- 1Immunology Program, Stanford University School of Medicine, Stanford, CA, United States
- 2Department of Medicine, Stanford University School of Medicine, Stanford, CA, United States
- 3Department of Statistics, Stanford University School of Medicine, Stanford, CA, United States
- 4Chan Zuckerberg Biohub, San Francisco, CA, United States
Natural killer (NK) cells are the predominant antiviral cells of the innate immune system, and may play an important role in acquisition and disease progression of HIV. While untreated HIV infection is associated with distinct alterations in the peripheral blood NK cell repertoire, less is known about how NK phenotype is altered in the setting of long-term viral suppression with antiretroviral therapy (ART), as well as how NK memory can impact functional responses. As such, we sought to identify changes in NK cell phenotype and function using high-dimensional mass cytometry to simultaneously analyze both surface and functional marker expression of peripheral blood NK cells in a cohort of ART-suppressed, HIV+ patients and HIV- healthy controls. We found that the NK cell repertoire following IL-2 treatment was altered in individuals with treated HIV infection compared to healthy controls, with increased expression of markers including NKG2C and CD2, and decreased expression of CD244 and NKp30. Using co-culture assays with autologous, in vitro HIV-infected CD4 T cells, we identified a subset of NK cells with enhanced responsiveness to HIV-1-infected cells, but no differences in the magnitude of anti-HIV NK cell responses between the HIV+ and HIV− groups. In addition, by profiling of NK cell receptors on responding cells, we found similar phenotypes of HIV-responsive NK cell subsets in both groups. Lastly, we identified clusters of NK cells that are altered in individuals with treated HIV infection compared to healthy controls, but found that these clusters are distinct from those that respond to HIV in vitro. As such, we conclude that while chronic, treated HIV infection induces a reshaping of the IL-2-stimulated peripheral blood NK cell repertoire, it does so in a way that does not make the repertoire more HIV-specific.
Introduction
Natural killer (NK) cells are critical effector cells of the innate immune system that can rapidly recognize and kill virally infected and tumor cells. NK cells express an array of activating and inhibitory receptors; the integration of signaling between these receptors determines NK cell activation and functional activity. This includes the release of cytolytic granules to induce target cell apoptosis, as well as the secretion of cytokines and chemokines including IFN-γ, MIP-1β (CCL4), and TNF-α.
Epidemiological and experimental evidence have highlighted the role of NK cells in the acquisition and disease progression of HIV-1. Increased constitutive NK cell activity is associated with protection from HIV acquisition in highly exposed seronegative individuals (1, 2). Similarly, the expression of the NK cell receptor KIR3DL1 and its cognate ligand HLA-Bw4-80I is associated with slower disease progression and improved suppression of autologous HIV-infected CD4 T cells (3–5). Specific NK cell receptors have also been implicated in HIV recognition and targeting. NKG2A-expressing NK cells have improved activity against HIV (6–8), and NKG2D ligands are upregulated on HIV-infected cells (9). As such, changes in expression of NK cell receptors can impact their ability to target HIV.
Chronic, untreated HIV infection is associated with significant changes in the NK cell repertoire, the most well-defined of which is the loss of CD56+ NK cells, and the concomitant expansion of a CD56neg NK cell subset (10). CD56neg NK cells are functionally impaired and thought to be exhausted, demonstrating reduced cytotoxicity and IFN-γ production (11–13). In addition, the expression of the inhibitory receptor Siglec-7 (14), as well as the expression of the activating receptors NKp30, NKp44 and NKp46 (15), are decreased in chronic, viremic HIV infection, whereas the expression of the inhibitory receptor TIGIT is increased (16, 17). After treatment with antiretroviral therapy (ART), the patterns of CD56+ and CD56neg NK cell subsets are restored to levels similar to seronegative, healthy individuals (12). However, less is known regarding how other NK cell subsets, as well as how the NK cell repertoire as a whole, may be altered in the setting of virological control by ART. In addition, the functional outcomes of these alterations, in particular with regards to how they may impact HIV-specific responses, are not well understood.
Contrary to their classic designation as an innate immune cell type, recent work has demonstrated the ability of human NK cells to form memory against viruses including cytomegalovirus, Epstein-Barr virus and varicella-zoster virus (18–24). In non-human primates, infection with simian immunodeficiency virus (SIV) or SHIV generates antigen-specific NK cells that react with presented Gag and Env. In addition, vaccination with Ad26 vectors containing Gag and Env antigens from HIV and SIV generates long-lived, antigen-specific NK cells, even in the absence of continuous antigen stimulation (25), raising the possibility that human NK cells in infected individuals could be similarly capable of generating and retaining memory responses against HIV antigens even without ongoing viral exposure. As such, we sought to understand whether previous HIV infection altered the functional capacity of peripheral blood NK cells to respond against a second, in vitro stimulation with autologous HIV-infected cells. Here, we use mass cytometry to profile NK cell receptor expression on a cohort of ART-suppressed, HIV + donors and healthy controls, to determine how changes in the NK cell repertoire that occur with HIV infection influence HIV-specific NK cell responses.
Materials and Methods
Study Subjects and Sample Processing
Cryopreserved peripheral blood mononuclear cells (PBMCs) from HIV-infected patients treated with antiretroviral therapy (ART) were obtained from the Stanford HIV Aging Cohort. This study was approved by the Institutional Review Board of Stanford University. For anonymous healthy HIV uninfected donors, leukoreduction system chambers were obtained from the Stanford Blood Bank. PBMCs were isolated by density gradient centrifugation using Ficoll-Paque PLUS (GE Healthcare), and cryopreserved in 10% DMSO (Sigma Aldrich) and 90% fetal bovine serum (FBS, Thermo Fisher).
CD4 and NK Cell Sorting and Cell Culture
Peripheral blood mononuclear cells were thawed, and stained with a panel consisting of 7-AAD viability staining solution (eBioscience), CD14-BV421 (clone M5E2), CD19-BV421 (clone HIB19), CD16-FITC (clone 3G8), CD3-PE (clone SK7), CD4-BV711 (clone OKT4), and CD56-PE Cy7 (clone HCD56, all antibodies from Biolegend), and sorted for CD4 T cells (CD14– CD19– CD3+ CD4+) and NK cells (CD14– CD19– CD3– CD56/CD16+) using a Sony SH800 sorter. Post-sorting, all cells were cultured in RPMI (Gibco), with 10% FBS (Thermo Fisher), 1% L-glutamine (Hyclone) and 1% penicillin/streptomycin/amphotericin (Thermo Fisher) (RP10). CD4 T cells were plated in RP10 with plate-bound anti-CD3 (clone OKT3, eBioscience), anti-CD28/CD49d (BD Biosciences) and PHA-L (eBioscience) for 48 h. NK cells were separately plated in RP10 with 300 IU/ml recombinant human IL-2 (R&D) for 72 h.
In vitro HIV Infection and NK Co-culture Assays
For all in vitro HIV infections, Q23-FL, a clone from early, subtype A infection (26), was used. The Q23-FL virus was produced by transfecting a plasmid encoding a full-length, replication competent clone into 293T cells, harvesting supernatant after 48 h and concentrating by ultracentrifugation. Viral stocks were titrated on TZM-bl cells as previously described (27). Activated CD4 T cells were infected with Q23-FL at an MOI of 25 (based on titrations in TZM-bl cells), using ViroMag magnetofection (OZ Biosciences). HIV-infected cells were used for co-cultures 24 h post infection. NK cells and CD4 T cells were co-cultured at a 1:4 effector:target (E:T) ratio, for 4 h, in the presence of brefeldin A (eBioscience), monensin (eBioscience), and anti-CD107a-APC (Biolegend).
Mass Cytometry
All antibodies were conjugated using MaxPar X8 labeling kits (Fluidigm), except for those purchased directly from Fluidigm; details of all antibodies is given in Supplementary Table S1. To maintain antibody stability and consistency in staining, all antibody panels were pre-mixed into separate surface and ICS cocktails (as indicated in Supplementary Table S1), aliquoted and frozen at −80°C until use. Palladium (Pd102, Pd104, Pd106, and Pd108) conjugated CD45 antibodies for barcoding were made as previously described (28).
At the end of co-culture, cells were stained for viability using 25 μM Cisplatin (Enzo) for 1 min and quenched with FBS, and samples were barcoded using palladium-based CD45 barcodes as previously described (28). After barcoding, cells were washed thrice, and all samples from a set of barcodes were combined. Samples were stained with the surface antibody panel for 30 min at 4°C, fixed with 2% paraformaldehyde (PFA, Electron Microscopy Sciences), permeabilized with Permeabilization Buffer (eBioscience), and stained with the intracellular staining (ICS) panel (made in Permeabilization Buffer) for 45 min at 4°C. Cells were suspended overnight in iridium interchelator (DVS Sciences) in 2% PFA, and resuspended in 1x EQ Beads (Fluidigm) before acquisition on a Helios mass cytometer (Fluidigm).
Data Analysis
Bead normalization1 and debarcoding (using the R package Premessa) were performed on all files post-acquisition. All CyTOF data was visualized and gated using FlowJo v10.1 (Tree Star); gated NK cells (CD3– CD56/CD16+), or functional+/functional– cells were exported as fcs files from FlowJo and used in downstream analyses. CD11a was excluded from all downstream analyses due to poor staining. The data supporting this publication is available at ImmPort2 under study accession SDY1620.
The open source statistical software (29) was used for analyses. For analyses using the generalized linear models as well as the clustering and the Uniform Manifold Approximation and Projection (UMAP) (30), raw channel values were transformed using the inverse hyperbolic sine (asinh) function with a cofactor equal to 5 to account for heteroskedasticity. This transformation was not applied for calculating mean signal intensity values. To compare frequencies of functionally responding cells, as well as frequency of the gated population between HIV+ and HIV− groups, t-tests were used. To compare frequencies of responding cells between the gated population and bulk NK cells in each donor, paired t-tests were used. To identify markers that are predictors of the HIV+ or HIV− conditions, we used the R package CytoGLMM (31) which uses a generalized linear model with bootstrap resampling. This model takes into account the distribution of each marker, and has a donor-specific variable to control for inter-individual variability. For the clustering analyses, the R package CATALYST was used (32, 33). This package provides a clustering method which combines the FlowSOM algorithm (34) which generates 100 high-resolution clusters, followed by the ConsensusClusterPlus metaclustering algorithm (35) which regroups these high-resolution clusters into metaclusters. Default parameters were used for clustering, and the number of metaclusters (10) was selected based on the delta area plot provided. To test for differential abundance of clusters between groups, the diffcyt-DA-GLMM method from the diffcyt package (32, 33) was used; the donor IDs were specified as a random effect. The UMAP was run using the scater package (36), with default settings.
Results
HIV+ Donors Have an Altered NK Cell Repertoire Even in the Setting of ART Suppression
To investigate the effect of HIV-1 infection on NK cell responses, we used CyTOF to profile NK cell receptor expression and functional activity in a cohort of 10 ART-suppressed, HIV+ donors (referred to as HIV+), and 10 healthy controls (referred to as HIV−). Patient demographics are given in Table 1. We first compared the expression of 28 NK cell receptors between the HIV+ and HIV− donors, in sorted, IL-2 activated NK cells. To look at overall NK receptor expression patterns between the two groups, we used a multidimensional scaling (MDS) plot to visualize all the NK cell samples (Figure 1A). NK cells from HIV− and HIV+ donors separated primarily on a diagonal axis; this separation was driven by multiple markers including CD2, NKp30 and NKp46 (as shown in the correlation circle). To further define the NK cell receptors whose expression is altered in HIV+ compared to HIV- individuals, we used a generalized linear model with bootstrap resampling to identify markers predictive of either the HIV+ or HIV− groups. Based on marker distribution, the model generates the log-odds that the expression of a given marker is predictive of either the HIV+ or HIV− group, together with the 95% confidence interval. In purified, IL-2 activated NK cells, the markers NKG2C, CD2, NKp46, and PD-1 were predictive of HIV+ (95% confidence interval does not contain the zero value) while CD244, NKp30, DNAM-1, and NKG2A were predictive of HIV− individuals (Figure 1B). To confirm these results, we also compared mean signal intensity (MSI) for the top 4 NK cell markers predictive of either the HIV− or HIV+ groups, and observed an increased trend of MSI for NKG2C, CD2, NKp46 and PD-1 in the HIV+ group, and a decreased trend in CD244, NKp30, DNAM-1, and NKG2A (Figure 1C).
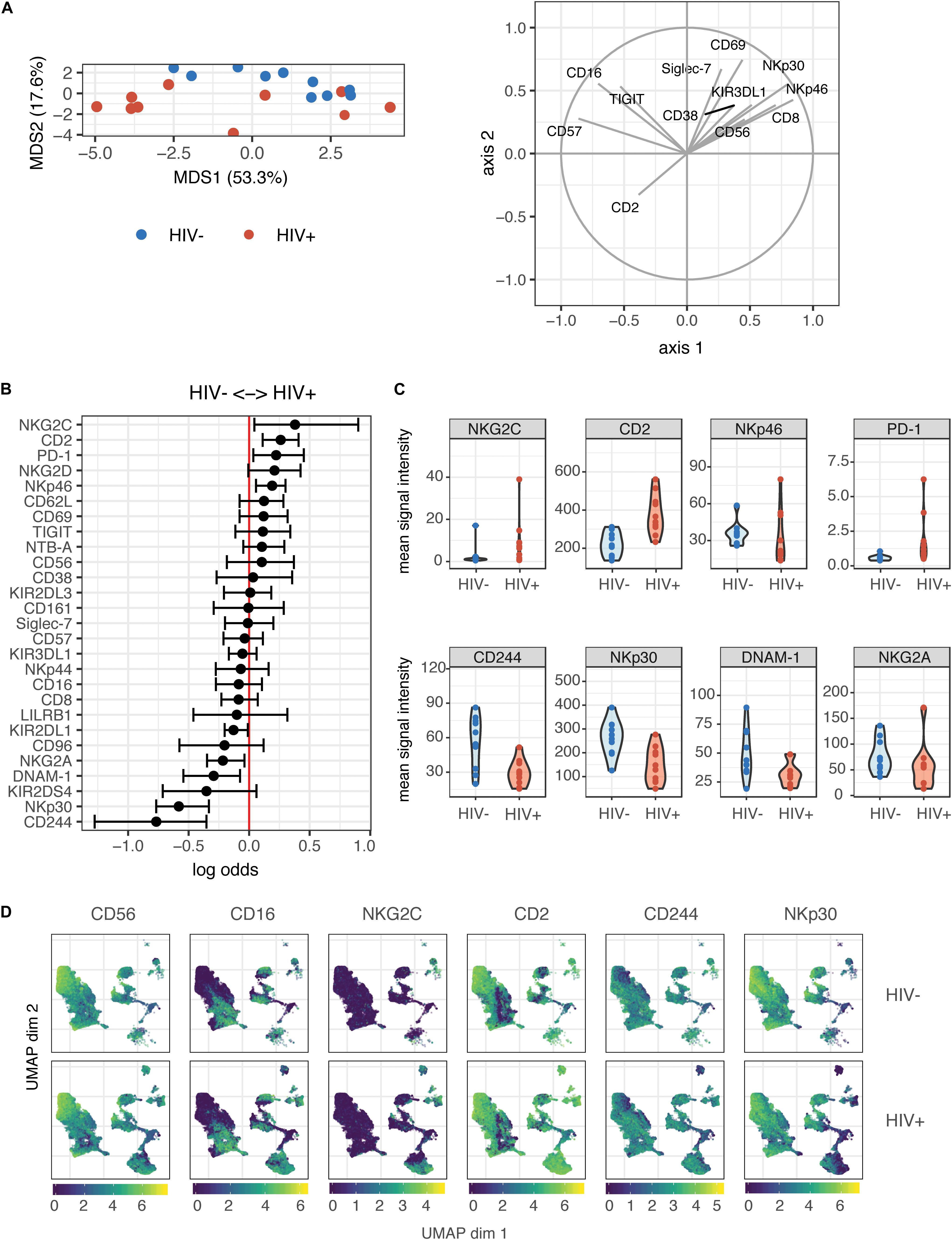
Figure 1. Profiling of NK cell repertoire using CyTOF in both healthy, HIV– and ART-suppressed, HIV+ donors demonstrates alterations in NK cell surface receptor expression. (A) Multidimensional scaling (MDS) plot showing separation of NK cells from HIV+ (n = 10) and HIV– donors (n = 10). Only markers whose contributions are greater than 0.25 in both MDS1 and MDS2 are displayed in the marker loadings. (B) A generalized linear model with bootstrap resampling was used to find receptors predictive of either HIV+ (right), or HIV– (left) donor NK cells. Log-odds are logarithm of ratios of the probability that a cell belongs to either group. For each marker, the 95% confidence interval is represented by the line surrounding the point estimate; a larger absolute log-odds value of the parameter indicates that the marker is a stronger predictor. (C) Mean signal intensity (MSI) of NKG2C, CD2, NKp46, and PD-1 (top 4 predictors of the HIV+ group; top), and CD244, NKp30, DNAM-1, and NKG2A (top 4 predictors of the HIV– group; bottom). (D) UMAP visualization of all NK cells from the HIV+ and HIV– groups, colored by expression of CD56, CD16, NKG2C, and CD2 (top 2 predictors of the HIV+ group), and CD244 and NKp30 (top 2 predictors of the HIV– group). Scales show asinh-transformed channel values.
To further visualize the changes in subsets of NK cells between HIV+ and HIV− groups, we used the Uniform Manifold Approximation and Projection (UMAP) to visualize purified NK cells from both groups (Figure 1D). To identify classic NK cell subsets, the expression of the markers CD56 and CD16 are shown, revealing the expected pattern in that the cells with highest expression of CD56 have low expression of CD16, identifying the canonical CD56brightCD16– and CD56dimCD16+ NK cell subsets. In addition, the expression of the top predictors for each of the HIV− (CD244 and NKp30) and HIV+ groups (NKG2C and CD2) are shown. These plots reveal that greatest differences in both cellular distribution and NK marker expression between HIV− and HIV + donors occurred on the right part of the UMAP plots. Thus, these data demonstrate that even in the setting of long-term virological suppression with ART, the NK cell repertoire following IL-2 treatment remains altered.
NK Cells From HIV+ Individuals Do Not Have an Increased Response Upon in vitro Restimulation With Autologous HIV-Infected Cells
Contrary to their classic designation as innate immune cells, NK cells have more recently been shown to demonstrate antigen-specific memory to viral antigens. As such, we were interested in determining whether prior HIV-1 exposure (in the HIV+ individuals) would alter the magnitude of the NK cell response or the phenotypes of responding cells, when restimulated with autologous HIV-infected cells in vitro. We co-cultured NK cells from HIV− and HIV+ donors with autologous CD4 T cells infected in vitro with the HIV strain Q23-FL (Figure 2A); infection levels in CD4 T cells after co-culture were similar between HIV+ and HIV− donors (Supplementary Figure S1). To identify NK cell responses, we looked at expression of functional markers on these cells by CyTOF. For each sample, we separately gated on NK cells that were positive for the expression of cytokines IFN-γ, MIP-1β (CCL4), TNF-α or the degranulation marker CD107a (Figure 2B), after 4 h of co-culture. All gating was performed based on samples of NK cells in the absence of target cells; these samples had generally low levels of expression of all functional markers. We found that the majority of responding cells were polyfunctional: for example, using the generalized linear model, the top predictors of CD107a+ cells included MIP-1β, TNF-α, and IFN-γ (Supplementary Figure S2). As such, we used Boolean gating to identify functionally responding cells (positive for any of the functional markers above, hereafter named as functional+), or non-functionally responding cells (negative for all of the markers above, named as functional–).
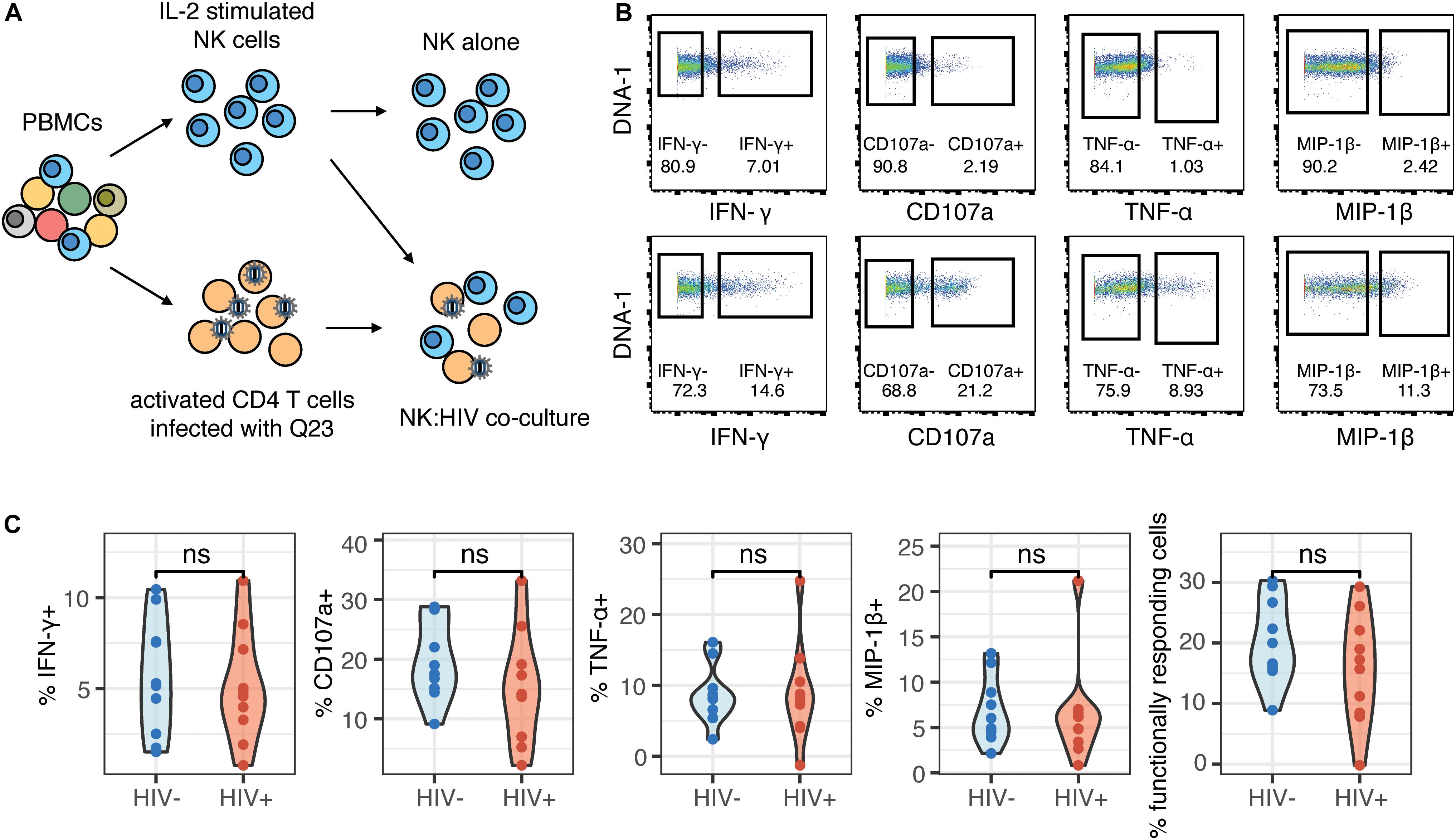
Figure 2. The magnitude of the HIV-specific response does not differ between HIV- and HIV+ donors. (A) Schematic of experimental set-up for NK co-culture assays. (B) Representative flow cytometry plots of CD107a, IFN-γ, MIP-1β, and TNF-α production (by frequency of positive cells), after 4 h co-culture without (top) or in the presence of HIV-infected autologous CD4+ T cells (bottom). (C) Summary data of CD107a, IFN-γ, MIP-1β and TNF-α production (by frequency of positive cells), after 4 h co-culture with HIV-infected autologous CD4+ T cells, in NK cells from HIV– (n = 10) and HIV+ (n = 10) donors. ns, not significant, by t-test.
To identify differences in the magnitude of NK cell responses to HIV-infected cells between HIV− and HIV+ donors, we gated on cells that were positive for functional markers, and sought to identify differences in frequency of these cells between the two groups. We applied background subtraction (by subtracting the frequency of NK cells expressing each functional marker in the NK alone condition) to account for variations in baseline NK activity. In response to autologous HIV-infected cells in vitro, the frequency of NK cells expressing any of the functional markers (IFN-γ, MIP-1β, TNF-α, CD107a) individually, or combined (functional+), was not significantly different between the HIV− and HIV+ groups (Figure 2C).
To further understand whether the NK cells that were generating a functional response were phenotypically similar between the HIV+ and HIV− groups, we used the generalized linear model to identify predictors of responding (functional+) and non-responding cells (functional–). Multiple NK cell receptors were strong predictors of functional+ or functional– cells, indicating a clearly distinct phenotype of cells that respond to HIV in vitro; these cells express higher levels of CD96, NKp30, TIGIT, and Siglec-7, and lower levels of CD62L, CD16, and NKp46 (Figure 3A). Notably, the top predictors of responding cells were very similar between the HIV- and the HIV+ group, including CD96, NKp30, and TIGIT. To identify a subset of responding cells, we included cells with high expression of positive predictors (predictors of functional+), and with negative expression of negative predictors (predictors of functional–), that had a log odds greater than 0.2 in both groups; the resultant phenotype was CD96hi NKp30hi TIGIThi CD16– CD62L– NKp46–. CD38, while being a strong predictor of functional+ cells in the HIV+ group, was excluded; it was not a predictor of responding cells in the HIV− group, and CD38 expression is increased in the context of HIV infection and remains high even after ART treatment (37). We gated on each marker individually (Figure 3B), and then used Boolean gating to generate a gated population that included all the features of the phenotype. Gating on this subset significantly enriched for functional+ cells to a similar extent in both the HIV+ and HIV− groups (Figure 3C), suggesting that, despite the alterations in NK cell repertoire, the NK cell subsets responding to HIV in vitro were not altered in HIV+ individuals. We also compared the frequency of this subset among total NK cells, in both HIV+ and HIV− groups (Figure 3D), and noted that, while the frequency was low (between 0.1 and 3%), there was no significant difference between the two groups, indicating that HIV infection does not alter the frequency of this subset.
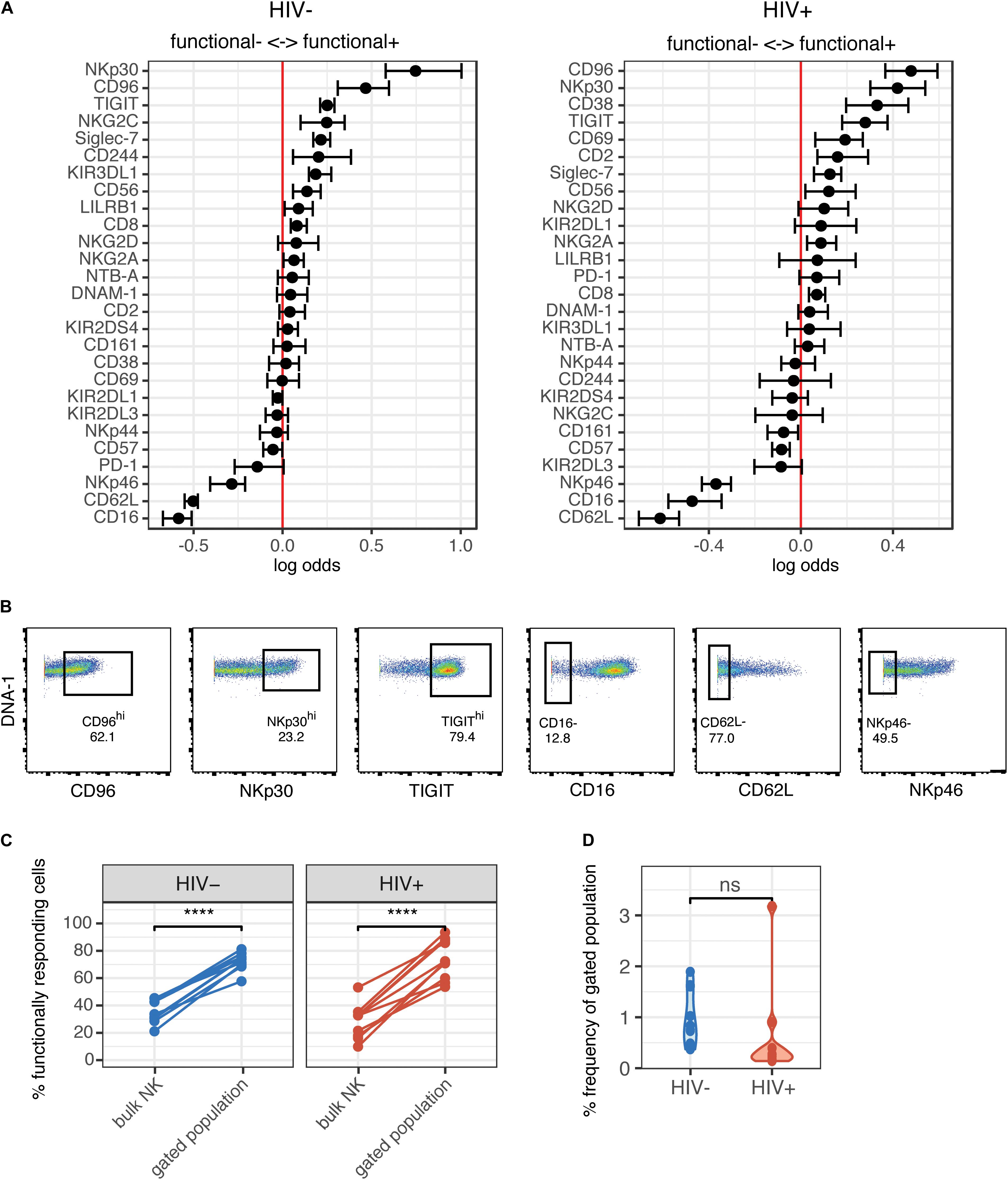
Figure 3. Phenotype of NK cells responding to HIV are similar between HIV- and HIV+ donors, and include many of the markers that are altered in ART-treated HIV infection. (A) A generalized linear model with bootstrap resampling was used to identify NK cell receptors predictive of either functionally responding (right), or non-responding (left) NK cells, in HIV– (n = 10) and HIV+ (n = 10) donors. Log-odds are logarithm of ratios of the probability that a cell belongs to either group. For each marker, the 95% confidence interval is represented by the line surrounding the point estimate; a larger absolute log-odds value of the parameter indicates that the marker is a stronger predictor. (B) Gating on markers that predict functionally responding cells (NKp30hi CD96hi TIGIThi CD62L- CD16- NKp46-), with a minimum log-odds threshold of 0.2 in both groups. (C) Comparison of functional activity in the gated population, compared to bulk NK cells. Summary data of percentage positive functionally responding (positive for any of CD107a, IFN-γ, MIP-1β, and TNF-α) in HIV– (n = 10) and HIV + groups (n = 10). ****p < 0.001, by paired t-test. (D) Comparison of the frequency of the gated population, as a percentage of total NK cells, between the HIV– (n = 10) and HIV+ (n = 10) groups. ns, not significant, by t-test.
NK Cells Clusters That Are Altered in HIV+ Individuals Are Distinct From Those That Are Functionally Responsive to HIV in vitro
As the expression of many of the markers on responding cells were markers we found to be altered in the HIV+ group compared to HIV− (Figure 1), it was surprising that these changes did not seem to have an effect on the magnitude or quality of the NK cell response to HIV in vitro. To better understand the populations of cells that are altered in HIV+ individuals, compared to those that are involved in the functional response, we used UMAP to visualize all NK cells from co-cultures with HIV-infected cells in vitro, in both groups. We also performed unsupervised clustering and metaclustering, using the FlowSOM and ConsensusClusterPlus algorithms, to identify 10 metaclusters of NK cells (Figure 4A); this included clusters that were shared between HIV+ and HIV− groups, such as cluster 1, but also clusters that were distinct to NK cells from HIV+ individuals (cluster 3), or HIV- individuals (cluster 5). To identify responding NK cells, we overlaid marker expression of functional markers onto the UMAP visualization (Figure 4B). All responding NK cells (positive for functional markers) clustered in a similar area on the left part of the plots that was shared between HIV+ and HIV− donors; many of these cells are positive for multiple functional markers, confirming that most responding cells were polyfunctional (Supplementary Figure S1). To identify which cluster these functionally responding cells belonged to, we looked at a heatmap of mean marker expression of all NK cell markers across all clusters (Figure 4C); this identified clusters 9 and 10 as the predominant clusters expressing functional markers, with cells in cluster 9 expressing high levels of CD107a, TNF-α, and MIP-1β, and cells in cluster 10 also expressing high levels of IFN-γ. In line with our previous analysis of the phenotypes of functionally responding cells, cluster 9 expresses high levels of NKp30, while cluster 10 has relatively high levels of TIGIT expression and low levels of NKp46 expression; both clusters have low CD16 and CD62L expression.
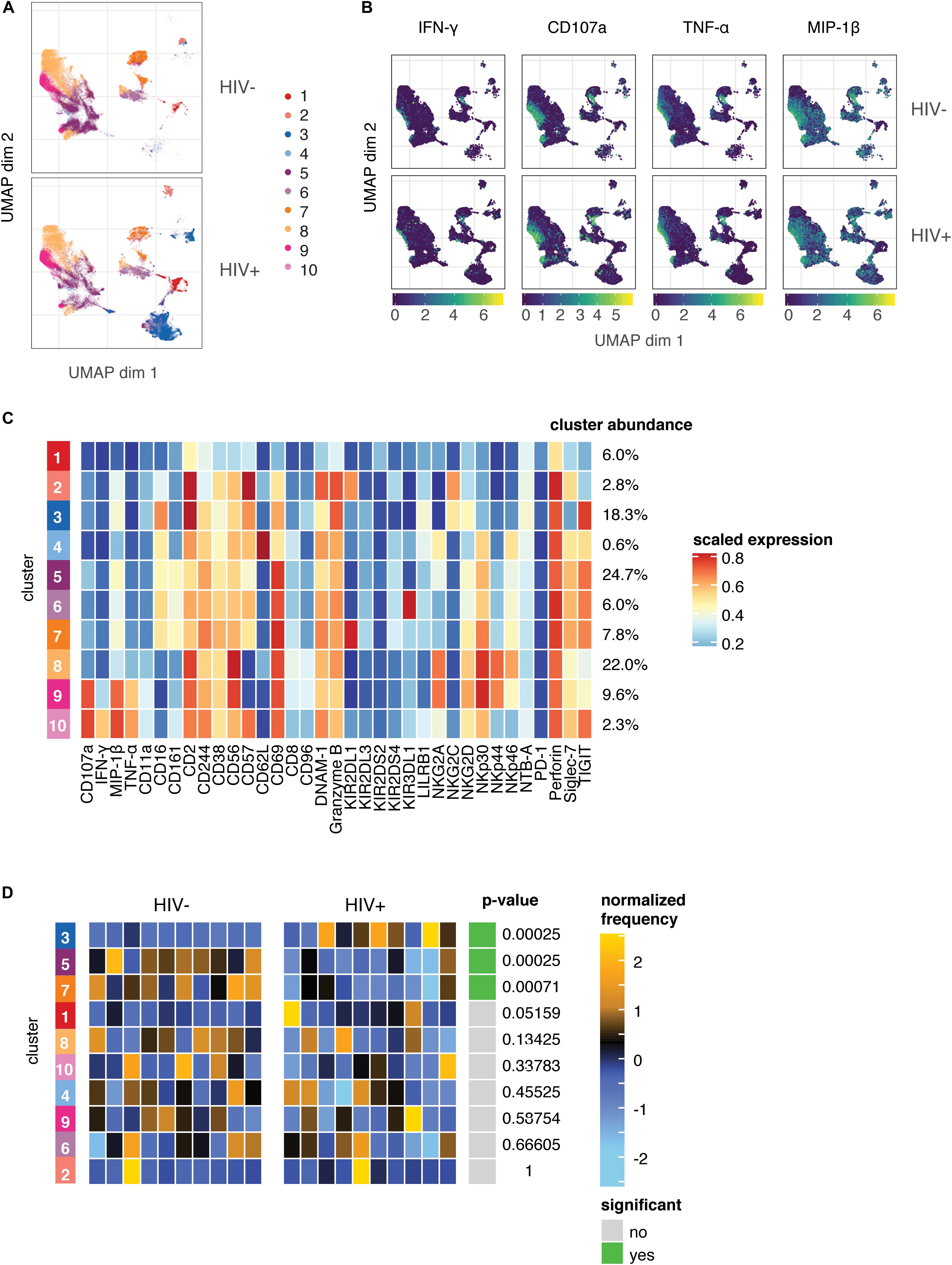
Figure 4. The predominant changes in the NK cell repertoire of HIV+ individuals occur in NK cell compartments that do not respond to HIV in in vitro restimulation. (A) UMAP visualization of all NK cells in co-culture with autologous HIV-infected cells in HIV– (n = 10) and HIV+ (n = 10) donors, colored by metacluster identity generated by ConsensusClusterPlus metaclustering. (B) UMAP visualization of all NK cells from the HIV+ and HIV– groups, colored by expression of functional markers CD107a, IFN-γ, MIP-1β, and TNF-α. Scales show asinh-transformed channel values. (C) Heatmap of scaled mean expression of all markers profiled, for each cluster 1 to 10. The abundance of each cluster (% of total cells) is given on the right of the heatmap. Functional markers (CD107a, IFN-γ, MIP-1β, and TNF-α) are on the left. (D) Heatmap of the relative abundance of each cluster between the HIV- (left) and HIV+ (right) groups. Each individual column represents a single donor. The heat represents the proportion of each metacluster in each donor, with yellow showing over-representation and blue showing under-representation. These proportions were first scaled with an arcsine-square-root transformation and then z-score normalized in each cluster. Clusters with a statistically significant (p < 0.05) difference in abundance between HIV– and HIV+ groups are highlighted in green; adjusted p-values (FDR) are shown beside it.
To identify if these clusters were differentially abundant between the HIV+ and HIV− groups, we performed differential abundance tests using the diffcyt package, which identified 3 clusters with significantly different abundance between the HIV+ and HIV− groups – clusters 3, 5, and 7 (Figure 4D). Notably, neither cluster 9 or 10 were differentially abundant between the HIV+ and HIV− groups, indicating that the functionally responding subsets remain unaltered in abundance or phenotype (Figure 3) in the setting of ART-treated HIV infection. Hence, although HIV infection induces a reshaping of the NK cell repertoire in vivo, it does so in a way that does not alter HIV-specific responses, as the predominant changes occur in NK cell compartments that do not respond to HIV in in vitro restimulation.
Discussion
Chronic HIV-1 infection is known to alter NK cell phenotype and function. To better understand how these changes occur in the setting of virological control with ART, as well as how HIV-specific function is impacted in this setting, we used mass cytometry to profile differences in NK cell receptor expression repertoire in peripheral blood NK cells between ART-treated, HIV+ individuals and healthy HIV- controls. We observed differences in the IL-2 stimulated NK cell repertoire between HIV+ and HIV− individuals, although these differences did not impact the HIV-specific response to an in vitro restimulation with HIV-infected CD4 T cells. In addition, we identified a unique phenotype of cells that is functionally responsive against HIV; this NK cell subset is shared between both HIV+ and HIV- donors and is similarly responsive in both.
During HIV-1 infection, the NK cell repertoire undergoes significant changes, but even in the setting of virological suppression with ART, the NK repertoire remains altered compared to healthy controls in IL-2 activated NK cells. We found increased expression of NKG2C, CD2, NKp46, and PD-1, and decreased expression of CD244, NKp30, DNAM-1 and NKG2A; many of these markers have been previously described to be altered in HIV infection. The decreased expression of NKp30 and DNAM-1 has been reported for ART-treated HIV-infected patients compared to healthy donors (38, 39); however, these studies also observed lower expression of NKp46 in HIV+ individuals, whereas we saw increased NKp46 expression in the HIV+ group. This discrepancy may be due to the IL-2 activation of NK cells in our study, which is known to increase NKp46 expression (40), and may do so to different extents in the HIV- and HIV+ groups. CD244 expression on NK cells is also known to decrease in HIV infection, although does recover over time after the initiation of ART (41). PD-1 expression is also increased in HIV infection even with ART treatment, and these cells have limited proliferative capacity and may contribute to NK cell dysfunction (42). The increased expression of NKG2C and CD2 may reflect an increase in a subset of NK cells with a more mature, adaptive phenotype that is known to expand during HIV infection, and that persists even during ART treatment (43). Indeed, we observed differential abundance of cluster 3 (Figure 4) between the HIV+ and HIV- groups; this cluster, which had greater abundance in donors of the HIV+ group, expressed high levels of CD2 and low levels of Siglec-7, and expressed both NKG2C and CD57, all known features of this subset.
We also identified other clusters of NK cells that are differentially expressed between HIV+ and HIV- groups (Figure 4). Clusters 5 and 7 have greater abundance in donors of the HIV- group; cells of these clusters have higher expression of CD244 and NKp30 in cluster 7, which we also identified in our GLM analyses (Figure 1B). These differentially abundant clusters may represent the loss of subsets of NK cells that occur after chronic HIV infection or ART treatment. The identification of these phenotypic features of NK cells can provide insight on how chronic, treated HIV infection shapes the activated NK cell repertoire, while the mechanisms and functional consequences of these alterations warrant further investigation.
We identified a unique NK cell subset that has higher functional activity in response to HIV-1-infected cells (Figure 3); this subset has the phenotype CD96hi NKp30hi TIGIThi CD16– CD62L– NKp46–, and its increased functional activity is present in both the HIV+ and HIV- groups. Individually, many of the markers represented in this subset have been previously implicated in immune-mediated control of HIV. For instance, in CD8 T cells, CD96 expression is positively associated with higher CD4 T cell counts in HIV-infected individuals, although CD96+ cells produce less perforin upon stimulation with phorbol myristate acetate/ionomycin (PMA/I), compared to CD96– (44). In NK cells, CD96+ NK cells from peripheral blood have reduced TNF-α and IFN-γ production following PMA/I stimulation (45). These prior data make it difficult to ascertain whether CD96 contributes to HIV control, but our observation that CD96hi cells have improved functional activity against HIV may reflect different mechanisms of activation between HIV infection and stimulation by PMA/I. In addition, NKp30 expression is induced upon IL-2 stimulation, and is correlated with IFN-γ production and inversely associated with the HIV reservoir, suggesting that cells that upregulate NKp30 upon IL-2 stimulation may have improved activity against HIV (46). This is particularly relevant as our study used IL-2 activated NK cells. TIGIT+ NK cells have also been previously implicated in HIV control - TIGIT expansion is markedly enhanced on NK cells in untreated HIV infection (16, 17). We also recently demonstrated that TIGIT expression marks a population of NK cells with an adaptive phenotype with greater functional activity against HIV-infected cells as well as other stimuli (17).
In contrast, the lack of expression of CD16 and NKp46 on these functionally responding cells may reflect downregulation of these receptors after NK cell activation by HIV-infected cells. After stimulation, downregulation of both CD16 and NKp46 have been reported to occur predominantly in activated NK cells that produce IFN-γ and CD107a; this downregulation can occur even in the absence of specific signaling through CD16 or NKp46 (47, 48). As such, these features of this subset may not necessarily reflect their involvement in the HIV-specific response, but instead mark activated NK cells. By using the combinatorial expression of all the markers we have identified, we were able to vastly enrich for cells that were responding against HIV – up to 90% of cells of this phenotype were functionally responding (Figure 3C). Although the frequency of this subset is low (0.1-3% of total NK cells, Figure 3D), and does not account for all responding cells, the high level of functional activity of these cells suggests that they are important in HIV-targeting activity.
While we did not find evidence of improved memory responses of peripheral blood NK cells in HIV+ individuals upon an in vitro re-stimulation with HIV-infected cells, this does not preclude the existence of these memory NK cells in other tissue locations, or in low frequency in the blood. In murine, humanized mice, and non-human primate studies of memory NK cells, memory NK cells were predominantly tissue-resident, particularly in the liver (24, 25, 49). A low frequency of these hepatic phenotype NK cells can be found in peripheral blood (24) and would have been included in our analyses, but may have been at too low a frequency to detect within the bulk of the response to HIV. Indeed, Reeves et al. have previously found that, in non-human primates, memory responses to Gag vaccination by peripheral blood NK cells were much lower in magnitude than splenic or hepatic NK cells, but were still observable (25). Even so, our observation that peripheral blood NK cells did not demonstrate HIV-specific memory responses may be due to the extremely low frequency of these cells, or differences between these memory NK cells in humans compared to mice or non-human primates or with different infections.
There are several limitations to our study. Due to sample availability, we profiled only NK cells in peripheral blood; tissue-resident NK cells may exhibit differences in both phenotype and the ability to generate memory responses, as discussed above. In addition, NK cells were not re-stimulated with the same HIV-1 strain as the primary in vivo infection, as we used a separate, in vitro infection with a different HIV strain. We used a subtype A strain for all in vitro HIV restimulations; however, as all our subjects were recruited in North America, where subtype B strains dominate (50), the mismatch in viral strain used for the secondary challenge may have contributed to the poor memory NK cell responses we observed. Even so, we have shown that infection with HIV-1 viruses across both subtype A and subtype B strains lead to similar patterns of alterations in expression of NK cell ligands on infected CD4 T cells (unpublished data), suggesting that strain-specific recognition is unlikely in NK cells, and the mismatch of strain subtype in primary infection and in vitro restimulation would not impair the detection of potential memory responses. Lastly, as our primary interest was in evaluating functional responses to HIV-infected cells, we profiled IL-2 activated NK cells, which may not entirely recapitulate NK cell phenotypes ex vivo. IL-2 induces changes in NK receptor expression, including upregulation of the natural cytotoxicity receptors (NCRs) NKp30 and NKp46; however, the expression of most other NK receptors remains unchanged (51). The differences in cytokine-induced upregulation of these NCRs between the HIV+ and HIV− groups can additionally be informative, as upregulation of NCRs have been implicated in control of HIV (46).
In summary, our data demonstrate that phenotypic alterations in peripheral blood NK cells that occur in individuals with ART-treated HIV-1 infection do not result in improved NK-mediated targeting of HIV. These phenotypic changes instead occur in distinct cellular subsets that are not involved in the functional response to HIV. Further work is required to understand whether other tissue-resident NK cells may exhibit differences in phenotypic alterations and functional responses in the course of treated HIV infection.
Data Availability Statement
The datasets generated for this study are available at ImmPort (https://www.immport.org) under study accession SDY1620.
Ethics Statement
The studies involving human participants were reviewed and approved by Institutional Review Board of Stanford University. The patients/participants provided their written informed consent to participate in this study.
Author Contributions
NZ and CB designed the experiments. NZ conducted the experiments. NZ and A-MF analyzed the data with statistical analysis input from SH. PG contributed samples to the study. NZ and CB wrote the manuscript. All authors contributed revisions.
Conflict of Interest
The authors declare that the research was conducted in the absence of any commercial or financial relationships that could be construed as a potential conflict of interest.
The handling Editor declared a past co-authorship with one of the authors CB.
Funding
This work was supported by the ITI/Bill & Melinda Gates Foundation Pilot Grant (CB), NIH/NIAID DP2 AI112193 (CB), NIH/NIDA Avant-Garde Award for HIV Research DP1 DA046089 (CB), and the Burroughs Wellcome Fund Investigators in the Pathogenesis of Infectious Diseases (CB). NZ was supported by a National Science Scholarship from the Agency of Science, Technology and Research (A∗STAR) Singapore. CB was the Tashia and John Morgridge Faculty Scholar in Pediatric Translational Medicine from the Stanford Maternal Child Health Research Institute, and an Investigator of the Chan Zuckerberg Biohub.
Acknowledgments
We would like to thank Thanmayi Ranganath for assistance with running mass cytometry samples, and the Human Immune Monitoring Core (HIMC) at Stanford University for use of their Helios machine.
Supplementary Material
The Supplementary Material for this article can be found online at: https://www.frontiersin.org/articles/10.3389/fimmu.2020.00829/full#supplementary-material
Footnotes
References
1. Alter G, Martin MP, Teigen N, Carr WH, Suscovich TJ, Schneidewind A, et al. Differential natural killer cell-mediated inhibition of HIV-1 replication based on distinct KIR/HLA subtypes. J Exp Med. (2007) 204:3027–36. doi: 10.1084/jem.20070695
2. Alter G, Teigen N, Davis BT, Addo MM, Suscovich TJ, Waring MT, et al. Sequential deregulation of NK cell subset distribution and function starting in acute HIV-1 infection. Blood. (2005) 106:3366–9. doi: 10.1182/blood-2005-03-1100
3. Bbosa N, Kaleebu P, Ssemwanga D. HIV subtype diversity worldwide. Curr Opin HIV AIDS. (2019) 14:153–60. doi: 10.1097/COH.0000000000000534
4. Bisio F, Bozzano F, Marras F, Di Biagio A, Moretta L, De Maria A. Successfully treated HIV-infected patients have differential expression of NK cell receptors (NKp46 and NKp30) according to AIDS status at presentation. Immunol Lett. (2013) 152:16–24. doi: 10.1016/j.imlet.2013.03.003
5. Brunetta E, Fogli M, Varchetta S, Bozzo L, Hudspeth KL, Marcenaro E, et al. The decreased expression of Siglec-7 represents an early marker of dysfunctional natural killer–cell subsets associated with high levels of HIV-1 viremia. Blood. (2009) 114:3822–30. doi: 10.1182/blood-2009-06-226332
6. Campos C, López N, Pera A, Gordillo JJ, Hassouneh F, Tarazona R, et al. Expression of NKp30, NKp46 and DNAM-1 activating receptors on resting and IL-2 activated NK cells from healthy donors according to CMV-serostatus and age. Biogerontology. (2015) 16:671–83. doi: 10.1007/s10522-015-9581-0
7. Davis ZB, Cogswell A, Scott H, Mertsching A, Boucau J, Wambua D, et al. A conserved HIV-1-derived peptide presented by HLA-E renders infected T-cells highly susceptible to attack by NKG2A/CD94-bearing natural killer cells. PLoS Pathog. (2016) 12:e1005421. doi: 10.1371/journal.ppat.1005421
8. Eriksson EM, Keh CE, Deeks SG, Martin JN, Hecht FM, Nixon DF. Differential expression of CD96 surface molecule represents CD8+ T cells with dissimilar effector function during HIV-1 infection. PLoS One. (2012) 7:e51696. doi: 10.1371/journal.pone.0051696
9. Foley B, Cooley S, Verneris MR, Curtsinger J, Luo X, Waller EK, et al. Human cytomegalovirus (CMV)-induced memory-like NKG2C+ NK cells are transplantable and expand in vivo in response to recipient CMV antigen. J Immunol. (2012) 189:5082–8. doi: 10.4049/jimmunol.1201964
10. Grzywacz B, Kataria N, Verneris MR. CD56(dim)CD16(+) NK cells downregulate CD16 following target cell induced activation of matrix metalloproteinases. Leukemia. (2007) 21:356–9. author reply 359. doi: 10.1038/sj.leu.2404499
11. Gumá M, Angulo A, Vilches C, Gómez-Lozano N, Malats N, López-Botet M. Imprint of human cytomegalovirus infection on the NK cell receptor repertoire. Blood. (2004) 104:3664–71. doi: 10.1182/blood-2004-05-2058
12. Gumá M, Budt M, Sáez A, Brckalo T, Hengel H, Angulo A, et al. Expansion of CD94/NKG2C+ NK cells in response to human cytomegalovirus-infected fibroblasts. Blood. (2006) 107:3624–31. doi: 10.1182/blood-2005-09-3682
13. Hammer Q, Rückert T, Borst EM, Dunst J, Haubner A, Durek P, et al. Peptide-specific recognition of human cytomegalovirus strains controls adaptive natural killer cells. Nat Immunol. (2018) 19:453–63. doi: 10.1038/s41590-018-0082-6
14. Hu P-F, Hultin LE, Hultin P, Hausner MA, Hirji K, Jewett A, et al. Natural killer cell immunodeficiency in HIV disease is manifest by profoundly decreased numbers of CD16 CD56 cells and expansion of a population of CD16dim CD56- cells with low lytic activity. J Acquir Immun Defici Syndrom Hum Retrovirol. (1995) 10:331–40. doi: 10.1097/00042560-199511000-00005
15. Kuri-Cervantes L, de Oca GS-M, Avila-Ríos S, Hernández-Juan R, Reyes-Terán G. Activation of NK cells is associated with HIV-1 disease progression. J Leukoc Biol. (2014) 96:7–16. doi: 10.1189/jlb.0913514
16. Lisovsky I, Isitman G, Song R, DaFonseca S. A higher frequency of NKG2A+ than of NKG2A- NK cells responds to autologous HIV-infected CD4 cells irrespective of whether or not they coexpress KIR3DL1. J Virol. (2015) 89:9909–19. doi: 10.1128/JVI.01546-15
17. Lopez-Verges S, Milush JM, Schwartz BS, Pando MJ, Jarjoura J, York VA, et al. Expansion of a unique CD57 NKG2Chi natural killer cell subset during acute human cytomegalovirus infection. Proc Natl Acad Sci USA. (2011) 108:14725–32. doi: 10.1073/pnas.1110900108
18. Marras F, Casabianca A, Bozzano F, Ascierto ML, Orlandi C, Di Biagio A, et al. Control of the HIV-1 DNA Reservoir Is Associated In Vivo and In Vitro with NKp46/NKp30 (CD335 CD337) Inducibility and Interferon Gamma Production by Transcriptionally Unique NK Cells. J. Virol. (2017) 91:647–617. doi: 10.1128/JVI.00647-17
19. Martin MP, Gao X, Lee J-H, Nelson GW, Detels R, Goedert JJ, et al. Epistatic interaction between KIR3DS1 and HLA-B delays the progression to AIDS. Nat Genet. (2002) 31:429–34. doi: 10.1038/ng934
20. Mavilio D, Benjamin J, Daucher M, Lombardo G, Kottilil S, Planta MA, et al. Natural killer cells in HIV-1 infection: dichotomous effects of viremia on inhibitory and activating receptors and their functional correlates. Proc Natl Acad Sci USA. (2003) 100:15011–6. doi: 10.1073/pnas.2336091100
21. Mavilio D, Lombardo G, Benjamin J, Kim D, Follman D, Marcenaro E, et al. Characterization of CD56–/CD16+ natural killer (NK) cells: A highly dysfunctional NK subset expanded in HIV-infected viremic individuals. Proc Natl Acad Sci USA. (2005) 102:2886–91. doi: 10.1073/pnas.0409872102
22. McCarthy DJ, Campbell KR, Lun ATL, Wills QF. Scater: pre-processing, quality control, normalization and visualization of single-cell RNA-seq data in R. Bioinformatics (2017) 33:1179–86. doi: 10.1093/bioinformatics/btw777
23. McInnes L, Healy J, Melville J. UMAP: uniform manifold approximation and projection for dimension reduction. arXiv. (2018) [Preprint]. arXiv:1802.03426v2.
24. Mei HE, Leipold MD, Schulz AR, Chester C, Maecker HT. Barcoding of live human peripheral blood mononuclear cells for multiplexed mass cytometry. J Immunol. (2015) 194:2022–31. doi: 10.4049/jimmunol.1402661
25. Milush JM, López-Vergès S, York VA, Deeks SG, Martin JN, Hecht FM, et al. CD56negCD16+NK cells are activated mature NK cells with impaired effector function during HIV-1 infection. Retrovirology. (2013) 10:158. doi: 10.1186/1742-4690-10-158
26. Nikzad R, Angelo LS, Aviles-Padilla K, Le DT, Singh VK, Bimler L, et al. Human natural killer cells mediate adaptive immunity to viral antigens. Sci Immunol. (2019) 4:eaat8116. doi: 10.1126/sciimmunol.aat8116
27. Norris S, Coleman A, Kuri-Cervantes L, Bower M, Nelson M, Goodier MR. PD-1 expression on natural killer cells and CD8(+) T cells during chronic HIV-1 infection. Viral Immunol. (2012) 25:329–32. doi: 10.1089/vim.2011.0096
28. Nowicka M, Krieg C, Crowell HL, Weber LM, Hartmann FJ, Guglietta S, et al. CyTOF workflow: differential discovery in high-throughput high-dimensional cytometry datasets. F1000Res. (2017) 6:748. doi: 10.12688/f1000research.11622.3
29. Ostrowski SR, Ullum H, Pedersen BK, Gerstoft J, Katzenstein TL. 2B4 expression on natural killer cells increases in HIV-1 infected patients followed prospectively during highly active antiretroviral therapy. Clin Exp Immunol. (2005) 141:526–33. doi: 10.1111/j.1365-2249.2005.02869.x
30. Parsons MS, Tang C-C, Jegaskanda S, Robert J, Center, Brooks AG, et al. Anti-HIV antibody–dependent activation of NK cells impairs NKp46 expression. J Immunology. (2014) 192:308–15. doi: 10.4049/jimmunol.1301247
31. Paust S, Blish CA, Reeves RK. Redefining memory: building the case for adaptive NK cells. J Virol. (2017) 91:169–117. doi: 10.1128/JVI.00169-17
32. Paust S, Gill HS, Wang B-Z, Flynn MP, Moseman EA, Senman B, et al. Critical role for the chemokine receptor CXCR6 in NK cell–mediated antigen-specific memory of haptens and viruses. Nat Immunol. (2010) 11:1127–35. doi: 10.1038/ni.1953
33. Peppa D, Pedroza-Pacheco I, Pellegrino P, Williams I, Maini MK, Borrow P. Adaptive reconfiguration of natural killer cells in HIV-1 infection. Front Immunol. (2018) 9:474. doi: 10.3389/fimmu.2018.00474
34. Poss M, Overbaugh J. Variants from the diverse virus population identified at seroconversion of a clade A human immunodeficiency virus type 1-infected woman have distinct biological properties. J Virol. (1999) 73: 5255–64.
35. Ramsuran V, Naranbhai V, Horowitz A, Qi Y, Martin MP, Yuki Y, et al. Elevated HLA-A expression impairs HIV control through inhibition of NKG2A-expressing cells. Science. (2018) 359:86–90. doi: 10.1126/science.aam8825
36. Ravet S, Scott-Algara D, Bonnet E, Tran HK, Tran T, Nguyen N, et al. Distinctive NK-cell receptor repertoires sustain high-level constitutive NK-cell activation in HIV-exposed uninfected individuals. Blood. (2007) 109:4296–305. doi: 10.1182/blood-2006-08-040238
37. Reeves RK, Li H, Jost S, Blass E, Li H, Schafer JL, et al. Antigen-specific NK cell memory in rhesus macaques. Nat Immunol. (2015) 16:927–32. doi: 10.1038/ni.3227
38. Richard J, Sindhu S, Pham TNQ, Belzile J-P, Cohen EA. HIV-1 Vpr up-regulates expression of ligands for the activating NKG2D receptor and promotes NK cell-mediated killing. Blood. (2010) 115:1354–63. doi: 10.1182/blood-2009-08-237370
39. Scott-Algara D, Truong LX, Versmisse P, David A, Luong TT, Nguyen NV, et al. Cutting edge: increased NK cell activity in HIV-1-exposed but uninfected Vietnamese intravascular drug users. J Immunol. (2003) 171:5663–7. doi: 10.4049/jimmunol.171.11.5663
40. Seiler C, Kronstad LM, Simpson LJ, Le Gars M, Vendrame E, Blish CA, et al. Uncertainty quantification in multivariate mixed models for mass cytometry data. arXiv. (2019) [Preprint]. arXiv:1903.07976.
41. Song R, Lisovsky I, Lebouché B, Routy J-P, Bruneau J, Bernard NF. HIV protective KIR3DL1/S1-HLA-B genotypes influence NK cell-mediated inhibition of HIV replication in autologous CD4 targets. PLoS Pathog. (2014) 10:e1003867. doi: 10.1371/journal.ppat.1003867
42. Strauss-Albee DM, Fukuyama J, Liang EC, Yao Y, Jarrell JA, Drake AL, et al. Human NK cell repertoire diversity reflects immune experience and correlates with viral susceptibility. Sci Transl Med (2015) 7:297ra115. doi: 10.1126/scitranslmed.aac5722
43. Sun H, Huang Q, Huang M, Wen H, Lin R, Zheng M, et al. Human CD96 Correlates to Natural Killer Cell Exhaustion and Predicts the Prognosis of Human Hepatocellular Carcinoma. Hepatology. (2019) 70:168–83. doi: 10.1002/hep.30347
44. R Core Team. R: A Language and Environment for Statistical Computing. (2019). Available online at: https://repo.bppt.go.id/cran/web/packages/dplR/vignettes/intro-dplR.pdf (accessed March 14, 2020).
45. Van Gassen S, Callebaut B, Van Helden MJ, Lambrecht BN, Demeester P, Dhaene T, et al. FlowSOM: Using self-organizing maps for visualization and interpretation of cytometry data. Cytometry A. (2015) 87:636–45. doi: 10.1002/cyto.a.22625
46. Vendrame E, Fukuyama J, Strauss-Albee DM, Holmes S, Blish CA. Mass cytometry analytical approaches reveal cytokine-induced changes in natural killer cells. Cytometry B Clin Cytom. (2017) 92:57–67. doi: 10.1002/cyto.b.21500
47. Vendrame E, Seiler C, Ranganath T, Zhao NQ, Vergara R, Alary M, et al. TIGIT is upregulated by HIV-1 infection and marks a highly functional adaptive and mature subset of natural killer cells. AIDS. (2020) 34:801–13. doi: 10.1097/QAD.0000000000002488
48. Weber LM, Nowicka M, Soneson C, Robinson MD. diffcyt: differential discovery in high-dimensional cytometry via high-resolution clustering. Commun Biol. (2019) 2:183. doi: 10.1038/s42003-019-0415-5
49. Wilkerson MD, Hayes DN. ConsensusClusterPlus: a class discovery tool with confidence assessments and item tracking. Bioinformatics. (2010) 26:1572–3. doi: 10.1093/bioinformatics/btq170
50. Yin X, Liu T, Wang Z, Ma M, Lei J, Zhang Z, et al. Expression of the Inhibitory Receptor TIGIT Is Up-Regulated Specifically on NK Cells With CD226 Activating Receptor From HIV-Infected Individuals. Front Immunol. (2018) 9:2341. doi: 10.3389/fimmu.2018.02341
Keywords: NK cells, HIV-1, memory, innate immunity, CyTOF
Citation: Zhao NQ, Ferreira A-M, Grant PM, Holmes S and Blish CA (2020) Treated HIV Infection Alters Phenotype but Not HIV-Specific Function of Peripheral Blood Natural Killer Cells. Front. Immunol. 11:829. doi: 10.3389/fimmu.2020.00829
Received: 07 March 2020; Accepted: 14 April 2020;
Published: 12 May 2020.
Edited by:
Silke Paust, The Scripps Research Institute, United StatesReviewed by:
Jacques Zimmer, Luxembourg Institute of Health, LuxembourgVincent Vieillard, Centre National de la Recherche Scientifique (CNRS), France
Copyright © 2020 Zhao, Ferreira, Grant, Holmes and Blish. This is an open-access article distributed under the terms of the Creative Commons Attribution License (CC BY). The use, distribution or reproduction in other forums is permitted, provided the original author(s) and the copyright owner(s) are credited and that the original publication in this journal is cited, in accordance with accepted academic practice. No use, distribution or reproduction is permitted which does not comply with these terms.
*Correspondence: Catherine A. Blish, Y2JsaXNoQHN0YW5mb3JkLmVkdQ==