- 1University of Colorado Denver Anschutz Medical Center, Aurora, CO, United States
- 2University of Witwatersrand, Johannesburg, South Africa
Background: HIV-exposed uninfected infants (HEU) are at higher risk of severe infections, hospitalizations and death compared with HIV-unexposed uninfected infants (HUU), but the immune deficit underlying it is not known. To address this gap, we investigated T cell functionality and its relationship to phenotypic profiles of T cells and antigen presenting cells (APC) in HEU and HUU.
Methods: Blood mononuclear cells from 55 HEU and 16 HUU were stimulated with Staphylococcal Enterotoxin B (SEB) or mock for 72 h, and tested by flow cytometry for proliferation and expression of Th1, Th2, and regulatory (Treg) markers. In parallel, cells were phenotypically assessed for differentiation profiles of Treg, conventional T cell (Tconv) and APC in unstimulated cells.
Results: HEU had lower CD4+ functional responses to SEB/mock and similar CD8+ responses compared with HUU. In the phenotypic T cell panel, HEU showed higher proportions of CD4+ and CD8+ Treg expressing IL10, FOXP3, and CD25; higher effector Tconv and Treg; and lower naïve and CD4+TGFβ+ Treg compared with HUU. In the phenotypic APC panel, HEU showed higher proportions of CD1c+ cDC2, CD123+ pDC, CD16+ inflammatory monocytes and cDC and higher expression of CD103 on CD1c-CD123-CD16-cDC1 compared with HUU. Regression analyses adjusted for HIV exposure and multiple comparisons showed that higher CD8+IL10+ and CD8+FOXP3+ Treg in unstimulated cells were associated with lower CD8+ T cell functional responses to SEB/mock. Functionality was not affected by Tconv differentiation, but higher APC activation in aggregate was associated with higher CD8+IL10+ Treg responses to SEB.
Conclusions: T cell functionality was decreased in HEU compared with HUU. High CD8+ Treg proportions were the most important predictors of decreased T cell functionality in HEU and HUU.
Introduction
Infants are at higher risk of severe infections and have lower immune responses to most vaccines than older children and young adults. This has been ascribed to an imbalance of T-helper (Th) Th1 and Th2 immune responses in infants (1–3). Neonates, however, also have strong immune regulatory mechanisms that promote their survival in utero while surrounded by foreign maternal antigens (4–7). An example of the role of neonatal regulatory T cells (Treg) in the risk of infectious morbidity is provided by the use of cord blood in allogeneic hematopoietic stem cell transplantation, which, compared with adult cell transplants, has been associated with higher risk of opportunistic infections (8, 9). Moreover, adaptive T-cell responses to foreign antigens that cross the placenta can be elicited using Treg-depleted cord blood mononuclear cells (CBMC) but not with undepleted CBMC (10). Collectively, these data indicate that it is reasonable to propose that high proportions of Treg may be associated with increased severity of infections in infants.
HIV-exposed uninfected infants (HEU) have a significantly higher incidence of severe infections, hospitalizations and death (11–24) and lower immune responses to some vaccines (25–31) than HIV-unexposed uninfected infants (HUU). Much of the excess morbidity and mortality of HEU is due to severe infections caused by respiratory viral pathogens and S. pneumoniae (24, 28, 31–33). It has been demonstrated that HEU generally have lower maternal antibodies against many of these pathogens compared with HUU (26, 31, 34). However, we recently found that antibody titers against respiratory viruses or S. pneumoniae in the first few days of life were not associated with the development of lower respiratory tract infections in HEU (35). Furthermore, antibody responses to tetanus vaccine also failed to discriminate between HEU who developed lower respiratory tract infections or not underscoring the lack of association between humoral immune responses and risk of severe infections in HEU (35). Collectively, these data suggested that defective T cell or innate immune responses may be primarily responsible for the morbidity and mortality of infections in HEU. The pathway leading to cellular immune defects in HEU is not known (25, 27, 36–46), but excessive immune regulation is a potential unifying explanation for the diverse immune defects of HEU, since Treg and other regulatory cells decrease both innate and adaptive immune responses (47–49). Pregnant women and other people living with HIV have higher markers of activation, inflammation and regulation than their uninfected counterparts. HEU also have higher levels of inflammation and T cell and dendritic cell (DC) activation compared with HUU (36, 50, 51). However, until now, there have been no published studies comparing Treg between HEU and HUU. Moreover, the effect of T cell and DC activation on functional T cell responses has not been studied.
To address this gap and to expand our understanding of the immunologic differences between HEU and HUU we performed hypotheses-generating analyses of T cells and antigen presenting cells (APC) in peripheral blood mononuclear cells (PBMC) collected in the first 1–2 days of life from HEU and in CBMC of HUU. In order to generate mechanistic hypotheses, we placed special emphasis on the relationship between functional T cell responses and phenotypic T cell and antigen presenting cell (APC) characteristics.
Samples and Methods
Samples
The study used a convenience sample of PBMC collected in the first 48 h of life in a previous study from 55 Black South African HEU, including 42% females and 86% term infants. The legal guardians of study participants signed informed consents and the Ethics Committee of the Witwatersrand University approved the use of the study. In lieu of HUU PBMC, we used CBMC from 16 HUU about to be discarded by the cord blood bank at the University of Colorado Denver Anschutz Medical Campus. The CMBC were obtained from term infants, including 50% White Caucasians, 44% non-Black Hispanics and 56% females. Due to limited number of PBMC from HEU, not all assays could be performed on each participant. In order to obtain roughly similar number of results for each test, we used a priority list among T cell functional, T cell phenotypic, and APC phenotypic assays that changed with every batch of HEU samples tested. PBMC included in each batch were selected in the order of the study identification numbers.
Flow Cytometry Methods
Cryopreserved CBMC/PBMC were thawed and processed immediately for phenotypic and functional assessment. Phenotypic profiling was performed using flow cytometry by staining with either the APC or the T cell panel. The APC panel consisted of surface staining with Zombie yellow (viability), CD14 Alexa488, CD103 PE, CD123 PerCP-Cy5.5, CD1c APC, PD-L1 BV421 (Biolegend) and CD16 PE-CF594, CD3 PE-Cy7, CD56 PE-Cy7, CD19 PE-Cy7, CD20 PE-Cy7, CD40 Alexa700, HLA-DR APC-H7 (BD Biosciences). The T cell panel consisted of surface staining with Zombie yellow (viability), CD28 PE-Dazzle594, CD27 BV421 (Biolegend) and CD39 FITC, CD4 PerCP-Cy5.5, CD25 APC-H7, CD3 Alexa700 (BD Biosciences) followed by fixation and permeabilization using the eBioscience Foxp3/Transcription Factor Staining Buffer Set. Intracellular staining was then performed with FoxP3 PE (eBioscience) and IL-10 PE-Cy7, TGFβ APC (Biolegend).
For the functional assay, cells were first stained with cell trace violet (LifeTechnologies) then stimulated for 72 h with Staphylococcal Enterotoxin B (SEB) or with no stimulation (media only). Brefeldin A and monensin (Sigma-Aldrich, 5 μg/ml each) were added to the cultures for the last 4 h. Cells were then surface stained with Zombie yellow (viability), CD39 FITC and CD4 PerCP-Cy5.5 (BD Biosciences) followed by fixation (BD lysing solution) and permeabilization (BD perm2 buffer) and stained intracellularly with IL-4 PE, CD3 Alexa700, IFNγ APC-H7 (BD Biosciences), and IL-10 PE-Cy7, TGFβ APC (Biolegend).
Samples were acquired on a Beckman Coulter Gallios cytometer and analyzed with FlowJo version 9.0 (BD Biosciences). Gating strategies are shown in Figure S1.
Statistical Methods
Frequencies (%) or means and standard deviations were calculated for baseline demographics. Heatmaps were drawn to show the Spearman correlation between cell populations. Correlations between HEU and HUU were assessed using simple linear regression models where cell populations were primary outcomes and maternal HIV status was the main covariate. Multiple linear regression models were used to assess relationships between various cell populations adjusting for maternal HIV status. Cell population distributions were examined using graphical inspection and log transformations were used when distributions were highly skewed. To account for multiple comparisons, a false discovery rate (FDR) correction was implemented for each set of comparisons among cell populations, and significance was evaluated at a FDR p-threshold of 0.05. Principal component analysis (PCA) using Euclidean distances were drawn for functional differences in SEB and no stimulation for CD4 and CD8 to evaluate potential differences in populations between HUU and HEU. Further, the first 3 PCs for the activation markers and CD4/CD8 T cell subsets were tested via linear regression for association with functional differences for CD4 and CD8. Functional outcomes were log transformed and the regression coefficients were back transformed to the original scale. Data analysis was performed using R software, version 3.4.0.
Results
Functional T Cell Characteristics of HEU and HUU
T cell proliferation and expression of Th1 (IFNγ, CD107a), Th2 (IL4), and Treg (IL10, TGFβ, and CD39) were investigated by stimulating PBMC from 22 HEU and 17 HUU with SEB and mock control and expressed as the ratio of stimulated over mock PBMC. The PCA visualization of the data (Figure 1A) showed separation of the CD4+ T cell outcome measures between HEU and HUU, but not of CD8+ T cell outcomes (Figure S2A). The linear regression analysis revealed significantly lower expression of IFNγ, IL4, IL10, TGFβ, and CD39 in HEU compared to HUU CD4+ T cells in response to SEB stimulation (Figure 1B), but not in CD8+ T cells (Figure S2B). Correlation analyses revealed strong positive associations (rho > 0.8) between CD4+CD107a+ and CD4+IL4+ responses to SEB in HEU and HUU (rho = 0.81 for both; Figures 2A,B). Similar analyses of CD8+ T cell responses to SEB showed strong positive correlations of CD8+CD107a+ with CD8+IL4+ and CD8+IFNγ+ in HEU (rho ≥ 0.81; Figure 2C) and strong positive correlations of CD8+ T cell expression of CD39, CD107, IFNγ, IL4 and/or IL10 in HUU (rho ≥ 0.81; Figure 2D). Importantly, there were no significant negative correlations between Treg responses to SEB and Th1 or Th2 responses to SEB.
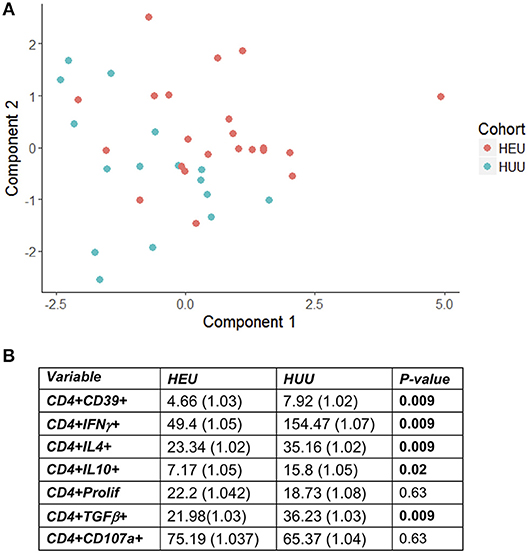
Figure 1. HEU and HUU functional CD4+ T cell responses. Data were derived from PBMC of 22 HEU and 17 HUU after stimulation with SEB and mock. Results were expressed as the ratio between SEB and mock stimulation. (A) shows the PCA distribution of the results. (B) shows results of the regression analysis.
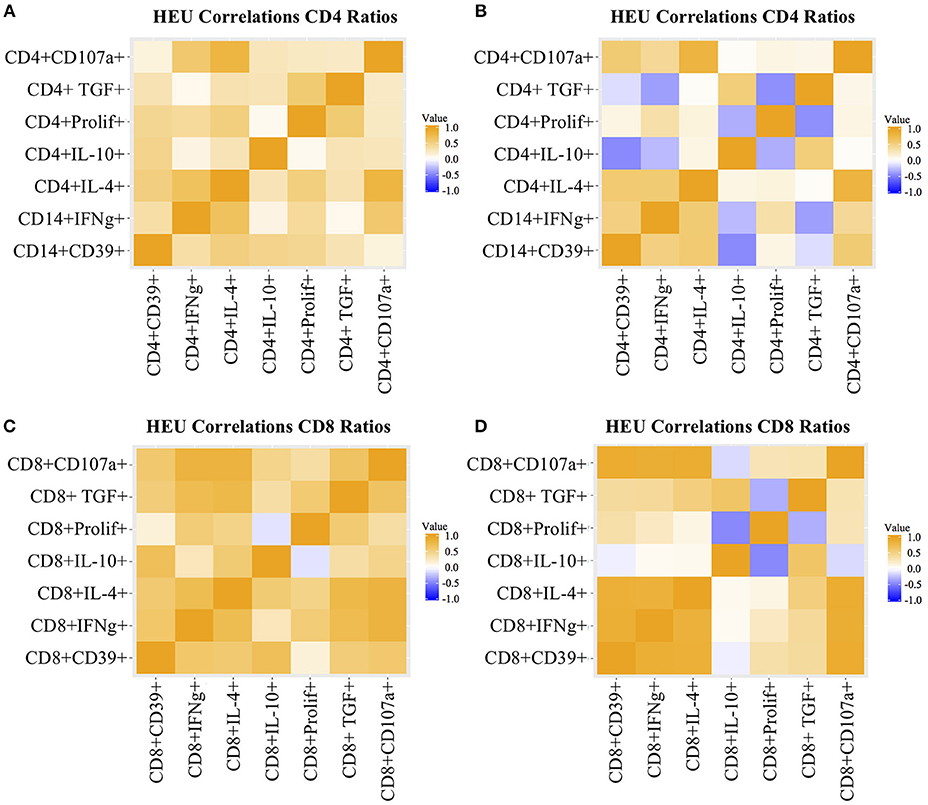
Figure 2. Heatmap representation of functional T cell response correlations. Data were derived from PBMC of 22 HEU and 17 HUU after stimulation with SEB and mock. Analytes are shown on the graph axes. The correlation factor scale is shown on the side of each heatmap. The data represented in panels (A–D) are labeled in each of the corresponding panels.
T Cell Phenotypic Characterization
We performed multiple pre-specified comparisons of the T cell phenotypes of HEU and HUU, including 28 Treg and Tconv subsets. Overall, the ratios of CD4+ to CD8+ T cell populations were similar in HEU and HUU. However, the proportions of multiple CD4+ and CD8+ T cell subsets significantly differed in unadjusted comparisons (Figure S3), 10 of which remained significantly different after FDR correction (Table 1). These included CD4+/CD8+FOXP3+, CD4+/CD8+FOXP3+CD25+, and CD8+IL10+ Treg, which were higher in HEU compared with HUU; CD4+TGFβ+ Treg, lower in HEU; CD8+FOXP3+CD27+CD28- and CD8+FOXP3+CD27-CD28-differentiated Treg, and CD8+CD27+CD28-differentiated Tconv higher in HEU; and CD8+FOXP3+CD27+CD28+ naïve Treg higher in HUU.
Phenotypic Characterization of APC
The unadjusted analyses identified multiple APC phenotypic differences in HEU compared with HUU (Figure S4). Overall, HEU had higher proportions of CD16+ Mono, CD16+CD123-CD1c- DC (CD16+ cDC) and CD123+ pDC compared with the HUU, but lower proportions of CD16- Mono (Mono1) and CD123-CD1c-CD16- DC (cDC1). HEU also had increased PDL1 expression on cDC1, cDC2, pDC, and Mono1 and increased expression of CD103 on cDC1 and cDC2. After FDR correction, the following observations remained significantly different: higher proportions of pDC, CD16+ cDC and cDC2 and CD16+ Mono out of APC, and higher CD103 expression on cDC1 in HEU compared with HUU (Table 2).
Correlation of T Cell Function With Treg and T Cell Differentiation Phenotypes
We investigated the effect of Treg, Tconv, and APC phenotypes on functional T cell responses to SEB stimulation in a regression analysis adjusted for maternal HIV status and for multiple comparisons. High frequencies of circulating CD8+IL10+ Treg were significantly associated with low CD8+IFNγ+, CD8+CD107a+, CD8+CD39+, and CD8+IL4+ responses to SEB stimulation (Figure 3). High frequencies of circulating CD8+FOXP3+ Treg were associated with low CD8+IL4+ and CD8+TGFβ+ responses to SEB stimulation (Figure 3). CD4+ Treg, CD4+ or CD8+ T cell differentiation and APC phenotypes were not associated with T cell responses to SEB (not shown).
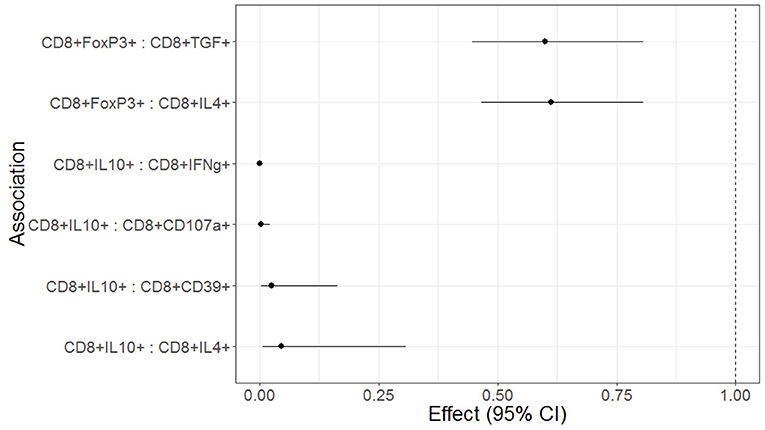
Figure 3. Forest plot representation of significant effects of Treg on functional T cell outcomes in HEU and HUU. Data were derived from 6 HEU and 16HUU. Filled circles and horizontal lines indicate mean and 95% CI of the effect of each Treg population on the functional outcome indicated on the abscissa (Treg : functional outcome). No effect is indicated by the dotted vertical line. The effect represents the decrease of the functional outcome with each unit increase in the Treg population. For example, for each 1% increase in CD8+FOXP3 Treg, there is a 40% decrease in the CD8+TGFβ T cells; and for each 1% increase in CD8+IL10+ Treg there is a 95% decrease in the CD8+IFNγ+ T cells.
Correlation of T Cell Function With APC Activation
None of the individual activation markers expressed by DC or Mono subsets showed significant effects on T cell functional outcomes. However, we reasoned that the high number of explanatory variables might have obscured significant associations. To decrease the dimensionality of the activated APC subset, we grouped them using PCA (Table 3). 95.7% of the variation of the activated APC subsets was explained by the first 3 PCs with 63% of the variation explained by PC1. The correlation analysis of PC1 with T cell functional outcomes revealed a significant positive association with CD8+IL10+ responses to SEB (effect estimate ±S.E. = 1.02 ± 1.01; p = 0.007). This indicates that PDL1+ cDC1, cDC2, pDC and CD16- Mono, as well as CD40+ cDC1 and cDC2, all moderately to highly correlated with PC1, may be codependently correlated with CD8+IL10+ responses to SEB. PC2 and PC3 did not significantly correlate with any of the functional outcome measures.
Discussion
The primary objective of this study was to identify factors that may explain decreased CMI responses to vaccines in HEU and that might be amenable to therapeutic interventions. Our primary focus was on Treg, because of their broad immune-attenuating and sometimes nonspecific activity and because of the association of HIV infection with increased Treg populations (52–58). In addition, preliminary results obtained from 7 South African HUU and 4 HEU showed significantly higher CD4+ and CD8+ FOXP3+ and FOXP3+CD25+ Treg in HEU compared with HUU. In the current study, we demonstrated an increased proportion of both CD4+ and CD8+ Treg in HEU compared with HUU using US CBMC to generate the HUU. Although the differences between US CBMC and South African HEU PBMC were similar to those between South African HUU and HEU, the use of CBMC was a limitation of this study. For example, the difference in Treg between HEU and HUU might have been even more significant if we had used HEU and HUU cells of the same age instead of PBMC from 1 to 2 days of age from HEU and CBMC from HUU. Recent findings showed that the proportions of Treg significantly decrease between birth and the first few days of life (59), suggesting that we might have underestimated the Treg differences HEU and HUU by virtue of using samples from slightly older HEU compared with HUU.
In this study, we showed for the first time to our knowledge that HEU have significant defects in T cell functionality at birth by comparison with HUU. This observation is highly relevant to the current landscape of HIV infection, in which there is a continuously growing number of HEU with increased risk of severe infections leading to hospitalization and/or death (11–24). It is important to note that this observation, originally made in countries with limited medical resources, has been extended to countries with high resources, including the US (60). HIV infection during pregnancy has been long known to decrease transplacental transfer of maternal antibodies (31, 61, 62), potentially increasing the vulnerability of HEU to infections. However, our most recent studies showed that maternal antibody levels transferred to the neonate did not predict the risk of lower respiratory tract infections in HEU (35). Furthermore, the antibody responses to vaccines of HEU has been comparable to that of HUU in studies originated in Sub-Saharan Africa, suggesting that HEU have intact humoral immunity (34, 63). A minority of studies, all of them including participants from Brazil, reported lower antibody responses in HEU compared with HUU (28, 31). Nevertheless, in our study comparing Brazilian HEU and HUU, despite showing decreased antibody responses to tetanus vaccine in HEU, the magnitude of the HEU antibody responses to the vaccine did not correlate with the risk of developing lower respiratory tract infections during the first 6 months of life, underscoring the lack of association between humoral immunity and risk of severe respiratory tract infection in HEU (35). Taken together, these data suggest that T cell immune defects play a more important role than antibodies in the increased susceptibility to infection of HEU.
Other studies that showed decreased T cell responses to BCG or tetanus vaccines during the first few months of life in HEU (11–24) support and complement our findings by indicating the persistence of T cell functional defects in HEU during the first year of life and possibly longer. It is also important to note that the increased risk of hospitalization and death due to infectious complications in HEU has been demonstrated for the first 1–2 years of life, further suggesting an association between decreased T cell functional responses and clinical outcomes.
The effect of increased proportions of Treg on functional responses in HEU was investigated by regression analysis adjusted for maternal HIV status. The adjustment was performed with the intent of increasing the probability of identifying causal associations. We reasoned that since HEU had both lower functional responses and higher Treg proportions, there was a considerable risk of finding both outcome measures in the same individual by chance, which we tried to prevent by controlling for the maternal HIV status. Nevertheless, the adjusted analysis identified CD8+FOXP3+ and CD8+IL10+ Treg as predictors of decreased CD8+ T cell functionality in HEU and HUU. It is important to note that the T cell functional responses that appear to be downregulated by Treg were not the same T cell functional responses that differed between HEU and HUU. This, however, was not unexpected due to the adjustment of the statistical analysis for the HIV maternal status.
We also found higher proportions of Treg and Tconv differentiated to the effector stage in HEU compared with HUU. Previous studies also showed higher level of T cell activation in HEU compared with HUU, which is in accordance with our findings, since effector T cells generally also express activation markers (36). It is well-known and accepted that T cell differentiation into effector and memory cells occurs in the presence of cognate antigen and is mediated by activated APC and lineage-specific cytokines. It is unclear what antigens triggered the T cell differentiation in HEU. However, we have previously shown higher pro-inflammatory cytokine milieu in HEU compared with HUU, including sTNFRI, IL6, and IP10 that could potentially lower the threshold of T cell activation and differentiation in HEU (50).
We also showed in this study increased expression of CD40, CD103, and PDL-1 in HEU compared with HUU in unadjusted analyses and CD103 on cDC1 after adjustment for multiple comparisons, indicating that increased activation is not limited to T cells, but also encompasses APC. None of the activated APC populations individually correlated with T cell function, but the PCA showed that activation in general, represented by either CD40 or PDL1 expression, was associated with increased CD8+IL10+ Treg proliferation in response SEB. Other functional T cell outcomes did not significantly correlate with APC.
Our study has several limitations, including the geographic and racial differences between HEU and HUU, the limited cell numbers in HEU samples, its exploratory nature and the lack of mechanistic experiments. As mentioned above, the age difference between HEU and HUU may have underestimated the Treg differences identified in our study. A strength of this study is the use of a superantigen as stimulant, which enlists the collaboration between T cells and APC into the response.
Several hypotheses can be formulated based on the results of this exploratory study. Firstly, we propose that increased Treg populations in HEU decrease their T cell responses to infections and vaccines. This hypothesis is being tested in studies that we are currently conducting. Secondly, we propose that the high inflammatory in utero milieu of HEU may lower the threshold for T cell activation and differentiation against antigens to which they are commonly exposed in utero and that do not trigger responses in HUU. Thirdly, there may be increased transplacental transfer of antigens in pregnancies complicated by HIV infection.
Data Availability
All datasets generated for this study are included in the manuscript and/or the Supplementary Files.
Author Contributions
EJ performed assays, analysis and wrote manuscript. KW and MK performed statistical analysis and wrote manuscript. MJ performed analysis and reviewed manuscript. CC, SM and MN enrolled study participants and reviewed the manuscript. AW designed the study, analyzed the data, and wrote the manuscript.
Conflict of Interest Statement
The authors declare that the research was conducted in the absence of any commercial or financial relationships that could be construed as a potential conflict of interest.
Acknowledgments
This study was supported by AI131360 (NIAID) and a grant from Bill and Melinda Gates Foundation.
Supplementary Material
The Supplementary Material for this article can be found online at: https://www.frontiersin.org/articles/10.3389/fimmu.2019.00595/full#supplementary-material
References
1. Kumar SK, Bhat BV. Distinct mechanisms of the newborn innate immunity. Immunol Lett. (2016) 173:42–54. doi: 10.1016/j.imlet.2016.03.009
2. Levy O. Innate immunity of the newborn,: basic mechanisms and clinical correlates. Nat Rev Immunol. (2007) 7:379–90. doi: 10.1038/nri2075
3. Debock I, Flamand V. Unbalanced neonatal CD4(+) T-cell immunity. Front Immunol. (2014) 5:393. doi: 10.3389/fimmu.2014.00393
4. Simon AK, Hollander GA, McMichael A. Evolution of the immune system in humans from infancy to old age. Proc Biol Sci. (2015) 282:20143085. doi: 10.1098/rspb.2014.3085
5. Mold JE, Michaelsson J, Burt TD, Muench MO, Beckerman KP, Busch MP, et al. Maternal alloantigens promote the development of tolerogenic fetal regulatory T cells in utero. Science. (2008) 322:1562–5. doi: 10.1126/science.1164511
6. Wang G, Miyahara Y, Guo Z, Khattar M, Stepkowski SM, Chen W. “Default” generation of neonatal regulatory T cells. J Immunol. (2010) 185:71–8. doi: 10.4049/jimmunol.0903806
7. Mold JE, Venkatasubrahmanyam S, Burt TD, Michaelsson J, Rivera JM, Galkina SA, et al. Fetal and adult hematopoietic stem cells give rise to distinct T cell lineages in humans. Science. (2010) 330:1695–9. doi: 10.1126/science.1196509
8. Quintela A, Escuret V, Roux S, Bonnafous P, Gilis L, Barraco F, et al. HHV-6 infection after allogeneic hematopoietic stem cell transplantation: from chromosomal integration to viral co-infections and T-cell reconstitution patterns. J Infect. (2016) 72:214–22. doi: 10.1016/j.jinf.2015.09.039
9. Konuma T, Tsukada N, Kanda J, Uchida N, Ohno Y, Miyakoshi S, et al. Comparison of transplant outcomes from matched sibling bone marrow or peripheral blood stem cell and unrelated cord blood in patients 50 years or older. Am J Hematol. (2016) 91:E284–92. doi: 10.1002/ajh.24340
10. Legrand FA, Nixon DF, Loo CP, Ono E, Chapman JM, Miyamoto M, et al. Strong HIV-1-specific T cell responses in HIV-1-exposed uninfected infants and neonates revealed after regulatory T cell removal. PLoS ONE. (2006) 1:e102. doi: 10.1371/journal.pone.0000102
11. Epalza C, Goetghebuer T, Hainaut M, Prayez F, Barlow P, Dediste A, et al. High incidence of invasive group B streptococcal infections in HIV-exposed uninfected infants. Pediatrics. (2010) 126:e631–8. doi: 10.1542/peds.2010-0183
12. Izadnegahdar R, Fox MP, Jeena P, Qazi SA, Thea DM. Revisiting pneumonia and exposure status in infants born to HIV-infected mothers. Pediatr Infect Dis J. (2014) 33:70–2. doi: 10.1097/INF.0b013e31829f0ade
13. Koyanagi A, Humphrey JH, Ntozini R, Nathoo K, Moulton LH, Iliff P, et al. Morbidity among human immunodeficiency virus-exposed but uninfected, human immunodeficiency virus-infected, and human immunodeficiency virus-unexposed infants in Zimbabwe before availability of highly active antiretroviral therapy. Pediatr Infect Dis J. (2011) 30:45–51. doi: 10.1097/INF.0b013e3181ecbf7e
14. Kuhn L, Kasonde P, Sinkala M, Kankasa C, Semrau K, Scott N, et al. Does severity of HIV disease in HIV-infected mothers affect mortality and morbidity among their uninfected infants? Clin Infect Dis. (2005) 41:1654–61. doi: 10.1086/498029
15. McNally LM, Jeena PM, Gajee K, Thula SA, Sturm AW, Cassol S, et al. Effect of age, polymicrobial disease, and maternal HIV status on treatment response and cause of severe pneumonia in South African children,: a prospective descriptive study. Lancet. (2007) 369:1440–51. doi: 10.1016/S0140-6736(07)60670-9
16. Mussi-Pinhata MM, Freimanis L, Yamamoto AY, Korelitz J, Pinto JA, Cruz ML, et al. Infectious disease morbidity among young HIV-1-exposed but uninfected infants in Latin American and Caribbean countries,: the National Institute of Child Health and Human Development International Site Development Initiative Perinatal Study. Pediatrics. (2007) 119:e694–704. doi: 10.1542/peds.2006-1856
17. Mussi-Pinhata MM, Motta F, Freimanis-Hance L, de Souza R, Szyld E, Succi RC, et al. Lower respiratory tract infections among human immunodeficiency virus-exposed, uninfected infants. Int J Infect Dis. (2010) 14(Suppl. 3):e176–82. doi: 10.1016/j.ijid.2010.01.006
18. Shapiro RL, Lockman S. Mortality among HIV-exposed infants: the first and final frontier. Clin Infect Dis. (2010) 50:445–7. doi: 10.1086/649887
19. Slogrove A, Reikie B, Naidoo S, De Beer C, Ho K, Cotton M, et al. HIV-exposed uninfected infants are at increased risk for severe infections in the first year of life. J Trop Pediatr. (2012) 58:505–8. doi: 10.1093/tropej/fms019
20. Slogrove AL, Cotton MF, Esser MM. Severe infections in HIV-exposed uninfected infants: clinical evidence of immunodeficiency. J Trop Pediatr. (2010) 56:75–81. doi: 10.1093/tropej/fmp057
21. Kourtis AP, Wiener J, Kayira D, Chasela C, Ellington SR, Hyde L, et al. Health outcomes of HIV-exposed uninfected African infants. AIDS. (2013) 27:749–59. doi: 10.1097/QAD.0b013e32835ca29f
22. Taron-Brocard C, Le Chenadec J, Faye A, Dollfus C, Goetghebuer T, Gajdos V, et al. Increased risk of serious bacterial infections due to maternal immunosuppression in HIV-exposed uninfected infants in a European country. Clin Infect Dis. (2014) 59:1332–45. doi: 10.1093/cid/ciu586
23. Kelly MS, Wirth KE, Steenhoff AP, Cunningham CK, Arscott-Mills T, Boiditswe SC, et al. Treatment failures and excess mortality among HIV-exposed, uninfected children with pneumonia. J Pediatric Infect Dis Soc. (2014) 4:e117–26. doi: 10.1093/jpids/piu092
24. von Mollendorf C, von Gottberg A, Tempia S, Meiring S, de Gouveia L, Quan V, et al. Increased risk for and mortality from invasive pneumococcal disease in HIV-exposed but uninfected infants aged < 1 year in South Africa 2009-2013. Clin Infect Dis. (2015) 60:1346–56. doi: 10.1093/cid/civ059
25. Mazzola TN, da Silva MT, Abramczuk BM, Moreno YM, Lima SC, Zorzeto TQ, et al. Impaired Bacillus Calmette-Guerin cellular immune response in HIV-exposed, uninfected infants. AIDS. (2011) 25:2079–87. doi: 10.1097/QAD.0b013e32834bba0a
26. Simani OE, Adrian PV, Violari A, Kuwanda L, Otwombe K, Nunes MC, et al. Effect of in-utero HIV exposure and antiretroviral treatment strategies on measles susceptibility and immunogenicity of measles vaccine. AIDS. (2013) 27:1583–91. doi: 10.1097/QAD.0b013e32835fae26
27. Van Rie A, Madhi SA, Heera JR, Meddows-Taylor S, Wendelboe AM, Anthony F, et al. Gamma interferon production in response to Mycobacterium bovis BCG and Mycobacterium tuberculosis antigens in infants born to human immunodeficiency virus-infected mothers. Clin Vaccine Immunol. (2006) 13:246–52. doi: 10.1128/CVI.13.2.246-252.2006
28. Abramczuk BM, Mazzola TN, Moreno YM, Zorzeto TQ, Quintilio W, Wolf PS, et al. Impaired humoral response to vaccines among HIV-exposed uninfected infants. Clin Vaccine Immunol. (2011) 18:1406–9. doi: 10.1128/CVI.05065-11
29. Gnanashanmugam D, Troy SB, Musingwini G, Huang C, Halpern MS, Stranix-Chibanda L, et al. Immunologic response to oral polio vaccine in human immunodeficiency virus-infected and uninfected Zimbabwean children. Pediatr Infect Dis J. (2012) 31:176–80. doi: 10.1097/INF.0b013e31823faa5f
30. Kidzeru EB, Hesseling AC, Passmore JA, Myer L, Gamieldien H, Tchakoute CT, et al. In-utero exposure to maternal HIV infection alters T-cell immune responses to vaccination in HIV-uninfected infants. AIDS. (2014) 28:1421–30. doi: 10.1097/QAD.0000000000000292
31. Weinberg A, Mussi-Pinhata MM, Yu Q, Cohen RA, Almeida VC, Amaral F, et al. Excess respiratory viral infections and low antibody responses among HIV-exposed, uninfected infants. AIDS. (2017) 31:669–79. doi: 10.1097/QAD.0000000000001393
32. Cohen C, Moyes J, Tempia S, Groome M, Walaza S, Pretorius M, et al. Epidemiology of acute lower respiratory tract infection in HIV-exposed uninfected infants. Pediatrics. (2016) 137:e20153272. doi: 10.1542/peds.2015-3272
33. Cutland CL, Schrag SJ, Thigpen MC, Velaphi SC, Wadula J, Adrian PV, et al. Increased risk for Group B Streptococcus sepsis in young infants exposed to HIV, Soweto, South Africa, 2004-2008(1). Emerg Infect Dis. (2015) 21:638–45. doi: 10.3201/eid2104.141562
34. Jones CE, Naidoo S, De Beer C, Esser M, Kampmann B, Hesseling AC. Maternal HIV infection and antibody responses against vaccine-preventable diseases in uninfected infants. JAMA. (2011) 305:576–84. doi: 10.1001/jama.2011.100
35. Weinberg A, Mussi-Pinhata MM, Yu Q, Cohen RA, Almeida VC, Amaral FR, et al. Factors associated with lower respiratory tract infections in HIV-exposed uninfected infants. AIDS Res Hum Retroviruses. (2018) 34:527–35. doi: 10.1089/AID.2017.0245
36. Clerici M, Saresella M, Colombo F, Fossati S, Sala N, Bricalli D, et al. T-lymphocyte maturation abnormalities in uninfected newborns and children with vertical exposure to HIV. Blood. (2000) 96:3866–71.
37. Miles DJ, Gadama L, Gumbi A, Nyalo F, Makanani B, Heyderman RS. Human immunodeficiency virus (HIV) infection during pregnancy induces CD4 T-cell differentiation and modulates responses to Bacille Calmette-Guerin (BCG) vaccine in HIV-uninfected infants. Immunology. (2010) 129:446–54. doi: 10.1111/j.1365-2567.2009.03186.x
38. Nielsen SD, Jeppesen DL, Kolte L, Clark DR, Sorensen TU, Dreves AM, et al. Impaired progenitor cell function in HIV-negative infants of HIV-positive mothers results in decreased thymic output and low CD4 counts. Blood. (2001) 98:398–404.
39. Rich KC, Siegel JN, Jennings C, Rydman RJ, Landay AL. Function and phenotype of immature CD4+ lymphocytes in healthy infants and early lymphocyte activation in uninfected infants of human immunodeficiency virus-infected mothers. Clin Diagnost Lab Immunol. (1997) 4:358–61.
40. Hygino J, Lima PG, Filho RG, Silva AA, Saramago CS, Andrade RM, et al. Altered immunological reactivity in HIV-1-exposed uninfected neonates. Clin Immunol. (2008) 127:340–7. doi: 10.1016/j.clim.2008.01.020
41. Hygino J, Vieira MM, Guillermo LV, Silva-Filho RG, Saramago C, Lima-Silva AA, et al. Enhanced Th17 phenotype in uninfected neonates born from viremic HIV-1-infected pregnant women. J Clin Immunol. (2011) 31:186–94. doi: 10.1007/s10875-010-9485-3
42. Ota MO, O'Donovan D, Marchant A, Yamuah L, Harding E, Jaffar S, et al. HIV-negative infants born to HIV-1 but not HIV-2-positive mothers fail to develop a Bacillus Calmette-Guerin scar. AIDS. (1999) 13:996–8.
43. Ono E, Nunes dos Santos AM, de Menezes Succi RC, Machado DM, de Angelis DS, Salomao R, et al. Imbalance of naive and memory T lymphocytes with sustained high cellular activation during the first year of life from uninfected children born to HIV-1-infected mothers on HAART. Braz J Med Biol Res. (2008) 41:700–8.
44. Gesner M, Papaevangelou V, Kim M, Chen SH, Moore T, Krasinski K, et al. Alteration in the proportion of CD4 T lymphocytes in a subgroup of human immunodeficiency virus-exposed-uninfected children. Pediatrics. (1994) 93:624–30.
45. Miyamoto M, Pessoa SD, Ono E, Machado DM, Salomao R, Succi RC, et al. Low CD4+ T-cell levels and B-cell apoptosis in vertically HIV-exposed noninfected children and adolescents. J Trop Pediatr. (2010) 56:427–32. doi: 10.1093/tropej/fmq024
46. Economides A, Schmid I, Anisman-Posner DJ, Plaeger S, Bryson YJ, Uittenbogaart CH. Apoptosis in cord blood T lymphocytes from infants of human immunodeficiency virus-infected mothers. Clin Diagnost Lab Immunol. (1998) 5:230–4.
47. Stephens GL, Andersson J, Shevach EM. Distinct subsets of FoxP3+ regulatory T cells participate in the control of immune responses. J Immunol. (2007) 178:6901–11.
48. Rosenblum MD, Way SS, Abbas AK. Regulatory T cell memory. Nat Rev Immunol. (2016) 16:90–101. doi: 10.1038/nri.2015.1
49. Philbin VJ, Levy O. Developmental biology of the innate immune response,: implications for neonatal and infant vaccine development. Pediatr Res. (2009) 65:98R−105R. doi: 10.1203/PDR.0b013e31819f195d
50. Mussi-Pinhata MM, Weinberg A, Yu Q, Cohen RA, Dirajlal-Fargo S, Funderburg N, et al. Increased inflammation and monocyte activation in HIV-exposed uninfected infant. In: Conference on Retroviruses and Opportunistic Infections. Boston, MA (2018).
51. Velilla PA, Montoya CJ, Hoyos A, Moreno ME, Chougnet C, Rugeles MT. Effect of intrauterine HIV-1 exposure on the frequency and function of uninfected newborns' dendritic cells. Clin Immunol. (2008) 126:243–50. doi: 10.1016/j.clim.2007.11.004
52. Gaardbo JC, Ronit A, Hartling HJ, Gjerdrum LM, Springborg K, Ralfkiaer E, et al. Immunoregulatory T cells may be involved in preserving CD4 T cell counts in HIV-infected long-term nonprogressors and controllers. J Acquired Immune Deficiency Syndromes. (2014) 65:10–8. doi: 10.1097/QAI.0b013e3182a7c932
53. Hunt PW, Landay AL, Sinclair E, Martinson JA, Hatano H, Emu B, et al. A low T regulatory cell response may contribute to both viral control and generalized immune activation in HIV controllers. PLoS ONE. (2011) 6:e15924. doi: 10.1371/journal.pone.0015924
54. Jenabian MA, El-Far M, Vyboh K, Kema I, Costiniuk CT, Thomas R, et al. Immunosuppressive tryptophan catabolism and gut mucosal dysfunction following early HIV infection. J Infect Dis. (2015) 212:355–66. doi: 10.1093/infdis/jiv037
55. Presicce P, Orsborn K, King E, Pratt J, Fichtenbaum CJ, Chougnet CA. Frequency of circulating regulatory T cells increases during chronic HIV infection and is largely controlled by highly active antiretroviral therapy. PLoS ONE. (2011) 6:e28118. doi: 10.1371/journal.pone.0028118
56. Moreno-Fernandez ME, Rueda CM, Velilla PA, Rugeles MT, Chougnet CA. cAMP during HIV infection: friend or foe? AIDS Res Hum Retroviruses. (2012) 28:49–53. doi: 10.1089/AID.2011.0265
57. Boasso A, Hardy AW, Anderson SA, Dolan MJ, Shearer GM. HIV-induced type I interferon and tryptophan catabolism drive T cell dysfunction despite phenotypic activation. PLoS ONE. (2008) 3:e2961.
58. Zhang M, Zhang H, Zhang T, Ji Y, Jiao Y, Wu H. Longitudinal changes of peripheral blood DC subsets and regulatory T cells in Chinese chronic HIV-1-infected patients during antiretroviral therapy. PLoS ONE. (2012) 7:e37966. doi: 10.1371/journal.pone.0037966
59. Olin A, Henckel E, Chen Y, Lakshmikanth T, Pou C, Mikes J, et al. Stereotypic immune system development in newborn children. Cell. (2018) 174:1277–1292.e14. doi: 10.1016/j.cell.2018.06.045
60. Labuda SM, Huo Y, Kacanek D, Patel K. Higher rates of hospitalization and infection-related hospitalization among HIV-exposed uninfected infants compared to HIV unexposed uninfected infants in the US. In: IDWeek 2018. San Francisco, CA (2018).
61. Dangor Z, Kwatra G, Izu A, Adrian P, van Niekerk N, Cutland CL, et al. HIV-1 is associated with lower group B Streptococcus capsular and surface-protein IGG antibody levels and reduced transplacental antibody transfer in pregnant women. J Infect Dis. (2015) 212:453–62. doi: 10.1093/infdis/jiv064
62. Abu-Raya B, Smolen KK, Willems F, Kollmann TR, Marchant A. Transfer of maternal antimicrobial immunity to HIV-exposed uninfected newborns. Front Immunol. (2016) 7:338. doi: 10.3389/fimmu.2016.00338
Keywords: HIV-exposed uninfected infants, regulatory T cells, T cell function, antigen presenting cells, T cell differentiation
Citation: Jalbert E, Williamson KM, Kroehl ME, Johnson MJ, Cutland C, Madhi SA, Nunes MC and Weinberg A (2019) HIV-Exposed Uninfected Infants Have Increased Regulatory T Cells That Correlate With Decreased T Cell Function. Front. Immunol. 10:595. doi: 10.3389/fimmu.2019.00595
Received: 08 December 2018; Accepted: 05 March 2019;
Published: 26 March 2019.
Edited by:
Francesca Chiodi, Karolinska Institutet (KI), SwedenReviewed by:
Martyn Andrew French, University of Western Australia, AustraliaSarah Rowland-Jones, University of Oxford, United Kingdom
Copyright © 2019 Jalbert, Williamson, Kroehl, Johnson, Cutland, Madhi, Nunes and Weinberg. This is an open-access article distributed under the terms of the Creative Commons Attribution License (CC BY). The use, distribution or reproduction in other forums is permitted, provided the original author(s) and the copyright owner(s) are credited and that the original publication in this journal is cited, in accordance with accepted academic practice. No use, distribution or reproduction is permitted which does not comply with these terms.
*Correspondence: Adriana Weinberg, YWRyaWFuYS53ZWluYmVyZ0B1Y2RlbnZlci5lZHU=