- 1Department of Biochemistry, Cell, and Molecular Biology, West African Centre for Cell Biology of Infectious Pathogens (WACCBIP), University of Ghana, Accra, Ghana
- 2Immunology Department, Noguchi Memorial Institute for Medical Research, College of Health Sciences, University of Ghana, Accra, Ghana
- 3African Institute for Mathematical Sciences, Cape Coast, Ghana
- 4Kintampo Health Research Centre, Kintampo, Ghana
- 5African Institute for Mathematical Sciences, University of Stellenbosch, Cape Town, South Africa
T cells play significant roles during Plasmodium falciparum infections. Their regulation of the immune response in symptomatic children with malaria has been deemed necessary to prevent immune associated pathology. In this study, we phenotypically characterized the expression of T cell inhibitory(PD-1, CTLA-4) and senescent markers (CD28(-), CD57) from children with symptomatic malaria, asymptomatic malaria and healthy controls using flow cytometry. We observed increased expression of T cell exhaustion and senescence markers in the symptomatic children compared to the asymptomatic and healthy controls. T cell senescence markers were more highly expressed on CD8 T cells than on CD4 T cells. Asymptomatically infected children had comparable levels of these markers with healthy controls except for CD8+ PD-1+ T cells which were significantly elevated in the asymptomatic children. Also, using multivariate regression analysis, CTLA-4 was the only marker that could predict parasitaemia level. The results suggest that the upregulation of immune exhaustion and senescence markers during symptomatic malaria may affect the effector function of T cells leading to inefficient clearance of parasites, hence the inability to develop sterile immunity to malaria.
Background
Clinical malaria is a disease of public health importance due to its associated morbidity and mortality (1). With the emergence of drug-resistant parasites and insecticide resistant vectors, there is a need to develop effective interventions (2–4). Despite promising results of candidate vaccines in naïve individuals, comparatively poorer responses are observed in people in endemic areas (5, 6), indicating that much effort needs to be focused on understanding host factors associated with the development of immunity, especially in malaria-endemic areas. Blood stage infection with malaria parasites may either result in asymptomatic malaria, uncomplicated malaria, or proceed to complications such as severe malaria anemia or cerebral malaria. Repeated exposure to parasites usually results in the acquisition of anti-disease immunity which is characterized by the absence of clinical symptoms, yet with susceptibility to the infection. This suggests that the naturally induced immune response generated against P. falciparum may not always be potent enough to eradicate the infection. Therefore, malaria vaccines that can protect against symptomatic disease and possibly also eliminate infections remain a global health priority.
Lymphocytes, including T cells, play a significant role in the generation of protective malaria-specific responses (7), and their mechanism of action may either be by controlling or decreasing parasitemia (8) or by exacerbating the infection promoting parasitemia (9). However, looking at natural infections it can be presumed that the inability to eliminate P. falciparum malaria may be associated with immune dysfunction resulting from the expression of markers that negatively regulate T cell activity or result in their ineffective response. These may lead to the exhaustion of T cells, which has been well-described in viral infections including HIV and hepatitis B (HBV) (10, 11) as well as in protozoan infections like Toxoplasmosis and Leishmania (12, 13).
In malaria, work in both human and murine models has reported the upregulation of immune inhibitory markers such as T-cell immunoglobin and mucin domain-3 (TIM-3), lymphocyte-activation gene-3 (LAG-3) and programmed cell death-1 (PD-1) during acute infections (14, 15). These have been shown to affect not only the effector functions of T cells including cell proliferation and cytokine production but also antibody generation by B cells (16). Specifically, PD-1 has been associated with decreased cytokine production and proliferation in T cells as well as enhancing disease progression, whereas CTLA-4 has been associated with T cell anergy and establishment of immunological tolerance (17, 18). Furthermore, it has been shown that the dysfunctional nature of exhausted T cells in murine models of malaria can be reversed by blockage of these receptors as this enhances effective parasite clearance and acquisition of immunity (16, 19).
In addition to immune exhaustion, infectious pathogens such as Cytomegalovirus (CMV) and Human Immune deficiency virus (HIV) have been associated with accelerated aging of the body's immune defense system through the upregulation of CD57, a classical marker for immune senescence (20, 21). CD57 is a terminally differentiated marker found on some cell subsets including T cells (22–24). Naïve T cells express CD28, a co-stimulatory molecule that provides signaling for T cell activation) after antigen recognition and this may bind to B7 proteins to provide co-stimulatory signals (25, 26). However, repeated T cell activation is associated with the progressive loss of CD28, a characteristic of memory or terminally differentiated cells, and the corresponding upregulation of CD57 (27–29). These senescent cells are characterized by shortened telomeres, replicative senescence, loss of CD27 resulting in a low proliferative capacity of the cells (30), eventually, leading to an inability to eradicate an infection. Importantly, the expression of CD57 is associated with repeated antigen stimulation (31) which was identified to accurately predict replicative senescence (22). In addition, CD57 expression on CD28- T cells has been shown to differ from the normal aging T cell phenotypes (CD28-CD57+, similarly observed in CMV) (31, 32) found in HIV infections (33).
Cellular aging has been described in wild birds chronically infected with malaria (34). Interestingly, a recent study reported evidence of cellular aging in travelers with single acute P. falciparum infections, characterized by decreased telomerase activity and increased levels of CDKN2A, a molecular marker associated with cellular aging (35). Nevertheless, it remains to be elucidated if frequent exposure to malaria is associated with increased expression of markers of T cell senescence in endemic areas. Here, we determined the expression profile of inhibitory or exhaustive, and immune-senescence markers on both CD4+ and CD8+ T cells. We characterized the expression of PD-1, CTLA-4, CD28 and CD57 markers in children with symptomatic malaria, asymptomatic malaria and healthy controls. In addition, we also determined the impact of these T cell phenotypes on parasitaemia and inflammation (using the platelet-to-lymphocyte ratio).
Materials and Methods
Ethics Statement
The study protocols were approved by the Institutional Review Board of the Noguchi Memorial Institute for Medical Research at the University of Ghana. All participants were children and informed consent was obtained from parents or guardians and assent properly received from the children before they were enrolled in the study. All methods were performed in accordance with the relevant guidelines and regulations.
Study Subjects
A total of 57 children within the age ranges of 1–12 years were recruited for the study, consisting of healthy children with no P. falciparum infections (n = 17), children with asymptomatic P. falciparum malaria (n = 18) and children with clinical malaria (n = 22) who were recruited from the Asutsuare and the Paakro sub-districts which are hyper-endemic areas for malaria transmission in Ghana. A volume of 5 ml of venous blood was collected from all study participants after recruitment. Parasites were identified using Giemsa stained thick and thin blood films. Clinical cases of malaria recruited from the health centers were defined by a history of fever within 24 h of health center attendance and presence of parasitaemia. For clinical cases, we collected venous blood samples from the children before anti-malarial treatment, based on the nationally recommended guidelines. Asymptomatic cases were recruited from the community and were defined by the presence of parasitaemia, absence of fever and no signs or symptoms of the disease. Healthy children, also recruited from the community were selected based on the absence of parasitaemia, fever and no signs or symptoms of the disease.
Peripheral Blood Mononuclear Cells (PBMC) and Plasma Isolation
Isolation of PBMCs was performed by density gradient centrifugation using ficoll paque. After isolation, PBMCs were enumerated and cryopreserved in fetal bovine serum with 10% dimethyl sulfoxide. PBMCs were kept at −80°C overnight and subsequently transferred to liquid nitrogen until required for the experiment.
Flow Cytometry Analysis
PBMCs were retrieved, thawed and washed. The viability was measured by the trypan blue dye exclusion method and cells with viability > 95% were used in the assay. All the antibodies were purchased from BD except anti-human FOXP3 fluorochrome-conjugated antibody (Biolegend). After washing, the cells were extracellularly stained with the following antibodies: anti-CD3-(APC H7), anti-CD4 (BUV 395), anti-CD8 (PerCP Cy5.5), anti-CD28 (APCR700), anti-CD57 (FITC) and anti-PD-1 (BUV737) on ice for 30 min. The cells were washed, fixed and permeabilized using FOXP3 buffer set (BD) according to manufacturer's instructions and intracellularly stained for FOXP3 (PE) and CTLA-4 (APC) on ice for 40 min. We gated for T cells by CD3, CD4, and CD8 lineage markers. Gates for inhibitory and senescence markers were defined using fluorescence minus one controls (Figure S1). Cells were acquired on a BD LSR Fortessa II-X20 cytometer. Data were compensated and analyzed using Flowjo V10 software (Tree Star, San Carlos, CA).
Statistical Analysis
Data analyses were performed with R-studio for statistical analysis (version 2) and the GraphPad Prism version 6.01 (GraphPad Software, Inc.). For comparing the markers of T cells among the three study populations, the Kruskal-Wallis test with a Dunn's post hoc test for multiple comparisons was used. Spearman's rank correlation was used to determine associations between markers. Principal component analysis (PCA) was conducted to identify and visualize significant features of T cell phenotypes (degree of variation) that can cluster our study populations by considering all phenotypes measured. PCA which is an unsupervised learning algorithm provides dimensions (linear combinations) along which the data are separable and reduces the noise associated with data whilst increasing its robustness. PCA was used since it reduces the data set to a small set of patterns and retain the significant features that are responsible for variation (separating the data into clusters). Multiple linear regression models with likelihood ratio test were also used to investigate the association between parasitaemia or inflammation and the measured cellular markers. Statistical significance was set at P < 0.05.
Results
Clinical Characteristics of the Study Participants
The study was approved by the Noguchi Memorial Institute for Medical Research Institutional Review Board. This was a cross-sectional study in which we recruited 57 children in the age range of 1–12 years. The participants included 22 symptomatic children, 18 asymptomatic children and 17 healthy controls (Table 1). The sexes of the children were comparable amongst the study groups (p < 0.05). Healthy children were older than the asymptomatic(p < 0.05) and symptomatic children (p < 0.05). Levels of parasitemia mirrored the intensity of infection, with symptomatic children having a higher parasite load compared to the asymptomatic children (p < 0.001). Hemoglobin levels were significantly decreased in the symptomatic children in comparison to the asymptomatic children (p < 0.05). Even though, the lymphocyte count was not significantly different amongst the study groups, they also mirrored the intensity of infection (p>0.05). We found the granulocyte count to be comparable amongst the study groups (p > 0.05). Also, the platelet-to-lymphocyte ratio (PLR) was found to be comparable between the healthy controls and asymptomatic groups (p < 0.05) but higher than the symptomatic group (p < 0.05).
Increased Expression of PD-1 and CTLA-4 Markers on T Cells in Children With Symptomatic P. falciparum Malaria
We first investigated the expression of the inhibitory markers PD-1 and CTLA-4 on T cells (Figure 1A). The expression levels of PD-1 were significantly upregulated in the symptomatic children compared to the asymptomatic (p < 0.0001) and healthy groups (p < 0.0001) for the CD4+ T cells (Figure 1B). Levels of PD-1 in the asymptomatic children and healthy children were comparable. Similarly, CD8+PD-1+ T cells were upregulated in children with symptomatic malaria compared to asymptomatic (p = 0.0312) and uninfected controls (p < 0.0001). Nevertheless, the expression of PD-1 on CD8+ T cells was increased significantly in the asymptomatic children compared to the healthy controls (p = 0.0359). Of note, the levels of PD-1 were higher in CD8+ T cells compared to the CD4+ T cells in all study groups. Also, the expression levels of CTLA-4 on CD4+ T cells were increased significantly in the symptomatic children compared to the asymptomatic (p < 0.001) and healthy controls (p < 0.05) whereas comparable levels of expression were found between asymptomatic children and healthy controls (Figure 1C). This trend was the same for the levels of CTLA-4 expression on CD8+ T cells among the study groups: symptomatic children had increased levels compared to asymptomatic (p < 0.001) and healthy children (p < 0.05).
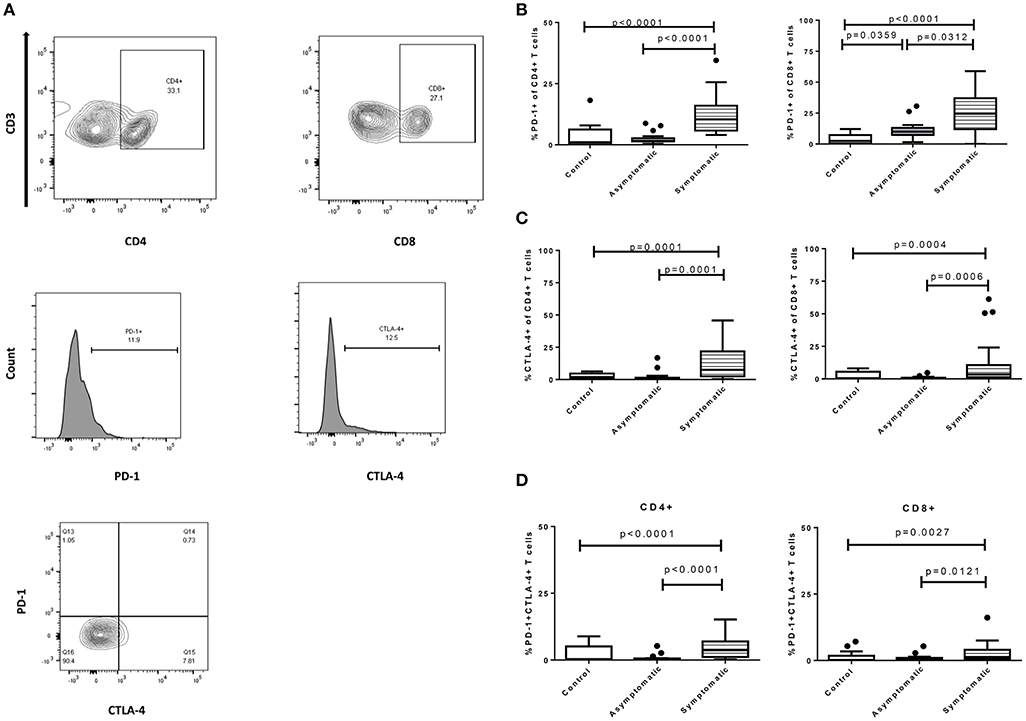
Figure 1. Expression profile of exhaustion and regulatory markers on CD4+ and CD8+ T cells among symptomatic malaria patients (n = 22), asymptomatic malaria(n = 18) and healthy children (n = 16). (A) The gating strategy to identify expression levels of PD-1 and CTLA-4 markers. The expression profile comparing levels of (B). PD-1, (C). CTLA-4, (D). PD-1 and CTLA-4 double-positive markers, on both CD4+ and CD8+ T cells across the study subjects. Levels of expression were compared using the Kruskal-Wallis with Dunn's test to correct for multiple comparisons. The data are presented as box plots with inter-quartile ranges. The 10th and 90th percentiles are presented as whiskers with medians indicated by the bold horizontal lines across the boxes. P < 0.05 were considered statistically significant.
Next, we assessed the expression of PD-1 and CTLA-4 double-positive markers on both T cell subsets. The symptomatic children had significantly higher levels of PD-1 and CTLA-4 double positive markers on CD4+ T cells in comparison to the asymptomatic children (p < 0.0001) and healthy controls (p = 0.0091). Similarly, levels on CD8+ T cells were higher in symptomatic children compared to the asymptomatic children (p = 0.0121) and healthy controls (p = 0.0098) (Figure 1D). In all, levels of PD-1 and CTLA-4 double positive markers between the asymptomatic children and healthy controls were comparable and not significantly different.
The significant levels of inhibitory markers observed in children with symptomatic malaria may be related to the inadequacy of effector functions in clearing parasitemia.
Symptomatic P. falciparum Infection Is Associated With the Upregulation of T-Cell Senescence Markers
We also determined if symptomatic malaria may be associated with the biological aging of T cells, by measuring senescent markers using CD28 and CD57 and comparing the proportions with the asymptomatic and healthy groups. We first determined the proportions of T cells expressing CD57 which were found to higher on CD8+ T cells compared to the CD4+ T cells. This trend was similar in all 3 study groups (Figure 2). Levels of CD4+CD57+ T cells were significantly higher in children with symptomatic malaria compared to asymptomatic (p = 0.0006) and healthy controls (p = 0.0041). A similar trend was observed for the CD8+ T cell subsets where a significant difference was observed between symptomatic and asymptomatic children (p = 0.0167) and healthy controls (p = 0.0050) (Figure 2A). Secondly, we checked for the percentage expression of CD28-CD57+ T cells, a marker frequently associated with T cell aging in the elderly. Levels of CD28-CD57+CD4+ T cells were also increased in children with symptomatic malaria compared to children with asymptomatic infections (p = 0.0002) and healthy controls (p = 0.0064). In contrast, levels of the CD28-CD57+ marker on CD8+ T cells did not differ between the symptomatic children and asymptomatic children (p = 0.1115), but was increased in the symptomatic group compared to healthy controls(p = 0.0178) (Figure 2B).
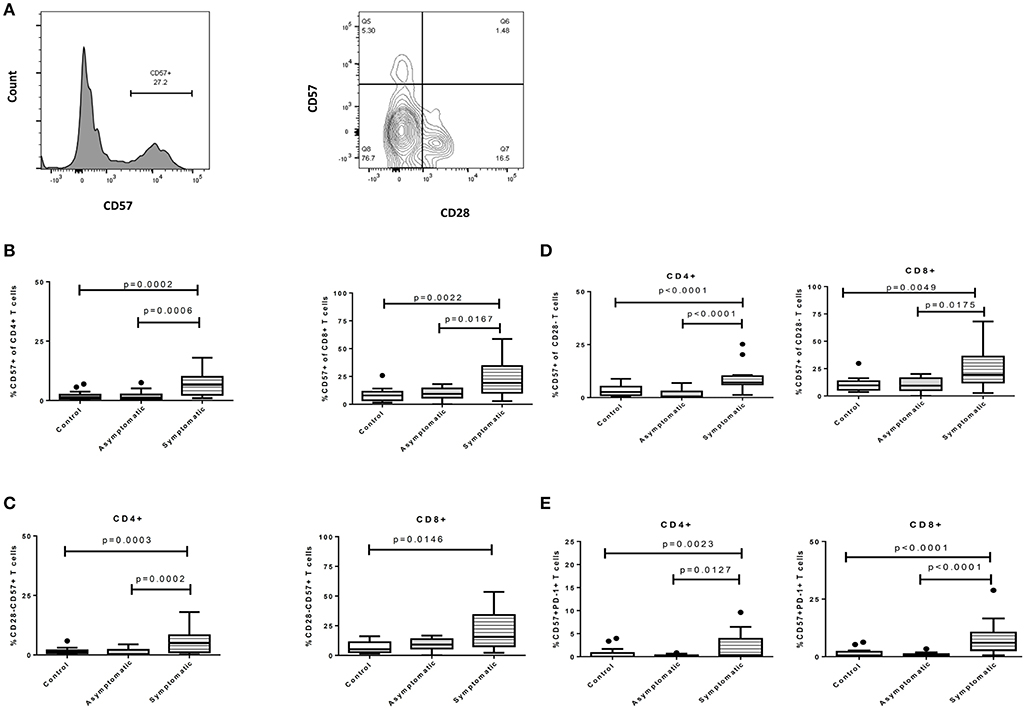
Figure 2. Symptomatic P. falciparum infection is associated with the upregulation of T-cell senescence markers. The expression profile of immune senescence markers on CD4+ and CD8+ T cells among symptomatic malaria patients, asymptomatic malaria and healthy children. (A) The gating strategy for identifying CD57 and CD28 surface markers. The expression profile comparing levels of (B). CD57, (C). CD28-CD57+, (D). CD57+ of CD28- and (E). PD-1+CD57+ markers on both CD4+ and CD8+ T cells across the study subjects. The data are presented as box plots with inter-quartile ranges. The 10th and 90th percentiles are presented as whiskers with medians indicated by the bold horizontal lines across the boxes. Levels of expression were compared among the study population using the Kruskal-Wallis with Dunn's test to correct for multiple comparisons. P < 0.05 were considered statistically significant.
Later, we gated on CD28- T cells and measured the expression of CD57 on CD28- T cells (CD57 of CD28- T cells) to determine if the expression levels may be similar or differ from what is observed in normal aging or HIV infections. We found that the percentage expression of CD57+ on CD28-CD4+ T cells remained increased in children with symptomatic malaria compared to children with asymptomatic infections (p < 0.0001) and healthy controls (p = 0.0261). Also, the percentage expression of CD57 on CD28- CD8+ T cells was significantly increased in children with symptomatic malaria compared to those with asymptomatic malaria (p = 0.0175) or healthy controls (p = 0.0147) (Figures 2B,C).
We further compared the expression of CD57 and PD-1 double-positive markers (commonly associated with increased apoptosis) on T cells in the study participants. We observed that CD4+ T cells expressing both CD57 and PD-1 were increased in children with symptomatic malaria compared to asymptomatic (p = 0.0127) and healthy controls (p = 0.0071; Figure 2D). This trend was similarly observed in the CD8+ T cells: levels of PD-1+CD57+CD8+ T cells were increased in children with symptomatic malaria in comparison to asymptomatic children (p < 0.0001) and healthy controls (p = 0.0001). Overall, T cells from symptomatic P. falciparum infected children showed phenotypic evidence of T cell senescence.
CTLA-4 Is a Major Predictor of Parasite Load During Plasmodium falciparum Infection4
The effect of cellular markers on parasitemia and inflammation was investigated using multivariate regression analysis. We analyzed 7 T cell phenotypic markers; inhibitory (PD-1+, CTLA-4+, PD-1CTLA-4+) and senescence (CD57+, CD28-CD57+, CD57+ of CD28-, PD-1+CD57+)each on both CD4+ and CD8+ T cells to determine if any of these markers could predict parasitaemia or inflammation (PLR). We defined inflammation by the ratio of platelet-to-lymphocyte count (PLR) (36–38). Before the multivariate analysis, we initially performed a correlation analysis to determine if any of the phenotypes may be significantly associated with PLR or parasitaemia. The proportions of PD-1 (r = −0.65, p < 0.01) and CTLA-4 (r = −0.506, p < 0.05) were inversely correlated with PLR (Figure S4) but positively correlated with parasitaemia (for PD-1, r = 0.4631, p < 0.05; CTLA-4, r = 0.4831, p < 0.05). However, using the multiple linear regression model and performing a likelihood ratio test, expression levels of CTLA-4 on both CD4+ and CD8+ T cells were found to significantly predict the level of parasitemia in the symptomatic children (Figure 3; Tables 2, 3). Likewise, the levels of CD8+CD28-CD57+ and CD57 on CD8+CD28- T cells could significantly explain some of the variation observed in parasitemia (Tables 2, 3). Even though all the coefficients from the regression analysis for the T cell phenotypes were inversely associated with inflammation, none could be a predictor of inflammation (Table S1).
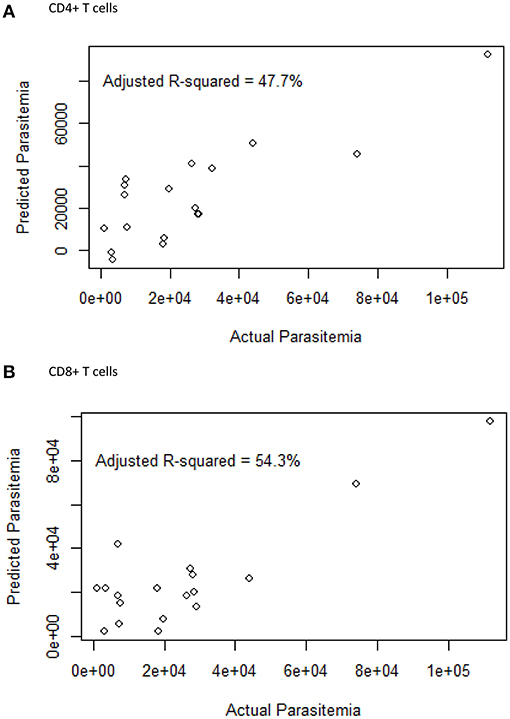
Figure 3. The relationship between the surface markers and parasitemia. A multiple linear regression plot. Actual parasitaemia levels were indicated on the x-axis with the predicted values of parasitaemia on the y-axis. The model was designed using 7 T cell phenotypes measured in (A) CD4 (PD-1, CTLA-4, CD57, PD-1CTLA-4, PD-1CD57, CD28-CD57+, CD57+ of CD28-) and (B) CD8 (PD-1, CTLA-4, CD57, PD-1CTLA-4, PD-1CD57, CD28-CD57+, CD57+ of CD28-) T cells from PBMCs obtained from the symptomatic children.
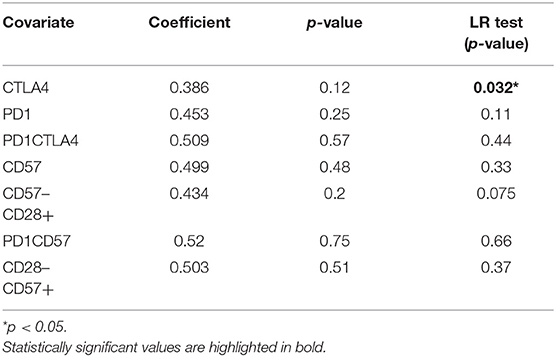
Table 2. The association of inhibitory markers on CD4+ T cells and parasitaemia for the symptomatic malaria population.
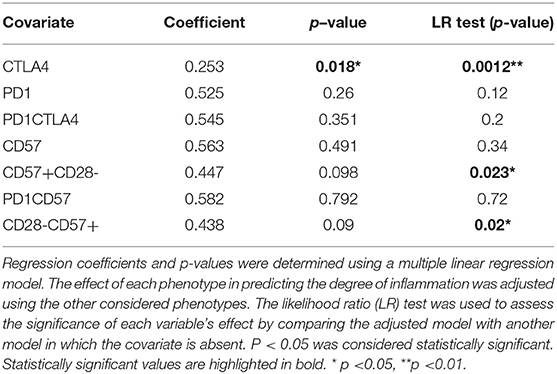
Table 3. The association of inhibitory markers on CD8+ T cells and parasitaemia for the symptomatic malaria population.
On the other hand, among the asymptomatic malaria group, levels of CD4+PD-1+ and CD4+PD-1+CD57+ could predict and explain some of the variation observed in parasitemia (p < 0.05; p < 0.0001) whereas for CD8+ T cells, the expression of CTLA-4 (p < 0.001) and PD-1+CTLA-4+ (p < 0.0001) were good predictors of parasitemia (Figure S3; Table S2).
Multivariate Analysis of T Cell Inhibitory and Senescent Markers
In order to identify significant immunological signatures (T cell phenotypes) that can explain the variation in our study population and separate our study population into clusters, we performed a principal component analysis (PCA). From the eigen values we obtained, we selected principal components that best explained the variations in the datasets. Components 1 and 2 for the CD4+ T cells accounted for 73.1% (62 and 11.7%, respectively) of the variation in data whereas, for CD8+ T cells, PC1 and PC2 accounted for 81.1% (56.3 and 24.8, respectively). From the plots, it can be observed that mostly the symptomatic group had higher PC values compared to the asymptomatic group. Using the entire datasets, the principal components clustered our population into three groups based on the frequencies of the phenotypes (Figures 4A,B). Also, from the CD8+ T cells, it can be observed that PC1 is associated with inhibitory markers located in the upper right quadrant whereas PC2 is associated with senescent markers, located in the lower right quadrant. In addition, the loadings of PD-1 and CD57 were significant for PC1 and PC2, respectively. Further analysis indicated the T cell phenotypes contributing to most of the variation for CD4 T cells were CTLA-4 and PD-1 whereas, for CD8 T cells, the important markers were PD-1, CD57 and CTLA4 (Figure S2).
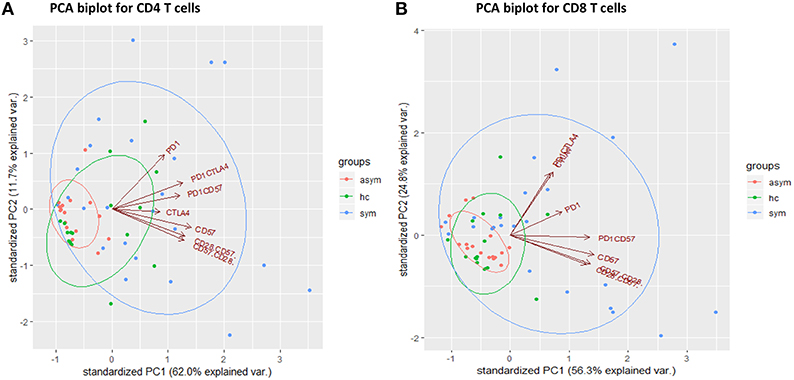
Figure 4. Principal component analysis of cellular markers determined in the healthy groups (n = 17), asymptomatic malaria (n = 18) and symptomatic malaria (n = 22), for (A) CD4 and (B) CD8 T cells. The green, red and blue symbols as well as ellipses denote the healthy, asymptomatic and symptomatic groups respectively. The red arrows indicate the cellular markers.
The Interrelationship Between the Cellular Inhibitory Markers
Next, we determined the interrelationship between the surface markers (including FOXP3) using partial correlation, a multiparametric correlation analysis that controls for confounding factors (Tables 4, 5). For instance, significant positive correlations for CD4 T cells were observed between FOXP3 and PD1, FOXP3 and CD57, PD-1CTLA-4 and CD57 (p < 0.05). Generally, significant correlations were all positively related.
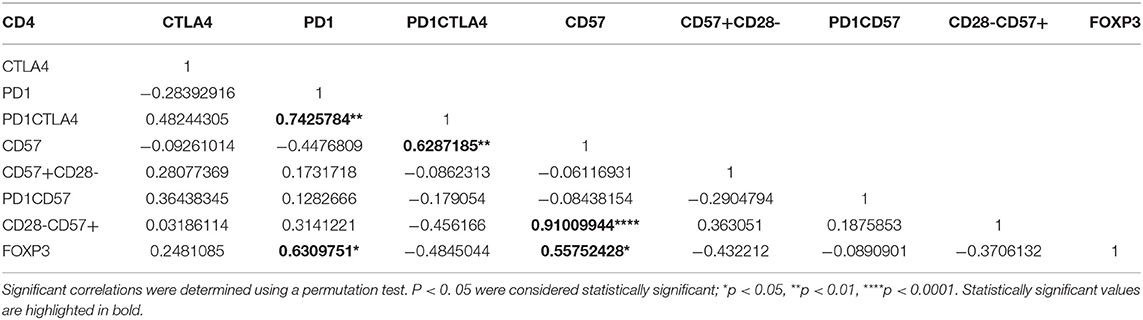
Table 4. A partial correlation matrix with covariates between the cellular markers measured on CD4+ T cells for the symptomatic malaria population.
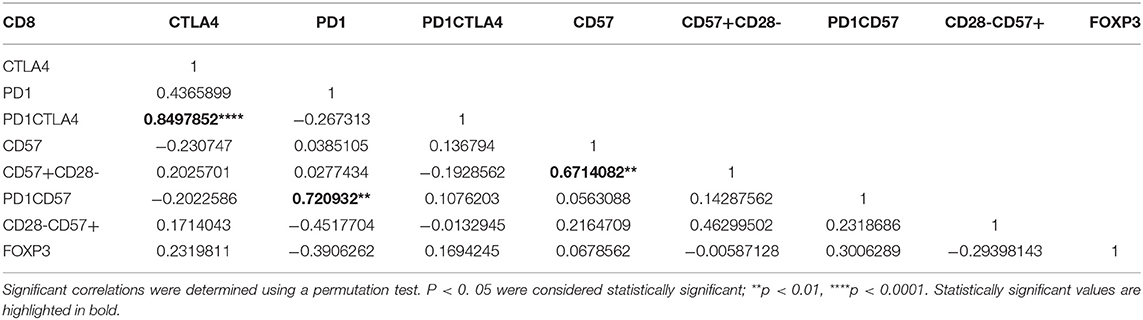
Table 5. A partial correlation matrix with covariates between the cellular markers measured on CD8+ T cells for the symptomatic malaria population.
Discussion
The upregulation of inhibitory and senescent markers on T cells has been associated with the impairment of effector T cell responses. In this study, we sought to identify T-cell immune signatures that may be associated with the development of symptomatic malaria. We analyzed the pattern of expression of co-inhibitory and senescent markers in children with symptomatic P. falciparum malaria, asymptomatic malaria and healthy controls. We found that the expression of these exhaustive and senescent markers was increased in children with symptomatic malaria compared to those with asymptomatic infections and healthy controls. Using multivariate regression analysis with likelihood ratio test, we found CTLA-4 to be a strong predictor of parasitemia levels. Also, none of the T cell phenotypes measured was a good predictor of inflammation, even though PD-1 and CTLA-4 were inversely correlated with inflammation. Using a principal component analysis, our study population was clustered into three groups based on the level of expression of the cellular markers. Further analysis revealed that, for CD4+ T cells, the levels of CD4+CTLA4+ and CD4+PD-1+ markers could explain the clustering pattern of the study groups, whereas for CD8+ T cells the important markers were CD8+PD-1+, CD8+CD57+ and CD8+CTLA-4+. In addition, we observed a lower platelet-to-lymphocyte ratio in the symptomatic malaria group probably resulting from the decreased platelets and lymphocytes counts that are associated with clinical malaria (39, 40).
The activation of T cells by pathogens leads to the induction of inhibitory receptors such as CTLA-4 and PD-1 (41). PD-1 and CTLA-4 are some of the well-characterized inhibitory receptors associated with the exhaustion of T cells (16, 42, 43). Levels of expression of the inhibitory markers PD-1 and CTLA-4 were upregulated in children with symptomatic malaria, confirming recent studies that have observed increased levels of PD-1 and CTLA-4 during acute infections (14, 44, 45), resulting in decreased production of cytokines (46). This, therefore, suggests that the increased frequency of inhibitory markers during clinical disease may alter the effector function of T cells.
It has been shown by Butler et al. (16) that levels of CD4+PD-1+ phenotypes correlate with parasitemia in clinical malaria. In this study, we found that CD4+PD-1+ and CD8+CTLA-4+ could also predict parasitemia levels in the asymptomatic malaria group. Importantly, in the symptomatic group, the expression of CTLA-4 was a major predictor in determining parasitemia load. This suggests that T cell exhaustion may induce tolerance which may promote parasitemia. Since both PD-1 and CTLA-4 are negative regulators of the immune response, their observed increase in symptomatic children may indicate that PD-1 and CTLA-4 contribute in regulating T cell activity or inflammation (14, 46). Interestingly, we found an inverse association between these inhibitory markers and inflammation, which is in line with previous observations in murine models that blockage of T cell inhibitory markers exacerbated the immune response, increased susceptibility to severe disease and decreased survival (47, 48). Furthermore, the strong association we observed between T cell exhaustion and clinical parameters such as parasitemia and inflammation suggests that T cell exhaustion plays a vital role in malaria pathogenesis.
We have previously shown that asymptomatic P. falciparum infections are characterized by a reduced frequency of regulatory T cells (49). Here, we hypothesized that asymptomatic infections may have reduced expression of inhibitory markers compared to symptomatic children. We found that the expression levels of inhibitory markers in asymptomatic and healthy controls were mostly comparable except for PD-1 which was increased in the P. falciparum infected groups compared to the healthy controls (43). It may indicate PD-1 expression may be driven by P. falciparum infections. This could also imply that continuous exposure to P. falciparum infections may render T cells to be defective in function. Unfortunately, we could not determine the functionality of these T cells to confirm this.
Immunosenescence is the aging of immune cells characterized by shortened telomeres and inability to replicate, (50) sensitivity to apoptosis and, phenotypically, the expression of CD57 (22). T cell senescent markers were more associated with CD8+ T cells compared to CD4+ T cells consistent with earlier reports that they accumulate at lower frequencies for CD4+ T cells in human periphery (51). Here, we found an increased expression of CD57+T cell subsets in children with symptomatic malaria (52). This may suggest that malaria accelerates the aging of the T cell pool. In addition, the increased expression of CD28-CD57+ marker observed in symptomatic children indicates a greater proportion of effector T cells in symptomatic malaria have a memory phenotype since these cell subsets have been described to be antigen experienced (22, 31, 51). It has previously been shown that PD-1+CD57+CD8+ T cells have increased sensitivity to apoptosis mediated by PD-1 (53). The observed increase in expression of CD57 and PD-1 double-positive markers on CD8+ T cells, therefore, indicates a greater risk of apoptosis of these cells in clinical malaria. Additionally, T cell aging has been well characterized in the elderly population, CMV and HIV infections (21, 30, 33). In contrast to CMV infections which leads to expansion of T cell senescent markers, HIV leads to a decrease in the expression of CD57 on CD28-CD8+ gated T cells, whereas levels of CD8+CD28-CD57+ remains unchanged. In our study, we observed that both the proportion of CD28- T cells that express CD57 were expanded in the symptomatic malaria group, suggesting that T cell aging in falciparum infections is more similar to that observed during CMV infections than in HIV infections. Together, these observed phenotypic changes might reduce the responsiveness of the T cell repertoire to P. falciparum antigens resulting in an impaired ability to eliminate parasitemia.
FOXP3 is an immune regulatory marker associated with preventing immunopathology during inflammation. Both FOXP3 and PD-1 have been shown to suppress host immune response. Importantly, P. falciparum infections has been reported to cause the induction of PD-1+CTLA4+ T cells that control T cell activity (14). In this study, the positive correlation observed between FOXP3 and PD-1 T cells as well as between PD-1 and PD-1CTLA4 T cells could indicate that these markers play complementary roles in mediating the increasing immune activation that is associated with symptomatic malaria. There are conflicting reports about the role of CD57+ T cells in clinical disease, with some reports describing them as immunosuppressive and others suggesting they exacerbate immune activity through IFNγproduction (31, 54). Here, we observed a significant positive correlation between CD4+CD57+ T cells and CD4+FOXP3+ T cells as well as between CD4+CD57+ and CD4+PD-1+CTLA-4+ T cell subsets. However, since we could not determine the functionality of the CD57 T cell subsets, we can only suggest that the positive relationship observed between CD4+CD57 and CD4+FOXP3+ as well as CD4+ PD-1+CTLA-4+ T cell subsets may indicate that CD4+CD57+ T cells play suppressive roles during clinical malaria. This further supports the view that Plasmodium infections induce immunosuppressive immune responses that enhance the development of tolerance to the parasite, a mechanism affecting the development of sterile immunity.
Also, the results from the principal component analysis may indicate that a selection panel of the considered markers may serve as a biomarker for identifying individuals with symptomatic disease. It may probably be used to predict the outcome or immune response to vaccination. These results provide a basis to perform functional assays to determine the impact of the considered markers on the acquisition of anti-disease immunity during P. falciparum infections, preferably in a longitudinal cohort.
Studies have reported a low ratio of platelet-to-lymphocyte count (PLR) as a marker for inflammation in various infectious diseases such as HBV (36) and HCV (37). In this study, even though none of our markers was a good predictor of inflammation as previously stated using the PLR, we show that symptomatic malaria is characterized by low ratio of platelet-to-lymphocyte counts, which is indicative of on-going inflammatory response. Nonetheless, additional studies are needed to determine the significance of other hematological markers of inflammation (such as the neutrophil to lymphocyte ratio) to ascertain their clinical relevance during symptomatic malaria.
This study had a number of limitations due to the cross-sectional nature. We could not determine the effect of anti-malarial treatment on the expression of these inhibitory and senescent markers since samples were taking before the initiation of treatment. Furthermore, we defined P. falciparum infections by microscopy which is not able to distinguish between microscopic and sub-microscopic infections. In addition, we could not determine the effect of these markers on T cell cytokine production since cytokine profile analysis was not performed.
Despite these shortcomings, this study shows evidence that the phenotypic defect of T cells during P. falciparum infections are more pronounced in clinical malaria and associated with higher expression of exhaustive and senescent markers compared to asymptomatic infections. CTLA-4 was a good predictor of parasitemia in both symptomatic and asymptomatic malaria groups. Also, using the platelet to lymphocyte ratio, none of the markers measured could predict inflammation. In addition, we observed that the aging phenotype of T cells in malaria infection is similar to that observed with normal aging and CMV infections. These may imply that the increased expression of these markers may be associated with the absence of sterile immunity to P. falciparum malaria.
Ethics Statement
This study was carried out in accordance with the recommendations of the Noguchi Memorial Institute for Medical Research, ethical review committee with written informed consent from all subjects. All subjects gave written informed consent and assent in accordance with the Declaration of Helsinki. The protocol was approved by the Institutional Review Board of the Noguchi Memorial Institute for Medical Research at the University of Ghana (NMIMR-IRB CPN 096/15-16).
Consent for Publication
All authors have read and agreed to the content of this manuscript and its publication upon acceptance.
Author Contributions
AF and MO conceived the idea and designed the experiments. WN, MO, and KK supervised the work. AF performed the experiments in the study and was assisted by DA-G and JA. AF, KK, MO and, WN wrote the paper. All authors read and approved the final manuscript.
Funding
Frimpong Augustina is supported by World Bank African Centres of Excellence Grant (ACE02-WACCBIP: Awandare) Ph.D. fellowship. This work was supported by grants from the International Development Research Center through the African Institute for Mathematical Sciences, Ghana, the L'Oreal-UNESCO for Women in Science, the Carnegie Corporation of New York and the University of Ghana BanGA.
Conflict of Interest Statement
The authors declare that the research was conducted in the absence of any commercial or financial relationships that could be construed as a potential conflict of interest.
Acknowledgments
The authors are grateful to the children, parents and guardians who participated in this study. We are also grateful to the clinical staffs at the Shai-Osudoku Health Directorate. We thank the technical staff at the Immunology Department, NMIMR for their technical support, Cell Biology and Immunology Lab, WACCBIP, and the University of Ghana's Malaria Centre of Excellence.
Supplementary Material
The Supplementary Material for this article can be found online at: https://www.frontiersin.org/articles/10.3389/fimmu.2019.01345/full#supplementary-material
References
2. Ranson H, Lissenden N. Insecticide resistance in African Anopheles mosquitoes: a worsening situation that needs urgent action to maintain malaria control. Trends Parasitol. (2016) 32:187–96. doi: 10.1016/j.pt.2015.11.010
3. Leang R, Taylor WR, Bouth DM, Song L, Tarning J, Char MC, et al. Evidence of Plasmodium falciparum malaria multidrug resistance to artemisinin and piperaquine in western Cambodia: dihydroartemisinin-piperaquine open-label multicenter clinical assessment. Antimicrob Agents Chemotherap. (2015) 59:4719–26. doi: 10.1128/AAC.00835-15
4. Ashley EA, Dhorda M, Fairhurst RM, Amaratunga C, Lim P, Suon S, et al. Spread of artemisinin resistance in Plasmodium falciparum malaria. N Engl J Med. (2014) 371:411–23. doi: 10.1056/NEJMoa1314981
5. Sissoko MS, Healy SA, Katile A, Omaswa F, Zaidi I, Gabriel EE, et al. Safety and efficacy of PfSPZ Vaccine against Plasmodium falciparum via direct venous inoculation in healthy malaria-exposed adults in Mali: a randomised, double-blind phase 1 trial. Lancet Infect Dis. (2017) 17:498–509. doi: 10.1016/S1473-3099(17)30104-4
6. White MT, Verity R, Griffin JT, Asante KP, Owusu-Agyei S, Greenwood B, et al. Immunogenicity of the RTS, S/AS01 malaria vaccine and implications for duration of vaccine efficacy: secondary analysis of data from a phase 3 randomised controlled trial. Lancet Infect Dis. (2015) 15:1450–58. doi: 10.1016/S1473-3099(15)00239-X
7. Nlinwe ON, Kusi KA, Adu B, Sedegah M. T-cell responses against Malaria: Effect of parasite antigen diversity and relevance for vaccine development. Vaccine. (2018) 36:2237–42 doi: 10.1016/j.vaccine.2018.03.023
8. Li X, Huang J, Zhang M, Funakoshi R, Sheetij D, Spaccapelo R, et al. Human CD8+ T cells mediate protective immunity induced by a human malaria vaccine in human immune system mice. Vaccine. (2016) 34:4501–06. doi: 10.1016/j.vaccine.2016.08.006
9. Walther M, Tongren JE, Andrews L, Korbel D, King E, Fletcher H, et al. Upregulation of TGF-β, FOXP3, and CD4+ CD25+ regulatory T cells correlates with more rapid parasite growth in human malaria infection. Immunity. (2005) 23:287–96. doi: 10.1016/j.immuni.2005.08.006
10. Blackburn SD, Shin H, Haining WN, Zou T, Workman CJ, Polley A, et al. (2009) Coregulation of CD8+ T cell exhaustion by multiple inhibitory receptors during chronic viral infection. Nat Immunol. 10:29. doi: 10.1038/ni.1679
11. Boni C, Fisicaro P, Valdatta C, Amadei B, Di Vincenzo P, Giuberti T, et al. Characterization of hepatitis B virus (HBV)-specific T-cell dysfunction in chronic HBV infection. J Virol. (2007) 81:4215–25. doi: 10.1128/JVI.02844-06
12. Hwang S, Cobb DA, Bhadra R, Youngblood B, Khan IA. Blimp-1–mediated CD4 T cell exhaustion causes CD8 T cell dysfunction during chronic toxoplasmosis. J Exp Med. (2016) 213:1799–818. doi: 10.1084/jem.20151995
13. Esch KJ, Juelsgaard R, Martinez PA, Jones DE. Petersen CA. Programmed Death 1–mediated T cell exhaustion during visceral leishmaniasis impairs phagocyte function. J Immunol. (2013) 191:5542–50. doi: 10.4049/jimmunol.1301810
14. Mackroth MS, Abel A, Steeg C, Schulze Zur Wiesch J, Jacobs T.Mackroth MS, Abel A, Steeg C, zur Wiesch JS, Jacobs T. Acute malaria induces PD1+ CTLA4+ effector T cells with cell-extrinsic suppressor function. PLoS Pathog. (2016) 12:e1005909. doi: 10.1371/journal.ppat.1005909
15. Horne-Debets JM, Faleiro R, Karunarathne DS, Liu XQ, Lineburg KE, Poh CM, et al. PD-1 dependent exhaustion of CD8+ T cells drives chronic malaria. Cell Rep. (2013) 5:1204–13. doi: 10.1016/j.celrep.2013.11.002
16. Butler NS, Moebius J, Pewe LL, Traore B, Doumbo OK, Tygrett LT, et al. Therapeutic blockade of PD-L1 and LAG-3 rapidly clears established blood-stage Plasmodium infection. Nat Immunol. (2012) 13:188. doi: 10.1038/ni.2180
17. Yang ZZ, Grote DM, Ziesmer SC, Xiu B, Novak AJ, Ansell SM. PD-1 expression defines two distinct T-cell sub-populations in follicular lymphoma that differentially impact patient survival. Blood Cancer J. (2015) 5:e281. doi: 10.1038/bcj.2015.1
18. Jago CB, Yates J, Câmara NO, Lechler RI, Lombardi G. Differential expression of CTLA-4 among T cell subsets. Clin Exp Immunol. (2004) 136:463–71. doi: 10.1111/j.1365-2249.2004.02478.x
19. Kurup SP, Obeng-Adjei N, Anthony SM, Traore B, Doumbo OK, Butler NS, et al. Regulatory T cells impede acute and long-term immunity to blood-stage malaria through CTLA-4. Nat Med. (2017) 23:1220. doi: 10.1038/nm.4395
20. Papagno L, Spina CA, Marchant A, Salio M, Rufer N, Little S, et al. Immune activation and CD8+ T-cell differentiation towards senescence in HIV-1 infection. PLoS Biol. (2004) 2:e20. doi: 10.1371/journal.pbio.0020020
21. Pawelec G, Derhovanessian E. Role of CMV in immune senescence. Virus Res. (2011) 157:175–9. doi: 10.1016/j.virusres.2010.09.010
22. Brenchley JM, Karandikar NJ, Betts MR, Ambrozak DR, Hill BJ, Crotty LE, et al. Expression of CD57 defines replicative senescence and antigen-induced apoptotic death of CD8+ T cells. Blood. (2003) 101:2711–20. doi: 10.1182/blood-2002-07-2103
23. Strauss-Albee DM, Horowitz A, Parham P, Blish CA. Coordinated regulation of NK receptor expression in the maturing human immune system. J Immunol. (2014) 193:4871–9. doi: 10.4049/jimmunol.1401821
24. Fletcher JM, Vukmanovic-Stejic M, Dunne PJ, Birch KE, Cook JE, Jackson SE, et al. Cytomegalovirus-specific CD4+ T cells in healthy carriers are continuously driven to replicative exhaustion. J Immunol. (2005) 175:8218–25. doi: 10.4049/jimmunol.175.12.8218
25. Effros RB. Loss of CD28 expression on T lymphocytes: a marker of replicative senescence. Dev Comp Immunol. (1997) 21:471–8. doi: 10.1016/S0145-305X(97)00027-X
26. Harding FA, McArthur JG, Gross JA, Raulet DH, Allison JP. (1992) CD28-mediated signalling co-stimulates murine T cells and prevents induction of anergy in T-cell clones. Nature. 356:607–9. doi: 10.1038/356607a0
27. Li G, Larregina AT, Domsic RT, Stolz DB, Medsger TA, Lafyatis R, et al. Skin-resident effector memory CD8+ CD28–T cells exhibit a profibrotic phenotype in patients with systemic sclerosis. J Invest Dermatol. (2017) 137:1042–50. doi: 10.1016/j.jid.2016.11.037
28. Yu HT, Park S, Shin EC, Lee WW. T cell senescence and cardiovascular diseases. Clin Exp Med. (2016) 16:257–63. doi: 10.1007/s10238-015-0376-z
29. Kaplan RC, Sinclair E, Landay AL, Lurain N, Sharrett AR, Gange SJ, et al. T cell activation and senescence predict subclinical carotid artery disease in HIV-infected women. J Infect Dis. (2011) 203:452–63. doi: 10.1093/infdis/jiq071
30. Olsson J, Wikby A, Johansson B, Löfgren S, Nilsson BO, Ferguson FG. Age-related change in peripheral blood T-lymphocyte subpopulations and cytomegalovirus infection in the very old: the Swedish longitudinal OCTO immune study. Mech Ageing Dev. (2001) 121:187–201. doi: 10.1016/S0047-6374(00)00210-4
31. Strioga M, Pasukoniene V, Characiejus D. CD8+ CD28- and CD8+ CD57+ T cells and their role in health and disease. Immunology. (2011) 134:17–32. doi: 10.1111/j.1365-2567.2011.03470.x
32. Onyema OO, Njemini R, Bautmans I, Renmans W, De Waele M, Mets T. Cellular aging and senescence characteristics of human T-lymphocytes. Biogerontology. (2012) 13:169–81. doi: 10.1007/s10522-011-9366-z
33. Lee SA, Sinclair E, Hatano H, Hsue PY, Epling L, Hecht FM, et al. Impact of HIV on CD8+ T cell CD57 expression is distinct from that of CMV and aging. PLoS ONE. (2014) 9:e89444. doi: 10.1371/journal.pone.0089444
34. Asghar M, Hasselquist D, Hansson B, Zehtindjiev P, Westerdahl H, Bensch S. Hidden costs of infection: chronic malaria accelerates telomere degradation and senescence in wild birds. Science. (2015) 347:436–8. doi: 10.1126/science.1261121
35. Asghar M, Yman V, Homann MV, Sondén K, Hammar U, Hasselquist D, et al. Cellular aging dynamics after acute malaria infection: a 12-month longitudinal study. Aging Cell. (2018) 17. doi: 10.1111/acel.12702
36. Zhao Z, Liu J, Wang J, Xie T, Zhang Q, Feng S, Zhao Z, et al. Platelet-to-lymphocyte ratio (PLR) and neutrophil-to-lymphocyte ratio (NLR) are associated with chronic hepatitis B virus (HBV) infection. Int Immunopharmacol. (2017) 51:1-8. doi: 10.1016/j.intimp.2017.07.007
37. Meng X, Wei G, Chang Q, Peng R, Shi G, Zheng P, et al. The platelet-to-lymphocyte ratio, superior to the neutrophil-to-lymphocyte ratio, correlates with hepatitis C virus infection. Int J Infect Dis. (2016) 45:72–7. doi: 10.1016/j.ijid.2016.02.025
38. Fest J, Ruiter R, Ikram MA, Voortman T, van Eijck CHJ, Stricker BH. Reference values for white blood-cell-based inflammatory markers in the Rotterdam Study: a population-based prospective cohort study. Sci Rep. (2018) 8:10566. doi: 10.1038/s41598-018-28646-w
39. Kho S, Barber BE, Johar E, Andries B, Poespoprodjo JR, Kenangalem E, et al. Platelets kill circulating parasites of all major Plasmodium species in human malaria. Blood. (2018) 132:1332–44. doi: 10.1182/blood-2018-05-849307
40. Kotepui M, Phunphuech B, Phiwklam N, Chupeerach C, Duangmano S. Effect of malarial infection on haematological parameters in population near Thailand-Myanmar border. Malaria J. (2014) 13:218. doi: 10.1186/1475-2875-13-218
41. Kaufmann DE, Walker BD. PD-1 and CTLA-4 inhibitory cosignaling pathways in HIV infection and the potential for therapeutic intervention. J Immunol. (2009) 182:5891–7. doi: 10.4049/jimmunol.0803771
42. Wherry EJ, Ha SJ, Kaech SM, Haining WN, Sarkar S, Kalia V, et al. Molecular signature of CD8+ T cell exhaustion during chronic viral infection. Immunity. (2007) 27:670–84. doi: 10.1016/j.immuni.2007.09.006
43. Illingworth J, Butler NS, Roetynck S, Mwacharo J, Pierce SK, Bejon P, et al. Chronic exposure to Plasmodium falciparum is associated with phenotypic evidence of B and T cell exhaustion. J Immunol. (2013) 190:1038–47. doi: 10.4049/jimmunol.1202438
44. Ruibal P, Oestereich L, Lüdtke A, Becker-Ziaja B, Wozniak DM, Kerber R, et al. Unique human immune signature of Ebola virus disease in Guinea. Nature. (2016) 533:100. doi: 10.1038/nature17949
45. Abel A, Steeg C, Aminkiah F, Addai-Mensah O, Addo M, Gagliani N, et al. Differential expression pattern of co-inhibitory molecules on CD4+ T cells in uncomplicated versus complicated malaria. Sci Rep. (2018) 8:4789. doi: 10.1038/s41598-018-36410-3
46. Costa PA, Leoratti FM, Figueiredo MM, Tada MS, Pereira DB, Junqueira C, et al. Induction of inhibitory receptors on T cells during Plasmodium vivax malaria impairs cytokine production. J Infect Dis. (2015) 212:1999–2010. doi: 10.1093/infdis/jiv306
47. Lepenies B, Gaworski I, Tartz S, Langhorne J, Fleischer B, Jacobs T. CTLA-4 blockade differentially influences the outcome of non-lethal and lethal Plasmodium yoelii infections. Micro Infect. (2007) 9:687–94. doi: 10.1016/j.micinf.2007.02.013
48. Hafalla JC, Claser C, Couper KN, Grau GE, Renia L, de Souza JB, et al. The CTLA-4 and PD-1/PD-L1 inhibitory pathways independently regulate host resistance to Plasmodium-induced acute immune pathology. PLoS Pathog. (2012) 8:e1002504-e1002504. doi: 10.1371/journal.ppat.1002504
49. Frimpong A, Kusi KA, Tornyigah B, Ofori MF, Ndifon W. Characterization of T cell activation and regulation in children with asymptomatic Plasmodium falciparum infection. Malaria J. (2018) 17:263. doi: 10.1186/s12936-018-2410-6
50. Gill Z, Nieuwoudt M, Ndifon W. The Hayflick limit and age-related adaptive immune deficiency. Gerontology. (2018) 64:135–9. doi: 10.1159/000478091
51. Valenzuela HF, Effros RB. Divergent telomerase and CD28 expression patterns in human CD4 and CD8 T cells following repeated encounters with the same antigenic stimulus. Clin Immunol. (2002) 105:117–25. doi: 10.1006/clim.2002.5271
52. Watanabe H, Weerasinghe A, Miyaji C, Sekikawa H, Toyabe S, Mannor MK, et al. Expansion of unconventional T cells with natural killer markers in malaria patients. Parasitol Int. (2003) 52:61–70. doi: 10.1016/S1383-5769(02)00085-5
53. Petrovas C, Chaon B, Ambrozak DR, Price DA, Melenhorst JJ, Hill BJ, et al. Differential association of programmed death-1 and CD57 with ex vivo survival of CD8+ T cells in HIV infection. J Immunol. (2009) 183:1120–32. doi: 10.4049/jimmunol.0900182
Keywords: malaria, Plasmodium falciparum, T-cell, exhaustion, immune senescence, PD-1, CTLA-4, CD57
Citation: Frimpong A, Kusi KA, Adu-Gyasi D, Amponsah J, Ofori MF and Ndifon W (2019) Phenotypic Evidence of T Cell Exhaustion and Senescence During Symptomatic Plasmodium falciparum Malaria. Front. Immunol. 10:1345. doi: 10.3389/fimmu.2019.01345
Received: 28 December 2018; Accepted: 28 May 2019;
Published: 18 June 2019.
Edited by:
Faith H. A. Osier, KEMRI Wellcome Trust Research Programme, KenyaReviewed by:
Johanna Daily, Albert Einstein College of Medicine, United StatesMaria Sophia Mackroth, University Medical Center Hamburg-Eppendorf, Germany
Copyright © 2019 Frimpong, Kusi, Adu-Gyasi, Amponsah, Ofori and Ndifon. This is an open-access article distributed under the terms of the Creative Commons Attribution License (CC BY). The use, distribution or reproduction in other forums is permitted, provided the original author(s) and the copyright owner(s) are credited and that the original publication in this journal is cited, in accordance with accepted academic practice. No use, distribution or reproduction is permitted which does not comply with these terms.
*Correspondence: Augustina Frimpong, tinafrimp@gmail.com; Wilfred Ndifon, wndifon@aims.ac.za