- 1TNO, Zeist, Netherlands
- 2Danone Food Safety Center, Utrecht, Netherlands
- 3Danone Nutricia Research, Utrecht, Netherlands
- 4Utrecht Institute of Pharmaceutical Sciences, Utrecht University, Utrecht, Netherlands
To assess the safety and efficacy of oral immune interventions, it is important and required by regulation to assess the impact of those interventions not only on the immune system, but also on other organs such as the gut as the porte d'entrée. Despite clear indications that the immune system interacts with several physiological functions of the gut, it is still unknown which pathways and molecules are crucial to assessing the impact of nutritional immune interventions on gut functioning. Here we used a network-based systems biology approach to clarify the molecular relationships between immune system and gut functioning and to identify crucial biomarkers to assess effects on gut functions upon nutritional immune interventions. First, the different gut functionalities were categorized based on literature and EFSA guidance documents. Moreover, an overview of the current assays and methods to measure gut function was generated. Secondly, gut-function related biological processes and adverse events were selected and subsequently linked to the physiological functions of the GI tract. Thirdly, database terms and annotations from the Gene ontology database and the Comparative Toxicogenomics Database (CTD) related to the previously selected gut-function related processes were selected. Next, database terms and annotations were used to identify the pathways and genes involved in those gut functionalities. In parallel, information from CTD was used to identify immune disease related genes. The resulting lists of both gut and immune function genes showed an overlap of 753 genes out of 1,296 gut-function related genes indicating the close gut-immune relationship. Using bioinformatics enrichment tools DAVID and Panther, the identified gut-immune markers were predicted to be involved in motility, barrier function, the digestion and absorption of vitamins and fat, regulation of the digestive system and gastric acid, and protection from injurious or allergenic material. Concluding, here we provide a promising systems biology approach to identify genes that help to clarify the relationships between immune system and gut functioning, with the aim to identify candidate biomarkers to monitor nutritional immune intervention assays for safety and efficacy in the general population. This knowledge helps to optimize future study designs to predict effects of nutritional immune intervention on gut functionalities.
Introduction
A well balanced immune system is key for overall health and well-being, therefore the concept of boosting immunity is gaining in popularity as today's complex world presents many potential health challenges. These health challenges range from environmental pollution, infections, the use of medication, the harmful effects of lifestyle stress and the effects of intense physical workouts on the body's natural ability to stay healthy. Risk reduction measures or immune health interventions may be effective for reducing the loss of health, loss of quality of life as well as the costs to society and health care due to immune related diseases and disorders.
Besides beneficial effects of oral immunotherapy and –prophylaxis on the functioning of the immune system itself (1–10), (re/un) balancing the immune system may also generate dis-immune toxicities. These so-called “immune-related adverse events” can come forward in the immune system itself [recently addressed in (11)], resulting in, for instance, increased incidences or severity of inflammatory diseases, but may also affect other immune system-interacting organ systems. The gut being the porte d'entrée of oral immunotherapy has been selected as one of the key priority organs to include in this study. However, despite established association studies that immunotherapy can influence gut functioning (12–16), it is still largely unknown which immune and gut function-related pathways and biomarkers are crucial to monitor in relation to gut functioning upon nutritional immune interventions.
Previously, immune cells and immune cell mediators have been described as affecting gut functions in several studies. Yu et al. showed that the mucosa of the gastrointestinal tract contains large numbers of immunocompetent cells, including mast cells, lymphocytes and granulocytes (17). These mast cells play an important role in normal physiology functions (regulation of vasodilation, vascular homeostasis, innate and adaptive immune responses, angiogenesis, and venom detoxification) and pathophysiology (including allergy, asthma, anaphylaxis, gastrointestinal disorders, many types of malignancies, and cardiovascular diseases) (18). For instance, mast cells are known to be involved in gastrointestinal motility, abdominal pain, discomfort, and gut barrier function. Mast cells are present in all compartments of the gastrointestinal (GI) tract. Upon activation, they release an array of inflammatory mediators including histamine, 5-hydroxytryptamine (5-HT), neutral proteases (tryptases, chymases, and carboxypeptidase A), prostaglandins, leukotrienes, platelet activating factor (PAF), and several cytokines including tumor necrosis factor-α (TNF-α), interleukin (IL)−3,−4,−5,−6 and granulocyte macrophage colony stimulating factor (GM-CSF) (17). When the gut is sensitized e.g., in case of irritable bowel syndrome, the infiltration of mast cells and the release of mediators are proven to be associated with disturbed motility (19). The motility is disturbed by, for example, an increase in colonic and intestinal myoelectric spike activity (20), the contraction of circular and longitudinal smooth muscle (21), and intense duodenal clusters of contraction (22). Additionally, mast cells have shown to play a role in chronic pain, mainly at the visceral level (23). There is also a positive relationship between the intestinal permeability and the number of mast cells with diarrhea predominant irritable bowel syndrome (24). The mast cell derived tryptase has been identified as a key factor that disrupts the intestinal barrier (25). Additionally mast cell mediators, such as interferon-γ (IFN-γ), TNF-α, IL-1β, IL-4, IL-13, and prostaglandin E2 (PGE2), have been shown to have destructive effects on both trans- and paracellular permeability (26).
Aside from mast cells, other immune cells and immune cell mediators can also affect gut functions, such as the intestinal barrier function. Multiple factors regulate the intestinal barrier, including exogenous factors, epithelial apoptosis, cytokines, and immune cells. Immune-induced intestinal barrier dysfunction is thought to be critical in the predisposition to and exacerbation of numerous autoimmune and inflammatory conditions, including inflammatory bowel disease (IBD), food allergy, celiac disease and diabetes (27, 28). Several mucosal immune cells have been implicated in the breakdown of intestinal barrier function such as gamma/delta-positive intestinal intraepithelial (iIELγδ+) T cells (29) and eosinophils (28). Their mediators, such as IFN-γ, TNF-α and some eosinophil granular proteins (e.g., major basic protein, eosinophil peroxidase, and eosinophilic cationic protein), promote the reorganization of several tight junction (TJ) proteins (e.g., zonula occludens-1, junctional adhesion molecule A, occludin, claudins-1 and−4) (30) or their expression (31), all resulting in a decreased epithelial barrier function. In contrast, IL-10 has been shown to positively regulate intestinal barrier function (32).
Food transit is another important feature of gut function. Food transit is influenced by the gastrointestinal motility which can be altered by inflammation and immune activation. Though the innate immune response does not seem to have a major role in muscle function, animal studies have shown that a Th1 immune response is associated with hypocontractility (33) and a Th2 immune response with hypercontractility (34) of inflamed intestinal smooth muscle (35). It has been reported that IL-1β plays an important role in decreased GI smooth muscle contractility in Th1 cytokines-dominant colitis, by downregulating C-kinase-activated protein phosphatase-1 (PP1) inhibitor, 17 kDa (CPI-17) expression (36–38). The inhibitory effect of IL-1β on the GI smooth muscle contraction can also be mediated by the upregulation of regulator of G protein signaling 4 (RGS4) expression by inhibiting NF-κB activation (38, 39). Th2 cytokines may have opposing mechanisms to downregulate RGS4 expression.
These examples illustrate that the risks and benefits of restoring or changing the immune balance by novel treatment strategies of immune-related disorders are not limited to immune resistance or the inflammatory status as such, but that these immune interventions can also impact gut physiology.
The aim of this study is to illustrate how systems biology can help in clarifying the relationships between the immune system and gut function, as well as the identification of crucial biomarkers to monitor effects on gut functions upon nutritional immune interventions in the general population. In this paper we will focus on effects of immunonutrition on five major physiological functions of the gut: transport/transit of ingested material, extracellular digestion of ingested material, intracellular digestion and metabolism, uptake of essential nutrients, and protection from injurious or allergenic material.
Materials and Methods
Literature Study on the Immune System—Gut Function Relationships
An inventory of the available literature regarding the relationship between the immune system and gut functioning was performed. The following biomedical databases were searched: CAB ABSTRACTS, Embase®, MEDLINE®, Current Contents® Search, and BIOSIS Previews®. The databases were searched between 15th of February 2017 and 15th of March 2018.
The following search string was used: ti,ab ((immune* OR immuno* OR immuni*) AND ((intestin* n/3 function) OR (gut P/0 barrier) OR (intestin* P/0 barrier) OR (gut P/0 integrity) OR (intestin* P/0 integrity) OR ((gut OR intestin*) AND (nutrient* P/0 absorption)) OR (intestin* p/0 brush p/0 border) OR (gut p/0 brush p/0 border) OR ((gut OR intestin*) AND (peristalsis OR motility Or peristaltic)) OR ((gut OR intestin*) AND (water p/0 absorption)) OR ((gut OR intestin*) AND (transit p/0 time))) NOT (HIV OR “immunodeficiency virus” OR AIDS OR “acquired immunodeficiency syndrome” OR “cell line” OR dog OR dogs OR canine OR cat OR cats OR feline OR horse OR horses OR cattle OR animal OR animals OR veterinary OR broiler OR broilers OR chick OR chicks OR chicken. The following criteria were applied to obtain the most relevant hits and reduce number of hits: 2007–2018; English; Excluding: Conference Abstract, Editorial Material, Editorial, Book Chapter, Short Survey, Conference Review, Letter, Chapter, Meeting Poster, Note, Erratum, Patent, Book, Corrected and Republished Article, Correction, Correspondence, Meeting Summary, Published Erratum, Thesis, News.
During the selection process of relevant manuscripts describing the interaction between the immune system and gut function, information from manuscripts was collected that describe several key functions and clinical endpoints of the gut. In addition, several guidance documents were studied to identify those key functions and clinical endpoints of the gut that are usually indicated by the regulatory authorities (40–42).
Selection of Candidate Biomarkers Linking the Immune System to Gut Functionality
First, the different gut functionalities were categorized based on literature and EFSA guidance documents, and an overview of the current assays and methods to measure gut function was generated. Secondly, related adverse events and biological processes were selected based on literature and expert knowledge and linked to the physiological functions of the GI tract. Thirdly, database terms and annotations from Gene Ontology [GO; (www.geneontology.org/) and CTD (http://ctdbase.org/); (43)] databases were selected that were related to the biological processes as identified previously. Next, key pathways involved in those gut functionalities and the involved genes were retrieved from the GO and CTD databases: The GO database links genes to processes (so called GO terms) related to gut function. The CTD database—enabled us to connect genes/proteins to diseases which are uniquely identified by MeSH identification numbers (so called MeSH IDs). Next, the gut-function related genes were compared with the previously identified set of immune health related genes (11). Neo4J is a graph-database with query-based calculations (Neo4j, Inc., San Mateo, CA, USA) and is used, together with Venn-diagrams (44) to visualize and calculate the unique and overlapping genes/proteins among the health endpoints/processes. Using bioinformatics enrichment tools DAVID 6.8 [https://david.ncifcrf.gov; bioinformatics resources using Kyoto Encyclopedia of Genes and Genomes (KEGG) pathway output, updated 2019] and Panther 14.1 [web-based software; www.pantherdb.org/pathway, updated 19th December 2018; (45)], the identified gut-immune markers were evaluated by predicting the effects of these gut-immune genes on gut functions. DAVID was used as tool to display the candidate biomarkers on pathway maps from KEGG to facilitate the biological interpretation in a network context. To this end, the gut-immune genes associated with a specific gut function were entered into DAVID after which the top 15 KEGG pathways related to the entered genes were retrieved.
Panther was used as tool to identify GO processes shared between the gut and immune system. Hereto, the gut-immune genes associated with a specific gut function were entered into Panther after which the top 15 GO processes related to the entered genes were retrieved. Figure 1 depicts the workflow and steps for the identification of potential candidate markers.
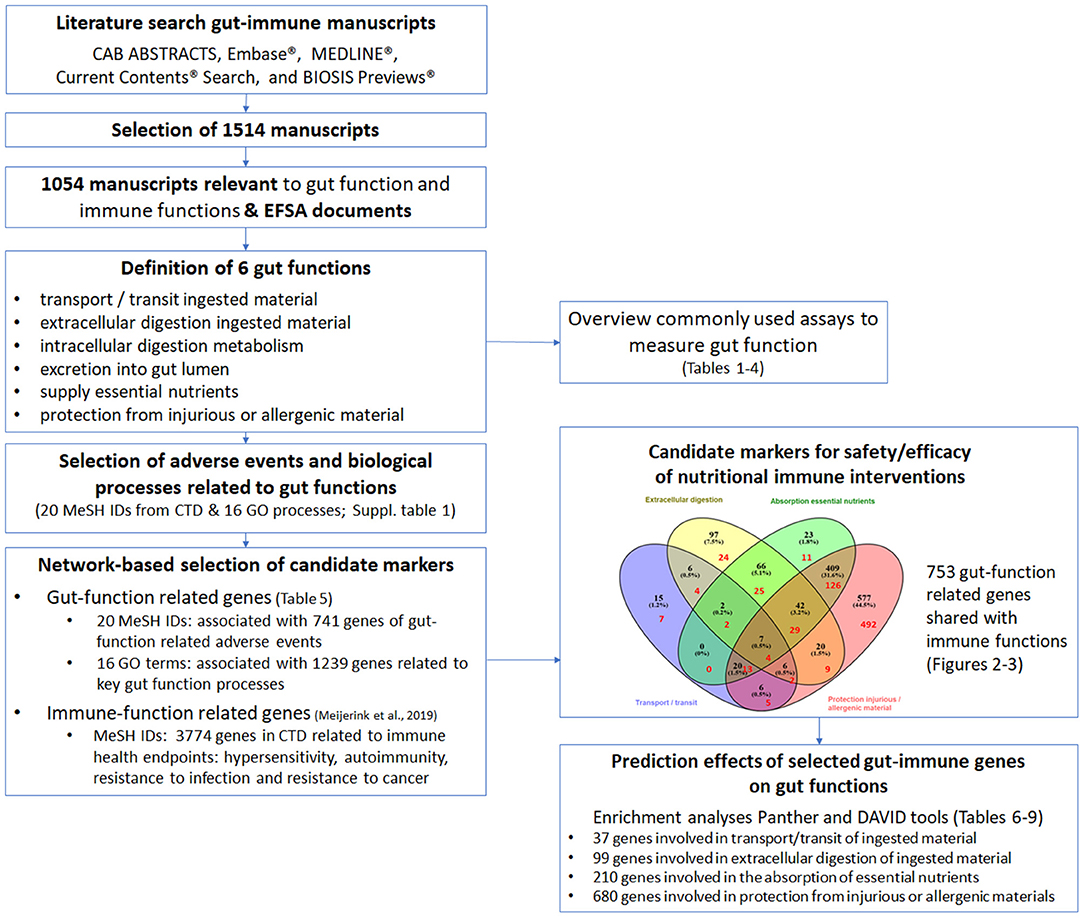
Figure 1. Schematic overview of the work flow to identify gut-function related genes possibly affected by immune interventions and to identify commonly used assays to measure gut function. The literature search resulted in a total of 1,514 manuscripts, of which 1,054 manuscripts were considered relevant to gut and immune functions. The information from the 1,054 manuscripts was collected resulting in the definition of six key gut functions. An overview was made of the commonly used assays to study gut function (depicted in Tables 1–4). The adverse events related to malfunctioning of the gut and key biological processes related to the gut functions were selected from the databases Comparative Toxicogenomics Database (CTD; 20 MeSH IDs) and GeneOntology (GO; 16 GO processes). Next the genes associated with the 16 GO processes and 20 MeSH IDs were retrieved from respectively GO (1,239 genes) and CTD (741 genes). Thereafter, CTD was used again to retrieve 3,774 immune disease related genes of the immune health endpoints hypersensitivity, autoimmunity, resistance to infection and resistance to cancer, as described previously (11). In total 753 genes were predicted to be involved in both gut functions and immune functions, indicating the strong relationship between the immune system and the gut functions. To study which biological processes in the different gut functions are predicted to be influenced by the 753 gut-immune related genes, a GO enrichment analysis (Panther web-based software; www.pantherdb.org/pathway) and an enrichment analysis using DAVID (bioinformatics resources using Kyoto Encyclopedia of Genes and Genomes (KEGG) pathway output) was performed, resulting in a top 15 selection of processes and pathways per gut function (depicted in Tables 6–9).
Proof of Principle/in silico Test Case
After selecting a large set of genes involved in the four gut functionalities, the next step was to check whether this set of genes could be validated by predicting whether an oral immune intervention can result in a disturbance of any of the gut functionalities. To this end, vitamin D was selected because it is known for its effects on (i) the immune response and (ii) adverse/beneficial effects on gut function are described, and (iii) top interacting genes are described in the CTD. The CTD contains curated data on the top interacting genes affected by a chemical/food substance. Using these curated data, the top interacting genes were compared with our previous set of gut-function related genes, to predict the effects on those gut-functionalities after which it was checked whether the predicted gut-effects could be confirmed in previously described adverse/beneficial effects on gut functionalities.
Results
Literature Study on Immune System—Gut Function Relationships
The literature study resulted in a total of 1514 articles which were reviewed. From these, 30.4% (460) were rejected and 69.6% (1, 54) were considered relevant. The reasons for rejections were: article not in English, species with low physiological similarity toward human GUT/immune system (e.g., fish, horses) or not the right focus. Of the remaining articles, 54.5% (575) were review articles and 44.5% (479) were original research articles. During the selection process of relevant articles about the interaction between immune system and gut function, information describing key functions and clinical endpoints of the gut was collected. In addition, several guidance documents were studied to identify those key functions and clinical endpoints of the gut that are usually specified by the regulatory authorities.
Key Gut Functions and Gut Assays to Study the Effects of Oral Immunotherapy
All collected clinical endpoints and coinciding analyzed parameters from literature and guidance documents were structured (see Tables 1–4). Based on this, we propose that the possible effects of immune interventions should be measured on the following four major physiological functions of the gut using the corresponding currently used assays:
1. Transport and transit of ingested material (Table 1):
The function of the healthy small intestine is to transport food, to mix it with bile and with pancreatic and intestinal secretions to facilitate digestion and absorption over the intestine mucosal surface. When there is a disturbance in gut health, common symptoms are abdominal pain or discomfort, diarrhea, constipation, fullness, and bloating. The underlying cause of constipation consists of poor intake of fluid or fiber, slow colonic transit, and outlet dysfunction in the anorectal area. Diarrhea is an increase in the volume of stool by fluid with or without frequency of defecation, and is categorized into osmotic, secretory, exudative, and altered intestinal motility. In addition, vomiting, reflux, and regurgitation are also adverse events related to this gut physiological function.
Frequently used methods to measure transit include dyes [e.g., trypan blue (46), carmine red solution (47), fluorescent labeled dextran or rhodamine B-conjugated dextran (48)] which after oral gavage the amounts are measured in tissue samples. In addition bead expulsion test (in animals) (51), X-ray contrast examination with barium meal (52) and fluorescent microscopy of whole mount preparation of the myenteric ganglia to study BIS-IR neurons (49) or GpBAR1-IR expression (50) are used to study (in)directly transit. The peristalsis/motility is often measured by smooth muscle cell contractility by exposing the muscle strip to e.g., increasing concentrations of the muscarinic agonist bethanechol (53), or indirectly by immunohistochemistry by measurement of neuronal nitric oxide synthase (54) and tyrosine kinase receptor (c-kit) and serotine (55, 56), ELISA by measurement of 5-HT and 5-Hydroxyindoleacetic acid (5-HIAA; in platelet poor/depleted plasma or urine) (57) or glucagon like peptide-1 (GLP-1), peptide YY (PYY) and somatostatin (58), vasoactive intestinal peptide (59), motilin (62), and neuropeptide Y (58) in plasma and quantification of GLP-1-Receptor (60) by real time PCR in the colon. Also the frequency of bowel movements of the jejunum and colon are often checked in animals (61).
The defecation pattern can be monitored using a consistency score of stools (e.g., Bristol Stool Form Scale), by measurement of the stool water content, frequency of defecation and production fecal pellets (63, 64). The gastrointestinal symptom score can be used for the assessment of a broad spectrum of gut related symptoms including included nausea, vomiting, bloating, abdominal cramps, early satiety, acidic eructation/heartburn, loss of appetite, retrosternal discomfort, and epigastric pain/upper abdominal pain (72).
2. Extracellular digestion of ingested material (Table 2):
Extracellular digestion is the process by which food is converted into substances that can be absorbed and assimilated. This is accomplished in the digestive tract by the mechanical and enzymatic breakdown of food constituents into simpler chemical compounds. Imbalances in extracellular digestion can lead to a level of GI discomfort, nutritional imbalances and allergy and infection susceptibility.
Methods to assess extracellular digestion include indirect calorimetry (65), ELISA by measurement of ghrelin (66) in plasma and gas chromatography or mass spectrometry to measure short-chain fatty acids (SCFAs) and branched fatty acids, which are produced by the gut microbes by fermentation (67).
3. Absorption of essential nutrients (Table 3):
Digested molecules of food, water and minerals from the diet are absorbed from the lumen of the upper and middle small intestine. The absorbed materials cross the mucosa into the blood or lymph and are carried off in the bloodstream to other parts of the body for storage (e.g., as energy source) or further chemical change. This process varies with different types of nutrients. Imbalances in absorption can lead to nutritional imbalances, disturbed defecation patterns and increased levels of GI discomfort.
Methods to assess the supply of essential nutrients are for instance blood galactose assay for measurement of in vivo nutrient absorption (68), the measurement of meal-stimulated, endogenous glucagon-like peptide-2 (GLP-2) secretion (64, 69). In plasma/blood, levels of vitamins, minerals, iron and lipids can be determined (71), whereas in breath hydrogen (measure for carbohydrate malabsorption as a product of colonic bacterial fermentation) (71) and carbon dioxide (measure for triglyceride uptake) (71) are often used. In addition metabolic chambers (70) are used to measure the ratio of carbon dioxide and oxygen along with nitrogen. Urine is also frequently collected to assess for instance protein oxidation. Symptom score for dyspeptic and malabsorption symptoms are also often used (72, 73).
4. Protection from injurious or allergenic material (Table 4):
In the intestinal tract, a single layer of epithelial cells forms a physical barrier between the intestinal lumen, the lamina propria and the mucosal-associated lymphoid tissue. In addition, mucus secreted by goblet cells in the epithelium serves to spatially compartmentalize the bacteria to the lumen and prevent bacterial colonization of the epithelium. Increased mucosal permeability and loss of epithelial integrity are recognized to play a role in the pathophysiology of a variety of (gastrointestinal) disorders and possibly allergy, including the challenge by intestinal pathogens. Similarly, the defecation pattern and the level of GI discomfort is affected by an impaired protection.
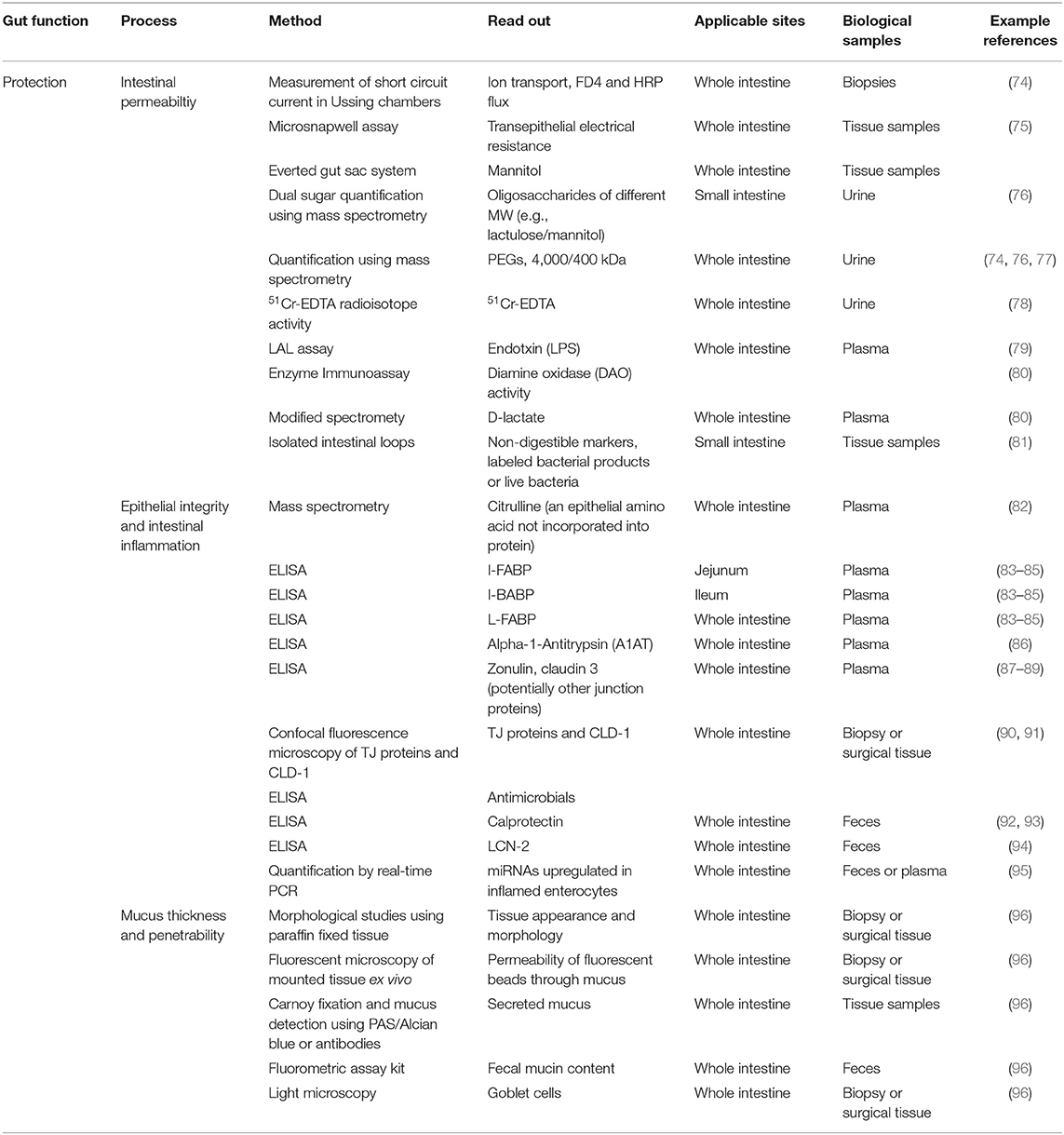
Table 4. Methods described in literature to measure protection from injurious or allergenic material.
Permeability of the small intestine is commonly evaluated by measurement of intestinal permeation and urinary excretion of orally administered water-soluble, non-metabolizable sugars that differ in size [e.g., lactulose, FITC-dextran or polyethylene glycols (PEGs) of 1,500–4,000 Da] and low-molecular-weight sugars such as mannitol and L-rhamnose, or low-molecular-weight PEG (400 Da) (74, 76, 77, 97). The 51Cr-EDTA test, which is performed by calculating the percentage of recovery from urine of an oral dose of 51Cr-EDTA, is also used as a permeability test (78). Another powerful model to assess intestinal permeability are creating isolated intestinal loops (81). Diamine oxidase (DAO) activity is measured in serum as it correlates inversely with intestinal permeability of the small intestine (80). Alpha-1-Antitrypsin (A1AT) in plasma and stool can also be used as a biomarker of intestinal permeability (86).
Potential biomarkers of epithelial integrity are fatty acid-binding proteins (FABPs) (83–85), TJ proteins (87–89), and citrulline (82) which can be measured in plasma and urine using ELISA. Detection of the inflammatory markers calprotectin (92, 93) and lipocalin 2 (94) in feces have also been used as a surrogate marker of epithelial integrity in many disease studies because excessive intestinal inflammation is known to increase epithelial permeability. Recently, non-coding microRNAs (miRNAs) such as miRNA-222, miRNA-30, miRNA-29b, miRNA-503, miRNA195, and miRNA-320a have been demonstrated to play a role in the regulation of epithelial regeneration, protection, and epithelial barrier function (95).
Ussing chamber (74) or microsnap well (75) are used to measure the short-circuit current which is an indicator of ion transport across the epithelium and to confirm the integrity and permeability using biopsies of patients and healthy subjects. Histological examination using biopsies or resected tissue from animals and humans is also a common experimental approach for studying aspects of barrier function by for instance immunofluorescent antibody detection of TJs (90) or adherens junctions (91) or to detect intestinal pathology including ulcerations of the mucosa and severe intestinal inflammation that will contribute to increased intestinal permeability. Carnoy fixation and paraffin embedding of intestinal tissue followed by immunofluorescent antibody staining of mucin Muc2 or periodic acid Schiff/Alcian blue staining can be used to assess mucus thickness in the colon of small rodents (96).
As shown, the symptoms of GI disorders reflect a broad spectrum of disturbance of GI physiology, including altered epithelial, muscle, intestinal, and enteric neural function which are at least in part, associated with immune activation. There are multiple assays available to address changes in gut functions but most emphasis is on transport and transit of ingested material and protection from injurious or allergenic material.
Selection of Candidate Biomarkers Linking the Immune System to Gut Functionalities
Based on the identified gut functions (transport/transit ingested material, extracellular digestion ingested material, intracellular digestion and metabolism, absorption essential nutrients and protection from injurious or allergenic material), the next step was to select candidate molecules that are affected by immunotherapy that might influence gut functions. Therefore, molecular databases were used to connect genes/proteins involved in gut functions using Gene Ontology/Panther database and CTD database (Supplementary Table 1). In Table 5 the results are shown for each different gut health function. In total 741 unique molecules were found using the CTD MeSH terms and 1,239 unique molecules using the Gene Ontology database, of which 100 molecules were overlapping.
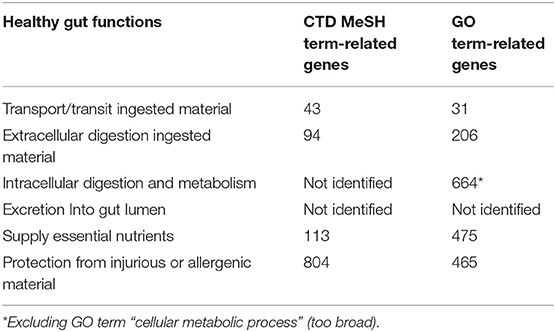
Table 5. Number of identified genes that are related to a gut function using the Comparative Toxicogenomics Database (CTD) and Gene Ontology (GO) database.
Next, the overlap of genes with multiple gut functions was evaluated and visualized in a Venn diagram (Figure 2). The names of the individual genes per gut function are depicted in the Supplementary Tables 2–5. Seven genes were overlapping between all gut functions: GUCY2C, EPCAM, MYO5B, PYY, SLC10A2, TNF, and NEUROG3.
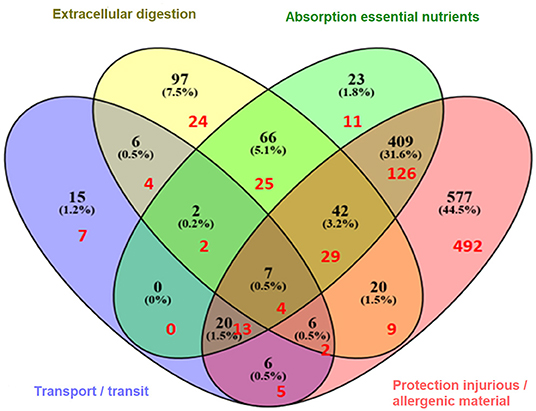
Figure 2. Venn diagram illustrating the (shared) sets of molecules involved in gut function-related processes: extracellular digestion ingested material, supply essential nutrients, transport/transit of ingested material, and protection from injurious or allergenic material (number of genes in black). In red are indicated the number of genes that are predicted to be shared with immune related diseases (hypersensitivity, autoimmunity, resistance to infection, and cancer) as previously described (11).
As referred to earlier, using the same approach as here, immune related genes were identified which are predicted to be involved in the four most common immune health endpoints:: hypersensitivity, infection, autoimmunity, and immune-mediated resistance to cancer (11). The overlap between the gut function related genes and immune disease related genes was determined revealing that in total 753 genes were shared (Figure 3), indicating the strong relationship between the immune system and gut function. These shared genes were evaluated and visualized in Figure 2 (indicated in red). The number of shared genes was as follows: transport/transit ingested material [37 out of 62 transport-related genes shared (60%)], extracellular digestion ingested material [99 out of 246 digestion-related genes shared (40%)], absorption essential nutrients [210 out of 569 absorption-related genes shared (37%)], and protection [680 out of 1100 protection-related genes shared (62%)]. Of the seven genes that were overlapping between all gut functions (GUCY2C, EPCAM, MYO5B, PYY, SLC10A2, TNF, and NEUROG3, as described previously) four genes were also immune disease related genes: GUCY2, EPCAM, MYO5B, and TNF.
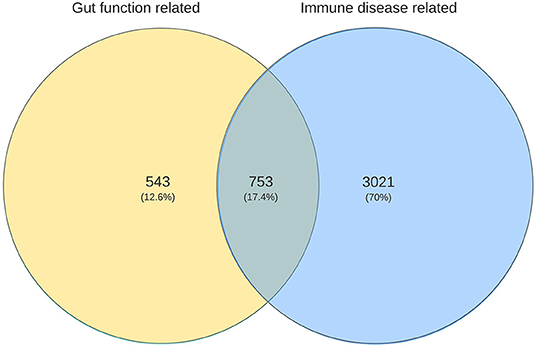
Figure 3. Venn diagram illustrating the numbers of unique and shared genes related to gut functions with immune disease related genes.
Prediction of Effects of Selected Gut-Immune Genes on Gut Functions
To study which biological GO processes and KEGG pathways in the different gut functions are predicted to be influenced by the immune system, a GO enrichment analysis was performed using Panther 14.1 and an KEGG pathway enrichment analysis using DAVID 6.8 were performed using the 753 gut-function related genes which are shared with the immune disease related genes.
Predicted Effects on Transport/Transit of Ingested Material
The 37 genes involved in transport/transit of ingested material which are shared with the immune system (Figure 2, red numbers in purple area) were used for the enrichment analysis. The top 15 biological GO processes and KEGG pathways involved in transport/immune are listed in Table 6. The biological processes in transport and transit are most likely to be affected in the regulation of the small intestinal transit, regulation of the hindgut contraction, regulation of the small intestine smooth muscle contraction, regulation of appetite and eating behavior, regulation of response to food, and regulation of gastric motility/gastric emptying.
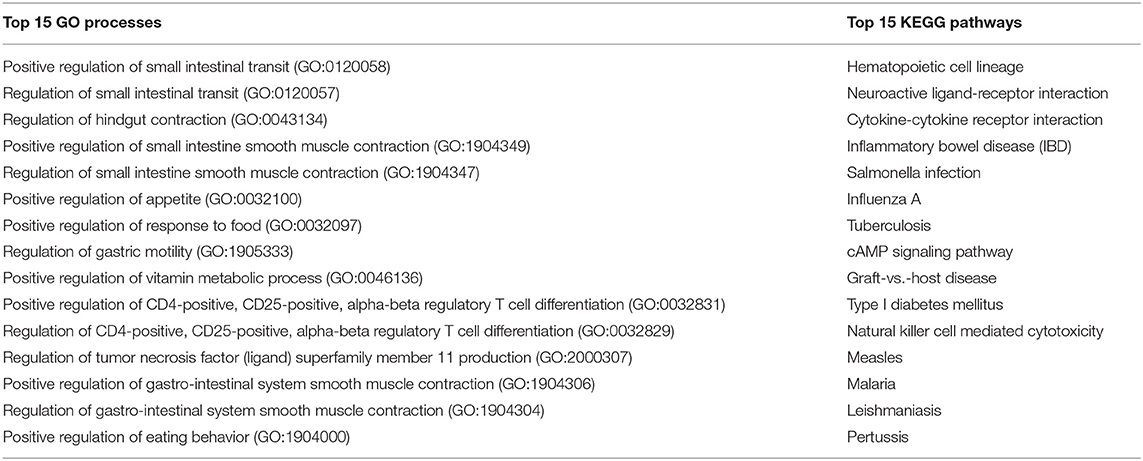
Table 6. Top 15 GO processes and KEGG pathways related to the selected 37 gut-immune genes involved in transport and transit of ingested material.
Of the top 15 KEGG pathways the Neuroactive ligand-receptor interaction pathway and the cAMP signaling pathway are the most biologically relevant. Genes of interest in above pathways are: DRD2, GHRL, GHSR, OPRM1, PTAFR, PTGER3, SSTR2, and TRPV1. As indicated by the presence of the genes in these pathways and processes, the immune system can affect the motility function of the gut, which is supportive for the applied approach.
Predicted Effects on Extracellular Digestion of Ingested Material
The 99 genes involved in extracellular digestion of ingested material which are shared with the immune system (Figure 2, red numbers in yellow area) were used for the enrichment analysis. The top 15 GO biological processes and KEGG pathways involved in extracellular digestion/immune are listed in Table 7. The biological processes in extracellular digestion are most likely to be affected by the immune system in the cysteine biosynthetic process from serine, regulation of pancreatic juice secretion, regulation of small intestinal transit, regulation of hindgut contraction, regulation of appetite, regulation of response to food, regulation of digestive system process, cysteine biosynthetic process via cystathionine, and intestinal D-glucose absorption.
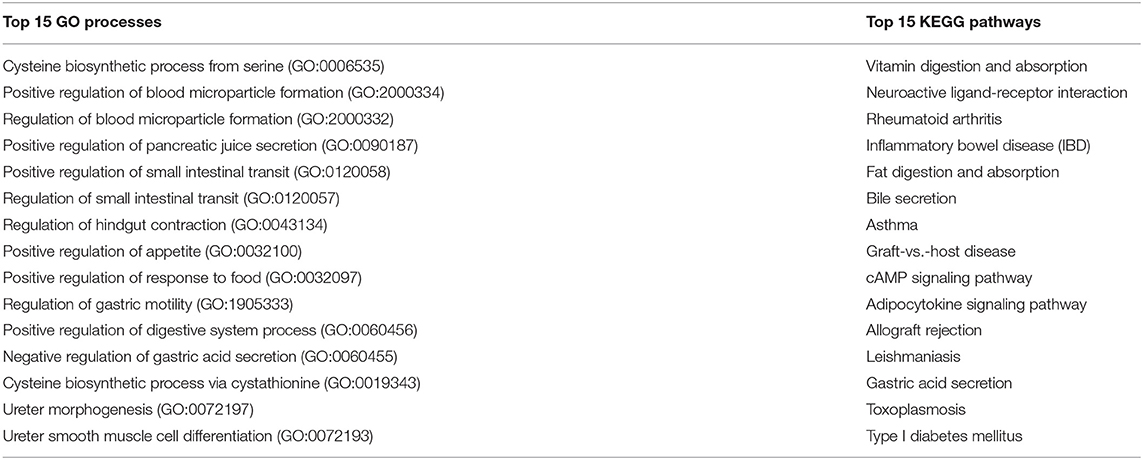
Table 7. Top 15 GO processes and KEGG pathways related to the selected 99 gut-immune genes involved in extracellular digestion.
Of the top 15 KEGG pathways the vitamin digestion and absorption, fat digestion and absorption, bile secretion, adipocytokine signaling pathway, and gastric acid secretion are the most biologically relevant. Genes of interest in above pathways are: ABCB4, ABCG5, CD36, APOA1, APOA4, AQP1, CUBN, EZR, HRH2, KCNQ1, LDLR, LEP, PPARGC1A, NPY, SCARB1, SSTR2, SST, SULT2A1, TCN2, and TNF. As indicated by the presence of the genes in these pathways and processes, the immune system can affect extracellular digestion, specifically on vitamin and fat digestion and absorption as well as on the regulation of the digestive system and gastric acid in general, again providing support to the applied approach.
Predicted Effects on Absorption of Essential Nutrients
The 210 genes involved in the absorption of essential nutrients which are shared with the immune system (Figure 2, red numbers in green area) were used for the enrichment analysis. The top 17 GO biological processes and KEGG pathways involved in absorption essential nutrients/immune are listed in Table 8. The biological processes related to absorption of essential nutrients most likely to be affected by the immune system are in the regulation of cell proliferation involved in outflow tract morphogenesis, protein localization to bicellular tight junction, Kit signaling pathway, cysteine biosynthetic process from serine, cellular response to stem cell factor stimulus, response to stem cell factor, and intestinal D-glucose absorption.
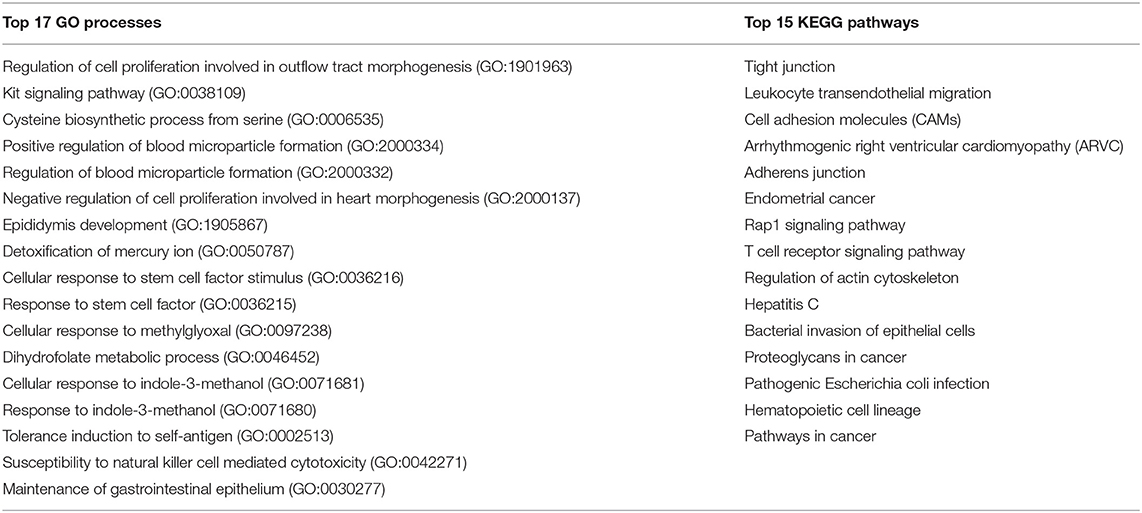
Table 8. Top 17 GO processes and KEGG pathways related to the selected 210 gut-immune genes involved in absorption of essential nutrients.
Of the top 15 KEGG pathways TJ, leukocyte transendothelial migration, cell adhesion molecules (CAMs), adherens junction and Rap1 signaling pathway are the most biologically relevant. Genes of interest in above pathways are: ACTN4, AKT1, ASH1L, PATJ, ACTN4, CADM1, CASK, CD2, CD226, CDC42, CDH1, CDH2, CDH3, CDH5, CTNNA1, CTNNA2, CTNNA3, CTNNB1, CNTNAP2, CTNNB1, CDC42, CLDN1, CLDN11, CLDN3, CLDN4, CLDN7, CLDN9, CDC42, CDH1, CDK4, EPB41L3, EZR, FGF13, FGFR4, IGSF5, ITGA6, ITGB1, INS, IQGAP1, KIT, KRIT1, MAGI1, MAGI2, MAP2K2, NECTIN1, OCLN, PATJ, PIK3R1, PLCG1, PRKCD, PRKCZ, PTPN6, PTPRJ, PVR, RHOA, SKAP1, SYMPK, TEK, TGFBR1, TJP3, TLN1, and VAV1. As indicated by the presence of the genes in these pathways and processes, the immune system can impact the barrier function of the gut as well as the absorption of nutrients.
Predicted Effects on Protection From Injurious or Allergenic Materials
The 680 genes involved in protection from injurious or allergenic materials which are shared with the immune system (Figure 2, red numbers in red area) were used for the enrichment analysis. The top 20 biological processes and KEGG pathways involved in protection from injurious or allergenic material/immune are listed in Table 9. The biological processes in protection most likely to be affected by the immune system are in regulation of nitrogen utilization, cellular response to triacyl bacterial lipopeptide, response to triacyl bacterial lipopeptide, toll-like receptor TLR1:TLR2 signaling pathway, fever generation, regulation of TRAIL-activated apoptotic signaling pathway, complement-mediated synapse pruning, nitric oxide transport, regulation of chronic inflammatory response to antigenic stimulus, regulation of type III interferon production, positive regulation of calcidiol 1-monooxygenase activity, positive regulation of vitamin D biosynthetic process, tolerance induction to self-antigen, negative regulation of interleukin-8 biosynthetic process, negative regulation of T-helper 2 cell cytokine production, interleukin-10 production, and activation of cysteine-type endopeptidase activity involved in apoptotic signaling pathway.
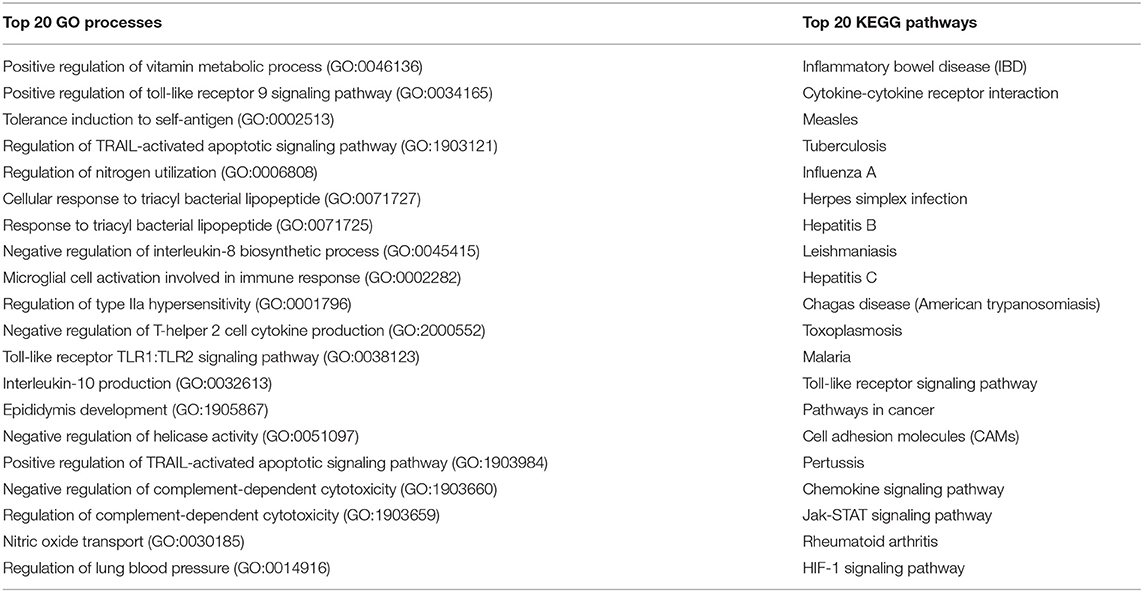
Table 9. Top 20 GO processes and KEGG pathways related to the selected 680 gut-immune genes involved in protection from injurious or allergenic material.
Of the top 20 KEGG pathways IBD, cytokine-cytokine receptor interaction, TLR signaling pathway, pathways in cancer, CAMs, chemokine signaling pathway, Jak-STAT signaling pathway, and viral/bacterial infection related pathways [Measles, Tuberculosis, Influenza A, Herpes simplex infection, Hepatitis B, Leishmaniasis, Hepatitis C, Chagas disease (American trypanosomiasis), Toxoplasmosis, Malaria, Pertussis and Epstein-Barr virus infection] are the most biologically relevant. These pathways include many interleukins, chemokines and surface cluster molecules such as: RELA, SMAD3, TBX21, FOXP3, IFN-GR1, IFN-G, IL-1A, IL-1B, IL-10, IL-12B, IL-13, IL-17A, IL-18, IL-2, IL-21R, IL-23R, IL-4R, IL-4, IL-5, IL-6, HLA-DPA1, HLA-DPB1, HLA-DQA1, HLA-DQB1, HLA-DRA, HLA-DRB1, STAT1, STAT3, STAT6, TLR2, TLR4, TLR5, and TGF-B1. As indicated by the presence of the genes in these pathways and processes, the immune system greatly impacts the protection function of the gut.
In conclusion, with this approach 1,296 molecules were identified to be involved in gut function, of which 753 molecules are likely to be affected by nutritional immune interventions.
Proof of Principle/in silico Test Case
The top interacting genes identified by the CTD for vitamin D were used for comparison with our set of 1,296 gut-function related genes, to check whether the previously described adverse/beneficial effects of vitamin D on gut function could be predicted accurately.
Vitamin D
Vitamin D is known to play an important role in bone metabolism through regulation of calcium and phosphate homeostasis and plays an important role in immune system regulation via, for instance, the suppression of mast cell activation and IgE synthesis from B cells (1), and increase the number of tolerogenic dendritic cells and IL-10-producing regulatory T cells (2). Vitamin D is produced by the body during exposure to sunlight, but is also found in oily fish, eggs and fortified food products and is used for nutritional interventions to check their immunomodulatory properties in food allergy (3), autoimmunity (4), respiratory tract infection (5–8), and cancer (9). In addition to causing rickets, vitamin D deficiency has been linked to respiratory infections such as pneumonia, tuberculosis and bronchiolitis (5–8). Vitamin D was checked in the CTD for its top interacting genes. Subsequently, those genes were checked to see whether they were involved in the four gut functions (Figure 4) and therefore potentially influence the gut functions. Interestingly, in all four of the gut functions the top interacting genes were present.
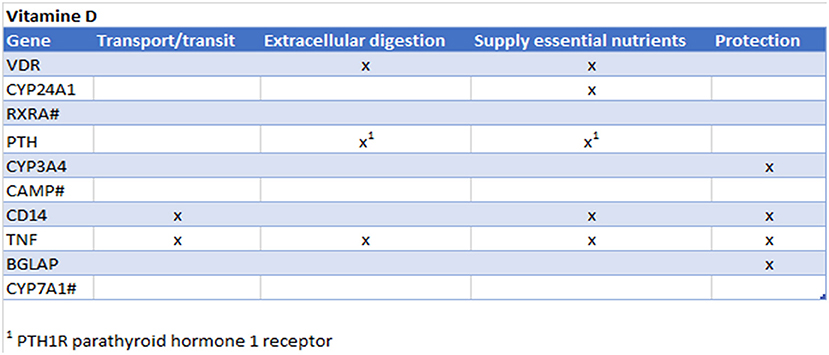
Figure 4. Prediction from CTD vitamin D. Gene names are listed of the top 10 interacting genes per chemical. Crosses (x) indicate in which of the four gut functions they are involved. #Gene not found in all gut functions.
These findings are confirmed in literature: Vitamin D plays an important role in calcium absorption, which is important for bone metabolism, but also to provide calcium to the nerve cells. In addition vitamin D has shown to protect intestinal microbiota (10–12). Adverse effects of severe hypercalcemia and hyperphoshatemia induces by exogenous vitamin D is also affecting the gastrointestinal system: including abdominal pain, anorexia, constipation, nausea, peptic ulcer, and vomiting (13, 14). Vitamin D deficiency exacerbates allergic diarrhea (15). Vitamin D is known to induce antimicrobial peptides (such as cathelicidins and defensins (16, 17) and has been shown in a clinical study that there is a direct correlation between vitamin D administration and serum cathelicidin levels in healthy subjects (18). Vitamin D is also known to enhance the gut epithelial barrier (19).
This example illustrates that the prediction with our systems biology approach is promising and can be used to find both negatively as positively correlated interactions. It must be noted that this approach is greatly influenced by the amount of data available on a specific compound; there will be more evidence on the top interacting genes when there are more data available. Therefore, it is of importance that the predicted biomarkers are properly validated in an appropriate assay/study.
Discussion
The aim of this study was to identify crucial biomarkers for the assessment of safety and efficacy of oral immune interventions on gut function by using systems biology approaches. An enormous advantage of using these systems approaches is that all information can be collected and selected in an automated fashion, thereby enabling the possibility to screen and select large databases with genes and their biological roles/pathways. The next step would be to validate their biological role.
By making use of these comprehensive databases such as PANTHER (www.geneontology.org/) and CTD (http://ctdbase.org/) we were able to determine the overlap between gut function related genes and immune disease related genes. In total 753 genes were shared, indicating the strong relationship between the immune system and gut functions. As expected, the gut function “protection from injurious or allergenic material” (from here on briefly referred to as “protection”) shares the highest number of genes with the immune disease related genes [680 shared (63%)]. However, as with the other gut functions there is quite some overlap: transport/transit ingested material [37 shared (69%)], extracellular digestion ingested material [99 shared (40%)], and absorption of essential nutrients [210 shared (37%)].
There is a multitude of assays available to study different functions of the gut physiology. However, it appears that most assays described in literature are related to the protection, transit and/or transport. In this study, several of the identified shared genes are already measured in currently existing assays, which are mostly related to protection, transit and/or transport. It would be very interesting to include the newly identified biomarkers in the assays related to the other gut functions as they are predicted to play a role both in immune as well as a gut function, thereby indicating the putative importance of such molecule during nutritional immune intervention.
By performing a GO enrichment analysis and an enrichment analysis using DAVID bioinformatics on the gene set shared between gut function transport and transit and immune disease related genes the neuroactive ligand-receptor interaction pathway and the cAMP signaling pathway were identified. Neurotransmitters (opoid) and cAMP are known to affect smooth muscle contractions and motility and the cAMP pathway generally reduces excitability and contraction by activating K+ channels and by reducing the Ca2+ sensitivity of the contractile apparatus (98). Therefore, neuron expression, tyrosine kinase receptor (c-kit) and serotonin are often studied to measure motility, however it could be useful to include molecules related to G protein-coupled receptors (not found with system biology approach directly).
Current methods to assess extracellular digestion include indirect calorimetry (65), ELISA by measurement of ghrelin (66) in plasma and gas chromatography or mass spectrometry to measure SCFAs and branched fatty acids (67). It could however also be interesting to measure apolipoprotein (APO) A1 and A4 in relation to lipid transport and CD36 in fat absorption.
Methods to assess the supply of essential nutrients mostly include absorption assays and metabolic chambers (70). Parathyroid hormones might also be interesting to measure as it is the most important endocrine regulator of calcium and phosphorus concentration in extracellular fluid (99). Interestingly, a recent meta-analysis of available clinical trials indicates that 1,000 IU vitamin D supplementation can suppress serum parathyroid hormone levels, while 4,000 IU of vitamin D3 was associated with the largest increase in serum 25-hydroxyvitamin D levels in an overweight and obese population (100).
Histological examination is a common experimental way of studying aspects of barrier function, for instance immunofluorescent antibody detection of TJs (90) or adherens junctions (91), or to detect intestinal pathology including ulcerations of the mucosa and severe intestinal inflammation that will cause and contribute to increased intestinal permeability. It would be a good opportunity to combine relevant molecules with histology, so the biological validation can also be performed. For instance this assay could include molecules of one of the central signaling pathway JAK-STAT, which regulates the adaptive and innate immune arms of mucosal immunity as well as epithelial repair and regeneration (101). Epithelial repair and regeneration are important in epithelial integrity as well as gut protection function. Another opportunity for study would be to include molecules that are involved in the activation of STAT3, which stimulates antimicrobial production (102). Measurement of the molecules related to this pathway will also help to evaluate gut function. However, it must be noted that some of the mentioned assessments may only be feasible in preclinical but not in clinical settings due to their invasive nature.
CD36 was identified as an interesting molecule as it is predicted to be involved in three different gut functions: extracellular digestion, absorption of essential nutrients and protection. For example, CD36-dependent signaling mediates fatty acid-induced gut release of secretin and cholecystokinin (103), and could therefore be a good marker of extracellular digestion. Furthermore, there is a direct mechanistic link between CD36 engagement and IL-10 induction, opening up new possibilities for using CD36 ligands, agents that increase CD36 expression or a combination of both to modulate inflammation and treat, or even prevent, an important set of chronic disorders (104).
The systems biology approach described here is a promising tool, but it does have its limitations. These limitations are based on the fact that it is essential that the data used are accurate, complete and up to date. The current approach used curated data from CTD and Panther databases. As not all information available from literature has been added to these databases, it is possible that not all relationships between the immune system and gut functioning have been captured by our systems biology approach. Moreover, data are continuously generated so the approach presented here needs continuous on-going scheduled refinements and improvements. Furthermore, it might be subjected to a reporting bias as it can be difficult to distinguish the absence of a relationship between molecules/pathways from a lack of evaluation of the relationship/pathways. In addition, molecules indicated in the databases are sometimes not annotated/linked to a specific cell type/organ whereas it is known that molecules in the context of specific cell types influence certain gut functions. For instance eosinophil related proteins (28) and markers for specific T cell subsets (29) are not included in our system immunology approach, and are apparently not included in the databases. This needs some further investigation. Another limitation of the approach concerns the simplification of the complexity of the immune system and the gut functionalities. In this approach, the connectivity between the processes, genes and diseases are elucidated but the nature of these relationships (co-expression, feedback, crosstalk, activation, etc.) at each layer and between layers are disregarded. In this approach, the key markers are identified but their roles in the complexity of the biological processes are not studied.
As an appropriately functioning immune system is a dynamic system which is in balance, an upregulation/activation of certain pathways/molecules does not automatically result in a malfunction of the immune system or influence gut functions, as a healthy dynamic immune system will eventually return to a balanced state (immune resilience). Therefore, it is of utmost importance that the selected biomarkers that are anticipated to play a role in the studied gut health functions after oral immune intervention, are validated in an appropriate assay/study to understand the biological relevance of induced changes.
A next step is to prioritize and validate useful biomarkers for in nutritional intervention studies. For instance, by making use of the identified crossroads to identify which of the proteins could best be used as part of a panel of biomarkers that distinguishes whether a nutritional intervention can affect specific gut functions. This can be performed by selecting a set of common and unique markers per gut functionality. In addition, the importance of the molecule and how essential it is in specific gut functions should be checked by performing pathway analysis. Lastly, the molecules should be checked for their feasibility to be measured on both gene expression level and at protein level. This will allow the measurement of gut function upon nutritional immune intervention.
In conclusion, we describe a systems biology approach that helps to clarify on one hand the relationships between immune system and gut functions, and on the other hand the identification of candidate biomarkers to monitor effects on gut functions upon nutritional immune interventions.
Data Availability Statement
All datasets generated for this study are included in the article/Supplementary Material.
Author Contributions
MM, TB, RD, LV, and JB contributed conception and design of the study. TB, RD, and LV organized the database. MM and JB performed databases searches. MM, LV, and JB wrote the manuscript. All authors contributed to manuscript revision, read, and approved the submitted version.
Funding
This research was financially supported by the Dutch Governmental TNO Research Cooperation Funds and Danone Nutricia Research and Food Safety center.
Conflict of Interest
JG is employed by Danone Food Safety Center. LK, LH, and KK are employed by Danone Nutricia Research.
The remaining authors declare that the research was conducted in the absence of any commercial or financial relationships that could be construed as a potential conflict of interest.
Supplementary Material
The Supplementary Material for this article can be found online at: https://www.frontiersin.org/articles/10.3389/fimmu.2019.02672/full#supplementary-material
References
1. Möbs C, Ipsen H, Mayer L, Slotosch C, Petersen A, Würtzen PA, et al. Birch pollen immunotherapy results in long-term loss of Bet v 1-specific TH2 responses, transient TR1 activation, and synthesis of IgE-blocking antibodies. J Allergy Clin Immunol. (2012) 130:1108–16.e6. doi: 10.1016/j.jaci.2012.07.056
2. Jones SM, Pons L, Roberts JL, Scurlock AM, Perry TT, Kulis M, et al. Clinical efficacy and immune regulation with peanut oral immunotherapy. J Allergy Clin Immunol. (2009) 124:292–300.e97. doi: 10.1016/j.jaci.2009.05.022
3. Skripak JM, Nash SD, Rowley H, Brereton NH, Oh S, Hamilton RG, et al. A randomized, double-blind, placebo-controlled study of milk oral immunotherapy for cow's milk allergy. J Allergy Clin Immunol. (2008) 122:1154–60. doi: 10.1016/j.jaci.2008.09.030
4. Vazquez-Ortiz M, Turner PJ. Improving the safety of oral immunotherapy for food allergy. Pediatr Allergy Immunol. (2015) 27:117–25. doi: 10.1111/pai.12510
5. Caspi RR. Immunotherapy of autoimmunity and cancer: The penalty for success. Nat Rev Immunol. (2008) 8:970–6. doi: 10.1038/nri2438
6. Chan KKW, Glenny AM, Weldon JC, Furness S, Worthington HV, Wakeford H. Interventions for the treatment of oral and oropharyngeal cancers: targeted therapy and immunotherapy. Cochrane database Syst Rev. (2015) 12:CD010341. doi: 10.1002/14651858.CD010341.pub2
7. Tam H, Calderon MA, Manikam L, Nankervis H, García Núñez I, Williams HC, et al. Specific allergen immunotherapy for the treatment of atopic eczema. Cochrane Database Syst Rev. (2016) 2:CD008774. doi: 10.1002/14651858.CD008774.pub2
8. Yeung JP, Kloda LA, McDevitt J, Ben-Shoshan M, Alizadehfar R. Oral immunotherapy for milk allergy. Cochrane Database Syst Rev Online. (2012) 11:CD009542. doi: 10.1002/14651858.CD009542.pub2
9. Romantsik O, Tosca MA, Zappettini S, Calevo MG. Oral and sublingual immunotherapy for egg allergy. Cochrane Database Syst Rev. (2014) 4:CD010638. doi: 10.1002/14651858.CD010638.pub2
10. Kostadinova AI, Willemsen LEM, Knippels LMJ, Garssen J. Immunotherapy - risk/benefit in food allergy. Pediatr Allergy Immunol. (2013) 24:633–44. doi: 10.1111/pai.12122
11. Meijerink M, van den Broek T, Dulos R, Neergaard Jacobsen L, Staudt Kvistgaard A, Garthoff J, et al. The impact of immune interventions: a systems biology strategy for predicting adverse and beneficial immune effects. Front Immunol. (2019) 10:231. doi: 10.3389/fimmu.2019.00231
12. Spain L, Diem S, Larkin J. Management of toxicities of immune checkpoint inhibitors. Cancer Treat Rev. (2016) 44:51–60. doi: 10.1016/j.ctrv.2016.02.001
13. Michot JM, Bigenwald C, Champiat S, Collins M, Carbonnel F, Postel-Vinay S, et al. Immune-related adverse events with immune checkpoint blockade: a comprehensive review. Eur J Cancer. (2016) 54:139–48. doi: 10.1016/j.ejca.2015.11.016
14. Johnston RL, Lutzky J, Chodhry A, Barkin JS. Cytotoxic T-lymphocyte-associated antigen 4 antibody-induced colitis and its management with infliximab. Dig Dis Sci. (2009) 54:2538–40. doi: 10.1007/s10620-008-0641-z
15. Bittar A, Sengupta U, Kayed R. Prospects for strain-specific immunotherapy in Alzheimer's disease and tauopathies. npj Vaccines. (2018) 3. doi: 10.1038/s41541-018-0046-8
16. James C, Bernstein DI. Allergen immunotherapy: an updated review of safety. Curr Opin Allergy Clin Immunol. (2017) 17:55–9. doi: 10.1097/ACI.0000000000000335
17. Yu LC, Perdue MH. Role of mast cells in intestinal mucosal function: studies in models of hypersensitivity and stress. Immunol Rev. (2001) 179:61–73. doi: 10.1034/j.1600-065x.2001.790107.x
18. Krystel-Whittemore M, Dileepan KN, Wood JG. Mast cell: a multi-functional master cell. Front Immunol. (2016) 6:620. doi: 10.3389/fimmu.2015.00620
19. Zhang L, Song J, Hou X. Mast cells and irritable bowel syndrome: from the bench to the bedside. J Neurogastroenterol Motil. (2016) 22:181–92. doi: 10.5056/jnm15137
20. Gasbarrini A, Lauritano EC, Garcovich M, Sparano L, Gasbarrini G. New insights into the pathophysiology of IBS: Intestinal microflora, gas production and gut motility. Eur Rev Med Pharmacol Sci. (2008) 12:111–7. doi: 10.1089/152460900318515
21. Santos J, Guilarte M, Alonso C, Malagelada JR. Pathogenesis of irritable bowel syndrome: the mast cell connection. Scand J Gastroenterol. (2005) 40:129–40. doi: 10.1080/00365520410009410
22. Murch S. Allergy and intestinal dysmotility - evidence of genuine causal linkage? Curr Opin Gastroenterol. (2006) 22:664–8. doi: 10.1097/01.mog.0000245546.18279.7e
23. Héron A, Dubayle D. A focus on mast cells and pain. J Neuroimmunol. (2013) 264:1–7. doi: 10.1016/j.jneuroim.2013.09.018
24. Lee H, Park JH, Park D Il, Kim HJ, Cho YK, Sohn C Il, et al. Mucosal mast cell count is associated with intestinal permeability in patients with diarrhea predominant irritable bowel syndrome. J Neurogastroenterol Motil. (2013) 19:244–50. doi: 10.5056/jnm.2013.19.2.244
25. Jacob C, Yang PC, Darmoul D, Amadesi S, Saito T, Cottrell GS, et al. Mast cell tryptase controls paracellular permeability of the intestine: Role of protease-activated receptor 2 and β-arrestins. J Biol Chem. (2005) 280:31936–48. doi: 10.1074/jbc.M506338200
26. Groschwitz KR, Hogan SP. Intestinal barrier function: molecular regulation and disease pathogenesis. J Allergy Clin Immunol. (2009) 124:3–20; quiz: 21–2. doi: 10.1016/j.jaci.2009.05.038
27. Meddings JB, Jarand J, Urbanski SJ, Hardin J, Gall DG. Increased gastrointestinal permeability is an early lesion in the spontaneously diabetic BB rat. Am J Physiol. (1999) 276:G951–7.
28. Furuta GT, Nieuwenhuis EES, Karhausen J, Gleich G, Blumberg RS, Lee JJ, et al. Eosinophils alter colonic epithelial barrier function: role for major basic protein. Am J Physiol Gastrointest Liver Physiol. (2005) 289:G890–7. doi: 10.1152/ajpgi.00015.2005
29. Dalton JE, Cruickshank SM, Egan CE, Mears R, Newton DJ, Andrew EM, et al. Intraepithelial gammadelta+ lymphocytes maintain the integrity of intestinal epithelial tight junctions in response to infection. Gastroenterology. (2006) 131:818–29. doi: 10.1053/j.gastro.2006.06.003
30. Zolotarevsky Y, Hecht G, Koutsouris A, Gonzalez DE, Quan C, Tom J, et al. A membrane-permeant peptide that inhibits MLC kinase restores barrier function in in vitro models of intestinal disease. Gastroenterology. (2002) 123:163–72. doi: 10.1053/gast.2002.34235
31. Mankertz J, Tavalali S, Schmitz H, Mankertz A, Riecken EO, Fromm M, et al. Expression from the human occludin promoter is affected by tumor necrosis factor alpha and interferon gamma. J Cell Sci. (2000) 113 (Pt 1):2085–90. doi: 10.1083/jcb.133.1.43
32. Madsen KL, Malfair D, Gray D, Doyle JS, Jewell LD, Fedorak RN. Interleukin-10 gene-deficient mice develop a primary intestinal permeability defect in response to enteric microflora. Inflamm Bowel Dis. (1999) 5:262–70. doi: 10.1097/00054725-199911000-00004
33. Akiho H. Low-grade inflammation plays a pivotal role in gastrointestinal dysfunction in irritable bowel syndrome. World J Gastrointest Pathophysiol. (2010) 1:97–105. doi: 10.4291/wjgp.v1.i3.97
34. Akiho H, Blennerhassett P, Deng Y, Collins SM. Role of IL-4, IL-13, and STAT6 in inflammation-induced hypercontractility of murine smooth muscle cells. Am J Physiol Gastrointest Liver Physiol. (2002) 282:G226–32. doi: 10.1152/ajpgi.2002.282.2.G226
35. Akiho H, Ihara E, Motomura Y, Nakamura K. Cytokine-induced alterations of gastrointestinal motility in gastrointestinal disorders. World J Gastrointest Pathophysiol. (2011) 2:72–81. doi: 10.4291/wjgp.v2.i5.72
36. Ohama T, Hori M, Fujisawa M, Kiyosue M, Hashimoto M, Ikenoue Y, et al. Downregulation of CPI-17 contributes to dysfunctional motility in chronic intestinal inflammation model mice and ulcerative colitis patients. J Gastroenterol. (2008) 43:858–65. doi: 10.1007/s00535-008-2241-2
37. Ohama T, Hori M, Sato K, Ozaki H, Karaki H. Chronic treatment with interleukin-1β attenuates contractions by decreasing the activities of CPI-17 and MYPT-1 in intestinal smooth muscle. J Biol Chem. (2003) 278:48794–48804. doi: 10.1074/jbc.M310166200
38. Ohama T, Hori M, Momotani E, Iwakura Y, Guo F, Kishi H, et al. Intestinal inflammation downregulates smooth muscle CPI-17 through induction of TNF-alpha and causes motility disorders. Am J Physiol Gastrointest Liver Physiol. (2007) 292:G1429–38. doi: 10.1152/ajpgi.00315.2006
39. Hu W, Mahavadi S, Li F, Murthy KS. Upregulation of RGS4 and downregulation of CPI-17 mediate inhibition of colonic muscle contraction by interleukin-1beta. AmJPhysiol Cell Physiol. (2007) 293:C1991–2000. doi: 10.1152/ajpcell.00300.2007
40. EFSA Panel on dietic products. Nutrition and allergies (NDA). Guidance on the scientific requirements for health claims related to gut and immune fuction. EFSA J. (2011) 9:1–12. doi: 10.2903/j.efsa.2011.1984.Available
41. EFSA NDA Panel. Guidance on the scientific requirements for health claims related to the gastro-intestinal tract, the immune system, and defence against pathogenic micoorganisms. EFSA J. (2016) 14:4369. doi: 10.2903/j.efsa.20YY.NNNN
42. Albers R, Antoine JM, Bourdet-Sicard R, Calder PC, Gleeson M, Lesourd B, et al. Markers to measure immunomodulation in human nutrition intervention studies. Br J Nutr. (2005) 94:452. doi: 10.1079/BJN20051469
43. Davis AP, Grondin CJ, Johnson RJ, Sciaky D, McMorran R, Wiegers J, et al. The comparative toxicogenomics database: update 2019. Nucleic Acids Res. (2018).
44. Oliveros JC. Venny. An interactive tool for comparing lists with Venn's diagrams (2007). Available online at: https://bioinfogp.cnb.csic.es/tools/venny/
45. The Gene Ontology Consortium. The Gene Ontology Resource: 20 years and still GOing strong. Nucleic Acids Res. (2019) 47:D330–8. doi: 10.1093/nar/gky1055
46. Huang HH, Ting CH, Syu YF, Chang SC, Chen CY. Correlation between colonic secretion and colonic motility in rats: role of Ghrelin. World J Gastroenterol. (2016) 22:10140–7. doi: 10.3748/wjg.v22.i46.10140
47. Dey N, Wagner VE, Blanton LV, Cheng J, Fontana L, Haque R, et al. Regulators of gut motility revealed by a gnotobiotic model of diet-microbiome interactions related to travel. Cell. (2015) 163:95–107. doi: 10.1016/j.cell.2015.08.059
48. Vilz TO, Overhaus M, Stoffels B, Websky MV, Kalff JC, Wehner S. Functional assessment of intestinal motility and gut wall inflammation in rodents: analyses in a standardized model of intestinal manipulation. J Vis Exp. (2012) 4086. doi: 10.3791/4086
49. Robinson AM, Sakkal S, Park A, Jovanovska V, Payne N, Carbone SE, et al. Mesenchymal stem cells and conditioned medium avert enteric neuropathy and colon dysfunction in guinea pig TNBS-induced colitis. Am J Physiol Gastrointest Liver Physiol. (2014) 307:G1115–29. doi: 10.1152/ajpgi.00174.2014
50. Poole DP, Godfrey C, Cattaruzza F, Cottrell GS, Kirkland JG, Pelayo JC, et al. Expression and function of the bile acid receptor GpBAR1 (TGR5) in the murine enteric nervous system. Neurogastroenterol Motil. (2010) 22:1–21. doi: 10.1111/j.1365-2982.2010.01487.x
51. Reed DE, Pigrau M, Lu J, Moayyedi P, Collins SM, Bercik P. Bead study: a novel method to measure gastrointestinal transit in mice. Neurogastroenterol Motil. (2014) 26:1663–68. doi: 10.1111/nmo.12442
52. Yan GW, Deng JF, Bhetuwal A, Yang GQ, Fu QS, Chen H, et al. A case report and literature review of barium sulphate aspiration during upper gastrointestinal examination. Medicine. (2017) 96:e8821. doi: 10.1097/MD.0000000000008821
53. Schmidt J, Stoffels B, Moore BA, Chanthaphavong RS, Mazie AR, Buchholz BM, et al. Proinflammatory role of leukocyte-derived Egr-1 in the development of murine postoperative ileus. Gastroenterology. (2008) 135:926–36, 936.e1–2. doi: 10.1053/j.gastro.2008.05.079
54. Bettolli M, De Carli C, Cornejo-Palma D, Jolin-Dahel K, Wang XY, Huizinga J, et al. Interstitial cell of Cajal loss correlates with the degree of inflammation in the human appendix and reverses after inflammation. J Pediatr Surg. (2012) 47:1891–9. doi: 10.1016/j.jpedsurg.2012.05.006
55. El-Salhy M, Gundersen D, Ostgaard H, Lomholt-Beck B, Hatlebakk JG, Hausken T. Low densities of serotonin and peptide YY cells in the colon of patients with irritable bowel syndrome. Dig Dis Sci. (2012) 57:873–8. doi: 10.1007/s10620-011-1948-8
56. Gibbons SJ, De Giorgio R, Faussone Pellegrini MS, Miller SM, Schmalz PF, Young-fadok TM, et al. Apoptotic cell death of human interstitial cells of Cajal. Neurogastroenterol Motil. (2009) 21:85–93. doi: 10.1111/j.1365-2982.2008.01185.x.Apoptotic
57. Liu HN, Ohya S, Nishizawa Y, Sawamura K, Iino S, Syed MM, et al. Serotonin augments gut pacemaker activity via 5-HT3 receptors. PLoS ONE. (2011) 6:e24928. doi: 10.1371/journal.pone.0024928
58. Gagnon J, Mayne J, Chen A, Raymond A, Woulfe J, Mbikay M, et al. PCSK2-null mice exhibit delayed intestinal motility, reduced refeeding response and altered plasma levels of several regulatory peptides. Life Sci. (2011) 88:212–7. doi: 10.1016/j.lfs.2010.11.010
59. Jacob P, Mueller MH, Hahn J, Wolk I, Mayer P, Nagele U, et al. Alterations of neuropeptides in the human gut during peritonitis. Langenbeck's Arch Surg. (2007) 392:267–71. doi: 10.1007/s00423-007-0168-3
60. Yang M, Fukui H, Eda H, Xu X, Kitayama Y, Hara K, et al. Involvement of gut microbiota in association between GLP-1/GLP-1 receptor expression and gastrointestinal motility. Am J Physiol Gastrointest Liver Physiol. (2017) 312:G367–73. doi: 10.1152/ajpgi.00232.2016
61. Farro G, Gomez-Pinilla PJ, Di Giovangiulio M, Stakenborg N, Auteri M, Thijs T, et al. Smooth muscle and neural dysfunction contribute to different phases of murine postoperative ileus. Neurogastroenterol Motil. (2016) 28:934–47. doi: 10.1111/nmo.12796
62. Wang Z, Xu L, Lu J. Effect of motilin on the contractility of gastric smooth muscle via NO pathway in guinea pig. Curr Zool. (2009) 55:67–74. doi: 10.1093/czoolo/55.1.67
63. Panigrahi MK, Kar SK, Singh SP, Ghoshal UC. Defecation frequency and stool form in a coastal eastern indian population. J Neurogastroenterol Motil. (2013) 19:374–80. doi: 10.5056/jnm.2013.19.3.374
64. Lund P, Sangild PT, Aunsholt L, Hartmann B, Holst JJ, Mortensen J, et al. Randomised controlled trial of colostrum to improve intestinal function in patients with short bowel syndrome. Eur J Clin Nutr. (2012) 66:1059–65. doi: 10.1038/ejcn.2012.93
65. Singer P, Singer J. Clinical guide for the use of metabolic carts: Indirect calorimetry - no longer the orphan of energy estimation. Nutr Clin Pract. (2016) 31:30–8. doi: 10.1177/0884533615622536
66. Peeters TL. Ghrelin: a new player in the control of gastrointestinal functions. Gut. (2005) 54:1638–49. doi: 10.1136/gut.2004.062604
67. Zheng X, Qiu Y, Zhong W, Baxter S, Su M, Li Q, et al. A targeted metabolomic protocol for short-chain fatty acids and branched-chain amino acids. Metabolomics. (2013) 9:818–27. doi: 10.1007/s11306-013-0500-6
68. Rasmussen SO, Martin L, Østergaard MV, Rudloff S, Li Y, Roggenbuck M, et al. Bovine colostrum improves neonatal growth, digestive function, and gut immunity relative to donor human milk and infant formula in preterm pigs. Am J Physiol Gastrointest Liver Physiol. (2016) 311:G480–91. doi: 10.1152/ajpgi.00139.2016
69. Martin GR, Wallace LE, Hartmann B, Holst JJ, Demchyshyn L, Toney K, et al. Nutrient-stimulated GLP-2 release and crypt cell proliferation in experimental short bowel syndrome. Am J Physiol Gastrointest Liver Physiol. (2005) 288:G431–8. doi: 10.1152/ajpgi.00242.2004
70. Lighton JRB. Limitations and requirements for measuring metabolic rates: a mini review. Eur J Clin Nutr. (2017) 71:301–5. doi: 10.1038/ejcn.2016.265
71. Nikaki K, Gupte GL. Assessment of intestinal malabsorption. Best Pract Res Clin Gastroenterol. (2016) 30:225–35. doi: 10.1016/j.bpg.2016.03.003
72. Adam B, Liebregts T, Saadat-Gilani K, Vinson B, Holtmann G. Validation of the gastrointestinal symptom score for the assessment of symptoms in patients with functional dyspepsia. Aliment Pharmacol Ther. (2005) 22:357–63. doi: 10.1111/j.1365-2036.2005.02572.x
73. Buckley MJ, Scanlon C, McGurgan P, O'Morain CA. A validated dyspepsia symptom score. Ital J Gastroenterol Hepatol. (1997) 29:495–500.
74. Herrmann JR, Turner JR. Beyond ussing's chambers: contemporary thoughts on integration of transepithelial transport. Am J Physiol Cell Physiol. (2016) 310:C423–31. doi: 10.1152/ajpcell.00348.2015
75. Yang Z, Sun R, Grinchuk V, Fernández-Blanco JA, Blanco JAF, Notari L, et al. IL-33-induced alterations in murine intestinal function and cytokine responses are MyD88, STAT6, and IL-13 dependent. Am J Physiol Gastrointest Liver Physiol. (2013) 304:G381–9. doi: 10.1152/ajpgi.00357.2012
76. van Nieuwenhoven MA, de Swart EA, van Eijk HM, Deutz NE, Brouns F, Brummer RJ. Effects of pre- and post-absorptive factors on the lactulose/rhamnose gut permeability test. Clin Sci. (2000) 98:349–53. doi: 10.1042/cs19990274
77. Farhadi A, Keshavarzian A, Holmes EW, Fields J, Zhang L, Banan A. Gas chromatographic method for detection of urinary sucralose: application to the assessment of intestinal permeability. J Chromatogr B Anal Technol Biomed life Sci. (2003) 784:145–54. doi: 10.1016/s1570-0232(02)00787-0
78. Pinier M, Fuhrmann G, Galipeau HJ, Rivard N, Murray JA, David CS, et al. The copolymer P(HEMA-co-SS) binds gluten and reduces immune response in gluten-sensitized mice and human tissues. Gastroenterology. (2012) 142:316–25.e12. doi: 10.1053/j.gastro.2011.10.038
79. Zhang JW, Du P, Gao J, Yang BR, Fang WJ, Ying C-M. Preoperative probiotics decrease postoperative infectious complications of colorectal cancer. Am J Med Sci. (2012) 343:199–205. doi: 10.1097/MAJ.0b013e31823aace6
80. Honzawa Y, Nakase H, Matsuura M, Chiba T. Clinical significance of serum diamine oxidase activity in inflammatory bowel disease: importance of evaluation of small intestinal permeability. Inflamm Bowel Dis. (2011) 17:E23–5. doi: 10.1002/ibd.21588
81. Wang L, Llorente C, Hartmann P, Yang AM, Chen P, Schnabl B. Methods to determine intestinal permeability and bacterial translocation during liver disease. J Immunol Methods. (2015) 421:44–53. doi: 10.1016/j.jim.2014.12.015
82. Lutgens LCHW, Blijlevens NMA, Deutz NEP, Donnelly JP, Lambin P, de Pauw BE. Monitoring myeloablative therapy-induced small bowel toxicity by serum citrulline concentration: a comparison with sugar permeability tests. Cancer. (2005) 103:191–9. doi: 10.1002/cncr.20733
83. Relja B, Szermutzky M, Henrich D, Maier M, de Haan JJ, Lubbers T, et al. Intestinal-FABP and liver-FABP: novel markers for severe abdominal injury. Acad Emerg Med. (2010) 17:729–35. doi: 10.1111/j.1553-2712.2010.00792.x
84. Reisinger KW, Derikx JPM, Thuijls G, van der Zee DC, Brouwers HAA, van Bijnen AA, et al. Noninvasive measurement of intestinal epithelial damage at time of refeeding can predict clinical outcome after necrotizing enterocolitis. Pediatr Res. (2013) 73:209–13. doi: 10.1038/pr.2012.160
85. Adriaanse MPM, Tack GJ, Passos VL, Damoiseaux JGMC, Schreurs MWJ, van Wijck K, et al. Serum I-FABP as marker for enterocyte damage in coeliac disease and its relation to villous atrophy and circulating autoantibodies. Aliment Pharmacol Ther. (2013) 37:482–90. doi: 10.1111/apt.12194
86. Alam AN, Sarker SA, Wahed MA, Khatun M, Rahaman MM. Enteric protein loss and intestinal permeability changes in children during acute shigellosis and after recovery: effect of zinc supplementation. Gut. (1994) 35:1707–11. doi: 10.1136/gut.35.12.1707
87. Fasano A. Zonulin, regulation of tight junctions, and autoimmune diseases. Ann N Y Acad Sci. (2012) 1258:25–33. doi: 10.1111/j.1749-6632.2012.06538.x
88. Sapone A, de Magistris Magistris L, Pietzak M, Clemente MG, Tripathi A, Cucca F, et al. Zonulin upregulation is associated with increased gut permeability in subjects with type 1 diabetes and their relatives. Diabetes. (2006) 55:1443–49. doi: 10.2337/db05-1593
89. Grootjans J, Thuijls G, Verdam F, Derikx JP, Lenaerts K, Buurman WA. Non-invasive assessment of barrier integrity and function of the human gut. World J Gastrointest Surg. (2010) 2:61–69. doi: 10.4240/wjgs.v2.i3.61
90. Bertiaux-Vandaele N, Youmba SB, Belmonte L, Lecleire S, Antonietti M, Gourcerol G, et al. The expression and the cellular distribution of the tight junction proteins are altered in irritable bowel syndrome patients with differences according to the disease subtype. Am J Gastroenterol. (2011) 106:2165–73. doi: 10.1038/ajg.2011.257
91. Wilcz-Villega E, McClean S, O'Sullivan M. Reduced E-cadherin expression is associated with abdominal pain and symptom duration in a study of alternating and diarrhea predominant IBS. Neurogastroenterol Motil. (2014) 26:316–25. doi: 10.1111/nmo.12262
92. Costa F, Mumolo MG, Ceccarelli L, Bellini M, Romano MR, Sterpi C, et al. Calprotectin is a stronger predictive marker of relapse in ulcerative colitis than in Crohn's disease. Gut. (2005) 54:364–8. doi: 10.1136/gut.2004.043406
93. Costa F, Mumolo MG, Bellini M, Romano MR, Ceccarelli L, Arpe P, et al. Role of faecal calprotectin as non-invasive marker of intestinal inflammation. Dig Liver Dis. (2003) 35:642–7. doi: 10.1016/S1590-8658(03)00381-5
94. Chassaing B, Srinivasan G, Delgado MA, Young AN, Gewirtz AT, Vijay-Kumar M. Fecal lipocalin 2, a sensitive and broadly dynamic non-invasive biomarker for intestinal inflammation. PLoS ONE. (2012) 7:e44328. doi: 10.1371/journal.pone.0044328
95. Peck BCE, Sincavage J, Feinstein S, Mah AT, Simmons JG, Lund PK, et al. miR-30 family controls proliferation and differentiation of intestinal epithelial cell models by directing a broad gene expression program that includes SOX9 and the ubiquitin ligase pathway. J Biol Chem. (2016) 291:15975–84. doi: 10.1074/jbc.M116.733733
96. Johansson ME V, Hansson GC. Preservation of mucus in histological sections, immunostaining of mucins in fixed tissue, and localization of bacteria with FISH. Methods Mol Biol. (2012) 842:229–35. doi: 10.1007/978-1-61779-513-8_13
97. Volynets V, Reichold A, Bárdos G, Rings A, Bleich A, Bischoff SC. Assessment of the intestinal barrier with five different permeability tests in healthy C57BL/6J and BALB/cJ mice. Dig Dis Sci. (2016) 61:737–46. doi: 10.1007/s10620-015-3935-y
98. Sanders KM, Koh SD, Ro S, Ward SM. Regulation of gastrointestinal motility-insights from smooth muscle biology. Nat Rev Gastroenterol Hepatol. (2012) 9:633–45. doi: 10.1038/nrgastro.2012.168
99. Peacock M. Calcium metabolism in health and disease. Clin J Am Soc Nephrol. (2010) 5: (Suppl. 1):S23–30. doi: 10.2215/CJN.05910809
100. Lotito A, Teramoto M, Cheung M, Becker K, Sukumar D. Serum parathyroid hormone responses to vitamin D supplementation in overweight/obese adults: a systematic review and meta-analysis of randomized clinical trials. Nutrients. (2017) 9:E241. doi: 10.3390/nu9030241
101. Heneghan AF, Pierre JF, Kudsk KA. JAK-STAT and intestinal mucosal immunology. Gut Microbes. (2014) 5:1–8. doi: 10.4161/jkst.25530
102. Wolk K, Kunz S, Witte E, Friedrich M, Asadullah K, Sabat R. IL-22 increases the innate immunity of tissues. Immunity. (2004) 21:241–54. doi: 10.1016/j.immuni.2004.07.007
103. Sundaresan S, Shahid R, Riehl TE, Chandra R, Nassir F, Stenson WF, et al. CD36-dependent signaling mediates fatty acid-induced gut release of secretin and cholecystokinin. FASEB J. (2013) 27:1191–202. doi: 10.1096/fj.12-217703
Keywords: gut assays, biomarkers, safety assessment, efficacy assessment, systems biology, immune intervention, network databases
Citation: Meijerink M, van den Broek TJ, Dulos R, Garthoff J, Knippels L, Knipping K, Harthoorn L, Houben G, Verschuren L and van Bilsen J (2019) Network-Based Selection of Candidate Markers and Assays to Assess the Impact of Oral Immune Interventions on Gut Functions. Front. Immunol. 10:2672. doi: 10.3389/fimmu.2019.02672
Received: 20 May 2019; Accepted: 29 October 2019;
Published: 13 November 2019.
Edited by:
Raquel Hontecillas, Virginia Tech, United StatesReviewed by:
Harry Wichers, Wageningen University & Research, NetherlandsVida Abedi, Geisinger Health System, United States
Copyright © 2019 Meijerink, van den Broek, Dulos, Garthoff, Knippels, Knipping, Harthoorn, Houben, Verschuren and van Bilsen. This is an open-access article distributed under the terms of the Creative Commons Attribution License (CC BY). The use, distribution or reproduction in other forums is permitted, provided the original author(s) and the copyright owner(s) are credited and that the original publication in this journal is cited, in accordance with accepted academic practice. No use, distribution or reproduction is permitted which does not comply with these terms.
*Correspondence: Marjolein Meijerink, marjolein.meijerink@tno.nl