- 1Shemyakin-Ovchinnikov Institute of Bioorganic Chemistry, Russian Academy of Sciences, Moscow, Russia
- 2Institute of Gene Biology, Russian Academy of Sciences, Moscow, Russia
- 3Pirogov Russian National Research Medical University, Moscow, Russia
- 4Neuroimmunological Department of the Federal Center of Cerebrovascular Diseases and Stroke, Moscow, Russia
- 5Sidney Kimmel Comprehensive Cancer Center, The Johns Hopkins University, Baltimore, MD, United States
- 6Vavilov Institute of General Genetics, Russian Academy of Sciences, Moscow, Russia
- 7Institute of Fundamental Medicine and Biology, Kazan (Volga) Federal University, Kazan, Russia
- 8National Research Center Institute of Immunology FMBA of Russia, Moscow, Russia
- 9Department of Microbiology, Thomas Jefferson University, Philadelphia, PA, United States
- 10Institute of Chemical Biology and Fundamental Medicine, Siberian Branch of Russian Academy of Sciences, Novosibirsk, Russia
- 11Department of Fundamental Medicine, Lomonosov Moscow State University, Moscow, Russia
Risk of the development of multiple sclerosis (MS) is known to be increased in individuals bearing distinct class II human leukocyte antigen (HLA) variants, whereas some of them may have a protective effect. Here we analyzed distribution of a highly polymorphous HLA-DRB1 locus in more than one thousand relapsing-remitting MS patients and healthy individuals of Russian ethnicity. Carriage of HLA-DRB1*15 and HLA-DRB1*03 alleles was associated with MS risk, whereas carriage of HLA-DRB1*01 and HLA-DRB1*11 was found to be protective. Analysis of genotypes revealed the compensatory effect of risk and resistance alleles in trans. We have identified previously unknown MBP153−161 peptide located at the C-terminus of MBP protein and MBP90−98 peptide that bound to recombinant HLA-DRB1*01:01 protein with affinity comparable to that of classical antigenic peptide 306-318 from the hemagglutinin (HA) of the influenza virus demonstrating the ability of HLA-DRB1*01:01 to present newly identified MBP153−161 and MBP90−98 peptides. Measurements of kinetic parameters of MBP and HA peptides binding to HLA-DRB1*01:01 catalyzed by HLA-DM revealed a significantly lower rate of CLIP exchange for MBP153−161 and MBP90−98 peptides as opposed to HA peptide. Analysis of the binding of chimeric MBP-HA peptides demonstrated that the observed difference between MBP153−161, MBP90−98, and HA peptide epitopes is caused by the lack of anchor residues in the C-terminal part of the MBP peptides resulting in a moderate occupation of P6/7 and P9 pockets of HLA-DRB1*01:01 by MBP153−161 and MBP90−98 peptides in contrast to HA308−316 peptide. This leads to the P1 and P4 docking failure and rapid peptide dissociation and release of empty HLA-DM–HLA-DR complex. We would like to propose that protective properties of the HLA-DRB1*01 allele could be directly linked to the ability of HLA-DRB1*01:01 to kinetically discriminate between antigenic exogenous peptides and endogenous MBP derived peptides.
Introduction
Human leukocyte antigen (HLA) genes encode proteins that are capable to bind and present antigenic peptides and, therefore, play a critical role in the immune responses against pathogens as well as those resulting in autoimmunity (1–4). Binding of antigenic peptides to HLA class II molecules produces binary peptide-HLA ligands displayed on the cell surface for recognition by T-cell receptors (5). Initially, nascent HLA proteins are protected by the invariant chain (6). In the endosome compartment, the invariant chain is partially degraded leaving HLA class II-associated Ii peptide (CLIP) in the binding groove (7, 8). Peptide antigens that are processed in the endosomes could then exchange with CLIP bound to the HLA molecules, a process that is facilitated by HLA-DM protein (9, 10). Finally, the peptide-HLA II complex is translocated to the surface of the antigen presenting cell (APC) for survey by T cells. While mechanisms of peptide presentation by HLA class II proteins is well-understood (11, 12), how generation and presentation of self-peptide-HLA class II ligands results in the development of autoimmune reactions is still unclear and remains a subject of a great interest. Indeed, identification of self-peptide-HLA class II ligands that are linked to autoimmune reactions promises to provide a clue for understanding of the pathogenesis of autoimmune disorders (13–15).
Multiple sclerosis (MS), a chronic autoimmune disease of the central nervous system (CNS), which is characterized by inflammation, demyelination, and neurodegeneration (16). The nature of genetic susceptibility to MS is complex and depends on the interplay between multiple genetic, epigenetic, and environmental factors (17). Since the early 2000s, genome-wide association studies have been exploited as a powerful tool for investigating the genetic basis of MS and have revealed more than 200 disease-associated loci; however, genes within HLA region are thought to exert a major genetic contribution to MS risk (18). Particular alleles of the highly polymorphous HLA class II DRB1 gene appear to be the strongest genetic determinant for MS and may influence both predisposition and resistance to the disease (19). Genetic heterogeneity in MS patients were observed in different populations. For instance, the HLA-DRB1*15:01 allele and its associated haplotype (DQB1*06:02, DQA1*01:02, DRB1*15:0, DRB5*01:01) have been known as a near-universal MS risk factor since the 1970s. Analysis of the HLA associations in Northern European MS populations uncovered many other HLA-DRB1 alleles (DRB1*03, *01, *10, *11, *14, *08) that were either positively or negatively associated with the disease (20). The distinct autoantigenic peptides presented by predisposing alleles have been identified. For instance, DRB1*15:01 binds peptide from myelin basic protein (MBP), i.e., MBP85−99 peptide (14), while DRB5*01:01 presents MBP86−105 peptide (13) and DRB1*04:01 can display MBP111−129 peptide (21). While these findings define disease-associated peptide-HLA ligands recognizable by T-cells (21–23), the mechanism providing resistance to MS by protective HLA alleles is not known. Here, on a representative cohort of ethnically Russian MS patients and healthy individuals, we show that group of alleles HLA-DRB1*01 is associated with resistance to MS. We have identified a novel MBP-derived peptide ligand presented by a particular HLA-DRB1*01:01 protein and have shown, that this HLA class II protein can kinetically discriminate between the MBP and virus peptides suggesting a mechanism responsible for resistance to MS of individuals that carry HLA-DRB1*01 alleles.
Materials and Methods
Patients and Controls
Five hundred and sixty five unrelated relapsing-remitting MS (hereinafter referred to as “MS”) patients from Moscow Multiple Sclerosis Center diagnosed according to the McDonald Criteria (24) and self-reported as Russians were selected for the study. Four hundred and seventy-one healthy individuals without neurological disorders and familial history of MS were included in the control group; they were also self-reported as Russians. All MS patients and healthy individuals lived in the Moscow region. Demographic and clinical data for all participants are presented in Table 1. No significant differences in demographic characteristics (age and sex ratio) were observed between two groups. This study was carried out in accordance with the recommendations of local ethics committee of the Moscow Multiple Sclerosis Center. All subjects gave written informed consent in accordance with the Declaration of Helsinki. The protocol #13 from 15 September 2014 was approved by the local ethics committee of the Moscow Multiple Sclerosis Center.
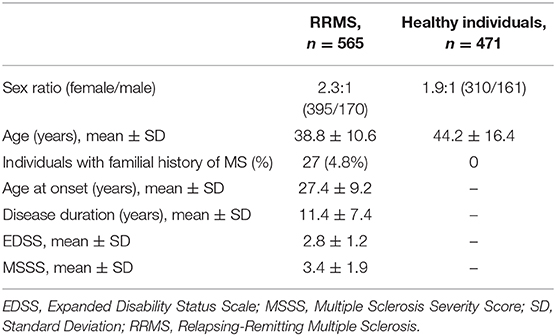
Table 1. Demographic and clinical data for relapsing-remitting multiple sclerosis patients and healthy individuals (all Russians).
Genotyping and Statistical Analysis
Genomic DNA was extracted from the peripheral blood samples with QIAamp DNA Blood Midi Kits (QIAGEN). Low-resolution (two-digital) genotyping of the HLA-DRB1 locus was performed using HLA-DRB1 real-time PCR Genotyping Kit (DNA-Technology). HLA-DRB1 alleles and genotypes associated with risk of MS were identified using the APSampler software [http://apsampler.sourceforge.net/] and validated with Fisher's exact test included in the software. Association was considered nominally significant; if uncorrected p-values (pf) for identified alleles/genotypes were <0.05 and 95% confidence interval (CI) for odds ratio (OR) did not cross 1. A standard permutation test with 100 APSampler runs was performed for each finding as a multiple hypothesis testing correction. The significance threshold was set at pperm <0.05.
Preparation of Human Dendritic Cells and Identification of Bound Peptides
The fraction of mononuclear cells (PBMC), containing dendritic cells (DC) progenitors, were isolated from human blood according standard protocol (25). 20–50 ml of blood from each donor was diluted 3 times with PBS-EDTA (PBS with 2 mM EDTA), carefully underlayered with 1/4 of volume with Ficoll solution (1.077 g/cm3, Paneco) and centrifuged at 750 g for 30 min at room temperature. The dense band of PBMCs was removed carefully, placed into 50 ml tube, diluted 3 times with PBS-EDTA, centrifuged at 200 g for 10 min at 4°C and the cell pellet was once washed with PBS-EDTA. Then it was solved in RPMI advanced medium with 10% bovine fetal serum, glutamax and antibiotic-antimicotic (ThermoFisher Scientific), seeded in 25 cm2 cultural flasks in 6*106 cell/ml concentration. After 2 h the unbound cells were removed and media was changed to fresh portion with DC growth factors—IL4 (100 ng/ml) and GM-CSF (50 ng/ml) (StemCells) and then cultivated for 6 days with a change of 1/2 of media volume each second day as described Markov et al. (26). After 6 days full media volume was changed to fresh portion with Bacterial LipoPolysaccharide (10 mkg/ml) and cultivated for 24 h for DC maturation. After 7 days DC were unbound by cell scrapper, lysed in PBS with 0.25% of sodium deoxycholate in presence of complete EDTA-free inhibitors (Roche), PMSF, Pepstatin, EDTA for 1 h at 4°C with following centrifugation at 16,000 g for 20 min. Then cell lysates were applied onto size-exclusion chromatography column Superdex75 (GE Healthcare). Presence of MHC II molecules in several high-molecular fractions, corresponding to MHCII tetramers, were verified by ELISA, where MHCII molecules were defined by binding with pre-immobilized mouse L243 antibody (anti-MHCII) and following successive interaction with rabbit anti-MHCII polyclonal serum and with anti-rabbit anti-whole molecule antibody-HRP (Sigma). These fractions were lyophilized.
LC-MS/MS and DATA Analysis
Isolated peptides were desalted using SDB-RPS StageTips as it was described earlier (27). LC-MS/MS analysis was performed using the Q Exactive HF benchtop Orbitrap mass spectrometer (Thermo Fisher Scientific) which was coupled to the Ultimate 3000 Nano LC System (Thermo Fisher Scientific) via a nanoelectrospray source (Thermo Fisher Scientific). The HPLC system was configured in a trap-elute mode. Peptide solution were loaded on an Acclaim PepMap 100 (100 μm × 2 cm) trap column and separated on an Acclaim PepMap 100 (75 μm × 50 cm) column (both from Thermo Fisher Scientific). Correlation of MS/MS spectra with peptide sequences was made using PEAKS Studio 8.0 build 20160908 software (28). Peptide lists generated by the PEAKS Studio were searched against the Homo sapiens Uniprot FASTA database (154257 species, version July 2016) and with methionine oxidations and asparagine/glutamine deamidations as variable modifications. The false discovery rate (FDR) for peptide-spectrum matches was set to 0.01 and was determined by searching a reverse database. Peptide identification was performed with an allowed initial precursor mass deviation up to 10 ppm and an allowed fragment mass deviation of 0.05 Da.
MHC Expression and Purification
The genetic constructions for recombinant HLA-DR (HLA-DRB1*01:01 and HLA-DRB1*15:01) α and β (with or without CLIP) chains expression were created based on pMT-V5/His and pRmHa vectors, respectively. All HLA-DRs carried parts of leucine zipper from c-jun and c-fos transcription factors as previously described (29). CLIP (PVSKMRMATPLLMQA) was covalently attached with the linker with a thrombin site at the N-terminus of β chain. The individual stable lines of Drosophila melanogaster S2 cells, carrying both genes of appropriate α and β (with or without CLIP) HLA-DR chains and separate plasmid pCoBlast (Invitrogen) with blasticidin resistance, were obtained. The HLA-DRB1 proteins with and without CLIP were expressed in SF900 III Media (Gibco) during 3–7 days after induction with 1 mM Cu2+ at 28°C with shaking. Then the cell culture concentrate was applied to affinity anti-MHC II (L243) resin in PBS, followed by elution with 50 mM glycine buffer (pH 11.5) and rapid neutralization of eluate by 2 M Tris-HCl (pH 8.0) (29). For the next step, impurities were removed with MonoQ column (GE Healthcare) in 0–1 M NaCl gradient. Constructions for recombinant HLA-DM α and β chain expression in eukaryotic suspension cells, HEK 293F, were previously created based on pFUSE vector encoding constant fragments of human immunoglobulin heavy chain (Fc) (29). The appropriate constructions were used for transit transfection of HEK293F cells with a following expression of HLA-DM, performed in serum-free FreeStyle medium (Gibco) until the percentage of living cells was lower than 60% (typically 5–7 days). Then the concentrated culture medium was loaded into a Protein G affinity column (GE Healthcare), followed by elution with 50 mM glycine buffer (pH 2.5) and rapid neutralization of eluate by 2 M Tris-HCl (pH 8.0). The second purification step comprised the use of an ion-exchange MonoQ column (GE Healthcare), following the same procedure as described above. Proteins were concentrated, transferred to 20 mM Tris-HCl (pH 8.0), 150 mM NaCl buffer and stored at 4°C.
Thioredoxin-Fused Peptides Expression and Purification
Thioredoxin-fused peptides were constructed and produced earlier as parts of an MBP epitope library (30). Twelve successive overlapping short fragments of MBP (25–30 aa) were placed on the C-terminus of bacterial thioredoxin via flexible linker (SGGGG)3S, carrying an His-tag for purification. The substrate construct, carrying only thioredoxin with the linker (TRX), was used as a control. New thioredoxin-fused peptides were created in this work, using MBP epitope library as a template. The genetic constructs, encoding HA, pp65, CLIP, MBP with point mutations and chimeric peptides, were obtained by insertion using overlapping PCR. All thioredoxin-fused substrates were produced in Escherichia coli BL21 (DE3) strain in soluble form, purified with Ni-NTA (Qiagen) and MonoS (GE Healthcare) columns (30). Thioredoxin-fused peptides were chemically biotinylated with EZ-Link Sulfo-NHS-LC-biotin (Thermo Fisher Scientific) in molar ratio 1:20 for 30 min at 25°C. Proteins were concentrated in PBS and stored at −20°C.
Peptide Binding Experiments and Dissociation Constant Determination
Both biotinylated synthetic and thioredoxin-fused MBP peptides (750 nM, which is much higher than measured KD to study maximal binding) from the MBP library and with point mutations, were incubated overnight at 37°C in PBS in 50 μl with HLA-DR (HLA-DRB1*01:01 or HLA-DRB1*15:01) (150 nM). DR/peptide complexes were then captured on the ELISA plate with immobilized L243 mAb (5 μg/ml in PBS) and blocked with 1% milk in PBS. HLA-DR-bound biotinylated peptide was quantitated with streptavidin-peroxidase (50 μl in PBST), using 3,3′,5,5′-tetramethylbenzidine (50 μl) as a substrate and stopping with 10% phosphoric acid (50 μl). ELISA was performed using standard protocol (https://www.abcam.com/protocols/sandwich-elisa-protocol).
In a competition assay, biotinylated thioredoxin-fused HA and pp65 peptides (150 nM) were incubated with HLA-DR in the presence of HA, pp65, MBP or chimeric peptides (1,000, 500, 250, 125, 62.5, 31.2, 15.6, and 7.8 nM) for 18 h. Whole procedure was the same as described above.
For KD determination, biotinylated synthetic and thioredoxin-fused peptides were incubated in the same conditions as described above with HLA-DR (5 nM) at the following concentrations: 10,000, 3,000, 1,000, 300, 100, 30, 10, 3, and 0.1 nM. DR/peptide complexes were quantitated using Europium-labeled streptavidin and enhancement solution. DELFIA was performed according to standard protocol (http://www.perkinelmer.com/lab-products-and-services/application-support-knowledgebase/delfia/delfia-immunoassays.html). All experiments were carried out in triplicates.
Kinetic Measurement of Peptide Loading on HLA-DR
A real-time ELISA assay was used to assess kinetics of peptide exchange on HLA-DR, catalyzed by HLA-DM. The linker connecting the CLIP peptide to the N-terminus of the HLA-DR β chain was cut with thrombin (1 h, 20 U/mg, at room temperature). DR-CLIP complexes (150 nM) were incubated with or without HLA-DM (150 nM, which was estimated as minimal concentration for maximal rate, see Figure S1) in the presence of biotinylated either synthetic or thioredoxin-fused HA, pp65, MBP, and chimeric peptides (150 nM, which was experimentally estimated as minimal concentration required for reliable detection), for 7, 5, 3, 1, and 0 h. Each time point was mixed separately starting from the end of incubation time (7 h) every 2 h, afterwards all time points were loaded into the plate.
In a competition assay, kinetic experiments were carried out as described above. Kinetics were measured in the presence of increasing concentrations (0, 30, 100, 300, and 1,000 nM) of thioredoxin-fused HA, pp65, MBP, or chimeric peptides at timepoints 8, 6, 4, 2, and 0 h. Thioredoxin without any peptide was used as a control. ELISA was performed as done previously. All experiments were carried out in triplicates.
Results
Genetic Association of HLA-DRB1 Gene Variants With MS
We analyzed HLA-DRB1 allelic distribution in 565 MS patients and 471 healthy individuals (all of Russian ethnicity) using low-resolution (two-digital) genotyping. Significant differences in carriage frequencies of several HLA-DRB1 groups of alleles (hereinafter “alleles”) were observed (Figure 1A and Table S1). Alleles HLA-DRB1*03 and *15 were strongly associated with high MS risk [pperm = 0.0056, OR = 1.77 (CI: 1.27–2.49) and pperm = 5.8 × 10−14, OR = 2.84 (CI: 2.17–3.72), respectively]. HLA-DRB1*01, *09, *11, and *12 alleles were significantly enriched in the control group. However, only alleles HLA-DRB1*01 and *11 were determined to be protective after correction for multiple comparisons [pperm = 0.00062, OR = 0.55 (CI: 0.41–0.74) and pperm = 0.0011, OR = 0.56 (CI: 0.42–0.76), respectively], while others simply remained nominally significant.
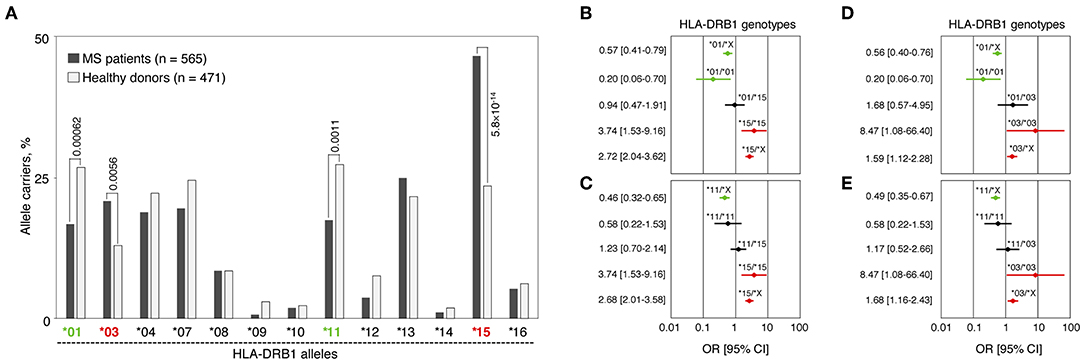
Figure 1. Genetic association of HLA-DRB1 gene variants with MS. (A) Carriage frequencies of HLA-DRB1 alleles in MS patients and healthy controls. pperm values for alleles DRB1*01, *03, *11 and *15, significantly associated with MS after correction for multiple comparisons, are presented. (B–E) Forest plots demonstrate odds ratios (ORs) and their 95% confidential intervals (95%CI) for the HLA-DRB1 genotypes that include identified MS predisposing and protective alleles as homozygotes and in heterozygous combinations with each other or with the rest of the HLA-DRB1 alleles. (B) HLA-DRB1*01 and *15 alleles; (C) HLA-DRB1*11 and *15 alleles; (D) HLA-DRB1*01 and *03 alleles; (E) HLA-DRB1*11 and *03 alleles. For each plot (B–E), a set of all the DRB1 alleles excluding the alleles of interest is denoted by the letter “X”; OR [95%CI] values are shown in red for positive association with MS genotypes, in green for negatively associated genotypes and in black for non-significant genotypes.
We further analyzed frequencies of heterozygous genotypes including one risk allele (HLA-DRB1*03 or *15) and one resistance allele (HLA-DRB1*01 or *11), i.e., *01/*15, *11/*15, *01/*03, and *11/*03 in MS patients and healthy individuals. Neither of those genotypes were associated with MS: 95% CI for OR crossing 1 (Figures 1B–E), while pf-values lay within the interval between 0.25 and 0.51 (not shown). These data suggest the compensatory effect of any identified protective and predisposal alleles in trans. Moreover, dose allele effect was observed when homozygotes *01/*01, *03/*03, and *15/*15 were compared with heterozygotes containing HLA-DRB1*01, *03, or *15 alleles in combination with alleles not associated with MS (denoted as “X” on Figures 1B–E). For HLA-DRB1*11 allele (Figures 1C,E), the dose effect was not observed.
Genetic constructs bearing HLA-DRB1*01:01 and *15:01 alleles were used for further analyses since these alleles are the most frequent among corresponding groups of alleles.
HLA-DRB1*01:01 Binds Encephalitogenic and C-Terminal Peptides of the Myelin Basic Protein
We further determined if recombinant HLA-DRB1*01:01, encoded by the most common allele among HLA-DRB1*01 groups [http://allelefrequencies.net/hla6006a.asp], may bind MBP-derived peptides utilizing a previously created MBP epitope library (30) (Figure 2A). HLA-DRB1*01:01 specifically bound MBP146−170 and less efficiently bound three other peptides MBP130−156, MBP81−104, and MBP65−92 (Figure 2B). As anticipated, HLA-DRB1*15:01 specifically recognized encephalitogenic peptide MBP81−104 (Figure 2B). Two more fragments, namely MBP25−54 and MBP130−156, were bound by HLA-DRB1*15:01; however, with significantly diminished efficiency. Obtained data were verified utilizing chemically synthesized biotinylated peptides (Figure 2C). The HLA-DRB1*01:01 recognized its classical ligand–immunodominant peptide 306-318 of the hemagglutinin (HA) of the influenza virus (31), and both myelin peptides, MBP81−104 and MBP146−170. The HLA-DRB1*15:01 bound only MBP81−104, and neither HLA-DRB1*01:01 nor HLA-DRB1*15:01 bound irrelevant MBP17−41 peptide. LC-MS/MS analysis of peptides associated with HLA exposed on the dendritic cells isolated from normal individual with heterozygous genotype HLA-DRB1*01:01/*15:01 revealed three main MBP regions, which corresponded to MBP81−104, MBP25−54, and MBP130−156, that were shown to be bound by HLA-DRB1*15:01. At the same time, we failed to detect any peptides related to MBP146−170, bound by HLA-DRB1*01:01 (Figure 2A). In line with these findings peptide MBP146−170 did not activate proliferation of CD4-positive T cells according to the CFSE assay (Figure S2).
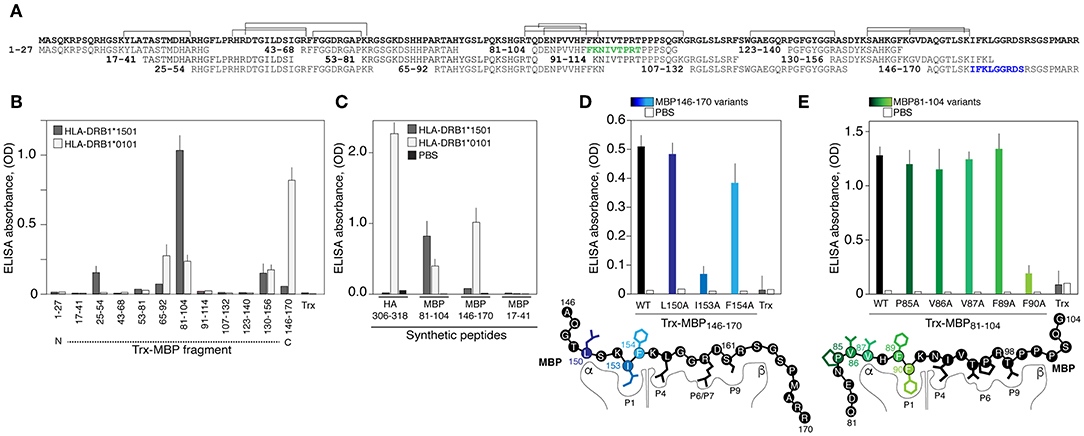
Figure 2. The HLA-DRB1*01:01 recognizes encephalitogenic and C-terminal parts of the myelin basic protein. (A) Amino acid sequence of the MBP. Overlapping peptides of the epitope library are indicated. Two epitopes of the HLA-DRB1*01:01 are marked by green and blue. Brackets correspond to the sequences of peptides associated with HLA exposed on the dendritic cells isolated from normal individual with heterozygous genotype HLA-DRB1*01:01/*15:01 according to LC-MS/MS analysis. (B) Analysis of binding of HLA-DRB1*15:01 (gray bars) and HLA-DRB1*01:01 (white bars) (150 nM) with the MBP epitope library (750 nM). Trx denotes thioredoxin. Standard deviation is indicated. (C) Recognition of the chemically synthesized peptides representing MBP fragments and HA306−318 peptide (750 nM) by the HLA-DRB1*15:01 (gray bars) and HLA-DRB1*01:01 (white bars) (150 nM). Black bars represent background signal (PBS). Standard deviation is indicated. (D,E) Binding of the thioredoxin-fused peptides and its variants (750 nM) with alanine point substitutions representing MBP146−170 (D) and MBP81−104 (E) with HLA-DRB1*01:01 (150 nM). Open bars represent background signal. Standard deviation is indicated. Point mutations are indicated by different overtones.
Myelin Peptides Recognized by HLA-DRB1*01:01 Contained Polar Residues in P6/P7 and P9
Next, it was important to determine binding epitopes recognized by HLA-DRB1*01:01, which are present in MBP81−104 and MBP146−170. Alanine scanning (substitutions of hydrophobic and aromatic residues) of the thioredoxin-fused MBP146−170 peptide (Figure 2D) revealed that the P1 pocket in the HLA-DRB1*01:01 carrying MBP146−170 is occupied by isoleucine at position 153 (human MBP1−170 nomenclature). Polar arginine and serine at positions 159 and 161 represent the P6/P7 and P9 residues, respectively. Alanine scanning of the thioredoxin-fused MBP81−104 peptide (Figure 2E) resulted in the determination of the phenylalanine at position 90 as a hydrophobic P1 anchor; in turn, this suggested that P6/P7 and P9 pockets in the HLA-DRB1*01:01 bound with MBP81−104 are occupied by threonine, proline, and threonine at positions 95, 96, and 98, respectively.
Loading of the HLA-DRB1*01:01 by Viral and Myelin Peptides Is Thermodynamically but Not Kinetically Equal
Dissociation constant (KD) of the HLA-peptide complex may characterize strength, which is required to remove a fully docked peptide from the HLA binding groove. To determine this, we measured KD for the complexes of the HLA-DRB1*01:01 with myelin peptides MBP81−104, MBP146−170, and viral HA306−318. For this purpose, we utilized both chemically synthesized peptides (Figure 3A) and thioredoxin-fused recombinant epitopes (Figure 3B). Calculated values of the KD revealed that HLA-DRB1*01:01 binds all epitopes with approximately similar efficacy (Figure 3C).
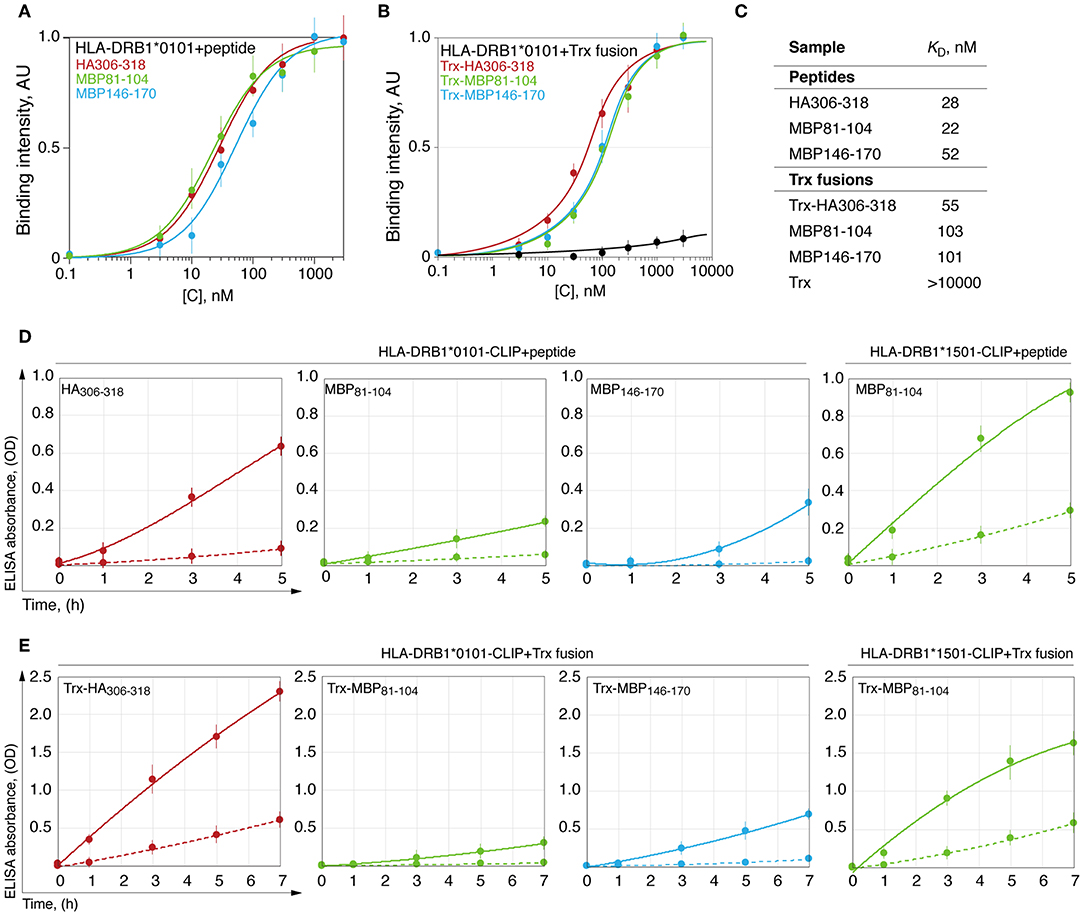
Figure 3. HLA-DRB1*01:01 kinetically discriminates peptides with similar thermodynamic affinities. Binding of chemically synthesized peptides HA306−318 (red curve), MBP81−104 (green curve) and MBP146−170 (blue curve) (A) and their thioredoxin-fused analogous (B) in different concentrations (0.1 nM−3 μM) with HLA-DRB1*01:01 (5 nM) measured by DELFIA with time-resolved fluorescence detection. AU denotes arbitrary units and Trx represents thioredoxin. Dissociation constants are summarized in (C). Kinetics of binding of chemically synthesized peptides (D) and their recombinant analogous as thioredoxin fusions (E) containing HA306−318 (red curve), MBP81−104 (green curve), and MBP146−170 (blue curve) (150 nM), to preloaded with CLIP HLA-DRB1*01:01 and HLA-DRB1*15:01 (150 nM) with (solid curves) or without (dashed curves) HLA-DM (150 nM).
Removing the CLIP and docking of the antigenic peptides on the HLA class II is a dynamic process that is catalyzed by the HLA-DM (32, 33). During this process, despite similar affinity, the HA peptide exchanged CLIP loaded onto HLA-DRB1*01:01 significantly more rapidly in comparison with MBP peptides 81-104 and 146-170, whereas HLA-DRB1*15:01 bound MBP81−104 to a similar rate of the HLA-DRB1*01:01–HA interaction (Figure 3D). Study of loading of these peptides as a part of thioredoxin fusion proteins on HLA resulted in identical results (Figure 3E).
C-Terminal P6/P7 and P9 Residues in Viral and Self-Peptides Make Their Kinetic Discrimination by HLA-DRB1*01:01 Possible
To determine the reason for the slow rate of C-terminal myelin peptide loading on HLA-DRB1*01:01, we created chimeric peptides representing combinations of N- and C-terminal parts of HA306−318, CLIP103−117, MBP151−164, and MBP88−100 (Figures 4A,B). Thioredoxin-fused chimeric peptides bearing the C-terminal part from the HA peptide (Trx-[HA], Trx-[MBP151−156-HA], Trx-[MBP88−93-HA], and Trx-[CLIP-HA]) were loaded on HLA-DRB1*01:01 with or without HLA-DM with a similar rate regardless of the N-terminal part. Conversely, none of the chimeric peptides assembled from the C-terminal part of MBP151−164 or MBP88−100 peptides (Trx-[MBP151−164], Trx-[HA-MBP157−164], Trx-[CLIP-MBP157−164], Trx-[MBP88−100], Trx-[HA-MBP94−100]) were capable of efficiently binding HLA-DRB1*01:01. Similar to these findings, substitution of the N-terminal part of the CLIP by fragments from either HA or MBP151−164 (Trx-[HA-CLIP]and Trx-[MBP151−156-CLIP]) had only a moderate effect on the HLA-DRB1*01:01 binding.
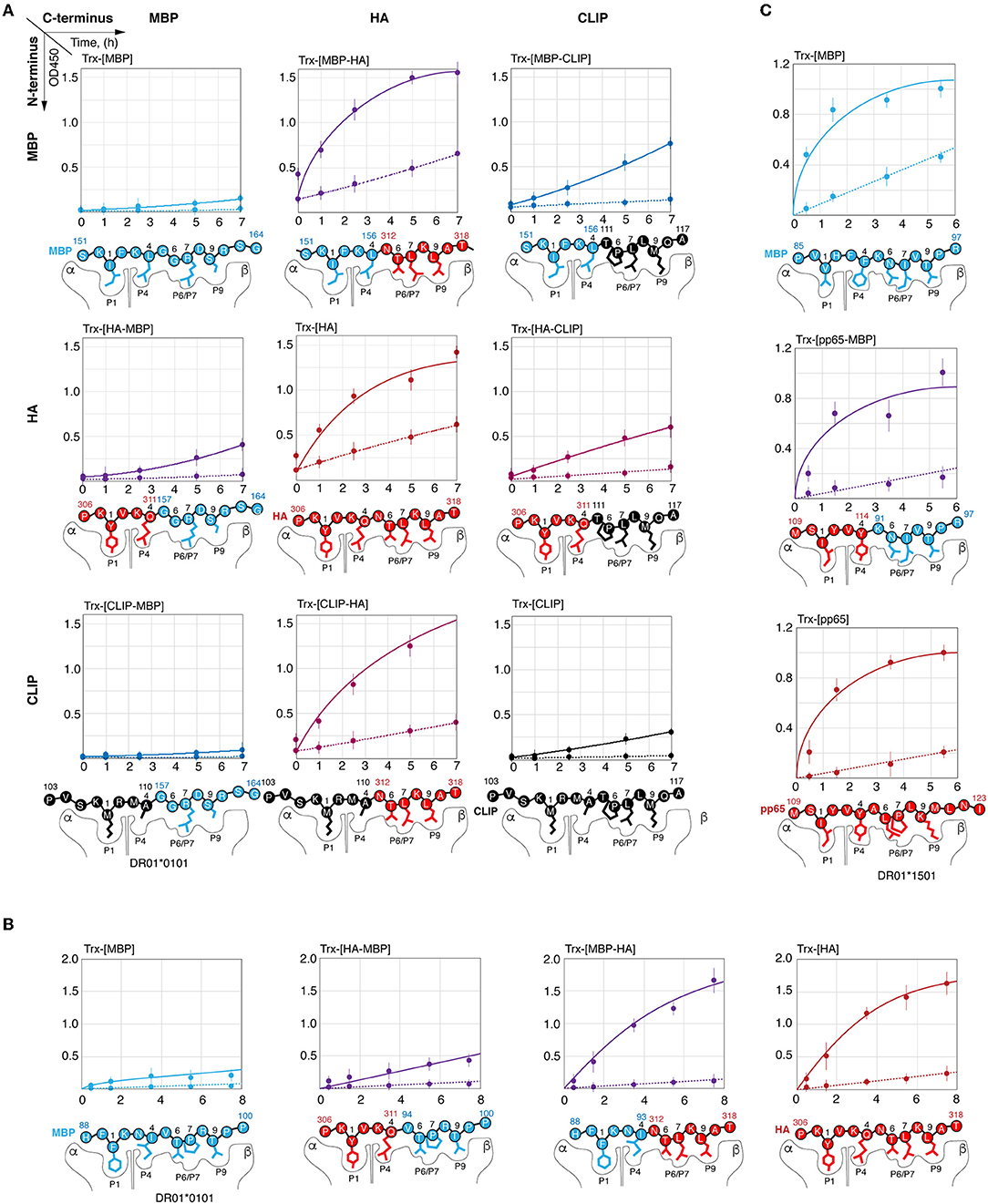
Figure 4. Kinetic discrimination of myelin and viral peptides by HLA-DRB1*01:01 is caused by their C-terminal residues. Kinetics of binding of thioredoxin fusions (150 nM) containing chimeric peptides assembled from N- and C-terminal parts of HA306−318 (red), MBP151−164 (blue), and CLIP (black) with HLA-DRB1*01:01 (150 nM) (A); of HA306−318 (red) and MBP88−100 (blue) with HLA-DRB1*01:01 (150 nM) (B); of pp65109−123 (red) and MBP85−97 (blue) with HLA-DRB1*15:01 (150 nM) (C) in the presence (solid lines) or absence (dashed lines) of HLA-DM (150 nM) measured by ELISA. Anchor residues are indicated.
In order to elucidate if there is a similar dependence on the peptide C-terminus in case of HLA-DRB1*15:01 and MBP85−97, we created chimeric peptide representing fusion of N-terminal part of pp65109−123 [antigenic peptide from cytomegalovirus structural protein (34) and C-terminal part of MBP85−97 (Figure 4C). All peptides (Trx-[pp65], Trx-[pp65-MBP91−97], and Trx-[MBP85−97]) were loaded on HLA-DRB1*15:01 with or without HLA-DM with a similarly high rate.
C-Terminal Part of Myelin Peptide Significantly Restricts Its Ability to Compete With HA Peptide in Terms of Loading on HLA-DRB1*01:01
We next determined the ability of MBP151−164 to compete with HA306−318 for HLA-DRB1*01:01 binding. To determine this, HLA-DRB1*01:01 was incubated with HLA-DM and biotinylated thioredoxin-fused HA306−318 (Trx-[HA]bio) in the presence of increasing concentrations of either Trx-HA306−318 (Trx-[HA]), Trx-MBP151−164 (Trx-[MBP151−164]) or their chimeric variants (Figure 5A). We determined that Trx-[MBP151−164] and Trx-[HA-MBP157−164] failed to compete with Trx-[HA]bio up to a concentration of 1 μM, whereas non-biotinylated Trx-[HA] and Trx-[MBP151−156-HA] decreased binding of Trx-[HA]bio starting from a concentration of 30 nM. To verify that observed difference is caused by inappropriate P6/P7 and P9 residues in MBP151−164 peptide, as was shown in case of kinetic discrimination of myelin and viral peptides, we determined the affinity of two chimeric peptides Trx-[HA-MBP157−164] and Trx-[MBP151−156-HA] (Figure 5B) in comparison with their ability to compete with Trx-[HA]bio for HLA-DRB1*01:01 binding (Figure 5C). Our data suggest that the comparable thermodynamically inhibition capacity of Trx-[HA-MBP157−164] is up to two orders of magnitude lower than Trx-[HA] and Trx-[MBP151−156-HA].
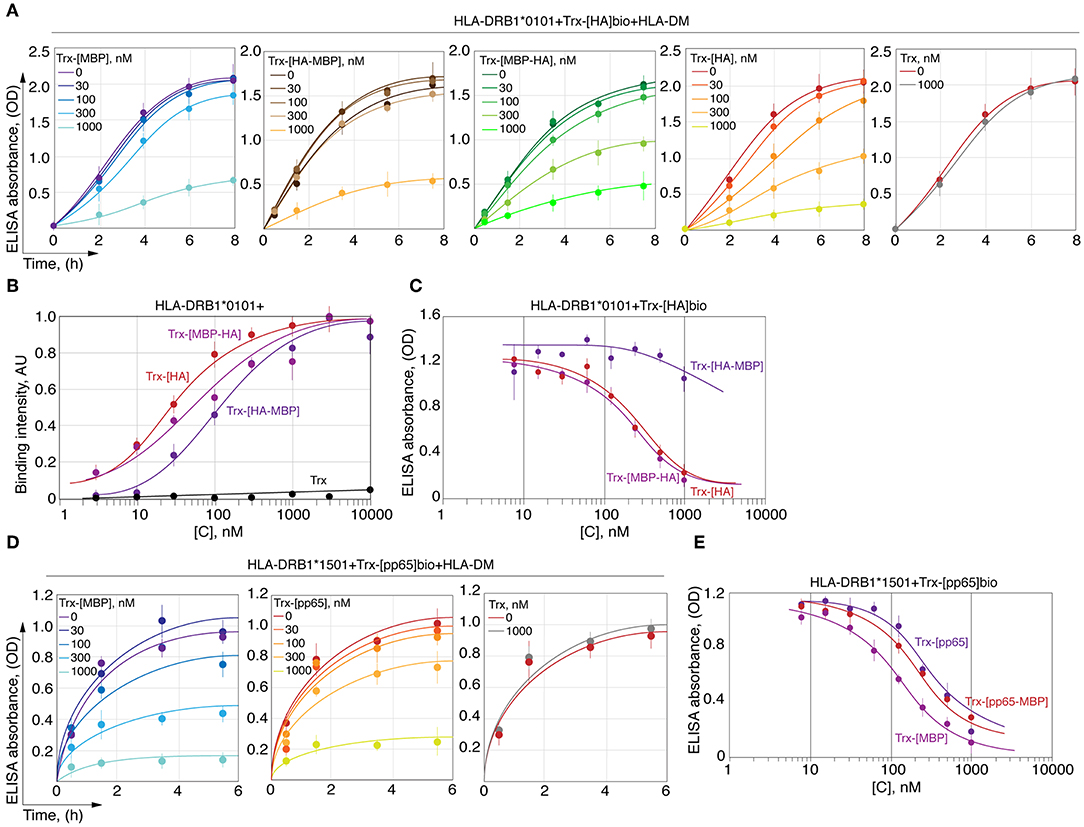
Figure 5. HA peptide prevents loading of MBP146−170 onto the HLA-DRB1*01:01. (A) Recombinant HLA-DRB1*01:01 (150 nM) and HLA-DM (150 nM) were incubated with biotinylated thioredoxin-fused HA306−318 (Trx-[HA]bio) (150 nM) in the presence of increasing concentrations (0-1 μM) of thioredoxin-fused MBP151−164 (Trx-[MBP]), HA306−311-MBP157−164 (Trx-[HA-MBP]), MBP151−156-HA312−318 (Trx-[MBP-HA]) and HA306−318 (Trx-[HA]). Thioredoxin (Trx) without any peptide was used as a control. At indicated timepoints 8, 6, 4, 2, and 0 h, the amount of bound Trx-[HA]bio was determined by addition of streptavidin-HRP. (B) Binding of thioredoxin fusions containing HA306−318 (red) and chimeric peptides assembled from N- and C-terminal parts of HA306−318 and MBP151−164 (Trx-[MBP-HA] and Trx-[HA-MBP]) in different concentrations (3 nM−10 μM) with recombinant HLA-DRB1*01:01 (5 nM) measured by DELFIA with time-resolved fluorescence detection. (C) Recombinant HLA-DRB1*01:01 (150 nM) was incubated with biotinylated thioredoxin-fused HA306−318 (Trx-[HA]bio) (150 nM) in the presence of increasing concentrations (7.8 nM−1 μM) of thioredoxin-fused HA306−318 (Trx-[HA]) and chimeric peptides assembled from N- and C-terminal parts of HA306−318 and MBP151−164 (Trx-[MBP-HA] and Trx-[HA-MBP]). After 18 h of incubation, the amount of bound Trx-[HA]bio was determined by addition of streptavidin-HRP. (D) Recombinant HLA-DRB1*15:01 (150 nM) and HLA-DM (150 nM) were incubated with biotinylated thioredoxin-fused pp65109−123 (Trx-[pp65]bio) (150 nM) in the presence of increasing concentrations (0-1 μM) of thioredoxin-fused MBP85−97 (Trx-[MBP]), pp65109−114-MBP91−97 (Trx-[pp65-MBP]), and pp65109−123 (Trx-[pp65]). Thioredoxin (Trx) without any peptide was used as a control. At indicated timepoints 5.5, 3.5, 1.5, and 0.5 h, the amount of bound Trx-[pp65]bio was determined by addition of streptavidin-HRP. (E) Recombinant HLA-DRB1*15:01 (150 nM) was incubated with biotinylated thioredoxin-fused pp65109−123 (Trx-[pp65]bio) (150 nM) in the presence of increasing concentrations (7.8 nM−1 μM) of thioredoxin-fused MBP85−97 (Trx-[MBP]), pp65109−114-MBP91−97 (Trx-[pp65-MBP]), and pp65109−123 (Trx-[pp65]). After 18 h of incubation, the amount of bound Trx-[pp65]bio was determined by addition of streptavidin-HRP.
We also determined the ability of MBP85−97 to compete with pp65109−123 for HLA-DRB1*15:01 binding. To determine this, HLA-DRB1*15:01 was incubated with HLA-DM and biotinylated thioredoxin-fused pp65109−123 (Trx-[pp65]bio) in the presence of increasing concentrations of either Trx-pp65109−123 (Trx-[pp65]) or Trx-MBP85−97 (Trx-[MBP85−97]) (Figure 5D). We showed that Trx-[MBP85−97] is capable to compete with Trx-[pp65]bio, similar to non-biotinylated Trx-[pp65], decreasing binding of Trx-[pp65]bio starting from a concentration of 100 nM. Our data suggest that thermodynamic inhibition capacity of Trx-[MBP85−97] in comparison with Trx-[pp65] and Trx-[pp65-MBP91−97] is up to half of order of magnitude greater (Figure 5E).
Discussion
In the present study, we have shown the strong association of HLA-DRB1*15 and *03 alleles with MS risk and the significant protective effect of HLA-DRB1*01 and *11 alleles in ethnic Russian people. The association between HLA-DRB1*15 allele and MS was previously shown based on the analysis of a limited independent cohort of ethnic Russians (35). For HLA-DRB1*15, which is widely known as the strongest genetic risk factor of MS, we observed that the OR value was equal to 2.84, which is similar to the results obtained for the majority of European populations (OR = 3.08) (18). Published data on the association of HLA-DRB1*03, *01, and *11 alleles with MS in different populations are presented in Table S2. Among 15 studies where these three alleles were investigated simultaneously (see references in Table S2), positive association with HLA-DRB1*03 was observed in five studies, negative association with HLA-DRB1*01 in seven and with *11 only in three reports. Results of the meta-analysis derived for Caucasians in 2011 revealed the associations of carriage (phenotype) frequencies of HLA-DRB1*03 and *01, but not for *11 with MS, and OR values for *03 and *01 alleles were close to those observed in our study (19). Therefore, our data suggest that Russians share MS-associated HLA-DRB1 *03 and *01 alleles with other Caucasians.
The OR values for HLA-DRB1*15, *03, and *01 were markedly higher in people who are homozygous for these allelic variants in comparison with heterozygotes individuals containing the same alleles (see Figures 1B, D). These data revealed a dose-depended effect not only for risk alleles HLA-DRB1*15 and *03, which was shown earlier (36), but also for the protective allele HLA-DRB1*01. For all genotypes containing one protective and one risk allele, we observed no significant differences in genotype frequencies between MS patients and healthy controls. The small number of persons carrying each of these heterozygous genotypes among patients or healthy individuals (from 5 to 32 persons) do not allow to reach definitive conclusions but estimated OR values close to 1 as well as relatively narrow CIs suggest the mutual compensation of allelic effects in heterozygotes. The most prominent compensatory effect was observed for the genotype HLA-DRB1*01/*15 [OR = 0.94 (CI: 0.47–1.91)]. Although the exact mechanisms by which HLA products encoded by different DRB1 alleles contribute to MS susceptibility are still unknown, the parameters of autoantigen binding to HLA proteins may be the key component determining predisposing or protective effects of HLA allelic variants on MS development.
We have found that the most abundant HLA-DRB1*01:01 allelic variant binds C-terminal and encephalitogenic peptide fragments of MBP with affinity comparable to that of exogenous viral peptides, such as we considered in this work, or even endogenous peptides with high affinity and slow dissociation kinetics (37). Loading of the peptide is mediated by a multistep mechanism including release of the CLIP, which is facilitated by the binding of HLA-DM to HLA-DRB1*01:01; tryptophan 43 of alpha chain of the HLA-DR protein plays an essential role in the binding (12) (Figure 6). Further, P6-P9 pockets are occupied by the C-terminal end of the peptide that facilitates interactions of the N-terminal “head” of the peptide with the P1 and P4 pockets followed by the release of the HLA-DM. These data provide evidence that HLA class II molecules are capable to discriminate kinetically between self- and exogenous peptides during HLA-DM-catalyzed CLIP exchange. Importantly, the affinity of the peptides for the HLA protein are very similar, while the on-rate of their loading onto the HLA are very different. This indicates that the binding kinetics of HLA ligands may be more essential characteristic as opposed to the affinity of the binding and is more relevant physiologically. It should be emphasized that nature proposes at least several other mechanisms to avoid autoimmunity in case of HLAs still capable for self-peptides loading. Molecular dynamics simulation of HLA-DR2—peptide interaction in the absence of DM reveled that protective allele DRB1*16:01 in contrast to the predisposing allele DRB1*15:01 forms more stable complex with the self-peptide in comparison with the viral one. This difference is further reasoned by more tight interaction of the C-terminal part of the self MBP peptide with DRB1*16:01. These data suggest that weak binding of HLA with mimicking viral peptide in case of presence of high affinity self-peptide may serve as a protective factor (38, 39). Recent reports also demonstrate that HLA molecules with high affinity toward self-antigens and associated with autoimmune protection may “steal epitopes” (40) or induce self-epitope specific T regulatory cells (41).
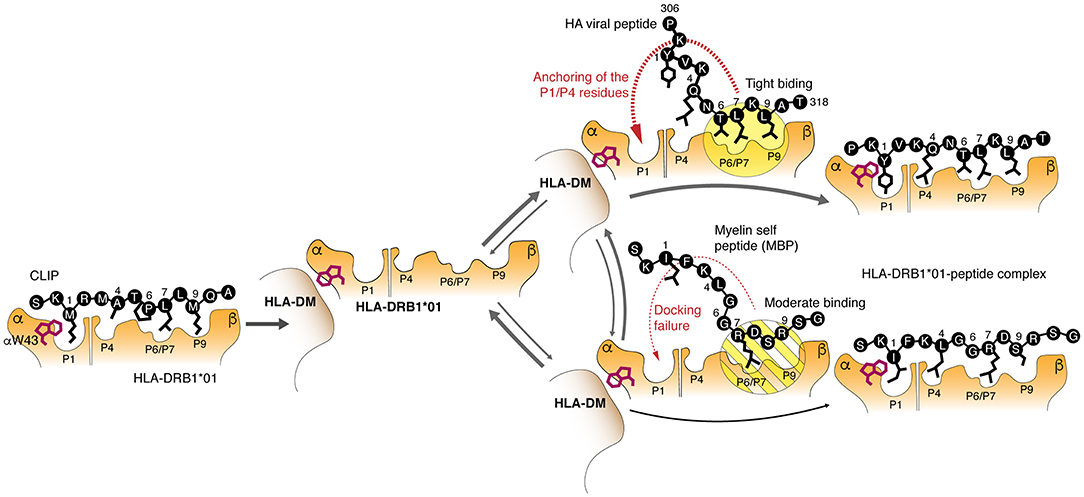
Figure 6. Molecular mechanism of the kinetic selection mediated by the HLA-DM–HLA-DRB1*01:01 complex, which restricts loading of the autoantigenic peptides. Release of the CLIP is followed by assembling of the HLA-DM–HLA-DRB1*01:01 complex, where tryptophan 43 of the HLA α chain is trapped by the HLA-DM. Myelin and exogenous (viral HA as representative example) peptides are competing with each other to bind this bimolecular complex utilizing P6/P7 and P9 residues. Moderate binding of polar P6/P7 and P9 residues in myelin peptides in contrast to the beneficial hydrophobic anchors in the HA peptide does not provide enough time for P1 residue to attack a respective pocket for complete docking.
Overall, the data suggest that the binding of MBP153−161 and MBP88−100 epitopes to HLA-DRB1*01:01 is inefficient, particularly at the first stage, because the P7 pocket is occupied by the basic bulky arginine residue or structurally unfavorable proline, respectively, instead of the highly hydrophobic leucine residue. Therefore, a moderate interaction of the peptide with P6/7 and P9 pockets in contrast to the binding of HA peptide leads to the P1 and P4 docking failure resulting in the peptide dissociation and release of the empty HLA-DM-HLA-DR complex (Figure 6). Because the time for HLA class II loading in the late endosome is restricted to several hours (42), low rate of peptide binding becomes more critical than high affinity of their interactions resulting in the inability to compete with peptides having fast kinetics and even lower affinity. For that reason, the HA peptide completely blocks loading of the myelin peptide onto the HLA-DRB1*01:01 in the equimolar concentration. Thus, it is unlikely that myelin-derived peptides will be presented in the context of the HLA-DRB1*01:01 on the cell surface of the antigen presenting cells at sufficient density required to initiate T cell response. It appears that protective properties of the HLA-DRB1*01 allele may be directly linked to the ability of HLA-DRB1*01:01 to kinetically discriminate between myelin and exogenous peptides. From the opposite side, risk allele HLA-DRB1*15:01 is capable to rapidly present myelin-derived peptides even in competition with exogenous peptides, such as viral pp65109−123. Ex vivo analysis of HLA-associated peptidome from heterozygous HLA-DRB1*01/*15-positive dendritic cells revealed presence of MBP fragments related to the HLA-DRB1*15 but not to HLA-DRB1*01. Even so the observed compensatory effect of protective HLA-DRB1*01 and MS-predisposing *15 alleles that are co-dominantly expressed in heterozygotes may be explained by dispersion of MBP-loaded HLA-DRB1*15 complexes by HLA-DRB1*01 molecules within MHC clusters, occurring in the immunological synapses formed between T cells and antigen presenting cells (43). Indeed, the density of functional MHC molecules within membrane clusters has proved to be an essential factor regulating T cell responses (44).
MS therapeutic glatiramer acetate (GA) or Copaxone is a 40–100 amino acids polypeptide of a random sequence composed from alanine, lysine, glutamate, and tyrosine at a molar ratio of 4.5:3.6:1.5:1, respectively, that with high affinity binds directly to the purified HLA-DR1, -DR2, and -DR4 molecules (45). Combinatorial chemistry, which lays in the basis of GA, may result in assembling of multiple HLA class II epitopes, that at least in part may be not simply thermodynamically but rather kinetically preferable. Concluding our data provide novel vector of optimization of altered peptide ligands in terms of kinetic discrimination of HLA class II antigens.
Future studies should determine if the proposed molecular mechanism of antigenic peptide loading to MHC II suggesting the kinetic discrimination step may have a more general significance in protecting humans from autoimmunity along with the central tolerance established during negative thymic selection of developing T cells.
Ethics Statement
This study was carried out in accordance with the recommendations of local ethics committee of the Moscow Multiple Sclerosis Center. All subjects gave written informed consent in accordance with the Declaration of Helsinki.
Author Contributions
AM, NV, IF, and MZ designed and performed experiments. AM, IK, AF, ISm, and ABe were responsible for statistical analysis and graphic design. AM, OK, OF, AG, and ABe designed the research. IK, VB, and NB performed data collection. MZ, ABo, YS, OF, and AG made intellectual contributions to data analysis, discussion, and coordination of the research team. ABo participated in MS diagnosis and sample collection. RZ performed LC-MS/MS analysis. AP, ISh, and MK performed CFSE proliferation assay. AM, OK, OF, YS, VV, AG, and ABe analyzed data and wrote the manuscript. All authors approved the final manuscript.
Funding
This study was supported by the Russian Science Foundation project #17-74-30019; ISm and YS obtained support from the RFBR-NIH #17-54-30025 (expression and purification of HLA). AF and OF received support from the RFBR-KOMFI #17-00-00295 (HLA genotyping). AG received support from Volkswagen foundation project #3004848 (kinetic analysis).
Conflict of Interest
The authors declare that the research was conducted in the absence of any commercial or financial relationships that could be construed as a potential conflict of interest.
Acknowledgments
We thank Lawrence J. Stern (University of Massachusetts Medical School, Worcester, MA, United States) for critical reading of the manuscript and meaningful discussion, O. Kuprienko (Institute of Bioorganic Chemistry, Minsk, Belarus), I. Mamedov (Institute of Bioorganic Chemistry, Moscow, Russia) and E. Osipova (Dmitry Rogachev National Medical Research Center, Moscow, Russia) for provided reagents, and Dmitriy M. Chudakov (Center for Precision Genome Editing and Genetic Technologies for Biomedicine, Pirogov Russian Medical State University, Moscow, Russia) for the valuable expert advice.
Supplementary Material
The Supplementary Material for this article can be found online at: https://www.frontiersin.org/articles/10.3389/fimmu.2019.03088/full#supplementary-material
References
1. Todd JA, Acha-Orbea H, Bell JI, Chao N, Fronek Z, Jacob CO, et al. A molecular basis for MHC class II–associated autoimmunity. Science. (1988) 240:1003–9. doi: 10.1126/science.3368786
2. Nepom GT, Erlich H. MHC class-II molecules and autoimmunity. Annu Rev Immunol. (1991) 9:493–525. doi: 10.1146/annurev.iy.09.040191.002425
3. Seliger B, Kloor M, Ferrone S. HLA class II antigen-processing pathway in tumors: molecular defects and clinical relevance. Oncoimmunology. (2017) 6:1–10. doi: 10.1080/2162402X.2016.1171447
4. Prentice HA, Tomaras GD, Geraghty DE, Apps R, Fong Y, Ehrenberg PK, et al. HLA class II genes modulate vaccine-induced antibody responses to affect HIV-1 acquisition. Sci Transl Med. (2015) 7:296ra112. doi: 10.1126/scitranslmed.aab4005
5. Huppa JB, Davis MM. T-cell-antigen recognition and the immunological synapse. Nat Rev Immunol. (2003) 3:973–83. doi: 10.1038/nri1245
6. Cresswell P. Assembly, transport, and function of MHC class II molecules. Annu Rev Immunol. (1994) 12:259–93. doi: 10.1146/annurev.iy.12.040194.001355
7. Ghosh P, Amaya M, Mellins E, Wiley DC. The structure of an intermediate in class II MHC maturation: CLIP bound to HLA-DR3. Nature. (1995) 378:457–62. doi: 10.1038/378457a0
8. Jasanoff a, Park SJ, Wiley DC. Direct observation of disordered regions in the major histocompatibility complex class II-associated invariant chain. Proc Natl Acad Sci USA. (1995) 92:9900–4. doi: 10.1073/pnas.92.21.9900
9. Sherman MA, Weber DA, Jensen PE. DM enhances peptide binding to class II MHC by release of invariant chain-derived peptide. Immunity. (1995) 3:197–205. doi: 10.1016/1074-7613(95)90089-6
10. Weber DA, Evavold BD, Jensen PE. Enhanced dissociation of HLA-DR-bound peptides in the presence of HLA-DM. Science. (1996) 274:618–20. doi: 10.1126/science.274.5287.618
11. Roche PA, Furuta K. The ins and outs of MHC class II-mediated antigen processing and presentation. Nat Rev Immunol. (2015) 15:203–16. doi: 10.1038/nri3818
12. Pos W, Sethi DK, Call MJ, Schulze MSED, Anders AK, Pyrdol J, et al. Crystal structure of the HLA-DM-HLA-DR1 complex defines mechanisms for rapid peptide selection. Cell. (2012) 151:1557–68. doi: 10.1016/j.cell.2012.11.025
13. Li Y, Li H, Martin R, Mariuzza RA. Structural basis for the binding of an immunodominant peptide from myelin basic protein in different registers by two HLA-DR2 proteins. J Mol Biol. (2000) 304:177–88. doi: 10.1006/jmbi.2000.4198
14. Smith KJ, Pyrdol J, Gauthier L, Wiley DC, Wucherpfennig KW. Crystal Structure of HLA-DR2 (DRA*0101, DRB1*1501) complexed with a peptide from human myelin basic protein. J Exp Med. (1998) 188:1511–20. doi: 10.1084/jem.188.8.1511
15. Yin Y, Li Y, Mariuzza RA. Structural basis for self-recognition by autoimmune T-cell receptors. Immunol Rev. (2012) 250:32–48. doi: 10.1111/imr.12002
16. Hemmer B, Kerschensteiner M, Korn T. Role of the innate and adaptive immune responses in the course of multiple sclerosis. Lancet Neurol. (2015) 14:406–19. doi: 10.1016/S1474-4422(14)70305-9
17. Sawcer S, Franklin RJM, Ban M. Multiple sclerosis genetics. Lancet Neurol. (2014) 13:700–9. doi: 10.1016/S1474-4422(14)70041-9
18. Canto E, Oksenberg JR. Multiple sclerosis genetics. Mult Scler. (2018) 24:75–9. doi: 10.1177/1352458517737371
19. Zhang Q, Lin CY, Dong Q, Wang J, Wang W. Relationship between HLA-DRB1 polymorphism and susceptibility or resistance to multiple sclerosis in Caucasians: a meta-analysis of non-family-based studies. Autoimmun Rev. (2011) 10:474–81. doi: 10.1016/j.autrev.2011.03.003
20. Ramagopalan SV, Ebers GC. Multiple sclerosis: major histocompatibility complexity and antigen presentation. Genome Med. (2009) 1:1–5. doi: 10.1186/gm105
21. Yin Y, Li Y, Kerzic MC, Martin R, Mariuzza RA. Structure of a TCR with high affinity for self-antigen reveals basis for escape from negative selection. EMBO J. (2011) 30:1137–48. doi: 10.1038/emboj.2011.21
22. Hahn M, Nicholson MJ, Pyrdol J, Wucherpfennig KW. Unconventional topology of self peptide-major histocompatibility complex binding by a human autoimmune T cell receptor. Nat Immunol. (2005) 6:490–6. doi: 10.1038/ni1187
23. Li Y, Huang Y, Lue J, Quandt JA, Martin R, Mariuzza RA. Structure of a human autoimmune TCR bound to a myelin basic protein self-peptide and a multiple sclerosis-associated MHC class II molecule. EMBO J. (2005) 24:2968–79. doi: 10.1038/sj.emboj.7600771
24. Polman CH, Reingold SC, Banwell B, Clanet M, Cohen JA, Filippi M, et al. Diagnostic criteria for multiple sclerosis: 2010 revisions to the McDonald criteria. Ann Neurol. (2011) 69:292–302. doi: 10.1002/ana.22366
25. Sreevalsan T. Isolation of dendritic cells from human blood for in vitro interaction studies with fungal antigens. Methods Mol Biol. (2009) 499:1–8. doi: 10.1007/978-1-60327-151-6_1
26. Markov OV, Mironova NL, Shmendel EV, Serikov RN, Morozova NG, Maslov MA, et al. Multicomponent mannose-containing liposomes efficiently deliver RNA in murine immature dendritic cells and provide productive anti-tumour response in murine melanoma model. J Control Release. (2015) 213:45–56. doi: 10.1016/j.jconrel.2015.06.028
27. Rappsilber J, Mann M, Ishihama Y. Protocol for micro-purification, enrichment, pre-fractionation and storage of peptides for proteomics using StageTips. Nat Protoc. (2007) 2:1896–906. doi: 10.1038/nprot.2007.261
28. Ma B, Zhang K, Hendrie C, Liang C, Li M, Doherty-Kirby A, Lajoie G. PEAKS: Powerful software for peptide de novo sequencing by tandem mass spectrometry. Rapid Commun Mass Spectrom. (2003) 17:2337–42. doi: 10.1002/rcm.1196
29. Mamedov AE, Ponomarenko NA, Belogurov AA, Gabibov AG. Erratum to: heterodimer HLA-DM fused with constant fragment of the heavy chain of the human immunoglobulin accelerates influenza hemagglutinin peptide HA306-318 loading to HLA-DR1. Bull Exp Biol Med. (2016) 161:442–6. doi: 10.1007/s10517-016-3353-y
30. Belogurov AA, Kurkova IN, Friboulet A, Thomas D, Misikov VK, Zakharova MY, et al. Recognition and degradation of myelin basic protein peptides by serum autoantibodies: novel biomarker for multiple sclerosis. J Immunol. (2008) 180:1258–67. doi: 10.4049/jimmunol.180.2.1258
31. Stern LJ, Brown JH, Jardetzky TS, Gorga JC, Urban RG, Strominger JL, et al. Crystal structure of the human class II MHC protein HLA-DR1 complexed with an influenza virus peptide. Nature. (1994) 368:215–21. doi: 10.1038/368215a0
32. Anders AK, Call MJ, Schulze MS, Fowler KD, Schubert DA, Seth NP, et al. HLA-DM captures partially empty HLA-DR molecules for catalyzed removal of peptide. Nat Immunol. (2011) 12:54–61. doi: 10.1038/ni.1967
33. Yin L, Maben ZJ, Becerra A, Stern LJ. Evaluating the role of HLA-DM in MHC Class II–peptide association reactions. J Immunol. (2015) 195:706–16. doi: 10.4049/jimmunol.1403190
34. Muixi L, Carrascal M, Alvarez I, Daura X, Marti M, Armengol MP, et al. Thyroglobulin peptides associate in vivo to HLA-DR in autoimmune thyroid glands. J Immunol. (2008) 181:795–807. doi: 10.4049/jimmunol.181.1.795
35. Sudomoina MA, Boiko AN, Demina TL, Gusev EI, Boldyreva MN, Trofimov DY, et al. Association of multiple sclerosis in the Russian population with HLA-DRB1 gene alleles. Mol Biol. (1998) 32:255–60.
36. Barcellos LF, Sawcer S, Ramsay PP, Baranzini SE, Thomson G, Briggs F, et al. Heterogeneity at the HLA-DRB1 locus and risk for multiple sclerosis. Hum Mol Genet. (2006) 15:2813–24. doi: 10.1093/hmg/ddl223
37. Yin L, Trenh P, Guce A, Wieczorek M, Lange S, Sticht J, et al. Susceptibility to HLA-DM protein is determined by a dynamic conformation of major histocompatibility complex class II molecule bound with peptide. J Biol Chem. (2014) 289:23449–64. doi: 10.1074/jbc.M114.585539
38. Kumar A, Cocco E, Atzori L, Marrosu MG, Pieroni E. Structural and dynamical insights on HLA-DR2 complexes that confer susceptibility to multiple sclerosis in sardinia: a molecular dynamics simulation study. PLoS ONE. (2013) 8:e59711. doi: 10.1371/journal.pone.0059711
39. Kumar A, Melis P, Genna V, Cocco E, Marrosu MG, Pieroni E. Antigenic peptide molecular recognition by the DRB1-DQB1 haplotype modulates multiple sclerosis susceptibility. Mol Biosyst. (2014) 10:2043–54. doi: 10.1039/C4MB00203B
40. van Lummel M, Buis DTP, Ringeling C, de Ru AH, Pool J, Papadopoulos GK, et al. Epitope stealing as a mechanism of dominant protection by HLA-DQ6 in type 1 diabetes. Diabetes. (2019) 68:787–95 doi: 10.2337/db18-0501
41. Ooi JD, Petersen J, Tan YH, Huynh M, Willett ZJ, Ramarathinam SH, et al. Dominant protection from HLA-linked autoimmunity by antigen-specific regulatory T cells. Nature. (2017) 545:243–7. doi: 10.1038/nature22329
42. Rabinowitz JD, Vrljic M, Kasson PM, Liang MN, Busch R, Boniface JJ, et al. Formation of a highly peptide-receptive state of class II MHC. Immunity. (1998) 9:699–709. doi: 10.1016/S1074-7613(00)80667-6
43. Vogt AB, Spindeldreher S, Kropshofer H. Clustering of MHC-peptide complexes prior to their engagement in the immunological synapse: lipid raft and tetraspan microdomains. Immunol Rev. (2002) 189:136–51. doi: 10.1034/j.1600-065X.2002.18912.x
44. Anikeeva N, Gakamsky D, Schøller J, Sykulev Y. Evidence that the density of self peptide-MHC ligands regulates T-cell receptor signaling. PLoS ONE. (2012) 7:e41466. doi: 10.1371/journal.pone.0041466
Keywords: myelin basic protein, multiple sclerosis, human leukocyte antigen, protective allele, epitope library, hemagglutinin, genetic predisposition to disease
Citation: Mamedov A, Vorobyeva N, Filimonova I, Zakharova M, Kiselev I, Bashinskaya V, Baulina N, Boyko A, Favorov A, Kulakova O, Ziganshin R, Smirnov I, Poroshina A, Shilovskiy I, Khaitov M, Sykulev Y, Favorova O, Vlassov V, Gabibov A and Belogurov A Jr (2020) Protective Allele for Multiple Sclerosis HLA-DRB1*01:01 Provides Kinetic Discrimination of Myelin and Exogenous Antigenic Peptides. Front. Immunol. 10:3088. doi: 10.3389/fimmu.2019.03088
Received: 18 May 2018; Accepted: 17 December 2019;
Published: 17 January 2020.
Edited by:
Fabienne Brilot, University of Sydney, AustraliaReviewed by:
Rudolf Valenta, Medical University of Vienna, AustriaEddie A. James, Benaroya Research Institute, United States
Maria Giovanna Marrosu, University of Cagliari, Italy
Copyright © 2020 Mamedov, Vorobyeva, Filimonova, Zakharova, Kiselev, Bashinskaya, Baulina, Boyko, Favorov, Kulakova, Ziganshin, Smirnov, Poroshina, Shilovskiy, Khaitov, Sykulev, Favorova, Vlassov, Gabibov and Belogurov. This is an open-access article distributed under the terms of the Creative Commons Attribution License (CC BY). The use, distribution or reproduction in other forums is permitted, provided the original author(s) and the copyright owner(s) are credited and that the original publication in this journal is cited, in accordance with accepted academic practice. No use, distribution or reproduction is permitted which does not comply with these terms.
*Correspondence: Alexander Gabibov, Z2FiaWJvdkBpYmNoLnJ1; Alexey Belogurov Jr., YmVsb2d1cm92QGliY2gucnU=