- 1Faculty of Pharmacy, King Abdulaziz University, Jeddah, Saudi Arabia
- 2Vaccines and Immunotherapy Unit, King Fahd Medical Research Center, King Abdulaziz University, Jeddah, Saudi Arabia
- 3Department of Pharmaceutics and Pharmaceutical Technology, College of Pharmacy, Taif University, Taif, Saudi Arabia
- 4College of Applied Medical Sciences, Taibah University, Medina, Saudi Arabia
- 5Department of Medical Laboratory Technology, University of Tabuk, Tabuk, Saudi Arabia
- 6Australian Institute for Bioengineering and Nanotechnology, The University of Queensland, Brisbane, QLD, Australia
- 7Australian Research Council Training Centre for Biopharmaceutical Innovation, The University of Queensland, Brisbane, QLD, Australia
- 8Department of Medical Microbiology and Parasitology, Faculty of Medicine, King Abdulaziz University, Jeddah, Saudi Arabia
Monoclonal antibodies (mAbs) have become one of the most important classes of biopharmaceutical products, and they continue to dominate the universe of biopharmaceutical markets in terms of approval and sales. They are the most profitable single product class, where they represent six of the top ten selling drugs. At the beginning of the 1990s, an in vitro antibody selection technology known as antibody phage display was developed by John McCafferty and Sir. Gregory Winter that enabled the discovery of human antibodies for diverse applications, particularly antibody-based drugs. They created combinatorial antibody libraries on filamentous phage to be utilized for generating antigen specific antibodies in a matter of weeks. Since then, more than 70 phage–derived antibodies entered clinical studies and 14 of them have been approved. These antibodies are indicated for cancer, and non-cancer medical conditions, such as inflammatory, optical, infectious, or immunological diseases. This review will illustrate the utility of phage display as a powerful platform for therapeutic antibodies discovery and describe in detail all the approved mAbs derived from phage display.
Monoclonal Antibodies (mAbs)
Monoclonal antibodies (mAbs) are versatile biomacromolecules that can bind with high specificity to a wide range of protein and non-protein targets (1–4). These mAbs can be engineered and produced into different formats to enhance their functionality and use (Figure 1) (5). To date, more than 80 mAbs have been approved for clinical applications with many more under pre-clinical and clinical development (6). They represent six of the top ten selling drugs (7) with annual sales exceeding $120 billion in 2017 (8) and are expected to reach $130–200 billion by 2022 (9). They also have a high success rate in clinical development; for instance, it has been reported that the probability of FDA approval for mAbs in phase I of development is ~14.1%, which is almost twice the approval rate of small molecule drugs (~7.6%) (10, 11). Such factors make biopharmaceutical companies more motivated and willing to sponsor the development of these pharmaceutical products.
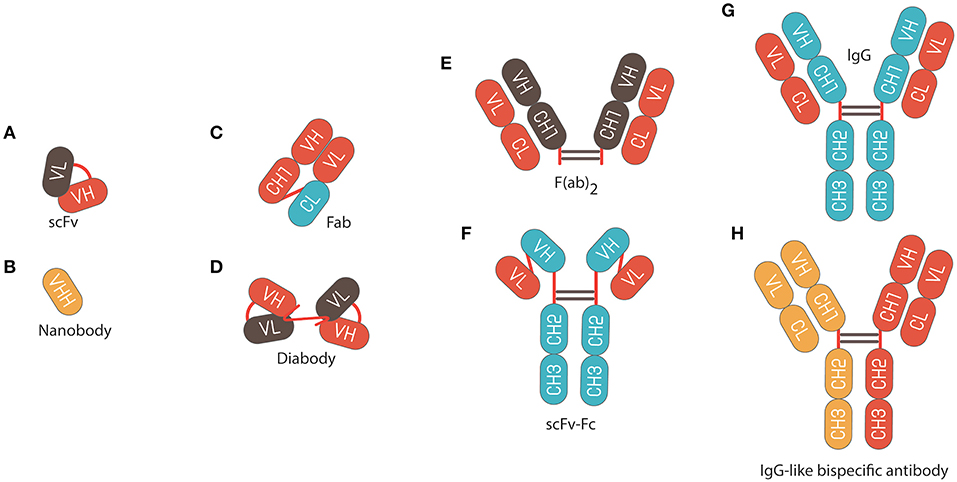
Figure 1. Schematic representation of different antibody formats. (A) Single chain fragment variable (scFv) composed of variable regions of the light chain (VL) linked to variable regions of the heavy chain (VH) by a flexible glycine-serine linker (Gly4Ser)3. (B) Nanobody fragments. (C) Fragment of antigen binding (Fab) composed of VL and a constant domain of the light chain (CL) linked to VH and constant domain 1 of the heavy chain (CH1) by a disulphide bond between the CL and CH1 domains. (D) Diabody composed of VL linked to variable heavy VH by a pentameric (Gly4Ser). (E) F(ab)2 fragment composed of 2 × Fab fragments joined by an Immunoglobulin G (IgG) hinge region. (F) scFv fusion with an Fc IgG. (G) IgG composed of constant fragment (Fc), which is able to bind and stimulate immune effector cells, and Fab, which comprises the variable domains that contain the antigen binding regions. (H) Bispecific IgG antibody.
During the last 120 years, the research and development of antibody-related technologies have been the subject of four Nobel Prizes. In 1901, Emil von Behring won the first Nobel Prize in Physiology or Medicine for the successful therapeutic use of horse hyperimmune serum containing neutralizing polyclonal antibodies against diphtheria and tetanus toxins (12). Kohler and Milstein received the 1984 Nobel Prize in Physiology or Medicine for developing the ground-breaking hybridoma technology which facilitated the isolation of mAbs and their subsequent production in laboratories (13). In 2018, George P. Smith and Sir Gregory P. Winter were awarded with the Nobel Prize in Chemistry for their development of phage display of peptide and antibodies (14–16). In the same year, James P. Allison and Tasuku Honjo were honored by the 2018 Nobel Prize in Physiology or Medicine for their discoveries of cancer immunotherapy via the use of antibody blockade of the T-cell inhibitory receptor (CTLA-4) and programmed cell death protein 1 (PD1) to enhance anti-tumor immune responses (17, 18).
Overview of Antibody Phage Display Libraries
Although hybridoma technology was ground-breaking at the time and still commonly used to produce antibodies as research reagents, murine-derived mAbs have limited therapeutic efficacy. Several reports have indicated that patients treated with murine-derived mAbs will develop a human anti-mouse antibody (HAMA) response, which accelerates mAb clearance, and could result in undesirable allergic reactions upon repeated administration (19, 20). Antibody engineering techniques have been subsequently utilized to create chimeric or humanized antibodies by utilizing the murine variable regions or complementary determining regions (CDRs), respectively, in conjunction with human constant regions, in order to maintain target specificity and reduce the HAMA response (21–23). Fully human antibodies are now generated using hybridoma technology in transgenic mice models, such as HuMabMouse and XenoMouse, whereby the mouse immunoglobulin (Ig) gene loci have been replaced with human loci within the transgenic mouse genome (24–26).
Development of antibody phage display libraries represents an alternative technique to the traditional hybridoma technology. They involve the isolation of fully human-derived mAbs from large Ig gene repertoires displayed on the surface of bacteriophages (16). In 1985, George P. Smith was the first to describe phage display technology by demonstrating that filamentous phages are able to display a peptide of interest on their surfaces after inserting a foreign DNA fragment into the filamentous phage coat protein gene (14). Subsequently, Parmley and Smith described a selection and affinity enrichment process known as “panning or biopanning,” that allowed for the isolation of peptide-phage fusions from a 108-fold excess of wild type phages based on their specific binding affinity to biotinylated antibodies specific for the peptides (27). Later, McCafferty and Winter were the first to utilize phage display technology in antibody discovery by creating combinatorial antibody libraries on filamentous phages to be utilized for generating antigen specific mAbs (15, 16).
M13 is one of the filamentous bacteriophages (Ff) of Escherichia coli (E. coli), and one of the most widely used phages for antibody phage display (28, 29). Filamentous bacteriophages only infect E. coli strains through an interaction between the expressed F pilus on the surface of hosts, and a phage coat protein (30). M13 is a flexible cylindrical-shaped virus particle containing a circular single-stranded DNA genome (6,407-base) consisting of nine genes encoding for five coat proteins (pIII, pVIII, pVI, pVII, and pIX), and six assembly and replication proteins (31, 32). Most major phage display systems are based on pIII-antibody fusion proteins, due to pIII structural flexibility and its ability to display large proteins without losing its function (33–36).
The discovery of smaller recombinant antibody formats, such as variable domain [Fv; variable regions of the heavy (VH) or light chain (VL)], single-chain variable domain (scFv), diabodies (bivalent scFvs), heavy-domain camelid and shark antibody fragments (VHHs, nanobodies), and fragment antigen binding (Fab), has helped to advance antibody phage display technology (Figure 1) (37–42). These smaller fragments are more amenable to expression in bacteria compared to full antibodies, which require assembly of four polypeptide chains and extensive disulfide bond formation. For instance, creating a combinatorial scFv library on the surface of M13 filamentous phage has been achieved through combining populations of VH and VL-domains, which are joined by a flexible, protease resistance glycine-serine linker (Gly4Ser)3, into a single DNA sequence (15). These antibody sequences are then introduced and cloned as a gene fusion with the bacteriophage pIII gene under the control of a weak promoter in a phagemid vector; a plasmid that carries an antibiotic resistance gene, bacterial and phage origins of replication (Figure 2) (43–46).
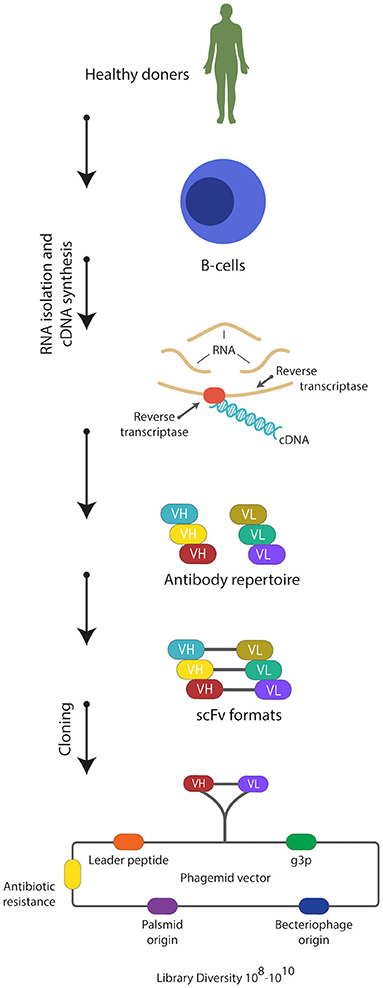
Figure 2. Strategy used for construction of naïve scFv-phage display libraries. Total RNA is isolated from B-lymphocytes from non-immunized healthy donors. Then cDNA is synthesized from the isolated RNA using reverse transcriptase enzyme. Then the repertoire of the VH and VL genes is amplified from the cDNA using forward and reverse primers hybridizing to the variable domains. scFvs are constructed and cloned into phagemid vector and a naïve phage library of 108-1010 is usually generated.
Co-infection of E. coli harboring a phagemid with a helper phage is essential for the formation of functional phage particles displaying pIII-antibody fusions (45). It causes E. coli to initiate the synthesis of all wild-type coat proteins needed for phage replication, and this is essential because the phagemid does not have all the genes necessary to encode a full bacteriophage in E. coli (47). The most commonly used helper phage is M13KO7, which is a derivative of M13 containing a kanamycin resistance gene and the P15A origin of replication that allows the genome to replicate as a plasmid in E. coli (48). A fully assembled phage particle contains five copies of the pIII protein, but since the wild type pIII gene from helper phage has superior expression levels compared to the phagemid-encoded pIII-antibody fusion gene, the majority of the produced phage population is expressed without a pIII-antibody fusion. However, only a portion of the population will contain monovalent display of the pIII-antibody fusion, with polyvalent display being much less frequent (49). The hyperphage system, which uses a helper phage lacking the pIII gene has been utilized for antibody pIII-antibody polyvalent display, because only the pIII-antibody gene of the phagemid is encoded (50). Nevertheless, monovalent display is the most popular display system because it allows for selection of higher affinity antibodies, avoiding the avidity effect of polyvalent display (43–46).
Biopanning for Target-Specific Antibodies
When purified antigens are available, they can be presented to a phage antibody library by immobilization on solid surfaces, such as nitrocellulose membranes, polystyrene tubes or plates, magnetic beads or column matrices (51–53). The use of blocking agents, such bovine serum albumin (BSA), milk or casein can block the remaining sites present on the solid surface to prevent non-specific phage binding to the surface (54, 55). After the phage library is exposed to the immobilized antigens, unbound phages are usually washed away (Figure 3). Such washing step is critical to remove non-specific binders, and to allow for some control over binding properties by manipulating the wash buffer and stringency of washing. For example, long wash times can be incorporated to ensure only clones with slow dissociation rates are selected. Detergents are usually included in wash buffers, but they can also be altered for factors, such as pH and salt concentration. The washing steps are gradually increased with every round of biopanning to increase the stringency in order to isolate higher affinity phage clones (46, 56).
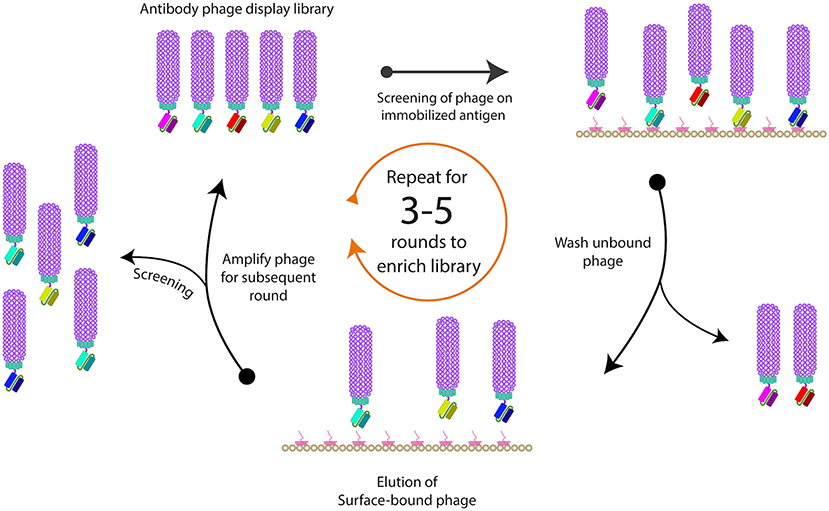
Figure 3. Schematic representation of phage biopanning. This is the basic method for sequential affinity screening of the phage display libraries for specific binding phage from a large excess of non-binding clones is often referred to as “panning or biopanning.” The phage antibody selection involves the immobilization of the ligand of interest on a solid support, followed by applying the phage display library (in the form of purified virions) to the immobilized ligand to allow binding of specific variants. To eliminate the adherent non-binders, multiple rounds of washing are usually performed, and remaining bound phages are eluted and re-amplified. At least three rounds of biopanning are usually required in order to amplify the binding variants and to exclude any non-specific binders.
To recover high-affinity phage antibodies from immobilized antigens, different elution conditions, including change in pH, proteolytic cleavage or competition with free antigens have been used. For pH elution, either acidic buffers, such as glycine or citric acid (52, 57), or alkaline triethylamine (TEA) can be used (51). It is crucial to neutralize the pH of eluted phage antibodies to be around 8, to avoid degradation of the phage and maintain infectivity. Some libraries have a cleavage site introduced between the antibody and the pIII protein to facilitate elution by using proteases, such as Genenase I or trypsin (58, 59).
After several rounds of biopanning, the pool of phages isolated from each round is tested, usually by ELISA, to determine if there is an enrichment of phage binders toward the specific antigen within the polyclonal pool. The polyclonal ELISA involves immobilizing antigen onto microtitre plates, followed by addition of various dilutions of the phage pool from each round and then detection of bound phage using an anti-M13 phage antibody. Individual clones from the round of biopanning exhibiting the maximum enrichment level are then further screened by ELISA to determine individual phage isolates with high specificity toward the antigen of interest. The procedure involves growing single colonies cell glycerol stock from the last performed biopanning round in a 96-well plate format, before adding the helper phage to induce production of phage particles. The positive clones derived from this experiment can then be analyzed by restriction fragment length polymorphism to determine the number of unique clones, or by sequencing which also determines the CDRs for both heavy and light chains (60). Once positive clones are isolated, downstream applications would determine how they are further processed. For example, a scFv gene from a phage clone can be re-cloned into a bacterial expression vector for large scale production or reformatted into a full mAb by inserting the variable regions into expression vectors containing the antibody constant regions (61). In vitro affinity maturation using mutated libraries of lead phage clones can also be conducted in order to increase the affinity and the stability of the selected antibodies (62, 63).
Although biopanning of immobilized antigen on solid surfaces is robust, it is often limited by the availability of purified protein and the possibility of its altered conformation when attached to solid surfaces. Therefore, alternative methods, such as in-solution biopanning, followed by affinity capture of antigens tagged with biotin (64), or calmodulin binding peptide have been used (65).
Some membrane proteins (66) are poorly soluble in an aqueous media, and due to their complexity, they do not form properly during recombinant expression (67). They might form aggregates and lose their tertiary structures when coated on immunotubes before biopanning (68–70), which as a result might lead to generate antibody binders that recognize epitopes that are not naturally exposed (71, 72). Thus, cell-based biopanning is often utilized to maintain membrane proteins native conformation (73–76). It can be applied to retrieve antibodies that are specific for either known or unknown antigens on cells surface, and it can be performed in case of unavailability of the targeted antigen in pure form (77–80). Furthermore, cell-based biopanning strategies allow for selecting binders to a specific conformational state of a cell surface receptor (81–85). Cell-based microselection approach, can be applied to retrieve unique binders, and identify novel biomarkers that are exclusively expressed on rare cells within a heterogeneous solution (86, 87).
The latest advancements in next-generation sequencing (NGS) technologies, bioinformatics and nanotechnology have tremendously improved the high-throughput screening of antibody discovery (88–91). For instance, a report from Raftery et al. (92) described a rapid selection of scFv-phage (PhageXpress) using electrohydrodynamic-manipulation of a solution containing phage library particles in combined with Oxford Nanopore Technologies' MinION sequencer. After a single round of biopanning and within 2 days compared to several weeks if applying traditional biopanning, they were able to identify 14 anti-dengue virus non-structural protein 1 scFv. Adopting similar approaches will significantly reduce the time and amount of laborious lab work required to discover putative antibodies, which are major obstacles in the traditional biopanning method, and will help accelerate developing therapeutic monoclonal antibodies during emerging infectious outbreaks (93).
Phage Display Libraries as an Antibody Discovery Platform
Antibody phage display is a versatile, in vitro selection technology that can be utilized to discover high affinity antibodies specific to a wide variety of antigens (94). However, specificity and high affinity are not the only attributes that account for successful therapeutic antibodies. Other antibody quality attributes, such as solubility, viscosity, expression yield, and thermal and long-term stability are vital to ensure the success of mAb lead candidates in biomanufacturing and clinical trials (95, 96). These biophysical properties of antibodies are strongly dependent on their amino acid sequences (97). Some mAbs might have poor developability profiles because of high immunogenicity, physicochemical instability, self-association, high viscosity, poly-specificity, short half-life, and poor expression (98, 99). For instance, low solubility can lead to issues during biomanufacturing (100–102), and could affect mAb potency, bioavailability and immunogenicity (103, 104). High thermal stability is crucial to maintain structural and functional integrity, and intrinsic properties, under different temperatures (105, 106). Furthermore, aggregation is one of the main challenges that limit the advancement of therapeutic mAb due to immunogenicity concerns (107–110).
Despite the several advantages of antibody phage display, such as bypassing animal immunization, the ability to isolate antibodies against toxic or non-immunogenic antigens and the ability to generate conformation-specific antibodies, the vast majority of the approved therapeutic antibodies are derived from immunized mice technologies. This is because the filtration process that imposed by the immune system enables mammalians derived antibodies to have better biophysical attributes compared to antibodies generated by phage display (111). In agreement with this, Jain et al. has comprehensively analyzed the biophysical attributes for 46 FDA approved therapeutic antibodies and 89 in advance clinical trials (96). They found that antibodies directly discovered by phage display or engineered at some point by phage biopanning exhibit significant developability risks' properties compared to than those derived from immunized mice. Further investigations found that phage display derived therapeutic antibodies have higher self-interaction and poly-reactivity due to the higher percentage of aliphatic residues in their CDRs compared to the non-phage derived antibodies (112).
Additionally, antibodies selected form phage display libraries are not glycosylated, because they are produced in E. coli, as a result, some candidates when glycosylation occur during mammalian cells expression; their binding, biodistribution, or pharmacokinetics might be negatively impacted (113–116). Therefore, using eukaryotic display platforms like yeast and mammalian display would be beneficial. For instance, in addition to their ability to produce glycosylated proteins, yeast and mammalian antibody libraries can be constructed to display full-length antibodies as well as antibody fragments, such as scFvs and fragment antigen-binding region (Fabs) (117–126), allowing the isolation of high affinity antibodies with definitive biological characteristics (122, 123). For example, Parthiban et al. has developed mammalian libraries that display around 10 million clones in IgG-format on the surface of HEK293 cells using CRISPR/Cas9 or transcription activator-like effector nucleases (TALENs) (127). These libraries can act as a quality filter for different antibody developability aspects, and to provide a very early insight into developability problems, such as aggregation and cross-reactivity. Each display system has its advantages and disadvantages, however, determining those are beyond the intended scope of this review, which is about the most commonly used type of antibody display, the phage display. Therefore, it is vital to generate phage libraries that allow for the isolation of highly specific and diverse mAbs with high affinity against diverse antigens with optimal developability potential (128–130).
Currently, as a common practice in industrial pipelines, biopharmaceutical companies are implementing extensive developability assessments to determine the biochemical and biophysical features of antibody candidates to help identifying candidates with more favorable biophysical properties and to avoid difficulties during the downstream process (131, 132). For example, in silico platforms, such as the Therapeutic Antibody Profiler (TAP) tool are used as a flagging system to predict mAbs with poor developability profiles by identifying anomalous values compared with therapeutic mAbs in clinical-stages. Indeed, features within the variable regions of mAbs, such as the total CDRs length, high hydrophobicity of VH and VL chains, lack of net charge symmetry, and/or the presence of patches of positive and negative charges were computationally predicted to be key factors in developability profiles of mAbs (133).
The probability of isolating high affinity, and more diverse mAbs that specifically bind random epitopes, increases significantly when biopanning campaigns are performed using larger antibody libraries. Library diversity is judged by how many functional antibody fragments are able to identify as many different antigens as possible (134). The bacterial transformation step during library construction; however, is a main practical bottleneck that limits the size of the library from exceeding 1011 antibody variants, even after optimization and performing numerous electroporation steps.
Ideally, antibody phage display libraries should not only be large and diverse, but also should display antibody variants as functional fragments. Issues related to the nucleotide sequences, such as the presence of stop codons, or the addition/deletion of nucleotides can occur during the library construction (135–137). These issues might inhibit the production of functional pIII-antibody fusions or change the reading frame of the antibody gene sequence, which could negatively affect their biophysical characteristics. Some in-frame antibody genes might also have poor expression levels from their phagemid, or produce aggregated, misfolded, or toxic antibody fragment to E. coli (138–141). However, such variants are usually displayed in lower percentage compared to other variants or phages that do not display any fusion protein.
Types of Antibody Phage Display Libraries
Phage libraries generated from human rearranged V-gene repertoires are constructed from mRNA or RNA extracted from B cells of immunized or naïve donors (Figure 2) (73, 142–144). Construction of immunized or naïve libraries involves using reverse transcription polymerase chain reaction (RT-PCR) to prepare the cDNA template. This is followed by the amplification of the repertoire of VL and VH genes by PCR, before cloning into the phagemid.
Immunized libraries are constructed from lymphoid tissues of individuals who carry a particular disease, such as metastatic cancer or particular infection, or have been immunized with a particular antigen (145–149). Such libraries are characterized by a biased antibody repertoire toward specific targets. Additionally, those antibodies tend to have much higher affinities for the desired antigen than antibodies isolated from naïve libraries of comparable size, because the VH and VL gene fragments have undergone the natural in vivo affinity maturation process (150). Naïve libraries, on the other hand, represent the germline diversity of antibody repertoire. These libraries are generated from healthy donor's mRNA or RNA without bias toward a particular disease state, and are used to yield mAbs against unlimited range of antigens (151). To generate a highly diverse naïve antibody phage library, it is recommended to use a large pool of donors from diverse ethnic groups, and to maximize the efficiency of antibody gene amplification in the process of library construction (152–155).
The CDRs play a significant role in antigen recognition (156), although some of the non-CDRs residues contribute to the antibody-antigen interaction (157). Each CDR loop contributes differently to antibody-antigen binding, and each residue within each CDR loop plays a different role in this interaction (158, 159). Among all the six CDR loops, the VH CDRs, especially VH's CDR3 (CDRH3), are more frequently involved in the antigen binding than those in the light chain (160, 161). The CDRH3 loop, which exists in a variety of different lengths (5–30 amino acids), is of particular importance due to its substantial impact on the canonical conformation and antigen binding compared to the other CDRs (156, 162–165). Noteworthy, the loop length of CDRH3 does not only affect the specificity and affinity of the antibody for its specific antigen, but also affects the nature of the binding of other CDRs. Specifically, for antibodies with long CDRH3 loops, these loops are responsible for most of the antibody-antigen interactions, while in antibodies with short CDRH3 loops, other CDRs loops usually assist in antigen binding (156). Thus, CDRH3 plays a major role in recognizing diverse targets, and generating interactions with acceptable affinity (166, 167).
The diversity of the V-gene segments can be designed and synthesized artificially by CDRs randomization. These libraries can be fully synthetic or semisynthetic. Synthetic libraries are made to maximize antibodies' functionality by making a large and highly diverse phage repertoire. This is usually achieved in vitro by using PCR and oligonucleotides to create a random integration of the CDRs as well as introduction of different CDRH3 loop sequences and lengths without disrupting the folding of the V regions (94, 168). Semisynthetic libraries combine natural and synthetic antibody diversity. They are constructed from non-rearranged V-genes from pre-B cells, or an antibody framework with randomization of the CDRH3 or several CDRs utilizing degenerated oligonucleotides (128, 169, 170).
Phage Display-Derived mAbs
Data collected for this review were obtained from different sources including PubMed, the clinical trial database (www.clinicaltrials.gov), patents, company websites, and international ImMunoGeneTics information system (www.imgt.org). A selection of phage display-derived therapeutics was described in great detail previously (171, 172), yet we here present an updated and comprehensive review of phage display-derived mAbs.
Two decades after McCafferty and Winter's seminal report in 1990, more than 70 phage–derived mAbs entered clinical studies, and 14 of them have been approved. The majority of these antibodies are generated by three company-owned libraries, Cambridge Antibody Technology (CAT), Dyax and MorphoSys's human combinatorial antibody libraries (HuCAL®) (Table 1, Figure 4). MorphoSys's HuCAL® has the highest number of mAbs (20 mAbs), wherein 19 are under clinical development, and one (Tremfya™) is approved. The majority of the MorphoSys's HuCAL® derived mAbs (12 mAbs) are in phase II clinical trials. CAT (AstraZenica) has the second highest number of phage derived mAbs (15 mAbs) in clinical trials, and the highest number of approved mAbs including Humira®, Benlysta®, Lumoxiti™, ABthrax®, and Gamifant®. Dyax has 13 mAbs in which four of them have been approved; Bavencio®, Portrazza™, Cyramza®, Takhzyro®.
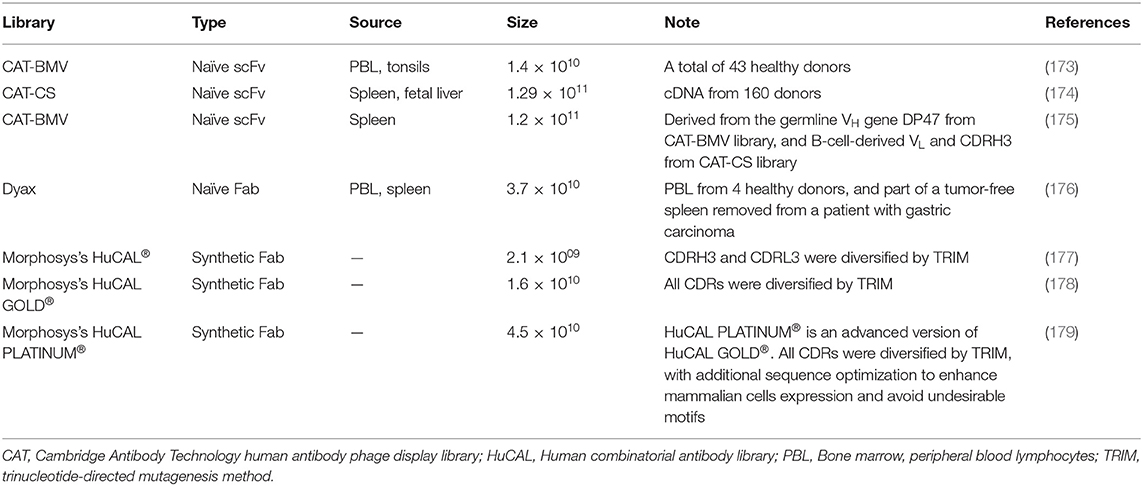
Table 1. The design, construction, and features of some major company-owned antibody-phage libraries.
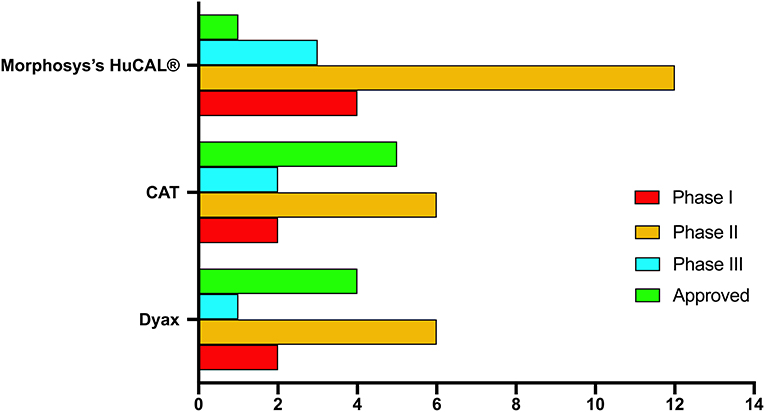
Figure 4. Highest development phase achieved for antibodies isolated from various major company-owned libraries.
Therapeutic mAbs from phage libraries can be successfully isolated to treat cancer, and non-cancer medical conditions, such as inflammatory, optical, infectious, or immunological diseases (Table 2). However, some of the aforementioned major libraries have a favorable therapeutic area of application. More mAbs for non-cancer indications in comparison to cancer indications (~63 vs. ~37%) were developed using CAT libraries. Among all the five approved CAT derived mAbs, Lumoxiti™ is the only one that is indicated to treat cancer. Unlike CAT, Dyax libraries have been remarkably useful in the development of therapeutic mAbs for oncology at the expense of non-oncology indications (~70 vs. ~30%), in which three out of the four approved mAbs are anti-cancer agents (Figure 5). MorphoSys's HuCAL® libraries have almost equally contributed to both cancer and non-cancer indications (Figure 5).

Table 2. A list of phage display-derived therapeutic antibodies that either are approved or have been investigated clinical trials.
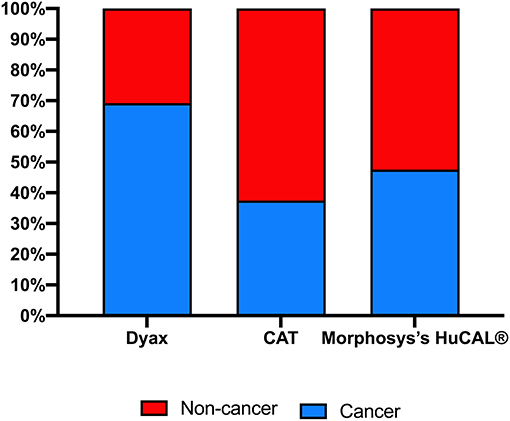
Figure 5. Indications for antibodies isolated from various major company-owned libraries. Clinical domains (cancer vs. non-cancer) of the approved or in clinical trials antibody-derived phage display.
The most dominant antibody format of the approved or under clinical investigations phage-derived antibodies is the Immunoglobulin G (IgG), yet other formats, such as antibody conjugates or nanobodies are also included (Table 2). MAbs isolated from the CAT libraries, for instance, belong to two IgG subclasses, IgG1 and IgG4, with the majority being IgG1-λ. MorphoSys's HuCAL® platforms show similar trend to CAT in addition to large number of mAbs from IgG2 subclass. MAbs from Dyax libraries, on the other hand, belong to IgG1 and IgG2 with the majority being IgG1-κ.
Having more than one mAb against a specific target or condition is essential especially that patients might acquire resistance against a prescribed therapeutic mAb, because of the possible immunogenicity and induction of anti-drug antibodies (ADAs) (459, 460). As a result, their pharmacokinetic, safety, and efficacy can be negatively impacted by the presence of ADAs (459–461). From this perspective, antibody phage display technology has enabled receptors like mesothelin (MSLN), human epidermal growth factor receptor 3 (HER3) and programmed cell death-ligand 1 (PD-L1) to have more than one specific therapeutic mAb (Table 2).
Phage Display-Derived mAbs
As discussed earlier, phage display technology demonstrated its robustness and reproducibility as a human antibodies discovery platform. To date, 14 approved mAbs and many others in pre-clinical development or in clinical trials have been derived using this technology. These antibodies and antibody fragments are indicated to treat several disease conditions (Table 2). In this section, we will discuss in detail all the approved phage display-derived antibodies to highlight the utility of antibody phage display technology in the universe of biopharmaceutical industry.
Atezolizumab (Tecentriq™)
Atezolizumab is a humanized IgG1-κ immune checkpoint inhibitor targets PD-L1 that commonly expressed on the surface of antigen presenting cells and tumor cells, and prevents its binding to the programmed cell death protein 1 (PD-1) receptor on T cells. PD-L1 is usually released by tumor cells and results in cancer immune evasion and decreases antitumor T-cell responses which are usually associated with poor clinical outcomes. Thus, utilizing atezolizumab could disrupt such T cell suppression by blocking PD-L1 binding to PD-1 and restore tumor-specific T-cell immunity in several cancer types (462–473).
In 2016, atezolizumab was approved by the US FDA for the treatment of urothelial carcinoma (UC) and metastatic lung cancer. Subsequently, it was granted accelerated approval for the treatment of advanced bladder cancer in 2017, and metastatic non-small-cell lung carcinoma (NSCLC) in combination with bevacizumab and chemotherapy in 2018. More recently, atezolizumab was approved for several indications, such as in combination with abraxane for patients with PD-L1-positive metastatic triple-negative breast cancer (PD-L1–positive TNBC), in combination with chemotherapy for the initial treatment of adults with extensive-stage small-cell lung carcinoma (SCLC), and in combination with abraxane and carboplatin for the initial treatment of metastatic non-squamous NSCLC (Table 2).
Clinical trials with atezolizumab are currently ongoing for multiple forms of solid tumors and hematologic malignancies. As of 2019, there are 249 ongoing trials with atezolizumab either as monotherapy or in combination with other anti-cancer agents. Ongoing clinical studies include several indications, such as NSCLC, UC, renal cell carcinoma (RCC), hepatocellular carcinoma, TNBC, colorectal cancer, and hematologic malignancies among other tumor types. Atezolizumab as a single treatment has shown a significant anti-tumor response in NSCLC (469, 474–476), UC (466, 477), glioblastoma multiforme (478), and RCC (479). In a randomized Phase II clinical trial for NSCLC, atezolizumab single treatment has shown an overall survival benefit compared to docetaxel (469).
Avelumab (Bavencio®)
Avelumab is a fully human IgG1-λ immune checkpoint inhibitor that targets PD-L1 protein and blocks its interaction with PD-1. Additionally, avelumab is thought to engage the innate immune system and elicits an antibody-dependent cellular cytotoxic (ADCC) response against PD-L1-expressing tumors (480). While ADCC has not been indicated to contribute to the clinical activity of avelumab (472), preclinical studies suggest a possible role of ADCC in the activity of avelumab (481, 482).
In early 2017, Avelumab was approved for metastatic Merkel cell carcinoma (mMCC) in adults and pediatric patients aged >12 years as the first approved medication for this indication in the USA (483). In Europe, the application of avelumab marketing authorization for the treatment of mMCC is under regulatory review, while in Australia, Japan, and Switzerland phase II trial has been initiated for mMCC (483).
In 2017, the US FDA approved avelumab in the treatment of locally advanced or metastatic urothelial carcinoma metastatic urothelial carcinoma based on the phase III JAVELIN Bladder 100 trial (NCT02603432). Additionally, in 2019 the US FDA approved avelumab for the treatment of advanced RCC in combination with the tyrosine kinase inhibitor, axitinib. The approval was based on the phase III JAVELIN Renal 101 trial (NCT02684006).
Avelumab is under phase III trial in several countries for breast cancer, head and neck cancer, NSCLC, ovarian cancer, B cell lymphoma, and gastric cancer. There are many other phase II clinical trials underway globally for glioblastoma, intestinal cancer, nasopharyngeal cancer, endometrial cancer, recurrent respiratory papillomatosis, and thymoma (483).
Moxetumomab Pasudotox-tdfk (Lumoxiti™)
Moxetumomab pasudotox-tdfk (CAT-8015) is a novel recombinant immunotoxin that consists of a recombinant murine scFv genetically fused to a truncated pseudomonas exotoxin (PE38), which targets CD22 antigen that is expressed on the surface of many types of malignant B cells including hairy cell leukemia (HCL) (263, 484). This mAb is the second generation of BL22/CAT-3888, whereby the CDRH3 has been affinity matured by phage display to increase the affinity by 14-fold toward CD22 (485, 486). After binding to CD22, moxetumomab pasudotox-tdfk is internalized, and the Pseudomonas exotoxin catalyzes inhibition of protein synthesis by ADP-ribosylation of elongation factor 2, resulting in apoptotic cell death (263).
HCL is a rare chronic disease that accounts for 2% of all leukemias with a 4:1 male-to-female predominance (487, 488). Outcomes with standard treatment are usually positive in 78% of patients however, relapses occur in ~50% of the patients (489). In 2018, the US FDA approved moxetumomab pasudotox-tdfk under the trade name of Lumoxiti™ (AstraZeneca Pharmaceuticals LP) to be utilized therapeutically for adult patients with relapsed or refractory HCL (R/R HCL) that no longer responding to other therapies, including purine analog (263, 490). Lumoxiti received US Orphan Drug designation and the FDA granted the application Fast Track and Priority Review designations because of the severity and rarity of the disease and was the first new therapy granted approval for HCL since cladribine in 1993.
Currently, the national cancer institute is sponsoring a phase I clinical trial to assess the safety of moxetumomab Pasudotox-tdfk in combination with rituximab in subjects with HCL or HCL variant (NCT03805932). Furthermore, an active phase III clinical trial (NCT04125290) aims to evaluate the post-marketing safety of moxetumomab pasudotox-tdfk for old patients (≥65 years), and/or patients with moderate renal impairment.
Necitumumab (Portrazza™)
Necitumumab is a fully human IgG1-κ mAb which selectively binds the epidermal growth factor receptor (EGFR). It binds to domain III of the extracellular region of EGFR and blocks ligand binding. Necitumumab prevents the proliferation of several cancer cell lines by affecting downstream signaling of the EGFR receptor involved in cell growth and angiogenesis (270, 491) which are crucial for promoting growth and spread of cancerous cells. Specifically, it inhibits downstream signaling pathways, such as mitogen-activated protein kinase (MAPK) and phosphatidylinositol-4,5-bisphosphate 3-kinase (PI3k)/Akt activation which in turn inhibit cancer cell proliferation, differentiation, adhesion, migration and survival (492–494). EGFR overexpression has been found in about 40–80% of lung cancer patients as well as in many other cancers including squamous NSCLC (495, 496).
Necitumumab was firstly approved by US FDA for the treatment of metastatic squamous NSCLC combined with gemcitabine and cisplatin in 2015 (497). Clinical trials for necitumumab were initiated in 2004 (498), and currently, it is being tested in 6 clinical trials (NCT02496663, NCT00982111, NCT02789345, NCT00981058, NCT03944772, NCT03387111) mostly on NSCLC.
Adalimumab (Humira®)
Adalimumab is the first phage display human IgG1-κ derived mAb developed by humanization with a “guided selection method” involving a mouse mAb (180). In 2002, adalimumab was the first human antibody derived from phage display that was granted approval for therapy by US FDA (Table 2) (172). Adalimumab is the biggest selling drug worldwide with $19.1 billion in 2019 and $82.5 billion cumulatively between 2014 and 2018 (7, 8). It shows very high specificity and sub-nanomolar affinity as it binds with tumor necrosis factor (TNF) and inhibits TNF receptors (TNF-R1 and -R2) binding and activation (499). This inhibition pathway leads to a wide range of anti-inflammatory responses as TNF is a key regulator for the initiation of proinflammatory cytokine cascade which ultimately leads to cell activation, inflammation, fever, and apoptosis (500).
Adalimumab was firstly indicated as a therapeutic option for some moderate and severe types of rheumatoid arthritis (RA) as monotherapy or in conjunction with MTX or other anti-rheumatic medications. Nowadays, adalimumab is one of the most prescribed medicines in immune-mediated disorders including RA (approved in 2002), psoriatic arthritis (PsA) (approved in 2005), ankylosing spondylitis (approved in 2006), Crohn's disease (approved in 2010), psoriasis and juvenile idiopathic arthritis (approved in 2008), ulcerative colitis (approved in 2012), hidradenitis suppurativa (HS) (approved in 2015), uveitis (approved in 2016), and fingernail psoriasis (approved in 2018). Currently, there are 118 clinical trials listed in ClinicalTrials.gov for adalimumab, and these studies ranging between phase I to phase IV.
Raxibacumab (Abthrax®)
Raxibacumab is a human IgG1-λ human mAb that was produced from a naive human scFv phage display library licensed from Cambridge Antibody Technology (CAT) by Human Genome Sciences (HGS), which has been later acquired by GlaxoSmithKline (GSK) (331, 501). In 2012, raxibacumab was first granted FDA approval under the trade name of Abthrax® to be indicated as a prophylaxis for the treatment of inhalational anthrax in combination with some antibiotics. Anthrax infection is caused by bacteria called Bacillus anthracis (B. anthracis) through skin abrasions, inhalation or ingestion, where its spores are usually phagocytosed by macrophages (502). Moreover, B. anthracis is categorized as a potential biological weapon according to the US Centers for Disease Control and Prevention (CDC) (503).
B. anthracis secretes the lethal toxin (LT) and the edema toxin (ET). The LT is formed when the lethal factor (LF) interacts with the protective antigen (PA), which a cell-binding protein, while the ET is formed by an interaction between the PA and the edema factor (EF) (504). Raxibacumab targets the PA in B. Anthracis with high affinity to the LT (505) and acts through neutralizing PA with a nanomolar concentrations (IC50 is ~0.21 nm and Kd is ~2.78 nM). The mechanism of action of raxibacumab depends on the downregulation of the cellular uptake of toxins to prevent the development of lethal complexes (332).
Currently, in the US, raxibacumab is not only indicated for the prophylaxis of inhaled anthrax but also when alternative therapeutic options do not exist or are not suitable, such as treatment for an antibiotic-resistant strain of B. anthracis. Raxibacumab monotherapy of antibiotic-resistant B. anthracis infection suggests a benefit for up to 6 days post-exposure (NCT00639678) (506).
Belimumab (Benlysta®)
Belimumab is a human IgG1-λ mAb that was discovered through a collaboration between HGS and CAT. Belimumab recognizes and binds to the soluble B lymphocyte stimulator (BLyS), preventing its interaction with its receptors (507). BLyS is a critical factor in the selection, maturation, and survival of B cells (508). BLyS is produced by a wide variety of cell types, including myeloid lineage cells, activated T cells, malignant B cells, and stromal cells (509–513). BLyS has three receptors that are expressed predominantly on B lineage cells, and some are found on subsets of activated T cells and dendritic cells (514, 515).
Patients with systemic lupus erythematosus (SLE) have elevated levels of BLyS, which correlate with high levels of autoantibodies and disease activity (516). Belimumab was FDA approved in 2011 and considered as the first biological drug, immunosuppressant, approved for the treatment of SLE. Long-term belimumab treatment causes a significant reduction of most plasma cells that are responsible for autoantibodies production (517, 518).
Belimumab is currently being tested in seven active clinical trials. These include a phase IV clinical trial to identify the side effects of belimumab when given with other SLE medications in adults with active SLE (NCT01705977). Also, in a Phase II study to evaluate the efficacy and safety of belimumab as a maintenance therapy in adults with refractory idiopathic inflammatory myositis (IIM) (NCT02347891). It is also being investigated in Phase II studies to evaluate its efficacy in combination with rituximab in adults with systemic SLE (NCT02426125) and in subjects with primary Sjogren's syndrome (NCT02631538).
Ramucirumab (Cyramza®)
Ramucirumab is a fully human IgG1-κ mAb that inhibits vascular endothelial growth factor receptor-2 (VEGFR-2), thus inhibiting downstream signaling and preventing angiogenesis within tumors (519). In the adults, VEGFR-2 is predominantly expressed on vascular endothelial cells of blood vessels (520). Increased levels of VEGFR-2 have been detected in mammary, colorectal cancer, NSCLC, UC, and several other cancers (521).
The FDA approved ramucirumab in 2014 for use in the second-line setting as a single-agent treatment for advanced or metastatic gastric cancer or gastroesophageal junction adenocarcinoma (522). Also, in 2014, ramucirumab was approved in combination with docetaxel, for treatment of metastatic NSCLC (523). In 2015, it was approved for use with FOLFIRI as a second-line treatment of metastatic colorectal cancer (524). In 2019, ramucirumab became the first FDA-approved biomarker-driven therapy in patients with hepatocellular carcinoma for people who have high levels of alpha-fetoprotein (525).
Currently, ramucirumab is being investigated in 19 different clinical trials for several other indications including a phase II randomized trial in combination with mFOLFIRINOX in patients with advanced pancreatic cancer (NCT02581215), phase III study in combination with chemotherapy treatment in previously untreated patients with HER2-negative, unresectable, locally-recurrent, or metastatic breast cancer (NCT00703326), and phase III trial in combination with docetaxel in patients with locally advanced or unresectable or metastatic UC (NCT02426125).
Guselkumab (Tremfya™)
Guselkumab is a human IgG1-λ mAb that neutralizes interleukin-23 (IL-23) functions (526). IL-23 is a pleiotropic, heterodimeric cytokine, consisting of a p19 and a p40 subunits, which are primarily secreted by antigen presenting cells, such as macrophages and dendritic cells (527). IL-23 belongs to the IL-6/IL-12 family of cytokines that have a crucial role in numerous immune responses (528). IL-23 specifically induces Th-17 proliferation and the subsequent release of IL-17 cytokine, which triggers inflammatory and autoimmune disorders, such as psoriasis (529). Guselkumab binds to IL-23p19 subunit with high affinity and specificity, inhibiting interaction with its receptor on the cell surface of certain immune cell subsets, most importantly on Th17 cells (529, 530). Such activity is responsible for preventing the activation of the IL-23 receptor and the subsequent production of several proinflammatory cytokines.
Guselkumab has demonstrated safety and efficacy in several clinical trials including a phase II proof-of-concept trial, which demonstrated efficacy in all endpoints linked to health-related quality of life as well as joint signs and symptoms, and skin disease (399, 400, 531). Patients from this study have also experienced a dramatic decrease in IL-17A, IL-17F, and C-reactive protein in their serum to normal levels compared with healthy controls, highlighting the significance of suppressing the IL-23/Th17 pathway for the treatment of skin and joint disorders (531). This encouraging trial has led to two pivotal phase III clinical trials, DISCOVER-1 (NCT03162796) and DISCOVER-2 (NCT03162796), where patients had experienced an improved joint, skin, physical function and health-related quality of life (532, 533). In 2017, this mAb has received approval from the US FDA to treat adults with moderate to severe plaque psoriasis who are candidates for systemic therapy or phototherapy (398) and is currently being evaluated in six active clinical trials at different phases. These include the evaluation of efficacy, safety and tolerability in patients with moderate to severe HS (NCT03628924) as well as patients with chronic plaque-type psoriasis refractory to ustekinumab treatment (NCT03553823).
Lanadelumab (Takhzyro®)
Lanadelumab is a fully human IgG1-κ mAb that inhibits the proteolytic activity of plasma kallikrein (PK) enzyme. PK enzyme induces the proteolysis of the coagulation factor XII and prekallikrein (pKal), and a non-enzymatic high-molecular-weight kininogen (HMWK) to generate bradykinin in response to tissue injury and pathophysiological stimuli (534–536). The increased level of bradykinin leads to angioedema episodes, an allergic skin swelling condition, through its excessive vasodilation effect (537, 538). This syndrome is a clinical feature of patients with hereditary angioedema (HAE), in which, a mutation in the SERPING1 gene leads to a reduced expression of C1 protein that lessens its function as a bradykinin regulator (539). Furthermore, certain mutations in the F12 gene result in the production of factor XII with increased activity leading to excessive production of bradykinin (540).
In phase I clinical trial, lanadelumab demonstrated a favorable safety profile with a potential inhibitory effect on HMWK and a long-term prevention of HAE attacks, enabling further evaluation in a larger trial (541, 542). Accordingly, a phase III randomized clinical trial (NCT02586805) evaluated the efficacy and safety of lanadelumab to prevent HAE attacks in patients with symptomatic HAE due to C1 inhibitor deficiency (C1-INH-HAE) disorder (403). In this trial, 150 or 300 mg were evaluated in subcutaneous injections setting, given every 2–4 weeks over a 6-months period. The findings from this study have demonstrated the efficacy of lanadelumab in preventing HAE attacks, leading to its approval by the US FDA in 2018 for the treatment of patients with type I or II HAE. In 2018, lanadelumab has received the US FDA approval for the prevention of the angioedema attacks in patients with hereditary angioedema. Currently, it is being evaluated in two active phase III clinical trials to prevent hereditary angioedema attacks in pediatric patients as well as in adolescent and adult patients suffering from acquired angioedema (AAE) due to C1-INH deficiency (NCT04070326 and NCT04206605).
Ixekizumab (Taltz®)
Ixekizumab is a humanized IgG4-κ mAb that targets IL-17A cytokine, which is a member of IL-17 cytokines family mainly produced by CD4-Th17 cells. Several other immune cells residing in the gut, lung and skin, including a subset of natural killer (NK) cells, Paneth cells and neutrophils also produce IL-17A in response to IL-23 cytokine stimulation (248, 543). The main function of Th17 cells is to clear pathogens not properly handled by the Th1 or Th2 immune response (544, 545). The infiltration of Th17 cells under the skin and excessive production of IL17A lead to the pathophysiology of psoriasis and PsA (546). The latter is an inflammatory disease with articular, peri-articular and extra-articular features that leads to skin and joint damage, and loss of functions (547). Several attributes lead to this condition including immunologic, genetic and environmental factors where a combination of two or more of these factors trigger the inflammatory immune response (548–551). There has been growing evidence suggesting the involvement of IL-17 signaling in PsA pathogenesis (552), which involves a persistent activation of Th-17 cells in response to synovial or skin antigens leading to tissue destruction and joint remodeling (552).
Several randomized clinical trials have assessed the efficacy of ixekizumab in patients with PsA achieving a primary endpoint of American College of Rheumatology 20% improvement (ACR20) (NCT01624233, NCT02349295, NCT01695239, NCT02584855). Moreover, ixekizumab has been proven to be superior to conventional rheumatic drugs as well as TNF-α inhibitors in two phase III clinical trials, indicating its safety and efficacy in delaying disease progression and supporting its use as a front-line therapy for PsA (249, 553, 554).
Lxekizumab was initially approved in 2016 by the US FDA for moderate to severe plaque psoriasis treatment in adult patients who are eligible for systematic therapy or phototherapy. The FDA approval was further expanded in 2017 for the treatment of adults suffering from active PsA. Furthermore, the US FDA approved Ixekizumab in 2019 for the treatment of active ankylosing spondylitis in adults. In early 2020, Ixekizumab has also granted the US FDA approval for the treatment of pediatric patients (ages 6 to 18) with moderate to severe plaque psoriasis who are also eligible for systemic therapy or phototherapy. Currently, ixekizumab is being evaluated in four active clinical trials. These include a phase IV clinical trial assessing the efficacy of ixekizumab in Japanese patients with generalized pustular psoriasis and erythrodermic psoriasis (NCT03942042). In addition, this mAb is also being tested in Chinese patients who have moderate to severe plaque psoriasis (NCT03364309).
Ranibizumab (Lucentis®)
Ranibizumab is a Fab antibody fragment derived from a recombinant humanized IgG1-κ mAb (murine Mab A.4.6.1) (555). Ranibizumab was created from the same parent mouse antibody as bevacizumab to target VEGF-A, both bind effectively and neutralize VEGF-A isoforms (555). VEGF-A and its receptors VEGFR-1 and VEGFR-2 promote angiogenesis throughout the body and represent the primary mediators of degenerative ocular conditions, such as diabetic retinopathy, retinal vein occlusions, age-related macular degeneration (AMD) including wet-AMD, the leading cause of blindness in the elderly population (556, 557). Ranibizumab has smaller size than bevacizumab and readily penetrates all layers of the retina after intravitreal injection (558). Importantly, ranibizumab is thought to be safer on normal healthy cells that express VEGF-A as it has shorter serum half-life and faster system clearance (559). The US FDA approved ranibizumab for the treatment of wet AMD in June 2006, macular edema following retinal vein occlusion in 2010, diabetic macular edema in 2012, diabetic retinopathy in people with diabetic macular edema in 2015, and myopic choroidal neovascularization in 2017.
Currently, there are 18 active clinical trials to evaluate ranibizumab as single or in combination with other treatments. One phase IV clinical study is comparing the safety and efficacy between ranibizumab as monotherapy and in combination with R:GEN (selective retina therapy) in clinically significant diabetic macular edema (NCT03759860). Also, another phase IV clinical study is comparing intravitreal ranibizumab and triamcinolone acetonide combination therapy vs. ranibizumab monotherapy in patients with polypoidal choroidal vasculopathy (NCT02806752). Long-term efficacy and safety of intravitreal ranibizumab compared with laser ablation therapy in patients who were treated for retinopathy of prematurity (ROP) (NCT02640664) is in phase III stage.
Caplacizumab (Cablivi™)
Caplacizumab is a humanized bivalent single-variable domain immunoglobulin (VHH) that consists of two identical, genetically linked, humanized building blocks (298). Caplacizumab was generated for the treatment of platelet adhesion diseases, such as acquired thrombotic thrombocytopenic purpura (aTTP) (298). Caplacizumab binds specifically to human von Willebrand factor (vWF) A1 domain, preventing its binding to the platelet glycoprotein 1b receptor (298). Acquired TTP is a rare blood disorder characterized by thrombosis in small blood vessels and low platelet count. It is caused by a severe deficiency in the vWF-cleaving protease (ADAMTS13) primarily due to acquired autoantibodies (560). Lacking ADAMTS13 enhances the accumulation of large multimers of vWF, vWF–platelet aggregation, and microvascular thrombosis of TTP, leading to low platelet count, ischemia, and organ dysfunction (560, 561).
Clinical studies showed that treatment with caplacizumab transiently reduced vWF levels and normalized platelet count compared with placebo (562). Target-bound caplacizumab is thought to be metabolized within the liver, while unbound caplacizumab is cleared renally (299). Caplacizumab received its first approval in September 2018 in the EU for the treatment of adults experiencing aTTP in conjunction with plasma exchange and immunosuppression (corticosteroids and increasingly also rituximab) (11). Caplacizumab is the first nanobody-based medication to receive approval in the US in 2019, for patients aged ≥18 years experiencing aTTP (11). Currently, one active phase III clinical trial (NCT02878603) is evaluating the long-term safety and efficacy of repeated use of caplacizumab in aTTP patients.
Emapalumab (Gamifant®)
Emapalumab is a human IgG1-λ mAb that neutralizes interferon gamma (IFNγ) activities and inhibits its binding to the interferon receptors (IFNγR1 and IFNγR2). It binds with high affinity to free and receptor-bound IFNγ, preventing the downstream signaling of JAK/STAT pathway and the subsequent cytokine storm release (563, 564). This blockade results in the attenuation of the adaptive and innate immune responses, which increase the susceptibility to infections (564). It has been primarily developed to treat patients with haemophagocytic lymphohistiocytosis (HLH) disorder refractory to conventional therapy (565, 566). HLH is a rare pathologic immune activation syndrome with excessive inflammation that occurs as a familial or sporadic disorder due to a variety of immune triggers (567).
A phase I dose-escalation clinical trial investigating the safety in healthy subjects, revealed a favorable safety profile warranting further clinical development (NCT01459562). Emapalumab was then evaluated in an open-label phase II/III trial in 34 pediatric patients with a confirmed HLH disorder. Emapalumab was administered with dexamethasone intravenously every 3–4 days for a minimum of 4 weeks and up to 8 weeks with a primary endpoint of overall response rate (ORR) (NCT01818492). Patients have experienced a response rates above 70 with a safe and tolerable profile and mild-moderate infusion-related reactions in 27% of the patients confirming its favorable outcomes (568). This trial has led to the US FDA approval of emapalumab in 2018 as an IFN blocking molecule for pediatric and adult patients with HLH refractory to conventional therapy (dexamethasone, etoposide, and intrathecal methotrexate) (565, 566). Currently, the efficacy of emapalumab is being evaluated in four active phase II/III clinical trials in patients with primary and secondary HLH (NCT03312751, NCT03985423, NCT01818492, NCT03311854). In addition, the safety and efficacy of emapalumab are also being tested in COVID-19 patients, with a primary endpoint of reducing the number of patients who need invasive mechanical ventilation or extracorporeal membrane oxygenation (NCT04324021).
Conclusion and Future Prospects
Antibody phage display is a versatile, reproducible, and functional technology that can be utilized to isolate antibody candidates for numerous disease indications. While it is the most common and well-established form of display technologies, the success of isolating useful antibodies is highly dependent on the quality and the nature of the targeted antigen used in biopanning and the size and quality of the library. By performing cell-based biopanning, antibody phage display can be used to identify new cell biomarkers, isolate antibodies that can discriminate between different antigen epitopes and conformations, or identify antibodies against antigens that are not available in pure form (74).
Phage display technology has been proven to be a powerful human mAb discovery platform. Not long ago the commercial use of phage display was restricted to a few selected biopharmaceutical companies with rights to phage display intellectual property (569). This explains why most of the approved mAbs or those in clinical trials sourced from phage display libraries belong to commercial entities with rights to the technology. However, most of the key patents covering phage display technology have expired in Europe and the US (569). Consequently, patent expiration should encourage academic and biotech start-ups to develop their own libraries to generate and develop more antibodies for translation to the clinic.
By reflecting on the collected data, antibody phage display has contributed to the isolation of antibodies for the treatment of many disease indications. There are 14 FDA approved phage display-derived antibodies and antibody fragments, and many others in clinical trials. Many research institutes, start-ups and industrial laboratories are continually developing methods for the design, construction and screening of developable antibody-phage libraries. Further improvements are expected to be achieved in the near future as this technology contributes significantly toward research, diagnosis, and therapy.
Author Contributions
MA and AH designed the review. MA, HA, AM, AA, and MJ wrote the main manuscript text. MA prepared the figures and analyzed the data. MJ, SM, and AH provided a substantial contribution to the discussion of the content and helped with reviewing and editing the article. All authors read and approved the final manuscript.
Funding
The authors extend their appreciation to the deputyship for Research and Innovation, Ministry of Education in Saudi Arabia for funding this research work through the project number (436).
Conflict of Interest
The authors declare that the research was conducted in the absence of any commercial or financial relationships that could be construed as a potential conflict of interest.
References
1. Weiner GJ. Building better monoclonal antibody-based therapeutics. Nat Rev Cancer. (2015) 15:361–70. doi: 10.1038/nrc3930
2. Schoonbroodt S, Steukers M, Viswanathan M, Frans N, Timmermans M, Wehnert A, et al. Engineering antibody heavy chain CDR3 to create a phage display Fab library rich in antibodies that bind charged carbohydrates. J Immunol. (2008) 181:6213–21. doi: 10.4049/jimmunol.181.9.6213
3. Zhang P, Sun F, Liu S, Jiang S. Anti-PEG antibodies in the clinic: Current issues and beyond PEGylation. J Control Release. (2016) 244:184–93. doi: 10.1016/j.jconrel.2016.06.040
4. Watanabe H, Nakanishi T, Umetsu M, Kumagai I. Human anti-gold antibodies: biofunctionalization of gold nanoparticles and surfaces with anti-gold antibodies. J Biol Chem. (2008) 283:36031–8. doi: 10.1074/jbc.M805547200
5. Saeed AF, Wang R, Ling S, Wang S. Antibody engineering for pursuing a healthier future. Front Microbiol. (2017) 8:495. doi: 10.3389/fmicb.2017.00495
6. Kaplon H, Muralidharan M, Schneider Z, Reichert JM. Antibodies to watch in 2020. mAbs. (2020) 12:1703531. doi: 10.1080/19420862.2019.1703531
7. Urquhart L. Top companies and drugs by sales in 2019. Nat Rev Drug Discov. (2020) 19:228. doi: 10.1038/d41573-019-00049-0
8. Walsh G. Biopharmaceutical benchmarks 2018. Nat Biotechnol. (2018) 36:1136–45. doi: 10.1038/nbt.4305
9. Grilo AL, Mantalaris A. The increasingly human and profitable monoclonal antibody market. Trends Biotechnol. (2019) 37:9–16. doi: 10.1016/j.tibtech.2018.05.014
10. Hay M, Thomas DW, Craighead JL, Economides C, Rosenthal J. Clinical development success rates for investigational drugs. Nat Biotechnol. (2014) 32:40–51. doi: 10.1038/nbt.2786
11. Kaplon H, Reichert JM. Antibodies to watch in 2019. mAbs. (2019) 11:219–38. doi: 10.1080/19420862.2018.1556465
12. Kaufmann SH. Remembering Emil von Behring: from tetanus treatment to antibody cooperation with phagocytes. MBio. (2017) 8:e00117-17. doi: 10.1128/mBio.00117-17
13. Kohler G, Milstein C. Continuous cultures of fused cells secreting antibody of predefined specificity. Nature. (1975) 256:495–7. doi: 10.1038/256495a0
14. Smith GP. Filamentous fusion phage: novel expression vectors that display cloned antigens on the virion surface. Science. (1985) 228:1315–7. doi: 10.1126/science.4001944
15. McCafferty J, Griffiths AD, Winter G, Chiswell DJ. Phage antibodies: filamentous phage displaying antibody variable domains. Nature. (1990) 348:552–4. doi: 10.1038/348552a0
17. Leach DR, Krummel MF, Allison JP. Enhancement of antitumor immunity by CTLA-4 blockade. Science. (1996) 271:1734–6. doi: 10.1126/science.271.5256.1734
18. Ledford H, Else H, Warren M. Cancer immunologists scoop medicine Nobel prize. Nature. (2018) 562:20–1. doi: 10.1038/d41586-018-06751-0
19. Legouffe E, Liautard J, Gaillard JP, Rossi JF, Wijdenes J, Bataille R, et al. Human anti-mouse antibody response to the injection of murine monoclonal antibodies against IL-6. Clin Exp Immunol. (1994) 98:323–9. doi: 10.1111/j.1365-2249.1994.tb06145.x
20. Berger M, Shankar V, Vafai A. Therapeutic applications of monoclonal antibodies. Am J Med Sci. (2002) 324:14–30. doi: 10.1097/00000441-200207000-00004
21. Morrison SL, Oi VT. Genetically engineered antibody molecules. Adv Immunol. (1989) 44:65–92. doi: 10.1016/S0065-2776(08)60640-9
22. Studnicka GM, Soares S, Better M, Williams RE, Nadell R, Horwitz AH. Human-engineered monoclonal antibodies retain full specific binding activity by preserving non-CDR complementarity-modulating residues. Protein Eng. (1994) 7:805–14. doi: 10.1093/protein/7.6.805
23. Jones PT, Dear PH, Foote J, Neuberger MS, Winter G. Replacing the complementarity-determining regions in a human antibody with those from a mouse. Nature. (1986) 321:522–5. doi: 10.1038/321522a0
24. Lonberg N, Taylor LD, Harding FA, Trounstine M, Higgins KM, Schramm SR, et al. Antigen-specific human antibodies from mice comprising four distinct genetic modifications. Nature. (1994) 368:856–9. doi: 10.1038/368856a0
25. Mendez MJ, Green LL, Corvalan JR, Jia XC, Maynard-Currie CE, Yang XD, et al. Functional transplant of megabase human immunoglobulin loci recapitulates human antibody response in mice. Nat Genet. (1997) 15:146–56. doi: 10.1038/ng0297-146
26. Lonberg N. Human antibodies from transgenic animals. Nat Biotechnol. (2005) 23:1117–25. doi: 10.1038/nbt1135
27. Parmley SF, Smith GP. Antibody-selectable filamentous FD phage vectors: affinity purification of target genes. Gene. (1988) 73:305–18. doi: 10.1016/0378-1119(88)90495-7
28. Huang JX, Bishop-Hurley SL, Cooper MA. Development of anti-infectives using phage display: biological agents against bacteria, viruses, and parasites. Antimicrob Agents Chemother. (2012) 56:4569–82. doi: 10.1128/AAC.00567-12
29. Sokullu E, Soleymani Abyaneh H, Gauthier AM. Plant/bacterial virus-based drug discovery, drug delivery, and therapeutics. Pharmaceutics. (2019) 11:211. doi: 10.3390/pharmaceutics11050211
30. O'Callaghan R, Bradley R, Paranchych W. The effect of M13 phage infection upon the F pili of E. coli. Virology. (1973) 54:220–9. doi: 10.1016/0042-6822(73)90131-1
31. Specthrie L, Bullitt E, Horiuchi K, Model P, Russel M, Makowski L. Construction of a microphage variant of filamentous bacteriophage. J Mol Biol. (1992) 228:720–4. doi: 10.1016/0022-2836(92)90858-H
32. van Wezenbeek PM, Hulsebos TJ, Schoenmakers JG. Nucleotide sequence of the filamentous bacteriophage M13 DNA genome: comparison with phage fd. Gene. (1980) 11:129–48. doi: 10.1016/0378-1119(80)90093-1
33. Rakonjac J, Bennett NJ, Spagnuolo J, Gagic D, Russel M. Filamentous bacteriophage: biology, phage display and nanotechnology applications. Curr Issues Mol Biol. (2011) 13:51–76. doi: 10.21775/cimb.013.051
34. Sidhu SS, Weiss GA, Wells JA. High copy display of large proteins on phage for functional selections. J Mol Biol. (2000) 296:487–95. doi: 10.1006/jmbi.1999.3465
35. Sheehan J, Marasco WA. Phage and yeast display. Microbiol Spectrum. (2015) 3:AID-0028–2014. doi: 10.1128/9781555817411.ch6
36. Kehoe JW, Kay BK. Filamentous phage display in the new millennium. Chem Rev. (2005) 105:4056–72. doi: 10.1021/cr000261r
37. Breitling F, Dubel S, Seehaus T, Klewinghaus I, Little M. A surface expression vector for antibody screening. Gene. (1991) 104:147–53. doi: 10.1016/0378-1119(91)90244-6
38. Clackson T, Hoogenboom HR, Griffiths AD, Winter G. Making antibody fragments using phage display libraries. Nature. (1991) 352:624–8. doi: 10.1038/352624a0
39. Bates A, Power AC. David vs. Goliath: the structure, function, and clinical prospects of antibody fragments. Antibodies. (2019) 8:28. doi: 10.3390/antib8020028
40. Barbas CF III, Kang AS, Lerner RA, Benkovic SJ. Assembly of combinatorial antibody libraries on phage surfaces: the gene III site. Proc Natl Acad Sci USA. (1991) 88:7978–82. doi: 10.1073/pnas.88.18.7978
41. Vincke C, Muyldermans S. Introduction to heavy chain antibodies and derived nanobodies. In: Saerens D, Muyldermans S, editors. Single Domain Antibodies: Methods and Protocols. Totowa, NJ: Humana Press (2012). p. 15–26. doi: 10.1007/978-1-61779-968-6_2
42. Kwon N-Y, Kim Y, Lee J-O. Structural diversity and flexibility of diabodies. Methods. (2019) 154:136–42. doi: 10.1016/j.ymeth.2018.09.005
43. Lowman HB, Bass SH, Simpson N, Wells JA. Selecting high-affinity binding proteins by monovalent phage display. Biochemistry. (1991) 30:10832–8. doi: 10.1021/bi00109a004
44. Bass S, Greene R, Wells JA. Hormone phage: an enrichment method for variant proteins with altered binding properties. Proteins. (1990) 8:309–14. doi: 10.1002/prot.340080405
45. Sidhu SS. Engineering M13 for phage display. Biomol Eng. (2001) 18:57–63. doi: 10.1016/S1389-0344(01)00087-9
47. Hammers CM, Stanley JR. Antibody phage display: technique and applications. J Invest Dermatol. (2014) 134:e17. doi: 10.1038/jid.2013.521
48. Rakonjac J, Jovanovic G, Model P. Filamentous phage infection-mediated gene expression: construction and propagation of the gIII deletion mutant helper phage R408d3. Gene. (1997) 198:99–103. doi: 10.1016/S0378-1119(97)00298-9
49. Ledsgaard L, Kilstrup M, Karatt-Vellatt A, McCafferty J, Laustsen AH. Basics of antibody phage display technology. Toxins (Basel). (2018) 10:236. doi: 10.3390/toxins10060236
50. Rondot S, Koch J, Breitling F, Dübel S. A helper phage to improve single-chain antibody presentation in phage display. Nat Biotechnol. (2001) 19:75–8. doi: 10.1038/83567
51. Marks JD, Hoogenboom HR, Bonnert TP, McCafferty J, Griffiths AD, Winter G. By-passing immunization. Human antibodies from V-gene libraries displayed on phage. J Mol Biol. (1991) 222:581–97. doi: 10.1016/0022-2836(91)90498-U
52. Kang AS, Barbas CF, Janda KD, Benkovic SJ, Lerner RA. Linkage of recognition and replication functions by assembling combinatorial antibody Fab libraries along phage surfaces. Proc Natl Acad Sci USA. (1991) 88:4363–6. doi: 10.1073/pnas.88.10.4363
53. Schirrmann T, Meyer T, Schütte M, Frenzel A, Hust M. Phage display for the generation of antibodies for proteome research, diagnostics and therapy. Molecules. (2011) 16:412–26. doi: 10.3390/molecules16010412
54. Shen W, Li S, Park M-K, Zhang Z, Cheng Z, Petrenko VA, et al. Blocking agent optimization for nonspecific binding on phage based magnetoelastic biosensors. J Electrochem Soc. (2012) 159:B818–23. doi: 10.1149/2.057210jes
55. Menendez A, Scott JK. The nature of target-unrelated peptides recovered in the screening of phage-displayed random peptide libraries with antibodies. Anal Biochem. (2005) 336:145–57. doi: 10.1016/j.ab.2004.09.048
56. Smith GP, Scott JK. Libraries of peptides and proteins displayed on filamentous phage. Methods Enzymol. (1993) 217:228–57. doi: 10.1016/0076-6879(93)17065-D
57. Haque A, Tonks NK. The use of phage display to generate conformation-sensor recombinant antibodies. Nat Protoc. (2012) 7:2127–43. doi: 10.1038/nprot.2012.132
58. Ward RL, Clark MA, Lees J, Hawkins NJ. Retrieval of human antibodies from phage-display libraries using enzymatic cleavage. J Immunol Methods. (1996) 189:73–82. doi: 10.1016/0022-1759(95)00231-6
59. Kristensen P, Winter G. Proteolytic selection for protein folding using filamentous bacteriophages. Fold Des. (1998) 3:321–8. doi: 10.1016/S1359-0278(98)00044-3
60. Pande J, Szewczyk MM, Grover AK. Phage display: concept, innovations, applications and future. Biotechnol Adv. (2010) 28:849–58. doi: 10.1016/j.biotechadv.2010.07.004
61. Jones ML, Seldon T, Smede M, Linville A, Chin DY, Barnard R, et al. A method for rapid, ligation-independent reformatting of recombinant monoclonal antibodies. J Immunol Methods. (2010) 354:85–90. doi: 10.1016/j.jim.2010.02.001
62. Thie H, Voedisch B, Dubel S, Hust M, Schirrmann T. Affinity maturation by phage display. Methods Mol Biol. (2009) 525:309–22, xv. doi: 10.1007/978-1-59745-554-1_16
63. Kobayashi N, Oyama H, Kato Y, Goto J, Soderlind E, Borrebaeck CA. Two-step in vitro antibody affinity maturation enables estradiol-17beta assays with more than 10-fold higher sensitivity. Anal Chem. (2010) 82:1027–38. doi: 10.1021/ac902283n
64. Fellouse FA, Esaki K, Birtalan S, Raptis D, Cancasci VJ, Koide A, et al. High-throughput generation of synthetic antibodies from highly functional minimalist phage-displayed libraries. J Mol Biol. (2007) 373:924–40. doi: 10.1016/j.jmb.2007.08.005
65. Mukherjee S, Ura M, Hoey RJ, Kossiakoff AA. A new versatile immobilization tag based on the ultra high affinity and reversibility of the calmodulin-calmodulin binding peptide interaction. J Mol Biol. (2015) 427:2707–25. doi: 10.1016/j.jmb.2015.06.018
66. Santos R, Ursu O, Gaulton A, Bento AP, Donadi RS, Bologa CG, et al. A comprehensive map of molecular drug targets. Nat Rev Drug Discov. (2016) 16:19–34. doi: 10.1038/nrd.2016.230
67. Sarkar CA, Dodevski I, Kenig M, Dudli S, Mohr A, Hermans E, et al. Directed evolution of a G protein-coupled receptor for expression, stability, and binding selectivity. Proc Natl Acad Sci USA. (2008) 105:14808–13. doi: 10.1073/pnas.0803103105
68. White SH, Wimley WC. Membrane protein folding and stability: physical principles. Annu Rev Biophys Biomol Struct. (1999) 28:319–65. doi: 10.1146/annurev.biophys.28.1.319
69. Butler JE, Navarro P, Sun J. Adsorption-induced antigenic changes and their significance in ELISA and immunological disorders. Immunol Invest. (1997) 26:39–54. doi: 10.3109/08820139709048914
70. Butler JE, Ni L, Nessler R, Joshi KS, Suter M, Rosenberg B, et al. The physical and functional behavior of capture antibodies adsorbed on polystyrene. J Immunol Methods. (1992) 150:77–90. doi: 10.1016/0022-1759(92)90066-3
71. Nagy P, Friedlander E, Tanner M, Kapanen AI, Carraway KL, Isola J, et al. Decreased accessibility and lack of activation of ErbB2 in JIMT-1, a herceptin-resistant, MUC4-expressing breast cancer cell line. Cancer Res. (2005) 65:473–82.
72. Lipes BD, Chen YH, Ma H, Staats HF, Kenan DJ, Gunn MD. An entirely cell-based system to generate single-chain antibodies against cell surface receptors. J Mol Biol. (2008) 379:261–72. doi: 10.1016/j.jmb.2008.03.072
73. Jones ML, Alfaleh MA, Kumble S, Zhang S, Osborne GW, Yeh M, et al. Targeting membrane proteins for antibody discovery using phage display. Sci Rep. (2016) 6:26240. doi: 10.1038/srep26240
74. Alfaleh AM, Jones LM, Howard BC, Mahler MS. Strategies for selecting membrane protein-specific antibodies using phage display with cell-based panning. Antibodies. (2017) 6:10. doi: 10.3390/antib6030010
75. Alfaleh AM, Arora N, Yeh M, de Bakker JC, Howard BC, Macpherson P, et al. Canine CD117-specific antibodies with diverse binding properties isolated from a phage display library using cell-based biopanning. Antibodies. (2019) 8:15. doi: 10.3390/antib8010015
76. McGuire MJ, Li S, Brown KC. Biopanning of phage displayed peptide libraries for the isolation of cell-specific ligands. Methods Mol Biol. (2009) 504:291–321. doi: 10.1007/978-1-60327-569-9_18
77. Siva AC, Kirkland RE, Lin B, Maruyama T, McWhirter J, Yantiri-Wernimont F, et al. Selection of anti-cancer antibodies from combinatorial libraries by whole-cell panning and stringent subtraction with human blood cells. J Immunol Methods. (2008) 330:109–19. doi: 10.1016/j.jim.2007.11.008
78. Krag DN, Shukla GS, Shen GP, Pero S, Ashikaga T, Fuller S, et al. Selection of tumor-binding ligands in cancer patients with phage display libraries. Cancer Res. (2006) 66:7724–33. doi: 10.1158/0008-5472.CAN-05-4441
79. Dantas-Barbosa C, de Macedo Brigido M, Maranhao AQ. Antibody phage display libraries: contributions to oncology. Int J Mol Sci. (2012) 13:5420–40. doi: 10.3390/ijms13055420
80. Rader C, Barbas CF III. Phage display of combinatorial antibody libraries. Curr Opin Biotechnol. (1997) 8:503–8. doi: 10.1016/S0958-1669(97)80075-4
81. Eisenhardt SU, Schwarz M, Bassler N, Peter K. Subtractive single-chain antibody (scFv) phage-display: tailoring phage-display for high specificity against function-specific conformations of cell membrane molecules. Nat Protoc. (2007) 2:3063–73. doi: 10.1038/nprot.2007.455
82. Schwarz M, Rottgen P, Takada Y, Le Gall F, Knackmuss S, Bassler N, et al. Single-chain antibodies for the conformation-specific blockade of activated platelet integrin alphaIIbbeta3 designed by subtractive selection from naive human phage libraries. FASEB J. (2004) 18:1704–6. doi: 10.1096/fj.04-1513fje
83. Schwarz M, Meade G, Stoll P, Ylanne J, Bassler N, Chen YC, et al. Conformation-specific blockade of the integrin GPIIb/IIIa: a novel antiplatelet strategy that selectively targets activated platelets. Circ Res. (2006) 99:25–33. doi: 10.1161/01.RES.0000232317.84122.0c
84. Eisenhardt SU, Schwarz M, Schallner N, Soosairajah J, Bassler N, Huang D, et al. Generation of activation-specific human anti-alphaMbeta2 single-chain antibodies as potential diagnostic tools and therapeutic agents. Blood. (2007) 109:3521–8. doi: 10.1182/blood-2006-03-007179
85. Paduch M, Koide A, Uysal S, Rizk SS, Koide S, Kossiakoff AA. Generating conformation-specific synthetic antibodies to trap proteins in selected functional states. Methods. (2013) 60:3–14. doi: 10.1016/j.ymeth.2012.12.010
86. Sorensen MD, Agerholm IE, Christensen B, Kolvraa S, Kristensen P. Microselection–affinity selecting antibodies against a single rare cell in a heterogeneous population. J Cell Mol Med. (2010) 14:1953–61. doi: 10.1111/j.1582-4934.2010.00896.x
87. Sorensen MD, Kristensen P. Selection of antibodies against a single rare cell present in a heterogeneous population using phage display. Nat Protoc. (2011) 6:509–22. doi: 10.1038/nprot.2011.311
88. Yoo DK, Lee SR, Jung Y, Han H, Lee HK, Han J, et al. Machine learning-guided prediction of antigen-reactive in silico clonotypes based on changes in clonal abundance through bio-panning. Biomolecules. (2020) 10:421. doi: 10.3390/biom10030421
89. DeKosky BJ, Ippolito GC, Deschner RP, Lavinder JJ, Wine Y, Rawlings BM, et al. High-throughput sequencing of the paired human immunoglobulin heavy and light chain repertoire. Nat Biotechnol. (2013) 31:166–9. doi: 10.1038/nbt.2492
90. Sormanni P, Aprile FA, Vendruscolo M. Third generation antibody discovery methods: in silico rational design. Chem Soc Rev. (2018) 47:9137–57. doi: 10.1039/C8CS00523K
91. Noh J, Kim O, Jung Y, Han H, Kim JE, Kim S, et al. High-throughput retrieval of physical DNA for NGS-identifiable clones in phage display library. MAbs. (2019) 11:532–45. doi: 10.1080/19420862.2019.1571878
92. Raftery LJ, Howard CB, Grewal YS, Vaidyanathan R, Jones ML, Anderson W, et al. Retooling phage display with electrohydrodynamic nanomixing and nanopore sequencing. Lab Chip. (2019) 19:4083–92. doi: 10.1039/C9LC00978G
93. Kelley B. Developing therapeutic monoclonal antibodies at pandemic pace. Nat Biotechnol. (2020) 38:540–5. doi: 10.1038/s41587-020-0512-5
94. Griffiths AD, Williams SC, Hartley O, Tomlinson IM, Waterhouse P, Crosby WL, et al. Isolation of high affinity human antibodies directly from large synthetic repertoires. EMBO J. (1994) 13:3245–60. doi: 10.1002/j.1460-2075.1994.tb06626.x
95. Chennamsetty N, Voynov V, Kayser V, Helk B, Trout BL. Prediction of aggregation prone regions of therapeutic proteins. J Phys Chem B. (2010) 114:6614–24. doi: 10.1021/jp911706q
96. Jain T, Sun T, Durand S, Hall A, Houston NR, Nett JH, et al. Biophysical properties of the clinical-stage antibody landscape. Proc Natl Acad Sci USA. (2017) 114:944–9. doi: 10.1073/pnas.1616408114
97. Lecerf M, Kanyavuz A, Lacroix-Desmazes S, Dimitrov JD. Sequence features of variable region determining physicochemical properties and polyreactivity of therapeutic antibodies. Mol Immunol. (2019) 112:338–46. doi: 10.1016/j.molimm.2019.06.012
98. Lavoisier A, Schlaeppi JM. Early developability screen of therapeutic antibody candidates using Taylor dispersion analysis and UV area imaging detection. mAbs. (2015) 7:77–83. doi: 10.4161/19420862.2014.985544
99. Dobson CL, Devine PW, Phillips JJ, Higazi DR, Lloyd C, Popovic B, et al. Engineering the surface properties of a human monoclonal antibody prevents self-association and rapid clearance in vivo. Sci Rep. (2016) 6:38644. doi: 10.1038/srep38644
100. Bye JW, Platts L, Falconer RJ. Biopharmaceutical liquid formulation: a review of the science of protein stability and solubility in aqueous environments. Biotechnol Lett. (2014) 36:869–75. doi: 10.1007/s10529-013-1445-6
101. Garidel P, Kuhn AB, Schafer LV, Karow-Zwick AR, Blech M. High-concentration protein formulations: how high is high? Eur J Pharm Biopharm. (2017) 119:353–60. doi: 10.1016/j.ejpb.2017.06.029
102. Trevino SR, Scholtz JM, Pace CN. Measuring and increasing protein solubility. J Pharm Sci. (2008) 97:4155–66. doi: 10.1002/jps.21327
103. Gibson TJ, McCarty K, McFadyen IJ, Cash E, Dalmonte P, Hinds KD, et al. Application of a high-throughput screening procedure with PEG-induced precipitation to compare relative protein solubility during formulation development with IgG1 monoclonal antibodies. J Pharm Sci. (2011) 100:1009–21. doi: 10.1002/jps.22350
104. Sormanni P, Amery L, Ekizoglou S, Vendruscolo M, Popovic B. Rapid and accurate in silico solubility screening of a monoclonal antibody library. Sci Rep. (2017) 7:8200. doi: 10.1038/s41598-017-07800-w
105. Seeliger D, Schulz P, Litzenburger T, Spitz J, Hoerer S, Blech M, et al. Boosting antibody developability through rational sequence optimization. mAbs. (2015) 7:505–15. doi: 10.1080/19420862.2015.1017695
106. Chennamsetty N, Voynov V, Kayser V, Helk B, Trout BL. Design of therapeutic proteins with enhanced stability. Proc Natl Acad Sci USA. (2009) 106:11937–42. doi: 10.1073/pnas.0904191106
107. Lowe D, Dudgeon K, Rouet R, Schofield P, Jermutus L, Christ D. Aggregation, stability, and formulation of human antibody therapeutics. Adv Protein Chem Struct Biol. (2011) 84:41–61. doi: 10.1016/B978-0-12-386483-3.00004-5
108. Perchiacca JM, Tessier PM. Engineering aggregation-resistant antibodies. Annu Rev Chem Biomol Eng. (2012) 3:263–86. doi: 10.1146/annurev-chembioeng-062011-081052
109. Rosenberg AS. Effects of protein aggregates: an immunologic perspective. AAPS J. (2006) 8:E501–7. doi: 10.1208/aapsj080359
110. Li W, Prabakaran P, Chen W, Zhu Z, Feng Y, Dimitrov SD. Antibody aggregation: insights from sequence and structure. Antibodies. (2016) 5:19. doi: 10.3390/antib5030019
111. Spencer S, Bethea D, Raju TS, Giles-Komar J, Feng Y. Solubility evaluation of murine hybridoma antibodies. mAbs. (2012) 4:319–25. doi: 10.4161/mabs.19869
112. Kaleli NE, Karadag M, Kalyoncu S. Phage display derived therapeutic antibodies have enriched aliphatic content: insights for developability issues. Proteins. (2019) 87:607–18. doi: 10.1002/prot.25685
113. Coloma MJ, Trinh RK, Martinez AR, Morrison SL. Position effects of variable region carbohydrate on the affinity and in vivo behavior of an anti-(1 → 6) dextran antibody. J Immunol. (1999) 162:2162.
114. Huang L, Biolsi S, Bales KR, Kuchibhotla U. Impact of variable domain glycosylation on antibody clearance: an LC/MS characterization. Anal Biochem. (2006) 349:197–207. doi: 10.1016/j.ab.2005.11.012
115. Hotzel I, Theil FP, Bernstein LJ, Prabhu S, Deng R, Quintana L, et al. A strategy for risk mitigation of antibodies with fast clearance. mAbs. (2012) 4:753–60. doi: 10.4161/mabs.22189
116. Almagro JC, Daniels-Wells TR, Perez-Tapia SM, Penichet ML. Progress and challenges in the design and clinical development of antibodies for cancer therapy. Front Immunol. (2017) 8:1751. doi: 10.3389/fimmu.2017.01751
117. Gera N, Hussain M, Rao BM. Protein selection using yeast surface display. Methods. (2013) 60:15–26. doi: 10.1016/j.ymeth.2012.03.014
118. Boder ET, Raeeszadeh-Sarmazdeh M, Price JV. Engineering antibodies by yeast display. Arch Biochem Biophys. (2012) 526:99–106. doi: 10.1016/j.abb.2012.03.009
119. Boder ET, Wittrup KD. Yeast surface display for screening combinatorial polypeptide libraries. Nat Biotechnol. (1997) 15:553–7. doi: 10.1038/nbt0697-553
120. Ho M, Pastan I. Display and selection of scFv antibodies on HEK-293T cells. Methods Mol Biol. (2009) 562:99–113. doi: 10.1007/978-1-60327-302-2_8
121. Ho M, Pastan I. Mammalian cell display for antibody engineering. Methods Mol Biol. (2009) 525:337–52, xiv. doi: 10.1007/978-1-59745-554-1_18
122. Ho M, Nagata S, Pastan I. Isolation of anti-CD22 Fv with high affinity by Fv display on human cells. Proc Natl Acad Sci USA. (2006) 103:9637–42. doi: 10.1073/pnas.0603653103
123. Akamatsu Y, Pakabunto K, Xu Z, Zhang Y, Tsurushita N. Whole IgG surface display on mammalian cells: application to isolation of neutralizing chicken monoclonal anti-IL-12 antibodies. J Immunol Methods. (2007) 327:40–52. doi: 10.1016/j.jim.2007.07.007
124. Zhou C, Jacobsen FW, Cai L, Chen Q, Shen WD. Development of a novel mammalian cell surface antibody display platform. mAbs. (2010) 2:508–18. doi: 10.4161/mabs.2.5.12970
125. Beerli RR, Bauer M, Buser RB, Gwerder M, Muntwiler S, Maurer P, et al. Isolation of human monoclonal antibodies by mammalian cell display. Proc Natl Acad Sci USA. (2008) 105:14336–41. doi: 10.1073/pnas.0805942105
126. Nguyen AW, Le KC, Maynard JA. Identification of high affinity HER2 binding antibodies using CHO Fab surface display. Protein Eng Des Sel. (2018) 31:91–101. doi: 10.1093/protein/gzy004
127. Parthiban K, Perera RL, Sattar M, Huang Y, Mayle S, Masters E, et al. A comprehensive search of functional sequence space using large mammalian display libraries created by gene editing. mAbs. (2019) 11:884–98. doi: 10.1080/19420862.2019.1618673
128. Valadon P, Pérez-Tapia SM, Nelson RS, Guzmán-Bringas OU, Arrieta-Oliva HI, Gómez-Castellano KM, et al. ALTHEA Gold Libraries™: antibody libraries for therapeutic antibody discovery. mAbs. (2019) 11:516–31. doi: 10.1080/19420862.2019.1571879
129. Wolf Pérez A-M, Sormanni P, Andersen JS, Sakhnini LI, Rodriguez-Leon I, Bjelke JR, et al. In vitro and in silico assessment of the developability of a designed monoclonal antibody library. mAbs. (2019) 11:388–400. doi: 10.1080/19420862.2018.1556082
130. Tiller T, Schuster I, Deppe D, Siegers K, Strohner R, Herrmann T, et al. A fully synthetic human Fab antibody library based on fixed VH/VL framework pairings with favorable biophysical properties. mAbs. (2013) 5:445–70. doi: 10.4161/mabs.24218
131. Jarasch A, Koll H, Regula JT, Bader M, Papadimitriou A, Kettenberger H. Developability assessment during the selection of novel therapeutic antibodies. J Pharm Sci. (2015) 104:1885–98. doi: 10.1002/jps.24430
132. Bauer J, Mathias S, Kube S, Otte K, Garidel P, Gamer M, et al. Rational optimization of a monoclonal antibody improves the aggregation propensity and enhances the CMC properties along the entire pharmaceutical process chain. mAbs. (2020) 12:1787121. doi: 10.1080/19420862.2020.1787121
133. Raybould MIJ, Marks C, Krawczyk K, Taddese B, Nowak J, Lewis AP, et al. Five computational developability guidelines for therapeutic antibody profiling. Proc Natl Acad Sci USA. (2019) 116:4025–30. doi: 10.1073/pnas.1810576116
134. Schofield DJ, Pope AR, Clementel V, Buckell J, Chapple S, Clarke KF, et al. Application of phage display to high throughput antibody generation and characterization. Genome Biol. (2007) 8:R254. doi: 10.1186/gb-2007-8-11-r254
135. Chan CEZ, Chan AHY, Lim APC, Hanson BJ. Comparison of the efficiency of antibody selection from semi-synthetic scFv and non-immune Fab phage display libraries against protein targets for rapid development of diagnostic immunoassays. J Immunol Methods. (2011) 373:79–88. doi: 10.1016/j.jim.2011.08.005
136. Gupta A, Shrivastava N, Grover P, Singh A, Mathur K, Verma V, et al. A novel helper phage enabling construction of genome-scale ORF-enriched phage display libraries. PLoS ONE. (2013) 8:e75212. doi: 10.1371/journal.pone.0075212
137. Cárcamo J, Ravera MW, Brissette R, Dedova O, Beasley JR, Alam-Moghé A, et al. Unexpected frameshifts from gene to expressed protein in a phage-displayed peptide library. Proc Natl Acad Sci USA. (1998) 95:11146. doi: 10.1073/pnas.95.19.11146
138. Lin B, Renshaw MW, Autote K, Smith LM, Calveley P, Bowdish KS, et al. A step-wise approach significantly enhances protein yield of a rationally-designed agonist antibody fragment in E. coli. Protein Expr Purif. (2008) 59:55–63. doi: 10.1016/j.pep.2008.01.002
139. Carmen S, Jermutus L. Concepts in antibody phage display. Brief Funct Genomic Proteomic. (2002) 1:189–203. doi: 10.1093/bfgp/1.2.189
140. Kulmala A, Huovinen T, Lamminmäki U. Effect of DNA sequence of Fab fragment on yield characteristics and cell growth of E. coli. Sci Rep. (2017) 7:3796. doi: 10.1038/s41598-017-03957-6
141. Krebber A, Burmester J, Pluckthun A. Inclusion of an upstream transcriptional terminator in phage display vectors abolishes background expression of toxic fusions with coat protein g3p. Gene. (1996) 178:71–4. doi: 10.1016/0378-1119(96)00337-X
142. Blazek D, Celer V. The production and application of single-chain antibody fragments. Folia Microbiol. (2003) 48:687–98. doi: 10.1007/BF02993480
143. Blazek D, Celer V, Navratilova I, Skladal P. Generation and characterization of single-chain antibody fragments specific against transmembrane envelope glycoprotein gp46 of maedi-visna virus. J Virol Methods. (2004) 115:83–92. doi: 10.1016/j.jviromet.2003.09.020
144. Kügler J, Tomszak F, Frenzel A, Hust M. Construction of human immune and naive scFv libraries. In: Hust M, Lim TS, editors. Phage Display: Methods and Protocols. New York, NY: Springer New York (2018). p. 3–24. doi: 10.1007/978-1-4939-7447-4_1
145. Clark MA, Hawkins NJ, Papaioannou A, Fiddes RJ, Ward RL. Isolation of human anti-c-erbB-2 Fabs from a lymph node-derived phage display library. Clin Exp Immunol. (1997) 109:166–74. doi: 10.1046/j.1365-2249.1997.4161316.x
146. Graus YF, Verschuuren JJ, Degenhardt A, van Breda Vriesman PJ, De Baets MH, Posner JB, et al. Selection of recombinant anti-HuD Fab fragments from a phage display antibody library of a lung cancer patient with paraneoplastic encephalomyelitis. J Neuroimmunol. (1998) 82:200–9. doi: 10.1016/S0165-5728(97)00199-9
147. Yip YL, Hawkins NJ, Clark MA, Ward RL. Evaluation of different lymphoid tissue sources for the construction of human immunoglobulin gene libraries. Immunotechnology. (1997) 3:195–203. doi: 10.1016/S1380-2933(97)00013-4
148. Rothe A, Klimka A, Tur MK, Pfitzner T, Huhn M, Sasse S, et al. Construction of phage display libraries from reactive lymph nodes of breast carcinoma patients and selection for specifically binding human single chain Fv on cell lines. Int J Mol Med. (2004) 14:729–35. doi: 10.3892/ijmm.14.4.729
149. Xu MY, Xu XH, Chen GZ, Deng XL, Li J, Yu XJ, et al. Production of a human single-chain variable fragment antibody against esophageal carcinoma. World J Gastroenterol. (2004) 10:2619–23. doi: 10.3748/wjg.v10.i18.2619
150. Bradbury AR, Marks JD. Antibodies from phage antibody libraries. J Immunol Methods. (2004) 290:29–49. doi: 10.1016/j.jim.2004.04.007
151. Nagano K, Imai S, Mukai Y, Nakagawa S, Abe Y, Kamada H, et al. Rapid isolation of intrabody candidates by using an optimized non-immune phage antibody library. Pharmazie. (2009) 64:238–41. doi: 10.1691/ph.2009.8352
152. Kim S, Park I, Park SG, Cho S, Kim JH, Ipper NS, et al. Generation, diversity determination, and application to antibody selection of a human naive Fab library. Mol Cells. (2017) 40:655–66. doi: 10.14348/molcells.2017.0106
153. Kügler J, Wilke S, Meier D, Tomszak F, Frenzel A, Schirrmann T, et al. Generation and analysis of the improved human HAL9/10 antibody phage display libraries. BMC Biotechnol. (2015) 15:10. doi: 10.1186/s12896-015-0125-0
154. Schwimmer LJ, Huang B, Giang H, Cotter RL, Chemla-Vogel DS, Dy FV, et al. Discovery of diverse and functional antibodies from large human repertoire antibody libraries. J Immunol Methods. (2013) 391:60–71. doi: 10.1016/j.jim.2013.02.010
155. Almagro CJ, Pedraza-Escalona M, Arrieta IH, Pérez-Tapia MS. Phage display libraries for antibody therapeutic discovery and development. Antibodies. (2019) 8:44. doi: 10.3390/antib8030044
156. Tsuchiya Y, Mizuguchi K. The diversity of H3 loops determines the antigen-binding tendencies of antibody CDR loops. Protein Sci. (2016) 25:815–25. doi: 10.1002/pro.2874
157. Sela-Culang I, Kunik V, Ofran Y. The structural basis of antibody-antigen recognition. Front Immunol. (2013) 4:302. doi: 10.3389/fimmu.2013.00302
158. Padlan EA, Abergel C, Tipper JP. Identification of specificity-determining residues in antibodies. FASEB J. (1995) 9:133–9. doi: 10.1096/fasebj.9.1.7821752
159. Kunik V, Ofran Y. The indistinguishability of epitopes from protein surface is explained by the distinct binding preferences of each of the six antigen-binding loops. Protein Eng Des Sel. (2013) 26:599–609. doi: 10.1093/protein/gzt027
160. Al-Lazikani B, Lesk AM, Chothia C. Standard conformations for the canonical structures of immunoglobulins. J Mol Biol. (1997) 273:927–48. doi: 10.1006/jmbi.1997.1354
161. Sela-Culang I, Alon S, Ofran Y. A systematic comparison of free and bound antibodies reveals binding-related conformational changes. J Immunol. (2012) 189:4890–9. doi: 10.4049/jimmunol.1201493
162. Chothia C, Lesk AM, Tramontano A, Levitt M, Smith-Gill SJ, Air G, et al. Conformations of immunoglobulin hypervariable regions. Nature. (1989) 342:877–83. doi: 10.1038/342877a0
163. Chothia C, Lesk AM. Canonical structures for the hypervariable regions of immunoglobulins. J Mol Biol. (1987) 196:901–17. doi: 10.1016/0022-2836(87)90412-8
164. Shirai H, Kidera A, Nakamura H. H3-rules: identification of CDR-H3 structures in antibodies. FEBS Lett. (1999) 455:188–97. doi: 10.1016/S0014-5793(99)00821-2
165. Kumar R, Parray HA, Shrivastava T, Sinha S, Luthra K. Phage display antibody libraries: a robust approach for generation of recombinant human monoclonal antibodies. Int J Biol Macromol. (2019) 135:907–18. doi: 10.1016/j.ijbiomac.2019.06.006
166. Xu JL, Davis MM. Diversity in the CDR3 region of V(H) is sufficient for most antibody specificities. Immunity. (2000) 13:37–45. doi: 10.1016/S1074-7613(00)00006-6
167. Solemani Zadeh A, Grässer A, Dinter H, Hermes M, Schindowski K. Efficient construction and effective screening of synthetic domain antibody libraries. Methods Protoc. (2019) 2:17. doi: 10.3390/mps2010017
168. Pini A, Viti F, Santucci A, Carnemolla B, Zardi L, Neri P, et al. Design and use of a phage display library. Human antibodies with subnanomolar affinity against a marker of angiogenesis eluted from a two-dimensional gel. J Biol Chem. (1998) 273:21769–76. doi: 10.1074/jbc.273.34.21769
169. Barbas CF III, Bain JD, Hoekstra DM, Lerner RA. Semisynthetic combinatorial antibody libraries: a chemical solution to the diversity problem. Proc Natl Acad Sci USA. (1992) 89:4457–61. doi: 10.1073/pnas.89.10.4457
170. Rauchenberger R, Borges E, Thomassen-Wolf E, Rom E, Adar R, Yaniv Y, et al. Human combinatorial Fab library yielding specific and functional antibodies against the human fibroblast growth factor receptor 3. J Biol Chem. (2003) 278:38194–205. doi: 10.1074/jbc.M303164200
171. Nixon AE, Sexton DJ, Ladner RC. Drugs derived from phage display: from candidate identification to clinical practice. mAbs. (2014) 6:73–85. doi: 10.4161/mabs.27240
172. Frenzel A, Schirrmann T, Hust M. Phage display-derived human antibodies in clinical development and therapy. mAbs. (2016) 8:1177–94. doi: 10.1080/19420862.2016.1212149
173. Vaughan TJ, Williams AJ, Pritchard K, Osbourn JK, Pope AR, Earnshaw JC, et al. Human antibodies with sub-nanomolar affinities isolated from a large non-immunized phage display library. Nat Biotechnol. (1996) 14:309–14. doi: 10.1038/nbt0396-309
174. Lloyd C, Lowe D, Edwards B, Welsh F, Dilks T, Hardman C, et al. Modelling the human immune response: performance of a 1011 human antibody repertoire against a broad panel of therapeutically relevant antigens. Protein Eng Des Sel. (2009) 22:159–68. doi: 10.1093/protein/gzn058
175. Groves M, Lane S, Douthwaite J, Lowne D, Gareth Rees D, Edwards B, et al. Affinity maturation of phage display antibody populations using ribosome display. J Immunol Methods. (2006) 313:129–39. doi: 10.1016/j.jim.2006.04.002
176. de Haard HJ, van Neer N, Reurs A, Hufton SE, Roovers RC, Henderikx P, et al. A large non-immunized human Fab fragment phage library that permits rapid isolation and kinetic analysis of high affinity antibodies. J Biol Chem. (1999) 274:18218–30. doi: 10.1074/jbc.274.26.18218
177. Knappik A, Ge L, Honegger A, Pack P, Fischer M, Wellnhofer G, et al. Fully synthetic human combinatorial antibody libraries (HuCAL) based on modular consensus frameworks and CDRs randomized with trinucleotides. J Mol Biol. (2000) 296:57–86. doi: 10.1006/jmbi.1999.3444
178. Rothe C, Urlinger S, Lohning C, Prassler J, Stark Y, Jager U, et al. The human combinatorial antibody library HuCAL GOLD combines diversification of all six CDRs according to the natural immune system with a novel display method for efficient selection of high-affinity antibodies. J Mol Biol. (2008) 376:1182–200. doi: 10.1016/j.jmb.2007.12.018
179. Prassler J, Thiel S, Pracht C, Polzer A, Peters S, Bauer M, et al. HuCAL PLATINUM, a synthetic Fab library optimized for sequence diversity and superior performance in mammalian expression systems. J Mol Biol. (2011) 413:261–78. doi: 10.1016/j.jmb.2011.08.012
180. Jespers LS, Roberts A, Mahler SM, Winter G, Hoogenboom HR. Guiding the selection of human antibodies from phage display repertoires to a single epitope of an antigen. Biotechnology. (1994) 12:899–903. doi: 10.1038/nbt0994-899
181. Burmester GR, Panaccione R, Gordon KB, McIlraith MJ, Lacerda AP. Adalimumab: long-term safety in 23,458 patients from global clinical trials in rheumatoid arthritis, juvenile idiopathic arthritis, ankylosing spondylitis, psoriatic arthritis, psoriasis and Crohn's disease. Ann Rheum Dis. (2013) 72:517–24. doi: 10.1136/annrheumdis-2011-201244
182. Lapadula G, Marchesoni A, Armuzzi A, Blandizzi C, Caporali R, Chimenti S, et al. Adalimumab in the treatment of immune-mediated diseases. Int J Immunopathol Pharmacol. (2014) 27:33–48. doi: 10.1177/03946320140270S103
183. Jemec GBE, Okun MM, Forman SB, Gulliver WPF, Prens EP, Mrowietz U, et al. Adalimumab medium-term dosing strategy in moderate-to-severe hidradenitis suppurativa: integrated results from the phase III randomized placebo-controlled PIONEER trials. Br J Dermatol. (2019) 181:697–75. doi: 10.1111/bjd.17919
184. Schadler ED, Ortel B, Mehlis SL. Biologics for the primary care physician: review and treatment of psoriasis. Dis Mon. (2019) 65:51–90. doi: 10.1016/j.disamonth.2018.06.001
185. Bitossi A, Bettiol A, Silvestri E, Di Scala G, Bacherini D, Lopalco G, et al. Adalimumab accounts for long-term control of noninfectious uveitis also in the absence of concomitant DMARD treatment: a multicenter retrospective study. Mediators Inflamm. (2019) 2019:1623847. doi: 10.1155/2019/1623847
186. Raum T, Gruber R, Riethmuller G, Kufer P. Anti-self antibodies selected from a human IgD heavy chain repertoire: a novel approach to generate therapeutic human antibodies against tumor-associated differentiation antigens. Cancer Immunol Immunother. (2001) 50:141–50. doi: 10.1007/PL00006684
187. Prang N, Preithner S, Brischwein K, Goster P, Woppel A, Muller J, et al. Cellular and complement-dependent cytotoxicity of Ep-CAM-specific monoclonal antibody MT201 against breast cancer cell lines. Br J Cancer. (2005) 92:342–9. doi: 10.1038/sj.bjc.6602310
188. Schmidt M, Ruttinger D, Sebastian M, Hanusch CA, Marschner N, Baeuerle PA, et al. Phase IB study of the EpCAM antibody adecatumumab combined with docetaxel in patients with EpCAM-positive relapsed or refractory advanced-stage breast cancer. Ann Oncol. (2012) 23:2306–13. doi: 10.1093/annonc/mdr625
189. Schmidt M, Scheulen ME, Dittrich C, Obrist P, Marschner N, Dirix L, et al. An open-label, randomized phase II study of adecatumumab, a fully human anti-EpCAM antibody, as monotherapy in patients with metastatic breast cancer. Ann Oncol. (2010) 21:275–82. doi: 10.1093/annonc/mdp314
190. Marschner N, Ruttinger D, Zugmaier G, Nemere G, Lehmann J, Obrist P, et al. Phase II study of the human anti-epithelial cell adhesion molecule antibody adecatumumab in prostate cancer patients with increasing serum levels of prostate-specific antigen after radical prostatectomy. Urol Int. (2010) 85:386–95. doi: 10.1159/000318055
191. Nagy ZA, Hubner B, Lohning C, Rauchenberger R, Reiffert S, Thomassen-Wolf E, et al. Fully human, HLA-DR-specific monoclonal antibodies efficiently induce programmed death of malignant lymphoid cells. Nat Med. (2002) 8:801–7. doi: 10.1038/nm736
192. Carlo-Stella C, Di Nicola M, Turco MC, Cleris L, Lavazza C, Longoni P, et al. The anti-human leukocyte antigen-DR monoclonal antibody 1D09C3 activates the mitochondrial cell death pathway and exerts a potent antitumor activity in lymphoma-bearing nonobese diabetic/severe combined immunodeficient mice. Cancer Res. (2006) 66:1799–808. doi: 10.1158/0008-5472.CAN-05-1200
193. Carlo-Stella C, Guidetti A, Di Nicola M, Lavazza C, Cleris L, Sia D, et al. IFN-gamma enhances the antimyeloma activity of the fully human anti-human leukocyte antigen-DR monoclonal antibody 1D09C3. Cancer Res. (2007) 67:3269–75. doi: 10.1158/0008-5472.CAN-06-3744
194. Golfier S, Kopitz C, Kahnert A, Heisler I, Schatz CA, Stelte-Ludwig B, et al. Anetumab ravtansine: a novel mesothelin-targeting antibody-drug conjugate cures tumors with heterogeneous target expression favored by bystander effect. Mol Cancer Ther. (2014) 13:1537–48. doi: 10.1158/1535-7163.MCT-13-0926
195. Quanz M, Hagemann UB, Zitzmann-Kolbe S, Stelte-Ludwig B, Golfier S, Elbi C, et al. Anetumab ravtansine inhibits tumor growth and shows additive effect in combination with targeted agents and chemotherapy in mesothelin-expressing human ovarian cancer models. Oncotarget. (2018) 9:34103–21. doi: 10.18632/oncotarget.26135
196. Wolska-Washer A, Robak T. Safety and tolerability of antibody-drug conjugates in cancer. Drug Saf. (2019) 42:295–314. doi: 10.1007/s40264-018-0775-7
197. Chowdhury PS, Viner JL, Beers R, Pastan I. Isolation of a high-affinity stable single-chain Fv specific for mesothelin from DNA-immunized mice by phage display and construction of a recombinant immunotoxin with anti-tumor activity. Proc Natl Acad Sci USA. (1998) 95:669–74. doi: 10.1073/pnas.95.2.669
198. Hassan R, Ebel W, L Routhier E, Patel R, Kline J, Zhang J, et al. Preclinical evaluation of MORAb-009, a chimeric antibody targeting tumor-associated mesothelin. Cancer Immun. (2007) 7:20.
199. Hassan R, Kindler HL, Jahan T, Bazhenova L, Reck M, Thomas A, et al. Phase II clinical trial of amatuximab, a chimeric antimesothelin antibody with pemetrexed and cisplatin in advanced unresectable pleural mesothelioma. Clin Cancer Res. (2014) 20:5927–36. doi: 10.1158/1078-0432.CCR-14-0804
200. Baldo P, Cecco S. Amatuximab and novel agents targeting mesothelin for solid tumors. Onco Targets Ther. (2017) 10:5337–53. doi: 10.2147/OTT.S145105
201. Herbst RS, Soria JC, Kowanetz M, Fine GD, Hamid O, Gordon MS, et al. Predictive correlates of response to the anti-PD-L1 antibody MPDL3280A in cancer patients. Nature. (2014) 515:563–7. doi: 10.1038/nature14011
202. Irving B, Chiu H, Maecker H, Mariathasan S, Lehar Sophie M, Wu YAN, et al. Anti-pd-l1 Antibodies, Compositions and Articles of Manufacture. US patent US 8217149 B2. U.S. Patent and Trademark Office (2012).
203. Shah NJ, Kelly WJ, Liu SV, Choquette K, Spira A. Product review on the Anti-PD-L1 antibody atezolizumab. Hum Vaccin Immunother. (2018) 14:269–76. doi: 10.1080/21645515.2017.1403694
204. Gray SG, Mutti L. Immunotherapy for mesothelioma: a critical review of current clinical trials and future perspectives. Transl Lung Cancer Res. (2020) 9:S100–19. doi: 10.21037/tlcr.2019.11.23
205. Inman BA, Longo TA, Ramalingam S, Harrison MR. Atezolizumab: a PD-L1-blocking antibody for bladder cancer. Clin Cancer Res. (2017) 23:1886–90. doi: 10.1158/1078-0432.CCR-16-1417
206. Santini FC, Rudin CM. Atezolizumab for the treatment of non-small cell lung cancer. Expert Rev Clin Pharmacol. (2017) 10:935–45. doi: 10.1080/17512433.2017.1356717
207. Rexer H, Doehn C. First-line treatment for advanced renal cell carcinoma: a phase 3, open-label, randomized study of atezolizumab (anti-PD-L1-Antibody) in combination with bevacizumab versus sunitinib in patients with untreated advanced renal cell carcinoma (“IMmotion”)–AN 37/15 der AUO. Urologe A. (2016) 55:1242–3. doi: 10.1007/s00120-016-0188-0
208. Reddy SM, Carroll E, Nanda R. Atezolizumab for the treatment of breast cancer. Expert Rev Anticancer Ther. (2020) 20:151–8. doi: 10.1080/14737140.2020.1732211
209. Shim H. Therapeutic antibodies by phage display. Curr Pharm Des. (2016) 22:6538–59. doi: 10.2174/1381612822666160923113714
210. Pujade-Lauraine E, Fujiwara K, Dychter SS, Devgan G, Monk BJ. Avelumab (anti-PD-L1) in platinum-resistant/refractory ovarian cancer: JAVELIN Ovarian 200 Phase III study design. Future Oncol. (2018) 14:2103–13. doi: 10.2217/fon-2018-0070
211. Bang YJ, Ruiz EY, Van Cutsem E, Lee KW, Wyrwicz L, Schenker M, et al. Phase III, randomised trial of avelumab versus physician's choice of chemotherapy as third-line treatment of patients with advanced gastric or gastro-oesophageal junction cancer: primary analysis of JAVELIN Gastric 300. Ann Oncol. (2018) 29:2052–60. doi: 10.1093/annonc/mdy264
212. Barlesi F, Vansteenkiste J, Spigel D, Ishii H, Garassino M, de Marinis F, et al. Avelumab versus docetaxel in patients with platinum-treated advanced non-small-cell lung cancer (JAVELIN Lung 200): an open-label, randomised, phase 3 study. Lancet Oncol. (2018) 19:1468–79. doi: 10.1016/S1470-2045(18)30673-9
213. Le Tourneau C, Hoimes C, Zarwan C, Wong DJ, Bauer S, Claus R, et al. Avelumab in patients with previously treated metastatic adrenocortical carcinoma: phase 1b results from the JAVELIN solid tumor trial. J Immunother Cancer. (2018) 6:111. doi: 10.1186/s40425-018-0424-9
214. Shirley M. Avelumab: a review in metastatic merkel cell carcinoma. Target Oncol. (2018) 13:409–16. doi: 10.1007/s11523-018-0571-4
215. Motzer RJ, Penkov K, Haanen J, Rini B, Albiges L, Campbell MT, et al. Avelumab plus axitinib versus sunitinib for advanced renal-cell carcinoma. N Engl J Med. (2019) 380:1103–15. doi: 10.1056/NEJMoa1816047
216. Rao A, Patel MR. A review of avelumab in locally advanced and metastatic bladder cancer. Ther Adv Urol. (2019) 11:1756287218823485. doi: 10.1177/1756287218823485
217. Baker KP, Edwards BM, Main SH, Choi GH, Wager RE, Halpern WG, et al. Generation and characterization of LymphoStat-B, a human monoclonal antibody that antagonizes the bioactivities of B lymphocyte stimulator. Arthritis Rheum. (2003) 48:3253–65. doi: 10.1002/art.11299
218. Stohl W, Hilbert DM. The discovery and development of belimumab: the anti-BLyS-lupus connection. Nat Biotechnol. (2012) 30:69–77. doi: 10.1038/nbt.2076
219. Benitez A, Torralba K, Ngo M, Salto LM, Choi KS, De Vera ME, et al. Belimumab alters transitional B-cell subset proportions in patients with stable systemic lupus erythematosus. Lupus. (2019) 28:1337–43. doi: 10.1177/0961203319869468
220. Jayne D, Blockmans D, Luqmani R, Moiseev S, Ji B, Green Y, et al. Efficacy and safety of belimumab and azathioprine for maintenance of remission in antineutrophil cytoplasmic antibody-associated vasculitis: a randomized controlled study. Arthritis Rheumatol. (2019) 71:952–63. doi: 10.1002/art.40802
221. Main S, Handy R, Wilton J, Smith S, Williams L, Fou LD, et al. A potent human anti-eotaxin1 antibody, CAT-213: isolation by phage display and in vitro and in vivo efficacy. J Pharmacol Exp Ther. (2006) 319:1395–404. doi: 10.1124/jpet.106.110734
222. Smith KA, Pulsipher A, Gabrielsen DA, Alt JA. Biologics in chronic rhinosinusitis: an update and thoughts for future directions. Am J Rhinol Allergy. (2018) 32:412–23. doi: 10.1177/1945892418787132
223. Amiot A, Peyrin-Biroulet L. Current, new and future biological agents on the horizon for the treatment of inflammatory bowel diseases. Therap Adv Gastroenterol. (2015) 8:66–82. doi: 10.1177/1756283X14558193
224. Izumi K, Bieber K, Ludwig RJ. Current clinical trials in pemphigus and pemphigoid. Front Immunol. (2019) 10:978. doi: 10.3389/fimmu.2019.00978
225. van der Star BJ, van Dijk CE, Zock JP, Smit LAM, Baliatsas C, Heederik DJJ, et al. Healthcare utilisation prior to the diagnosis of inflammatory bowel diseases and the influence of livestock exposure: a longitudinal case-control study. PLoS ONE. (2018) 13:e0195305. doi: 10.1371/journal.pone.0195305
226. Lach-Trifilieff E, Minetti GC, Sheppard K, Ibebunjo C, Feige JN, Hartmann S, et al. An antibody blocking activin type II receptors induces strong skeletal muscle hypertrophy and protects from atrophy. Mol Cell Biol. (2014) 34:606–18. doi: 10.1128/MCB.01307-13
227. Garito T, Roubenoff R, Hompesch M, Morrow L, Gomez K, Rooks D, et al. Bimagrumab improves body composition and insulin sensitivity in insulin-resistant individuals. Diabetes Obes Metab. (2018) 20:94–102. doi: 10.1111/dom.13042
228. Hanna MG, Badrising UA, Benveniste O, Lloyd TE, Needham M, Chinoy H, et al. Safety and efficacy of intravenous bimagrumab in inclusion body myositis (RESILIENT): a randomised, double-blind, placebo-controlled phase 2b trial. Lancet Neurol. (2019) 18:834–44. doi: 10.1016/S1474-4422(19)30200-5
229. Rooks D, Praestgaard J, Hariry S, Laurent D, Petricoul O, Perry RG, et al. Treatment of sarcopenia with bimagrumab: results from a phase II, randomized, controlled, proof-of-concept study. J Am Geriatr Soc. (2017) 65:1988–95. doi: 10.1111/jgs.14927
230. Rooks DS, Laurent D, Praestgaard J, Rasmussen S, Bartlett M, Tanko LB. Effect of bimagrumab on thigh muscle volume and composition in men with casting-induced atrophy. J Cachexia Sarcopenia Muscle. (2017) 8:727–34. doi: 10.1002/jcsm.12205
231. Molfino A, Amabile MI, Rossi Fanelli F, Muscaritoli M. Novel therapeutic options for cachexia and sarcopenia. Expert Opin Biol Ther. (2016) 16:1239–44. doi: 10.1080/14712598.2016.1208168
232. Carton JM, Sauerwald T, Hawley-Nelson P, Morse B, Peffer N, Beck H, et al. Codon engineering for improved antibody expression in mammalian cells. Protein Expr Purif. (2007) 55:279–86. doi: 10.1016/j.pep.2007.05.017
233. Brana I, Calles A, LoRusso PM, Yee LK, Puchalski TA, Seetharam S, et al. Carlumab, an anti-C-C chemokine ligand 2 monoclonal antibody, in combination with four chemotherapy regimens for the treatment of patients with solid tumors: an open-label, multicenter phase 1b study. Target Oncol. (2015) 10:111–23. doi: 10.1007/s11523-014-0320-2
234. Sandhu SK, Papadopoulos K, Fong PC, Patnaik A, Messiou C, Olmos D, et al. A first-in-human, first-in-class, phase I study of carlumab (CNTO 888), a human monoclonal antibody against CC-chemokine ligand 2 in patients with solid tumors. Cancer Chemother Pharmacol. (2013) 71:1041–50. doi: 10.1007/s00280-013-2099-8
235. Pienta KJ, Machiels JP, Schrijvers D, Alekseev B, Shkolnik M, Crabb SJ, et al. Phase 2 study of carlumab (CNTO 888), a human monoclonal antibody against CC-chemokine ligand 2 (CCL2), in metastatic castration-resistant prostate cancer. Invest New Drugs. (2013) 31:760–8. doi: 10.1007/s10637-012-9869-8
236. Raghu G, Martinez FJ, Brown KK, Costabel U, Cottin V, Wells AU, et al. CC-chemokine ligand 2 inhibition in idiopathic pulmonary fibrosis: a phase 2 trial of carlumab. Eur Respir J. (2015) 46:1740–50. doi: 10.1183/13993003.01558-2014
237. Rowinsky EK, Youssoufian H, Tonra JR, Solomon P, Burtrum D, Ludwig DL. IMC-A12, a human IgG1 monoclonal antibody to the insulin-like growth factor I receptor. Clin Cancer Res. (2007) 13:5549s–55s. doi: 10.1158/1078-0432.CCR-07-1109
238. Argiris A, Lee JW, Stevenson J, Sulecki MG, Hugec V, Choong NW, et al. Phase II randomized trial of carboplatin, paclitaxel, bevacizumab with or without cixutumumab (IMC-A12) in patients with advanced non-squamous, non-small-cell lung cancer: a trial of the ECOG-ACRIN Cancer Research Group (E3508). Ann Oncol. (2017) 28:3037–43. doi: 10.1093/annonc/mdx534
239. Abou-Alfa GK, Capanu M, O'Reilly EM, Ma J, Chou JF, Gansukh B, et al. A phase II study of cixutumumab (IMC-A12, NSC742460) in advanced hepatocellular carcinoma. J Hepatol. (2014) 60:319–24. doi: 10.1016/j.jhep.2013.09.008
240. Weigel B, Malempati S, Reid JM, Voss SD, Cho SY, Chen HX, et al. Phase 2 trial of cixutumumab in children, adolescents, and young adults with refractory solid tumors: a report from the Children's Oncology Group. Pediatr Blood Cancer. (2014) 61:452–6. doi: 10.1002/pbc.24605
241. Bakker AB, Marissen WE, Kramer RA, Rice AB, Weldon WC, Niezgoda M, et al. Novel human monoclonal antibody combination effectively neutralizing natural rabies virus variants and individual in vitro escape mutants. J Virol. (2005) 79:9062–8. doi: 10.1128/JVI.79.14.9062-9068.2005
242. Kramer RA, Marissen WE, Goudsmit J, Visser TJ, Clijsters-Van der Horst M, Bakker AQ, et al. The human antibody repertoire specific for rabies virus glycoprotein as selected from immune libraries. Eur J Immunol. (2005) 35:2131–45. doi: 10.1002/eji.200526134
243. Bedinger D, Lao L, Khan S, Lee S, Takeuchi T, Mirza AM. Development and characterization of human monoclonal antibodies that neutralize multiple TGFbeta isoforms. mAbs. (2016) 8:389–404. doi: 10.1080/19420862.2015.1115166
244. de Gramont A, Faivre S, Raymond E. Novel TGF-beta inhibitors ready for prime time in onco-immunology. Oncoimmunology. (2017) 6:e1257453. doi: 10.1080/2162402X.2016.1257453
245. Vincenti F, Fervenza FC, Campbell KN, Diaz M, Gesualdo L, Nelson P, et al. A phase 2, double-blind, placebo-controlled, randomized study of fresolimumab in patients with steroid-resistant primary focal segmental glomerulosclerosis. Kidney Int Rep. (2017) 2:800–10. doi: 10.1016/j.ekir.2017.03.011
246. Rice LM, Padilla CM, McLaughlin SR, Mathes A, Ziemek J, Goummih S, et al. Fresolimumab treatment decreases biomarkers and improves clinical symptoms in systemic sclerosis patients. J Clin Invest. (2015) 125:2795–807. doi: 10.1172/JCI77958
247. Formenti SC, Lee P, Adams S, Goldberg JD, Li X, Xie MW, et al. Focal irradiation and systemic TGFbeta blockade in metastatic breast cancer. Clin Cancer Res. (2018) 24:2493–504. doi: 10.1158/1078-0432.CCR-17-3322
248. Liu L, Lu J, Allan BW, Tang Y, Tetreault J, Chow CK, et al. Generation and characterization of ixekizumab, a humanized monoclonal antibody that neutralizes interleukin-17A. J Inflamm Res. (2016) 9:39–50. doi: 10.2147/JIR.S100940
249. Mease P, Roussou E, Burmester GR, Goupille P, Gottlieb A, Moriarty SR, et al. Safety of ixekizumab in patients with psoriatic arthritis: results from a pooled analysis of three clinical trials. Arthritis Care Res (Hoboken). (2019) 71:367–78. doi: 10.1002/acr.23738
250. Genovese MC, Greenwald M, Cho CS, Berman A, Jin L, Cameron GS, et al. A phase II randomized study of subcutaneous ixekizumab, an anti-interleukin-17 monoclonal antibody, in rheumatoid arthritis patients who were naive to biologic agents or had an inadequate response to tumor necrosis factor inhibitors. Arthritis Rheumatol. (2014) 66:1693–704. doi: 10.1002/art.38617
251. Deodhar A, Poddubnyy D, Pacheco-Tena C, Salvarani C, Lespessailles E, Rahman P, et al. Efficacy and safety of ixekizumab in the treatment of radiographic axial spondyloarthritis: sixteen-week results from a phase III randomized, double-blind, placebo-controlled trial in patients with prior inadequate response to or intolerance of tumor necrosis factor inhibitors. Arthritis Rheumatol. (2019) 71:599–611. doi: 10.1002/art.40753
252. Okubo Y, Mabuchi T, Iwatsuki K, Elmaraghy H, Torisu-Itakura H, Morisaki Y, et al. Long-term efficacy and safety of ixekizumab in Japanese patients with erythrodermic or generalized pustular psoriasis: subgroup analyses of an open-label, phase 3 study (UNCOVER-J). J Eur Acad Dermatol Venereol. (2019) 33:325–32. doi: 10.1111/jdv.15287
253. Pukac L, Kanakaraj P, Humphreys R, Alderson R, Bloom M, Sung C, et al. HGS-ETR1, a fully human TRAIL-receptor 1 monoclonal antibody, induces cell death in multiple tumour types in vitro and in vivo. Br J Cancer. (2005) 92:1430–41. doi: 10.1038/sj.bjc.6602487
254. Belch A, Sharma A, Spencer A, Tarantolo S, Bahlis NJ, Doval D, et al. A multicenter randomized phase II trial of mapatumumab, a TRAIL-R1 agonist monoclonal antibody, in combination with bortezomib in patients with relapsed/refractory multiple myeloma (MM). Blood. (2010) 116:5031. doi: 10.1182/blood.V116.21.5031.5031
255. Trarbach T, Moehler M, Heinemann V, Kohne CH, Przyborek M, Schulz C, et al. Phase II trial of mapatumumab, a fully human agonistic monoclonal antibody that targets and activates the tumour necrosis factor apoptosis-inducing ligand receptor-1 (TRAIL-R1), in patients with refractory colorectal cancer. Br J Cancer. (2010) 102:506–12. doi: 10.1038/sj.bjc.6605507
256. von Pawel J, Harvey JH, Spigel DR, Dediu M, Reck M, Cebotaru CL, et al. Phase II trial of mapatumumab, a fully human agonist monoclonal antibody to tumor necrosis factor-related apoptosis-inducing ligand receptor 1 (TRAIL-R1), in combination with paclitaxel and carboplatin in patients with advanced non-small-cell lung cancer. Clin Lung Cancer. (2014) 15:188–96 e2. doi: 10.1016/j.cllc.2013.12.005
257. Younes A, Vose JM, Zelenetz AD, Smith MR, Burris HA, Ansell SM, et al. A phase 1b/2 trial of mapatumumab in patients with relapsed/refractory non-Hodgkin's lymphoma. Br J Cancer. (2010) 103:1783–7. doi: 10.1038/sj.bjc.6605987
258. Burmester GR, Feist E, Sleeman MA, Wang B, White B, Magrini F. Mavrilimumab, a human monoclonal antibody targeting GM-CSF receptor-alpha, in subjects with rheumatoid arthritis: a randomised, double-blind, placebo-controlled, phase I, first-in-human study. Ann Rheum Dis. (2011) 70:1542–9. doi: 10.1136/ard.2010.146225
259. Weinblatt ME, McInnes IB, Kremer JM, Miranda P, Vencovsky J, Guo X, et al. A randomized phase IIb study of mavrilimumab and golimumab in rheumatoid arthritis. Arthritis Rheumatol. (2018) 70:49–59. doi: 10.1002/art.40323
260. Crotti C, Agape E, Becciolini A, Biggioggero M, Favalli EG. Targeting granulocyte-monocyte colony-stimulating factor signaling in rheumatoid arthritis: future prospects. Drugs. (2019) 79:1741–55. doi: 10.1007/s40265-019-01192-z
261. Gosain R, Abdou Y, Singh A, Rana N, Puzanov I, Ernstoff MS. COVID-19 and cancer: a comprehensive review. Curr Oncol Rep. (2020) 22:53. doi: 10.1007/s11912-020-00934-7
262. Kreitman RJ, Pastan I. Antibody fusion proteins: anti-CD22 recombinant immunotoxin moxetumomab pasudotox. Clin Cancer Res. (2011) 17:6398–405. doi: 10.1158/1078-0432.CCR-11-0487
263. Nobre CF, Newman MJ, DeLisa A, Newman P. Moxetumomab pasudotox-tdfk for relapsed/refractory hairy cell leukemia: a review of clinical considerations. Cancer Chemother Pharmacol. (2019) 84:255–63. doi: 10.1007/s00280-019-03875-6
264. Kreitman RJ, Dearden C, Zinzani PL, Delgado J, Karlin L, Robak T, et al. Moxetumomab pasudotox in relapsed/refractory hairy cell leukemia. Leukemia. (2018) 32:1768–77. doi: 10.1038/s41375-018-0210-1
265. Krinner EM, Hepp J, Hoffmann P, Bruckmaier S, Petersen L, Petsch S, et al. A highly stable polyethylene glycol-conjugated human single-chain antibody neutralizing granulocyte-macrophage colony stimulating factor at low nanomolar concentration. Protein Eng Des Sel. (2006) 19:461–70. doi: 10.1093/protein/gzl031
266. Krinner EM, Raum T, Petsch S, Bruckmaier S, Schuster I, Petersen L, et al. A human monoclonal IgG1 potently neutralizing the pro-inflammatory cytokine GM-CSF. Mol Immunol. (2007) 44:916–25. doi: 10.1016/j.molimm.2006.03.020
267. Taylor PC, Saurigny D, Vencovsky J, Takeuchi T, Nakamura T, Matsievskaia G, et al. Efficacy and safety of namilumab, a human monoclonal antibody against granulocyte-macrophage colony-stimulating factor (GM-CSF) ligand in patients with rheumatoid arthritis (RA) with either an inadequate response to background methotrexate therapy or an inadequate response or intolerance to an anti-TNF (tumour necrosis factor) biologic therapy: a randomized, controlled trial. Arthritis Res Ther. (2019) 21:101. doi: 10.1186/s13075-019-1879-x
268. Worth C, Bowness P, Hussein Al-Mossawi M. Novel therapeutic targets in axial spondyloarthritis. Curr Treatm Opt Rheumatol. (2018) 4:174–82. doi: 10.1007/s40674-018-0095-1
269. Papp KA, Gooderham M, Jenkins R, Vender R, Szepietowski JC, Wagner T, et al. Granulocyte-macrophage colony-stimulating factor (GM-CSF) as a therapeutic target in psoriasis: randomized, controlled investigation using namilumab, a specific human anti-GM-CSF monoclonal antibody. Br J Dermatol. (2019) 180:1352–60. doi: 10.1111/bjd.17195
270. Li S, Kussie P, Ferguson KM. Structural basis for EGF receptor inhibition by the therapeutic antibody IMC-11F8. Structure. (2008) 16:216–27. doi: 10.1016/j.str.2007.11.009
271. Liu M, Zhang H, Jimenez X, Ludwig D, Witte L, Bohlen P, et al. Identification and characterization of a fully human antibody directed against epidermal growth factor receptor for cancer therapy. Cancer Research. (2004) 64:163.
272. Thatcher N, Hirsch FR, Szczesna A, Ciuleanu T-E, Szafranski W, Dediu M, et al. A randomized, multicenter, open-label, phase III study of gemcitabine-cisplatin (GC) chemotherapy plus necitumumab (IMC-11F8/LY3012211) versus GC alone in the first-line treatment of patients (pts) with stage IV squamous non-small cell lung cancer (sq-NSCLC). J Clin Oncol. (2014) 32:8008. doi: 10.1200/jco.2014.32.15_suppl.8008
273. Diaz-Serrano A, Sanchez-Torre A, Paz-Ares L. Necitumumab for the treatment of advanced non-small-cell lung cancer. Future Oncol. (2019) 15:705–16. doi: 10.2217/fon-2018-0594
274. Elez E, Hendlisz A, Delaunoit T, Sastre J, Cervantes A, Varea R, et al. Phase II study of necitumumab plus modified FOLFOX6 as first-line treatment in patients with locally advanced or metastatic colorectal cancer. Br J Cancer. (2016) 114:372–80. doi: 10.1038/bjc.2015.480
275. Kuenen B, Witteveen PO, Ruijter R, Giaccone G, Dontabhaktuni A, Fox F, et al. A phase I pharmacologic study of necitumumab (IMC-11F8), a fully human IgG1 monoclonal antibody directed against EGFR in patients with advanced solid malignancies. Clin Cancer Res. (2010) 16:1915–23. doi: 10.1158/1078-0432.CCR-09-2425
276. Pepinsky RB, Shao Z, Ji B, Wang Q, Meng G, Walus L, et al. Exposure levels of anti-LINGO-1 Li81 antibody in the central nervous system and dose-efficacy relationships in rat spinal cord remyelination models after systemic administration. J Pharmacol Exp Ther. (2011) 339:519–29. doi: 10.1124/jpet.111.183483
277. Cadavid D, Mellion M, Hupperts R, Edwards KR, Calabresi PA, Drulovic J, et al. Safety and efficacy of opicinumab in patients with relapsing multiple sclerosis (SYNERGY): a randomised, placebo-controlled, phase 2 trial. Lancet Neurol. (2019) 18:845–56. doi: 10.1016/S1474-4422(19)30137-1
278. Cadavid D, Balcer L, Galetta S, Aktas O, Ziemssen T, Vanopdenbosch L, et al. Safety and efficacy of opicinumab in acute optic neuritis (RENEW): a randomised, placebo-controlled, phase 2 trial. Lancet Neurol. (2017) 16:189–99. doi: 10.1016/S1474-4422(16)30377-5
279. Lee WS, Pyun B-J, Kim S-W, Shim SR, Nam JR, Yoo JY, et al. TTAC-0001, a human monoclonal antibody targeting VEGFR-2/KDR, blocks tumor angiogenesis. mAbs. (2015) 7:957–68. doi: 10.1080/19420862.2015.1045168
280. Burmester J, Plückthun A. Construction of scFv fragments from hybridoma or spleen cells by PCR assembly. In: Kontermann R, Dübel S, editors. Antibody Engineering. Berlin; Heidelberg: Springer Berlin Heidelberg (2001). p. 19–40. doi: 10.1007/978-3-662-04605-0_2
281. Lee SJ, Lee SY, Lee WS, Yoo JS, Sun J-M, Lee J, et al. Phase I trial and pharmacokinetic study of tanibirumab, a fully human monoclonal antibody to vascular endothelial growth factor receptor 2, in patients with refractory solid tumors. Invest New Drugs. (2017) 35:782–90. doi: 10.1007/s10637-017-0463-y
282. Cher L, Nowak AK, Iatropoulos G, Lee WS, Lee SY, Shim SR, et al. A multicenter, 3-arm, open-label, phase IIa clinical trial to evaluate the safety and efficacy of tanibirumab (VEGFR2 mAb), in patients with recurrent glioblastoma (GBM). J Clin Oncol. (2017) 35:e13522. doi: 10.1200/JCO.2017.35.15_suppl.e13522
283. Fisher TS, Kamperschroer C, Oliphant T, Love VA, Lira PD, Doyonnas R, et al. Targeting of 4–1BB by monoclonal antibody PF-05082566 enhances T-cell function and promotes anti-tumor activity. Cancer Immunol Immunother. (2012) 61:1721–33. doi: 10.1007/s00262-012-1237-1
284. Tolcher AW, Sznol M, Hu-Lieskovan S, Papadopoulos KP, Patnaik A, Rasco DW, et al. Phase Ib study of utomilumab (PF-05082566), a 4–1BB/CD137 agonist, in combination with pembrolizumab (MK-3475) in patients with advanced solid tumors. Clin Cancer Res. (2017) 23:5349–57. doi: 10.1158/1078-0432.CCR-17-1243
285. Pegram MD, Zong Y, Yam C, Goetz MP, Moulder SL. Innovative Strategies: Targeting Subtypes in Metastatic Breast Cancer. Am Soc Clin Oncol Educ Book. (2018) 38:65–77. doi: 10.1200/EDBK_200715
286. Zhang J, Medeiros LJ, Young KH. Cancer immunotherapy in diffuse large B-cell lymphoma. Front Oncol. (2018) 8:351. doi: 10.3389/fonc.2018.00351
287. Chavez JC, Bachmeier C, Kharfan-Dabaja MA. CAR T-cell therapy for B-cell lymphomas: clinical trial results of available products. Ther Adv Hematol. (2019) 10:2040620719841581. doi: 10.1177/2040620719841581
288. Chaudhari K, Rizvi S, Syed B. Non-Hodgkin lymphoma therapy landscape. Nat Rev Drug Discov. (2019) 18:663–4. doi: 10.1038/d41573-019-00051-6
289. Beltran PJ, Mitchell P, Chung Y-A, Cajulis E, Lu J, Belmontes B, et al. AMG 479, a fully human anti–insulin-like growth factor receptor type I monoclonal antibody, inhibits the growth and survival of pancreatic carcinoma cells. Mol Cancer Ther. (2009) 8:1095. doi: 10.1158/1535-7163.MCT-08-1171
290. Calzone FJ, Cajulis E, Chung Y-A, Tsai M-M, Mitchell P, Lu J, et al. Epitope-specific mechanisms of IGF1R inhibition by ganitumab. PLoS ONE. (2013) 8:e55135. doi: 10.1371/journal.pone.0055135
291. Cohn AL, Tabernero J, Maurel J, Nowara E, Sastre J, Chuah BYS, et al. A randomized, placebo-controlled phase 2 study of ganitumab or conatumumab in combination with FOLFIRI for second-line treatment of mutant KRAS metastatic colorectal cancer. Ann Oncol. (2013) 24:1777–85. doi: 10.1093/annonc/mdt057
292. Fuchs CS, Azevedo S, Okusaka T, Van Laethem JL, Lipton LR, Riess H, et al. A phase 3 randomized, double-blind, placebo-controlled trial of ganitumab or placebo in combination with gemcitabine as first-line therapy for metastatic adenocarcinoma of the pancreas: the GAMMA trial. Ann Oncol. (2015) 26:921–7. doi: 10.1093/annonc/mdv027
293. Bailey K, Cost C, Davis I, Glade Bender J, Grohar P, Houghton P, et al. Emerging novel agents for patients with advanced Ewing sarcoma: a report from the Children's Oncology Group (COG) New Agents for Ewing Sarcoma Task Force. F1000Research. (2019) 8:F1000 Faculty Rev-493. doi: 10.12688/f1000research.18139.1
294. Bready JV, Lee K, Jacobsen R, Graham K, Estrada J, Kaufman SA, et al. Abstract 1022: development and preclinical testing of AMG 780, a fully human antibody targeting angiopoietin 1 (Ang1) and angiopoietin 2 (Ang2). Cancer Res. (2014) 74:1022. doi: 10.1158/1538-7445.AM2014-1022
295. Dowlati A, Vlahovic G, Natale RB, Rasmussen E, Singh I, Hwang YC, et al. A phase I, first-in-human study of AMG 780, an angiopoietin-1 and−2 inhibitor, in patients with advanced solid tumors. Clin Cancer Res. (2016) 22:4574–84. doi: 10.1158/1078-0432.CCR-15-2145
296. Hamers-Casterman C, Atarhouch T, Muyldermans S, Robinson G, Hammers C, Songa EB, et al. Naturally occurring antibodies devoid of light chains. Nature. (1993) 363:446–8. doi: 10.1038/363446a0
297. Harmsen MM, De Haard HJ. Properties, production, and applications of camelid single-domain antibody fragments. Appl Microbiol Biotechnol. (2007) 77:13–22. doi: 10.1007/s00253-007-1142-2
298. Ulrichts H, Silence K, Schoolmeester A, de Jaegere P, Rossenu S, Roodt J, et al. Antithrombotic drug candidate ALX-0081 shows superior preclinical efficacy and safety compared with currently marketed antiplatelet drugs. Blood. (2011) 118:757. doi: 10.1182/blood-2010-11-317859
299. Duggan S. Caplacizumab: first global approval. Drugs. (2018) 78:1639–42. doi: 10.1007/s40265-018-0989-0
300. Scully M, Cataland SR, Peyvandi F, Coppo P, Knöbl P, Kremer Hovinga JA, et al. Results of the randomized, double-blind, placebo-controlled, phase 3 Hercules study of caplacizumab in patients with acquired thrombotic thrombocytopenic purpura. Blood. (2017) 130:LBA-1. doi: 10.1182/blood.V130.Suppl_1.LBA-1.LBA-1
301. Lu D, Jimenez X, Zhang H, Bohlen P, Witte L, Zhu Z. Selection of high affinity human neutralizing antibodies to VEGFR2 from a large antibody phage display library for antiangiogenesis therapy. Int J Cancer. (2002) 97:393–9. doi: 10.1002/ijc.1634
302. Lu D, Shen J, Vil MD, Zhang H, Jimenez X, Bohlen P, et al. Tailoring in vitro selection for a picomolar affinity human antibody directed against vascular endothelial growth factor receptor 2 for enhanced neutralizing activity. J Biol Chem. (2003) 278:43496–507. doi: 10.1074/jbc.M307742200
303. Chiorean EG, Hurwitz HI, Cohen RB, Schwartz JD, Dalal RP, Fox FE, et al. Phase I study of every 2- or 3-week dosing of ramucirumab, a human immunoglobulin G1 monoclonal antibody targeting the vascular endothelial growth factor receptor-2 in patients with advanced solid tumors. Ann Oncol. (2015) 26:1230–7. doi: 10.1093/annonc/mdv144
304. Imbimbo M, Vitali M, Fabbri A, Ottaviano M, Pasello G, Petrini I, et al. RELEVENT trial: phase II trial of ramucirumab, carboplatin, and paclitaxel in previously untreated thymic carcinoma/B3 thymoma with area of carcinoma. Clin Lung Cancer. (2018) 19:e811–4. doi: 10.1016/j.cllc.2018.06.005
305. Mackey JR, Ramos-Vazquez M, Lipatov O, McCarthy N, Krasnozhon D, Semiglazov V, et al. Primary results of ROSE/TRIO-12, a randomized placebo-controlled phase III trial evaluating the addition of ramucirumab to first-line docetaxel chemotherapy in metastatic breast cancer. J Clin Oncol. (2014) 33:141–8. doi: 10.1158/0008-5472.SABCS13-S5-04
306. Petrylak DP, de Wit R, Chi KN, Drakaki A, Sternberg CN, Nishiyama H, et al. Ramucirumab plus docetaxel versus placebo plus docetaxel in patients with locally advanced or metastatic urothelial carcinoma after platinum-based therapy (RANGE): a randomised, double-blind, phase 3 trial. Lancet. (2017) 390:2266–77. doi: 10.1016/S0140-6736(17)32365-6
307. Khan U, Shah MA. Ramucirumab for the treatment of gastric or gastro-esophageal junction cancer. Expert Opin Biol Ther. (2019) 19:1135–41. doi: 10.1080/14712598.2019.1656715
308. Garon EB, Ciuleanu T-E, Arrieta O, Prabhash K, Syrigos KN, Goksel T, et al. Ramucirumab plus docetaxel versus placebo plus docetaxel for second-line treatment of stage IV non-small-cell lung cancer after disease progression on platinum-based therapy (REVEL): a multicentre, double-blind, randomised phase 3 trial. Lancet. (2014) 384:665–73. doi: 10.1016/S0140-6736(14)60845-X
309. Verdaguer H, Tabernero J, Macarulla T. Ramucirumab in metastatic colorectal cancer: evidence to date and place in therapy. Ther Adv Med Oncol. (2016) 8:230–42. doi: 10.1177/1758834016635888
310. Zhu AX, Kang Y-K, Yen C-J, Finn RS, Galle PR, Llovet JM, et al. Ramucirumab after sorafenib in patients with advanced hepatocellular carcinoma and increased α-fetoprotein concentrations (REACH-2): a randomised, double-blind, placebo-controlled, phase 3 trial. Lancet Oncol. (2019) 20:282–96. doi: 10.1016/S1470-2045(18)30937-9
311. Presta LG, Chen H, Connor SJ, Chisholm V, Meng YG, Krummen L, et al. Humanization of an anti-vascular endothelial growth factor monoclonal antibody for the therapy of solid tumors and other disorders. Cancer Res. (1997) 57:4593.
312. Muller YA, Chen Y, Christinger HW, Li B, Cunningham BC, Lowman HB, et al. VEGF and the Fab fragment of a humanized neutralizing antibody: crystal structure of the complex at 2.4 Å resolution and mutational analysis of the interface. Structure. (1998) 6:1153–67. doi: 10.1016/S0969-2126(98)00116-6
313. Chen Y, Wiesmann C, Fuh G, Li B, Christinger HW, McKay P, et al. Selection and analysis of an optimized anti-VEGF antibody: crystal structure of an affinity-matured fab in complex with antigen. J Mol Biol. (1999) 293:865–81. doi: 10.1006/jmbi.1999.3192
314. Wykoff CC, Eichenbaum DA, Roth DB, Hill L, Fung AE, Haskova Z. Ranibizumab induces regression of diabetic retinopathy in most patients at high risk of progression to proliferative diabetic retinopathy. Ophthalmol Retina. (2018) 2:997–1009. doi: 10.1016/j.oret.2018.06.005
315. Gale RP, Mahmood S, Devonport H, Patel PJ, Ross AH, Walters G, et al. Action on neovascular age-related macular degeneration (nAMD): recommendations for management and service provision in the UK hospital eye service. Eye. (2019) 33:1–21. doi: 10.1038/s41433-018-0300-3
316. Brown DM, Campochiaro PA, Singh RP, Li Z, Gray S, Saroj N, et al. Ranibizumab for macular edema following central retinal vein occlusion: six-month primary end point results of a phase III study. Ophthalmology. (2010) 117:1124–33.e1. doi: 10.1016/j.ophtha.2010.02.022
317. Dervenis N, Mikropoulou AM, Tranos P, Dervenis P. Ranibizumab in the treatment of diabetic macular edema: a review of the current status, unmet needs, and emerging challenges. Adv Ther. (2017) 34:1270–82. doi: 10.1007/s12325-017-0548-1
318. Ohno-Matsui K, Suzaki M, Teshima R, Okami N. Real-world data on ranibizumab for myopic choroidal neovascularization due to pathologic myopia: results from a post-marketing surveillance in Japan. Eye. (2018) 32:1871–8. doi: 10.1038/s41433-018-0192-2
319. Stewart MW. A review of ranibizumab for the treatment of diabetic retinopathy. Ophthalmol Ther. (2017) 6:33–47. doi: 10.1007/s40123-017-0083-9
320. Tesar M. Fully human antibody MOR202 against CD38 for the treatment of multiple myeloma and other blood-borne malignancies. J Clin Oncol. (2007) 25:8106. doi: 10.1200/jco.2007.25.18_suppl.8106
321. Raab MS, Chatterjee M, Goldschmidt H, Agis H, Blau IW, Einsele H, et al. Phase I/IIa study of the human anti-CD38 antibody MOR202 (MOR03087) in relapsed or refractory multiple myeloma. Blood. (2015) 126:3035. doi: 10.1182/blood.V126.23.3035.3035
322. Carnemolla B, Borsi L, Balza E, Castellani P, Meazza R, Berndt A, et al. Enhancement of the antitumor properties of interleukin-2 by its targeted delivery to the tumor blood vessel extracellular matrix. Blood. (2002) 99:1659. doi: 10.1182/blood.V99.5.1659
323. Johannsen M, Spitaleri G, Curigliano G, Roigas J, Weikert S, Kempkensteffen C, et al. The tumour-targeting human L19-IL2 immunocytokine: preclinical safety studies, phase I clinical trial in patients with solid tumours and expansion into patients with advanced renal cell carcinoma. Eur J Cancer. (2010) 46:2926–35. doi: 10.1016/j.ejca.2010.07.033
324. Tang A, Harding F. The challenges and molecular approaches surrounding interleukin-2-based therapeutics in cancer. Cytokine. (2019) 1:100001. doi: 10.1016/j.cytox.2018.100001
325. Borsi L, Balza E, Carnemolla B, Sassi F, Castellani P, Berndt A, et al. Selective targeted delivery of TNFalpha to tumor blood vessels. Blood. (2003) 102:4384–92. doi: 10.1182/blood-2003-04-1039
326. Hemmerle T, Probst P, Giovannoni L, Green AJ, Meyer T, Neri D. The antibody-based targeted delivery of TNF in combination with doxorubicin eradicates sarcomas in mice and confers protective immunity. Br J Cancer. (2013) 109:1206–13. doi: 10.1038/bjc.2013.421
327. Murer P, Neri D. Antibody-cytokine fusion proteins: a novel class of biopharmaceuticals for the therapy of cancer and of chronic inflammation. Nat Biotechnol. (2019) 52:42–53. doi: 10.1016/j.nbt.2019.04.002
328. Borsi L, Balza E, Bestagno M, Castellani P, Carnemolla B, Biro A, et al. Selective targeting of tumoral vasculature: comparison of different formats of an antibody (L19) to the ED-B domain of fibronectin. Int J Cancer. (2002) 102:75–85. doi: 10.1002/ijc.10662
329. Poli GL, Bianchi C, Virotta G, Bettini A, Moretti R, Trachsel E, et al. Radretumab radioimmunotherapy in patients with brain metastasis: a 124I-L19SIP dosimetric PET study. Cancer Immunol Res. (2013) 1:134. doi: 10.1158/2326-6066.CIR-13-0007
330. Erba PA, Sollini M, Orciuolo E, Traino C, Petrini M, Paganelli G, et al. Radioimmunotherapy with radretumab in patients with relapsed hematologic malignancies. J Nucl Med. (2012) 53:922–7. doi: 10.2967/jnumed.111.101006
332. Tsai CW, Morris S. Approval of raxibacumab for the treatment of inhalation anthrax under the US Food and Drug Administration “Animal Rule”. Front Microbiol. (2015) 6:1320. doi: 10.3389/fmicb.2015.01320
333. Steidl S, Ratsch O, Brocks B, Durr M, Thomassen-Wolf E. In vitro affinity maturation of human GM-CSF antibodies by targeted CDR-diversification. Mol Immunol. (2008) 46:135–44. doi: 10.1016/j.molimm.2008.07.013
334. Buckley C, Campos JS, Zhdan V, Becker B, Chauhan D, Davy K, et al. OP0228 GSK3196165 an investigational anti-GM-CSF monoclonal antibody, improves patient reported outcomes in a phase IIb study of patients with rheumatoid arthritis (RA). Ann Rheum Dis. (2019) 78:191. doi: 10.1136/annrheumdis-2019-eular.6035
335. Cook AD, Hamilton JA. Investigational therapies targeting the granulocyte macrophage colony-stimulating factor receptor-α in rheumatoid arthritis: focus on mavrilimumab. Ther Adv Musculoskelet Dis. (2018) 10:29–38. doi: 10.1177/1759720X17752036
336. Schoeberl B, Pace EA, Fitzgerald JB, Harms BD, Xu L, Nie L, et al. Therapeutically targeting ErbB3: a key node in ligand-induced activation of the ErbB receptor–PI3K axis. Sci Signal. (2009) 2:ra31. doi: 10.1126/scisignal.2000352
337. Wassaf D, Kuang G, Kopacz K, Wu QL, Nguyen Q, Toews M, et al. High-throughput affinity ranking of antibodies using surface plasmon resonance microarrays. Anal Biochem. (2006) 351:241–53. doi: 10.1016/j.ab.2006.01.043
338. Curley MD, Sabnis GJ, Wille L, Adiwijaya BS, Garcia G, Moyo V, et al. Seribantumab, an anti-ERBB3 antibody, delays the onset of resistance and restores sensitivity to letrozole in an estrogen receptor-positive breast cancer model. Mol Cancer Ther. (2015) 14:2642–52. doi: 10.1158/1535-7163.MCT-15-0169
339. Sequist LV, Gray JE, Harb WA, Lopez-Chavez A, Doebele RC, Modiano MR, et al. Randomized phase II trial of seribantumab in combination with erlotinib in patients with EGFR wild-type non-small cell lung cancer. Oncologist. (2019) 24:1095–102. doi: 10.1634/theoncologist.2018-0695
340. Thom G, Cockroft AC, Buchanan AG, Candotti CJ, Cohen ES, Lowne D, et al. Probing a protein-protein interaction by in vitro evolution. Proc Natl Acad Sci USA. (2006) 103:7619–24. doi: 10.1073/pnas.0602341103
341. May RD, Monk PD, Cohen ES, Manuel D, Dempsey F, Davis NH, et al. Preclinical development of CAT-354, an IL-13 neutralizing antibody, for the treatment of severe uncontrolled asthma. Br J Pharmacol. (2012) 166:177–93. doi: 10.1111/j.1476-5381.2011.01659.x
342. Panettieri RA Jr, Sjöbring U, Péterffy A, Wessman P, Bowen K, et al. Tralokinumab for severe, uncontrolled asthma (STRATOS 1 and STRATOS 2): two randomised, double-blind, placebo-controlled, phase 3 clinical trials. Lancet Respir Med. (2018) 6:511–25. doi: 10.1016/S2213-2600(18)30184-X
343. Wollenberg A, Howell MD, Guttman-Yassky E, Silverberg JI, Kell C, Ranade K, et al. Treatment of atopic dermatitis with tralokinumab, an anti-IL-13 mAb. J Allergy Clin Immunol. (2019) 143:135–41. doi: 10.1016/j.jaci.2018.05.029
344. Parameswaran R, Lim M, Fei F, Abdel-Azim H, Arutyunyan A, Schiffer I, et al. Effector-mediated eradication of precursor B acute lymphoblastic leukemia with a novel Fc-engineered monoclonal antibody targeting the BAFF-R. Mol Cancer Ther. (2014) 13:1567. doi: 10.1158/1535-7163.MCT-13-1023
345. Yamane-Ohnuki N, Kinoshita S, Inoue-Urakubo M, Kusunoki M, Iida S, Nakano R, et al. Establishment of FUT8 knockout Chinese hamster ovary cells: an ideal host cell line for producing completely defucosylated antibodies with enhanced antibody-dependent cellular cytotoxicity. Biotechnol Bioeng. (2004) 87:614–22. doi: 10.1002/bit.20151
346. McWilliams EM, Lucas CR, Chen T, Harrington BK, Wasmuth R, Campbell A, et al. Anti-BAFF-R antibody VAY-736 demonstrates promising preclinical activity in CLL and enhances effectiveness of ibrutinib. Blood Adv. (2019) 3:447–60. doi: 10.1182/bloodadvances.2018025684
347. Dörner T, Posch MG, Li Y, Petricoul O, Cabanski M, Milojevic JM, et al. Treatment of primary Sjögren's syndrome with ianalumab (VAY736) targeting B cells by BAFF receptor blockade coupled with enhanced, antibody-dependent cellular cytotoxicity. Ann Rheum Dis. (2019) 78:641. doi: 10.1136/annrheumdis-2018-214720
348. Dubey D, Forsthuber T, Flanagan EP, Pittock SJ, Stuve O. B-cell-targeted therapies in relapsing forms of MS. Neurol Neuroimmunol Neuroinflamm. (2017) 4:e405. doi: 10.1212/NXI.0000000000000405
349. Brack SS, Silacci M, Birchler M, Neri D. Tumor-targeting properties of novel antibodies specific to the large isoform of tenascin-C. Clin Cancer Res. (2006) 12:3200. doi: 10.1158/1078-0432.CCR-05-2804
350. Mårlind J, Kaspar M, Trachsel E, Sommavilla R, Hindle S, Bacci C, et al. Antibody-mediated delivery of interleukin-2 to the stroma of breast cancer strongly enhances the potency of chemotherapy. Clin Cancer Res. (2008) 14:6515. doi: 10.1158/1078-0432.CCR-07-5041
351. Viti F, Nilsson F, Demartis S, Huber A, Neri D. Design and use of phage display libraries for the selection of antibodies and enzymes. Methods Enzymol. (2000) 326:480–505. doi: 10.1016/S0076-6879(00)26071-0
352. Schliemann C, Gutbrodt KL, Kerkhoff A, Pohlen M, Wiebe S, Silling G, et al. Targeting interleukin-2 to the bone marrow stroma for therapy of acute myeloid leukemia relapsing after allogeneic hematopoietic stem cell transplantation. Cancer Immunol Res. (2015) 3:547. doi: 10.1158/2326-6066.CIR-14-0179
353. Rabinowits G. Systemic therapy for merkel cell carcinoma: what's on the horizon? Cancers (Basel). (2014) 6:1180–94. doi: 10.3390/cancers6021180
354. List T, Neri D. Immunocytokines: a review of molecules in clinical development for cancer therapy. Clin Pharmacol. (2013) 5:29–45. doi: 10.2147/CPAA.S49231
355. Friedbichler K, Hofmann MH, Kroez M, Ostermann E, Lamche HR, Koessl C, et al. Pharmacodynamic and antineoplastic activity of BI 836845, a fully human IGF ligand-neutralizing antibody, and mechanistic rationale for combination with rapamycin. Mol Cancer Ther. (2014) 13:399. doi: 10.1158/1535-7163.MCT-13-0598
356. Park K, Cho BC, Lee KH, Su W-C, Kim S-W, Lin C-C, et al. P3.02b-005 phase Ib trial of afatinib and BI 836845 in advanced NSCLC: dose escalation and safety results: topic: EGFR biomarkers. J Thorac Oncol. (2017) 12:S1187–8. doi: 10.1016/j.jtho.2016.11.1672
357. Rihawi K, Ong M, Michalarea V, Bent L, Buschke S, Bogenrieder T, et al. Phase I dose escalation study of 3-weekly BI 836845, a fully human, affinity optimized, insulin-like growth factor (IGF) ligand neutralizing antibody, in patients with advanced solid tumors. J Clin Oncol. (2014) 32:2622. doi: 10.1200/jco.2014.32.15_suppl.2622
358. Simpson A, Petnga W, Macaulay VM, Weyer-Czernilofsky U, Bogenrieder T. Insulin-like growth factor (IGF) pathway targeting in cancer: role of the IGF axis and opportunities for future combination studies. Target Oncol. (2017) 12:571–97. doi: 10.1007/s11523-017-0514-5
359. Schmid P, Rugo HS, Cortes J, Huang C-L, Crossley K, Massey D, et al. XENERA-1: A phase II trial of xentuzumab (Xe) in combination with everolimus (Ev) and exemestane (Ex) in patients with hormone receptor-positive (HR+)/human epidermal growth factor receptor 2-negative (HER2-) metastatic breast cancer (mBC) and non-visceral involvement. J Clin Oncol. (2019) 37:TPS1103. doi: 10.1200/JCO.2019.37.15_suppl.TPS1103
360. Kneissel M, Halleux C, Hu S-I, Diefenbach-Streiber B, Prassler J, Novartis AG. Compositions and Methods for Use for Antibodies Against Sclerostin. US patent US 7879322 B2. U.S. Patent and Trademark Office (2011).
361. Glorieux FH, Devogelaer JP, Durigova M, Goemaere S, Hemsley S, Jakob F, et al. BPS804 anti-sclerostin antibody in adults with moderate osteogenesis imperfecta: results of a randomized phase 2a trial. J Bone Miner Res. (2017) 32:1496–504. doi: 10.1002/jbmr.3143
362. Seefried L, Baumann J, Hemsley S, Hofmann C, Kunstmann E, Kiese B, et al. Efficacy of anti-sclerostin monoclonal antibody BPS804 in adult patients with hypophosphatasia. J Clin Invest. (2017) 127:2148–58. doi: 10.1172/JCI83731
363. MacNabb C, Patton D, Hayes JS. Sclerostin antibody therapy for the treatment of osteoporosis: clinical prospects and challenges. J Osteoporos. (2016) 2016:6217286. doi: 10.1155/2016/6217286
364. Persaud K, Tille JC, Liu M, Zhu Z, Jimenez X, Pereira DS, et al. Involvement of the VEGF receptor 3 in tubular morphogenesis demonstrated with a human anti-human VEGFR-3 monoclonal antibody that antagonizes receptor activation by VEGF-C. J Cell Sci. (2004) 117:2745–56. doi: 10.1242/jcs.01138
365. Saif MW, Knost JA, Chiorean EG, Kambhampati SR, Yu D, Pytowski B, et al. Phase 1 study of the anti-vascular endothelial growth factor receptor 3 monoclonal antibody LY3022856/IMC-3C5 in patients with advanced and refractory solid tumors and advanced colorectal cancer. Cancer Chemother Pharmacol. (2016) 78:815–24. doi: 10.1007/s00280-016-3134-3
366. Sommer A, Kopitz C, Schatz CA, Nising CF, Mahlert C, Lerchen H-G, et al. Preclinical efficacy of the auristatin-based antibody–drug conjugate BAY 1187982 for the treatment of FGFR2-positive solid tumors. Cancer Res. (2016) 76:6331. doi: 10.1158/0008-5472.CAN-16-0180
367. Söderlind E, Strandberg L, Jirholt P, Kobayashi N, Alexeiva V, Åberg A-M, et al. Recombining germline-derived CDR sequences for creating diverse single-framework antibody libraries. Nat Biotechnol. (2000) 18:852–6. doi: 10.1038/78458
368. Schatz CA, Kopitz C, Wittemer-Rump S, Sommer A, Lindbom L, Osada M, et al. Abstract 4766: pharmacodynamic and stratification biomarker for the anti-FGFR2 antibody (BAY1179470) and the FGFR2-ADC. Cancer Res. (2014) 74:4766. doi: 10.1158/1538-7445.AM2014-4766
369. Wang Z, Murphy JE, Pan J, Jiang H, Liu B, Bayer Health Care LLC. Monoclonal Antibodies Against Tissue Factor Pathway Inhibitor (TFPI). US patent US 20180334509 A1. U.S. Patent and Trademark Office (2018).
370. Bauzon M, Liu B, Grudzinska-Goebel J, Tebbe J, Pan J, Cifrese J, et al. Characterization of a high-affinity fully human IgG2 antibody against tissue factor pathway inhibitor as a bypass agent for the treatment of hemophilia. Blood. (2015) 126:3513. doi: 10.1182/blood.V126.23.3513.3513
371. Paz P, Xie J, Aswad F. Antibody engineering of anti-TFPI bypass therapeutic BAY 1093884: isotype selection and sequence optimization. Blood. (2015) 126:3496. doi: 10.1182/blood.V126.23.3496.3496
372. Lenting PJ. Laboratory monitoring of hemophilia A treatments: new challenges. Blood Adv. (2020) 4:2111–8. doi: 10.1182/bloodadvances.2019000849
373. Thomas D, Thelen K, Kraff S, Schwers S, Schiffer S, Unger S, et al. BAY 1213790, a fully human IgG1 antibody targeting coagulation factor XIa: first evaluation of safety, pharmacodynamics, and pharmacokinetics. Res Pract Thromb Haemost. (2019) 3:242–53. doi: 10.1002/rth2.12186
374. Schaefer M, Buchmueller A, Dittmer F, Strassburger J, Wilmen A. Allosteric inhibition as a new mode of action for BAY 1213790, a neutralizing antibody targeting the activated form of coagulation factor XI. J Mol Biol. (2019) 431:4817–33. doi: 10.1016/j.jmb.2019.09.008
375. Quan ML, Pinto DJP, Smallheer JM, Ewing WR, Rossi KA, Luettgen JM, et al. Factor XIa inhibitors as new anticoagulants. J Med Chem. (2018) 61:7425–47. doi: 10.1021/acs.jmedchem.8b00173
376. Almagro Juan C, Della Ducata D, Elloso M, Luo J, Malia T, Naso M, et al. Il-17a Antibodies. US patent US 8519107 B2. U.S. Patent and Trademark Office (2013).
377. Mease PJ, Jeka S, Jaller JJ, Kitumnuaypong T, Louthrenoo W, Mann H, et al. CNTO6785, a fully human antiinterleukin 17 monoclonal antibody, in patients with rheumatoid arthritis with inadequate response to methotrexate: a randomized, placebo-controlled, phase II, dose-ranging study. J Rheumatol. (2018) 45:22. doi: 10.3899/jrheum.161238
378. Eich A, Urban V, Jutel M, Vlcek J, Shim JJ, Trofimov VI, et al. A randomized, placebo-controlled phase 2 trial of CNTO 6785 in chronic obstructive pulmonary disease. COPD. (2017) 14:476–83. doi: 10.1080/15412555.2017.1335697
379. Cunningham M, Feng Y, Heeringa K, Luo J, Rauchenberger R, Rutz M, et al. Toll-like Receptor 3 Antagonists. US patent US 8409567 B2. U.S. Patent and Trademark Office (2013).
380. Silkoff PE, Flavin S, Gordon R, Loza MJ, Sterk PJ, Lutter R, et al. Toll-like receptor 3 blockade in rhinovirus-induced experimental asthma exacerbations: a randomized controlled study. J Allergy Clin Immunol. (2018) 141:1220–30. doi: 10.1016/j.jaci.2017.06.027
381. Kimball AB, Gordon KB, Langley RG, Menter A, Chartash EK, Valdes J, et al. Safety and efficacy of ABT-874, a fully human interleukin 12/23 monoclonal antibody, in the treatment of moderate to severe chronic plaque psoriasis: results of a randomized, placebo-controlled, phase 2 trial. JAMA Dermatol. (2008) 144:200–7. doi: 10.1001/archdermatol.2007.63
382. Ding C, Xu J, Li J. ABT-874, a fully human monoclonal anti-IL-12/IL-23 antibody for the potential treatment of autoimmune diseases. Curr Opin Investig Drugs. (2008) 9:515–22.
383. Vollmer TL, Wynn DR, Alam MS, Valdes J. A phase 2, 24-week, randomized, placebo-controlled, double-blind study examining the efficacy and safety of an anti-interleukin-12 and−23 monoclonal antibody in patients with relapsing-remitting or secondary progressive multiple sclerosis. Mult Scler. (2011) 17:181–91. doi: 10.1177/1352458510384496
384. Strober BE, Crowley JJ, Yamauchi PS, Olds M, Williams DA. Efficacy and safety results from a phase III, randomized controlled trial comparing the safety and efficacy of briakinumab with etanercept and placebo in patients with moderate to severe chronic plaque psoriasis. Br J Dermatol. (2011) 165:661–8. doi: 10.1111/j.1365-2133.2011.10419.x
385. Ettenberg S, Cong F, Shulok J, Urlinger S, Bardroff M, Charlat O, et al. BHQ880, a novel anti-DKK1 neutralizing antibody, inhibits tumor-induced osteolytic bone disease. Cancer Research. (2008) 68:3987.
386. Padmanabhan S, Beck JT, Kelly KR, Munshi NC, Dzik-Jurasz A, Gangolli E, et al. A phase I/II study of BHQ880, a novel osteoblat activating, anti-DKK1 human monoclonal antibody, in relapsed and refractory multiple myeloma (MM) patients treated with zoledronic acid (Zol) and anti-myeloma therapy (MM Tx). Blood. (2009) 114:750. doi: 10.1182/blood.V114.22.750.750
387. Munshi NC, Abonour R, Beck JT, Bensinger W, Facon T, Stockerl-Goldstein K, et al. Early evidence of anabolic bone activity of BHQ880, a fully human anti-DKK1 neutralizing antibody: results of a phase 2 study in previously untreated patients with smoldering multiple myeloma at risk for progression. Blood. (2012) 120:331. doi: 10.1182/blood.V120.21.331.331
388. Roghanian A, Teige I, Martensson L, Cox KL, Kovacek M, Ljungars A, et al. Antagonistic human FcgammaRIIB (CD32B) antibodies have anti-tumor activity and overcome resistance to antibody therapy in vivo. Cancer Cell. (2015) 27:473–88. doi: 10.1016/j.ccell.2015.03.005
389. Teige I, Martensson L, Frendeus BL. Targeting the antibody checkpoints to enhance cancer immunotherapy-focus on FcgammaRIIB. Front Immunol. (2019) 10:481. doi: 10.3389/fimmu.2019.00481
390. Villa A, Trachsel E, Kaspar M, Schliemann C, Sommavilla R, Rybak J-N, et al. A high-affinity human monoclonal antibody specific to the alternatively spliced EDA domain of fibronectin efficiently targets tumor neo-vasculature in vivo. Int J Cancer. (2008) 122:2405–13. doi: 10.1002/ijc.23408
391. Galeazzi M, Sebastiani G, Voll R, Viapiana O, Dudler J, Zufferey P, et al. FRI0118 Dekavil (F8IL10)–update on the results of clinical trials investigating the immunocytokine in patients with rheumatoid arthritis. Ann Rheum Dis. (2018) 77:603.2–4. doi: 10.1136/annrheumdis-2018-eular.5550
392. Schier R, Marks JD, Wolf EJ, Apell G, Wong C, McCartney JE, et al. In vitro and in vivo characterization of a human anti-c-erbB-2 single-chain Fv isolated from a filamentous phage antibody library. Immunotechnology. (1995) 1:73–81. doi: 10.1016/1380-2933(95)00007-0
393. Zhou Y, Zhao L, Marks JD. Selection and characterization of cell binding and internalizing phage antibodies. Arch Biochem Biophys. (2012) 526:107–13. doi: 10.1016/j.abb.2012.05.007
394. Miller K, Cortes J, Hurvitz SA, Krop IE, Tripathy D, Verma S, et al. HERMIONE: a randomized phase 2 trial of MM-302 plus trastuzumab versus chemotherapy of physician's choice plus trastuzumab in patients with previously treated, anthracycline-naïve, HER2-positive, locally advanced/metastatic breast cancer. BMC Cancer. (2016) 16:352. doi: 10.1186/s12885-016-2385-z
395. Munster P, Krop IE, LoRusso P, Ma C, Siegel BA, Shields AF, et al. Safety and pharmacokinetics of MM-302, a HER2-targeted antibody–liposomal doxorubicin conjugate, in patients with advanced HER2-positive breast cancer: a phase 1 dose-escalation study. Br J Cancer. (2018) 119:1086–93. doi: 10.1038/s41416-018-0235-2
396. Benson J, Carton J, Cunningham M, Orlovsky Yevgeniya I, Rauchenberger R, Sweet R, et al. Human Anti-il-23 Antibodies, Compositions, Methods and Uses. US patent US 9353181 B2. U.S. Patent and Trademark Office (2016).
397. Teplyakov A, Luo J, Obmolova G, Malia TJ, Sweet R, Stanfield RL, et al. Antibody modeling assessment II. Structures and models. Proteins. (2014) 82:1563–82. doi: 10.1002/prot.24554
398. Markham A. Guselkumab: first global approval. Drugs. (2017) 77:1487–92. doi: 10.1007/s40265-017-0800-7
399. Smolen JS, Agarwal SK, Ilivanova E, Xu XL, Miao Y, Zhuang Y, et al. A randomised phase II study evaluating the efficacy and safety of subcutaneously administered ustekinumab and guselkumab in patients with active rheumatoid arthritis despite treatment with methotrexate. Ann Rheum Dis. (2017) 76:831. doi: 10.1136/annrheumdis-2016-209831
400. Terui T, Kobayashi S, Okubo Y, Murakami M, Hirose K, Kubo H. Efficacy and safety of guselkumab, an anti–interleukin 23 monoclonal antibody, for palmoplantar pustulosis: a randomized clinical trial. JAMA Dermatol. (2018) 154:309–16. doi: 10.1001/jamadermatol.2017.5937
401. Willuda J, Linden L, Lerchen H-G, Kopitz C, Stelte-Ludwig B, Pena C, et al. Preclinical antitumor efficacy of BAY 1129980—a novel auristatin-based anti-C4.4A (LYPD3) antibody–drug conjugate for the treatment of non–small cell lung cancer. Mol Cancer Ther. (2017) 16:893. doi: 10.1158/1535-7163.MCT-16-0474
402. Kenniston JA, Faucette RR, Martik D, Comeau SR, Lindberg AP, Kopacz KJ, et al. Inhibition of plasma kallikrein by a highly specific active site blocking antibody. J Biol Chem. (2014) 289:23596–608. doi: 10.1074/jbc.M114.569061
403. Banerji A, Riedl MA, Bernstein JA, Cicardi M, Longhurst HJ, Zuraw BL, et al. Effect of lanadelumab compared with placebo on prevention of hereditary angioedema attacks: a randomized clinical trial. JAMA. (2018) 320:2108–21. doi: 10.1001/jama.2018.16773
404. Syed YY. Lanadelumab: first global approval. Drugs. (2018) 78:1633–7. doi: 10.1007/s40265-018-0987-2
405. Merchant MS, Geller JI, Baird K, Chou AJ, Galli S, Charles A, et al. Phase I trial and pharmacokinetic study of lexatumumab in pediatric patients with solid tumors. J Clin Oncol. (2012) 30:4141–7. doi: 10.1200/JCO.2012.44.1055
406. Sikic BI, Wakelee HA, von Mehren M, Lewis N, Calvert AH, Plummer ER, et al. A phase Ib study to assess the safety of lexatumumab, a human monoclonal antibody that activates TRAIL-R2, in combination with gemcitabine, pemetrexed, doxorubicin or FOLFIRI. J Clin Oncol. (2007) 25:14006. doi: 10.1200/jco.2007.25.18_suppl.14006
407. Hay CM, Sult E, Huang Q, Mulgrew K, Fuhrmann SR, McGlinchey KA, et al. Targeting CD73 in the tumor microenvironment with MEDI9447. OncoImmunology. (2016) 5:e1208875. doi: 10.1080/2162402X.2016.1208875
408. Overman MJ, LoRusso P, Strickler JH, Patel SP, Clarke SJ, Noonan AM, et al. Safety, efficacy and pharmacodynamics (PD) of MEDI9447 (oleclumab) alone or in combination with durvalumab in advanced colorectal cancer (CRC) or pancreatic cancer (panc). J Clin Oncol. (2018) 36:4123. doi: 10.1200/JCO.2018.36.15_suppl.4123
409. Rizvi N, Shu C, Goldberg S, Papadimitrakopoulou V, Camidge DR, Patel S, et al. P3.04–23 phase 1b/2 study to evaluate novel combinations with oleclumab (MEDI9447) in previously treated advanced EGFRm NSCLC. J Thorac Oncol. (2018) 13:S930–1. doi: 10.1016/j.jtho.2018.08.1730
410. Schmid P, Nunes AT, Lall R, Cruz C, Grinsted L, Lanasa MC. Abstract OT3–01-01: BEGONIA: phase Ib/II open-label, platform study of safety and efficacy of durvalumab, paclitaxel and other novel oncology therapy agents as first-line (1L) therapy in patients with metastatic triple negative breast cancer (mTNBC). Cancer Res. (2019) 79:OT3-01. doi: 10.1158/1538-7445.SABCS18-OT3-01-01
411. Yen W-C, Fischer MM, Axelrod F, Bond C, Cain J, Cancilla B, et al. Targeting notch signaling with a Notch2/Notch3 antagonist (tarextumab) inhibits tumor growth and decreases tumor-initiating cell frequency. Clin Cancer Res. (2015) 21:2084. doi: 10.1158/1078-0432.CCR-14-2808
412. Smith DC, Chugh R, Patnaik A, Papadopoulos KP, Wang M, Kapoun AM, et al. A phase 1 dose escalation and expansion study of tarextumab (OMP-59R5) in patients with solid tumors. Invest New Drugs. (2019) 37:722–30. doi: 10.1007/s10637-018-0714-6
413. O'Reilly EM, Sahai V, Bendell JC, Bullock AJ, LoConte NK, Hatoum H, et al. Results of a randomized phase II trial of an anti-notch 2/3, tarextumab (OMP-59R5, TRXT, anti-Notch2/3), in combination with nab-paclitaxel and gemcitabine (Nab-P+Gem) in patients (pts) with untreated metastatic pancreatic cancer (mPC). J Clin Oncol. (2017) 35:279. doi: 10.1200/JCO.2017.35.4_suppl.279
414. Hu ZI, Bendell JC, Bullock A, LoConte NK, Hatoum H, Ritch P, et al. A randomized phase II trial of nab-paclitaxel and gemcitabine with tarextumab or placebo in patients with untreated metastatic pancreatic cancer. Cancer Med. (2019) 8:5148–57. doi: 10.1002/cam4.2425
415. Ramos EK, Hoffmann AD, Gerson SL, Liu H. New opportunities and challenges to defeat cancer stem cells. Trends Cancer. (2017) 3:780–96. doi: 10.1016/j.trecan.2017.08.007
416. Garner AP, Bialucha CU, Sprague ER, Garrett JT, Sheng Q, Li S, et al. An antibody that locks HER3 in the inactive conformation inhibits tumor growth driven by HER2 or neuregulin. Cancer Res. (2013) 73:6024. doi: 10.1158/0008-5472.CAN-13-1198
417. Prassler J, Steidl S, Urlinger S. In vitro affinity maturation of HuCAL antibodies: complementarity determining region exchange and RapMAT technology. Immunotherapy. (2009) 1:571–83. doi: 10.2217/imt.09.23
418. Reynolds KL, Bedard PL, Lee S-H, Lin C-C, Tabernero J, Alsina M, et al. A phase I open-label dose-escalation study of the anti-HER3 monoclonal antibody LJM716 in patients with advanced squamous cell carcinoma of the esophagus or head and neck and HER2-overexpressing breast or gastric cancer. BMC Cancer. (2017) 17:646. doi: 10.1186/s12885-017-3641-6
419. Takahashi S, Kobayashi T, Tomomatsu J, Ito Y, Oda H, Kajitani T, et al. LJM716 in Japanese patients with head and neck squamous cell carcinoma or HER2-overexpressing breast or gastric cancer. Cancer Chemother Pharmacol. (2017) 79:131–8. doi: 10.1007/s00280-016-3214-4
420. Malm M, Frejd FY, Ståhl S, Löfblom J. Targeting HER3 using mono- and bispecific antibodies or alternative scaffolds. mAbs. (2016) 8:1195–209. doi: 10.1080/19420862.2016.1212147
421. Mishra R, Patel H, Alanazi S, Yuan L, Garrett JT. HER3 signaling and targeted therapy in cancer. Oncol Rev. (2018) 12:355. doi: 10.4081/oncol.2018.355
422. Bohrmann B, Baumann K, Benz J, Gerber F, Huber W, Knoflach F, et al. Gantenerumab: a novel human anti-abeta antibody demonstrates sustained cerebral amyloid-beta binding and elicits cell-mediated removal of human amyloid-beta. J Alzheimers Dis. (2012) 28:49–69. doi: 10.3233/JAD-2011-110977
423. Ostrowitzki S, Lasser RA, Dorflinger E, Scheltens P, Barkhof F, Nikolcheva T, et al. A phase III randomized trial of gantenerumab in prodromal Alzheimer's disease. Alzheimers Res Ther. (2017) 9:95. doi: 10.1186/s13195-017-0318-y
424. Gurney A, Axelrod F, Bond CJ, Cain J, Chartier C, Donigan L, et al. Wnt pathway inhibition via the targeting of Frizzled receptors results in decreased growth and tumorigenicity of human tumors. Proc Natl Acad Sci USA. (2012) 109:11717. doi: 10.1073/pnas.1120068109
425. Smith DC, Rosen LS, Chugh R, Goldman JW, Xu L, Kapoun A, et al. First-in-human evaluation of the human monoclonal antibody vantictumab (OMP-18R5; anti-Frizzled) targeting the WNT pathway in a phase I study for patients with advanced solid tumors. J Clin Oncol. (2013) 31:2540. doi: 10.1200/jco.2013.31.15_suppl.2540
426. Fischer MM, Cancilla B, Yeung VP, Cattaruzza F, Chartier C, Murriel CL, et al. WNT antagonists exhibit unique combinatorial antitumor activity with taxanes by potentiating mitotic cell death. Sci Adv. (2017) 3:e1700090. doi: 10.1126/sciadv.1700090
427. Cohen ES, Dobson CL, Käck H, Wang B, Sims DA, Lloyd CO, et al. A novel IgE-neutralizing antibody for the treatment of severe uncontrolled asthma. mAbs. (2014) 6:755–63. doi: 10.4161/mabs.28394
428. Sheldon E, Schwickart M, Li J, Kim K, Crouch S, Parveen S, et al. Pharmacokinetics, pharmacodynamics, and safety of MEDI4212, an anti-IgE monoclonal antibody, in subjects with atopy: a phase I study. Adv Ther. (2016) 33:225–51. doi: 10.1007/s12325-016-0287-8
429. Adams C, Totpal K, Lawrence D, Marsters S, Pitti R, Yee S, et al. Structural and functional analysis of the interaction between the agonistic monoclonal antibody Apomab and the proapoptotic receptor DR5. Cell Death Differ. (2008) 15:751–61. doi: 10.1038/sj.cdd.4402306
430. Camidge DR, Herbst RS, Gordon MS, Eckhardt SG, Kurzrock R, Durbin B, et al. A phase I safety and pharmacokinetic study of the death receptor 5 agonistic antibody PRO95780 in patients with advanced malignancies. Clin Cancer Res. (2010) 16:1256. doi: 10.1158/1078-0432.CCR-09-1267
431. Wittebol S, Ferrant A, Wickham NW, Fehrenbacher L, Durbin-Johnson B, Bray GL. Phase II study of PRO95780 plus rituximab in patients with relapsed follicular non-Hodgkin's lymphoma (NHL). J Clin Oncol. (2010) 28:e18511. doi: 10.1200/jco.2010.28.15_suppl.e18511
432. Karapetis CS, Clingan PR, Leighl NB, Durbin-Johnson B, O'Neill V, Spigel DR. Phase II study of PRO95780 plus paclitaxel, carboplatin, and bevacizumab (PCB) in non-small cell lung cancer (NSCLC). J Clin Oncol. (2010) 28:7535. doi: 10.1200/jco.2010.28.15
433. Roguska M, Splawski I, Diefenbach-Streiber B, Dolan E, Etemad-Gilbertson B, Rondeau J-M, et al. Generation and characterization of LFG316, a fully-human anti-C5 antibody for the treatment of age-related macular degeneration. Invest Ophthalmol Visual Sci. (2014) 55:3433.
434. Nebbioso M, Lambiase A, Cerini A, Limoli PG, La Cava M, Greco A. Therapeutic approaches with intravitreal injections in geographic atrophy secondary to age-related macular degeneration: current drugs and potential molecules. Int J Mol Sci. (2019) 20:1693. doi: 10.3390/ijms20071693
435. Ricklin D, Mastellos DC, Reis ES, Lambris JD. The renaissance of complement therapeutics. Nat Rev Nephrol. (2018) 14:26–47. doi: 10.1038/nrneph.2017.156
436. Ferlin W, Fischer N, Elson G, Leger O, NovImmune SA. Anti-interferon Gamma Antibodies and Methods of Use Thereof. US patent US 9682142 B2. U.S. Patent and Trademark Office (2017).
437. Al-Salama ZT. Emapalumab: first global approval. Drugs. (2019) 79:99–103. doi: 10.1007/s40265-018-1046-8
438. Kerschbaumer R, Scheiflinger F, Rieger M, Thiele M, Mudde CG, Muellberg J, et al. Anti Mif Antibodies. EP patent EP 2231707 B1. European Patent Office (2014).
439. Mahalingam D, Patel M, Sachdev J, Hart L, Halama N, Ramanathan R, et al. PD-011Safety and efficacy analysis of imalumab, an anti-oxidized macrophage migration inhibitory factor (oxMIF) antibody, alone or in combination with 5-fluorouracil/leucovorin (5-FU/LV) or panitumumab, in patients with metastatic colorectal cancer (mCRC). Ann Oncol. (2016) 27:ii105. doi: 10.1093/annonc/mdw200.11
440. Meyer L, Suidan R, Sun C, Westin S, Coleman RL, Mills GB. The management of malignant ascites and impact on quality of life outcomes in women with ovarian cancer. Expert Rev Qual Life Cancer Care. (2016) 1:231–8. doi: 10.1080/23809000.2016.1185369
441. Lehrer-Graiwer J, Singh P, Abdelbaky A, Vucic E, Korsgren M, Baruch A, et al. FDG-PET imaging for oxidized LDL in stable atherosclerotic disease: a phase II study of safety, tolerability, and anti-inflammatory activity. JACC Cardiovasc Imaging. (2015) 8:493–4. doi: 10.1016/j.jcmg.2014.06.021
442. Wichert S, Juliusson G, Johansson A, Sonesson E, Teige I, Wickenberg AT, et al. A single-arm, open-label, phase 2 clinical trial evaluating disease response following treatment with BI-505, a human anti-intercellular adhesion molecule-1 monoclonal antibody, in patients with smoldering multiple myeloma. PLoS ONE. (2017) 12:e0171205. doi: 10.1371/journal.pone.0171205
444. Pettersson K, Camber OLA, Sexton DAN, Nixon Andrew E, Athera Biotechnologies Ab. Dyax Corp. New Antibodies Against Phosphorylcholine. EP patent EP 2742068 B1. European Patent Office (2019).
445. Pettersson K, Ewing Mark M, de Vries Margreet R, Atout R, Nordzell M, Sexton Daniel J, et al. Abstract 15644: a fully human monoclonal IgG phosphorylcholine antibody prevents accelerated atherosclerosis in mice. Circulation. (2011) 124:A15644.
446. Nilsson J, Hansson GK. Vaccination strategies and immune modulation of atherosclerosis. Circ Res. (2020) 126:1281–96. doi: 10.1161/CIRCRESAHA.120.315942
447. Zhu Z, Dimitrov AS, Bossart KN, Crameri G, Bishop KA, Choudhry V, et al. Potent neutralization of Hendra and Nipah viruses by human monoclonal antibodies. J Virol. (2006) 80:891–9. doi: 10.1128/JVI.80.2.891-899.2006
448. Zhu Z, Bossart KN, Bishop KA, Crameri G, Dimitrov AS, McEachern JA, et al. Exceptionally potent cross-reactive neutralization of Nipah and Hendra viruses by a human monoclonal antibody. J Infect Dis. (2008) 197:846–53. doi: 10.1086/528801
449. Playford EG, Munro T, Mahler SM, Elliott S, Gerometta M, Hoger KL, et al. Safety, tolerability, pharmacokinetics, and immunogenicity of a human monoclonal antibody targeting the G glycoprotein of henipaviruses in healthy adults: a first-in-human, randomised, controlled, phase 1 study. Lancet Infect Dis. (2020) doi: 10.1016/S1473-3099(19)30634-6
450. Silence K, Dreier T, Moshir M, Ulrichts P, Gabriels SM, Saunders M, et al. ARGX-110, a highly potent antibody targeting CD70, eliminates tumors via both enhanced ADCC and immune checkpoint blockade. mAbs. (2014) 6:523–32. doi: 10.4161/mabs.27398
451. Klarenbeek A, El Mazouari K, Desmyter A, Blanchetot C, Hultberg A, de Jonge N, et al. Camelid Ig V genes reveal significant human homology not seen in therapeutic target genes, providing for a powerful therapeutic antibody platform. mAbs. (2015) 7:693–706. doi: 10.1080/19420862.2015.1046648
452. Shinkawa T, Nakamura K, Yamane N, Shoji-Hosaka E, Kanda Y, Sakurada M, et al. The absence of fucose but not the presence of galactose or bisecting N-acetylglucosamine of human IgG1 complex-type oligosaccharides shows the critical role of enhancing antibody-dependent cellular cytotoxicity. J Biol Chem. (2003) 278:3466–73. doi: 10.1074/jbc.M210665200
453. Ochsenbein AF, Riether C, Bacher U, Müller R, Höpner S, Banz Y, et al. Argx-110 targeting CD70, in combination with azacitidine, shows favorable safety profile and promising anti-leukemia activity in newly diagnosed AML patients in an ongoing phase 1/2 clinical trial. Blood. (2018) 132:2680. doi: 10.1182/blood-2018-99-118302
454. Aftimos P, Rolfo C, Rottey S, Offner F, Bron D, Maerevoet M, et al. Phase I dose-escalation study of the anti-CD70 antibody ARGX-110 in advanced malignancies. Clin Cancer Res. (2017) 23:6411–20. doi: 10.1158/1078-0432.CCR-17-0613
455. Basilico C, Hultberg A, Blanchetot C, de Jonge N, Festjens E, Hanssens V, et al. Four individually druggable MET hotspots mediate HGF-driven tumor progression. J Clin Invest. (2014) 124:3172–86. doi: 10.1172/JCI72316
456. Hultberg A, Morello V, Huyghe L, De Jonge N, Blanchetot C, Hanssens V, et al. Depleting MET-expressing tumor cells by ADCC provides a therapeutic advantage over inhibiting HGF/MET signaling. Cancer Res. (2015) 75:3373–83. doi: 10.1158/0008-5472.CAN-15-0356
457. Vaccaro C, Bawdon R, Wanjie S, Ober RJ, Ward ES. Divergent activities of an engineered antibody in murine and human systems have implications for therapeutic antibodies. Proc Natl Acad Sci USA. (2006) 103:18709–14. doi: 10.1073/pnas.0606304103
458. Aftimos PG, Barthelemy P, Rolfo CD, Hanssens V, De Jonge N, Silence K, et al. A phase I, first-in-human study of argx-111, a monoclonal antibody targeting c-met in patients with solid tumors. J Clin Oncol. (2015) 33:2580. doi: 10.1200/jco.2015.33.15_suppl.2580
459. Pineda C, Castaneda Hernandez G, Jacobs IA, Alvarez DF, Carini C. Assessing the immunogenicity of biopharmaceuticals. BioDrugs. (2016) 30:195–206. doi: 10.1007/s40259-016-0174-5
460. Carter PJ, Lazar GA. Next generation antibody drugs: pursuit of the ‘high-hanging fruit’. Nat Rev Drug Discov. (2018) 17:197–223. doi: 10.1038/nrd.2017.227
461. Bartelds GM, Krieckaert CL, Nurmohamed MT, van Schouwenburg PA, Lems WF, Twisk JW, et al. Development of antidrug antibodies against adalimumab and association with disease activity and treatment failure during long-term follow-up. JAMA. (2011) 305:1460–8. doi: 10.1001/jama.2011.406
462. Brahmer J, Reckamp KL, Baas P, Crinò L, Eberhardt WE, Poddubskaya E, et al. Nivolumab versus docetaxel in advanced squamous-cell non–small-cell lung cancer. N Engl J Med. (2015) 373:123–35. doi: 10.1056/NEJMoa1504627
463. Herbst RS, Baas P, Kim D-W, Felip E, Pérez-Gracia JL, Han J-Y, et al. Pembrolizumab versus docetaxel for previously treated, PD-L1-positive, advanced non-small-cell lung cancer (KEYNOTE-010): a randomised controlled trial. Lancet. (2016) 387:1540–50. doi: 10.1016/S0140-6736(15)01281-7
464. Motzer RJ, Escudier B, McDermott DF, George S, Hammers HJ, Srinivas S, et al. Nivolumab versus everolimus in advanced renal-cell carcinoma. N Engl J Med. (2015) 373:1803–13. doi: 10.1056/NEJMoa1510665
465. Robert C, Schachter J, Long GV, Arance A, Grob JJ, Mortier L, et al. Pembrolizumab versus ipilimumab in advanced melanoma. N Engl J Med. (2015) 372:2521–32. doi: 10.1056/NEJMoa1503093
466. Rosenberg JE, Hoffman-Censits J, Powles T, Van Der Heijden MS, Balar AV, Necchi A, et al. Atezolizumab in patients with locally advanced and metastatic urothelial carcinoma who have progressed following treatment with platinum-based chemotherapy: a single-arm, multicentre, phase 2 trial. Lancet. (2016) 387:1909–20. doi: 10.1016/S0140-6736(16)00561-4
467. Cassler NM, Merrill D, Bichakjian CK, Brownell I. Merkel cell carcinoma therapeutic update. Curr Treat Opt Oncol. (2016) 17:36. doi: 10.1007/s11864-016-0409-1
468. Alsaab HO, Sau S, Alzhrani R, Tatiparti K, Bhise K, Kashaw SK, et al. PD-1 and PD-L1 checkpoint signaling inhibition for cancer immunotherapy: mechanism, combinations, and clinical outcome. Front Pharmacol. (2017) 8:561. doi: 10.3389/fphar.2017.00561
469. Fehrenbacher L, Spira A, Ballinger M, Kowanetz M, Vansteenkiste J, Mazieres J, et al. Atezolizumab versus docetaxel for patients with previously treated non-small-cell lung cancer (POPLAR): a multicentre, open-label, phase 2 randomised controlled trial. Lancet. (2016) 387:1837–46. doi: 10.1016/S0140-6736(16)00587-0
470. Ribas A, Puzanov I, Dummer R, Schadendorf D, Hamid O, Robert C, et al. Pembrolizumab versus investigator-choice chemotherapy for ipilimumab-refractory melanoma (KEYNOTE-002): a randomised, controlled, phase 2 trial. Lancet Oncol. (2015) 16:908–18. doi: 10.1016/S1470-2045(15)00083-2
471. Gulley JL, Rajan A, Spigel DR, Iannotti N, Chandler J, Wong DJ, et al. Avelumab for patients with previously treated metastatic or recurrent non-small-cell lung cancer (JAVELIN Solid Tumor): dose-expansion cohort of a multicentre, open-label, phase 1b trial. Lancet Oncol. (2017) 18:599–610. doi: 10.1016/S1470-2045(17)30240-1
472. Heery CR, O'Sullivan-Coyne G, Madan RA, Cordes L, Rajan A, Rauckhorst M, et al. Avelumab for metastatic or locally advanced previously treated solid tumours (JAVELIN Solid Tumor): a phase 1a, multicohort, dose-escalation trial. Lancet Oncol. (2017) 18:587–98. doi: 10.1016/S1470-2045(17)30239-5
473. Kelly K, Heery CR, Patel MR, Infante JR, Iannotti N, Leach JW, et al. Avelumab (MSB0010718C; anti-PD-L1) in patients with advanced cancer: safety data from 1300 patients enrolled in the phase 1b JAVELIN Solid Tumor trial. J Clin Oncol. (2016) 34:3055. doi: 10.1200/JCO.2016.34.15_suppl.3055
474. Besse B, Johnson M, Janne P, Garassino M, Eberhardt W, Peters S, et al. 16LBA Phase II, single-arm trial (BIRCH) of atezolizumab as first-line or subsequent therapy for locally advanced or metastatic PD-L1-selected non-small cell lung cancer (NSCLC). Eur J Cancer. (2015) 51:S717–8. doi: 10.1016/S0959-8049(16)31938-4
475. Horn L, Spigel DR, Gettinger SN, Antonia SJ, Gordon MS, Herbst RS, et al. Clinical activity, safety and predictive biomarkers of the engineered antibody MPDL3280A (anti-PDL1) in non-small cell lung cancer (NSCLC): update from a phase Ia study. J Clin Oncol. (2015) 33:8029. doi: 10.1200/jco.2015.33.15_suppl.8029
476. Spigel DR, Chaft JE, Gettinger SN, Chao BH, Dirix LY, Schmid P, et al. Clinical activity and safety from a phase II study (FIR) of MPDL3280A (anti-PDL1) in PD-L1–selected patients with non-small cell lung cancer (NSCLC). J Clin Oncol. (2015) 33:8028. doi: 10.1200/jco.2015.33.15_suppl.8028
477. Petrylak DP, Powles T, Bellmunt J, Braiteh FS, Loriot Y, Cruz Zambrano C, et al. A phase Ia study of MPDL3280A (anti-PDL1): updated response and survival data in urothelial bladder cancer (UBC). J Clin Oncol. (2015) 33:4501. doi: 10.1200/jco.2015.33.15_suppl.4501
478. Lukas RV, Rodon J, Becker K, Wong ET, Shih K, Touat M, et al. Clinical activity and safety of atezolizumab in patients with recurrent glioblastoma. J Neurooncol. (2018) 140:317–28. doi: 10.1007/s11060-018-2955-9
479. McDermott DF, Sosman JA, Sznol M, Massard C, Gordon MS, Hamid O, et al. Atezolizumab, an anti–programmed death-ligand 1 antibody, in metastatic renal cell carcinoma: long-term safety, clinical activity, and immune correlates from a phase Ia study. J Clin Oncol. (2016) 34:833–42. doi: 10.1200/JCO.2015.63.7421
480. Grenga I, Donahue RN, Lepone LM, Richards J, Schlom J. A fully human IgG1 anti-PD-L1 MAb in an in vitro assay enhances antigen-specific T-cell responses. Clin Transl Immunol. (2016) 5:e83. doi: 10.1038/cti.2016.27
481. Boyerinas B, Jochems C, Fantini M, Heery CR, Gulley JL, Tsang KY, et al. Antibody-dependent cellular cytotoxicity activity of a novel anti–PD-L1 antibody avelumab (MSB0010718C) on human tumor cells. Cancer Immunol Res. (2015) 3:1148–57. doi: 10.1158/2326-6066.CIR-15-0059
482. Kaufman HL, Russell J, Hamid O, Bhatia S, Terheyden P, D'Angelo SP, et al. Avelumab in patients with chemotherapy-refractory metastatic Merkel cell carcinoma: a multicentre, single-group, open-label, phase 2 trial. Lancet Oncol. (2016) 17:1374–85. doi: 10.1016/S1470-2045(16)30364-3
483. Kim ES. Tivozanib: first global approval. Drugs. (2017) 77:1917–23. doi: 10.1007/s40265-017-0825-y
484. Aruna G. Immunotoxins: a review of their use in cancer treatment. J Stem Cells Regen Med. (2006) 1:31–6. doi: 10.46582/jsrm.0101005
485. Alderson RF, Kreitman RJ, Chen T, Yeung P, Herbst R, Fox JA, et al. CAT-8015: a second-generation pseudomonas exotoxin A-based immunotherapy targeting CD22-expressing hematologic malignancies. Clin Cancer Res. (2009) 15:832–9. doi: 10.1158/1078-0432.CCR-08-1456
486. Salvatore G, Beers R, Margulies I, Kreitman RJ, Pastan I. Improved cytotoxic activity toward cell lines and fresh leukemia cells of a mutant anti-CD22 immunotoxin obtained by antibody phage display. Clin Cancer Res. (2002) 8:995–1002.
487. Teras LR, DeSantis CE, Cerhan JR, Morton LM, Jemal A, Flowers CR. 2016 US lymphoid malignancy statistics by World Health Organization subtypes. CA Cancer J Clin. (2016) 66:443–59. doi: 10.3322/caac.21357
488. Flandrin G, Sigaux F, Sebahoun G, Bouffette P. Hairy cell leukemia: clinical presentation and follow-up of 211 patients. Semin Oncol. (1984) 11:458–71.
489. Else M, Dearden CE, Matutes E, Garcia-Talavera J, Rohatiner AZ, Johnson SA, et al. Long-term follow-up of 233 patients with hairy cell leukaemia, treated initially with pentostatin or cladribine, at a median of 16 years from diagnosis. Br J Haematol. (2009) 145:733–40. doi: 10.1111/j.1365-2141.2009.07668.x
490. Kreitman RJ, Pastan I. Contextualizing the use of moxetumomab pasudotox in the treatment of relapsed or refractory hairy cell leukemia. Oncologist. (2020) 25:e170–7. doi: 10.1634/theoncologist.2019-0370
491. Schmitz KR, Ferguson KM. Interaction of antibodies with ErbB receptor extracellular regions. Exp Cell Res. (2009) 315:659–70. doi: 10.1016/j.yexcr.2008.10.008
492. Lu D, Zhang H, Koo H, Tonra J, Balderes P, Prewett M, et al. A fully human recombinant IgG-like bispecific antibody to both the epidermal growth factor receptor and the insulin-like growth factor receptor for enhanced antitumor activity. J Biol Chem. (2005) 280:19665–72. doi: 10.1074/jbc.M500815200
493. Normanno N, Bianco C, Strizzi L, Mancino M, Maiello MR, De Luca A, et al. The ErbB receptors and their ligands in cancer: an overview. Curr Drug Targets. (2005) 6:243–57. doi: 10.2174/1389450053765879
494. Seshacharyulu P, Ponnusamy MP, Haridas D, Jain M, Ganti AK, Batra SK. Targeting the EGFR signaling pathway in cancer therapy. Expert Opin Ther Targets. (2012) 16:15–31. doi: 10.1517/14728222.2011.648617
495. Hirsch FR, Dziadziuszko R, Thatcher N, Mann H, Watkins C, Parums DV, et al. Epidermal growth factor receptor immunohistochemistry: comparison of antibodies and cutoff points to predict benefit from gefitinib in a phase 3 placebo-controlled study in advanced nonsmall-cell lung cancer. Cancer. (2008) 112:1114–21. doi: 10.1002/cncr.23282
496. Rusch V, Baselga J, Cordon-Cardo C, Orazem J, Zaman M, Hoda S, et al. Differential expression of the epidermal growth factor receptor and its ligands in primary non-small cell lung cancers and adjacent benign lung. Cancer Res. (1993) 53:2379–85.
497. Fala L. Portrazza (Necitumumab), an IgG1 monoclonal antibody, FDA approved for advanced squamous non-small-cell lung cancer. Am Health Drug Benefits. (2016) 9:119–22.
498. Thatcher N, Hirsch FR, Luft AV, Szczesna A, Ciuleanu TE, Dediu M, et al. Necitumumab plus gemcitabine and cisplatin versus gemcitabine and cisplatin alone as first-line therapy in patients with stage IV squamous non-small-cell lung cancer (SQUIRE): an open-label, randomised, controlled phase 3 trial. Lancet Oncol. (2015) 16:763–74. doi: 10.1016/S1470-2045(15)00021-2
499. Kaymakcalan Z, Sakorafas P, Bose S, Scesney S, Xiong L, Hanzatian DK, et al. Comparisons of affinities, avidities, and complement activation of adalimumab, infliximab, and etanercept in binding to soluble and membrane tumor necrosis factor. Clin Immunol. (2009) 131:308–16. doi: 10.1016/j.clim.2009.01.002
500. Bazzoni F, Beutler B. The tumor necrosis factor ligand and receptor families. N Engl J Med. (1996) 334:1717–25. doi: 10.1056/NEJM199606273342607
501. Kummerfeldt CE. Raxibacumab: potential role in the treatment of inhalational anthrax. Infect Drug Resist. (2014) 7:101–9. doi: 10.2147/IDR.S47305
502. Ascenzi P, Visca P, Ippolito G, Spallarossa A, Bolognesi M, Montecucco C. Anthrax toxin: a tripartite lethal combination. FEBS Lett. (2002) 531:384–8. doi: 10.1016/S0014-5793(02)03609-8
503. Froude JW, Stiles B, Pelat T, Thullier P. Antibodies for biodefense. mAbs. (2011) 3:517–27. doi: 10.4161/mabs.3.6.17621
504. Inglesby TV, O'Toole T, Henderson DA, Bartlett JG, Ascher MS, Eitzen E, et al. Anthrax as a biological weapon, 2002: updated recommendations for management. JAMA. (2002) 287:2236–52. doi: 10.1001/jama.287.17.2236
505. Liu S, Moayeri M, Leppla SH. Anthrax lethal and edema toxins in anthrax pathogenesis. Trends Microbiol. (2014) 22:317–25. doi: 10.1016/j.tim.2014.02.012
506. Migone TS, Subramanian GM, Zhong J, Healey LM, Corey A, Devalaraja M, et al. Raxibacumab for the treatment of inhalational anthrax. N Engl J Med. (2009) 361:135–44. doi: 10.1056/NEJMoa0810603
507. Blair HA, Duggan ST. Belimumab: a review in systemic lupus erythematosus. Drugs. (2018) 78:355–66. doi: 10.1007/s40265-018-0872-z
508. Cancro MP, D'Cruz DP, Khamashta MA. The role of B lymphocyte stimulator (BLyS) in systemic lupus erythematosus. J Clin Invest. (2009) 119:1066–73. doi: 10.1172/JCI38010
509. Gorelik L, Gilbride K, Dobles M, Kalled SL, Zandman D, Scott ML. Normal B cell homeostasis requires B cell activation factor production by radiation-resistant cells. J Exp Med. (2003) 198:937–45. doi: 10.1084/jem.20030789
510. Lavie F, Miceli-Richard C, Quillard J, Roux S, Leclerc P, Mariette X. Expression of BAFF (BLyS) in T cells infiltrating labial salivary glands from patients with Sjogren's syndrome. J Pathol. (2004) 202:496–502. doi: 10.1002/path.1533
511. Nardelli B, Belvedere O, Roschke V, Moore PA, Olsen HS, Migone TS, et al. Synthesis and release of B-lymphocyte stimulator from myeloid cells. Blood. (2001) 97:198–204. doi: 10.1182/blood.V97.1.198
512. Novak AJ, Bram RJ, Kay NE, Jelinek DF. Aberrant expression of B-lymphocyte stimulator by B chronic lymphocytic leukemia cells: a mechanism for survival. Blood. (2002) 100:2973–9. doi: 10.1182/blood-2002-02-0558
513. Scapini P, Nardelli B, Nadali G, Calzetti F, Pizzolo G, Montecucco C, et al. G-CSF-stimulated neutrophils are a prominent source of functional BLyS. J Exp Med. (2003) 197:297–302. doi: 10.1084/jem.20021343
514. Day ES, Cachero TG, Qian F, Sun Y, Wen D, Pelletier M, et al. Selectivity of BAFF/BLyS and APRIL for binding to the TNF family receptors BAFFR/BR3 and BCMA. Biochemistry. (2005) 44:1919–31. doi: 10.1021/bi048227k
515. Cancro MP. The BLyS/BAFF family of ligands and receptors: key targets in the therapy and understanding of autoimmunity. Ann Rheum Dis. (2006) 65:iii34–6. doi: 10.1136/ard.2006.058412
516. Elbirt D, Asher I, Mahlab-Guri K, Bezalel-Rosenberg S, Edelstein V, Sthoeger Z. BLyS levels in sera of patients with systemic lupus erythematosus: clinical and serological correlation. Isr Med Assoc J. (2014) 16:491–6.
517. Jacobi AM, Huang W, Wang T, Freimuth W, Sanz I, Furie R, et al. Effect of long-term belimumab treatment on B cells in systemic lupus erythematosus: extension of a phase II, double-blind, placebo-controlled, dose-ranging study. Arthritis Rheum. (2010) 62:201–10. doi: 10.1002/art.27189
518. Ramos-Casals M, Sanz I, Bosch X, Stone JH, Khamashta MA. B-cell-depleting therapy in systemic lupus erythematosus. Am J Med. (2012) 125:327–36. doi: 10.1016/j.amjmed.2011.09.010
519. Poole RM, Vaidya A. Ramucirumab: first global approval. Drugs. (2014) 74:1047–58. doi: 10.1007/s40265-014-0244-2
520. Smith NR, Baker D, James NH, Ratcliffe K, Jenkins M, Ashton SE, et al. Vascular endothelial growth factor receptors VEGFR-2 and VEGFR-3 are localized primarily to the vasculature in human primary solid cancers. Clin Cancer Res. (2010) 16:3548–61. doi: 10.1158/1078-0432.CCR-09-2797
521. Holzer TR, Fulford AD, Nedderman DM, Umberger TS, Hozak RR, Joshi A, et al. Tumor cell expression of vascular endothelial growth factor receptor 2 is an adverse prognostic factor in patients with squamous cell carcinoma of the lung. PLoS ONE. (2013) 8:e80292. doi: 10.1371/journal.pone.0080292
522. Casak SJ, Fashoyin-Aje I, Lemery SJ, Zhang L, Jin R, Li H, et al. FDA approval summary: ramucirumab for gastric cancer. Clin Cancer Res. (2015) 21:3372–6. doi: 10.1158/1078-0432.CCR-15-0600
523. Fala L. Cyramza (Ramucirumab) approved for the treatment of advanced gastric cancer and metastatic non-small-cell lung cancer. Am Health Drug Benefits. (2015) 8:49–53.
524. Lee JJ, Sun W. Options for second-line treatment in metastatic colorectal cancer. Clin Adv Hematol Oncol. (2016) 14:46–54.
525. Syed YY. Ramucirumab: a review in hepatocellular carcinoma. Drugs. (2020) 80:315–22. doi: 10.1007/s40265-020-01263-6
526. Nogueira M, Torres T. Guselkumab for the treatment of psoriasis–evidence to date. Drugs Context. (2019) 8:212594. doi: 10.7573/dic.212594
527. Shimozato O, Ugai S, Chiyo M, Takenobu H, Nagakawa H, Wada A, et al. The secreted form of the p40 subunit of interleukin (IL)-12 inhibits IL-23 functions and abrogates IL-23-mediated antitumour effects. Immunology. (2006) 117:22–8. doi: 10.1111/j.1365-2567.2005.02257.x
528. Tang C, Chen S, Qian H, Huang W. Interleukin-23: as a drug target for autoimmune inflammatory diseases. Immunology. (2012) 135:112–24. doi: 10.1111/j.1365-2567.2011.03522.x
529. Puig L. The role of IL 23 in the treatment of psoriasis. Expert Rev Clin Immunol. (2017) 13:525–34. doi: 10.1080/1744666X.2017.1292137
530. Kryczek I, Zhao E, Liu Y, Wang Y, Vatan L, Szeliga W, et al. Human TH17 cells are long-lived effector memory cells. Sci Transl Med. (2011) 3:104ra0. doi: 10.1126/scitranslmed.3002949
531. Deodhar A, Gottlieb AB, Boehncke WH, Dong B, Wang Y, Zhuang Y, et al. Efficacy and safety of guselkumab in patients with active psoriatic arthritis: a randomised, double-blind, placebo-controlled, phase 2 study. Lancet. (2018) 391:2213–24. doi: 10.1016/S0140-6736(18)30952-8
532. Deodhar A, Helliwell PS, Boehncke WH, Kollmeier AP, Hsia EC, Subramanian RA, et al. Guselkumab in patients with active psoriatic arthritis who were biologic-naive or had previously received TNFalpha inhibitor treatment (DISCOVER-1): a double-blind, randomised, placebo-controlled phase 3 trial. Lancet. (2020) 395:1115–25. doi: 10.1016/S0140-6736(20)30265-8
533. McHugh J. IL-23 inhibitor guselkumab shows promise for PsA. Nat Rev Rheumatol. (2020) 16:247. doi: 10.1038/s41584-020-0420-6
534. Wu Y. Contact pathway of coagulation and inflammation. Thromb J. (2015) 13:17. doi: 10.1186/s12959-015-0048-y
535. Colman RW, Schmaier AH. Contact system: a vascular biology modulator with anticoagulant, profibrinolytic, antiadhesive, and proinflammatory attributes. Blood. (1997) 90:3819–43. doi: 10.1182/blood.V90.10.3819
536. Suffritti C, Zanichelli A, Maggioni L, Bonanni E, Cugno M, Cicardi M. High-molecular-weight kininogen cleavage correlates with disease states in the bradykinin-mediated angioedema due to hereditary C1-inhibitor deficiency. Clin Exp Allergy. (2014) 44:1503–14. doi: 10.1111/cea.12293
537. Hornig B, Kohler C, Drexler H. Role of bradykinin in mediating vascular effects of angiotensin-converting enzyme inhibitors in humans. Circulation. (1997) 95:1115–8. doi: 10.1161/01.CIR.95.5.1115
538. Kaplan AP, Joseph K. Pathogenesis of hereditary angioedema: the role of the bradykinin-forming cascade. Immunol Allergy Clin North Am. (2017) 37:513–25. doi: 10.1016/j.iac.2017.04.001
539. Pappalardo E, Caccia S, Suffritti C, Tordai A, Zingale LC, Cicardi M. Mutation screening of C1 inhibitor gene in 108 unrelated families with hereditary angioedema: functional and structural correlates. Mol Immunol. (2008) 45:3536–44. doi: 10.1016/j.molimm.2008.05.007
540. Dewald G, Bork K. Missense mutations in the coagulation factor XII (Hageman factor) gene in hereditary angioedema with normal C1 inhibitor. Biochem Biophys Res Commun. (2006) 343:1286–9. doi: 10.1016/j.bbrc.2006.03.092
541. Chyung Y, Vince B, Iarrobino R, Sexton D, Kenniston J, Faucette R, et al. A phase 1 study investigating DX-2930 in healthy subjects. Ann Allergy Asthma Immunol. (2014) 113:460–6 e2. doi: 10.1016/j.anai.2014.05.028
542. Banerji A, Busse P, Shennak M, Lumry W, Davis-Lorton M, Wedner HJ, et al. Inhibiting plasma kallikrein for hereditary angioedema prophylaxis. N Engl J Med. (2017) 376:717–28. doi: 10.1056/NEJMoa1605767
543. Cua DJ, Tato CM. Innate IL-17-producing cells: the sentinels of the immune system. Nat Rev Immunol. (2010) 10:479–89. doi: 10.1038/nri2800
544. Harrington LE, Hatton RD, Mangan PR, Turner H, Murphy TL, Murphy KM, et al. Interleukin 17-producing CD4+ effector T cells develop via a lineage distinct from the T helper type 1 and 2 lineages. Nat Immunol. (2005) 6:1123–32. doi: 10.1038/ni1254
545. Kara EE, Comerford I, Fenix KA, Bastow CR, Gregor CE, McKenzie DR, et al. Tailored immune responses: novel effector helper T cell subsets in protective immunity. PLoS Pathog. (2014) 10:e1003905. doi: 10.1371/journal.ppat.1003905
546. Martin DA, Towne JE, Kricorian G, Klekotka P, Gudjonsson JE, Krueger JG, et al. The emerging role of IL-17 in the pathogenesis of psoriasis: preclinical and clinical findings. J Invest Dermatol. (2013) 133:17–26. doi: 10.1038/jid.2012.194
547. Sankowski AJ, Lebkowska UM, Cwikla J, Walecka I, Walecki J. Psoriatic arthritis. Pol J Radiol. (2013) 78:7–17. doi: 10.12659/PJR.883763
548. van der Fits L, Mourits S, Voerman JS, Kant M, Boon L, Laman JD, et al. Imiquimod-induced psoriasis-like skin inflammation in mice is mediated via the IL-23/IL-17 axis. J Immunol. (2009) 182:5836–45. doi: 10.4049/jimmunol.0802999
549. Sagoo GS, Tazi-Ahnini R, Barker JW, Elder JT, Nair RP, Samuelsson L, et al. Meta-analysis of genome-wide studies of psoriasis susceptibility reveals linkage to chromosomes 6p21 and 4q28-q31 in Caucasian and Chinese Hans population. J Invest Dermatol. (2004) 122:1401–5. doi: 10.1111/j.0022-202X.2004.22607.x
550. International Psoriasis Genetics C. The International Psoriasis Genetics Study: assessing linkage to 14 candidate susceptibility loci in a cohort of 942 affected sib pairs. Am J Hum Genet. (2003) 73:430–7. doi: 10.1086/377159
551. Eder L, Law T, Chandran V, Shanmugarajah S, Shen H, Rosen CF, et al. Association between environmental factors and onset of psoriatic arthritis in patients with psoriasis. Arthritis Care Res (Hoboken). (2011) 63:1091–7. doi: 10.1002/acr.20496
552. Blauvelt A, Chiricozzi A. The immunologic role of IL-17 in psoriasis and psoriatic arthritis pathogenesis. Clin Rev Allergy Immunol. (2018) 55:379–90. doi: 10.1007/s12016-018-8702-3
553. Dougados M, Wei JC, Landewe R, Sieper J, Baraliakos X, Van den Bosch F, et al. Efficacy and safety of ixekizumab through 52 weeks in two phase 3, randomised, controlled clinical trials in patients with active radiographic axial spondyloarthritis (COAST-V and COAST-W). Ann Rheum Dis. (2020) 79:176–85. doi: 10.1136/annrheumdis-2019-216118
554. Langley RG, Kimball AB, Nak H, Xu W, Pangallo B, Osuntokun OO, et al. Long-term safety profile of ixekizumab in patients with moderate-to-severe plaque psoriasis: an integrated analysis from 11 clinical trials. J Eur Acad Dermatol Venereol. (2019) 33:333–9. doi: 10.1111/jdv.15242
555. Papadopoulos N, Martin J, Ruan Q, Rafique A, Rosconi MP, Shi E, et al. Binding and neutralization of vascular endothelial growth factor (VEGF) and related ligands by VEGF Trap, ranibizumab and bevacizumab. Angiogenesis. (2012) 15:171–85. doi: 10.1007/s10456-011-9249-6
556. Shibuya M. Vascular endothelial growth factor (VEGF) and its receptor (VEGFR) signaling in angiogenesis: a crucial target for anti- and pro-angiogenic therapies. Genes Cancer. (2011) 2:1097–105. doi: 10.1177/1947601911423031
557. Ma IT, McConaghy S, Namachivayam K, Halloran BA, Kurundkar AR, MohanKumar K. VEGF mRNA and protein concentrations in the developing human eye. Pediatr Res. (2015) 77:500–5. doi: 10.1038/pr.2015.15
558. Gaudreault J, Fei D, Beyer JC, Ryan A, Rangell L, Shiu V, et al. Pharmacokinetics and retinal distribution of ranibizumab, a humanized antibody fragment directed against VEGF-A, following intravitreal administration in rabbits. Retina. (2007) 27:1260–6. doi: 10.1097/IAE.0b013e318134eecd
559. Bakri SJ, Snyder MR, Reid JM, Pulido JS, Ezzat MK, Singh RJ. Pharmacokinetics of intravitreal ranibizumab (Lucentis). Ophthalmology. (2007) 114:2179–82. doi: 10.1016/j.ophtha.2007.09.012
560. Kremer Hovinga JA, Coppo P, Lammle B, Moake JL, Miyata T, Vanhoorelbeke K. Thrombotic thrombocytopenic purpura. Nat Rev Dis Primers. (2017) 3:17020. doi: 10.1038/nrdp.2017.20
561. Peyvandi F, Ferrari S, Lavoretano S, Canciani MT, Mannucci PM. von Willebrand factor cleaving protease (ADAMTS-13) and ADAMTS-13 neutralizing autoantibodies in 100 patients with thrombotic thrombocytopenic purpura. Br J Haematol. (2004) 127:433–9. doi: 10.1111/j.1365-2141.2004.05217.x
562. Peyvandi F, Scully M, Kremer Hovinga JA, Cataland S, Knobl P, Wu H, et al. Caplacizumab for acquired thrombotic thrombocytopenic purpura. N Engl J Med. (2016) 374:511–22. doi: 10.1056/NEJMoa1505533
563. Vallurupalli M, Berliner N. Emapalumab for the treatment of relapsed/refractory hemophagocytic lymphohistiocytosis. Blood. (2019) 134:1783–6. doi: 10.1182/blood.2019002289
564. Das R, Guan P, Sprague L, Verbist K, Tedrick P, An QA, et al. Janus kinase inhibition lessens inflammation and ameliorates disease in murine models of hemophagocytic lymphohistiocytosis. Blood. (2016) 127:1666–75. doi: 10.1182/blood-2015-12-684399
565. Lounder DT, Bin Q, de Min C, Jordan MB. Treatment of refractory hemophagocytic lymphohistiocytosis with emapalumab despite severe concurrent infections. Blood Adv. (2019) 3:47–50. doi: 10.1182/bloodadvances.2018025858
566. Henter JI, Samuelsson-Horne A, Arico M, Egeler RM, Elinder G, Filipovich AH, et al. Treatment of hemophagocytic lymphohistiocytosis with HLH-94 immunochemotherapy and bone marrow transplantation. Blood. (2002) 100:2367–73. doi: 10.1182/blood-2002-01-0172
567. Farquhar JW, Claireaux AE. Familial haemophagocytic reticulosis. Arch Dis Child. (1952) 27:519–25. doi: 10.1136/adc.27.136.519
568. The 45th annual meeting of the European Society for blood and marrow transplantation: physicians–oral session. Bone Marrow Transplant. (2019) 54:16–141. doi: 10.1038/s41409-019-0562-9
Keywords: monoclonal antibodies, phage display, antibody libraries, biopanning, biopharmaceuticals
Citation: Alfaleh MA, Alsaab HO, Mahmoud AB, Alkayyal AA, Jones ML, Mahler SM and Hashem AM (2020) Phage Display Derived Monoclonal Antibodies: From Bench to Bedside. Front. Immunol. 11:1986. doi: 10.3389/fimmu.2020.01986
Received: 29 May 2020; Accepted: 23 July 2020;
Published: 28 August 2020.
Edited by:
Rajko Reljic, St. George's, University of London, United KingdomReviewed by:
Victor Greiff, University of Oslo, NorwayFatima Ferreira, University of Salzburg, Austria
Copyright © 2020 Alfaleh, Alsaab, Mahmoud, Alkayyal, Jones, Mahler and Hashem. This is an open-access article distributed under the terms of the Creative Commons Attribution License (CC BY). The use, distribution or reproduction in other forums is permitted, provided the original author(s) and the copyright owner(s) are credited and that the original publication in this journal is cited, in accordance with accepted academic practice. No use, distribution or reproduction is permitted which does not comply with these terms.
*Correspondence: Anwar M. Hashem, YW1oYXNoZW1Aa2F1LmVkdS5zYQ==