- 1Cancer Centre, Faculty of Health Sciences, University of Macau, Avenida de Universidade, Taipa, China
- 2Key Laboratory of Optoelectronic Science and Technology for Medicine of Ministry of Education, College of Life Sciences, Fujian Normal University, Fuzhou, China
Targeting PD-L1 and PD-1 interactions is a relatively new therapeutic strategy used to treat cancer. Inhibitors of PD-1/PD-L1 include peptides, small molecule chemical compounds, and antibodies. Several approved antibodies targeting PD-1 or PD-L1 have been patented with good curative effect in various cancer types in clinical practices. While the current antibody therapy is facing development bottleneck, some companies have tried to develop PD-L1 companion tests to select patients with better diagnosis potential. Meanwhile, many companies have recently synthesized small molecule inhibitors of PD-1/PD-L1 interactions and focused on searching for novel biomarker to predict the efficacy of anti-PD-1/PD-L1 drugs. This review summarized clinical studies and patent applications related to PD-1/PD-L1 targeted therapy and also discussed progress in inhibitors of PD-1/PD-L1.
Introduction
Programmed cell death protein 1, also referred to as cluster of differentiation 279 (CD279), is a surface protein that can regulate the immune system by inhibiting T-cell activity. PD-1 is constitutively expressed on activated T-cells, B cells, natural killer (NK) cells, macrophages, and dendritic cells (DCs) (1). Programmed death-ligand 1 (PD-L1), also referred to as B7-H1 or CD274, is constitutively expressed on antigen-presenting cells, lymphoid, endothelial, and epithelial cells (2). Interferon gamma (IFN-γ) and tumor necrosis factor (TNF-α) secreted by activated T-cells can also induce PD-L1 expression on tumor cells and antigen-presenting cells (APCs) (3). Figure 1 shows that naïve T-cells are activated through binding between T cell receptors (TCR) and the peptide-MHC complex presented by (APC); T-cell activation can lead to transient upregulation of PD-1, which is the receptor of PD-L1. Binding between PD-1 and PD-L1 negatively regulates downstream signaling mediated by co-activation of TCR and CD28 (4). When PD-L1 interacts with PD-1, the immunoreceptor tyrosine-based inhibitory motifs (ITIM) and immunoreceptor tyrosine-based switch motifs (ITSM), which are on the intracellular domain of PD-1, can be phosphorylated. The Src homology 2 domain-containing protein tyrosine phosphatase 1 (SHP-1) and Src homology 2 domain-containing protein tyrosine phosphatase 1 (SHP-2) are then recruited and bind to ITIM to further inhibit the signaling downstream of the TCR (5). After inhibiting the TCR-mediated signaling pathway, PD-1 prevents the activation of the pathway mediated by PI3K/Akt or Ras/MEK/Erk. This further inhibits the function of CD8+ T-cells (6). Programmed cell death 1 ligand 2 (also known as PD-L2, B7-DC), which is the second ligand of PD-1, is expressed on tumor cells, APCs, cancer associated fibroblasts, and macrophages (7–9). PD-L2 plays an inhibitory role on the functioning of T-cells, which is similar to that of PD-L1. Meanwhile, PD-L1 also interacts with the surface protein CD80 (B7-1) expressed on activated T-cells. Interacting with PD-L1, CD80 could induce increased expression of Bim, which contributes to the apoptosis of CD8+ T-cells (10). As a result, the PD-1/PD-L1 signaling pathway promotes tumor cells escaping immune surveillance by inhibiting cell survival and activation of T-cells.
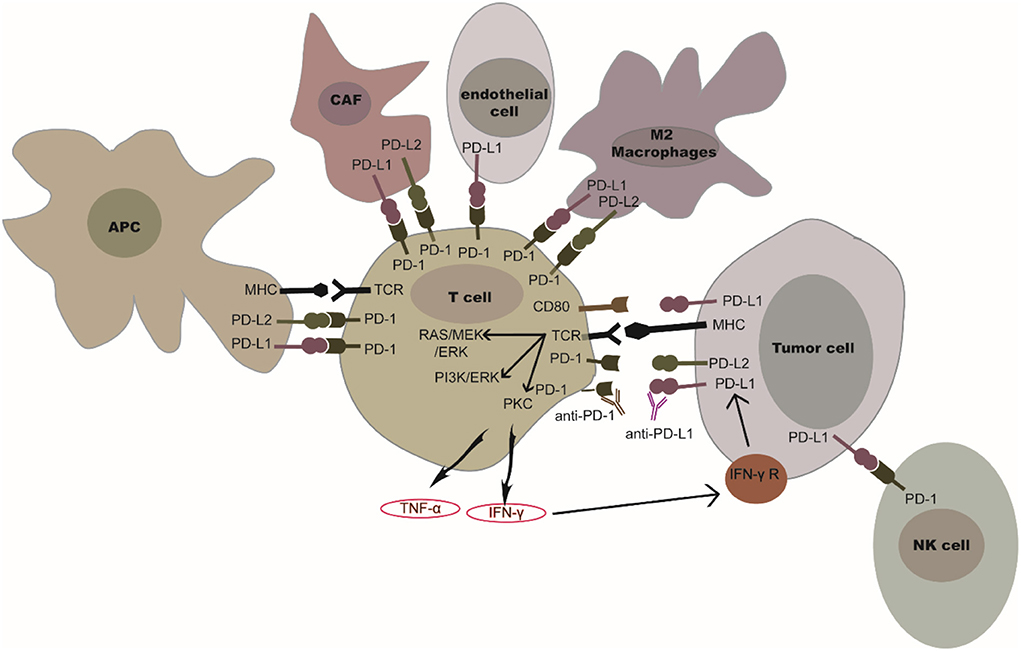
Figure 1. PD-1/PD-L1 or PD-1/PD-L2 in the tumor microenvironment. PD-1 is expressed on T-cells and NK cells. PD-L1 is expressed in tumor cells, antigen presenting cells, cancer associated fibroblasts, and in several immune cells (myeloid cells, endothelial cells, M2 macrophages). The binding of PD-L1 or PD-L2 to PD-1 could inhibit the functioning of T-cells and NK cells. IFN-γ secreted by activated T-cells mediates the up-regulation of tumor PD-L1. The blockade of PD-1/PD-L1 or PD-1/PD-L2 interaction by PD-1 or PD-L1 inhibitors could restore T-cell or NK cell activation.
Targeting PD-L1 and PD-1 interactions is a novel therapeutic strategy used for cancer treatment. Antibodies targeting PD-1 or PD-L1 have marked a breakthrough in cancer immunotherapy and have become a hot topic in cancer therapy. Many companies have therefore begun studies on cancer immunotherapy and applied a series of related patents and patent applications in this field. To date, there have been about 5,000 patents published, and the number of patents continues to increase (Figure 2). In this review, we demonstrate the development of PD-1/PD-L1 directed immunotherapy and progress in inhibitors disrupting PD-1/PD-L1 binding. Moreover, patents or patent applications related to PD-1/PD-L1 signaling pathway and its inhibitors will also be discussed in this review, which will provide an update on PD-1/PD-L1 targeted cancer therapy.
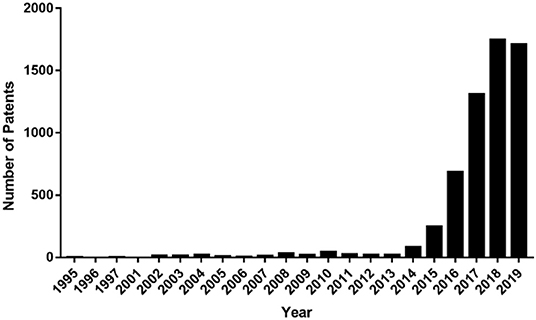
Figure 2. Numbers of international patent applications published per year containing the word “PD-1” or “PD-L1” in the title, claim, or abstract.
Historical Overview of Relevant Patents of PD-1/PD-L1 Targeting Cancer Immunotherapy
The PD-1 protein was discovered by Tasuku Honjo in 1992, and he was awarded the Nobel Prize in physiology and medicine in 2018. The patent published in 1995 by Honjo firstly proposed the sequence of PD-1 protein and gene encoded PD-1 (11). Honjo's discovery also showed that PD-1 is a protein that negatively regulates the immune system (12). Later, Gordon Freeman identified B7–4 as one of the ligands to PD-1 (13). Meanwhile, Dr. Lieping Chen and his team independently discovered B7-H1. The sequence of B7-H1 protein and gene encoded B7-H1 was published in 1999 by Dong et al. (14). However, they did not mention the correlation between B7-H1 and PD-1. Based on his own findings of B7-H1, Chen et al. applied a series of patents related to B7-H1 protein. Meanwhile, in 2000, Freeman et al. published a paper mentioning that B7-4 was renamed to PD-L1 and is the same as B7-H1 protein discovered by Freeman et al. (15). Freeman also mentioned that PD-L1 is one of the members of the CD28/B7 immunoglobulin superfamily that could inhibit the T-cell function through PD-1/PD-L1 interactions (15). Table 1 shows patents and patent applications for the finding of PD-1 and PD-L1 proteins and the development of PD-1/PD-L1 blockade therapy.
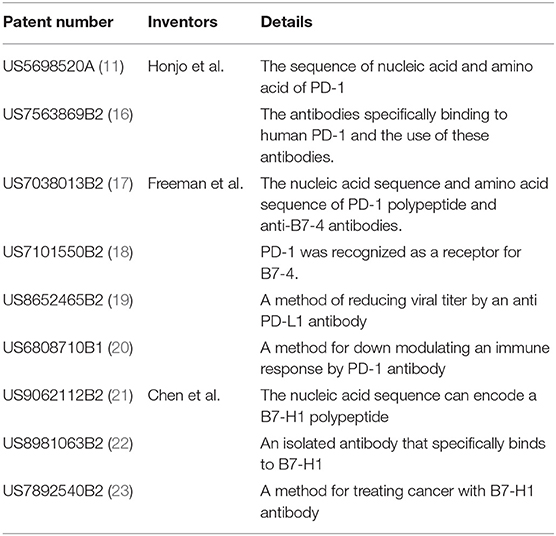
Table 1. Patents and patent applications naming Honjo, Freeman, and Dr. Chen as inventors that are related to PD-1 and PD-L1.
Honjo's studies suggested that suppression of the PD-1 protein could be effective in cancer treatment (12). Studies have shown that expression of PD-1 and PD-L1 was enhanced in cancer cells and was related to defective immune responses (24). These studies suggested that two immune checkpoint molecules may be important therapeutic targets for cancer and infectious disease treatment. Thus, the blockade of PD-1/PD-L1 interactions using inhibitors may be a novel and effective strategy for immunotherapy. Additionally, a previous study showed that blockade of the PD-1/PD-L1 pathway using PD-L1 antibody could inhibit T-cell apoptosis (25). This study also showed that PD-L1 antibody affected the survival of tumor cells in vivo (25). These results proved that PD-L1 antibodies can enhance T-cell growth to further inhibit tumor growth—this suggests that inhibition of the PD-1/PD-L1 interaction could be a new method of cancer treatment.
Honjo cooperated with Ono Pharmaceutical Co. and Medarex to develop an anti-cancer medication targeting PD-1, named nivolumab. Two studies of nivolumab conducted in Phase III trials showed impressive efficacy for this antibody in advanced melanoma (26, 27). The results of a phase III trial showed that the overall survival rate at 1 year was significantly different between the nivolumab group (72.9%) and dacarbazine group (42.1%) of previously untreated patients who had advanced melanoma without a BRAF mutation (26). In addition, nivolumab showed higher response rates and lower toxicity rates than ipilimumab and chemotherapy (27). Following the results of these two clinical trials, the Food and Drug Administration (FDA) approved nivolumab for the treatment of advanced melanoma in 2014. The discovery of the PD-1/PD-L1 signaling pathway attracted researchers' attention on developing antibodies against this pathway. The PD-1 protein has led to breakthroughs in cancer immunotherapies in the past decades. Many companies have filed patents related to antibodies during these past 20 years. Table 2 shows the core patents related to FDA-approved antibodies while Table 3 shows patents related to antibodies.
Structure Analysis of Antibodies Targeting PD-1 and PD-L1
Several structures and classes of antibodies inhibiting the PD-1/PD-L1 interaction have been published recently. Most of these anti-PD-1 antibodies are fully human immunoglobulin G4 (IgG4) antibodies with the S228P mutation, including nivolumab, pembrolizumab, cemiplimab, dostarlimab, MEDI-0680, and SSI-361. These antibodies have similar binding properties to the natural IgG4, which reduce ADCC function and eliminate CDC function, but they still retain function in binding to FcγRI and FcγRIIb. Spartalizumab is a humanized IgG4κ monoclonal antibody with S228P mutations and K447 deletion (44). Tislelizumab was generated via the introduction of several mutations (including S228P, E233P, F234V, L235A, D265A, and R409K) in IgG4 antibodies (45). AMP-224 is an anti-PD-1 recombinant fusion protein that contains the extracellular domain of PD-L2 and Fc domain of human IgG1 (46).
Moreover, the crystal structures of PD-1/Anti-PD-1 antibodies have also been explored. The N-terminal extension, BC-loops, and FG-loops are crucial for binding of nivolumab and PD-1. The VL chain of nivolumab and PD-L1 residues shared an overlapping binding surface on the FG loop (47). The C'D loop of PD-1 mainly contributes to the interaction with pembrolizumab (48). Anti-PD-1 antibodies inhibit the PD-1/PD-L1 interaction by competing with PD-L1 while binding to PD-1. The epitopes of these antibodies directly occupy the partial binding site of the PD-L1 protein. In addition, the binding of PD-1 and its antibodies induces optimal conformational changes in the PD-1 protein, which blocks PD-1/PD-L1 interactions, because PD-1 also interacts with PD-L1 in distinct conformations. Tislelizumab interacts with an IgV-like domain of PD-1 and is different from pembrolizumab and nivolumab, as shown by its unique binding epitopes, including Gln75, Thr76, Asp77, and Arg86 (45). Although SHR-1210 was reported to have unspecific interactions with some human receptors driving angiogenesis, the optimization of complementary determining region (CDR) domains successfully eliminated off-target binding (49). Meanwhile, the binding properties of SHR-1210 have not been reported.
Unlike anti-PD-1 antibodies, three approved anti-PD-L1 antibodies include human IgG1 antibodies. Atezolizumab and durvalumab are antibodies of eliminated FcγR-binding and effector functions while avelumab was designed to retain intact Fc functions (50). BMS-936559 is differentiated from three approved PD-L1 antibodies and is an IgG4 mAb with S228P mutations (50). KN035 is a fusion protein containing a single domain of the humanized anti-PD-L1 antibody and the Fc of an IgG1 (51). CX-072 is a human PD-L1 specific protease-activatable antibody prodrug. CX-072 was designed by linking the masking peptide links to the targeted antibody (52).
Recently, the crystal structures of the PD-L1/avelumab complex revealed that avelumab/atezolizumab/BMS-936559 binds to the IgV domain of PD-L1 through its heavy chain (VH) and light chain (VL). These are dominated by the VH chain (53). A comparison of the PD-L1/antibody and human PD-1/PD-L1 complexes demonstrates that antibodies directly occupy the partial binding site of the PD-1 protein. In contrast, the PD-L1/durvalumab Fab complex demonstrated that the binding sites of the antibody are in the N-terminal region of the PD-L1 protein (53). The KN035/PD-L1 complex showed a different pattern. The paratope of KN035 is limited to only two complementary determining regions (CDRs)—one of which contributes to binding with high-affinity (54). This narrow binding area provides an opportunity for rationally designing peptides or small-molecule inhibitors that imitate the nanobody/PD-L1 interface.
Clinical Application of PD-1/PD-L1 Targeting Cancer Immunotherapy
There have been more than 2,000 clinical trials of anti-PD-1 antibodies and over 1,000 clinical trials of anti-PD-L1 antibodies (Figure 3). Based on the data from several clinical trials, some of these drugs have been approved by the FDA, the National Medical Products Administration (NMPA), and the European Medicines Agency (EMA) for use in the treatment of various cancers. Nivolumab and pembrolizumab, two anti-PD-1 antibodies, obtained approval for cancer therapy in 2014. After that, more PD-1 and PD-L1 drugs got FDA approval following positive results from clinical trials. There are currently several FDA-approved antibodies, including nivolumab (trade name: Opdivo), pembrolizumab (trade name: Keytruda), cemiplimab (trade name: Libtayo), atezolizumab (trade name: Tecentriq), durvalumab (trade name: Imfinzi), and avelumab (trade name: Bavencio) (Table 4) (50). In addition, camrelizumab and toripalimab were approved by NMPA for marketing.
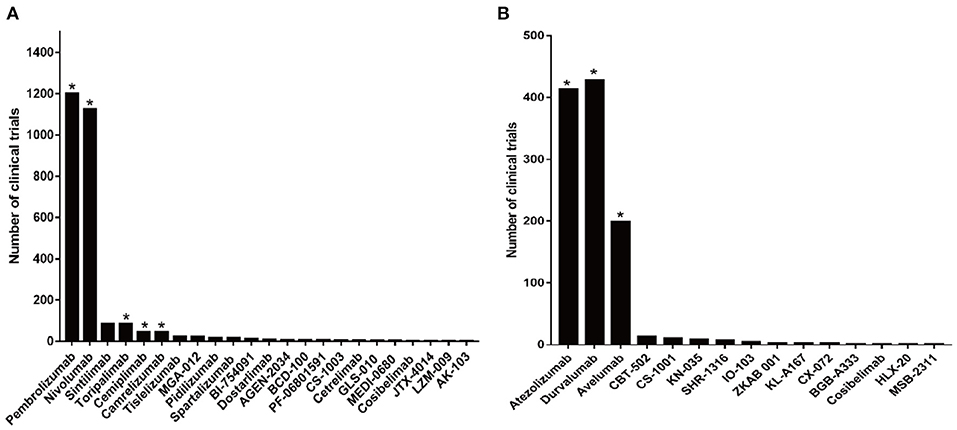
Figure 3. Clinical trials related to anti-PD-1/PD-L1 antibodies. (A) Numbers of clinical trials of anti-PD-1 antibodies. (B) Numbers of clinical trials of anti-PD-L1 antibodies. Antibodies that obtained approval for cancer therapy are indicated by an asterisk.
Clinical trials of most antibodies have just started, and the results require further updating. Table 5 summarizes several clinical trials of anti-PD-1/PD-L1 antibodies that are currently being developed with the latest data. The data from clinical trials revealed that newly developed antibodies also showed a durable response. Table 5 also demonstrates that anti-PD-1/PD-L1 antibodies can cause treatment-related adverse effects (TRAEs) and immune-related adverse effects (IRAEs). In some patients, these AEs led to treatment discontinuation and treatment interruption. The Objective response rate (ORR) is 47% among the 75 patients with metastatic CSCC who received cemiplimab-rwlc. Complete response was achieved in 4% of patients (81). Among patients with relapsed/refractory cHL (NCT02961101 and NCT03250962), the response duration rate at 6 months was 76% in patients treated with camrelizumab monotherapy (n = 19) compared to 100% in those treated with decitabine plus camrelizumab (n = 42) (95). Among the 127 patients with advanced melanoma (NCT03013101), the ORR is 17.3% in overall population after treatment with toripalimab. The disease control rate (DCR) was 57.5% and median progression free survival (PFS) was 3.6 months (96). Based on the clinical results shown above, cemiplimab, camrelizumab, and toripalimab were approved for clinical use.
The Current Optimization of Anti-PD-1/PD-L1 Treatment Strategy
Several clinical trials using antibodies targeting the interaction of PD-1 and PD-L1 for cancer treatment have shown promising abilities in prolonging survival, but not all patients respond to PD-1/PD-L1 inhibitors (97). In addition, clinical results have also shown that anti-PD-1 or anti-PD-L1 treatment caused TRAEs and IRAEs, although anti-PD-1/PD-L1 drugs have shown lower toxicity than standard chemotherapy (98). Most seriously, AEs caused by these antibodies sometimes could lead to treatment discontinuation and treatment interruption (98). Due to the limited success and disadvantages of anti-PD-1/PD-L1 antibodies, effective strategies are needed to improve the efficacy of PD-1/PD-L1 targeted immunotherapy. Detecting PD-L1 expression in tumor cells and tumor infiltrated T-cells would be useful for targeting patients with a big likelihood of responding to PD-1/PD-L1 treatment. Meanwhile, it is also crucial to search for potential biomarkers that could selectively reflect the efficacy and feasibility of anti-PD1/PD-L1 therapy. Furthermore, small molecule inhibitors targeting PD-1 and PD-L1 are emerging as their potential advantages are realized vs. monoclonal antibodies.
The Application of PD-L1 Immunohistochemistry (IHC) Assays
Some clinical trials have shown that more than half of patients had no response to anti-PD-1 drugs, and some responders even experience tumor relapse within 2 years after treatment of anti-PD-1 drugs (26, 99). Studies suggest that clinical efficacy of PD-1/PD-L1 targeted immunotherapies may be predicted by PD-L1 expression on tumor cells and tumor-infiltrating immune cells (100). Developing PD-L1 IHC test compounds have begun to attract scientists' attention during the past 5 years. Several companies have designed commercially available PD-L1 IHC tests, including 22C3, 28-8, SP263, SP142, E1L3N, and 73-10 assays. Merck developed a PD-L1 IHC test using 22C3 antibody and also applied for a patent (US9709568B2), which claimed the use of the 22C3 antibody for diagnostic purposes (101). In addition, BMS designed a different companion assay for PD-L1 expression using its 28-8 antibody and had a patent application (WO2013173223A1) that described a method of detecting PD-L1 expression using the clone 28-8 antibody (102). The SP142 assay was developed by Ventana and was described in patent application WO2015181343A2 (103).
These PD-L1 IHC assays are currently being tested in clinical trials, and some of them have been approved by the FDA as companion diagnostics for PD-1/PD-L1 targeted immunotherapies. Table 6 shows that PD-L1 expression was first reported to be associated with higher response rates to pembrolizumab/atezolizumab and was approved by the FDA to guide the selection of patients for anti-PD-1/PD-L1 treatment. For example, the DAKO 22C3 IHC assay is approved for use as a companion diagnostic with pembrolizumab immunotherapy in NSCLC, gastric cancer, cervical cancer, HNSCC, and ESCC (68, 104–108). In addition, the Ventana PD-L1 (SP142) assay has also been approved as a companion diagnostics test for atezolizumab in UC and TNBC (109, 110). IHC 28–8 and SP263 (nivolumab and durvalumab, respectively) are complementary diagnostics and have not been approved by the FDA. Recent studies (e.g., shown in the meta-analysis) have also confirmed that efficacy of PD-1/PD-L1 inhibitors was more sensitive in PD-L1 positive patients compared to negative groups (111). Each PD-L1 IHC assay, performed in different IHC staining platforms, is independently developed for a specific anti-PD-1 or anti-PD-L1 drug. As is shown in Table 7, differences between six commonly used PD-L1 IHC assay were shown by detection system, staining platform, and antibody epitope. Thus, each assay potentially displayed different staining sensitivities. Different PD-L1 IHC assays and different PD-L1 tumor expression cut-off points are used in clinical trials, which raises concerns about whether the tests can be used interchangeably. The Blueprint PD-L1 IHC Assay Comparison Project was founded to enable a better understanding of the similarities and differences between these four PD-L1 IHC systems. This project is an industrial-academic partnership seeking to harmonize IHC PD-L1 testing. The result from phase I of the Blueprint project showed that the 22C3, 28-8, and SP263 assays displayed comparable sensitivity and the SP142 assay showed significantly less sensitivity (112). The phase I of the Blueprint project detect PD-L1 expression on TCs using four PD-L1 IHC assays performed in different staining platforms, and the result of staining was evaluated independently by three pathologists (112). Phase 2 of the Blueprint project compares 73-10 assay with four other PD-L1 IHC assay (including 22C3, 28-8, SP263). The results from phase 2 showed highly comparable sensitivity between 22C3, 28-8, and SP263 assays, less sensitivity with SP142 assay, and higher sensitivity with 73-10 assay when detecting PD-L1 expression on TC (113). The high concordance was observed between scorings by glass slide and scorings by digital image (113). Most importantly, a recent study has investigated the cause of distinct immunohistochemical staining generated by SP142 assay. The results suggested that discordances are more likely caused by differences of staining platform rather than antibody epitope (114).
The Current Potential Biomarkers Used to Evaluate the Feasibility of Anti-PD-1/PD-L1 Therapy
PD-1/PD-L1 inhibitors represent a breakthrough in cancer therapy. However, the response rates of PD-1/PD-L1 inhibitors in patients is, overall, unsatisfactory and results in limited applications in clinical practice. Therefore, searching for biomarkers predicting the efficacy of PD-1/PD-L1 inhibitors is crucial for patient selection. There are several biomarkers associated with the response to anti-PD-1/anti-PD-L1 therapy (Table 8) including PD-L1 expression, lactate dehydrogenase (LDH), mismatch-repair (MMR) deficiency, gene alteration, tumor mutational burden, etc. A clinical study conducted by Diem showed that patients with an elevated baseline LDH showed a significantly shorter OS (P = 0.0292) and lower response rate compared with patients with normal LDH at baseline and during treatment. This suggests that LDH could predict early response or progression in advanced melanoma patients with anti-PD-1 therapy (115, 116). In addition, patients who achieved clinical benefit after treatment of anti–PD-1 therapy were detected with a higher percentage of Bim+PD-1+CD8+ T-cells in the peripheral blood (117). The levels of Bim in PD-1+CD11ahiCD8+ T-cells (also indicated tumor reactive T cell) could be a predictive factor of clinical benefit in patients with metastatic melanoma treated with anti–PD-1 therapy (117). High pretreatment lymphocyte count (LC) and relative eosinophil count (REC) were associated with improved overall survival of melanoma patients with pembrolizumab treatment (118). Patients with T-cells expressing SRY-Box 2 (SOX-2) experienced disease regression following the treatment of nivolumab, suggesting that SOX-2 is associated with a clinical response upon immunotherapy with anti-PD-1 monoclonal antibodies (119). A retrospective study showed that the median PFS of patients with a neutrophil-lymphocyte ratio (NLR) of ≥3 was shorter than that in patients with a NLR of < 3 (2.0 vs. 5.3 months, p = 0.00515) at 4 weeks after treatment (120). The clinical data suggested that the NLR ratio might be an indicator of a poor prognosis in patients with advanced NSCLC receiving nivolumab (120). Patients with a 1.5-fold increase in circulating soluble PD-L1 (sPD-L1) concentrations were more likely to achieve partial responses to anti–PD-1 antibodies after 5 months upon anti-PD-1 therapy. This shows the predictive effect of sPD-L1 on clinical response to anti-PD-1 therapy (121). Among 36 EGFR-mutated metastatic NSCLC patients, compared with patients detecting decreased levels of sPD-1, patients with an increased or stable sPD-1 level achieved longer PFS (p = 0.004) and OS (p = 0.002) after two cycles of nivolumab (122). In melanoma, the pre-treatment tumors in responding patients were detected with higher expressions of IFN-γ and IFN-γ-inducible genes, including indoleamine 2,3-dioxygenase 1 (IDO1) and C-X-C motif Chemokine Ligand 9 (CXCL9) (123). These associations were also found in NSCLC or renal cell carcinoma patients (123). In addition, genetic aberrations within tumors were also found to be associated with clinical efficacy in anti-PD-1/PD-L1 therapy. For example, among 155 patients, six patients with MDM2/MDM4 amplification and seven of eight patients with Epidermal Growth Factor Receptor (EGFR) alterations were found to have time-to-treatment failure (TTF) <2 months (124). Meanwhile, hyper-progressors harbored MDM2/4 amplifications or EGFR alterations (124). A retrospective analysis showed that EGFR-mutant and ALK-positive NSCLC patients receiving anti-PD-1/PD-L1 therapy showed lower ORR (P = 0.053) (125). Immunotherapeutic analysis and prospective observation suggested that patients harboring TP53 or KRAS mutations—especially co-mutations of TP53/KRAS—showed significantly better clinical responses to anti-PD-1 therapy (126). Among the 174 lung adenocarcinoma (LUAC) patients with KRAS mutations, patients harboring (Serine/Threonine Kinase 11) STK11 alterations showed lower ORR to PD-1 inhibitors vs. LUAC patients with mutant KRAS and wildtype STK11 (P < 0.001) (127). Another study evaluated the clinical efficacy of PD-1 inhibitors in patients with MMR-deficient tumors across 12 tumor types. ORR was achieved in 53% of patients, disease control was achieved in 77% of patients, and complete responses were achieved in 21% of patients (71). The MMR deficiency was defined by the presence of either MSI-H or by loss of MutL Homolog 1 (MLH1), MutS Homolog 2 (MSH2), MutS Homolog 6 (MSH6), or PMS1 Homolog 2 (PMS2) protein expression. Among the 35 patients with clear cell renal cell carcinoma (ccRCC), a clinical benefit was associated with loss-of-function mutations in the Polybromo 1 (PBRM1) gene (p = 0.012) after treatment of pembrolizumab and nivolumab (128). The presence of DNA damage response gene (DDR) alteration was associated with a higher response rate (P < 0.001) (129). The most commonly altered genes were ATM (n = 7), DNA Polymerase Epsilon (POLE) (n = 3), and BRCA2, ERCC2, FA Complementation Group A (FANCA), and MutS Homolog 6 (MSH6) (n = 2) (129). Gene variations that occur in at least 1% of the population used to be called polymorphism. Single nucleotide polymorphisms (SNPs) of tumor microenvironment-related genes (including CCL2, NOS3, IL1RN, IL12B, CXCR3, and IL6R) were significantly associated with ORR of patients treated with anti-PD-1/PD-L1 therapies (130). And safety of anti-PD-1/PD- L1 targeted therapies was significantly associated with gene SNPs including UNG, IFNW1, CTLA4, PD-L1, and IFNL4 genes (130). Besides that, rs17388568, which maps to a locus of IL2 gene and IL21 gene, was correlated with a higher response to anti-PD-1 targeting therapy (131). CD8, PD-1, and PD-L1 expression in the tumor and at the invasive margin significantly correlated with treatment outcome (P = 0.001) (132). Versus the progression group, the response group had significantly higher numbers of CD8+, PD-1+, and PD-L1+ cells (CD8, P = 0.0001; PD-1, P = 0.0002; PD-L1, P = 0.006) (132). Among HNSCC patients treated with pembrolizumab, PD-L2-positive patients showed higher ORR compared with PD-L2-negative patients (133). And longer PFS and OS were observed in PD-L2–positive patients (133).
Except for the biomarkers mentioned above, the tumor mutation burden/load (TMB) also served as a predictive or prognostic factor for response to anti-PD-1/PD-L1 immunotherapy. TMB is an estimate of somatic mutations by accessing the data from whole exome sequencing (WES) or sequencing a select panel of genes. Foundation Medicine has developed clinical testing platforms to measure TMB using hybrid capture-based next generation sequencing. FDA has approved FoundationOne CDx to be used as a companion diagnostic for therapy selection. Several studies have shown that TMB is associated with a clinical response to anti-PD-1/PD-L1 treatment in melanoma and NSCLC (Table 9). Recently, a novel blood-based TMB (bTMB) assay was developed for cell-free DNA by researchers from Foundation Medicine. A retrospective analysis using bTMB assay showed that bTMB is correlated with significant PFS benefit (P = 0.013) and TMB (Spearman rank correlation = 0.64) in patients with NSCLC treated with atezolizumab (139). Neoantigens derived from mutated genes are tumor-specific and show significant correlation with the clinical response to anti-PD-1/PD-L1 treatment. A significantly higher candidate neoantigen burden was detected in patients with CB vs. those with NCB and associated with improved PFS (median 14.5 vs. 3.5 months, log-rank P = 0.002) (134). The PFS in patients with a higher non-synonymous burden were higher than those with low non-synonymous burden (median PFS 14.5 vs. 3.7 months, log-rank P = 0.01) (134). These data suggested that higher non-synonymous mutation or candidate neoantigen burden in tumors were associated with improved PFS of anti-PD-1-treated NSCLC patients. A recent study has shown that a minority of somatic mutations in tumors could lead to neoantigens and TMB could be used to estimate tumor neoantigen load (140).
Discovery of Small Molecule Compounds Inhibiting PD-1/PD-L1 Interactions
The limited success and disadvantage of antibodies prompted researchers to search for more effective strategies for PD-1/PD-L1 targeted therapy and improve the efficacy of cancer immunotherapy. Thus, studies on the discovery of low-molecular-weight compounds inhibiting PD-1/PD-L1 interaction have begun to attract scientist's attention. During the past 5 years, many companies, such as Arising International Inc, Chemocentryx Inc, Institute of Materia Medica, Guangzhou Maxinovel Pharmaceuticals Co, Incyte Corporation, Bristol Myers Squibb (BMS), and Aurigene, have discovered a series of small molecule chemical compounds and peptides.
Meanwhile, these companies have applied for a series of patents related to inhibitors (Table 10). Most of these patents presented not only the structure of PD-1/PD-L1 inhibitors, but also the method of compound synthesis and the use of inhibitors as immunomodulators. In addition, the patents showed verified inhibitory effects of these inhibitors. Some of these inhibitors could only block PD-L1/PD-1 interactions. Other inhibitors, such as the peptides discovered by BMS company, could inhibit interactions of PD-L1 with PD-1 or CD80. All inhibitors discovered by Aurigene, including small molecule chemical compounds and peptides, showed an inhibitory effect on the PD-1 signaling pathway.
BMS has published biphenyl derivatives as immunomodulators, and these are the first reported small compounds inhibiting PD-1/PD-L1 interaction. Interestingly, most of the inhibitory compounds showed IC50 values of 1 μM or even 0.018 μM as measured by the PD-1/PD-L1 homogenous time-resolved fluorescence (HTRF) binding assay (141). Further modification of the BMS compounds, such as hydrophobic modifications, enhanced the potency of compounds (lowest IC50 = 0.48 nM) (143). Moreover, the introduction of symmetric biaryl scaffolds could also improve binding affinities (lowest IC50 = 0.04 nM)(144). Arising International LLC published symmetric or semi-symmetric compounds as immunomodulators (IC50 values from 0.1 to 25 μM) (146, 147). ChemoCentryx reported 4-phenyl-2,3-dihydro-1H-inden-1-ol derivatives as inhibitors of the PD-1/PD-L1 interaction (147). The Institute of Materia Medica at the Chinese Academy of Medical Sciences has also discovered a series of bromo benzyl ether derivative and phenylate derivative blocking PD-1/PD-L1 interaction (IC50: 1 × 10−4 nM−1 nM) (149–151). Guangzhou Maxinovel Pharmaceuticals Co., Ltd reported that aromatic acetylene or aromatic ethylene compounds had a significant inhibitory effect on PD-1 and PD-L1 (152). A series of oxadiazole- and thiadiazole- compounds have been developed to inhibit the PD-1/PD-L1 pathway by Aurigene Discovery Technologies Limited (153–160). Incyte Corporation identified a series of heterocyclic compounds as inhibitors for PD-1/PD-L1 protein/protein interaction (IC50 values range from the nanomolar to micromolar) (161–169). Meanwhile, Aurigene Discovery Technologies Limited has designed a series of tripeptide peptidomimetics and developed cyclopeptides and macrocyclic-peptides based on peptidomimetics (171–176). Furthermore, BMS developed a series of macrocyclic peptides against the PD-1/PD-L1 pathway (177–184).
However, the discovery of PD-L1/PD-1 inhibitors has only just started. Nearly all inhibitors are still being investigated in preclinical studies. Only CA-170, a PD-L1 inhibitor discovered by Aurigene and Curis, has entered Phase I clinical trial (No: NCT02812875). This has shown acceptable safety of CA-170 (185). The phase II study of CA-170 showed a positive response in two patients with Hodgkin's lymphoma, and the clinical benefit rate is 68.18% (186). Due to its short half-life (6–8 h) vs. other long-lasting antibodies, CA-170 showed less sequalae after being permanently discontinued (186). In addition, preclinical data of the compound CCX4503, published by ChemoCentryx, markedly reduced tumor growth in a human melanoma/peripheral blood mononuclear cell co-implantation model. This preclinical result suggested that the small molecule inhibitors may offer effective anti-tumor therapy (187).
Discussion and Perspective
Anti-PD-1/PD-L1 antibodies have achieved success in the field of cancer immunotherapy during the past decade and mark a breakthrough in oncology. Eight antibodies blocking PD-1 and PD-L1 interactions have been approved for several indications. Despite the promising results reported in some clinical trials, limited drug efficacy caused by IRAEs has been observed and durable responses have been found in only a limited number of patients. In addition, immune-related adverse events caused by anti-PD-1 drugs have been reported in several clinical trials. Due to the limited successes and disadvantages of anti-PD-1/PD-L1 antibodies, more attention has been given to developing more effective strategies to improve clinical response rates. However, using PD-L1 expression as a biomarker of response is important in identifying patients who could obtain a positive clinical response from PD-1/PD-L1 targeted immunotherapy. The use of a single PD-L1 IHC assay with immunotherapy using a specific anti-PD-1/PD-L1 antibody would be one strategy for improving clinical trial outcomes. However, responses were also seen in patients with negative or low PD-L1 expression. For example, in three trials (CheckMate 017, CheckMate 025, and OAK), favorable long-term outcomes were achieved in PD-L1-negative patients (26, 188, 189). The CheckMate 227 trial among NSCLC patients with a high tumor mutational burden showed that progression-free survival was significantly longer with first line nivolumab plus ipilimumab than with chemotherapy, regardless of PD-L1 status (190). These studies also suggested that a higher mutation or neoantigen load could potentially result in a higher likelihood of response to PD-1 or PD-L1 inhibitors. Apart from TMB, there are several other biomarkers including LDH, MMR-deficiency, gene alteration, and IFN-γ related gene. These are useful biomarkers for the response to anti-PD-1/PD-L1 cancer therapy in solid tumors. Some studies have shown dynamic PD-L1 expression in the tumor cells further limits the feasibility of PD-L1 IHC (191). PD-L1 expression could be regulated through extrinsic and intrinsic signaling pathways such as mitogen-activated protein kinase (MAPK) signaling pathway, Janus kinase/signal transducers and activators of transcription (JAK/STAT) signaling pathway, miRNA-related pathway, as well as IFN-γ and TNF-α (192–194). An understanding of the mechanism of regulation of dynamic PD-L1 expression may be useful for developing novel strategies to improve the efficacy of anti-PD-1/PD-L1 drugs. On the other hand, small molecules are expected to reduce immune-related adverse events and promote higher efficacy. Studies on small molecule PD-1/PD-L1 inhibitors have just begun within the preclinical stage. CA-170 is the first PD-1/PD-L1 inhibitor successfully entering clinical trial, and it is potentially a small molecule PD-1/PD-L1 inhibitor in cancer therapy. Future clinical trial results of CA-170 would be important for developing small molecule inhibitors.
Author's Note
This review has made a summary about clinical studies and patent application of PD-1/PD-L1 targeted therapies. The paper has also shown the promising result of anti-PD-1/PD-L1 drug in various cancer types and several kinds of strategies improving efficacy of anti-PD-1/PD-L1 drug have been mentioned in the paper, including developing companion PD-L1 test, searching for biomarkers, and discovering small molecule PD-1/PD-L1 inhibitors. The paper has shown the development of anti-PD-1/PD-L1 therapies and provided broad knowledge of PD-1/PD-L1 targeted therapies.
Author Contributions
LG, RW, and HK contributed conception and design of the review article. LG organized the database collection. LG and RW wrote the first draft of the manuscript. HK wrote and revised sections of the manuscript. All authors contributed to manuscript revision, read and approved the submitted version.
Funding
This work was funded by the FDCT-MoST Joint Funding Project—Science and Technology Development Fund, Macau SAR (File no. 023/2015/AMJ), and International S&T Cooperation Program of China (File no. 2016YFE0121900).
Conflict of Interest
The authors declare that the research was conducted in the absence of any commercial or financial relationships that could be construed as a potential conflict of interest.
Acknowledgments
The authors would like to thank Ms. Ema Zivkovic who kindly reviewed the manuscript for English editing.
References
1. Agata Y, Kawasaki A, Nishimura H, Ishida Y, Tsubata T, Yagita H, et al. Expression of the PD-1 antigen on the surface of stimulated mouse T and B lymphocytes. Int Immunol. (1996) 8:765–72. doi: 10.1093/intimm/8.5.765
2. Gibbons JR, Dong H. Functional expression of programmed death-ligand 1 (B7-H1) by immune cells and tumor cells. Front Immunol. (2017) 8:961. doi: 10.3389/fimmu.2017.00961
3. Ni L, Lu J. Interferon gamma in cancer immunotherapy. Cancer Med. (2018) 7:4509–16. doi: 10.1002/cam4.1700
4. Granier C, De Guillebon E, Blanc C, Roussel H, Badoual C, Colin E, et al. Mechanisms of action and rationale for the use of checkpoint inhibitors in cancer. Esmo Open. (2017) 2:e000213. doi: 10.1136/esmoopen-2017-000213
5. Riley JL. PD-1 signaling in primary T cells. Immunol Rev. (2009) 229:114–25. doi: 10.1111/j.1600-065X.2009.00767.x
6. Patsoukis N, Sari D, Boussiotis VA. PD-1 inhibits T cell proliferation by upregulating p27 and p15 and suppressing Cdc25A. Cell Cycle. (2012) 11:4305–9. doi: 10.4161/cc.22135
7. Yang H, Zhang Q, Xu M, Wang L, Chen X, Feng Y, et al. CCL2-CCR2 axis recruits tumor associated macrophages to induce immune evasion through PD-1 signaling in esophageal carcinogenesis. Mol Cancer. (2020) 19:41. doi: 10.1186/s12943-020-01165-x
8. Nazareth MR, Broderick L, Simpson-Abelson MR, Kelleher RJJ, Yokota SJ, Bankert RB. Characterization of human lung tumor-associated fibroblasts and their ability to modulate the activation of tumor-associated T cells. J Immunol. (2007) 178:5552–62. doi: 10.4049/jimmunol.178.9.5552
9. Rozali EN, Hato SV, Robinson BW, Lake RA, Lesterhuis WJ. Programmed death ligand 2 in cancer-induced immune suppression. Clin Dev Immunol. (2012) 2012:656340. doi: 10.1155/2012/656340
10. Rollins MR, Gibbons Johnson RM. CD80 Expressed by CD8(+) T cells contributes to PD-L1-induced apoptosis of activated CD8(+) T cells. J Immunol Res. (2017) 2017:7659462. doi: 10.1155/2017/7659462
11. Honjo T, Ishida Y, Shinohara T. Peptide Related to Human Programmed Cell Death and DNA Encoding the Same. US5698520A. Alexandria, VA: USPTO (1997).
12. Okazaki T, Honjo T. PD-1 and PD-1 ligands: from discovery to clinical application. Int Immunol. (2007) 19:813–24. doi: 10.1093/intimm/dxm057
13. Seung E, Dudek TE, Allen TM, Freeman GJ, Luster AD, Tager AM. PD-1 Blockade in chronically HIV-1-infected humanized mice suppresses viral loads. PLoS ONE. (2013) 8:e77780. doi: 10.1371/journal.pone.0077780
14. Dong H, Zhu G, Tamada K, Chen L. B7-H1, a third member of the B7 family, co-stimulates T-cell proliferation and interleukin-10 secretion. Nat Med. (1999) 5:1365–9. doi: 10.1038/70932
15. Freeman GJ, Long AJ, Iwai Y, Bourque K, Chernova T, Nishimura H, et al. Engagement of the PD-1 immunoinhibitory receptor by a novel B7 family member leads to negative regulation of lymphocyte activation. J Exp Med. (2000) 192:1027–34. doi: 10.1084/jem.192.7.1027
16. Honjo T, Shibayama S, Takeda K, Matsuo M, Yoshida T, Miyamoto M. Substance Specific to Human PD-1. US7563869B2. Alexandria, VA: USPTO (2009).
17. Freeman G, Boussiotis V, Chernova T, Malenkovich N. B7-4 Polypeptides and Uses Therefor. US7038013B2. Alexandria, VA: USPTO (2006).
18. Wood C, Freeman GJ. PD-1, a Receptor for B7-4, and Uses Therefor. US7101550B2. Alexandria, VA: USPTO (2006).
19. Freeman G, Sharpe A, Dorfman DM, Ahmed R, Barber D, Wherry EJ. Methods and Compositions for the Treatment of Persistent Infections. US8652465B2. Alexandria, VA: USPTO (2014).
20. Wood C, Freeman GJ. Downmodulating an Immune Response With Multivalent Antibodies to PD-1. US6808710B1. Alexandria, VA: USPTO (2004).
23. Chen L, Strome SE, Kwon ED. B7-H1 and Methods of Diagnosis, Prognosis, and Treatment of Cancer. US7892540B2. Alexandria, VA: USPTO (2011).
24. Lyford-Pike S, Peng S, Young GD, Taube JM, Westra WH, Akpeng B, et al. Evidence for a role of the PD-1:PD-L1 pathway in immune resistance of HPV-associated head and neck squamous cell carcinoma. Cancer Res. (2013) 73:1733–41. doi: 10.1158/0008-5472.CAN-12-2384
25. Dong H, Strome SE, Salomao DR, Tamura H, Hirano F, Flies DB, et al. Tumor-associated B7-H1 promotes T-cell apoptosis: a potential mechanism of immune evasion. Nat Med. (2002) 8:793–800. doi: 10.1038/nm730
26. Robert C, Long GV, Brady B, Dutriaux C, Maio M, Mortier L, et al. Nivolumab in previously untreated melanoma without BRAF mutation. New Engl J Med. (2015) 372:320–30. doi: 10.1056/NEJMoa1412082
27. Schadendorf D, Hodi FS, Robert C, Weber JS, Margolin K, Hamid O, et al. Pooled analysis of long-term survival data from phase II and Phase III trials of ipilimumab in unresectable or metastatic melanoma. J Clin Oncol. (2015) 33:1889–94. doi: 10.1200/JCO.2014.56.2736
28. Honjo T, Minato N, Iwai Y, Shibayama S. Method for Treatment of Cancer by Inhibiting the Immunosuppressive Signal Induced by PD-1. US7595048B2. Alexandria, VA: USPTO (2004).
29. Carven GJ, van Eenennaam H, Dulos GJ. Antibodies to Human Programmed Death Receptor PD-1. US8952136B2. Alexandria, VA: USPTO (2013).
30. Nastri HG, Iffland C, Leger O, An Q, Cartwright M, McKenna SD, et al. Anti-pd-l1 Antibodies and Uses Thereof. US20140341917A1. Alexandria, VA: USPTO (2014).
31. Irving B, Chiu H, Maecker H, Mariathasan S, Lehar SM, Wu Y, et al. Anti-PD-L1 Antibodies, Compositions and Articles of Manufacture. US8217149B2. Alexandria, VA: USPTO (2010).
32. Queva C, Morrow M, Hammond S, Alimzhanov M, Babcook J, Foltz IN, et al. Targeted Binding Agents Against B7-H1. US8779108B2. Alexandria, VA: USPTO (2014).
33. Freeman GJ, Sharpe AH, Blattler WA, Mataraza JM, Sabatos-peyton CA, Chang HW, et al. Antibody Molecules to PD-1 and Uses Thereof. WO2015112900. Geneva: WIPO (2015).
34. Papadopoulos NJ, Murphy AJ, Thurston G, Burova EI. Human Antibodies to PD-1. US20150203579A1. Alexandria, VA: USPTO (2015).
35. Yuan J, Qu X, Lin J, Ye X, Cao G, Tao W, et al. Pd-1 Antibody, Antigen-Binding Fragment Thereof, and Medical Application Thereof. US20160376367A1. Alexandria, VA: USPTO (2016).
36. Li K, Zhang T, Song J, Xu L, Liu Q, Peng H. Anti-PD1 Antibodies and Their Use as Therapeutics and Diagnostics. US20150079109A1. Alexandria, VA: USPTO (2015).
37. King DJ, Kehry M. Antibodies Directed Against Programmed Death-1 (PD-1). US9815897B2. Alexandria, VA: USPTO (2017).
38. Langermann S, Liu L. Compositions of PD-1 Antagonists And Methods of Use. US8609089B2. Alexandria, VA: USPTO (2013).
39. Wang J, Dong L, Wang Y. Anti-pd-1 Antibodies and Therapeutic Uses Thereof. US20180346569A1. Alexandria, VA: USPTO (2018).
40. Langermann S. Simultaneous Inhibition of pd-l1/pd-l2. US20130017199A1. Alexandria, VA: USPTO (2013).
41. West JW, Mei L, Moore SJ, Nguyen MTL, Hostetter DR, Vasiljeva O, et al. Anti-pdl1 Antibodies, Activatable Anti-pdl1 Antibodies, and Methods of Use Thereof. US20160311903A1. Alexandria, VA: USPTO (2016).
42. Korman AJ, Selby MJ, Wang C, Srinivasan M, Passmore DB, Huang H, et al. Human Monoclonal Antibodies to Programmed Death Ligand 1 (PD-L1). US7943743B2. Alexandria, VA: USPTO (2011).
43. Xu T, Dong Y, Wang P, Chen T. Single Domain Antibody and Derivative Proteins Thereof Against Programmed Death-Ligand (pdl1). US20180327494A1. Alexandria, VA: USPTO (2018).
44. Dumet C, Pottier J, Gouilleux-Gruart V, Watier H. Insights into the IgG heavy chain engineering patent landscape as applied to IgG4 antibody development. Mabs-Austin. (2019) 11:1341–50. doi: 10.1080/19420862.2019.1664365
45. Qin SK, Finn RS, Kudo M, Meyer T, Vogel A, Ducreux M, et al. RATIONALE 301 study: tislelizumab versus sorafenib as first-line treatment for unresectable hepatocellular carcinoma. Future Oncol. (2019) 15:1811–22. doi: 10.2217/fon-2019-0097
46. Floudas CS, Brar G, Mabry-Hrones D, Duffy AG, Wood B, Levy E, et al. A pilot study of the PD-1 targeting agent AMP-224 used with low-dose cyclophosphamide and stereotactic body radiation therapy in patients with metastatic colorectal cancer. Clin Colorectal Canc. (2019) 18:e349–60. doi: 10.1016/j.clcc.2019.06.004
47. Tan S, Zhang H, Chai Y, Song H, Tong Z, Wang Q, et al. An unexpected N-terminal loop in PD-1 dominates binding by nivolumab. Nat Commun. (2017) 8:14369. doi: 10.1038/ncomms14369
48. Na Z, Yeo SP, Bharath SR, Bowler MW, Balikci E, Wang CI, et al. Structural basis for blocking PD-1-mediated immune suppression by therapeutic antibody pembrolizumab. Cell Res. (2017) 27:147–50. doi: 10.1038/cr.2016.77
49. Finlay WJ, Coleman JE, Edwards JS, Johnson KS. Anti-PD1 ‘SHR-1210’ aberrantly targets pro-angiogenic receptors and this polyspecificity can be ablated by paratope refinement. In: Proceedings of Mabs-Austin. (2019) 11:26–44. doi: 10.1080/19420862.2018.1550321
50. Chen X, Song X, Li K, Zhang TJF. FcγR-binding is an important functional attribute for immune checkpoint antibodies in cancer immunotherapy. Front Immunol. (2019) 10:292. doi: 10.3389/fimmu.2019.00292
51. Zhang F, Wei H, Wang X, Bai Y, Wang P, Wu J, et al. Structural basis of a novel PD-L1 nanobody for immune checkpoint blockade. Cell Discov. (2017) 3:17004. doi: 10.1038/celldisc.2017.4
52. Autio KA, Boni V, Humphrey RW, Naing A. Probody therapeutics: an emerging class of therapies designed to enhance on-target effects with reduced off-tumor toxicity for use in immuno-oncology. Clin Canc. Res. (2019) 26:984–89. doi: 10.1158/1078-0432.CCR-19-1457
53. Tan S, Liu K, Chai Y, Zhang CW, Gao S, Gao GF, et al. Distinct PD-L1 binding characteristics of therapeutic monoclonal antibody durvalumab. Protein Cell. (2018) 9:135–9. doi: 10.1007/s13238-017-0412-8
54. Zak KM, Grudnik P, Magiera K, Domling A, Dubin G, Holak TA. Structural biology of the immune checkpoint receptor PD-1 and its ligands PD-L1/PD-L2. Structure. (2017) 25:1163–74. doi: 10.1016/j.str.2017.06.011
55. Overman MJ, McDermott R, Leach JL, Lonardi S, Lenz HJ, Morse MA, et al. Nivolumab in patients with metastatic DNA mismatch repair-deficient or microsatellite instability-high colorectal cancer (CheckMate 142): an open-label, multicentre, phase 2 study. Lancet Oncol. (2017) 18:1182–91.
56. Weber JS, D'Angelo SP, Minor D, Hodi FS, Gutzmer R, Neyns B, et al. Nivolumab versus chemotherapy in patients with advanced melanoma who progressed after anti-CTLA-4 treatment (CheckMate 037): a randomised, controlled, open-label, phase 3 trial. Lancet Oncol. (2015) 16:375–84. doi: 10.1016/S1470-2045(15)70076-8
57. Brahmer J, Reckamp KL, Baas P, Crino L, Eberhardt WEE, Poddubskaya E, et al. Nivolumab versus docetaxel in advanced squamous-cell non-small-cell lung cancer. New Engl J Med. (2015) 373:123–35. doi: 10.1056/NEJMoa1504627
58. Borghaei H, Paz-Ares L, Horn L, Spigel DR, Steins M, Ready NE, et al. Nivolumab versus docetaxel in advanced nonsquamous non-small-cell lung cancer. New Engl J Med. (2015) 373:1627–39. doi: 10.1056/NEJMoa1507643
59. Sharma P, Retz M, Siefker-Radtke A, Baron A, Necchi A, Bedke J, et al. Nivolumab in metastatic urothelial carcinoma after platinum therapy (CheckMate 275): a multicentre, single-arm, phase 2 trial. Lancet Oncol. (2017) 18:312–22. doi: 10.1016/S1470-2045(17)30065-7
60. Motzer RJ, Escudier B, McDermott DF, George S, Hammers HJ, Srinivas S, et al. Nivolumab versus everolimus in advanced renal-cell carcinoma. New Engl J Med. (2015) 373:1803–13. doi: 10.1056/NEJMoa1510665
61. Ansell SM, Lesokhin AM, Borrello I, Halwani A, Scott EC, Gutierrez M, et al. PD-1 blockade with nivolumab in relapsed or refractory Hodgkin's lymphoma. N Engl J Med. (2015) 372:311–9. doi: 10.1056/NEJMoa1411087
62. Younes A, Santoro A, Shipp M, Zinzani PL, Timmerman JM, Ansell S, et al. Nivolumab for classical Hodgkin's lymphoma after failure of both autologous stem-cell transplantation and brentuximab vedotin: a multicentre, multicohort, single-arm phase 2 trial. Lancet Oncol. (2016) 17:1283–94. doi: 10.1016/S1470-2045(16)30167-X
63. El-Khoueiry AB, Sangro B, Yau T, Crocenzi TS, Kudo M, Hsu C, et al. Nivolumab in patients with advanced hepatocellular carcinoma (CheckMate 040): an open-label, non-comparative, phase 1/2 dose escalation and expansion trial. Lancet. (2017) 389:2492–502. doi: 10.1016/S0140-6736(17)31046-2
64. Ferris RL, Blumenschein G, Fayette J, Guigay J, Colevas AD, Licitra L, et al. Nivolumab for recurrent squamous-cell carcinoma of the head and neck. New Engl J Med. (2016) 375:1856–67. doi: 10.1056/NEJMoa1602252
65. Hamid O, Robert C, Daud A, Hodi FS, Hwu WJ, Kefford R, et al. Safety and tumor responses with lambrolizumab (Anti-PD-1) in melanoma. New Engl J Med. (2013) 369:134–44. doi: 10.1056/NEJMoa1305133
66. Robert C, Ribas A, Wolchok JD, Hodi FS, Hamid O, Kefford R, et al. Anti-programmed-death-receptor-1 treatment with pembrolizumab in ipilimumab-refractory advanced melanoma: a randomised dose-comparison cohort of a phase 1 trial. Lancet. (2014) 384:1109–17. doi: 10.1016/S0140-6736(14)60958-2
67. Sul J, Blumenthal GM, Jiang X, He K, Keegan P, Pazdur R. FDA approval summary: pembrolizumab for the treatment of patients with metastatic non-small cell lung cancer whose tumors express programmed death-ligand 1. Oncologist. (2016) 21:643–50. doi: 10.1634/theoncologist.2015-0498
68. Balar AV, Castellano D, O'Donnell PH, Grivas P, Vuky J, Powles T, et al. First-line pembrolizumab in cisplatin-ineligible patients with locally advanced and unresectable or metastatic urothelial cancer (KEYNOTE-052): a multicentre, single-arm, phase 2 study. Lancet Oncol. (2017) 18:1483–92. doi: 10.1016/S1470-2045(17)30616-2
69. Bellmunt J, de Wit R, Vaughn DJ, Fradet Y, Lee JL, Fong L, et al. Pembrolizumab as second-line therapy for advanced urothelial carcinoma. New Engl J Med. (2017) 376:1015–26. doi: 10.1056/NEJMoa1613683
70. Seiwert TY, Burtness B, Mehra R, Weiss J, Berger R, Eder JP, et al. Safety and clinical activity of pembrolizumab for treatment of recurrent or metastatic squamous cell carcinoma of the head and neck (KEYNOTE-012): an open-label, multicentre, phase 1b trial. Lancet Oncol. (2016) 17:956–65. doi: 10.1016/S1470-2045(16)30066-3
71. Le DT, Durham JN, Smith KN, Wang H, Bartlett BR, Aulakh LK, et al. Mismatch repair deficiency predicts response of solid tumors to PD-1 blockade. Science. (2017) 357:409–13. doi: 10.1126/science.aan6733
72. Fuchs CS, Doi T, Jang RWJ, Muro K, Satoh T, Machado M, et al. KEYNOTE-059 cohort 1: efficacy and safety of pembrolizumab (pembro) monotherapy in patients with previously treated advanced gastric cancer. J Clin Oncol. (2017) 35:4003. doi: 10.1200/JCO.2017.35.15_suppl.4003
73. Chung HC, Schellens JHM, Delord JP, Perets R, Italiano A, Shapira-Frommer R. Pembrolizumab treatment of advanced cervical cancer: updated results from the phase 2 KEYNOTE-158 study. J Clin Oncol. (2018) 36:5522. doi: 10.1200/JCO.2018.36.15_suppl.5522
74. Apolo AB, Infante JR, Balmanoukian A, Patel MR, Wang D, Kelly K, et al. Avelumab, an anti-programmed death-ligand 1 antibody, in patients with refractory metastatic urothelial carcinoma: results from a multicenter, phase Ib study. J Clin Oncol. (2017) 35:2117–24. doi: 10.1200/JCO.2016.71.6795
75. Kaufman HL, Russell J, Hamid O, Bhatia S, Terheyden P, D'Angelo SP, et al. Avelumab in patients with chemotherapy-refractory metastatic Merkel cell carcinoma: a multicentre, single-group, open-label, phase 2 trial. Lancet Oncol. (2016) 17:1374–85. doi: 10.1016/S1470-2045(16)30364-3
76. Fehrenbacher L, Spira A, Ballinger M, Kowanetz M, Vansteenkiste J, Mazieres J, et al. Atezolizumab versus docetaxel for patients with previously treated non-small-cell lung cancer (POPLAR): a multicentre, open-label, phase 2 randomised controlled trial. Lancet. (2016) 387:1837–46. doi: 10.1016/S0140-6736(16)00587-0
77. Rittmeyer A, Barlesi F, Waterkamp D, Park K, Ciardiello F, von Pawel J, et al. Atezolizumab versus docetaxel in patients with previously treated non-small-cell lung cancer (OAK): a phase 3, open-label, multicentre randomised controlled trial. Lancet. (2017) 389:255–65. doi: 10.1016/S0140-6736(16)32517-X
78. Rosenberg JE, Hoffman-Censits J, Powles T, van der Heijden MS, Balar AV, Necchi A, et al. Atezolizumab in patients with locally advanced and metastatic urothelial carcinoma who have progressed following treatment with platinum-based chemotherapy: a single-arm, multicentre, phase 2 trial. Lancet. (2016) 387:1909–20. doi: 10.1016/S0140-6736(16)00561-4
79. Antonia SJ, Villegas A, Daniel D, Vicente D, Murakami S, Hui R, et al. Durvalumab after chemoradiotherapy in stage III non-small-cell lung cancer. N Engl J Med. (2017) 377:1919–29. doi: 10.1056/NEJMoa1709937
80. Powles T, O'Donnell PH, Massard C, Arkenau HT, Friedlander TW, Hoimes CJ, et al. Efficacy and safety of durvalumab in locally advanced or metastatic urothelial carcinoma: updated results from a phase 1/2 open-label study. JAMA Oncol. (2017) 3:e172411. doi: 10.1001/jamaoncol.2017.2411
81. Migden MR, Rischin D, Schmults CD, Guminski A, Hauschild A, Lewis KD, et al. PD-1 blockade with cemiplimab in advanced cutaneous squamous-cell carcinoma. N Engl J Med. (2018) 379:341–51. doi: 10.1056/NEJMoa1805131
82. Westin JR, Chu F, Zhang M, Fayad LE, Kwak LW, Fowler N, et al. Safety and activity of PD1 blockade by pidilizumab in combination with rituximab in patients with relapsed follicular lymphoma: a single group, open-label, phase 2 trial. Lancet Oncol. (2014) 15:69–77. doi: 10.1016/S1470-2045(13)70551-5
83. Long GV, Lebbe C, Atkinson V, Mandalà M, Nathan PD, Arance Fernandez AM, et al. The anti–PD-1 antibody spartalizumab (S) in combination with dabrafenib (D) and trametinib (T) in previously untreated patients (pts) with advanced BRAF V600–mutant melanoma: Updated efficacy and safety from parts 1 and 2 of COMBI-i. In: Proceedings of 2019 ASCO Annual Meeting, Chicago, IL (2019).
84. Fang W, Yang Y, Ma Y, Hong S, Lin L, He X, et al. Camrelizumab (SHR-1210) alone or in combination with gemcitabine plus cisplatin for nasopharyngeal carcinoma: results from two single-arm, phase 1 trials. Lancet Oncol. (2018) 19:1338–50. doi: 10.1016/S1470-2045(18)30495-9
85. Wang S, Huang X, Bai Y-X, Yuan Y, Li J, Wang Z, et al. Preliminary results with tislelizumab, an investigational anti-PD-1 antibody, in Chinese patients with nasopharyngeal cancer (NPC). In: Proceedings of 2019 ASCO Annual Meeting, Chicago, IL (2019).
86. Chi Z, Tang B, Sheng X, Si L, Cui C, Kong Y, et al. A phase II study of JS001, a humanized PD-1 mAb, in patients with advanced melanoma in China. In: Proceedings of 2018 ASCO Annual Meeting. Chicago, IL (2018) 1–5.
87. Moreno V, Barretina-Ginesta M-P, Guo W, Lu S, Jenkins D, McEachern K, et al. Abstract CT053: preliminary safety, efficacy, and PK/PD characterization from GARNET, a phase 1 clinical trial of the anti-PD-1 monoclonal antibody, TSR-042, in patients with recurrent or advanced NSCLC and MSI-H endometrial cancer. In: Proceedings of AACR Annual Meeting 2018, Chicago, IL (2018).
88. Moore KN, Dresher C, Liu J, O'Malley DM, Wang EW, Wang JS-Z, et al. Phase 1/2 open-label, multiple ascending dose trial of AGEN2034, an anti-PD-1 monoclonal antibody, in advanced solid malignancies: results of dose escalation. In: Proceedings of 2018 ASCO Annual Meeting, Chicago, IL (2018).
89. Shi Y, Su H, Song Y, Jiang W, Sun X, Qian W, et al. Safety and activity of sintilimab in patients with relapsed or refractory classical Hodgkin lymphoma (ORIENT-1): a multicentre, single-arm, phase 2 trial. Lancet Haematol. (2019) 6:e12–9. doi: 10.1016/S2352-3026(18)30192-3
90. Fogt S, Shustova M, Demidov LV, Moiseyenko V, Tjulandin S, Semiglazova T, et al. Phase II trial (BCD-100-2/MIRACULUM) of the novel PD-1 inhibitor (BCD-100) in patients with advanced melanoma. In: Proceedings of 2019 ASCO Annual Meeting, Chicago, IL (2019).
91. Song Y, Zhu J, Lin N, Zhang C, Zhang M, Bai H, et al. GLS-010, a novel anti-PD-1 mAb in Chinese patients with relapsed or refractory classical Hodgkin's lymphoma: preliminary result of a phase II clinical trial. In: Proceedings of 2019 ASCO Annual Meeting, Chicago, IL (2019).
92. Boni V, Garcia-Corbacho J, Ott PA, Cho D, Autio KA, Uboha N, et al. Preliminary results of PROCLAIM-CX-072: the first-in-human, dose-finding trial of PD-L1 probody therapeutic CX-072 as monotherapy in patients (pts) with advanced solid tumors. In: Proceedings of ESMO 2018 Congress, Munich (2018).
93. Shen L, Cao J, Li J, Pan H, Xu N, Zhang Y, et al. A phase Ia/Ib trial of the anti-PD-L1 human monoclonal antibody (mAb), CS1001, in patients (pts) with advanced solid tumors or lymphomas. In: Proceedings of 2019 ASCO Annual Meeting, Chicago, IL (2019).
94. Checkpoint Therapeutics Announces Positive Interim Clinical Results of Anti-PD-L1 Antibody Cosibelimab. Availabe online at: https://www.globenewswire.com/news-release/2019/05/01/1813426/0/en/Checkpoint-Therapeutics-Announces-Positive-Interim-Clinical-Results-of-Anti-PD-L1-Antibody-Cosibelimab.html (accessed May 01, 2019).
95. Nie J, Wang C, Liu Y, Yang Q, Mei Q, Dong L, et al. Addition of low-dose decitabine to anti–PD-1 antibody camrelizumab in relapsed/refractory classical hodgkin lymphoma. J Clin Oncol. (2019) 37:1479–89. doi: 10.1200/JCO.18.02151
96. Keam SJ. Toripalimab: first global approval. Drugs. (2019) 79:573–8. doi: 10.1007/s40265-019-01076-2
97. Topalian SL, Hodi FS, Brahmer JR, Gettinger SN, Smith DC, McDermott DF, et al. Safety, activity, and immune correlates of anti-PD-1 antibody in cancer. N Engl J Med. (2012) 366:2443–54. doi: 10.1056/NEJMoa1200690
98. Man J, Ritchie G, Links M, Lord S, Lee CK. Treatment-related toxicities of immune checkpoint inhibitors in advanced cancers: a meta-analysis. Asia Pac J Clin Oncol. (2018) 14:141–52. doi: 10.1111/ajco.12838
99. Postow MA, Callahan MK, Wolchok JD. Immune checkpoint blockade in cancer therapy. J Clin Oncol. (2015) 33:1974–82. doi: 10.1200/JCO.2014.59.4358
100. Cree IA, Booton R, Cane P, Gosney J, Ibrahim M, Kerr K, et al. PD-L1 testing for lung cancer in the UK: recognizing the challenges for implementation. Histopathology. (2016) 69:177–86. doi: 10.1111/his.12996
101. Pierce RH, Bourne P, Liang L, Bigler M. Antibodies That Bind to Human Programmed Death Ligand 1 (PD-L1). US9709568B2. Alexandria, VA: USPTO (2017).
102. Cogswell JP, Goldberg SM, Gupta AK, Jure-Kunkel M, Wang XT, Wigginton JM. Cancer Immunotherapy by Disrupting pd-1/pd-l1 Signaling. WO2013173223A1. Geneva: WIPO (2013).
103. Nitta H, Vennapusa B, Dennis E. Multiplex Assay for Improved Scoring of Tumor Tissues Stained for pd-l1. WO2015181343A2. Geneva: WIPO (2015).
104. Hui R, Garon E, Goldman J, Leighl N, Hellmann M, Patnaik A, et al. Pembrolizumab as first-line therapy for patients with PD-L1-positive advanced non-small cell lung cancer: a phase 1 trial. Ann Oncol. (2017) 28:874–81. doi: 10.1093/annonc/mdx008
105. Wainberg Z, Jalal S, Muro K, Yoon H, Garrido M, Golan T, et al. LBA28_PRKEYNOTE-059 update: efficacy and safety of pembrolizumab alone or in combination with chemotherapy in patients with advanced gastric or gastroesophageal (G/GEJ) cancer. Ann Oncol. (2017) 28:616–17. doi: 10.1093/annonc/mdx440.020
106. Chung HC, Ros W, Delord J-P, Perets R, Italiano A, Shapira-Frommer R, et al. Efficacy and safety of pembrolizumab in previously treated advanced cervical cancer: results from the phase II KEYNOTE-158 study. J Clin Oncol. (2019) 37:1470–8. doi: 10.1200/JCO.18.01265
107. Burtness B, Harrington K, Greil R, Soulières D, Tahara M, De Castro G Jr, et al. LBA8_PR KEYNOTE-048: phase III study of first-line pembrolizumab (P) for recurrent/metastatic head and neck squamous cell carcinoma (R/M HNSCC). ESMO Meeting. (2018) 29:mdy424. 045. doi: 10.1093/annonc/mdy424.045
108. Kojima T, Muro K, Francois E, Hsu C-H, Moriwaki T, Kim S-B, et al. Pembrolizumab versus chemotherapy as second-line therapy for advanced esophageal cancer: phase III KEYNOTE-181 study. In: Proceedings of 2019 Gastrointestinal Cancers Symposium, San Francisco, CA (2019).
109. Aggen DH, Drake CG. Biomarkers for immunotherapy in bladder cancer: a moving target. J Immunother Cancer. (2017) 5:94. doi: 10.1186/s40425-017-0299-1
110. Emens LA, Cruz C, Eder JP, Braiteh F, Chung C, Tolaney SM, et al. Long-term clinical outcomes and biomarker analyses of atezolizumab therapy for patients with metastatic triple-negative breast cancer: a phase 1 study. JAMA Oncol. (2019) 5:74–82. doi: 10.1001/jamaoncol.2018.4224
111. Shen X, Zhao B. Efficacy of PD-1 or PD-L1 inhibitors and PD-L1 expression status in cancer: meta-analysis. BMJ. (2018) 362:k3529. doi: 10.1136/bmj.k3529
112. Hirsch FR, McElhinny A, Stanforth D, Ranger-Moore J, Jansson M, Kulangara K, et al. PD-L1 immunohistochemistry assays for lung cancer: results from phase 1 of the blueprint PD-L1 IHC assay comparison project. J Thorac Oncol. (2017) 12:208–22. doi: 10.1016/j.jtho.2016.11.2228
113. Tsao MS, Kerr KM, Kockx M, Beasley MB, Borczuk AC, Botling J, et al. PD-L1 immunohistochemistry comparability study in real-life clinical samples: results of blueprint phase 2 project. J Thorac Oncol. (2018) 13:1302–11. doi: 10.1016/j.jtho.2018.05.013
114. Lawson NL, Dix CI, Scorer PW, Stubbs CJ, Wong EM, Hutchinson L, et al. Mapping the binding sites of antibodies utilized in programmed cell death ligand-1 predictive immunohistochemical assays for use with immuno-oncology therapies. Modern Pathol. (2019) 33:758–8. doi: 10.1038/s41379-019-0379-5
115. Diem S, Kasenda B, Spain L, Martin-Liberal J, Marconcini R, Gore M, et al. Serum lactate dehydrogenase as an early marker for outcome in patients treated with anti-PD-1 therapy in metastatic melanoma. Br J Cancer. (2016) 114:256–61. doi: 10.1038/bjc.2015.467
116. Ribas A, Hamid O, Daud A, Hodi FS, Wolchok JD, Kefford R, et al. Association of pembrolizumab with tumor response and survival among patients with advanced melanoma. JAMA. (2016) 315:1600–9. doi: 10.1001/jama.2016.4059
117. Dronca RS, Liu X, Harrington SM, Chen L, Cao S, Kottschade LA, et al. T cell Bim levels reflect responses to anti–PD-1 cancer therapy. JCI Insight. (2016) 1:e86014. doi: 10.1172/jci.insight.86014
118. Weide B, Martens A, Hassel JC, Berking C, Postow MA, Bisschop K, et al. Baseline biomarkers for outcome of melanoma patients treated with pembrolizumab. Clin Cancer Res. (2016) 22:5487–96. doi: 10.1158/1078-0432.CCR-16-0127
119. Dhodapkar KM, Gettinger SN, Das R, Zebroski H, Dhodapkar MVJO. SOX2-specific adaptive immunity and response to immunotherapy in non-small cell lung cancer. Oncoimmunology. (2013) 2:e25205. doi: 10.4161/onci.25205
120. Nakaya A, Kurata T, Yoshioka H, Takeyasu Y, Niki M, Kibata K, et al. Neutrophil-to-lymphocyte ratio as an early marker of outcomes in patients with advanced non-small-cell lung cancer treated with nivolumab. Int J Clin Oncol. (2018) 23:634–40. doi: 10.1007/s10147-018-1250-2
121. Zhou J, Mahoney KM, Giobbie-Hurder A, Zhao F, Lee S, Liao X, et al. Soluble PD-L1 as a biomarker in malignant melanoma treated with checkpoint blockade. Cancer Immunol Res. (2017) 5:480–92. doi: 10.1158/2326-6066.CIR-16-0329
122. Tiako Meyo M, Jouinot A, Giroux-Leprieur E, Fabre E, Wislez M, Alifano M, et al. Predictive value of soluble PD-1, PD-L1, VEGFA, CD40 ligand and CD44 for nivolumab therapy in advanced non-small cell lung cancer: a case-control study. Cancers. (2020) 12:473. doi: 10.3390/cancers12020473
123. Herbst RS, Soria J-C, Kowanetz M, Fine GD, Hamid O, Gordon MS, et al. Predictive correlates of response to the anti-PD-L1 antibody MPDL3280A in cancer patients. Nature. (2014) 515:563–7. doi: 10.1038/nature14011
124. Kato S, Goodman A, Walavalkar V, Barkauskas DA, Sharabi A, Kurzrock RJCCR. Hyperprogressors after immunotherapy: analysis of genomic alterations associated with accelerated growth rate. Clin Cancer Res. (2017) 23:4242–50. doi: 10.1158/1078-0432.CCR-16-3133
125. Gainor JF, Shaw AT, Sequist LV, Fu X, Azzoli CG, Piotrowska Z, et al. EGFR mutations and ALK rearrangements are associated with low response rates to PD-1 pathway blockade in non–small cell lung cancer: a retrospective analysis. Clin Cancer Res. (2016) 22:4585–93. doi: 10.1158/1078-0432.CCR-15-3101
126. Dong Z, Zhong W, Zhang X, Su J, Xie Z, Liu S, et al. Potential predictive value of TP53 and KRAS mutation status for response to PD-1 blockade immunotherapy in lung adenocarcinoma. Clin Cancer Res. (2017) 23:3012–24. doi: 10.1016/j.jtho.2016.11.504
127. Skoulidis F, Goldberg ME, Greenawalt DM, Hellmann MD, Awad MM, Gainor JF, et al. STK11/LKB1 mutations and PD-1 inhibitor resistance in KRAS-mutant lung adenocarcinoma. Cancer Discov. (2018) 8:822–35. doi: 10.1158/2159-8290.CD-18-0099
128. Miao D, Margolis CA, Gao W, Voss MH, Li W, Martini DJ, et al. Genomic correlates of response to immune checkpoint therapies in clear cell renal cell carcinoma. Science. (2018) 359:801–6. doi: 10.1126/science.aan5951
129. Teo MY, Seier K, Ostrovnaya I, Regazzi AM, Kania BE, Moran MM, et al. Alterations in DNA damage response and repair genes as potential marker of clinical benefit from PD-1/PD-L1 blockade in advanced urothelial cancers. J CLin Oncol. (2018) 36:1685–94. doi: 10.1200/JCO.2017.75.7740
130. Refae S, Gal J, Ebran N, Otto J, Borchiellini D, Peyrade F, et al. Germinal Immunogenetics predict treatment outcome for PD-1/PD-L1 checkpoint inhibitors. Invest New Drugs. (2020) 38:160–71. doi: 10.1007/s10637-019-00845-w
131. Chat V, Ferguson R, Simpson D, Kazlow E, Lax R, Moran U, et al. Autoimmune genetic risk variants as germline biomarkers of response to melanoma immune-checkpoint inhibition. Cancer Immunol Immun. (2019) 68:897–905. doi: 10.1007/s00262-019-02318-8
132. Tumeh PC, Harview CL, Yearley JH, Shintaku IP, Taylor EJ, Robert L, et al. PD-1 blockade induces responses by inhibiting adaptive immune resistance. Nature. (2014) 515:568–71. doi: 10.1038/nature13954
133. Yearley JH, Gibson C, Yu N, Moon C, Murphy E, Juco J, et al. PD-L2 expression in human tumors: relevance to anti-PD-1 therapy in cancer. Clin Cancer Res. (2017) 23:3158–67. doi: 10.1158/1078-0432.CCR-16-1761
134. Rizvi NA, Hellmann MD, Snyder A, Kvistborg P, Makarov V, Havel JJ, et al. Mutational landscape determines sensitivity to PD-1 blockade in non–small cell lung cancer. Science. (2015) 348:124–8. doi: 10.1126/science.aaa1348
135. Campesato LF, Barroso-Sousa R, Jimenez L, Correa BR, Sabbaga J, Hoff PM, et al. Comprehensive cancer-gene panels can be used to estimate mutational load and predict clinical benefit to PD-1 blockade in clinical practice. Oncotarget. (2015) 6:34221. doi: 10.18632/oncotarget.5950
136. Hellmann MD, Callahan MK, Awad MM, Calvo E, Ascierto PA, Atmaca A, et al. Tumor mutational burden and efficacy of nivolumab monotherapy and in combination with ipilimumab in small-cell lung cancer. Cancer Cell. (2018) 33:853–61.e854. doi: 10.1016/j.ccell.2018.04.001
137. Johnson DB, Frampton GM, Rioth MJ, Yusko E, Xu Y, Guo X, et al. Targeted next generation sequencing identifies markers of response to PD-1 blockade. Cancer Immunol Res. (2016) 4:959–67. doi: 10.1158/2326-6066.CIR-16-0143
138. Goodman AM, Kato S, Bazhenova L, Patel SP, Frampton GM, Miller V, et al. Tumor mutational burden as an independent predictor of response to immunotherapy in diverse cancers. Mol Cancer Ther. (2017) 16:2598–608. doi: 10.1158/1535-7163.MCT-17-0386
139. Gandara DR, Paul SM, Kowanetz M, Schleifman E, Zou W, Li Y, et al. Blood-based tumor mutational burden as a predictor of clinical benefit in non-small-cell lung cancer patients treated with atezolizumab. Nat Med. (2018) 24:1441–8. doi: 10.1038/s41591-018-0134-3
140. Chan TA, Yarchoan M, Jaffee E, Swanton C, Quezada SA, Stenzinger A, et al. Development of tumor mutation burden as an immunotherapy biomarker: utility for the oncology clinic. Ann Oncol. (2019) 30:44–56. doi: 10.1093/annonc/mdy495
142. Chupak LS, Ding M, Martin SW, Zheng X, Hewawasam P, Connolly TP, et al. Compounds Useful as Immunomodulators. WO2015160641A2. Geneva: WIPO (2015).
143. Yeung K-S, Grant-Young KA, Zhu J, Saulnier MG, Frennesson DB, Meng Z, et al. 1,3-Dihydroxy-Phenyl Derivatives Useful as Immunomodulators. WO2018009505A1. Geneva: WIPO (2018).
144. Yeung K-S, Connolly TP, Frennesson DB, Grant-Young KA, Hewawasam P, Langley DR, et al. Compounds Useful as Immunomodulators. WO2017066227A1. Geneva: WIPO (2017).
145. Yeung K-S, Grant-Young KA, Zhu J, Saulnier MG, Frennesson DB, Langley DR, et al. Biaryl Compounds Useful as Immunomodulators. WO2018044963A1. Geneva: WIPO (2018).
146. Wang M. Symmetric or Semi-Symmetric Compounds Useful as Immunomodulators. WO2018026971A1. Geneva: WIPO (2018).
147. Webber SE, Almassy RJ. Immune Checkpoint Inhibitors, Compositions and Methods Thereof. WO2018045142A1. Geneva: WIPO (2018).
148. Lange C, Malathong V, Mcmurtrie DJ, Punna S, Singh R, Yang J, et al. Immunomodulator Compounds. WO2018005374A1. Geneva: WIPO (2018).
149. Feng Z, Chen X, Zhang L, Yang Y, Lai F, Ji M, et al. Bromo Benzyl Ether Derivative, Preparation Method Therefor, and Pharmaceutical Composition and Uses Thereof. WO2017202275A1. Geneva: WIPO (2017).
150. Feng Z, Chen X, Yang Y, Lai F, Ji M, Zhang L, et al. Benzyl Phenyl Ether Derivative, Preparation Method Therefor, and Pharmaceutical Composition and Uses Thereof. WO2017202273A1. Geneva: WIPO (2017).
151. Feng Z, Chen X, Yang Y, Zhou C, Lai F, Jin X, et al. Phenylate Derivative, Preparation Method Therefor, and Pharmaceutical Composition and Uses Thereof. WO2017202276A1. Geneva: WIPO (2017).
152. Wang Y, Xu Z, Wu T, He M, Zhang N. Aromatic Acetylene or Aromatic Ethylene Compound, Intermediate, Preparation Method, Pharmaceutical Composition and Use Thereof. WO2018006795A1. Geneva: WIPO (2018).
153. Sasikumar PN, Ramachandra M, Naremaddepalli SS. 1,3,4-Oxadiazole and Thiadiazole Compounds as Immunomodulators. WO2016142852A1. Geneva: WIPO (2016).
154. Sasikumar PN, Ramachandra M, Prasad A, Naremaddepalli SS. 3-Substituted 1,3,4-Oxadiazole and Thiadiazole Compounds as Immunomodulators. WO2016142894A1. Geneva: WIPO (2016).
155. Sasikumar PN, Ramachandra M, Naremaddepalli SS. 1,3,4-Oxadiazole and 1,3,4-Thiadiazole Derivatives as Immunomodulators. WO2015033301A1. Geneva: WIPO (2015).
156. Sasikumar PN, Ramachandra M, Naremaddepalli SS. 1,2,4-Oxadiazole Derivatives as Immunomodulators. WO2015033299A1. Geneva: WIPO (2015).
157. Sasikumar PN, Ramachandra M, Prasad A, Naremaddepalli SS. 3-Substituted-1,2,4-Oxadiazole and Thiadiazole Compounds as Immunomodulators. WO2016142886A2. Geneva: WIPO (2016).
158. Sasikumar PN, Ramachandra M, Naremaddepalli SS. 1,2,4-Oxadiazole and Thiadiazole Compounds as Immunomodulators. WO2016142833A1. Geneva: WIPO (2016).
159. Sasikumar PN, Ramachandra M, Prasad A, Naremaddepalli SS. Cyclic Substituted-1,3,4-Oxadiazole and Thiadiazole Compounds as Immunomodulators. WO2018051255A1. Geneva: WIPO (2018).
160. Sasikumar PN, Prasad A, Naremaddepalli SS, Ramachandra M. Cyclic Substituted-1,2,4-Oxadiazole Compounds as Immunomodulators. WO2018051254A1. Geneva: WIPO (2018).
161. Lu L, Qian D, Wu L, Yao W. Heterocyclic Compounds as Immunomodulators. WO2017205464A1. Geneva: WIPO (2017).
162. Wu L, Shen B, Li J, Li Z, Liu K, Zhang F. Heterocyclic Compounds as Immunomodulators. US20170107216A1. Alexandria, VA: USPTO (2017).
163. Wu L, Shen B, Li J, Li Z, Liu K, Zhang F, et al. Heterocyclic Compounds as Immunomodulators. WO2017070089A1. Geneva: WIPO (2017).
164. Wu L, Yu Z, Zhang F, Yao W. N-Phenyl-Pyridine-2-Carboxamide Derivatives and Their Use as pd-1/pd-l1 Protein/Protein Interaction Modulators. WO2017106634A1. Geneva: WIPO (2017).
165. Lajkiewicz N, Wu L, Yao W. Heterocyclic Compounds as Immunomodulators. US20170174679A1. Alexandria, VA: USPTO (2017).
166. Wu L, Zhang F, Mei S, Yao W. Heterocyclic Compounds as Immunomodulators. US20180057486A1. Alexandria, VA: USPTO (2018).
167. Yu Z, Wu L, Yao W. Heterocyclic Compounds as Immunomodulators. WO2018013789A1. Geneva: WIPO (2018).
168. Xiao K, Zhang F, Wu L, Yao W. Heterocyclic Compounds as Immunomodulators. US20170362253A1. Alexandria, VA: USPTO (2017).
169. Li Z, Wu L, Yao W. Heterocyclic Compounds as Immunomodulators. WO2017192961A1. Geneva: WIPO (2017).
170. Alexander D. Inhibitors of the pd-1/pd-l1 Protein/Protein Interaction. WO2017118762A1. Geneva: WIPO (2017).
171. Sasikumar PN, Ramachandra M, Vadlamani SK, Shrimali KR, Subbarao K. Therapeutic Compounds for Immunomodulation. US9096642B2. Alexandria, VA: USPTO (2014).
172. Sasikumar PN, Ramachandra M, Naremaddepalli SS. Immunomodulating Peptidomimetic Derivatives. WO2015036927A1. Geneva: WIPO (2015).
173. Sasikumar PN, Ramachandra M, Naremaddepalli SS. Therapeutic Immunomodulating Compounds. WO2015044900A1. Geneva: WIPO (2015).
174. Sasikumar PN, Ramachandra M. Immunomodulating Cyclic Compounds. US9422339B2. Alexandria, VA: USPTO (2016).
175. Sasikumar PN, Ramachandra M, Naremaddepalli SS. Cyclic Peptidomimetic Compounds as Immunomodulators. WO2015033303A1. Geneva: WIPO (2015).
176. Sasikumar PN, Ramachandra M, Naremaddepalli SS. Therapeutic Cyclic Compounds as Immunomodulators. WO2016142835A1. Geneva: WIPO (2016).
177. Miller MM, Mapelli C, Allen MP, Bowsher MS, Boy KM, Gillis EP, et al. Macrocyclic Inhibitors of the pd-1/pd-l1 and cd80(b7-1)/pd-l1 Protein/Protein Interactions. US9308236B2. Alexandria, VA: USPTO (2016).
178. Miller MM, Allen MP, Bowsher MS, Boy KM, Gillis EP, Langley DR, et al. Macrocyclic Inhibitors of the PD-1/PD-L1 and CD80(B7-1)/PD-L1 Protein/Protein Interactions. US9879046B2. Alexandria, VA: USPTO (2018).
179. Miller MM, Mapelli C, Allen MP, Bowsher MS, Gillis EP, Langley DR, et al. Macrocyclic Inhibitors of the pd-1/pd-l1 and cd80 (b7-1)/pd-li Protein/Protein Interactions. WO2016039749A1. Geneva: WIPO (2016).
180. Miller MM, Allen MP, Li L, Bowsher MS, Gillis EP, Mull E, et al. Macrocyclic Inhibitors of the pd-1/pd-l1 and cd80/pd-l1 Protein/Protein Interactions. WO2017176608A1. Geneva: WIPO (2017).
181. Gillman KW, Goodrich J, Boy KM, Zhang Y, Mapelli C, Poss MA, et al. Macrocyclic Peptides Useful as Immunomodulators. WO2016077518A1. Geneva: WIPO (2016).
182. Sun L, Zhao Q, Gillis EP, Miller MM, Allen MP, Mull E, et al. Immunomodulators. WO2016100608A1. Geneva: WIPO (2016).
183. Allen MP, Gillis EP, Langley DR, Miller MM, Mull E, Sun L, et al. Immunomodulators. US20170252432A1. Alexandria, VA: USPTO (2017).
184. Miller MM, Allen MP, Li L, Mapelli C, Poirier MA, Sun L, et al. Immunomodulators. WO2016126646A1. Geneva: WIPO (2016).
185. Lee JJ, Powderly JD, Patel MR, Brody J, Hamilton EP, Infante JR, et al. Phase 1 trial of CA-170, a novel oral small molecule dual inhibitor of immune checkpoints PD-1 and VISTA, in patients (pts) with advanced solid tumor or lymphomas. J Clin Oncol. (2017) 35:3099. doi: 10.1200/JCO.2017.35.15_suppl.TPS3099
186. Radhakrishnan VS, Bakhshi S, Prabhash K, Deshmukh C, Nag S, Lakshmaiah K, et al. Phase 2 trial of CA-170, a novel oral small molecule dual inhibitor of immune checkpoints VISTA and PD-1, in patients (pts) with advanced solid tumor and Hodgkin lymphoma. In: Proceedings of 33rd Annual Meeting & Pre-Conference Programs of the Society for Immunotherapy of Cancer (SITC 2018), Washington, DC (2018).
187. Vilalta Colomer M, Punna S, Li S, Malathong V, Lange C, McMurtrie D, et al. A small molecule human PD-1/PD-L1 inhibitor promotes T cell immune activation and reduces tumor growth in a preclinical model. In: Proceedings of ESMO Immuno-Oncology Congress 2018, Geneva. (2018).
188. Horn L, Spigel DR, Vokes EE, Holgado E, Ready N, Steins M, et al. Nivolumab versus docetaxel in previously treated patients with advanced non-small-cell lung cancer: two-year outcomes from two randomized, open-label, phase III trials (checkMate 017 and CheckMate 057). J Clin Oncol. (2017) 35:3924–33. doi: 10.1200/JCO.2017.74.3062
189. Tomita Y, Fukasawa S, Shinohara N, Kitamura H, Oya M, Eto M, et al. Nivolumab versus everolimus in advanced renal cell carcinoma: Japanese subgroup analysis from the CheckMate 025 study. Jpn J Clin Oncol. (2017) 47:639–46. doi: 10.1093/jjco/hyx049
190. Hellmann MD, Ciuleanu TE, Pluzanski A, Lee JS, Otterson GA, Audigier-Valette C, et al. Nivolumab plus ipilimumab in lung cancer with a high tumor mutational burden. N Engl J Med. (2018) 378:2093–104. doi: 10.1056/NEJMoa1801946
191. Yue C, Jiang Y, Li P, Wang Y, Xue J, Li N, et al. Dynamic change of PD-L1 expression on circulating tumor cells in advanced solid tumor patients undergoing PD-1 blockade therapy. Oncoimmunology. (2018) 7:e1438111. doi: 10.1080/2162402X.2018.1438111
192. Wei R, Guo L, Wang Q, Miao J, Kwok HF, Lin Y. Targeting PD-L1 protein: translation, modification and transport. Curr Protein Pept Sci. (2019) 20:82–91. doi: 10.2174/1389203719666180928105632
193. Wang Q, Lin W, Tang X, Li S, Guo L, Lin Y, et al. The roles of microRNAs in regulating the expression of PD-1/PD-L1 immune checkpoint. Int J Mol Sci. (2017) 18:2540. doi: 10.3390/ijms18122540
Keywords: patent, PD-1, PD-L1, immunotherapy, clinical trial
Citation: Guo L, Wei R, Lin Y and Kwok HF (2020) Clinical and Recent Patents Applications of PD-1/PD-L1 Targeting Immunotherapy in Cancer Treatment—Current Progress, Strategy, and Future Perspective. Front. Immunol. 11:1508. doi: 10.3389/fimmu.2020.01508
Received: 01 April 2020; Accepted: 09 June 2020;
Published: 07 July 2020.
Edited by:
Sophie Lucas, Université Catholique de Louvain, BelgiumReviewed by:
Alessandro Poggi, San Martino Hospital (IRCCS), ItalyAmorette Barber, Longwood University, United States
Copyright © 2020 Guo, Wei, Lin and Kwok. This is an open-access article distributed under the terms of the Creative Commons Attribution License (CC BY). The use, distribution or reproduction in other forums is permitted, provided the original author(s) and the copyright owner(s) are credited and that the original publication in this journal is cited, in accordance with accepted academic practice. No use, distribution or reproduction is permitted which does not comply with these terms.
*Correspondence: Yao Lin, eWFvbGluQGZqbnUuZWR1LmNu; Hang Fai Kwok, aGZrd29rQHVtLmVkdS5tbw==