- 1School of Municipal and Environmental Engineering, Harbin Institute of Technology, Harbin, China
- 2State Key Laboratory Breeding Base of Marine Genetic Resources, Key Laboratory of Marine Genetic Resources, Third Institute of Oceanography, State Oceanic Administration, Collaborative Innovation Center for Exploitation and Utilization of Marine Biological Resources, Key Laboratory of Marine Genetic Resources of Fujian Province, Xiamen, China
A multilocus sequence analysis (MLSA) was established and performed on the genus Thioclava, including 23 strains isolated from diverse marine environments, with the aim of better differentiation of strains and species within this genus. The study was based on sequences of 16S rRNA gene and five protein-coding housekeeping genes, gyrB, rpoD, dnaK, trpB, and recA. In contrast to 16S rRNA gene-based tree that was unable to separate some species within this genus, each tree based on a single housekeeping gene and MLSA had consistently defined seven clades, corresponding to the five established ones and two novel ones. The digital DNA-DNA hybridization and average nucleotide identity analyses based on genome sequences of the representative strains reconfirmed the validity of the MLSA analysis, and recommended a 97.3% MLSA similarity as the soft species threshold and nine species representing the five known and four putative novel species. Two of the four new species were identified as Thioclava sediminum sp. nov. (type strain TAW-CT134T = MCCC 1A10143T = LMG 29615T) and Thioclava marinus sp. nov. (type strain 11.10-0-13T = MCCC 1A03502T = LMG 29618T) by using a polyphasic taxonomic approach. Taken together, the newly established MLSA in this study first described the variability and phylogeny of the genus Thioclava which contributes to better understanding its ecology and evolution.
Introduction
Thioclava is a genus consisting of Gram-staining-negative, moderately halophilic and facultatively aerobic bacteria belonging to the family Rhodobacteraceae within the class Alphaproteobacteria (Sorokin et al., 2005). At the time of writing, the genus Thioclava consisted of the five published species, including the type species Thioclava pacifica (Sorokin et al., 2005), along with Thioclava dalianensis (Zhang et al., 2013), Thioclava atlantica (Lai et al., 2014a), Thioclava indica (Liu et al., 2015a), and the newly described Thioclava nitratireducens (Liu et al., 2017b). Until now, a large number of isolates of this genus were obtained from diverse marine environments and preliminarily identified by 16S rRNA gene sequencing. Some strains were found to capable of sulfur oxidation (Sorokin et al., 2005), degradation of crude oil (Wang et al., 2014), decomposition of dibenzothiophene (unpublished data), fixation of carbon dioxide (unpublished data), and power generation as electrochemically active bacteria (Rowe et al., 2015). Therefore, we speculate that the Thioclava bacteria may play a crucial role in the biogeochemical cycles of sulfur and carbon.
PCR-based methods combined with DNA sequencing have been widely applied in phylogenetic analyses of bacteria, especially for some groups that cannot be distinguished by conventional methods. The 16S rRNA gene is the most popular genetic marker for taxonomic identification of Bacteria and Archaea (Yarza et al., 2014). However, 16S rRNA gene sequence is unable to distinguish certain closely related strains and even species owing to the relatively high conservation, the heterogeneity of multiple copies, and horizontal gene transfer (HGT) in previously published studies (Kitahara and Miyazaki, 2013; Liu et al., 2013, 2015b; Tian et al., 2015). Similarly, almost all of Thioclava bacteria from our group and GenBank database could not be well classified with respect to the relatively high similarities of their 16S rRNA gene sequences (above 97%).
Since 2005, multilocus sequence analysis (MLSA) has become a powerful tool and has been performed frequently to elucidate taxonomic relationship and phylogenetic analysis of closely related strains (Gevers et al., 2005; Vinuesa, 2010; Peeters et al., 2016; Whatmore et al., 2016). In general, several housekeeping genes are chosen preferentially in MLSA schemes, such as gyrB (DNA gyrase, beta subunit), rpoD (RNA polymerase, σ70 factor), dnaK (Chaperone protein DnaK), trpB (tryptophan synthase, beta subunit), and recA (recombinase A), because they encode core metabolic enzymes (Charlebois and Doolittle, 2004); and more importantly, they own much higher discrimination than 16S rRNA gene (Glaeser and Kämpfer, 2015). Furthermore, the concatenated housekeeping genes could minimize the weight of HGT (Macheras et al., 2011) and/or recombination (Timilsina et al., 2015) and then provide accurate taxonomic position for closely related species and strains (Glaeser and Kämpfer, 2015). In addition, digital DNA-DNA hybridization (dDDH) and average nucleotide identity (ANI) analyses based on genome sequences of bacteria are rapid and reliable methods for identification of closely related strains (Konstantinidis and Tiedje, 2005; Meier-Kolthoff et al., 2013; Garrido-Sanz et al., 2016). In recent years, these approaches have been successfully used for numerous bacteria, e. g. the Bacillus cereus group (Liu et al., 2015b, 2017a), Thalassospira (Lai et al., 2014c), Hyphomonas (Li et al., 2014), Burkholderia (Peeters et al., 2016), but without considering members of the genus Thioclava to date. Consequently, whether these approaches can be used for the Thioclava bacteria remains to be further investigated.
In recent years, multiple strains of bacteria assigned to the genus Thioclava have been isolated from various marine environments, suggesting a critical role of this genus in distinct habitats. This study proposes MLSA analyses to resolve the taxonomic affiliation of novel strains and species within this genus. In this study, we created the new MLSA scheme based on five housekeeping genes with gyrB, rpoD, recA, trpB, and dnaK as well as 16S rRNA gene to resolve the taxonomic affiliation of a collection of the Thioclava bacteria isolated from various marine environments, and further verified by dDDH and ANI analyses. Four putative novel species were discovered and two of them were subjected to a polyphasic taxonomic characterization.
Materials and Methods
Strains and Culture Conditions
Many Thioclava strains were isolated in recent years by our group, the representatives of which have been deposited in the Marine Culture Collection of China (MCCC). In this report, 23 strains were chosen for phylogenetic study (Table 1), among which, five were type strains, T. pacifica MCCC 1A06460T, T. dalianensis MCCC 1A03957T, T. atlantica MCCC 1A02612T, T. indica MCCC 1A00513T, and T. nitratireducens MCCC 1A07302T. As listed in Table 1, all strains were isolated from marine environments; 15 strains were isolated from coastal areas of China, such as Beihai Sea, Dalian Bay, Yellow Sea; two from the Pacific Ocean; one from the Indian Ocean; one from the Atlantic Ocean; and two from the Bering Sea. Other two strains, Thioclava sp. ES.031 and Thioclava sp. ES.031, were isolated from the Monterey Bay near Monterey city in California on the basis of the annotated information from IMG database (http://genome.jgi.doe.gov/). Unless otherwise specified, strains were grown aerobically on marine agar 2216 and in marine broth 2216 (BD, Difco) for 48 h at 28°C.
DNA Preparation, Gene Selection, PCR Amplification, and Sequencing
Bacterial genomic DNAs for the strains were extracted using the SBS extraction kit (SBS Genetech Co., Ltd. in Shanghai, China) following the manufacturer's instructions. In this study, the above-mentioned five housekeeping genes were chosen on the basis of the following criteria: (1) the common presence in all strains belonged to the core genes; (2) single copy; (3) a relatively high discrimination power relative to 16S rRNA gene; (4) no or little HGT and recombination events; (5) the same or similar evolutionary rate. PCR amplification and sequencing of 16S rRNA and gyrB gene were performed using the primers as described previously (Lai et al., 2014c), and included in Table S1. The four pair specific primers for other genes, rpoD, recA, trpB, and dnaK, were designed from the available genome sequences of five type strains in GenBank database and two in IMG database using the software Primer Premier version 5.0, which were marked, respectively in Table S1. These genes were amplified under nearly identical conditions. The 50 μL reaction mixtures contained 37 μL of double distilled water, 5 μL of 10 × Ex Taq buffer (Mg2+ Plus), 4 μL of dNTP mixture (10 mM), 1 μL of each primer (20 μM), 1 μL of genomic DNA template (10–30 ng/μL), 1 μL of Ex Taq™ DNA polymerase (TaKaRa, 5 U/μL). PCR amplifications were performed according to the following parameters in a Biometra T-Professional thermocycler (Biometra; Goettingen, Germany): initial denaturation at 94°C for 5 min; then 30 cycles of 30 s of denaturation at 94°C, 30 s of annealing at 55°C, and 1.0–1.5 min of extension at 72°C; and a final extension at 72°C for 10 min. More detail information was listed in Table S1. After amplification, PCR products and their concentration were verified by electrophoresis of 3 μL products on a 1% agarose gel and stained with ethidium bromide. A DL2000 marker (TaKaRa, China) was included to estimate the length of the amplification products. And then, the PCR products were purified using the PCR purification kit (Axygen Scientific, Inc., USA) according to the manufacturer's instructions. Finally, the purified products were sequenced with the ABI3730xl platform (Shanghai Majorbio Bio-Pharm Technology Co., Ltd., Shanghai, China) using the corresponding sequencing primers (Table S1).
The ambiguous bases and/or gaps of sequences for all genes of 16 strains were modified artificially on the basis of the height of the original sequencing peak using the software DNAMAN version 7.0 (Lynnon Biosoft, Quebec, Canada). The gene sequences of other seven strains were obtained from their genome sequences using a local BLAST function implemented in the software BioEdit version 7.0.9.0 (http://www.mbio.ncsu.edu/BioEdit/bioedit.html). All sequences were submitted to GenBank database. The accession numbers were assigned and listed in Table S2.
Analysis of Nucleotide Diversity
All gene sequences were aligned using the software MAFFT version 7.311 under the default settings (Katoh and Standley, 2013) for nucleotide diversity analysis. Diversity indices of 16S rRNA gene, single housekeeping gene, and concatenated housekeeping genes sequences, such as numbers of allele and parsimony informative sites, mean G+C content (mol%), and Ka/Ks ratios (Ka: the number of non-synonymous substitutions per non-synonymous site, Ks: the number of synonymous substitutions per synonymous site), were analyzed using the software DnaSP version 5.10 (http://www.ub.edu/dnasp/). The ranges of similarities and mean similarities of single gene and concatenated genes sequences were analyzed using DNAMAN 7.0 under default parameters.
Phylogenetic Analysis
Prior to the phylogenetic analysis, all genes were subjected to an examination of sequence substitution saturation and recombination events. A substitution saturation assessment was performed using the software DAMBE version 5.3.10 (Xia, 2013). Putative recombination events were detected using the software RDP version 4.16 (Martin et al., 2015). This software was used for searching the occurrence of recombination events when different parts of the sequence data result in discordant topologies using different algorithms, RDP, Bootscan, MaxChi, Chimera, Geneconv, and SiScan. Statistical significance was set at P ≤ 0.01.
The five housekeeping gene sequences were imported into the software MEGA version 5.05 (Tamura et al., 2011) for alignment by amino acid. Once the open reading frame was determined, sequences were translated to amino acid and then aligned by Muscle (Codons) (Edgar, 2004) implemented in MEGA 5.05. The amino acid alignment was then back-translated to nucleotides, retaining the codon positions. The 16S rRNA gene sequences were aligned using MAFFT 7.311. The appropriate nucleotide substitution models for gene(s) were determined using the software jModelTest version 2.1.7 (Darriba et al., 2012) under the Akaike Information Criterion (Posada and Buckley, 2004). The phylogenetic trees were constructed, respectively by maximum likelihood (ML) (Felsenstein, 1981) implemented in the software RaxmlGUI version 1.3 (Silvestro and Michalak, 2012) under default settings using the GTR+I model for 16S rRNA gene and the GTR+I+G model for single housekeeping gene and the concatenated genes (in the following order: gyrB, rpoD, dnaK, trpB, and recA). The type strain Rhodovulum sulfidophilum DSM 1374T (Accession number: CP015418) was used as an outgroup in all phylogenetic analysis. Bootstrap confidence analysis was carried out with 1,000 replicates for evaluating the robustness of tree topologies. >70% Bootstrap values were shown at nodes of all trees. The bar represented nucleotide substitution rate (Knuc) units in each tree.
Genome Sequencing, dDDH and ANI Calculation, and DNA G+C Content
The representative strains were selected on the basis of MLSA analysis. The genome sequences of these strains were determined by Shanghai Majorbio Bio-pharm Technology Co., Ltd. using Solexa paired-end (500 bp library) sequencing technology. About 500 Mbp of clean data were generated with an Illumina/Solexa Genome Analyzer IIx (Illumina, SanDiego, CA), reaching approximately 100-fold coverage depth, for each strain. The high quality reads were assembled SOAPdenovo version 1.05 with default parameters. And then the assembled contigs were submitted in GenBank database, and the assigned accession numbers and assembly statistics of them were listed in Table S3. The dDDH and ANI values for representative strains were calculated using the genome-to-genome distance calculator website service (GGDC 2.1) with the recommended formula 2 (http://ggdc.dsmz.de/distcalc2.php) and the EzGenome web service (http://www.ezbiocloud.net/ezgenome/ani), respectively. A previous study demonstrated that the dDDH values at the upper 95% confidence interval (CI) were in better agreement with those of ANI for delineating species (Colston et al., 2014). Therefore, the upper 95% CI dDDH values were used for analysis in this study. Correlation analyses between the dDDH values and MLSA similarities were simulated using a nonlinear interpolation analysis method with the default option of the Curve Fitting Tool implemented in the commercially available software MATLAB version R2013a (MathWorks Inc., USA). Additionally, the genomic DNA G+C contents of representative strains were determined based on the respective genomic sequences.
Morphological and Physiological Characterization
Cell and colony morphology, motility, Gram-staining, and anaerobic growth of representative strains were performed according to the previously described methods (Liu et al., 2017b). Catalase and oxidase activity was determined according to established procedures (Liu et al., 2016). The ranges and optimum of temperature, pH and NaCl were performed according to the methods previously described (Lai et al., 2014b). Other biochemical tests were carried out using API ZYM, API 20NE and API 20E strips (bioMérieux) according to the manufacturer's instructions, with the modification of adjusting the NaCl concentration to 3.0% in all tests.
Fatty Acid, Polar Lipid, and Quinone Characterization
For fatty acid analysis, the cells were harvested from the third quadrants on MA medium. The cells were saponified, methylated, and extracted using the standard MIDI (Sherlock Microbial Identification System, version 6.0B) protocol. The fatty acids were then analyzed by gas chromatography (Agilent Technologies 6850) (Sasser, 1990). Analysis of the respiratory quinones and polar lipids were carried out according to the previously described method (Collins, 1985; Kates, 1986).
Results
Individual Gene Analyses
Almost full-length 16S rRNA gene sequences and partial nucleotide sequences of five housekeeping genes, including gyrB, rpoD, dnaK, trpB, and recA, were determined for all strains. The features of each gene and five concatenated genes are displayed in Table 2.
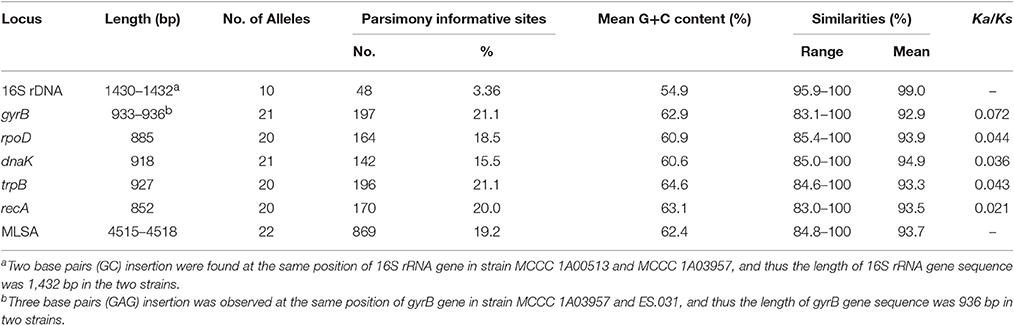
Table 2. Characteristics of the 16S rRNA gene, single housekeeping gene and the concatenated genes from all strains.
The trimmed 16S rRNA gene sequences with 1,430/1,432 bp in length of all isolates were used for phylogenetic analysis. Among 23 strains, the number of alleles was ten, and the numbers and proportion of parsimony informative sites (S) were, respectively, 48 and 3.36%. The mean G+C content was 54.9 mol%. The similarities ranged from 95.9 to 100%, with a mean value of 99.0%. As shown in Figure 1, 23 strains in ML tree based on 16S rRNA gene can be grouped into six clades. The first clade, designated Clade 1, included two branches; the first branch only contained one strains; the second contained seven strains with two sequence types (ST) (type strain MCCC 1A10143T). The second clade, named Clade 2, comprised the type strain MCCC 1A07302T and other five strains, exhibiting the same ST. The third clade, called Clade “3 and 4,” consisted of the two type strains (MCCC 1A06460T and MCCC 1A03502T) and other three strains, with three STs. The remaining three clades, marked Clade 5, 6, and 7, corresponded to the three species T. atlantica, T. dalianensis, and T. indica. The pairwise similarities of 16S rRNA gene sequences among all strains used in this study were higher than the threshold value of 97% that is used as the standard of the species boundary (Stackebrandt and Goebel, 1994), with exception of the type strain T. indica MCCC 1A00513T and 11 strains belonging to Clade 2, 3, and 4 (referred to MLSA analysis below). Therefore, 16S rRNA gene is inadequate to discriminate closely related species and strains.
The sequence lengths for the five housekeeping genes (Table 2) were from 852 bp (recA) to 933/936 bp (gyrB). Among 23 strains, the numbers of alleles (20–22) for these genes were roughly the same. The gyrB gene possessed the most parsimony informative sites with 197, followed by trpB with 196, recA with 170, rpoD with 164, and dnaK with 142. The percentages of parsimony informative sites for all genes were higher than 15%, suggesting more genetic information against 16S rRNA gene. The mean G+C contents of all genes were from 60.6 to 64.6 mol%. The ranges and mean values of the five gene similarities were not remarkable difference. In addition, a slight variation of the Ka/Ks ratio for the five housekeeping genes was observed in Table 2, suggesting that two genes, dnaK and recA, were under a higher negative selective pressure relative to the three genes, rpoD, trpB, and gyrB. However, all values were far below 1, indicating that these genes were under strong purifying selection (Hurst, 2002) and thereby suitable for MLSA. These results clearly demonstrated that each housekeeping gene owns a much higher discriminatory power compared to the 16S rRNA gene.
Test for substitution saturation of all genes sequences showed that they were not saturated in substitutions. Meanwhile, no recombination events were found in any of them, as determined by RDP 4.16. These results demonstrated that all gene sequences could provide essential phylogenetic information.
In comparison with 16S rRNA gene tree, all single-gene trees based on nucleotide sequences showed much higher resolution on the basis of more branches and higher bootstrap values (Figure S1). Moreover, these single-gene trees are generally of the same topology with seven clades (see the detailed description in MLSA section). However, some differences were observed in the deep branches of trees. For example, Clade 6 was a sister taxon to Clade 7 in the trees based on gyrB, rpoD, dnaK, or recA gene, while it was a deep branch in the tree based on trpB gene, and far related to Clade 7 (Figure S1). Clade 5 exhibited the varied positions in the five trees (Figure S1). In addition, some bacteria in Clade 1 and Clade 2 shifted from one position to another in different single-gene trees. Such as in the tree of gyrB, strain MCCC 1A03974 neighbored with MCCC 1A10143T, while it is by side of MCCC 1A02813 in the recA tree. Therefore, the single housekeeping gene cannot accurately infer the phylogenetic relationships of some members of the genus Thioclava, although it is effective in differentiating different species, and even different strains within a species.
MLSA
The concatenated sequences of the five housekeeping genes with 4,518 bp in length contained 22 alleles and 869 parsimony informative sites (19.2%) with a mean G+C content of 62.4 mol% (Table 2). The MLSA similarities among all tested strains ranged from 84.8 to 100%, with a mean value of 93.7% (Table S4). The MLSA tree showed a similar topology to the single housekeeping gene tree as described above, but showed much higher bootstrap values.
The 23 strains were divided into seven clades denoted as Clade 1 to Clade 7 in the MLSA tree (Figure 2). Specifically, Clade 1 had eight branches with eight STs, each of which represents a single strain. The Clade 2 was separated into six branches with six STs corresponding to the type strain MCCC 1A07302T and other five strains. The Clade 3 only contained the type strain MCCC 1A06460T. The Clade 4 was split into three branches including four strains with three STs. The Clade 5 included two strains with the same genotype, the type strain MCCC 1A02612T and MCCC 1A07323. The Clade 6 and Clade 7 were, respectively represented by the type strains MCCC 1A03957T and MCCC 1A00513T. Of them, bacteria in Clade 1 and Clade 4 cannot be allocated to any previously described species, and therefore, they are potential novel species.
General Genome Features
The general genome features of the eight newly sequenced strains and seven previously sequenced strains are summarized in Table S3. The genome sizes of these strains ranged from 3,653,331 to 4,962,820 bp. Contigs number ranged from one to 105, depending on the strain. The longest contigs of the genome was 3,938,466 bp from strain ES.032. The N50 length for these contigs showed significant differences, ranging from 116,979 to 2,098,934. The G+C content of these strains ranged from 60.3 mol% (MCCC 1A00513T) to 65.3 mol% (MCCC 1A02612T).
The dDDH and ANI Values and Comparison with MLSA
In order to further confirm the results of MLSA, the genome sequences of representative strains from seven clades were determined (at least one per clade). The pairwise dDDH and ANI values of 15 genome sequences were, respectively, calculated according to the method mentioned above, including five from GenBank database and two from IMG database. As listed in Table S5, most of dDDH values were <70%, that is often used as the gold standard for bacterial species definition (Wayne et al., 1987). Likewise, almost all ANI values were under a narrowed boundary of 95–96% (Table S6), which was proposed as the species definition for prokaryotes (Richter and Rossello-Mora, 2009). It is noteworthy that the dDDH values among the six MCCC strains in Clade 1 were slightly higher than 70%. Furthermore, the ANI values among the six strains were above 96%. Therefore, they belonged to the same species. Other two strains in Clade 1, strain ES.031 and ES.031, each represented a putative novel species on the basis of the dDDH and ANI values. Evidently, Clade 1 comprised three putative novel species. For other six clades, each represented one unique species, among which Clade 4 represented a novel species. Therefore, a total of four putative novel species were recommended in this study. Here, only two novel isolates from our group, one in Clade 1, and the one in Clade 4, were characterized further in taxonomy, and named as Thioclava sediminum sp. nov. and Thioclava marinus sp. nov. as below. The two novel species represented by ES.031 and ES.032 in Clade 1 had no culture in our lab and thus could not be characterized in the present study.
The correlation between the dDDH values and MLSA similarities for 15 strains was determined by a nonlinear interpolation analysis method. The dDDH values were highly correlated with the MLSA similarities (R2 = 0.9906). Based on the simulative equation of y = 90.87*exp(0.0009749*x)−982.7*exp(−0.2097*x), a 70% dDDH value was equivalent to a 97.3% MLSA similarity (Figure 3), which could be used to be species threshold for the members of the genus Thioclava. However, a gap of MLSA similarities from 97.3 to 96.6% was shown in Figure 3. Concretely speaking, there were three situations based on the current analyses in this study: (1) the strains were definitely the same species when MLSA similarities were above 97.3%; (2) the strains were the same or different species when they range from 97.3 to 96.6%; (3) the strains were affirmably different species when they were below 96.6%. As a result, the MLSA cutoff should be used as a soft rather than hard boundry for demarcating species within the genus Thioclava.
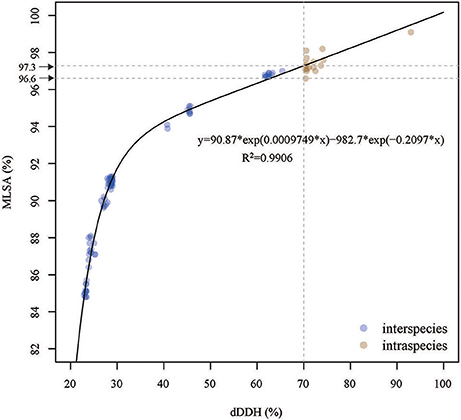
Figure 3. The Correlation analysis between the dDDH values and MLST similarities for resprestative Thicolava strains. The vertical line indicates the 70% dDDH threshold. The horizontal line (y = 97.3) indicates the estimated MLSA similarity threshold corresponding to 70% dDDH threshold.
Phenotypic Characteristics of Thioclava sediminum and Thioclava marinus
The cell shape of Thioclava sediminum TAW-CT134T and Thioclava marinus 11.10-0-13T, are the same as those of reference strains (short rods) (Figure S2). By contrast, the type strain T. pacifica MCCC 1A06460T varied from long filaments with swollen ends, clustered in aggregates, to single small rods (Sorokin et al., 2005). Colonies of all strains are circular, non-translucent, and smooth with entire margins after 3 days at 28°C. T. sediminum TAW-CT134T is motile by means of a polar flagellum, while T. marinus 11.10-0-13T does not possess flagella. All strains are Gram-staining-negative, aerobic, catalase- and oxidase-positive. The growth and optimal temperature, pH and NaCl ranges for growth of these two strains are similar to the ones of reference strains (Liu et al., 2017b). In the API ZYM, API 20NE and API 20E tests, all strains present similar physiological characteristics, indicating that the two strains belong to the genus Thioclava. However, as shown in Table 3 and in the species description below, some morphological and physiological characteristics distinguish both strains from other known species.
Below are the chemotaxonomic features of the two strains, analyzed in parallel with other reference strains. As a result, the composition of fatty acids for the two novel strains are almost consistent with those of closely related type strains, with Summed Feature 8 (C18:1ω6c and/or C18:1ω7c) as the major component (Table S7), and supporting their allocation in the genus Thioclava (Liu et al., 2017b). However, the proportions of fatty acids varied to some extent among them (Table S7). The quinone of the two novel strains is identified as Q-10, which is in accordance with data from reference strains (Liu et al., 2017b). The types of polar lipids for the two novel species are almost identical, with the presence of phosphatidylglycerol (PG), phosphatidylethanolamine (PE), unidentified glycolipid (GL), and several unidentified phospholipids (PLs) (Figure S3). The distinctive feature is the presence of aminophospholipid in type strain MCCC 1A02612T (Lai et al., 2014a) and MCCC 1A03957T (Zhang et al., 2013). The DNA G+C content of two novel strains TAW-CT134T and 11.10-0-13T are, respectively, 64.0 and 64.1 mol%, which are in the same range than the ones of the five type strains (60.3–65.3 mol%) (Liu et al., 2017b).
Discussion
The members of the genus Thioclava are ubiquitous in marine environments and distributed in a variety of ecological niches, such as surface and deep seawater, deep sea sediment, halobios in global oceans like the Pacific Ocean, the Atlantic Ocean, the Indian Ocean and even the Arctic Ocean (Wang et al., 2012, 2014; Rowe et al., 2015). However, the phylogeny of bacteria within this genus has been not well understood so far. In this report, a MLSA scheme based on five housekeeping genes with gyrB, rpoD, recA, trpB, and dnaK was established for referring the phylogeny of the species known and other putative novel species.
As mentioned above, 16S rRNA gene as a phylogenetic marker often provides low resolution in differentiation of some strains and species. Mutiple previous studies demonstrated that some species shared >97%, and even 100% of 16S rRNA gene sequences (Das et al., 2014; Yarza et al., 2014; Liu et al., 2015b). Likewise, in this study, we found that the strains affiliated to three species in Clade 1 sharing 99.9% similarities between 16S rRNA gene sequences, which is quite higher than the traditionally recognized threshold of 97% (Stackebrandt and Goebel, 1994), even the latest recommended threshold of 98.65% (Kim et al., 2014). Therefore, we should keep in mind the fact that 16S rRNA gene has been widely used to identify phylogenetic relationships for all microorganisms on earth, but is not good indicator in determining the accurate taxonomic position of some bacteria, for example the members of the genus Thioclava in this study.
In recent years, the MLSA approach has shed new light on prokaryotic systematics and phylogeny (Martens et al., 2008; Vinuesa, 2010; Glaeser and Kämpfer, 2015), and has been extensively used in the classification and identification of diverse bacteria. For example, the phylogenetic relationships of the type strains for 107 species within the genus Pseudomonas was performed using an atypia MLSA based on the four core housekeeping genes (16S rRNA gene, gyrB, rpoB, and rpoD) and on this basis a 97% MLSA similarity was proposed to be as a threshold for species delineation (Mulet et al., 2010). During the process of investigation of the evolution and taxonomy of native mesorhizobia nodulating medicinal Glycyrrhiza species in China, the eight tested strains actually belonged to the same putative new species of the genus Mesorhizobium by MLSA (Ampomah et al., 2017). It was stated that the MLSA scheme can be applied in diverse Streptomyces clades that contain species with high 16S rRNA gene sequence similarities; a 0.007 of nucleotide sequence distance of the five genes corresponding to a 70% DDH value could be considered as the species cut-off for the whole genus (Rong and Huang, 2010, 2012). Similarly, a well-established MLSA scheme for the genus Thioclava in our study proved a powerful method for discrimination of the Thioclava bacteria. Moreover, a 97.3% MLSA similarity was proposed as the soft species threshold of the genus Thioclava based on the correlation analysis between dDDH and MLSA, leading to the discovery of four putative novel species.
Although MLSA is an easy, practical, reliable, and robust technique for identification of bacterial isolates as alternatives to 16S rRNA gene sequence (Glaeser and Kämpfer, 2015), it lacks a uniform cut-off value for identifying them to the species level (Vinuesa, 2010; Glaeser and Kämpfer, 2015). Over the years, many different threshold values of MLSA for species distinction have been recommended in previous studies, for example, 96.16–97.32% for the genus Thalassospira (Lai et al., 2014c), 97.74% for the Bacillus cereus group (Liu et al., 2015b), 97% for the Burkholderia cepacia complex (Peeters et al., 2013) and in the genus Pseudomonas (Mulet et al., 2010), and 97.3% for the genus Thioclava in the present study. Moreover, different housekeeping genes from different strains and with analytical methodologies make it difficult to set up a canonical scheme. In addition, MLSA is limited by a few reference sequences of housekeeping genes against a tremendous amount of commonly used rRNA gene sequences in public database. Therefore, MLSA still needs improvements in order to make the application more feasible and generally applicable in the future. Luckily, the advent of the genome sequencing era and the appearance of dDDH and ANI will contribute to compensate the shortcomings of MLSA (Konstantinidis and Tiedje, 2005; Liu et al., 2015b; Hahnke et al., 2016).
In summary, the bacterial phylogeny and taxonomy of the genus Thioclava were determined by a newly established MLSA in combination with dDDH, ANI, and other approaches in this report. The four novel species have been added to the genus with five other established species. We established a robust framework for the discrimination of Thioclava isolates, which will provide a great avenue for a better understanding their geographic distribution and ecological roles in diverse marine environments.
Description of Thioclava sediminum sp. nov
Thioclava sediminum (se.di.mi′num. L. gen. pl. n. sediminum of sediments, pertaining to source of isolation).
Cells are Gram-staining-negative, aerobic, short-rod-shaped, motile by means of a polar flagellum, 0.6–0.7 μm in width and 1.0–1.2 μm in length (Figure S2). Colonies are light brown-colored, circular, non-translucent, smooth with entire margins, and approximately 1–2 mm in diameter on MA medium after 3 days at 28°C. Growth occurs at 10–43°C (optimum 28–32°C), at pH 6–10 (optimum, pH 7-8), and in 0–12% NaCl (optimum 3%, w/v). Catalase and oxidase are positive. In the API ZYM tests, positive for alkaline phosphatase, esterase (C4), esterase lipase (C8), leucine arylamidase, valine arylamidase, naphthol-AS-BI-phosphohydrolase, acid phosphatase, α-galactosidase, β-galactosidase, α-glucosidase, and β-glucosidase; negative for lipase (C14), cystine arylamidase, trypsin, α-chymotrypsin, β-glucuronidase, N-acetyl-β-Glucosaminidase, α-mannosidase, and α-fucosidase. In the API 20NE tests, positive for nitrate reduction, urease, β-glucosidase (Aesculin hydrolysis), β-galactosidase, utilization of D-glucose, L-arabinose, D-mannose, D-mannitol, D-maltose, potassium gluconate, malic acid, and trisodium citrate; negative for denitrification, indole production, D-glucose fermentation, arginine dihydrolase, gelatin hydrolysis, utilization of N-acetyl-glucosamine, capric acid, adipic acid, and phenylacetic acid. In the API 20E tests, positive for β-galactosidase, citrate utilization and urease; negative for arginine dihydrolase, lysine decarboxylase, ornithine decarboxylase, H2S production, tryptophane deaminase, indole production, acetoin production (Voges Proskauer), gelatinase, acid production of glucose, mannitol, inositol, sorbitol, rhamnose, saccharose, melibiose, amygdalin and arabinose. The major fatty acid is Summed Feature 8. The isoprenoid quinone is Q-10. The polar lipids comprised PE, PG, GL, and PLs.
The type strain, TAW-CT134T (=MCCC 1A10143T = LMG 29615T), was isolated the coastal sediment around Xiamen Island, Fujian province, China. The DNA G+C content of the type strain is 64.0 mol%.
Description of Thioclava marinus sp. nov
Thioclava marinus (ma.ri′nus. L. masc. adj. marinus of the sea, the origin of the sample from which the type strain was isolated).
Cells are Gram-staining-negative, aerobic, short-rod-shaped, non-motile, 0.5–0.6 μm in width and 1.4–1.6 μm in length (Figure S2). Colonies are light yellow-colored, circular, non-translucent, smooth with entire margins and approximately 1–2 mm in diameter on MA medium after 3 days at 28°C. Growth occurs at 10–45°C (optimum 28–32°C), at pH 6-9 (optimum, pH 7-8), and in 0–12% NaCl (optimum 1–3%, w/v). Catalase and oxidase are positive. In the API ZYM tests, positive for alkaline phosphatase, esterase (C4), esterase lipase (C8), lipase (C14), leucine arylamidase, valine arylamidase, acid phosphatase, naphthol-AS-BI-phosphohydrolase, α-glucosidase, and β-glucosidase; negative for cystine arylamidase, trypsin, α-chymotrypsin, α-galactosidase, β-galactosidase, N-acetyl-β-Glucosaminidase, β-glucuronidase, α-mannosidase, and α-fucosidase. In the API 20NE tests, positive for nitrate reduction, denitrification, urease, utilization of D-glucose, D-mannose, D-mannitol, D-maltose, potassium gluconate, and malic acid; negative for indole production, D-glucose fermentation, arginine dihydrolase, β-glucosidase (Aesculin hydrolysis), gelatin hydrolysis, β-galactosidase, utilization of L-arabinose, N-acetyl-glucosamine, capric acid, adipic acid, trisodium citrate, and phenylacetic acid. In the API 20E tests, positive for citrate utilization and urease; negative for β-galactosidase, arginine dihydrolase, lysine decarboxylase, ornithine decarboxylase, H2S production, tryptophane deaminase, indole production, acetoin production (Voges Proskauer), gelatinase, acid production of glucose, mannitol, inositol, sorbitol, rhamnose, saccharose, melibiose, amygdalin, and arabinose. The major fatty acid is Summed Feature 8. The isoprenoid quinone is Q-10. The polar lipids comprised PE, PG, GL, and PLs.
The type strain, 11.10-0-13T (=MCCC 1A03502T = LMG 29618T), was isolated from the coastal seawaters of Yellow Sea, China. The DNA G+C content of the type strain is 64.1 mol%.
Author Contributions
YL and ZS conceived the experiment. YL conducted the experiment, analyzed the result and wrote the paper. YL, QL, and ZS reviewed the manuscript.
Funding
This work was financially supported by the China Ocean Mineral Resources R&D Association program (No. DY135) and National Infrastructure of Microbial Resources of China (NIMR-2017-9).
Conflict of Interest Statement
The authors declare that the research was conducted in the absence of any commercial or financial relationships that could be construed as a potential conflict of interest.
Acknowledgments
We are very grateful these sequence data of the two strains (Thioclava sp. ES.031, Thioclava sp. ES.032) from the US Department of Energy Joint Genome Institute (http://www.jgi.doe.gov/) in collaboration with the user community.
Supplementary Material
The Supplementary Material for this article can be found online at: http://journal.frontiersin.org/article/10.3389/fmicb.2017.01321/full#supplementary-material
References
Ampomah, O. Y., Mousavi, S. A., Lindstrom, K., and Huss-Danell, K. (2017). Diverse Mesorhizobium bacteria nodulate native Astragalus and Oxytropis in arctic and subarctic areas in Eurasia. Syst. Appl. Microbiol. 40, 51–58. doi: 10.1016/j.syapm.2016.11.004
Charlebois, R. L., and Doolittle, W. F. (2004). Computing prokaryotic gene ubiquity: rescuing the core from extinction. Genome Res. 14, 2469–2477. doi: 10.1101/gr.3024704
Collins, M. D. (1985). “Isoprenoid quinone analyses in bacterial classification and identification,” in Society for Applied Bacteriology. Technical Series, eds M. Goodfellow and D. E. Minikin (London, UK: Academic Press), 267–287.
Colston, S. M., Fullmer, M. S., Beka, L., Lamy, B., Gogarten, J. P., and Graf, J. (2014). Bioinformatic genome comparisons for taxonomic and phylogenetic assignments using Aeromonas as a test case. MBio 5:e02136. doi: 10.1128/mBio.02136-14
Darriba, D., Taboada, G. L., Doallo, R., and Posada, D. (2012). jmodeltest 2: more models, new heuristics and parallel computing. Nat. Methods 9:772. doi: 10.1038/nmeth.2109
Das, S., Dash, H. R., Mangwani, N., Chakraborty, J., and Kumari, S. (2014). Understanding molecular identification and polyphasic taxonomic approaches for genetic relatedness and phylogenetic relationships of microorganisms. J. Microbiol. Methods 103, 80–100. doi: 10.1016/j.mimet.2014.05.013
Edgar, R. C. (2004). MUSCLE: multiple sequence alignment with high accuracy and high throughput. Nucleic Acids Res. 32, 1792–1797. doi: 10.1093/nar/gkh340
Felsenstein, J. (1981). Evolutionary trees from DNA sequences: a maximum likelihood approach. J. Mol. Evol. 17, 368–376. doi: 10.1007/BF01734359
Garrido-Sanz, D., Meier-Kolthoff, J. P., Göker, M., Martin, M., Rivilla, R., and Redondo-Nieto, M. (2016). Genomic and genetic diversity within the Pseudomonas fluorescens complex. PLoS ONE 11:e0150183. doi: 10.1371/journal.pone.0150183
Gevers, D., Cohan, F. M., Lawrence, J. G., Spratt, B. G., Coenye, T., Feil, E. J., et al. (2005). Opinion: re-evaluating prokaryotic species. Nat. Rev. Microbiol. 3, 733–739. doi: 10.1038/nrmicro1236
Glaeser, S. P., and Kämpfer, P. (2015). Multilocus sequence analysis (MLSA) in prokaryotic taxonomy. Syst. Appl. Microbiol. 38, 237–245. doi: 10.1016/j.syapm.2015.03.007
Hahnke, R. L., Meier-Kolthoff, J. P., Garcia-Lopez, M., Mukherjee, S., Huntemann, M., Ivanova, N. N., et al. (2016). Genome-based taxonomic classification of bacteroidetes. Front. Microbiol. 7:2003. doi: 10.3389/fmicb.2016.02003
Hurst, L. D. (2002). The Ka/Ks ratio: diagnosing the form of sequence evolution. Trends Genet. 18:486. doi: 10.1016/S0168-9525(02)02722-1
Kates, M. (1986). Techniques of Lipidology. Sole Distributors for the USA and Canada. New York, NY: Elsevier Science Pub. Co.
Katoh, K., and Standley, D. M. (2013). MAFFT multiple sequence alignment software version 7: improvements in performance and usability. Mol. Biol. Evol. 30, 772–780. doi: 10.1093/molbev/mst010
Kim, M., Oh, H. S., Park, S. C., and Chun, J. (2014). Towards a taxonomic coherence between average nucleotide identity and 16S rRNA gene sequence similarity for species demarcation of prokaryotes. Int. J. Syst. Evol. Microbiol. 64, 346–351. doi: 10.1099/ijs.0.059774-0
Kitahara, K., and Miyazaki, K. (2013). Revisiting bacterial phylogeny: natural and experimental evidence for horizontal gene transfer of 16S rRNA. Mob. Genet. Elements 3:e24210. doi: 10.4161/mge.24210
Konstantinidis, K. T., and Tiedje, J. M. (2005). Genomic insights that advance the species definition for prokaryotes. Proc. Natl. Acad. Sci. U.S.A. 102, 2567–2572. doi: 10.1073/pnas.0409727102
Lai, Q., Li, S., Xu, H., Jiang, L., Zhang, R., and Shao, Z. (2014a). Thioclava atlantica sp. nov., isolated from deep sea sediment of the Atlantic Ocean. Antonie Van Leeuwenhoek 106, 919–925. doi: 10.1007/s10482-014-0261-x
Lai, Q., Liu, Y., and Shao, Z. (2014b). Bacillus xiamenensis sp. nov., isolated from intestinal tract contents of a flathead mullet (Mugil cephalus). Antonie Van Leeuwenhoek 105, 99–107. doi: 10.1007/s10482-013-0057-4
Lai, Q., Liu, Y., Yuan, J., Du, J., Wang, L., Sun, F., et al. (2014c). Multilocus sequence analysis for assessment of phylogenetic diversity and biogeography in Thalassospira bacteria from diverse marine environments. PLoS ONE 9:e106353. doi: 10.1371/journal.pone.0106353
Li, C., Lai, Q., Li, G., Liu, Y., Sun, F., and Shao, Z. (2014). Multilocus sequence analysis for the assessment of phylogenetic diversity and biogeography in hyphomonas bacteria from diverse marine environments. PLoS ONE 9:e101394. doi: 10.1371/journal.pone.0101394
Liu, Y., Lai, Q., Dong, C., Sun, F., Wang, L., Li, G., et al. (2013). Phylogenetic diversity of the Bacillus pumilus group and the marine ecotype revealed by multilocus sequence analysis. PLoS ONE 8:e80097. doi: 10.1371/journal.pone.0080097
Liu, Y., Lai, Q., Du, J., and Shao, Z. (2016). Bacillus zhangzhouensis sp. nov. and Bacillus australimaris sp. nov. Int. J. Syst. Evol. Microbiol. 66, 1193–1199. doi: 10.1099/ijsem.0.000856
Liu, Y., Lai, Q., Du, J., and Shao, Z. (2017a). Genetic diversity and population structure of the Bacillus cereus group bacteria from diverse marine environments. Sci. Rep. 7, 689. doi: 10.1038/s41598-017-00817-1
Liu, Y., Lai, Q., Du, J., Xu, H., Jiang, L., and Shao, Z. (2015a). Thioclava indica sp. nov., isolated from surface seawater of the Indian Ocean. Antonie Van Leeuwenhoek 107, 297–304. doi: 10.1007/s10482-014-0320-3
Liu, Y., Lai, Q., Göker, M., Meier-Kolthoff, J. P., Wang, M., Sun, Y., et al. (2015b). Genomic insights into the taxonomic status of the Bacillus cereus group. Sci. Rep. 5:14082. doi: 10.1038/srep14082
Liu, Y., Lai, Q. L., and Shao, Z. Z. (2017b). Thioclava nitratireducens sp. nov., isolated from surface seawater. Int. J. Syst. Evol. Microbiol. doi: 10.1099/ijsem.0.001844. [Epub ahead of print].
Macheras, E., Roux, A. L., Bastian, S., Leão, S. C., Palaci, M., Sivadon-Tardy, V., et al. (2011). Multilocus sequence analysis and rpoB sequencing of Mycobacterium abscessus (sensu lato) strains. J. Clin. Microbiol. 49, 491–499. doi: 10.1128/JCM.01274-10
Martens, M., Dawyndt, P., Coopman, R., Gillis, M., De Vos, P., and Willems, A. (2008). Advantages of multilocus sequence analysis for taxonomic studies: a case study using 10 housekeeping genes in the genus Ensifer (including former Sinorhizobium). Int. J. Syst. Evol. Microbiol. 58, 200–214. doi: 10.1099/ijs.0.65392-0
Martin, D. P., Murrell, B., Golden, M., Khoosal, A., and Muhire, B. (2015). RDP4: detection and analysis of recombination patterns in virus genomes. Virus Evol. 1:vev003. doi: 10.1093/ve/vev003
Meier-Kolthoff, J. P., Auch, A. F., Klenk, H. P., and Göker, M. (2013). Genome sequence-based species delimitation with confidence intervals and improved distance functions. BMC Bioinformatics 14:60. doi: 10.1186/1471-2105-14-60
Mulet, M., Lalucat, J., and Garcia-Valdes, E. (2010). DNA sequence-based analysis of the Pseudomonas species. Environ. Microbiol. 12, 1513–1530. doi: 10.1111/j.1462-2920.2010.02181
Peeters, C., Meier-Kolthoff, J. P., Verheyde, B., De Brandt, E., Cooper, V. S., and Vandamme, P. (2016). Phylogenomic study of Burkholderia glathei-like organisms, proposal of 13 novel Burkholderia species and emended descriptions of Burkholderia sordidicola, Burkholderia zhejiangensis, and Burkholderia grimmiae. Front. Microbiol. 7:877. doi: 10.3389/fmicb.2016.00877
Peeters, C., Zlosnik, J. E., Spilker, T., Hird, T. J., LiPuma, J. J., and Vandamme, P. (2013). Burkholderia pseudomultivorans sp. nov., a novel Burkholderia cepacia complex species from human respiratory samples and the rhizosphere. Syst. Appl. Microbiol. 36, 483–489. doi: 10.1016/j.syapm.2013.06.003
Posada, D., and Buckley, T. R. (2004). Model selection and model averaging in phylogenetics: advantages of akaike information criterion and bayesian approaches over likelihood ratio tests. Syst. Biol. 53, 793–808. doi: 10.1080/10635150490522304
Richter, M., and Rossello-Mora, R. (2009). Shifting the genomic gold standard for the prokaryotic species definition. Proc. Natl. Acad. Sci. U.S.A. 106, 19126–19131. doi: 10.1073/pnas.0906412106
Rong, X., and Huang, Y. (2010). Taxonomic evaluation of the Streptomyces griseus clade using multilocus sequence analysis and DNA-DNA hybridization, with proposal to combine 29 species and three subspecies as 11 genomic species. Int. J. Syst. Evol. Microbiol. 60, 696–703. doi: 10.1099/ijs.0.012419-0
Rong, X., and Huang, Y. (2012). Taxonomic evaluation of the Streptomyces hygroscopicus clade using multilocus sequence analysis and DNA-DNA hybridization, validating the MLSA scheme for systematics of the whole genus. Syst. Appl. Microbiol. 35, 7–18. doi: 10.1016/j.syapm.2011.10.004
Rowe, A. R., Chellamuthu, P., Lam, B., Okamoto, A., and Nealson, K. H. (2015). Marine sediments microbes capable of electrode oxidation as a surrogate for lithotrophic insoluble substrate metabolism. Front. Microbiol. 5:784. doi: 10.3389/fmicb.2014.00784
Sasser, M. (1990). Identification of Bacteria by Gas Chromatography of Cellular Fatty Acids, MIDI Technical Note 101. Newark, DE: MIDI Inc.
Silvestro, D., and Michalak, I. (2012). Raxmlgui: a graphical front-end for RAxML. Org. Divers. Evol. 12, 335–337. doi: 10.1007/s13127-011-0056-0
Sorokin, D. Y., Tourova, T. P., Spiridonova, E. M., Rainey, F. A., and Muyzer, G. (2005). Thioclava pacifica gen. nov., sp. nov., a novel facultatively autotrophic, marine, sulfur-oxidizing bacterium from a near-shore sulfidic hydrothermal area. Int. J. Syst. Evol. Microbiol. 55, 1069–1075. doi: 10.1099/ijs.0.63415-0
Stackebrandt, E., and Goebel, B. (1994). Taxonomic note: a place for DNA-DNA reassociation and 16S rRNA sequence analysis in the present species definition in bacteriology. Int. J. Syst. Evol. Microbiol. 44, 846–849. doi: 10.1099/00207713-44-4-846
Tamura, K., Peterson, D., Peterson, N., Stecher, G., Nei, M., and Kumar, S. (2011). MEGA5: molecular evolutionary genetics analysis using maximum likelihood, evolutionary distance, and maximum parsimony methods. Mol. Biol. Evol. 28, 2731–2739. doi: 10.1093/molbev/msr121
Tian, R. M., Cai, L., Zhang, W. P., Cao, H. L., and Qian, P. Y. (2015). Rare events of intragenus and intraspecies horizontal transfer of the 16S rRNA gene. Genome Biol. Evol. 7, 2310–2320. doi: 10.1093/gbe/evv143
Timilsina, S., Jibrin, M. O., Potnis, N., Minsavage, G. V., Kebede, M., Schwartz, A., et al. (2015). Multilocus sequence analysis of xanthomonads causing bacterial spot of tomato and pepper plants reveals strains generated by recombination among species and recent global spread of Xanthomonas gardneri. Appl. Environ. Microbiol. 81, 1520–1529. doi: 10.1128/AEM.03000-14
Vinuesa, P. (2010). “Multilocus sequence analysis and bacterial species phylogeny” in Molecular Phylogeny of Microorganisms, eds O. Aharon and R. Thane Papke (Caister: Academic Press), 45–64.
Wang, J., Dong, C., Lai, Q., Lin, L., and Shao, Z. (2012). Diversity of C16H33Cl-degrading bacteria in surface seawater of the Arctic Ocean. Acta Microbiol. Sin. 52, 1011–1020. doi: 10.13343/j.cnki.wsxb.2012.08.011
Wang, W., Zhang, R., Shan, D., and Shao, Z. (2014). Indigenous oil-degrading bacteria in crude oil-contaminated seawater of the Yellow sea, China. Appl. Microbiol. Biotechnol. 98, 7253–7269. doi: 10.1007/s00253-014-5817-1
Wayne, L., Brenner, D., Colwell, R., Grimont, P., Kandler, O., Krichevsky, M., et al. (1987). Report of the ad hoc committee on reconciliation of approaches to bacterial systematics. Int. J. Syst. Evol. Microbiol. 37, 463–464. doi: 10.1099/00207713-37-4-463
Whatmore, A. M., Koylass, M. S., Muchowski, J., Edwards-Smallbone, J., Gopaul, K. K., and Perrett, L. L. (2016). Extended multilocus sequence analysis to describe the global population structure of the genus Brucella: phylogeography and relationship to biovars. Front. Microbiol. 7:2049. doi: 10.3389/fmicb.2016.02049
Xia, X. (2013). DAMBE5: a comprehensive software package for data analysis in molecular biology and evolution. Mol. Biol. Evol. 30, 1720–1728. doi: 10.1093/molbev/mst064
Yarza, P., Yilmaz, P., Pruesse, E., Glöckner, F. O., Ludwig, W., Schleifer, K. H., et al. (2014). Uniting the classification of cultured and uncultured bacteria and archaea using 16S rRNA gene sequences. Nat. Rev. Microbiol. 12, 635–645. doi: 10.1038/nrmicro3330
Keywords: Thioclava, MLSA, genome, dDDH, polyphasic taxonomy
Citation: Liu Y, Lai Q and Shao Z (2017) A Multilocus Sequence Analysis Scheme for Phylogeny of Thioclava Bacteria and Proposal of Two Novel Species. Front. Microbiol. 8:1321. doi: 10.3389/fmicb.2017.01321
Received: 28 April 2017; Accepted: 29 June 2017;
Published: 13 July 2017.
Edited by:
Frank T. Robb, University of Maryland, Baltimore, United StatesReviewed by:
Alfons Stams, Wageningen University and Research, NetherlandsMatthew S. Fullmer, University of Connecticut, United States
Juan M. Gonzalez, Consejo Superior de Investigaciones Científicas (CSIC), Spain
Copyright © 2017 Liu, Lai and Shao. This is an open-access article distributed under the terms of the Creative Commons Attribution License (CC BY). The use, distribution or reproduction in other forums is permitted, provided the original author(s) or licensor are credited and that the original publication in this journal is cited, in accordance with accepted academic practice. No use, distribution or reproduction is permitted which does not comply with these terms.
*Correspondence: Zongze Shao, shaozz@163.com