- School of Animal and Veterinary Sciences, The University of Adelaide, Roseworthy, SA, Australia
Members of Salmonella enterica are frequently involved in egg and egg product related human food poisoning outbreaks worldwide. In Australia, Salmonella Typhimurium is frequently involved in egg and egg product related foodborne illness and Salmonella Mbandaka has also been found to be a contaminant of the layer farm environment. The ability possessed by Salmonella Enteritidis to colonize reproductive organs and contaminate developing eggs has been well-described. However, there are few studies investigating this ability for Salmonella Typhimurium. The hypothesis of this study was that the Salmonella Typhimurium can colonize the gut for a prolonged period of time and that horizontal infection through feces is the main route of egg contamination. At 14 weeks of age hens were orally infected with either S. Typhimurium PT 9 or S. Typhimurium PT 9 and Salmonella Mbandaka. Salmonella shedding in feces and eggs was monitored for 15 weeks post-infection. Egg shell surface and internal contents of eggs laid by infected hens were cultured independently for detection of Salmonella spp. The mean Salmonella load in feces ranged from 1.54 to 63.35 and 0.31 to 98.38 most probable number/g (MPN/g) in the S. Typhimurium and S. Typhimurium + S. Mbandaka group, respectively. No correlation was found between mean fecal Salmonella load and frequency of egg shell contamination. Egg shell contamination was higher in S. Typhimurium + S. Mbandaka infected group (7.2% S. Typhimurium, 14.1% S. Mbandaka) compared to birds infected with S. Typhimurium (5.66%) however, co-infection had no significant impact on egg contamination by S. Typhimurium. Throughout the study Salmonella was not recovered from internal contents of eggs laid by hens. Salmonella was isolated from different segments of oviduct of hens from both the groups, however pathology was not observed on microscopic examination. This study investigated Salmonella shedding for up to 15 weeks p.i which is a longer period of time compared to previously published studies. The findings of current study demonstrated intermittent but persistent fecal shedding of Salmonella after oral infection for up to 15 weeks p.i. Further, egg shell contamination, with lack of internal egg content contamination and the low frequency of reproductive organ infection suggested that horizontal infection through contaminated feces is the main route of egg contamination with S. Typhimurium in laying hens.
Introduction
Foodborne gastric infections due to Salmonella enterica are of major concern worldwide. Typically contaminated eggs and egg related products are primary vehicles for human salmonellosis. Globally, S. Enteritidis represents a dominant serotype in commercial poultry isolated from eggs and is frequently involved in egg related food poisoning in humans (Foley et al., 2011). S. Enteritidis, however, is not endemic in Australian poultry flocks (OzFoodNet Working Group, 2009). This niche has been filled by S. Typhimurium, which is a leading cause of foodborne outbreaks linked to contaminated egg and egg related products (OzFoodNet Working Group, 2009). In 2010, S. Typhimurium was the most commonly notified Salmonella serotype accounting for 5241 (44%) cases of all Salmonella notified infections in Australia (OzFoodNet Working Group, 2012).
The external and internal egg contamination by Salmonella during poultry production is a complex issue, influenced by many variables. As a result, implementation of appropriate control measures is extremely difficult (Whiley and Ross, 2015). Egg contamination can occur by two routes, vertical or horizontal. Vertical transmission is a result of reproductive organ colonization (ovary and oviduct) before shell formation, whereas horizontal transmission occurs due to external egg shell contamination (De Reu et al., 2006).
Oral challenge of both S. Enteritidis and S. Typhimurium has the potential to invade the reproductive organs. However, only S. Enteritidis has been recovered from egg contents (Keller et al., 1997; Okamura et al., 2001a; Gast et al., 2004, 2007, 2013; Gantois et al., 2008). The intrinsic properties and resistance to antibacterial compounds enabling S. Enteritidis to colonize the oviduct and contaminate egg internal contents are well-known (Gantois et al., 2009). There is, however, limited information on the long term shedding, colonization of reproductive organs and egg contamination by S. Typhimurium.
Previous studies have examined reproductive organ colonization and egg contamination by S. Typhimurium in laying hens. Results from these experiments, however, are inconsistent due to variation in experimental design, route of inoculation, inoculum dose as well as the strain of S. Typhimurium selected (Baker et al., 1980; Williams et al., 1998; Leach et al., 1999; Okamura et al., 2001a,b, 2010). Moreover, the majority of these previous studies examined the capability of S. Typhimurium to colonize reproductive organs and/or egg contamination frequency up to 3 weeks post-infection, which could fail to unveil the ability of S. Typhimurium to cause egg contamination over a prolonged period (Wales and Davies, 2011). Altogether, there is a lack of published data arising from long term experiments aimed at fecal shedding, reproductive organ colonization and egg contamination by S. Typhimurium in laying hens.
On commercial layer farms environmental contamination with multiple Salmonella serovars is common and represents a serious concern for poultry industries world-wide (Gole et al., 2014c; Im et al., 2015). A recent epidemiological survey examining the prevalence of Salmonella spp. on layer farms demonstrated that S. Mbandaka (54.40%, 68/125) was the most frequently recovered serovar along with S. Typhimurium (11.54%, 15/130) (Gole et al., 2014a,c). S. Mbandaka has also been isolated from egg shell, animals, feed, and sporadic cases of human salmonellosis (Hoszowski and Wasyl, 2001; Little et al., 2007; Im et al., 2015). Given the diversity of poultry associated Salmonella serovars, there are few reports on how the presence of commonly isolated serovars from layer farm environments (such as S. Mbandaka) might influence the shedding patterns of S. Typhimurium. In addition, how two Salmonella serovars have an effect upon organ invasion and egg contamination in vivo is still unclear.
Given the potential public health threat by S. Typhimurium associated with consumption of contaminated egg and egg products, this study sought to investigate the dynamics of egg contamination over an extended time course. In this study the duration of fecal shedding, its relation to frequency of egg contamination and reproductive organ colonization after oral infection with S. Typhimurium alone and in combination with S. Mbandaka was investigated in commercial layer hens. To our knowledge, this is a first report of a Salmonella oral challenge model conducted in controlled environment employing strict biosecurity measures for up to 30 weeks of age.
Materials and Methods
Experimental Animals
Fertile eggs were obtained from a commercial layer parent flock. Eggs were fumigated using formaldehyde as previously described (Samberg and Meroz, 1995) and incubated for 21 days at 37.7°C. Relative humidity was maintained at 45–55% until day 18 and increased to 55–65% up to hatching. A total of 32 birds were hatched, raised in pens until week 10 and then shifted in cages contained within positive pressure rooms at Roseworthy Campus of The University of Adelaide, until the end of experiment (week 30).
Sample size for this study was calculated using Openepi-Tool (Dean et al., 2011). This tool along with the sample size determines the power of the experimental trial. For sample size calculation, assumed percent with outcome in S. Typhimurium and S. Typhimurium + S. Mbandaka infected group was 20% and 70% respectively with the confidence interval of 95%. This gave an 80% chance of detecting differences between treatment groups with normal approximation.
Prior to experiments all animal rooms and equipment were fumigated with formaldehyde and cleaned with commercial disinfectants (Chemtel, Australia). Throughout the experiment, feed was sterilized by fumigation (Samberg and Meroz, 1995) and water purification tablets (Aquatabs, Ireland) were added to drinking water. Feed and water was provided ad libitum. The recommended lighting program specified in the commercial management guide of Hy-Line Australia Pty Ltd was followed in this study. Feces, feed, and water samples were tested at fortnightly intervals for detection of Salmonella spp. by the culture method as described previously (Gole et al., 2014a). All experiments were conducted according to the protocol approved by the institutional animal ethics committee of The University of Adelaide (Protocol No. S-2014-008) and in compliance with the Australian code for the care and use of animals for scientific purposes.
Bacterial Strains, Culture, and Inoculum Preparation
Salmonella isolates used for oral infection in this study were recovered previously from layer hen fecal samples (Gole et al., 2014a,c). S. Typhimurium PT 9 has been frequently implicated in egg product related human Salmonellosis in Australia (OzFoodNet Working Group, 2009, 2012). Hence, this strain was selected. The antimicrobial resistance profile of Salmonella isolates was characterized earlier (Pande et al., 2015). S. Typhimurium PT 9 isolate used in this study was resistant to amoxicillin, ampicillin, and tetracycline. This isolate was susceptible to trimethoprim, cefotaxime, cephalothin, chloramphenicol, gentamycin, neomycin, and streptomycin. On other hand, S. Mbandaka isolate used in this study was resistant to amoxicillin, ampicillin, and trimethoprim and susceptible to cefotaxime, cephalothin, chloramphenicol, gentamycin, neomycin, streptomycin, and tetracycline (Pande et al., 2015).
For oral inoculation, stocks of bacterial strains were cultured overnight at 37°C on nutrient agar. Twenty-four hours prior to infection, a single colony of each Salmonella strain was added to a separate tube containing 5 ml of Luria Bertani (LB) broth (Oxoid, Australia) and incubated 6 h with shaking (110 rpm). From this LB culture, 10 μl was transferred to 5 ml of LB and grown overnight at 37°C with shaking. Bacterial suspensions were diluted to 109 bacteria per ml for oral inoculation. Bacterial cell counts (CFU) were determined by plating 10-fold serial dilutions of the inoculum on nutrient agar to confirm dose.
Experimental Design
At week 10 after hatch, birds were divided in three treatment groups and housed in separate rooms in individual cages. At the age of 14 weeks, birds were orally challenged with either 109 CFU of S. Typhimurium PT 9 (T group, n = 14) or 109 CFU of S. Typhimurium PT 9 and S. Mbandaka (TM group, n = 14). Control birds (C group, n = 4) received only sterile LB broth. Following infection, all experimental birds were monitored twice a day for clinical signs of infection. All hens were humanely euthanized at the age of week 30. Ovary and segments of the oviduct (infundibulum, magnum, isthmus, uterus (shell gland) and vagina) were removed aseptically and processed for bacteriological and histopathological analysis. Throughout the study, all eggs laid (n = 1004) during 5, 7, 9, 11, 13, 15 weeks post-infection (p.i.) were tested for presence of Salmonella spp.
Enumeration and Isolation of Salmonella from Feces
Fecal samples were aseptically collected from individual hens in Whirl- Pack plastic bags (Thermo Fisher Scientific, Australia) on days 0, 1, 3, 6, 9, and 12 followed by weeks 3, 5, 7, 9, 11, 13, and 15 p.i.
Fecal samples were processed for enumeration of Salmonella by three tube most probable number (MPN) method (Santos et al., 2005; Pavic et al., 2010). Briefly, 10 g of fecal sample were weighed in sterile Whirl-Pack plastic bag (Thermo scientific, Australia) followed by the addition of 90 ml Buffered peptone water (BPW, (Oxoid, Australia) (1:10); bags were then homogenized for 1 min. From this bag 10 ml of homogenate was placed into three different sterile tubes (100 dilution). Then 1 ml of homogenate sample was transferred to three different tubes containing 9 ml of BPW, and then serially diluted in triplicate tubes of BPW. The tubes were incubated overnight at 37°C. After incubation, 10 μl of BPW from each MPN tube was plated on modified semisolid Rappaport–Vassiliadis (MSRV, Oxoid, Australia) agar plates and incubated at 42°C for 24 h. A loopful of media from the leading edge of white zones from MRSV plate was streaked onto XLD and or Salmonella Brilliance agar plates (Oxoid, Australia) for confirmation of Salmonella.
Bacteriological Analysis of Egg Shell and Internal Contents
Eggs from both control and Salmonella infected hens were collected aseptically in individual Whirl-Pack plastic bags. Each egg was processed for the presence of Salmonella on the egg shell and in the internal contents. Briefly, an individual egg was immersed in 10 ml of BPW in Whirl- Pack plastic bag, massaged for 2 min and then removed. The egg shell rinse was then processed for Salmonella isolation as previously described (Gole et al., 2014a). Each egg was dipped in 70% ethanol for 2 min to prevent internal content contamination from the egg shell surface. Each egg was then broken aseptically and contents emptied into a Whirl-Pack plastic bag. The egg contents were homogenized thoroughly. Five ml of internal egg contents were mixed with 45 ml of BPW (1:10) and incubated at 37°C overnight. Salmonella enrichment and isolation from egg shell and internal content samples was carried out as described previously (Gole et al., 2014a). Salmonella positive egg shell wash enriched in Rappaport-Vassiliadis (RVS) broth was stored in 20% glycerol at −80°C for further PCR testing.
Bacteriological Analysis of Reproductive Organs
Samples (0.1–0.2 g) of the ovary, infundibulum, magnum, isthmus, uterus (shell gland), and vagina were collected in sterile tubes. The tissue samples were homogenized using a Bullet Blender®(Next Advance Inc. USA) at full speed for 2 min and serial 10-fold dilutions were prepared in phosphate buffer saline (PBS). From each dilution 100 μl was spread directly onto XLD agar plates (Oxoid, Australia) and incubated overnight at 37°C. After 24 h the number of colonies was enumerated and concentration of Salmonella in tissues was expressed as mean log10 CFU/g of tissue.
DNA Extractions from Fecal Samples, Egg Shell Wash, and Reproductive Organs
DNA was extracted from all fecal samples of control, T and TM groups using QIAamp DNA Stool Mini Kit (Qiagen, Australia) according to manufacturer instructions. DNA extraction from all Salmonella isolates recovered from egg shell washes of T and TM hens was performed as previously described (Pande et al., 2015). Briefly, the frozen stock of RVS broth was thawed and 50 μl of broth was mixed with 450 μl of LB broth and incubated overnight at 37°C. One hundred microliter of overnight bacterial culture was mixed to 1 ml of sterile water and centrifuged at 14,000 g for 2 min. After decanting the supernatant, the bacterial pellet was re-suspended in 200 μl of 6% Chelex® (Bio-Rad, Sydney, NSW, Australia) prepared in TE (10 mM Tris and 1 mM EDTA). Tubes were incubated at 56°C for 20 min, vortexed and further incubated at 100°C for 8 min. Samples were placed on ice for 5 min and centrifuged at 14,000 g for 10 min. Supernatants were recovered from each sample and used as a DNA template for PCR.
DNA was extracted from reproductive organs using DNeasy Blood & Tissue Kit (Qiagen, Australia) as per manufacturer instructions.
PCR Detection of S. Typhimurium and S. Mbandaka
Salmonella positive egg shell wash samples from T and TM group, all fecal samples and culture positive reproductive organs from T and TM groups were screened for Salmonella specific invA gene and S. Typhimurium serovar specific genomic region TSR3 (Akiba et al., 2011) by multiplex PCR to detect S. Typhimurium PT9. TSR3 gene was not amplified in S. Mbandaka isolates (Akiba et al., 2011). Further, to differentiate S. Mbandaka from S. Typhimurium PT 9 in the TM group, DNA extracted from feces, egg shell wash and reproductive organs were tested for dhfrV gene that confers resistance to trimethoprim (Pande et al., 2015). Samples from T infected group were also tested for dhfrV gene. S. Typhimurium PT9 used in this study was sensitive to trimethoprim and negative for dhfrV gene (Pande et al., 2015). PCR reactions for invA and TSR3 gene were performed in a total reaction volume of 20 μl including 2 μl DNA template. PCR reaction mixture consisted of final concentration of 1.5 mM MgCl2, 2.5 μM of each dNTP (Bioline, Australia), 0.5 μM each forward and reverse primer and 2.5 U of Taq polymerase (Bioline, Australia). DNA amplification was carried out in T100 thermal cycler (Bio-Rad, Australia) using the following protocol: 2 min initial denaturation at 94°C, following 30 cycles of denaturation at 95°C for 30 s, annealing at 60°C for 30 s, extension at 68°C for 30 s and a final extension at 72°C for 5 min.
PCR reactions for dhfrV gene were performed in a total reaction volume of 25 μl including 2 μl DNA templates. Each PCR reaction mixture consisted of final concentration of 1.5 mM MgCl2, 2.5 μM of each dNTP (Bioline, Australia), 0.28 μM of each primer and 2.5U of Taq polymerase (Bioline, Australia) using the following PCR cycle conditions: 2 min initial denaturation at 95°C, following 30 cycles of denaturation at 94°C for 30 s, annealing at 64°C for 30 s, extension at 72°C for 30 s, and a final extension at 72°C for 5 min.
PCR products were electrophoresed at 60 V for 1.5 h on 1.5% agarose gel in 0.5X Tris borate EDTA buffer and stained with GelRed™ nucleic acid gel stain (Biotium, USA). The size of PCR products was determined by comparing with standard 100 bp ladders (Thermo Fisher, Australia). Negative and positive controls were used in each PCR reaction for all the samples.
In order to investigate the detection limit of S. Typhimurium by multiplex PCR, Salmonella negative fecal samples were spiked with S. Typhimurium or S. Typhimurium + S. Mbandaka at doses ranging from 101 to 109 CFU/ml. Following DNA extractions from spiked samples using QIAamp DNA Stool Mini Kit (Qiagen, Australia), multiplex PCR was performed as described above.
Histopathology of Reproductive Organs
Infundibulum, magnum, isthmus, uterus, and vagina were collected individually to evaluate histomorphological alterations in response to Salmonella infection. Tissue samples of reproductive organs were fixed in 10% neutral buffered formalin, embedded in paraffin wax and 5 μm sections were stained with Haematoxylin and Eosin stain (H &E).
Statistical Analysis
Significant differences between groups in the isolation rate of Salmonella from feces and eggs were determined by Fisher's exact probability test. MPN data was analyzed by two way analysis of variance. The relationship between recovery of Salmonella (MPN/g) from feces and isolation of Salmonella from egg shell was determined by Pearson correlation test (R2-value). All data generated in this study was analyzed statistically either using GraphPad Prism version 6 software or IBM®SPSS Statistics® version 21. P < 0.05 were considered statistically significant.
Results
Clinical Symptoms and Mortality
During the first week p.i., mucoid and blood tinged feces were observed in two birds from each treatment group. No mortality was recorded in any of the treatment groups throughout this study.
Fecal Shedding of Salmonella at Different p.i. Intervals
All fecal, water and feed samples collected from experimental birds before oral challenge were negative for Salmonella spp. The number of Salmonella positive fecal samples for both T (S. Typhimurium) and TM (S. Typhimurium and S. Mbandaka) groups over the course of the experiment is presented in Table 1. No significant difference (p = 0.848) was observed in number of fecal samples positive for Salmonella between T (152/168, 90.47%) and TM groups (154/168, 91.66%). There were more fecal positive samples until week 5 p.i.
An overall decline after week 5 in the number of birds shedding Salmonella in feces was observed in both groups. Overall, persistent Salmonella shedding in feces was observed in both groups throughout the experimental period after oral infection. Salmonella spp. was not isolated from any bird in the control group (data not shown).
Enumeration of Salmonella from Feces by MPN Method
The viable counts of Salmonella (MPN/g) in feces over the course of the experiment are presented in Figure 1. Throughout the experimental period viable counts of Salmonella were detected in the feces with a mean frequency ranging from 1.54 to 63.35 and 0.31 to 98.38 MPN/g in T and TM groups, respectively. The mean Salmonella load peaked at week 5 p.i. and thereafter, a decline in the viable Salmonella load was observed in both groups (Figure 1). Mean Salmonella counts were variable between days p.i. and group over the course of the experiment. Mean load of Salmonella was significantly higher (p = 0.0001) in the TM group compared to the T group at day 12 p.i. Variables such as group and days p.i. revealed significant differences in viable Salmonella count recovered from feces of orally infected birds (p = 0.0004).
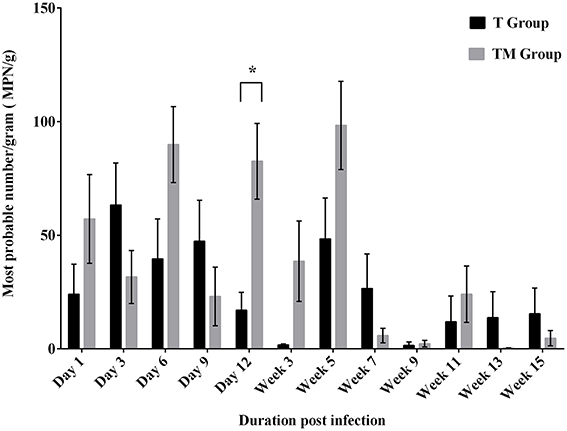
Figure 1. Enumeration of Salmonella (MPN/g) from feces of birds orally infected with 109 CFU of S. Typhimurium (T group) or S. Typhimurium + S. Mbandaka (TM group). Values are Mean ± SEM. Comparison between group marked with an asterisk (*) is significantly different at p < 0.05 based on ANOVA.
Analysis of Salmonella from Egg Shell
All eggs tested (n = 136) from control hens were negative for Salmonella. The frequency of egg shell contamination after oral infection ranged from 0 to 16.67 and 16.67 to 21.11% in T and TM group, respectively. Overall the frequency of egg shell contamination was significantly higher (p = 0.001) in the TM group (18.69%, 83/444) as compared to the T group (5.66%, 24/424) (Table 2).
In order to determine the effect of co-infection (TM group) on the recovery rate of S. Typhimurium on egg shell surface, multiplex PCR that specifically differentiates S. Typhimurium from S. Mbandaka was carried out. Overall the frequency of recovery of S. Typhimurium from egg shells of TM group (7.20%, 32/444) did not differ significantly from T group (5.66%, 24/424). PCR results indicated that overall, 14.1% (63/444) egg shell samples were positive for S. Mbandaka (Table 2). Correlation between Salmonella shedding in feces (MPN/g) and subsequent egg shell contamination was analyzed using a Pearson correlation test. No correlation was evident between mean fecal Salmonella load and observed frequency of contaminated eggs laid by orally infected birds of T and TM group (p = 0.624, R2 = 0.002 T group; p = 0.177, R2 = 0.022 TM group). Fecal Shedding and egg contamination data per bird/egg over time is presented in Supplementary Table 1. In TM group, Salmonella shedding in feces and eggs was variable in individual birds across 15 weeks p.i.
Comparison between Culture and PCR Based Detection of S. Typhimurium
The sensitivity of multiplex PCR for invA and TSR3 gene to detect S. Typhimurium was determined by spiking fecal samples with various doses of S. Typhimurium or S. Typhimurium + S. Mbandaka. The PCR detection limit for S. Typhimurium was 102 CFU/reaction whereas it was 104 CFU/reaction when fecal samples were spiked with both S. Typhimurium and S. Mbandaka. The PCR detection limit for dhfrV gene to detect S. Mbandaka was 104 CFU/reaction, when fecal samples were spiked with both S. Typhimurium and S. Mbandaka. The details of fecal and egg shell samples positive and negative for Salmonella at different days p.i. by culture and PCR method are described in Tables 1, 2. Fecal samples from T infected group tested negative for dhfrV gene. Overall, S. Typhimurium was detected in 133/168 (79.16%) fecal samples and 32/444 (7.20%) egg shell samples in TM group. Similarly, S. Mbandaka was detected in 121/168 (72.02%) fecal samples and 63/444 (14.18%) egg shell samples in TM group.
Analysis of Salmonella from Internal Egg Contents
Over the course of the experiment, Salmonella was not isolated from the internal content of eggs (n = 1004) laid by either control or infected hens.
Bacteriological and Histopathological Analysis of Reproductive Organs
The recovery rate of Salmonella from reproductive organs is summarized in Table 3. Colonization of Salmonella in reproductive organs of laying hens after oral infection was observed in both groups. In the T group birds Salmonella was recovered from different segments of oviduct: ovary (1/14), infundibulum (2/14), magnum (2/14), isthmus (3/14), uterus (3/14), and vagina (3/14) collected after 15 weeks p.i. However, in the TM group Salmonella was only recovered from infundibulum (1/14), uterus (2/14), and vagina (1/14) (Table 3). Mean concentration of Salmonella (mean log10 CFU/g) was highest in vagina (3.54 ± 0.64) and uterus (3.00 ± 0.45) of the T and TM group birds, respectively (Table 3). Colonization of reproductive organs was not frequent and only 0–3 hens of the 14 hens for each of the groups showed Salmonella in the bacteriological analysis of their reproductive organs, and no histopathological lesions were detected in any case.
Detection of S. Typhimurium in Reproductive Tissues by PCR
The reproductive organs from the T and TM groups found positive for Salmonella by culture method were analyzed by multiplex PCR to detect S. Typhimurium. Only one reproductive tissue (uterus) from T group was found positive for S. Typhimurium by multiplex PCR assay (data not shown). All other samples from T group tested negative by PCR for Salmonella spp.
Discussion
The present experiment was designed to study the long term shedding, egg contamination and colonization of oviduct by S. Typhimurium. It is considered that adult birds are more resistant to salmonellae than young chicks due to the developed gut microflora (Gast, 2008). Continued harboring of the organism and intermittent fecal shedding has also been noted for up to 1 year following infection of day old chicks (Gast, 2008) however, in our study older birds (14 wk) were infected with Salmonella. Previous studies reported low colonization of S. Typhimurium in adult birds (Groves, 2011), however, the results of the current study demonstrate that S. Typhimurium can colonize the gut and shed bacteria up to 15 weeks p.i.
In this study, intermittent but prolonged fecal shedding of bacteria was observed in both infected groups. A significant difference between the T and TM group at day 12 p.i. could be due to the intermittent Salmonella shedding. The magnitude of Salmonella shedding was higher up to 5 week p.i. Thereafter, the level of Salmonella in feces dropped but persisted for 15 weeks p.i. The increased Salmonella shedding in feces observed up to 5 week p.i. in this study could be attributed to the stress associated with the onset of lay. In layer birds, the stress occurring as a result of lay could negatively impact their immunity (El-Lethey et al., 2003; Humphrey, 2006) consequently resulting in higher shedding of Salmonella. Higher rate of fecal Salmonella shedding at the early onset of lay has also been reported previously (Gole et al., 2014a). The decrease in Salmonella load in feces after 5 weeks p.i. in both treatment groups could be the result of recovery from laying stress or development of effective humoral response. In addition, previous studies have reported that gastrointestinal microflora of older birds was responsible for protection against food poisoning Salmonella serovars (Barrow et al., 1988; Gast, 2008).
Fecal Salmonella counts from this study could not be compared with previous reports because the majority of these studies have examined post-infection fecal shedding of Salmonella for a shorter duration. A field survey investigating the prevalence of Salmonella shedding on commercial layer farms found significant variability in Salmonella prevalence at various stages of lay (Gole et al., 2014a). On farm, shedding of S. Typhimurium from the known positive laying hens can be intermittent and remain undetected for several weeks (Gole et al., 2014c). Such results suggest that Salmonella spp. can remain in the caeca for long periods of time and persistently infected hens could transmit the infection to unexposed and susceptible birds thereby maintaining the Salmonella infection cycle in the flock (Lister and Barrow, 2008). Hence, it is essential to frequently monitor the Salmonella free status of the birds used for the infection trials.
No correlation between fecal Salmonella counts and the recovery of bacteria from egg shell surface in experimentally infected birds was observed in this study. A recent longitudinal survey on two commercial layer farms found a significant relationship between Salmonella fecal contamination and egg shells testing positive for Salmonella (odds ratio 91.8; p < 0.001) (Gole et al., 2014c). In contrast, egg shells were found negative for S. Typhimurium in experimental infections although the bacterium was excreted in the feces (Baker et al., 1980; Okamura et al., 2001a,b). In the present study, though the egg shell contamination failed to positively relate with fecal shedding of Salmonella, fecal carriage of Salmonella was observed throughout the experimental period. The egg shell surface contamination observed in this study stresses the importance of proper egg handling and hygienic practices in food preparation and processing premises to avoid cross contamination of other food products.
The multiplex PCR was validated to detect S. Typhimurium positive samples in T and TM groups. In experimentally spiked fecal samples, the multiplex PCR demonstrated a good sensitivity and was able to detect 102 CFU/reaction of S. Typhimurium. On the other hand, PCR assay was able to detect 104 CFU/reaction of S. Typhimurium and S. Mbandaka in the fecal samples spiked with S. Typhimurium + S. Mbandaka. The poor detection limit observed in the feces experimentally spiked with S. Typhimurium + S. Mbandaka may under-represent the positive samples detected in the TM group using the PCR assay. The poor PCR sensitivity compared with the standard culture method to detect S. Typhimurium in fecal samples is similar to previous studies and could be attributed to the gradual reduction in Salmonella in feces, presence of PCR inhibitors and other abundant microflora DNA interfering with the PCR assays (Wilson, 1997; Gole et al., 2014a,c).
This study has examined egg shell contamination following oral infection with Salmonella for a prolonged period (15 weeks p.i.) compared to previous short term experimental infection studies (up to 3 weeks) and our results demonstrated that egg shell contamination by Salmonella occurred for longer p.i. intervals. Egg shell contamination following oral infection of S. Typhimurium observed in this study has also been reported previously (Cox et al., 1973). In the current study, the overall rate of egg shell contamination by Salmonella was significantly higher in the co-infected group (TM group) compared to the T group. However, the effect of co-infection on egg shell contamination analyzed by PCR demonstrated no significant difference in number of S. Typhimurium positive egg shells between T (S. Typhimurium) and TM groups (S. Typhimurium + S. Mbandaka). There is a little literature indicating the effect of mixed Salmonella infection on egg contamination after oral infection. The high experimental infection doses used in our study does not mimic field situations and had non-significant effects on the recovery rate of S. Typhimurium from the egg shell in the coinfected group. To compare these results with the field scenario further experiments using different routes and doses of multiple Salmonella serotypes are needed.
In the present study internal egg contents laid down by birds infected with S. Typhimurium alone or in combination with S. Mbandaka were negative for Salmonella up to week 15 p.i. The results of this study are also in agreement with the field surveys in Australia (Daughtry et al., 2005; Gole et al., 2013, 2014c) and previous reports in which oral or crop infection with S. Typhimurium was not associated with the contamination of egg contents (Cox et al., 1973; Baker et al., 1980; Keller et al., 1997; Okamura et al., 2010). On the other hand, contamination of egg internal contents with S. Typhimurium has been documented after experimental infection of hens at the onset of lay via oral and aerosol routes (Williams et al., 1998; Leach et al., 1999; Okamura et al., 2010). Altogether, the possibility of egg content contamination with S. Typhimurium seems to be a rare event. However, in those studies where experimental infection has caused internal contamination, sexual maturity, or the onset of lay was found to be an important factor for internal egg contamination.
It is well-known that colonization of reproductive organs with S. Enteritidis results in the deposition of bacteria within the egg contents of developing eggs in experimentally infected laying hens (Thiagarajan et al., 1994; Keller et al., 1995). However, the frequency of S. Typhimurium isolation from reproductive organs and corresponding frequency of internal egg content contamination is unclear. The present study determined that colonization of reproductive organs of S. Typhimurium infected (T group) hens and coinfected (TM group) hens varied after oral infection. The magnitude of S. Typhimurium recovery from each section of oviduct except for uterus was higher in the T group than TM group where Salmonella was localized to certain parts of the oviduct. To assess the effect of mixed infection, reproductive tissues from T and TM groups found positive for Salmonella by culture method were also analyzed by multiplex PCR to detect S. Typhimurium. In spite of positive culture results, S. Typhimurium was recovered from only one reproductive tissue (uterus) by multiplex PCR assay. This finding suggests that culture methods are more sensitive than multiplex PCR in detecting S. Typhimurium. The lack of additional stand-alone S. Mbandaka group and sacrifice of birds at regular intervals are some of the limitations of this study. However, it is interesting to note that despite the low Salmonella colonization in the oviduct of hens from TM group, frequency of egg shell contamination was significantly higher in the TM group (particularly for S. Mbandaka) as compared to the T group.
The results of prolonged Salmonella fecal shedding observed in this study indicated that colonization was present somewhere within the animal after several weeks p.i. However, though the persistence of Salmonella in the reproductive tissues of very few infected birds was evident after a long p.i. interval, the internal egg contents were negative throughout the experimental period in both T and TM groups. Moreover, this study demonstrates that the mere presence of S. Typhimurium in reproductive tissues would not give rise to the production of internally contaminated eggs.
The observations of the present study also support the previous findings which concluded that S. Typhimurium has the potential to colonize both the reproductive organs and developing eggs prior to oviposition but cannot be recovered from internal egg contents after oviposition (Keller et al., 1997; Okamura et al., 2001a; Gantois et al., 2008). Overall, the results of the present and previous studies demonstrate that S. Typhimurium was found to colonize the reproductive organs of laying hens. However, why S. Typhimurium is not associated with contamination of laid eggs is still unclear.
S. Typhimurium is able to penetrate and survive in the egg albumin and the yolk at 20 or 25°C (Gantois et al., 2008; Gole et al., 2014b). In addition, the S. Typhimurium genome possesses virulence associated genes involved in cellular adhesion, invasion and survival of S. Typhimurium (McWhorter et al., 2015). Therefore, it could be possible that S. Typhimurium is unable to survive and proliferate in egg contents during egg formation at host body temperature (42°C) or there could be down regulation of genes critical to colonization of S. Typhimurium. This could partly explain why S. Typhimurium despite their colonization in reproductive organs was never isolated from egg contents in this study. Salmonella pathogenicity islands (SPIs) are the gene clusters that encode virulence factors present in Salmonella genome (Foley et al., 2013). It has been observed that SPI-1 and SPI-2 contribute to the colonization of caecum, liver, and spleen in chickens (Dieye et al., 2009; Rychlik et al., 2009). A recent study also demonstrated that poultry body temperature may regulate systemic colonization (Troxell et al., 2015). However, the role of these pathogenicity islands in reproductive organ colonization in laying hens is less understood and needs further research. In addition, the possible role of several factors such as immunoglobulins, iron sequestering, and proteins inhibiting bacterial protease and antibacterial enzymes present in the egg yolk and albumin have been identified to inhibit the growth of Salmonella before shell formation is complete and eggs are laid (Keller et al., 1995; Gantois et al., 2009; Bedrani et al., 2013).
In order to determine the course of reproductive organ invasion after oral Salmonella infection, histopathology of reproductive tissues was carried out in this experiment. The regions of reproductive tract which were found positive after cultural analysis did not show lesions suggestive of bacterial infection. As there is lack of published information on histopathological alterations in oviduct tissue after prolonged infection interval, these findings could not be compared to previous studies. In addition, examination of infected birds at periodic intervals was not a part of this study but may have identified a time window for establishment of oviduct lesions as a result of bacterial infection. The possible explanation for the absence of inflammatory lesions after a long p.i. interval in response to oral Salmonella infection in this study could be related to either the low level of tissue colonization or development of strong immune response to clear the infection. Further, research examining the localization of Salmonella at different time intervals, cellular involvement and why Salmonella clearance from reproductive tissues does not take place is warranted.
In summary, intermittent but persistent fecal shedding of Salmonella after oral infection was observed up to 15 weeks p.i. Further, egg shell contamination together with lack of internal egg contents contamination and the low frequency of reproductive organ infection suggested that horizontal infection through contaminated feces is the main route of egg contamination with S. Typhimurium during lay. Previously, it has been hypothesized that effective and more immune response generated by S. Typhimurium compared to S. Enteritidis is likely to limit the disease progression and quickly clears the S. Typhimurium infection from birds (Wales and Davies, 2011). The egg shell contamination observed in this study also stresses the importance of proper egg handling and hygienic practices in food preparation and processing premises to avoid cross contamination of other food products. Considering the productive life span of commercial laying hens (75–80 weeks) further studies are required to study the shedding of S. Typhimurium beyond 15 weeks p.i.
Author Contributions
Conception and designed the experiments: KC, AM, and VP. Performed the experiments: VP, RD, PS, KC, and AM. Data acquisition and analysis: VP, KC, and AM. Drafting of article and revisions: VP, AM, and KC.
Conflict of Interest Statement
The authors declare that the research was conducted in the absence of any commercial or financial relationships that could be construed as a potential conflict of interest.
Acknowledgments
This research work was supported by Australian Egg Corporation Limited (AECL) Australia. Mr. VP is a recipient of post-graduate research scholarship of The University of Adelaide Australia. We thank Dr Vaibhav Gole and Dr Rebecca Forder for technical help in this study.
Supplementary Material
The Supplementary Material for this article can be found online at: http://journal.frontiersin.org/article/10.3389/fmicb.2016.00203
References
Akiba, M., Kusumoto, M., and Iwata, T. (2011). Rapid identification of Salmonella enterica serovars, Typhimurium, Choleraesuis, Infantis, Hadar, Enteritidis, Dublin and Gallinarum, by multiplex PCR. J. Microbiol. Methods 85, 9–15. doi: 10.1016/j.mimet.2011.02.002
Baker, R. C., Goff, J. P., and Mulnix, E. J. (1980). Salmonellae recovery following oral and intravenous inoculation of laying hens. Poult. Sci. 59, 1067–1072. doi: 10.3382/ps.0591067
Barrow, P. A., Simpson, J. M., and Lovell, M. A. (1988). Intestinal colonisation in the chicken by food-poisoning Salmonella serotypes; microbial characteristics associated with faecal excretion. Avian Pathol. 17, 571–588. doi: 10.1080/03079458808436478
Bedrani, L., Helloin, E., Guyot, N., Réhault-Godbert, S., and Nys, Y. (2013). Passive maternal exposure to environmental microbes selectively modulates the innate defences of chicken egg white by increasing some of its antibacterial activities. BMC Microbiol. 13:128. doi: 10.1186/1471-2180-13-128
Cox, N. A., Davis, B. H., Watts, A. B., and Colmer, A. R. (1973). Salmonella in the laying hen. 1. Salmonella recovery from viscera, feces and eggs following oral inoculation. Poult Sci. 52, 661–666. doi: 10.3382/ps.0520661
Daughtry, B. J., Sumner, J., Hooper, G., Thomas, C. J., Grimes, T., Horn, R., et al. (2005). National Food Safety Risk Profile of Eggs and Egg Products. Sydney: Australian Egg Corporation Limited.
Dean, A., Sullivan, K., and Soe, M. (2011). OpenEpi: Open Source Epidemiologic Statistics for Public Health, Version 2.3.1. Available online at: http://www.openepi.com/Menu/OE_Menu.htm
De Reu, K., Grijspeerdt, K., Messens, W., Heyndrickx, M., Uyttendaele, M., Debevere, J., et al. (2006). Eggshell factors influencing eggshell penetration and whole egg contamination by different bacteria, including Salmonella Enteritidis. Int. J. Food Microbiol. 112, 253–260. doi: 10.1016/j.ijfoodmicro.2006.04.011
Dieye, Y., Ameiss, K., Mellata, M., and Curtiss, R. III. (2009). The Salmonella Pathogenicity Island (SPI) 1 contributes more than SPI2 to the colonization of the chicken by Salmonella enterica serovar Typhimurium. BMC Microbiol. 9:3. doi: 10.1186/1471-2180-9-3
El-Lethey, H., Huber-Eicher, B., and Jungi, T. W. (2003). Exploration of stress-induced immunosuppression in chickens reveals both stress-resistant and stress-susceptible antigen responses. Vet. Immunol. Immunopathol. 95, 91–101. doi: 10.1016/S0165-2427(02)00308-2
Foley, S. L., Johnson, T. J., Ricke, S. C., Nayak, R., and Danzeisen, J. (2013). Salmonella pathogenicity and host adaptation in chicken-associated serovars. Microbiol. Mol. Biol. Rev. 77, 582–607. doi: 10.1128/MMBR.00015-13
Foley, S. L., Nayak, R., Hanning, I. B., Johnson, T. J., Han, J., and Ricke, S. C. (2011). Population dynamics of Salmonella enterica serotypes in commercial egg and poultry production. Appl. Environ. Microbiol. 77, 4273–4279. doi: 10.1128/AEM.00598-11
Gantois, I., Ducatelle, R., Pasmans, F., Haesebrouck, F., Gast, R., Humphrey, T. J., et al. (2009). Mechanisms of egg contamination by Salmonella Enteritidis. FEMS Microbiol. Rev. 33, 718–738. doi: 10.1111/j.1574-6976.2008.00161.x
Gantois, I., Eeckhaut, V., Pasmans, F., Haesebrouck, F., Ducatelle, R., and van Immerseel, F. (2008). A comparative study on the pathogenesis of egg contamination by different serotypes of Salmonella. Avian Pathol. 37, 399–406. doi: 10.1080/03079450802216611
Gast, R. K. (2008). “Paratyphoid infections,” in Diseases of Poultry, eds. Y. M. Saif, A. M. Fadly, J. R. Glisson, L. R. McDougald, L. K. Nolan, and D. E. Swayne (Ames: Iowa State University Press; Blackwell Publishing), 636–655.
Gast, R. K., Guard-Bouldin, J., and Holt, P. S. (2004). Colonization of reproductive organs and internal contamination of eggs after experimental infection of laying hens with Salmonella heidelberg and Salmonella Enteritidis. Avian Dis. 48, 863–869. doi: 10.1637/7204-05050R
Gast, R. K., Guraya, R., Guard-Bouldin, J., Holt, P. S., and Moore, R. W. (2007). Colonization of specific regions of the reproductive tract and deposition at different locations inside eggs laid by hens infected with Salmonella Enteritidis or Salmonella heidelberg. Avian Dis. 51, 40–44. doi: 10.1637/0005-2086(2007)051[0040:COSROT]2.0.CO;2
Gast, R. K., Guraya, R., and Guard, J. (2013). Salmonella Enteritidis deposition in eggs after experimental infection of laying hens with different oral doses. J. Food Prot. 76, 108–113. doi: 10.4315/0362-028X.JFP-12-268
Gole, V. C., Caraguel, C. G., Sexton, M., Fowler, C., and Chousalkar, K. K. (2014a). Shedding of Salmonella in single age caged commercial layer flock at an early stage of lay. Int. J. Food Microbiol. 189, 61–66. doi: 10.1016/j.ijfoodmicro.2014.07.030
Gole, V. C., Chousalkar, K. K., and Roberts, J. R. (2013). Survey of Enterobacteriaceae contamination of table eggs collected from layer flocks in Australia. Int. J. Food Microbiol. 164, 161–165. doi: 10.1016/j.ijfoodmicro.2013.04.002
Gole, V. C., Chousalkar, K. K., Roberts, J. R., Sexton, M., May, D., Tan, J., et al. (2014b). Effect of egg washing and correlation between eggshell characteristics and egg penetration by various Salmonella Typhimurium strains. PLoS ONE 9:e90987. doi: 10.1371/journal.pone.0090987
Gole, V. C., Torok, V., Sexton, M., Caraguel, C. G., and Chousalkar, K. K. (2014c). Association between the indoor environmental contamination of Salmonella with egg contamination on layer farms. J. Clin. Microbiol. 52, 3250–3258. doi: 10.1128/JCM.00816-14
Groves, P. (2011). Salmonella Control in Layer Chickens. A Report for the Australian Egg Corporation Limited. Sydney, NSW: Poultry Research Foundation, The University of Sydney.
Hoszowski, A., and Wasyl, D. (2001). Typing of Salmonella enterica subsp. enterica serovar Mbandaka isolates. Vet. Microbiol. 80, 139–148. doi: 10.1016/S0378-1135(00)00382-5
Humphrey, T. (2006). Are happy chickens safer chickens? Poultry welfare and disease susceptibility. Br. Poult Sci. 47, 379–391. doi: 10.1080/00071660600829084
Im, M. C., Jeong, S. J., Kwon, Y. K., Jeong, O. M., Kang, M. S., and Lee, Y. J. (2015). Prevalence and characteristics of Salmonella spp. isolated from commercial layer farms in Korea. Poult Sci. 94, 1691–1698. doi: 10.3382/ps/pev137
Keller, L. H., Benson, C. E., Krotec, K., and Eckroade, R. J. (1995). Salmonella Enteritidis colonization of the reproductive tract and forming and freshly laid eggs of chickens. Infect. Immun. 63, 2443–2449.
Keller, L. H., Schifferli, D. M., Benson, C. E., Aslam, S., and Eckroade, R. J. (1997). Invasion of chicken reproductive tissues and forming eggs is not unique to Salmonella Enteritidis. Avian Dis. 41, 535–539. doi: 10.2307/1592142
Leach, S. A., Williams, A., Davies, A. C., Wilson, J., Marsh, P. D., and Humphrey, T. J. (1999). Aerosol route enhances the contamination of intact eggs and muscle of experimentally infected laying hens by Salmonella typhimurium DT104. FEMS Microbiol. Lett. 171, 203–207. doi: 10.1111/j.1574-6968.1999.tb13433.x
Lister, S. A., and Barrow, P. (2008). “Enterobacteriaceae,” in Poultry Diseases, 6th Edn, eds M. Pattison, P. F. McMullin, J. M. Bradbury, and D. J. Alexander (Edinburgh: W.B.Saunders), 111–125.
Little, C. L., Walsh, S., Hucklesby, L., Surman-Lee, S., Pathak, K., Gatty, Y., et al. (2007). Survey of Salmonella contamination of non–United Kingdom produced raw shell eggs on retail sale in the northwest of England and London, 2005 to 2006. J. Food Prot. 70, 2259–2265.
McWhorter, A. R., Davos, D., and Chousalkar, K. K. (2015). Pathogenicity of Salmonella strains isolated from egg shells and the layer farm environment in Australia. Appl. Environ. Microbiol. 81, 405–414. doi: 10.1128/AEM.02931-14
Okamura, M., Kamijima, Y., Miyamoto, T., Tani, H., Sasai, K., and Baba, E. (2001a). Differences among six Salmonella serovars in abilities to colonize reproductive organs and to contaminate eggs in laying hens. Avian Dis. 45, 61–69. doi: 10.2307/1593012
Okamura, M., Miyamoto, T., Kamijima, Y., Tani, H., Sasai, K., and Baba, E. (2001b). Differences in abilities to colonize reproductive organs and to contaminate eggs in intravaginally inoculated hens and in vitro adherences to vaginal explants between Salmonella Enteritidis and other Salmonella serovars. Avian Dis. 45, 962–971. doi: 10.2307/1592875
Okamura, M., Sonobe, M., Obara, S., Kubo, T., Nagai, T., Noguchi, M., et al. (2010). Potential egg contamination by Salmonella enterica serovar Typhimurium definitive type 104 following experimental infection of pullets at the onset of lay. Poult. Sci. 89, 1629–1634. doi: 10.3382/ps.2010-00774
OzFoodNet Working Group (2009). Monitoring the incidence and causes of diseases potentially transmitted by food in Australia: annual report of the OzFoodNet Network, 2008. Commun. Dis. Intell. Q. Rep. 33, 389–413.
OzFoodNet Working Group (2012). Monitoring the incidence and causes of diseases potentially transmitted by food in Australia: annual report of the OzFoodNet network, 2010. Commun. Dis. Intell. Q. Rep. 36, E213–E241.
Pande, V. V., Gole, V. C., McWhorter, A. R., Abraham, S., and Chousalkar, K. K. (2015). Antimicrobial resistance of non-typhoidal Salmonella isolates from egg layer flocks and egg shells. Int. J. Food Microbiol. 203, 23–26. doi: 10.1016/j.ijfoodmicro.2015.02.025
Pavic, A., Groves, P. J., Bailey, G., and Cox, J. M. (2010). A validated miniaturized MPN method, based on ISO 6579:2002, for the enumeration of Salmonella from poultry matrices. J. Appl. Microbiol. 109, 25–34. doi: 10.1111/j.1365-2672.2009.04649.x
Rychlik, I., Karasova, D., Sebkova, A., Volf, J., Sisak, F., Havlickova, H., et al. (2009). Virulence potential of five major pathogenicity islands (SPI-1 to SPI-5) of Salmonella enterica serovar Enteritidis for chickens. BMC Microbiol. 9:268. doi: 10.1186/1471-2180-9-268
Samberg, Y., and Meroz, M. (1995). Application of disinfectants in poultry hatcheries. Rev. Sci. Tech. 14, 365–380.
Santos, F., Li, X., Payne, J., and Sheldon, B. (2005). Estimation of most probable number Salmonella populations on commercial North Carolina turkey farms. J. Appl. Poult. Res. 14, 700–708. doi: 10.1093/japr/14.4.700
Thiagarajan, D., Saeed, A. M., and Asem, E. K. (1994). Mechanism of transovarian transmission of Salmonella Enteritidis in laying hens. Poult. Sci. 73, 89–98. doi: 10.3382/ps.0730089
Troxell, B., Petri, N., Daron, C., Pereira, R., Mendoza, M., Hassan, H. M., et al. (2015). Poultry body temperature contributes to invasion control through reduced expression of Salmonella pathogenicity island 1 genes in Salmonella enterica Serovars Typhimurium and Enteritidis. Appl. Environ. Microbiol. 81, 8192–8201. doi: 10.1128/AEM.02622-15
Wales, A. D., and Davies, R. H. (2011). A critical review of Salmonella Typhimurium infection in laying hens. Avian Pathol. 40, 429–436. doi: 10.1080/03079457.2011.606799
Whiley, H., and Ross, K. (2015). Salmonella and Eggs: from production to plate. Int. J. Environ. Res. Public Health 12, 2543–2556. doi: 10.3390/ijerph120302543
Williams, A., Davies, A. C., Wilson, J., Marsh, P. D., Leach, S., and Humphrey, T. J. (1998). Contamination of the contents of intact eggs by Salmonella Typhimurium DT104. Vet. Rec. 143, 562–563. doi: 10.1136/vr.143.20.562
Keywords: Salmonella Typhimurium, laying hens, oviduct, egg contamination
Citation: Pande VV, Devon RL, Sharma P, McWhorter AR and Chousalkar KK (2016) Study of Salmonella Typhimurium Infection in Laying Hens. Front. Microbiol. 7:203. doi: 10.3389/fmicb.2016.00203
Received: 25 November 2015; Accepted: 08 February 2016;
Published: 25 February 2016.
Edited by:
Jean-Christophe Augustin, Ecole Nationale Vétérinaire d'Alfort, FranceReviewed by:
Brian Brunelle, National Animal Disease Center, USAAvelino Alvarez-Ordóñez, Teagasc Food Research Centre, Ireland
Copyright © 2016 Pande, Devon, Sharma, McWhorter and Chousalkar. This is an open-access article distributed under the terms of the Creative Commons Attribution License (CC BY). The use, distribution or reproduction in other forums is permitted, provided the original author(s) or licensor are credited and that the original publication in this journal is cited, in accordance with accepted academic practice. No use, distribution or reproduction is permitted which does not comply with these terms.
*Correspondence: Kapil K. Chousalkar, a2FwaWwuY2hvdXNhbGthckBhZGVsYWlkZS5lZHUuYXU=