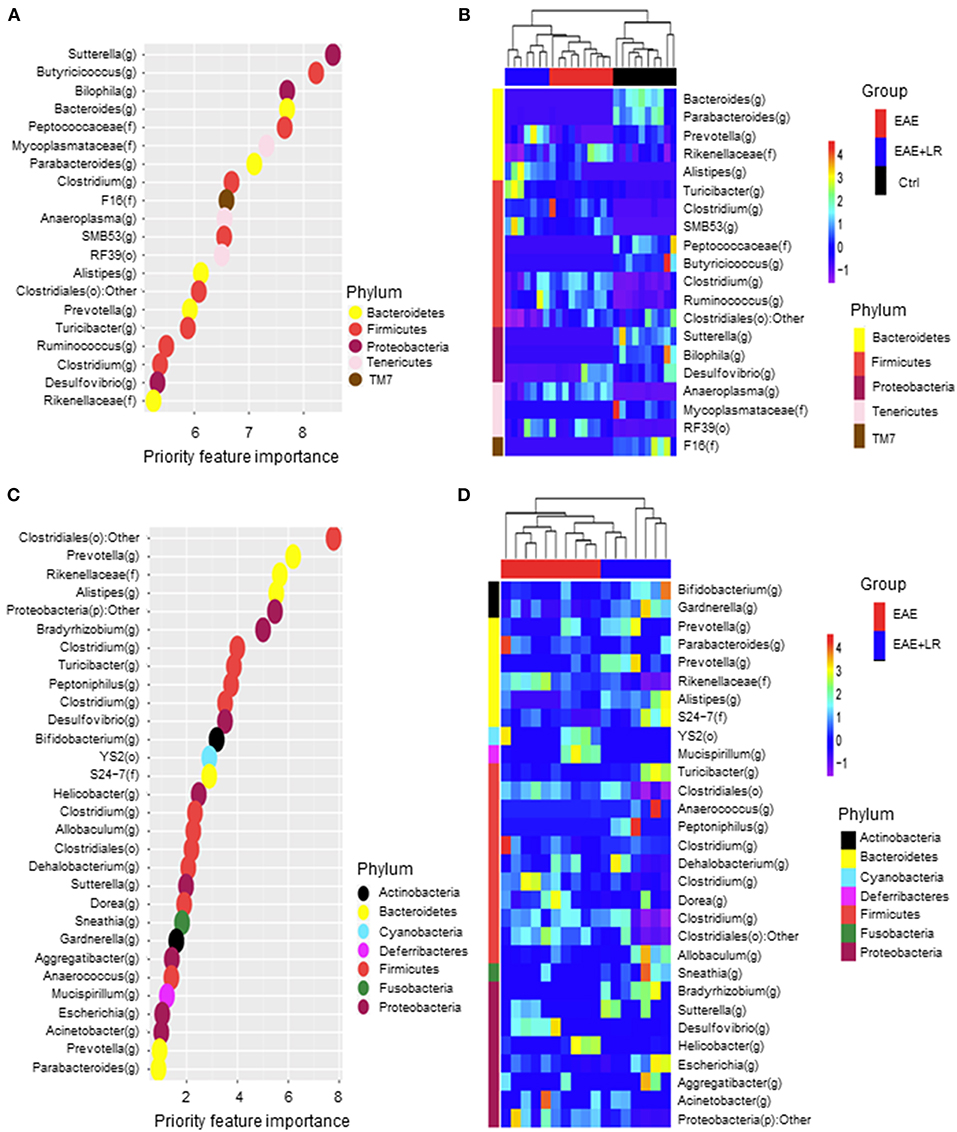
Figure 6. Predictive model based on the relative abundance profile at the genus level using Random Forest analysis. (A) Predictive power of individual genera (Top 20 most important bacteria at genus level) with three group (Ctrl, EAE, and EAE+LR) comparisons assessed by Random Forest (RF) analysis (o, order; f, family; g, genus). (B) Heatmap based on the relative abundance of bacteria from (A) top 20 most important bacteria at the genus level of Ctrl, EAE, and EAE+LR mice. (C) Predictive power of individual genera (Top 30 most important bacteria at genus level) with two group (EAE and EAE+LR) comparisons assessed by RF analysis. (D) Heatmap based on the relative abundance of bacteria from (C) top 30 most important bacteria at the genus level of EAE and EAE+LR mice. Hierarchical clustering shows that Ctrl, EAE, or EAE+LR samples tend to cluster together, respectively.
Discussion
Our current study demonstrates that mice with EAE have a distinct gut microbiota compared to normal mice. Certain gut microbes showed positive or negative correlations with the clinical EAE scores. Probiotic L. reuteri treatment of EAE mice modified the relative abundance of EAE-associated microbiota, reducing the clinical symptoms and inflammation during the EAE development. Probiotics have beneficial effects on the host by regulating the intestinal microbial communities and modulating of inflammatory immune responses—both locally in the gut and systemically. Early administration of L. reuteri reduces pathogen colonization (24) and has the potential to reduce the risk of necrotizing enterocolitis (NEC) in infants (25, 26). In animal models of NEC, we have demonstrated that L. reuteri reduces incidence and severity of NEC via modulation of Toll-like receptor-4 (TLR4) and nuclear factor kappa-light-chain-enhancer of activated B cells (NF-kB) signaling in the intestine (27). L. reuteri coordinately modulates inflammatory effector T cells counterbalanced by Foxp3+ Treg cells in the intestinal mucosa during NEC, a beneficial effect which is mediated by TLR2 (16, 28, 29).
L. reuteri prolongs the survival of mice suffering from Foxp3+ Treg-deficiency-induced autoimmune disease (called the scurfy mouse). We have extensively studied this model of a disease characterized by immune dysregulation, polyendocrinopathy, and enteropathy with X-linked inheritance (IPEX syndrome) that is seen in humans (13). We recently identified a novel mechanism by which this probiotic and its metabolite inosine act on an adenosine receptor expressed on inflammatory T cell to inhibit inflammation (13, 30). Because we observed that the L. reuteri strain 17938 inhibits the differentiations of naïve CD4+ T cells into TH1, TH2 (13), and TH17 cells in vitro (data not shown), we decided to “put this strain to the test” in the EAE model of MS, a condition mediated primarily by TH1 and TH17 cells (31).
We began orally feeding L. reuteri by gavage simultaneously with the first inoculation of MOG35−55 (d0), and we gave the probiotic until the end of the experiment at d20. Mouse EAE symptoms were visible as early as d10. Oral administration of L. reuteri significantly reduced the incidence of EAE and EAE severity scores starting on d10 until d20 post-immunization. Previous studies of probiotics given for EAE used different regimens for prophylaxis. Various authors gave a probiotic daily beginning 3 weeks before (32, 33), 12 days before (34), 7 days before (35–37) or 0 days before the first inoculation of MOG35−55 (that is, on d0) (38). For other MS treatments (medications), study groups have administered preventative treatment on d0 (39–41) or d2-post-immunization (42). Our protocol of simultaneous oral administration at the same time as with MOG35−55 sensitization in this study falls into the prophylactic window. For therapeutic regimens, compounds or probiotics have been administered starting at d11 or d20 post-immunization (39, 42).
Previous studies showed that the effects of probiotic Lactobacilli on EAE autoimmunity are strain-, and EAE model-dependent (43, 44). Lactobacillus casei strain Shirota (LcS) has been reported to be associated with increases in TH1-associated cytokines in a Lewis rat EAE model (35), which raised concerns about the safety of this strain in MS patients. Further studies evaluated the safety of Lactobacillus casei strain Shirota (LcS) together with Bifidobacterium breve strain Yakult (BbY) when given to Lewis rats with EAE. However, in this series of experiments investigators found that neither LcS nor SbY exacerbates EAE (45). Another study demonstrated that even though LcS upregulated peripheral IL-17 responses; it did not exacerbate neurological symptoms in EAE (37). Another probiotic mixture, IRT5, representing a combination of five probiotic strains, Streptococcus thermophilus, L. reuteri, Bifidobacterium bifidium, Lactobacillus acidophilus, and Lactobacillus casei, was given as pretreatment 3 weeks before disease induction. Investigators found that treatment with IRT5 during induction of EAE delayed the disease onset (32). Previous studies demonstrated that Bifidobacterium animalis PTCC1631 in combination with L. plantarum A7 also ameliorated neuroinflammation in the EAE mouse model (38).
Recently, investigators using this MS model tested different probiotic strains and selected mixtures, including Lactobacillus crispatus LMG P-23257, Lactobacillus rhamnosus ATCC 53103, Bifidobacterium animalis subspecies Lactis BB12®, and Bifidobacterium animalis subspecies Lactis LMG S-28195. Their results indicated that selective probiotic mixtures effectively modulate disease symptoms in the EAE model (36). Administration of the selected mixtures altered CD4+ T cell subset balance, inhibiting the pro-inflammatory TH1/TH17 polarization while inducing IL10-producing Foxp3+ Treg cells (36, 38). In our study, L. reuteri reduced the inflammatory infiltration in the spinal cord, especially invading T cells (CD3+) and macrophages (CD68+), reducing IFN-γ-producing TH1 and IL-17-producing TH17 cells. However, we did not observe a difference with respect to the levels of circulating IL4-, and IL-10-producing T cells (and their associated cytokines IL-4 and IL-10 in plasma (data not shown). This finding indicates that strain L. reuteri DSM 17938 specifically acts on TH1 and TH17 subsets in the EAE model.
Other studies in the EAE model which tested a single probiotic strain, Lactobacillus helveticus SBT2171 (33) or another combination probiotic Enterococcus faecium strain L-3 (46) with Prevotella histicola (47) showed differential modulation of immune cells and amelioration of EAE. Gut microbes and their products therefore must participate as key participants in the development of MS. Germ-free mice are fully protected from spontaneous EAE (6), while gut microbiota from MS patients when transferred facilitates the spontaneous development of EAE in mice (48). As final evidence, antibiotics can alter the severity of MS (10, 11).
In the current study, we analyzed the changes of gut microbiota derived from colonic contents, revealing significant differences among the groups in the beta diversity distance matrix (PCoA). For alpha-diversity, among five metrics (Chao1, Observed Species, PD whole tree, Shannon, and Simpson), only the PD whole tree analysis showed significantly differences. At the phylum level, L. reuteri treated EAE mice had restoration toward normal relative abundance of Bacteroidetes, but continued to show reduced relative abundances of Proteobacteria and Deferribacteres. We conclude that L. reuteri promotes the growth of beneficial commensal microbes (Bacteroidetes) and while reducing the abundance of pathobionts (Proteobacteria) or potentially pathogenic (Deferribacteres) gram-negative organisms.
Recent studies noted that the interaction between Bacteroidetes and their animal host is one of mutualism rather than commensalism (49). Mutualism refers to a situation in which both organisms benefit, whereas commensalism refers to a state in which one species benefits but the other is not harmed. Bacteroidetes are also beneficial to the normal development of the gastrointestinal tract (GIT), enhancing the immune system and activation of T-cell mediated responses (50). Furthermore, Bacteroidetes limit the colonization of the gastrointestinal tract by potential pathogenic bacteria (51). Gut Bacteroidetes produce butyrate, facilitate bile acid metabolism, and can transform toxic compounds (52, 53).
At the genus level, several EAE-associated bacteria (Anaeroplasma and Rikenellaceae) were reduced by L. reuteri treatment, at the same time as the low relative abundance of Prevotella and S24-7 rebounded back to normal. Human studies of MS patients have also indicated that the relative abundance of Prevotella and Lactobacilli are decreased compared to healthy controls (7, 8) and these 2 taxa increase after treatment with MS-directed phamacotherapy (7). Interestingly, Prevotella in particular has been associated with phytoestrogen metabolism (54–56). Metabolites derived from phytoestrogens play a critical role in producing anti-inflammatory responses (57). Treatment with certain phytoestrogens can suppress and/or protect mice from EAE (58–60). Future studies should investigate the role of Prevotella-derived phytoestrogen products in the prevention and therapy of autoimmune disease. In addition, Prevotella and Lactobacillus can ferment carbohydrates to yield short chain fatty acids (SCFA) which are known for their beneficial immunoregulatory functions (61–63).
After analyzing the correlation between the significantly changed bacteria with clinical EAE scores, we concluded that among all the bacteria, 16 genera bacteria positively correlated with clinical EAE scores, while 17 negatively correlated with clinical EAE scores. Our data therefore further the understanding EAE–associated bacteria and provide additional information that may assist in modulating gut bacteria to change the development of MS. Toward this end, we used predictive models to confirm the bacterial taxa differentially expressed and their importance in the control of the disease. Prevotella and Rikenellaceae had priority importance to EAE and EAE with LR treatment (Figure 6). They are members of the phylum Bacteroidetes, but as shown in Figure 5, L. reuteri increased the abundance of Prevotella that were decreased in EAE while L. reuteri reduced the abundance of Rikenellaceae. This is significant because Prevotella negatively and Rikenellaceae positively correlated with clinical disease severity. Our results provide clear evidence that resetting gut microbiota should be considered as an adjunctive therapeutic strategy for treating MS.
In summary, we have found alterations in the gut microbiota that correlate with changes of neuroinflammation in a mouse model of MS. A novel therapeutic strategy for MS may consist of the use of probiotics, prebiotics, defined microbial communities, or even fecal microbiota transplantation—aiming to remodel the microbiome in this disease.
Author Contributions
BH, YL, JL, and JR conceived and designed the experiments. BH, TH, JF, SP, and YL performed all experiments and analyzed the data. XT, CT, EB, and ML contributed to microbiota analysis. JL guided EAE mouse model and clinical EAE scoring. MB and JL contributed the spinal cord sample histological processing and analysis. JC and DT assisted flow cytometry and immune cell analysis. BH, YL, XT, CT, MB, JL, DT, and JR wrote the paper and edited the manuscript. All authors read and approved the final manuscript.
Funding
This study was supported by National Institutes of Health/National Center for Complementary and Integrative Health (NIH/NCCIH) grant R01AT007083, and, in part, by US Public Health Service (P30DK56338), which funds the Texas Medical Center Digestive Diseases Center.
Conflict of Interest Statement
The authors declare that the research was conducted in the absence of any commercial or financial relationships that could be construed as a potential conflict of interest.
Acknowledgments
We thank Melissa Stephens (Histology Laboratory at Department of Pathology and Laboratory Medicine of our Institute) for histological technical assistance.
References
1. Frohman EM, Racke MK, Raine CS. Multiple sclerosis–the plaque and its pathogenesis. N Engl J Med. (2006) 354:942–55. doi: 10.1056/NEJMra052130
2. McFarland HF, Martin R. Multiple sclerosis: a complicated picture of autoimmunity. Nat Immunol. (2007) 8:913–9. doi: 10.1038/ni1507
3. Goverman J. Autoimmune T cell responses in the central nervous system. Nat Rev Immunol. (2009) 9:393–407. doi: 10.1038/nri2550
4. Nylander A, Hafler DA. Multiple sclerosis. J Clin Invest. (2012) 122:1180–8. doi: 10.1172/JCI58649
5. Dendrou CA, Fugger L, Friese MA. Immunopathology of multiple sclerosis. Nat Rev Immunol. (2015) 15:545–58. doi: 10.1038/nri3871
6. Berer K, Mues M, Koutrolos M, Rasbi ZA, Boziki M, Johner C, et al. Commensal microbiota and myelin autoantigen cooperate to trigger autoimmune demyelination. Nature. (2011) 479:538–41. doi: 10.1038/nature10554
7. Chen J, Chia N, Kalari KR, Yao JZ, Novotna M, Soldan MM, et al. Multiple sclerosis patients have a distinct gut microbiota compared to healthy controls. Sci Rep. (2016) 6:28484. doi: 10.1038/srep28484
8. Jangi S, Gandhi R, Cox LM, Li N, von GF, Yan R, et al. Alterations of the human gut microbiome in multiple sclerosis. Nat Commun. (2016) 7:12015. doi: 10.1038/ncomms12015
9. Newland PK, Heitkemper M, Zhou Y. The emerging role of the gut microbiome in adult patients with multiple sclerosis. J Neurosci Nurs. (2016) 48:358–64. doi: 10.1097/JNN.0000000000000252
10. Ochoa-Reparaz J, Mielcarz DW, Ditrio LE, Burroughs AR, Foureau DM, Haque-Begum S, et al. Role of gut commensal microflora in the development of experimental autoimmune encephalomyelitis. J Immunol. (2009) 183:6041–50. doi: 10.4049/jimmunol.0900747
11. Yokote H, Miyake S, Croxford JL, Oki S, Mizusawa H, Yamamura T. NKT cell-dependent amelioration of a mouse model of multiple sclerosis by altering gut flora. Am J Pathol. (2008) 173:1714–23. doi: 10.2353/ajpath.2008.080622
12. Lee YK, Menezes JS, Umesaki Y, Mazmanian SK. Proinflammatory T-cell responses to gut microbiota promote experimental autoimmune encephalomyelitis. Proc Natl Acad Sci USA. (2011) 108(Suppl 1):4615–22. doi: 10.1073/pnas.1000082107
13. He B, Hoang TK, Wang T, Ferris M, Taylor CM, Tian X, et al. Resetting microbiota by Lactobacillus reuteri inhibits T reg deficiency-induced autoimmunity via adenosine A2A receptors. J Exp Med. (2017) 214:107–23. doi: 10.1084/jem.20160961
14. Bittner S, Afzali AM, Wiendl H, Meuth SG. Myelin oligodendrocyte glycoprotein (MOG35-55) induced experimental autoimmune encephalomyelitis (EAE) in C57BL/6 mice. J Vis Exp. (2014) 86:51275. doi: 10.3791/51275
15. Miller SD, Karpus WJ, Davidson TS. Experimental autoimmune encephalomyelitis in the mouse. Curr Protoc Immunol. (2010) Chapter 15, Unit.1. doi: 10.1002/0471142735.im1501s88
16. Liu Y, Fatheree NY, Dingle BM, Tran DQ, Rhoads M. Lactobacillus reuteri DSM 17938 changes the frequency of Foxp3+ regulatory T cells in the intestine and mesenteric lymph node in experimental necrotizing enterocolitis. PLoS ONE. (2013) 8:e56547. doi: 10.1371/journal.pone.0056547
17. Bruce-Keller AJ, Salbaum JM, Luo M, Blanchard E, Taylor CM, Welsh DA, et al. Obese-type gut microbiota induce neurobehavioral changes in the absence of obesity. Biol Psychiatry. (2015) 77:607–15. doi: 10.1016/j.biopsych.2014.07.012
18. Edgar RC. UPARSE: highly accurate OTU sequences from microbial amplicon reads. Nat Methods. (2013) 10:996–8. doi: 10.1038/nmeth.2604
19. Caporaso JG, Kuczynski J, Stombaugh J, Bittinger K, Bushman FD, Costello EK, et al. QIIME allows analysis of high-throughput community sequencing data. Nat Methods. (2010) 7:335–6. doi: 10.1038/nmeth.f.303
20. He B, Xu W, Santini PA, Polydorides AD, Chiu A, Estrella J, et al. Intestinal bacteria trigger T cell-independent immunoglobulin A(2) class switching by inducing epithelial-cell secretion of the cytokine APRIL. Immunity. (2007) 26:812–26. doi: 10.1016/j.immuni.2007.04.014
21. He B, Nohara K, Ajami NJ, Michalek RD, Tian X, Wong M, et al. Transmissible microbial and metabolomic remodeling by soluble dietary fiber improves metabolic homeostasis. Sci Rep. (2015) 5:10604. doi: 10.1038/srep10604
22. Schloss PD, Iverson KD, Petrosino JF, Schloss SJ. The dynamics of a family's gut microbiota reveal variations on a theme. Microbiome. (2014) 2:25. doi: 10.1186/2049-2618-2-25
23. Roehm NW, Rodgers GH, Hatfield SM, Glasebrook AL. An improved colorimetric assay for cell proliferation and viability utilizing the tetrazolium salt XTT. J Immunol Methods. (1991) 142:257–65. doi: 10.1016/0022-1759(91)90114-U
24. Savino F, Fornasero S, Ceratto S, De MA, Mandras N, Roana J, et al. Probiotics and gut health in infants: A preliminary case-control observational study about early treatment with Lactobacillus reuteri DSM 17938. Clin Chim Acta. (2015) 451:82–7. doi: 10.1016/j.cca.2015.02.027
25. Athalye-Jape G, Rao S, Patole S. Lactobacillus reuteri DSM 17938 as a Probiotic for preterm neonates: a strain-specific systematic review. JPEN J Parenter Enteral Nutr. (2016) 40:783–94. doi: 10.1177/0148607115588113
26. Hunter C, Dimaguila MA, Gal P, Wimmer JE Jr, Ransom JL, Carlos RQ, et al. Effect of routine probiotic, Lactobacillus reuteri DSM 17938, use on rates of necrotizing enterocolitis in neonates with birthweight < 1000 grams: a sequential analysis. BMC Pediatr. (2012) 12:142. doi: 10.1186/1471-2431-12-142
27. Liu Y, Fatheree NY, Mangalat N, Rhoads JM. Lactobacillus reuteri strains reduce incidence and severity of experimental necrotizing enterocolitis via modulation of TLR4 and NF-kappaB signaling in the intestine. Am J Physiol Gastrointest Liver Physiol. (2012) 302:G608–17. doi: 10.1152/ajpgi.00266.2011
28. Hoang TK, He B, Wang T, Tran DQ, Rhoads JM, Liu Y. Protective effect of Lactobacillus reuteri DSM 17938 against experimental necrotizing enterocolitis is mediated by Toll-like receptor 2. Am J Physiol Gastrointest Liver Physiol. (2018) 315:G231–40. doi: 10.1152/ajpgi.00084.2017
29. Liu Y, Tran DQ, Fatheree NY, Marc RJ. Lactobacillus reuteri DSM 17938 differentially modulates effector memory T cells and Foxp3+ regulatory T cells in a mouse model of necrotizing enterocolitis. Am J Physiol Gastrointest Liver Physiol. (2014) 307:G177–86. doi: 10.1152/ajpgi.00038.2014
30. He B, Hoang TK, Tran DQ, Rhoads JM, Liu Y. Adenosine A2A receptor deletion blocks the beneficial effects of Lactobacillus reuteri in regulatory T-deficient scurfy mice. Front Immunol. (2017) 8:1680. doi: 10.3389/fimmu.2017.01680
31. Kaskow BJ, Baecher-Allan C. Effector T cells in multiple sclerosis. Cold Spring Harb Perspect Med. (2018) 8:a029025. doi: 10.1101/cshperspect.a029025
32. Kwon HK, Kim GC, Kim Y, Hwang W, Jash A, Sahoo A, et al. Amelioration of experimental autoimmune encephalomyelitis by probiotic mixture is mediated by a shift in T helper cell immune response. Clin Immunol. (2013) 146:217–27. doi: 10.1016/j.clim.2013.01.001
33. Yamashita M, Ukibe K, Matsubara Y, Hosoya T, Sakai F, Kon S, et al. Lactobacillus helveticus SBT2171 attenuates experimental autoimmune encephalomyelitis in mice. Front Microbiol. (2017) 8:2596. doi: 10.3389/fmicb.2017.02596
34. Lavasani S, Dzhambazov B, Nouri M, Fak F, Buske S, Molin G, et al. A novel probiotic mixture exerts a therapeutic effect on experimental autoimmune encephalomyelitis mediated by IL-10 producing regulatory T cells. PLoS ONE. (2010) 5:e9009. doi: 10.1371/journal.pone.0009009
35. Baken KA, Ezendam J, Gremmer ER, de KA, Pennings JL, Matthee B, et al. Evaluation of immunomodulation by Lactobacillus casei Shirota: immune function, autoimmunity and gene expression. Int J Food Microbiol. (2006) 112:8–18. doi: 10.1016/j.ijfoodmicro.2006.06.009
36. Consonni A, Cordiglieri C, Rinaldi E, Marolda R, Ravanelli I, Guidesi E, et al. Administration of bifidobacterium and lactobacillus strains modulates experimental myasthenia gravis and experimental encephalomyelitis in Lewis rats. Oncotarget. (2018) 9:22269–87. doi: 10.18632/oncotarget.25170
37. Kobayashi T, Suzuki T, Kaji R, Serata M, Nagata T, Ando M, et al. Probiotic upregulation of peripheral IL-17 responses does not exacerbate neurological symptoms in experimental autoimmune encephalomyelitis mouse models. Immunopharmacol Immunotoxicol. (2012) 34:423–33. doi: 10.3109/08923973.2010.617755
38. Salehipour Z, Haghmorad D, Sankian M, Rastin M, Nosratabadi R, Soltan Dallal MM, et al. Bifidobacterium animalis in combination with human origin of Lactobacillus plantarum ameliorate neuroinflammation in experimental model of multiple sclerosis by altering CD4+ T cell subset balance. Biomed Pharmacother. (2017) 95:1535–48. doi: 10.1016/j.biopha.2017.08.117
39. Green LK, Zareie P, Templeton N, Keyzers RA, Connor B, La Flamme AC. Enhanced disease reduction using clozapine, an atypical antipsychotic agent, and glatiramer acetate combination therapy in experimental autoimmune encephalomyelitis. Mult Scler J Exp Transl Clin. (2017) 3:2055217317698724. doi: 10.1177/2055217317698724
40. Toker A, Slaney CY, Backstrom BT, Harper JL. Glatiramer acetate treatment directly targets CD11b(+)Ly6G(-) monocytes and enhances the suppression of autoreactive T cells in experimental autoimmune encephalomyelitis. Scand J Immunol. (2011) 74:235–43. doi: 10.1111/j.1365-3083.2011.02575.x
41. Xie L, Gong W, Chen J, Xie HW, Wang M, Yin XP, et al. The flavonoid kurarinone inhibits clinical progression of EAE through inhibiting Th1 and Th17 cell differentiation and proliferation. Int Immunopharmacol. (2018) 62:227–36. doi: 10.1016/j.intimp.2018.06.022
42. Li W, Wu H, Gao C, Yang D, Yang D, Shen J. Radix rehmanniae extract ameliorates experimental autoimmune encephalomyelitis by suppressing macrophage-derived nitrative damage. Front Physiol. (2018) 9:864. doi: 10.3389/fphys.2018.00864
43. Maassen CB, van Holten JC, Balk F, Heijne den Bak-Glashouwer MJ, Leer R, Laman JD, et al. Orally administered Lactobacillus strains differentially affect the direction and efficacy of the immune response. Vet Q. (1998) 20(Suppl. 3):S81–3. doi: 10.1080/01652176.1998.9694976
44. Maassen CB, Claassen E. Strain-dependent effects of probiotic lactobacilli on EAE autoimmunity. Vaccine. (2008) 26:2056–7. doi: 10.1016/j.vaccine.2008.02.035
45. Kobayashi T, Kato I, Nanno M, Shida K, Shibuya K, Matsuoka Y, et al. Oral administration of probiotic bacteria, Lactobacillus casei and Bifidobacterium breve, does not exacerbate neurological symptoms in experimental autoimmune encephalomyelitis. Immunopharmacol Immunotoxicol. (2010) 32:116–24. doi: 10.3109/08923970903200716
46. Abdurasulova IN, Matsulevich AV, Tarasova EA, Kudryavtsev IV, Serebrjakova MK, Ermolenko EI, et al. Enterococcus faecium strain L-3 and glatiramer acetate ameliorate experimental allergic encephalomyelitis in rats by affecting different populations of immune cells. Benef Microbes. (2016) 7:719–29. doi: 10.3920/BM2016.0018
47. Mangalam A, Shahi SK, Luckey D, Karau M, Marietta E, Luo N, et al. Human gut-derived commensal bacteria suppress cns inflammatory and demyelinating disease. Cell Rep. (2017) 20:1269–77. doi: 10.1016/j.celrep.2017.07.031
48. Berer K, Gerdes LA, Cekanaviciute E, Jia X, Xiao L, Xia Z, et al. Gut microbiota from multiple sclerosis patients enables spontaneous autoimmune encephalomyelitis in mice. Proc Natl Acad Sci USA. (2017) 114:10719–24. doi: 10.1073/pnas.1711233114
49. Thomas F, Hehemann JH, Rebuffet E, Czjzek M, Michel G. Environmental and gut bacteroidetes: the food connection. Front Microbiol. (2011) 2:93. doi: 10.3389/fmicb.2011.00093
50. Mazmanian SK, Round JL, Kasper DL. A microbial symbiosis factor prevents intestinal inflammatory disease. Nature. (2008) 453:620–5. doi: 10.1038/nature07008
51. Mazmanian SK. Capsular polysaccharides of symbiotic bacteria modulate immune responses during experimental colitis. J Pediatr Gastroenterol Nutr. (2008) 46(Suppl. 1):E11–2. doi: 10.1097/01.mpg.0000313824.70971.a7
52. Kim YS, Milner JA. Dietary modulation of colon cancer risk. J Nutr. (2007) 137:2576S−9S. doi: 10.1093/jn/137.11.2576S
53. Wexler HM. Bacteroides: the good, the bad, and the nitty-gritty. Clin Microbiol Rev. (2007) 20:593–621. doi: 10.1128/CMR.00008-07
54. Korkina L, Kostyuk V, De LC, Pastore S. Plant phenylpropanoids as emerging anti-inflammatory agents. Mini Rev Med Chem. (2011) 11:823–35. doi: 10.2174/138955711796575489
55. Schogor AL, Huws SA, Santos GT, Scollan ND, Hauck BD, Winters AL, et al. Ruminal prevotella spp. may play an important role in the conversion of plant lignans into human health beneficial antioxidants PLoS ONE. (2014) 9:e87949. doi: 10.1371/journal.pone.0087949
56. Toh H, Oshima K, Suzuki T, Hattori M, Morita H. Complete genome sequence of the equol-producing bacterium Adlercreutzia equolifaciens DSM 19450T. Genome Announc. (2013). 1:e00742–13. doi: 10.1128/genomeA.00742-13
57. Moussa L, Bezirard V, Salvador-Cartier C, Bacquie V, Lencina C, Leveque M, et al. A low dose of fermented soy germ alleviates gut barrier injury, hyperalgesia and faecal protease activity in a rat model of inflammatory bowel disease. PLoS ONE. (2012) 7:e49547. doi: 10.1371/journal.pone.0049547
58. De Paula ML, Rodrigues DH, Teixeira HC, Barsante MM, Souza MA, Ferreira AP. Genistein down-modulates pro-inflammatory cytokines and reverses clinical signs of experimental autoimmune encephalomyelitis. Int Immunopharmacol. (2008) 8:1291–7. doi: 10.1016/j.intimp.2008.05.002
59. Muthian G, Bright JJ. Quercetin, a flavonoid phytoestrogen, ameliorates experimental allergic encephalomyelitis by blocking IL-12 signaling through JAK-STAT pathway in T lymphocyte. J Clin Immunol. (2004) 24:542–52. doi: 10.1023/B:JOCI.0000040925.55682.a5
60. Razeghi JS, Arrefhosseini SR, Ghaemi A, Alizadeh A, Moradi TH, Togha M. Alleviation of experimental allergic encephalomyelitis in C57BL/6 mice by soy daidzein. Iran J Allergy Asthma Immunol. (2014) 13:256–64. doi: 10.1016/j.ijdevneu.2015.04.295
61. Correa-Oliveira R, Fachi JL, Vieira A, Sato FT, Vinolo MA. Regulation of immune cell function by short-chain fatty acids. Clin Transl Immunol. (2016) 5:e73. doi: 10.1038/cti.2016.17
62. Kahouli I, Malhotra M, Tomaro-Duchesneau C, Saha S, Marinescu D, Rodes LS, et al. Screening and in vitro analysis of Lactobacillus reuteri strains for short chain fatty acids production, stability and therapeutic potentials in colorectal cancer. J Bioequiv Availab. (2015) 7:039–50. doi: 10.4172/jbb.1000212
Keywords: experimental autoimmune encephalomyelitis, Lactobacillus reuteri, TH1/TH17 cells, IFN-γ/IL-17, gut microbiota
Citation: He B, Hoang TK, Tian X, Taylor CM, Blanchard E, Luo M, Bhattacharjee MB, Freeborn J, Park S, Couturier J, Lindsey JW, Tran DQ, Rhoads JM and Liu Y (2019) Lactobacillus reuteri Reduces the Severity of Experimental Autoimmune Encephalomyelitis in Mice by Modulating Gut Microbiota. Front. Immunol. 10:385. doi: 10.3389/fimmu.2019.00385
Received: 02 July 2018; Accepted: 14 February 2019;
Published: 07 March 2019.
Edited by:
Fabienne Brilot, University of Sydney, AustraliaReviewed by:
Djordje Miljkovic, University of Belgrade, SerbiaLaura Piccio, Washington University in St. Louis, United States
Copyright © 2019 He, Hoang, Tian, Taylor, Blanchard, Luo, Bhattacharjee, Freeborn, Park, Couturier, Lindsey, Tran, Rhoads and Liu. This is an open-access article distributed under the terms of the Creative Commons Attribution License (CC BY). The use, distribution or reproduction in other forums is permitted, provided the original author(s) and the copyright owner(s) are credited and that the original publication in this journal is cited, in accordance with accepted academic practice. No use, distribution or reproduction is permitted which does not comply with these terms.
*Correspondence: Jon Marc Rhoads, Si5NYXJjLlJob2Fkc0B1dGgudG1jLmVkdQ==
Yuying Liu, WXV5aW5nLkxpdUB1dGgudG1jLmVkdQ==