- 1Department of Plant Agriculture, University of Guelph, Guelph, ON, Canada
- 2Department of Microbiology and Immunology, Faculty of Pharmacy, Damanhour University, Damanhour, Egypt
The cucurbit vegetables, including cucumbers, melons and pumpkins, have been cultivated for thousands of years without fungicides. However, their seed germination stage is prone to be infected by soil-borne fungal and oomycete pathogens. Endophytes are symbionts that reside inside plant tissues including seeds. Seed endophytes are founders of the juvenile plant microbiome and can promote host defense at seed germination and later stages. We previously isolated 169 bacterial endophytes associated with seeds of diverse cultivated cucurbits. We hypothesized that these endophytes can antagonize major fungal and oomycete pathogens. Here we tested the endophytes for in vitro antagonism (dual culture assays) against important soil-borne pathogens (Rhizoctonia solani, Fusarium graminearum, Phytophthora capsici, Pythium aphanidermatum). The endophytes were also assayed in planta (leaf disk and detached leaf bioassays) for antagonism against a foliar pathogen of global importance, Podosphaera fuliginea, the causative agent of cucurbit powdery mildew. The endophytes were further tested in vitro for secretion of volatile organic compounds (VOCs) known to induce plant defense. Extracellular ribonuclease activity was also tested, as a subset of pathogenesis-related (PR) proteins of plant hosts implicated in suppression of fungal pathogens, displays ribonuclease activity. An unexpected majority of the endophytes (70%, 118/169) exhibited antagonism to the five phytopathogens, of which 68% (50/73) of in vitro antagonists belong to the genera Bacillus and Paenibacillus. All Lactococcus and Pantoea endophytes exhibited anti-oomycete activity. However, amongst the most effective inoculants against Podosphaera fuliginea were Pediococcus and Pantoea endophytes. Interestingly, 67% (113/169) of endophytes emitted host defense inducing VOCs (acetoin/diacetyl) and 62% (104/169) secreted extracellular ribonucleases in vitro, respectively. These results show that seeds of cultivated cucurbits package microbes with significant disease-suppression potential. As seeds can act as vectors for genetic transmission of endophytes across host generations, it is interesting to hypothesize whether humans, when selecting seeds of healthy hosts, may have inadvertently selected for disease-suppressing seed endophytes. As the majority of pathogen-suppressing endophytes belong to Bacillus and Paenibacillus, and since Bacilli are widely used as commercial biocontrol agents of vegetables, we propose that these agents are mimicking the ecological niche established by their endophytic cousins.
Introduction
Endophytes including fungi and bacteria are symbionts that colonize the internal tissues of plants without eliciting disease symptoms. They can act as probiotics for their hosts contributing to host fitness and diversity (Ruiza et al., 2011; Hardoim et al., 2015). The endophytic microbiome can improve plant growth and health through four main routes including: (1) protection from biological enemies via production of antimicrobial metabolites and stimulation of plant defense responses; (2) enhancement of host tolerance to abiotic stress; (3) promotion of nutrient acquisition; and (4) secretion of plant growth promoting phytohormones (Berg, 2009; Truyens et al., 2015; Bacon and White, 2016; Khalaf and Raizada, 2016). Beneficial bacteria can emit diverse volatile compounds (VOCs) as signaling molecules to regulate plant growth and immunity in response to biotic and abiotic stresses (Bitas et al., 2013; Asari et al., 2016; Chung et al., 2016). These VOCs, including acetoin and diacetyl, exert their signaling functions by stimulating the transcription of plant host genes employed in metabolic, physiological and defensive activities (Asari et al., 2016). As part of their cascade of defense responses, plants can secrete pathogenesis related proteins (PR proteins), including those with ribonuclease (RNase) activity, that can directly antagonize fungal phytopathogens by penetrating fungal cells or by inducing programmed cell death of plant cells under attack (Filipenko et al., 2013; Miranda et al., 2017; Moosa et al., 2017), though whether endophytes supplement this host activity is not clear.
Beneficial microbes colonizing healthy seeds have the potential to establish the plant microbiome following germination, providing their host plants with nutritional and defensive functions (Johnston-Monje and Raizada, 2011; Truyens et al., 2015; Khalaf and Raizada, 2016; Mitter et al., 2016). Seeds may serve as vectors to transmit endophytes across plant host generations (Hardoim et al., 2015), demonstrated by the relative conservation of seed endophytic diversity across wild plant ancestors and their cultivated domesticates (Johnston-Monje and Raizada, 2011). Though relatively unexploited, seed endophytes are starting to be recognized as promising sources of microbial inoculants, including as biofertilizers (Ruiza et al., 2011; Truyens et al., 2015; Khalaf and Raizada, 2016) but more recently as biocontrol agents (Berg et al., 2017).
The cucurbit plant family is taxonomically divided into 118–122 genera including 940–980 species that evolutionarily span the world’s continents (Renner et al., 2007; Schaefer et al., 2009; Sebastian et al., 2010; Heneidak and Khalik, 2015; Khalaf and Raizada, 2016; Paris, 2016a). Cucurbits are popular vegetable crops, of which the most economically important globally are cucumber (Cucumis sativus), melon (Cucumis melo), watermelon (Citrullus lanatus), pumpkin and squash (Cucurbita sp.). Other cucurbit genera such as Luffa and Lagenaria are popularly utilized in particular regions of the world (Robinson and Decker-Walters, 1997; Specht et al., 2014; Lebeda et al., 2016; Paris, 2016b). The archaeological remains (mostly seeds) of wild progenitors of major cucurbits reflect their diverse origins spanning the Old World (Africa: watermelon; Asia: melon and cucumber) and the New World (Americas: pumpkins and squash) (Figure 1) (Paris, 2016b) including the tropics and humid sub-tropics (Sebastian et al., 2010; Paris, 2016b; Pető et al., 2016; Kates et al., 2017), which are regions that favor oomycete and fungal pathogens.
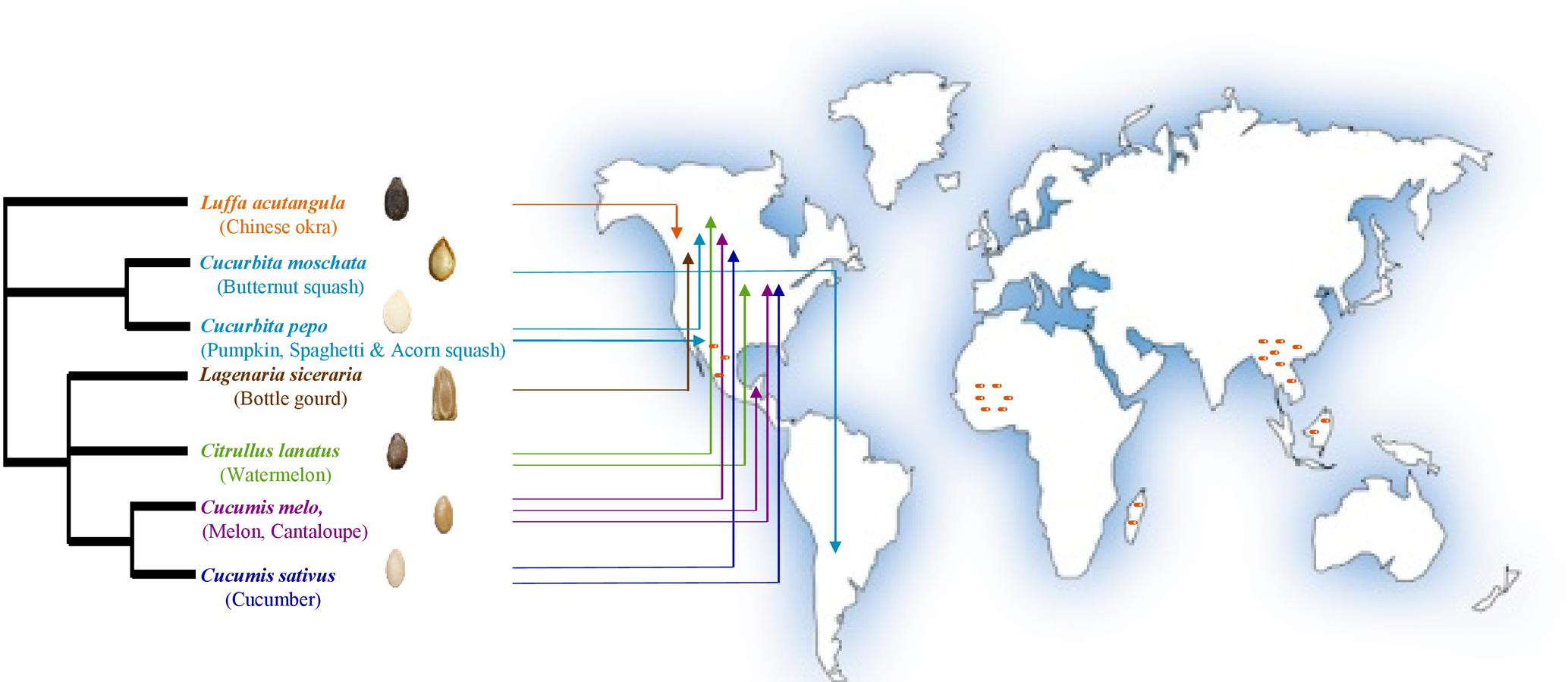
FIGURE 1. Geographic origins of the cucurbit seeds used in the current study as sources of endophytic bacteria (adapted from Khalaf and Raizada, 2016). Arrows point to the direct geographical origins of seeds and/or fruits. Dark orange dashes refer to the hotspots of cucurbit diversification based on archaeological remains. PhyloT phylogenetic tree online generator was used to construct the phylogenetic tree based on NCBI taxonomic data.
As with all plants, cucurbit seeds germinate in the soil, and early seed germination is prone to serious soil-derived pathogens that influence seedling survival. Soil pathogens particularly fungi and oomycetes threaten global crop production and food security (Strange and Scott, 2005; Heydari and Pessarakli, 2010; Pliego et al., 2011; Lamichhane et al., 2017). In general, 14.4% of cultivated crops globally are lost every year due to plant diseases with total losses estimated at $220 billion USD (Agrios, 2005; Kandel et al., 2017). Among the most economically important soil-borne oomycetes are Phytophthora spp. and Pythium spp., while major soil-borne fungal pathogens include Rhizoctonia solani and Fusarium spp. (Pliego et al., 2011). These soil-borne phytopathogens can infect a wide range of hosts and are able to exist as saprophytes, explaining their ability to spread through soil even in the absence of a host plant (Lamichhane et al., 2017). Today, there is interest in understanding the interactions between the plant microbiome and oomycetes as a major step toward protecting plants against oomycetous infections (Larousse and Galiana, 2017). Although the oomycete Phytopthora capsici was first identified as a chili pepper pathogen in Mexico in 1922, it was subsequently discovered to be a devastating vegetable crop pathogen infecting numerous species of plants in different plant families such as the Solanaceae (e.g., potato, tomato) and cucurbit families (Hausbeck and Lamour, 2004). Pythium sp. enter cucumber roots through the root hairs (Yang et al., 2004), and within this genus, Pythium aphanidermatum causes dramatic losses in greenhouse cucumbers in particular when using hydroponic systems due to the resulting root rot (Punja and Yip, 2003). Among the fungal pathogens of crops, R. solani is devastating and difficult to manage as the fungus develops sclerotia and is difficult to control chemically. The fungus is normally found in low densities in the soil as a saprotrophic and facultative parasite that enables it to infect a broad spectrum of plant species causing serious losses to trees, major grain and vegetable crops including cucurbits, in particular cucumber in both field and greenhouse production (Val-Moraes, 2015; Karimi et al., 2016; Justyna et al., 2017). Fusarium spp. such as F. oxysporum are known to cause root rot in cucurbits, however F. graminearum is ranked fourth among the top 10 most severe fungal pathogens of crops based on voting by the international research community (Dean et al., 2012). Biocontrol of F. graminearum in cereals is of global interest due to its serious detrimental effect of reducing the grain quality and producing mycotoxins (Mousa et al., 2016).
At later stages following germination, plants are vulnerable to airborne pathogens. In cucurbits, powdery mildew is an airborne foliar disease of global importance in field and greenhouse production, leading to serious yield losses and poor quality fruits. Infection frequently occurs by two air-borne obligate pathogens, Golovinomyces orontii and Podosphaera xanthii (Braun and Cook, 2012; Lebeda et al., 2016). Podosphaera xanthii, previously known as Sphaerotheca fusca or Sphaerotheca fulginiea, is frequently reported in warmer climates such as subtropical and tropical regions and under greenhouse conditions (Cohen, 1993; Shishkoff, 2000; Lebeda et al., 2016).
Primary screening of potentially beneficial microbes for antagonism of culturable phytopathogens is typically performed using the in vitro dual culture assay where the pathogen target and the candidate biocontrol agents are cultured together to assay for direct pathogen growth suppression (Pliego et al., 2011; Bosmans et al., 2016). This method has been shown to predict their interactions in planta (Shehata et al., 2016). As this option is not available for obligate pathogens such as P. xanthii, leaf disk and detached plant organ assays are used where the pathogen is maintained on plant tissues in Petri dishes supplemented with water and nutrients. These in planta assays have been used to screen for candidates as biocontrol agents and their extracts against powdery mildew in cucumber (Cohen, 1993; Lebeda et al., 2016).
We previously reported an extensive collection of seed associated bacterial endophytes from cultivated cucurbit crops along with their exhibited plant growth promoting activity but we did not test them for potential biocontrol traits (Khalaf and Raizada, 2016). Given the cultivation of cucurbits over thousands of years in warm, moist environments, including more recently in greenhouses, here we used the endophyte library to test the hypothesis that endophytes associated with seeds of the world’s human-selected cucurbits have the ability to restrain soil-borne oomycete and fungal pathogens (the culturable pathogens, P. capsici, P. aphanidermatum, R. solani and F. graminearum) known to infect cucurbits and other plants following seed germination. We also tested whether the endophytes can suppress the later cucumber foliar disease, powdery mildew, caused by the airborne fungal pathogen Podosphaera xanthii. For the culturable pathogens, the in vitro dual culture assay was used. For the obligate pathogen, the leaf disk and detached whole leaf assays were used. To test for the potential ability of the endophytes to stimulate host defense responses, they were in vitro screened for secretion of the VOC compounds, acetoin and diacetyl. The endophyte library was also screened for extracellular ribonuclease activity.
Results
Predominance of Endophytic Functional Traits Known to Promote Plant Disease Resistance
A majority of the cucurbit seed associated endophytes expressed functional traits known to enhance plant disease resistance. Approximately 67% (113/169) and 62% (104/169) of the tested endophytic bacteria produced acetoin and/or diacetyl in vitro and displayed ribonuclease (RNase) activity, respectively (Figures 2, 3 and Supplementary Table S1). Cucumber seeds, specifically, were the primary source of the positive candidates accounting for 44% (49/113) of the acetoin/diacetyl producers and 37% (38/104) of strains with RNase activity. The genus Bacillus accounted for 59% (67/113) of acetoin/diacetyl producers and 73% (76/104) of RNase positive isolates. Furthermore, the vast majority of the 83 Bacillus isolates displayed acetoin/diacetyl activity (81%, 67/83) and RNAse activity (92%, 76/83) (Figure 3). In addition, 49% (18/37) and 27% (10/37) of the Paenibacillus isolates demonstrated acetoin/diacetyl production and RNase activity in vitro, respectively. Regarding the identified Enterobacteriaceae family members, 75% (15/20) were acetoin/ diacetyl producers and 30% (6/20) showed RNase activity. All isolated bacterial endophytes of the genera Cronobacter, Pantoea, Microbacterium, and Staphylococcus displayed these traits.
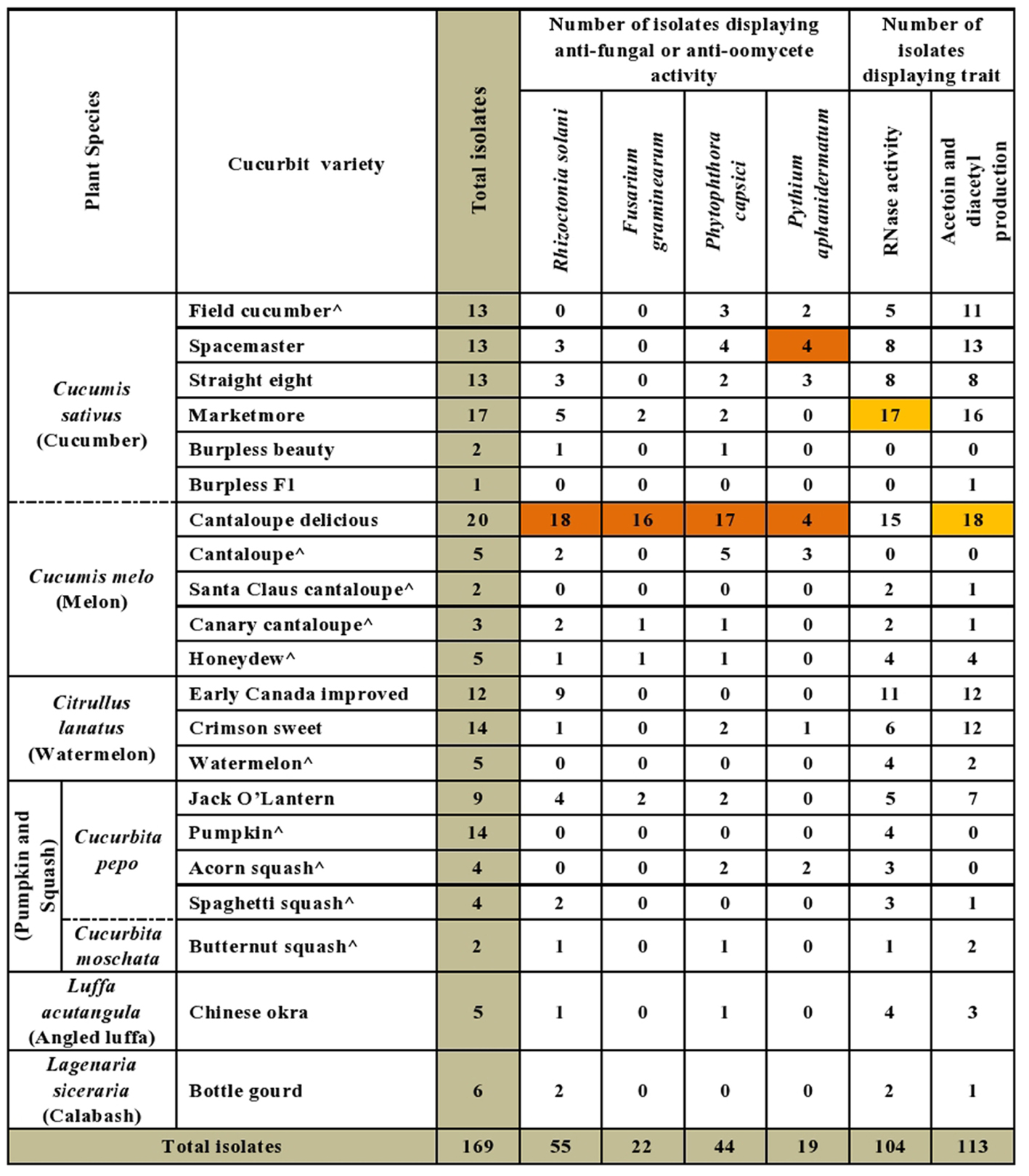
FIGURE 2. Summary of in vitro antagonistic and functional activities displayed by the endophytic bacteria associated with cucurbit seeds, organized by host taxonomy. Each orange highlight denotes the crop variety that possesses the greatest number of endophytes that display the corresponding beneficial functional trait (vertical column) in vitro. The circumflex accent (ˆ) denotes that fresh fruits were used as the seed source.
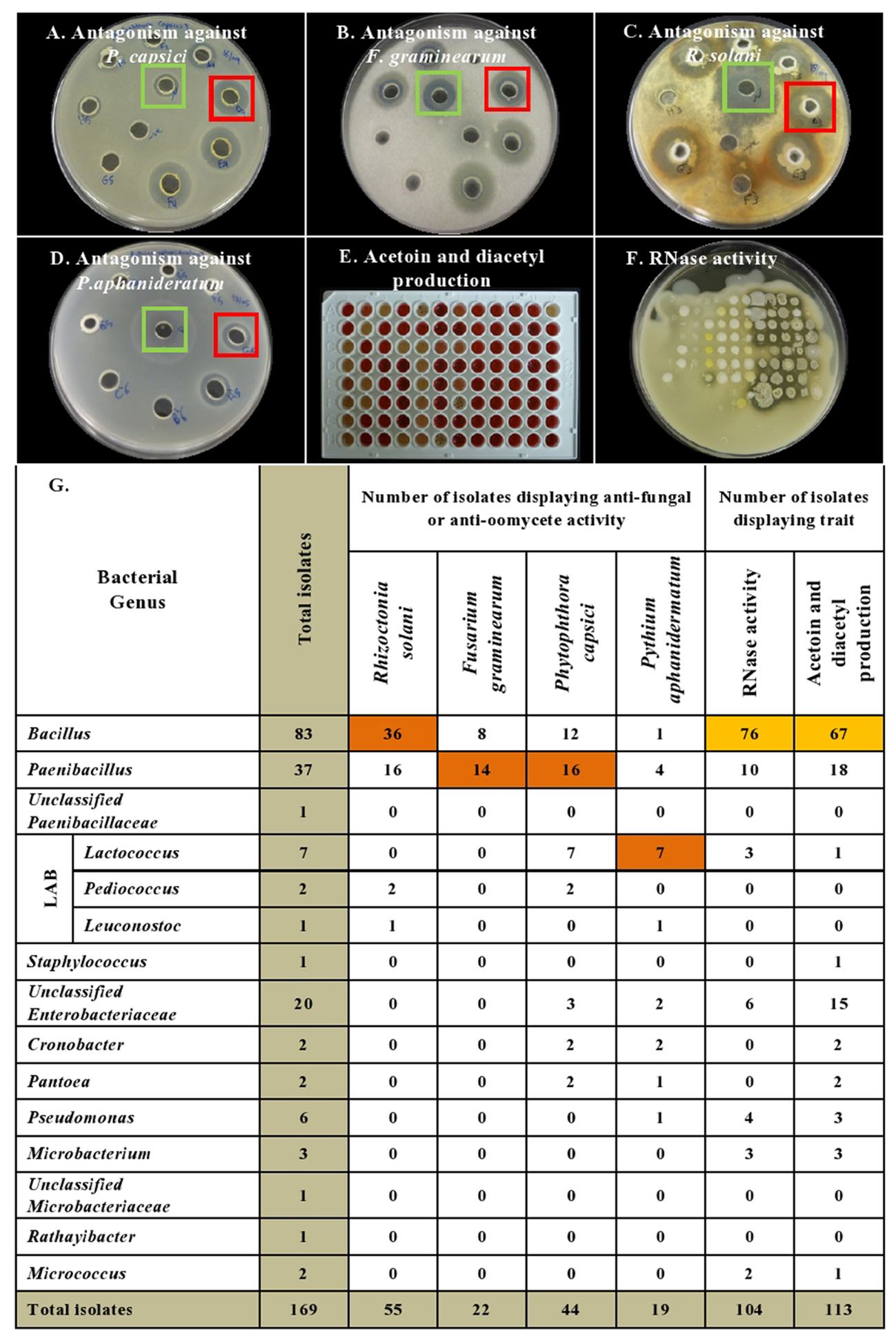
FIGURE 3. Summary of in vitro antagonistic and functional activities displayed by the endophytic bacteria associated with cucurbit seeds, organized by bacterial taxonomy. (A–D) Examples of endophytes that antagonize the growth of pathogens in vitro using the disk diffusion technique. Green squares indicate positive controls (fungicide), while red squares indicate antagonistic endophytes. Shown are antagonism assays for: (A) Phytophthora capsici, (B) Fusarium graminearum, (C) Rhizoctonia solani, and (D) Pythium aphanidermatum. (E,F) Examples of endophytes that display functional traits known to promote host plant defense, specifically: (E) Acetoin and/or diacetyl production (red/pink is a positive result), and (F) RNase activity (clear halo around colony is a positive result). (G) Summary of the bacterial endophytes that display the tested in vitro activities. Each orange highlight denotes the bacterial genus that possesses the greatest number of endophytes that display the corresponding beneficial functional trait (vertical column) in vitro.
Predominance of in Vitro Anti-pathogenic Activity of Cultivated Cucurbit Seed-Associated Endophytes
The bacterial endophytes were tested for their ability to suppress growth in vitro of a spectrum of plant pathogens with diverse host ranges including cucurbits. In total, 43% (73/169) of isolates showed anti-pathogenic traits in vitro including against both oomycete pathogens (P. capsici, P. aphanidermatum) and fungal pathogens (R. solani, F. graminearum) of which F. graminearum (control) is not known to cause disease in cucurbits (Figure 2). For each pathogen tested, antagonistic endophytes were identified that originated from diverse, major cucurbits. For example, the potential R. solani antagonists originated from the five cucurbit genera and 15 different varieties. The seeds of diverse varieties of melon (C. melo), in particular variety Cantaloupe delicious (Figure 2 and Supplementary Table S1), hosted the highest number and broadest spectrum (fungal, oomycetes) of in-vitro antagonistic endophytic bacteria. For example, 82% (18/22) of cucurbit family endophytes that displayed anti-F. graminearum activity originated from melon seed; this number was 55% (24/44) for P. capsici and 42% (23/55) for R. solani (Supplementary Table S1). Despite desiccation and long-term storage, dry seeds (variety names without asterisks, Figure 2 and Supplementary Table S1) were the main supplier of bacterial endophytes in this study (113/169) and constituted the majority of the antagonists (77%, 56/73 of antagonists). Fresh seeds were also sources of antagonists, however, (e.g., melon, C. melo; Figure 2 and Supplementary Table S1).
With respect to the tested fungal pathogens, of the 55 strains that antagonized R. solani in vitro, 52 belonged to the genera Bacillus and Paenibacillus though they only constituted 71% (120/169) of the endophyte library (Figure 3 and Supplementary Table S1). Similarly, of the 22 strains that antagonized F. graminearum, all belonged to Bacillus and Paenibacillus. By contrast of the 20 Enterobacteriaceae endophytes tested, none suppressed the fungal pathogens (Figure 3 and Supplementary Table S1).
A broader taxonomic spectrum of endophytes suppressed growth of the tested oomycete pathogens. Nevertheless, of the 44 endophytes that suppressed growth of P. capsici in vitro, 64% (28/44) belonged to Bacillus and Paenibacillus, with the remainder belonging to 5 other genera including Lactococcus (16%, 7/44). There were 19 taxonomically diverse strains that suppressed P. aphanidermatum in vitro, of which Lactococcus endophytes were the most prevalent (41%, 7/19), whereas only 5 Bacillus and Paenibacillus strains (combined) were antagonists. It is noteworthy that all 7 isolates of the genus Lactococcus showed in vitro anti-oomycete activity (Figure 3 and Supplementary Table S1).
Leaf Disk Bioassay for Antagonism against Cucumber Powdery Mildew
The endophytes were also tested for antagonism against Podosphaera fuliginea, the causative agent of cucumber powdery mildew disease. As the pathogen is obligate, the bioassay employed leaf disks (Figures 4A–D). The disease index (DI%) and preventive effect (PE%) were calculated for all tested endophytes (Supplementary Tables S2B,C). In total, 37% (62/169) of the tested endophytic bacteria demonstrated significant suppression of powdery mildew symptoms (p < 0.05, mean DI%) in comparison to disks treated with LB media (negative control) (Figures 4D, 5A and Supplementary Tables S3, S4). Noteworthy, these promising 62 strains originated from the five tested cucurbit genera (Figure 5B and Supplementary Table S2A). Interestingly, most of the remaining endophytes displayed adverse effects on cucumber leaf health in the presence of the pathogen (Figure 4E and Supplementary Tables S2A–C). The beneficial endophytes displayed mean positive PE values of up to 89%. Of these beneficial strains, 66% (41/62) and 79% (49/62) were statistically equal or more effective (One-Way ANOVA Dunnett’s multiple comparisons) than the fungicide, prothioconazole [(positive control, concentration optimized in a pre-experiment (see Materials and Methods; data not shown)] (Figure 4A) and the biofungicide, Bacillus subtilis strain QST 713 (Figure 4B), respectively.
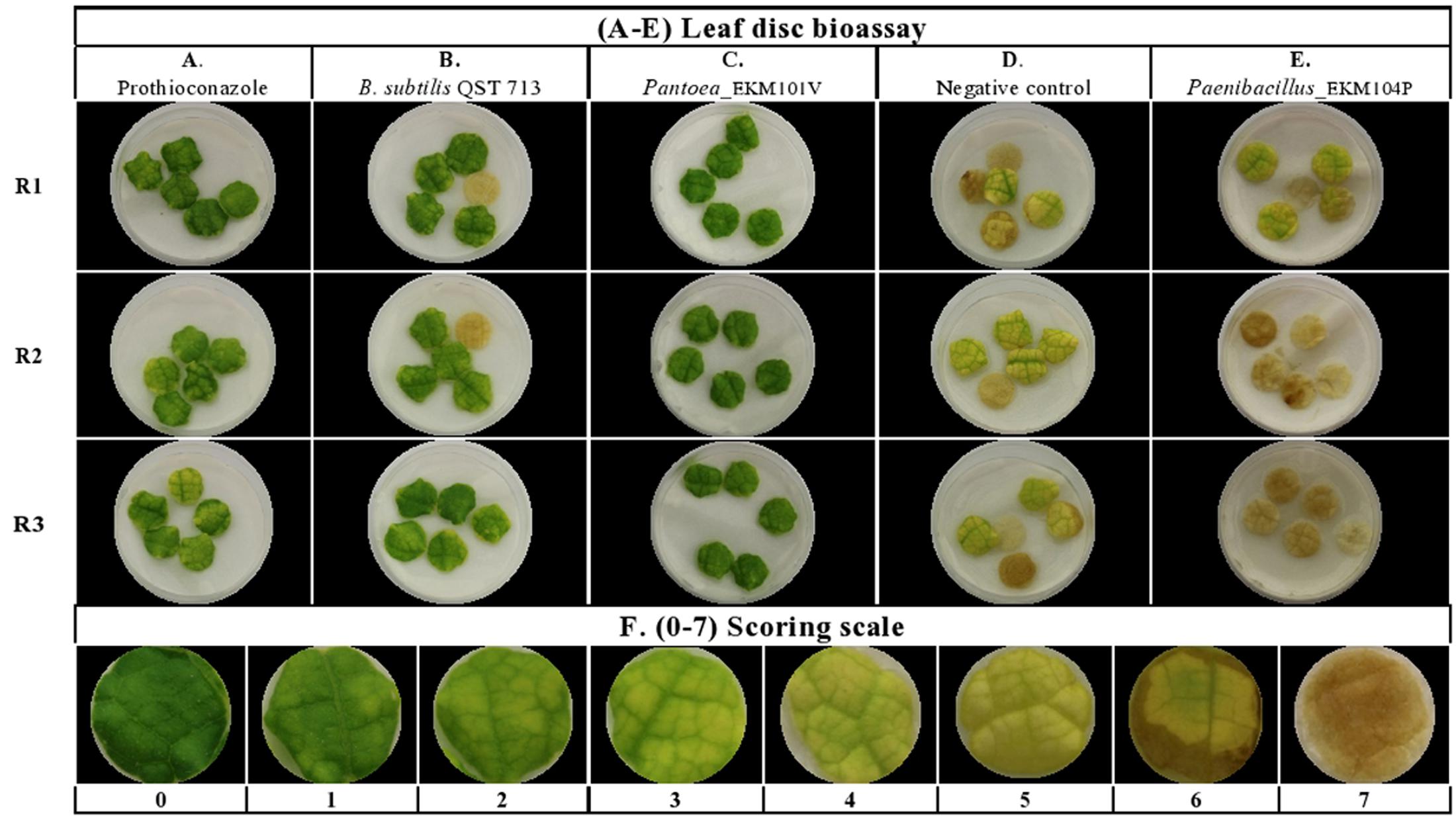
FIGURE 4. Screening of cucurbit seed associated endophytic bacteria for antagonism against cucumber powdery mildew disease using a leaf disk bioassay. (A–E) Representative pictures of: (A) chemical fungicide positive control (Prothioconazole), (B) commercial biocontrol agent positive control (Bacillus subtilis strain QST 713), (C) example of a typical promising endophytic bacteria; Pantoea EKM101V, (D) the negative control (LB broth amended with filter sterilized 0.01% Tween 20) and (E) example of an endophytic bacteria displaying adverse effects in the presence of the pathogen. Paenibacillus EKM104P. (F) Represents pictures of 0–7 scoring scale used as references to guide the visual disease assessments. R (1–3) denotes replicate number.
Unlike in vitro antimicrobial activity, fresh cucurbit seeds were the major sources (61%, 38/62) of antagonists that significantly suppressed powdery mildew in planta (Supplementary Tables S2A, S3). Furthermore, cucumber, pumpkin, cantaloupe and watermelon seeds contributed many of the antagonists, accounting for 23% (14/62), 19% (12/62), 18% (11/62) and 16% (10/62) of the significantly (p < 0.05) effective endophytes, respectively. With respect to endophytic taxonomy, the strains that significantly restrained symptoms of the disease belonged to 14 different bacterial genera (Figure 5B and Supplementary Tables S3, S4), of which the most prevalent (in rank order) were Bacillus (37%, 23/62), Paenibacillus (16%, 10/62) and Unclassified Enterobacteriaceae (14.5%, 9/62). However, amongst the most effective antagonists were Pediococcus and Pantoea endophytes (Figure 4C).
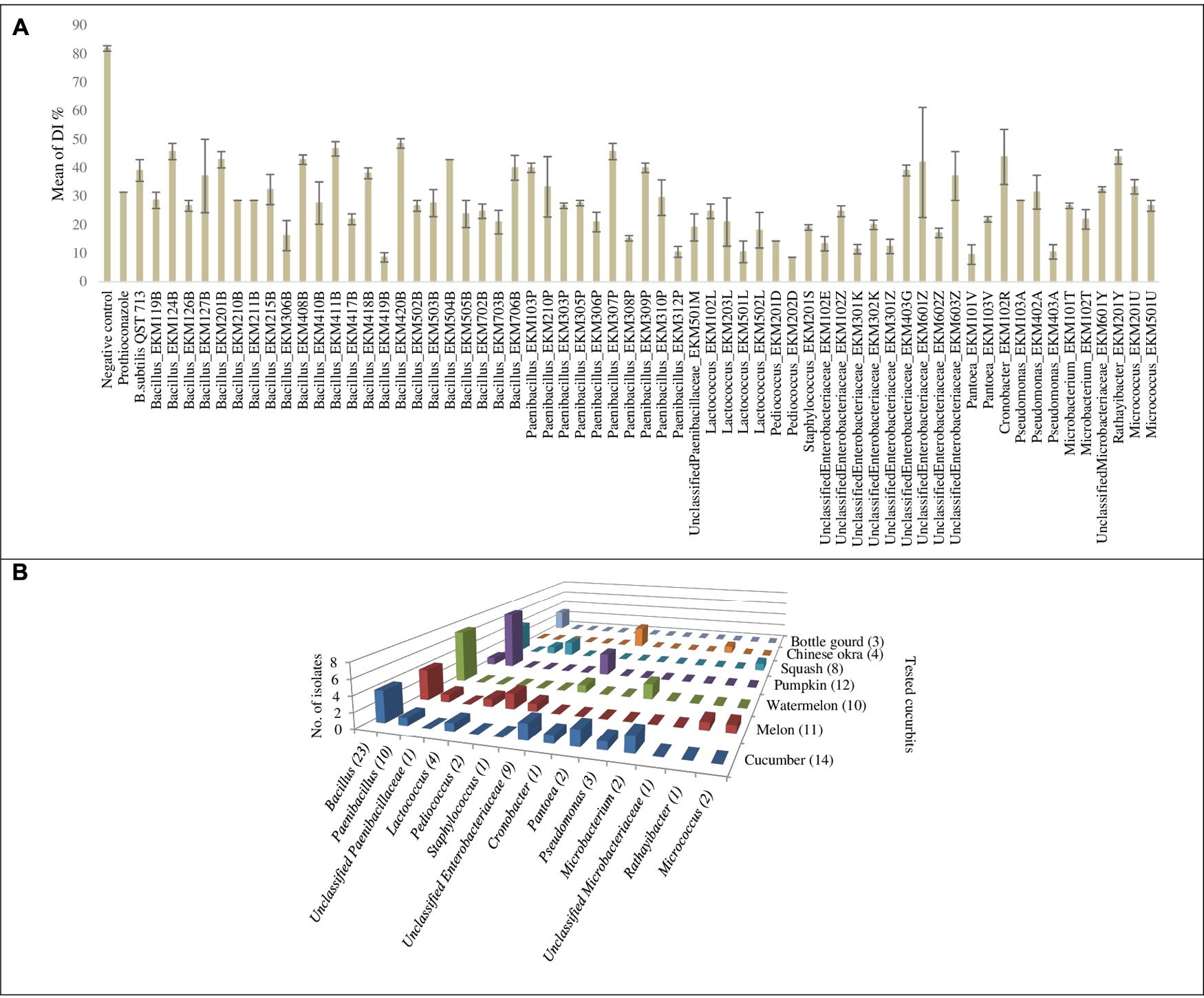
FIGURE 5. Graphical representation for endophytic bacteria that exhibited significant suppression of powdery mildew in the leaf disk bioassay: (A) Graphical representation of calculated means of the disease index percentage (DI%) displayed by controls and endophytic bacteria that exhibited significant suppression of powdery mildew (p < 0.05) in the leaf disk bioassay. Error bars show the standard error of mean (SEM). (B) Graph chart summarizing endophytic bacterial genera that exhibited significant suppression of powdery mildew symptoms at p < 0.05, cross referenced with the original endophyte seed sources. Numbers between brackets displayed on the horizontal axis denote the total number of strains in each corresponding bacterial genus that showed antagonism. Numbers between brackets displayed on the depth axis denote the total number of strains identified from each corresponding cucurbit crop that showed antagonism. For detailed information by endophyte strain, see Supplementary Tables S3, S4.
Detached Whole Leaf Bioassay for Antagonism against Cucumber Powdery Mildew
To independently validate the leaf disk results and to make the assay more robust, the endophytes that were effective against powdery mildew in comparison to prothioconazole fungicide (41 strains, Supplementary Table S2D) were re-tested using detached whole cucumber leaves infected with the pathogen (Figures 6A–E and Supplementary Figure S1). In total, 66% (27/41) of endophytes were confirmed to inhibit powdery mildew symptoms visually in comparison to leaves treated with LB media (negative control), with PE values ranging from 8–46% (Figure 6E, Supplementary Figures S1A–E, and Supplementary Table S2D). However, only six strains showed significant suppression of powdery mildew disease when compared to the negative control (independent t-test, one-tailed, unequal variance, using visual scores at p < 0.1) (Figure 6E and Supplementary Figure S1). As above, the majority of the remaining endophytes displayed adverse effects on cucumber leaf health in the presence of the pathogen (Supplementary Figure S1E and Supplementary Table S2D). The fresh seeds were the major sources of beneficial microbes, accounting for 89% (24/27) of disease antagonists (Figure 6E). Pumpkin seeds were the main source of promising antagonists accounting for 30% (8/27) of candidates, followed by cucumber seeds 22% (6/27), cantaloupe (19%, 5/27) and watermelon seeds (19%, 5/27). Nevertheless, 50% (3/6) of strains that exhibited significant disease suppression originated from fresh cucumber seeds and belong to the Enterobacteriaceae family (Figure 6E). Bacillus endophytes were the most prevalent antagonists, comprising 26% (7/27) of the positive strains. However, a Pediococcus endophyte from fresh cantaloupe seeds displayed the highest PE% against the disease (46%), equivalent to the positive controls (Supplementary Table S2D).
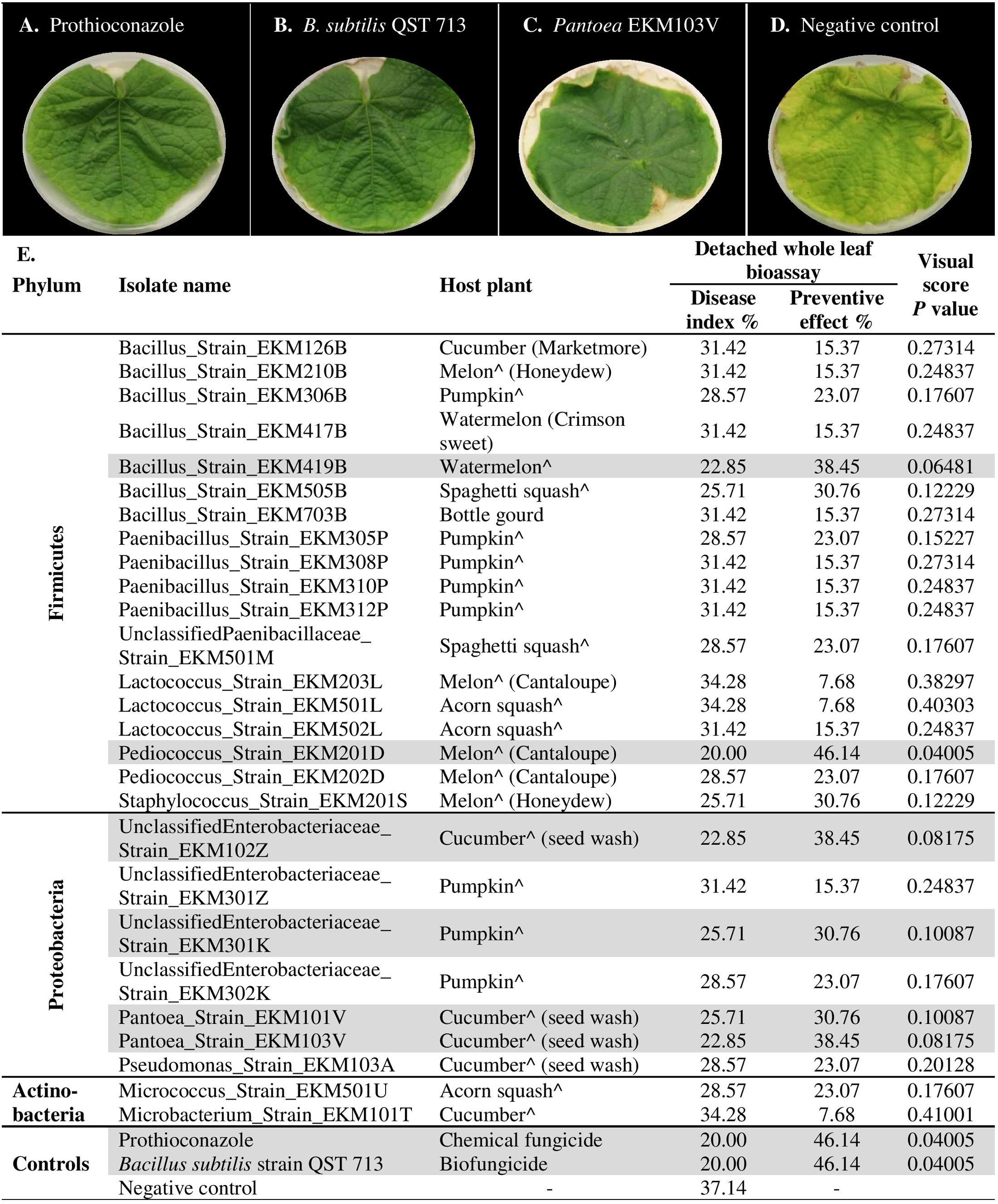
FIGURE 6. In planta screening of promising endophytes from the leaf disk bioassay for antagonism against cucumber powdery mildew using a detached whole leaf bioassay. (A–D) Representative pictures of: (A) chemical fungicide positive control (Prothioconazole), (B) commercial biocontrol agent positive control (Bacillus subtilis strain QST 713), (C) example of a typical promising endophytic bacteria; Pantoea EKM103V, (D) and the negative control (LB broth amended with filter sterilized 0.01% Tween 20). (E) List of tested endophytic bacterial strains, their respective plant seed source, corresponding disease index, preventive effect values and visual score p-values (calculated using independent samples t-test, one-tailed and unequal variance). The rows highlighted in gray denote promising strains that significantly (p < 0.1) suppressed the disease in comparison to the negative controls. A greater Disease Index indicates increased disease symptoms, whereas a greater Preventative Effect denotes improved control of the disease. The circumflex accent (ˆ) denotes that fresh fruits were used as the seed source.
Discussion
The Cucurbitaceae is an economically important crop family, associated with human civilizations for thousands of years (Jeffrey, 1980) and able to grow in diverse ecosystems and climates around the world (Figure 1) (Pessarakli, 2016). These crops originated and were domesticated in the tropics and humid sub-tropics across the world’s continents, and today also grow in warm, moist greenhouses (Sebastian et al., 2010; Paris, 2016b; Pető et al., 2016; Kates et al., 2017); these environments favor fungal pathogens. Unlike endophytes that inhabit other plant organs, seed endophytes have the potential to transmit across plant generations and are uniquely able to establish the plant microbiome in seedlings (Johnston-Monje and Raizada, 2011; Truyens et al., 2015; Huang et al., 2016; Johnston-Monje et al., 2016; Khalaf and Raizada, 2016; Mitter et al., 2016). Previous studies have shown that up to one third of in vitro tested endophytes exhibit activity against plant pathogens (Berg, 2009). For these reasons, we hypothesized that the seeds of domesticated cucurbits may possess endophytes with anti-pathogen traits. We previously isolated cucurbit seed-associated endophytes and showed that they express plant growth promoting traits including nutrient acquisition and phytohormone biosynthesis (Khalaf and Raizada, 2016).
Here we tested the seed associated endophytes against the most important soil-borne pathogenic genera of crops (Fusarium, Rhizoctonia, Pythium and Phytophthora) known to be detrimental to early seed germination (Nelson, 2017), along with the most devastating foliar disease of cucurbits, powdery mildew (Glawe, 2008). Our findings showed that a surprisingly large number of seed-associated endophytes (70%, 118/169) could antagonize the five tested phytopathogens. when agar disk assay results (4 pathogens, 73/118) were combined with a detached leaf disk bioassay (one obligate pathogen, 45/118). Furthermore, approximately two-thirds of the library exhibited RNase activity and secrete the VOCs, acetoin and/or diacetyl, known to be implicated in triggering the plant immune defense. Combined, the results show that the majority of the seed associated bacteriome of the domesticated cucurbits possess potential biocontrol traits.
Importance of Bacillus and Paenibacillus Seed Endophytes
Bacillus and Paenibacillus are cosmopolitan aerobic bacterial genera in agroecosystems (McSpadden Gardener, 2004). Their ubiquitous existence has been confirmed by culture dependent techniques, while metagenomic analyses have revealed additional species diversity (Borneman et al., 1996; Mahaffee and Kloepper, 1997; Garbeva et al., 2003). Moreover, they are known for specific attributes that favor them as crop inoculants including their ability: (1) to form stress tolerant endospores; (2) to secrete diverse antimicrobial secondary metabolites (peptide antibiotics); (3) to secrete extracellular enzymes and signaling molecules; (4) to scavenge nutrients from the environment; (5) to biosynthesize phytohormones; and (6) to induce host systemic defense. Various beneficial strains of Bacillus and Paenibacillus are commercially available as biopesticides and biofertilizers (McSpadden Gardener and Fravel, 2002; McSpadden Gardener, 2004; Choudhary and Johri, 2009; Böhme et al., 2016).
Despite an earlier focus of biocontrol research on the use of Gram negative bacteria such as Pseudomonas, Agrobacterium and Erwinia, later studies starting in 1999 introduced Bacillus species as alternative biocontrol agents (Emmert and Handelsman, 1999; Nagórska et al., 2007; Rybakova et al., 2016). The above-noted biological traits of Bacilli facilitated their commercial formulation as marketable biopesticides (Ongena and Jacques, 2008; Rybakova et al., 2016). For example, in a recent study (Karimi et al., 2016), 4/8 Bacillus strains (including B. amyloliquefaciens, B. pumilus and B. siamensis) isolated from the sugar beet rhizosphere, and shoots and roots of apple, showed significant suppression of pathogenic R. solani infecting sugar beet under greenhouse conditions.
Interestingly, here, 43% (36/83) of Bacillus strains isolated from cucurbit seeds exhibited antagonism in vitro against diverse fungal and oomycete pathogens including R. solani (isolated from sugar beet), F. graminearum (from maize), P. capsici (from pepper) and P. aphanidermatum (from cucumber). These Bacillus isolates originated from seeds of all tested cucurbit species (Figure 2) and were predicted to be diverse species (Khalaf and Raizada, 2016). In a previous study, various Bacillus strains (B. subtilis, B. amyloliquefaciens, and B. cereus) isolated from cucurbit fruits (but not seeds) (C. melo, C. callosus, Citrullus lanatus and Bryonia cretica) demonstrated in vitro anatagonism against four fungal pathogens of melons including F. oxysporum F. sp. melonis and F. oxysporum, and F. sp. radicis-cucumerinum (Glassner et al., 2015). Furthermore, 71% of tested Bacillus microbes against Fusarium wilt in cucumber were effective (Raza et al., 2017). Fusarium wilt disease is a devastating disease of cucumber, caused by Fusarium oxysporum (Raza et al., 2017). We do not know if the anti-F. graminearum strains identified in this study showed cross-antagonism toward other Fusarium species, but it may explain the reason why cucurbits possess endophytes that antagonize a non-cucurbit pathogen.
With regard to the role of Bacillus in managing cucurbit powdery mildew, in a previous study, four Bacillus strains isolated from the healthy phyllosphere and rhizosphere of cucurbits infected with powdery mildew demonstrated antagonism against the fungus Podosphaera fusca on detached melon leaves and seedlings; the suppressive efficacy was up to 80% (Romero et al., 2004). In another study, Bacillus subtilis strain UMAF6639 demonstrated reduction of powdery mildew disease severity in infected melon leaves (García-Gutiérrez et al., 2012). The antagonism involved three major mechanisms including induction of host systemic resistance (García-Gutiérrez et al., 2012), stimulation of plant signaling pathways mediated by jasmonate and salicylic acid, and finally induction of host defense mediated by a surfactin lipopeptide. Generally, however, powdery mildew antagonism by biocontrol agents has been shown to be caused by microbial production of the antifungal compounds, iturin and fengycin (García-Gutiérrez et al., 2013).
Since the first characterization of the genus Paenibacillus 20 years ago, numerous studies of its role as an endophyte have been reported (Rybakova et al., 2016). For example, P. polymyxa strains identified in our lab from seeds and roots of maize exhibited anti-F. graminearum activity in vitro as well as under greenhouse conditions (Mousa et al., 2015). The displayed antagonism was confirmed by molecular detection of the fusA gene and biochemical detection of produced fusaricidin derivatives. Similarly, 3 out of 4 isolates belonging to the genus Paenibacillus (and one isolate belonging to the genus Pantoea) identified from wheat seeds of a commercial variety exhibited anti-F. graminearum activity in the dual culture assay (Díaz Herrera et al., 2016). Moreover, a P. polymyxa strain isolated from the vinegar industry showed effective suppression of Fusarium wilt in cucumber and promoted other beneficial microbes to successfully compete against the causative agent (F. oxysporum, a soil pathogen) for colonizing the rhizospheric niche of cucumber (Shi et al., 2017). P. polymyxa PB71 identified from seeds of Styrian oil pumpkin demonstrated antagonism against fungal diseases caused by Didymella bryoniae under greenhouse condition as well as suppression of powdery mildew during field trials, and improved pumpkin yield (Fürnkranz et al., 2012). In a recent study (Liu et al., 2017), endophytic bacterial strain RSE1, isolated from rice (Oryza sativa L.) seeds and identified as P. polymyxa, showed in vitro antagonism against the fungal pathogen Ustilaginoidea oryzae, the causative agent of rice false smut. The genetic basis of the biocontrol trait was predicted through computational analysis of the draft genome of the endophytic strain as a glucanase gene. In our study, 17/37 (46%) of Paenibacillus strains isolated from commercial cucurbit seeds exhibited in vitro antagonism when results of all tested crop pathogens were combined (Supplementary Table S1). These candidates included 16 antagonists of R. solani and P. capsici, and 14 strains against F. graminearum in vitro (Figure 3). Intriguingly, the R. solani antagonists were isolated from all tested cucurbit genera and hence may have been inadvertently selected. Almost all of these promising candidates were acetoin and/or diacetyl producers (Supplementary Table S1). Seven strains of Paenibacillus recovered from fresh seeds of pumpkin showed significant reduction of powdery mildew symptoms (p < 0.01–0.0001) in the leaf disk bioassay (Supplementary Tables S3, S4). Our results are congruent with the reported patents of Paenibacillus species as biocontrol agents antagonizing a wide range of plant pathogens comprising pathogenic species of Fusarium, Rhizoctonia, Pythium, Phytophthora, Botrytis, Penicillium, Sclerotinia and Cladosporium (Rybakova et al., 2016).
Importance of Other Endophytic Genera
The genus Pantoea was first reported in the literature as a plant pathogen (Muraschi et al., 1965; Nadarasah and Stavrinides, 2014), but more recently various strains have been shown to exert beneficial biocontrol activity to host plants through various mechanisms including competitive colonization, production of antibiotics and/or induction of host systemic defense. Pantoea species have been shown to target a wide spectrum of plant pathogens including bacteria, fungi, oomycetes and parasitic nematodes via secretion of antimicrobials such as pantocins, herbicolins, microcins, and phenazines (Walterson and Stavrinides, 2015). Commercially formulated Pantoea-based biopesticides are currently available such as BlightBan C9-1 (P. vagans C9-1) and Bloomtime Biological (P. agglomerans E325) which are used for combating fire blight in apples and pears (Johnson and Stockwell, 1998; Pusey, 2002). In a recently published paper, three endophytic bacteria isolated from surface-sterilized rice (Oryza sativa L.) seeds promoted in vitro rice seedling development along with protection against F. oxysporum. Using 16S rRNA sequencing, the strains were identified as Pantoea dispersa, along with Enterobacter asburiae and Pseudomonas putida (Verma et al., 2017). In our study, we isolated two Pantoea strains from fresh cucumber seed mucilage that exhibited in vitro antagonism against the tested oomycetes (Supplementary Table S1) and showed significant suppression of powdery mildew symptoms in the leaf disk bioassay (p < 0.05) and detached whole leaf bioassay (p < 0.1) (Figures 4C, 6C and Supplementary Tables S2, S3). These strains were also producers of acetoin and diacetyl (Supplementary Table S1). Our findings suggest that Pantoea may hold potential as inoculants that can target a new, wider anti-pathogenic spectrum.
Another recent study (Sandhya et al., 2017) showed the potential of seed endophytes as biocontrol agents. In that study, maize seeds and roots were used as sources of bacterial endophytes; 10 out of 39 endophytic bacteria demonstrated diverse in vitro activities comprising growth promotion and antagonism against 6 fungal phytopathogens (Macrophomina phaseolina, Rhizoctonia solani, Fusarium oxysporum, Sclerotium rolfsii, Pythium aphanidermatum and Alternaria sp.) under drought stress. These endophytic bacteria were identified to the genus level as Pseudomonas, Acinetobacter, Enterobacter, and Sinorhizobium.
In the food industry, various species of lactic acid bacteria (LAB) belonging to the genera Enterococcus, Lactobacillus, Lactococcus, Pediococcus and Leuconostoc, are well known safe biopreservatives and biological control agents (Gajbhiye and Kapadnis, 2016; Porto et al., 2017). Their antagonism is mediated by secretion of cyclic dipeptides, diacetyl, organic aids, ethanol, antifungal metabolites (such as phenyllactic acid or fatty acids), bacteriocins and antibiotics (such as reutericyclin) (Akbar et al., 2016; Gajbhiye and Kapadnis, 2016). In our study, the results revealed 10 antifungal LAB strains belonging to the genera Lactococcus, Pediococcus and Leuconostoc. All Lactococcus strains showed in vitro antagonism against oomycetes (Supplementary Table S1) whereas four strains antagonized Podosphaera fuliginea in planta (Supplementary Table S2A). The two identified Pediococcus strains were shown here to exhibit a broad antimicrobial target spectrum including against R. solani, P. capsici and Podosphaera fuliginea, but not F. graminearum. However, in a previous study, a Pediococcus pentosaceus strain isolated from dairy products antagonized F. graminearum growth (Sellamani et al., 2016). Moreover, the broad antifungal spectrum of Pediococcus acidilactici LAB 5 strain was first reported in a study by Mandal et al. (2007) and included antagonism against Alternaria solani, Aspergillus fumigaus, A. parasiticus, Cladosporium herbarum, Colletotrichum acutatum, Curvularia lunata, Fusarium oxysporum, Microsporium sp. Mucor sp. and Penicillium sp. (Mandal et al., 2007). In this study, a single Leuconostoc strain demonstrated antagonism toward R. solani and P. aphanidermatum, though in an earlier study, the intimate association between R. solani and Leuconostoc bacteria in sugar beet roots was interpreted as a synergistic interaction that promoted root rot (Strausbaugh, 2015). Combining these previous reports with our study, it would appear that the probiotic characteristics of LAB are not limited to food preservation, but may extend to counteract plant pathogens during crop cultivation.
Acetoin and Diacetyl Production
As noted earlier, acetoin (3-hydroxy-2-butanone) and its major bioanalogs (2,3-butanediol and diacetyl), are important volatile organic compounds (VOCs) produced by several microbes including bacteria. In earlier research, B. subtilis, B. amyloliquefaciens and P. polymyxa demonstrated growth promotion and induction of systemic resistance in Arabidopsis thaliana by secretion of these VOCs (Ryu et al., 2003, 2004; Lee et al., 2012). In our study, perhaps surprisingly, production of acetoin and diacetyl volatiles correlated to antagonism of fungi and oomycetes despite the lack of a plant host in our in vitro assay. For example, 95% (21/22), 82% (45/55), 77% (34/44) and 58% (11/19), respectively, of F. graminearum, R. solani, P. capsici and P. aphanidermatum in vitro antagonists, were acetoin and diacetyl producers (Supplementary Table S1). Furthermore, 43.5% (27/62) of promising candidates that significantly (p < 0.05) reduced disease severity against powdery mildew in the in planta leaf disk bioassay were VOC producers (Supplementary Table S2). It may be that these endophytes have been selected to have multiple anti-pathogen mechanisms, including both direct antibiosis (evident in our dual-culture assays) and induction of host defense. The majority of the promising VOC-producing antagonists in this study belong to the phylum Firmicutes, in particular the genera Bacillus and Paenibacillus (Figure 3). Similarly, in an earlier study, four different VOC-producing B. amyloliquefaciens subspecies demonstrated in vitro antagonism against several plant pathogenic fungi (Asari et al., 2016). Therefore, our findings point to the promise of using endophytic bacteria displaying VOC activity in biological control applications (Vespermann et al., 2007; Asari et al., 2016), though further confirmation of the impact of the VOCs produced by the cucurbit endophytes will be required. Specifically, VOCs of promising biocontrol candidates could be collected in sealed plates and applied to the plant tissue in the presence of the relevant pathogen.
Role of Ribonuclease Activity in Biocontrol
In our study, of the 104 RNase positive isolates, 91% (20/22) of F. graminearum antagonists displayed RNase activity followed by 73% (40/55) for R. solani, 55% (24/44) for P. capsici, 42% (8/19) of P. aphanidermatum, and 53% (33/62) for powdery mildew (of those that significantly suppressed the disease in the leaf disk bioassay at p < 0.05). Interestingly, 83% (86/104 RNase positive strains) belonged to Bacillus (76/104) and Paenibacillus (10/104). In previous studies, Bacilli have been shown to produce RNAses, for example, Bacillus amyloliquefaciens has been shown to produce barnase, a ribonuclease and antibiotic protein (Khan et al., 2016). Noteworthy, the antifungal activity of some pathogenesis-related (PR) proteins secreted by host plants has been associated with their ribonuclease activity, including the PR-4 and PR-10 families (Filipenko et al., 2013; Miranda et al., 2017; Moosa et al., 2017). The associated mechanism(s) of action of these ribonucleases is not clear but may include direct antagonism (by penetrating fungal cells and degrading native mRNA) or indirect antagonism (by inducing host programmed cell death) (Filipenko et al., 2013; Miranda et al., 2017; Moosa et al., 2017). Further experiments will be required to determine whether the extracellular RNase activity of any of the endophytes plays a role in their phytopathogen suppression, and if so, their mechanism of action.
Potential Role of Lytic Enzymes in Phytopathogen Suppression
In our previous study (Khalaf and Raizada, 2016), we reported that extracellular lytic enzyme activities, including cellulase, pectinase and protease function, were displayed by numerous cucurbit seed-associated bacterial endophytes. Our previous observation may be relevant here, as several biocontrol agents have been shown to exert their antagonistic activity through secretion of lytic enzymes that protect the plant either directly or indirectly. The direct mechanism has been shown to involve breaking down of essential complex polymers within the pathogen such as chitin, protein, cellulose and DNA (Pliego et al., 2011). By contrast, the lysis products (e.g., chitin fragments) can be indirectly employed in plant protection by eliciting host defence responses (Heydari and Pessarakli, 2010; Pliego et al., 2011; Duran-Flores and Heil, 2016). Further experiments will be needed to verify this hypothesis.
Study Limitations, Future Experiments and Biocontrol Applications
Surprisingly, we noticed adverse effects from some endophytic bacteria in the cucumber leaf disk bioassay (Supplementary Table S2B), suggesting that they were being recognized as pathogens or added to plant stress, perhaps due to their high titre in combination with plant wounding. Some endophytes have been shown to be helpful to specific hosts but pathogenic to others (Brader et al., 2017). Alternatively, some of the observed variation between the endophytes may have been due to differences in their growth phase. In a study by Romero et al. (2004), bacterial cells in stationary phase showed relatively greater inhibition than log phase cells with respect to growth of the obligate pathogen Podosphaera fuliginea (Romero et al., 2004). With respect to the strain biosafety, promising endophytes must be subject to further study to evaluate their impacts on human health and the surrounding environment. Potential risks could be identified through the rules and regulations of the safety assessment charter laid down by the American Biological Safety Association (ABSA) (Bharti et al., 2017).
Our study represents only the first step toward the development of novel biocontrol inoculants. Apart from powdery mildew, the study was limited to in vitro screening, and likely revealed endophytes that exhibit direct antagonism, along with traits such as VOCs pertinent to the induction of the host defense response. Other implicated biocontrol mechanisms such as pathogen/endophyte-plant interactions and competition with natural microflora will require in planta assays (Eljounaidi et al., 2016). More generally, future biological control applications of promising antagonists will require replicated field and greenhouse trials, which are forthcoming.
The focus of this study was bacterial, not fungal, endophytes as potential biocontrol agents, because vegetable growers spray with fungicides, and hence bacterial biocontrol agents can be integrated with chemical approaches. Furthermore, bacteria are easily handled and rapidly grow to permit inoculant preparation (Whipps, 2001). Although very limited, a small number of successful trials of seed bacterial endophytes have been reported for the management of fungal phytopathogens (Mukhopadhyay et al., 1996; Loper and Henkels, 1999; Cottyn et al., 2001; Ruiza et al., 2011; Gagne-Bourgue et al., 2013; Truyens et al., 2015), suggesting that the microbes identified in this study may have future biocontrol potential. Optimistically with the advent of next generation sequencing technology, omics tools could help in performing studies of comparative genomics between de novo inoculants and their biocontrol cousins of the same genus. These studies may facilitate the selection of promising candidates for further in vivo testing based on their genomes encoding biocontrol genes (Douriet-Gámez et al., 2017).
Study Implications
Cucurbit fruits and their seeds have been widely grown by humans for thousands of years, able to grow in diverse climates where fungi and oomycetes can thrive. As with all plants, cucurbit seeds germinate in the soil. Here we demonstrate that the majority of seed associated endophytes of cultivated cucurbits have the potential to suppress fungal and oomycete pathogens that affect early seed germination as well as a later foliar disease. Furthermore, we demonstrate that the majority of these antagonistic microbes are Bacillus and Paenabacillus strains. Bacilli are widely used as commercial biocontrol agents of vegetable crops, and it is interesting to speculate whether these biocontrol agents are mimicking the ecological niche established by their endophytic cousins. The endophytes characterized in this study may assist with the implementation of alternative pest management strategies to address growing concerns for safe food production globally.
Materials and Methods
Source of Tested Endophytic Bacteria
One hundred and sixty nine endophytic bacteria were previously cultured from seeds of diverse cucurbits and classified based on their 16S rRNA sequences (Supplementary Table S1) (Khalaf and Raizada, 2016).
In Vitro Testing of Functional Traits That Promote Host Resistance
Acetoin and Diacetyl Production
The method was adapted from previous protocols (Phalip et al., 1994; Johnston-Monje and Raizada, 2011). Autoclaved lysogeny broth (LB) medium (composed of 10 g NaCl, 5 g yeast extract, 10 g tryptone, per liter) was amended with filter sterilized 0.5% glucose and distributed as 1 ml aliquots in 96 deep well plates (#07-200-700, Fisher). The plates were inoculated with overnight grown bacterial endophytes using a flame-sterilized 96-pin replicator, sealed with a breathable membrane and incubated in a shaking incubator (150 rpm at 28°C) for 5 days. On the fifth day, 100 μl of each bacterial culture was transferred to a 96 well white fluorometer plate using a multichannel pipet. Subsequently, 100 μl/well of freshly prepared Barritt’s Reagents A and B was added, and then left to stand for 15 min. The development of red/pink color was scored as a positive result compared to the copper color of the reagent (negative control). For confirmation, the test was performed in triplicate. Barritt’s Reagents A and B were prepared in a proportion of 3:1 (v/v) by mixing 3 parts of 0.5% (w/v) creatine solution with 1 part of freshly prepared 7.5% (w/v) α-naphthol in 2.5 N sodium hydroxide.
Ribonuclease Activity
In 10 ml of 0.1 M Na2HPO4 (pH 8), 1.5 g of torula yeast RNA (#R6625, Sigma–Aldrich) was dissolved, filter sterilized and added to 250 ml of autoclaved LB agar media (Hole et al., 2004). Bacterial endophytes were inoculated onto agar plates using a flame sterilized pin replicator, and incubated at 28°C. After 3 days, 70% perchloric acid was flooded over the plates for 15 min. A clear halo around a colony was scored as a positive result. The test was performed in triplicate.
In Vitro Anti-microbial Screening
Source of Plant Pathogens
The Rhizoctonia solani strain was isolated from sugar beet cultivated in Ontario, Canada, and identified phenotypically at the University of Guelph (Ridgetown campus) and by Dr. Linda Hanson (United States Department of Agriculture, USDA) in Michigan, United States. Phytophthora capsici was isolated from pepper grown in Simcoe Station, Ontario and identified phenotypically by Dr. Catarina Saude (University of Guelph) with completion of Koch’s postulates. Pythium aphanidermatum was obtained from cucumber grown in Quebec, identified and supplied by Prof. Mary Ruth McDonald (University of Guelph). Fusarium graminearum was isolated from maize and supplied by Agriculture and Agri-food Canada (AAFC), Guelph, ON, Canada.
Agar Disk Diffusion Technique
Using the agar disk diffusion technique (Shehata et al., 2016), the tested soil-borne fungal plant pathogens and oomycetes were grown in YPD broth (#Y1375, Sigma–Aldrich) for 3–5 days (based on the fungus growth rate) at 25°C with gentle shaking. Pre-melted cooled PDA media was inoculated with the mycelia (25 ml/150 ml PDA for R. solani, 1 ml/50 ml PDA for P. capsici and F. graminearum, and 1 ml/100ml PDA for P. aphanidermatum) and 50 ml were poured into each sterile Petri dish (150 mm × 15 mm), then left to solidify. Using sterile glass tubes, 11 mm diameter cups (wells) were made in the inoculated agar plates. Cups were loaded with 100 μl of overnight LB culture of each bacterial endophyte with adjusted OD600 0.4–0.6. The fungicide Nystatin (N 581, PhytoTechnology Laboratories, United States) was dissolved in dimethyl sulfoxide (DMSO) and used as a positive control (≈202 U/100 μl for R. solani, 454 U/100 μl for F. graminearum and 1817 U/100 μl for P. capsici), and DMSO was used as the negative control. Propamocarb hydrochloride (722 g/L, aqueous solution, UVP:05933765, Bayer CropScience Inc., Canada) was used as a positive control for P. aphanidermatum at its commercial concentration, and autoclaved ddH2O was used as the negative control. The plates were incubated at 25°C for 3–5 days in darkness. The anti-microbial activity was scored by measuring the diameter of the inhibition zone around each agar cup. The assay was performed in triplicate for each bacterial endophyte.
In Planta Techniques for Screening for Antagonism against Powdery Mildew
Plant Variety and Growth Conditions
The National Pickling cucumber variety was purchased from William Dam Seeds Ltd. (Dundas, ON, Canada). Seeds were germinated for 4–6 days, then transplanted and grown in a growth room at 25/18°C for 16/8 h (day/night), respectively. The humidity was maintained at 75% during the experiment, and the light intensity (cool white fluorescent bulbs) was measured (150–220 μmol m-2 sec-1) at the top of the plants using a photon flux meter in the photosynthetically active radiation (PAR) range of 400–700 nm (model BQM-01, Apogee Instruments Inc., Logan, UT, United States). For the leaf biocontrol assays, 30–35 days old plants (after sowing) were used.
Source and Maintenance of the Pathogen
As Podosphaera fuliginea is an obligate pathogen, cucumber leaves infected with powdery mildew were obtained from a commercial greenhouse (Leamington, ON, Canada) in collaboration with the Ontario Ministry of Agriculture, Food and Rural Affairs. The causative pathogen was maintained on leaves of healthy cucumber plants (National Pickling cucumber variety) by tapping the infected leaves over the healthy ones to mechanically transfer the fungal spores. The leaves were then gently misted with water droplets, and the plants were covered with plastic bags for 24 h to raise the humidity. The bags were then removed and the plants were maintained in a growth chamber at 24/18°C for 16/8 h (day/night). The humidity was adjusted to 60% during the day and 80% at night, and the light intensity was as noted above. The early signs of disease appeared on the infected leaves within 1 week.
Molecular Identification of the Powdery Mildew Causative Pathogen
A heavily infected leaf was selected and the conidia were collected by spraying with autoclaved 0.01% Triton X-100 in ddH2O. The spore suspension was centrifuged and washed using the same solution at 10,000 rpm for 10 min. The pelleted spores were ground under liquid nitrogen in a mortar and pestle (Chen et al., 2008; Glawe, 2008). Fungal DNA was isolated using a DNeasy®Plant Mini kit (#69106, Qiagen, United States) and quantified using a Nanodrop (Thermo Scientific, United States). PCR was performed using primer pair ITS4 (forward, 5′-TCCTCCGCTTATTGATATGC-3′) and ITS1F (reverse, 5′-CTTGGTCATTTAGAGGAAGTAA-3′) (Amend et al., 2010). Approximately 50 ng of total DNA was added to a PCR mixture containing 4 μl Standard Taq Buffer (M791B, Promega), 0.4 μl of 25 mM dNTP mix, 0.5 μl of each primer (10 mM working stock), 0.6 μl of 50 mM MgCl2, 0.2 μl of Standard Taq (New England Biolabs) and H2O to a final volume of 20 μl. A PCR reaction was undertaken using the following conditions: an initial denaturation at 95°C for 10 min, then 34 cycles (95°C for 1 min, 51°C for 1 min, 72°C for 1 min) and a final extension at 72°C for 7 min. Amplicons (600 bp) were gel purified, sequenced and then searched against fungal ITS deposits within RDP (Ribosomal Database Project) and also BLASTN searched against NCBI using the default parameters (Supplementary Table S2E).
Leaf Disk Bioassay
Adapting a previous protocol (Sun et al., 2013), the leaves from the 2nd to 5th node of cucumber plants were detached and 0.5 inch-diameter leaf-disks were created by using a paper puncher. Fifteen leaf-disks were used for each endophytic bacterium (3 Petri dishes, 5 disks per dish) by soaking them in a bacterial suspension of LB broth amended with filter sterilized 0.01% Tween 20 and adjusted to OD600 ∼0.3–0.5 for 1 min with gentle shaking. Then five disks were transferred into each Petri dish (6 cm diameter) using flame sterilized forceps where they were placed on a damp filter paper (5.5 cm filter paper Whatman No.1) wetted with 2 ml of nutrient solution optimized for cucumbers [per 1 L of tap water: 950 mg Ca(NO3)2].4H2O; 810 mg KNO3; 500 mg MgSO4; 7H2O; 155 mg NH4H2PO4; 3 mg H3BO3; 2 mg; ZnSO4.7H2O; 0.05 mg CuSO4.5H2O; 0.02 mg NaMoO4; and 25 mg NaFeEDTA (Pramanik et al., 2000). The leaf disks were placed with their adaxial side up. The Petri dishes were incubated in darkness at 30°C for 24 h. After 24 h, the conidial suspension was prepared by adapting a previous protocol (Kim et al., 2007): 10 μl of the freshly prepared conidial suspension (adjusted to 1 × 105 CFU/ml, in autoclaved ddH2O supplemented with 0.01% Triton X-100) were placed on the center of each leaf disk as a pathogen inoculum. The Petri dishes were then sealed with breathable tape and incubated in a growth chamber using the same conditions used for pathogen maintenance (above). Prothioconazole fungicide (used as 24 μg/ml concentration and prepared from 480 g/L stock solution) and a commercial biocontrol agent (Bacillus subtilis strain QST 713, formulated in a 1 mg/ml suspension of water dispersible granules) were used as positive controls.
LB broth supplemented with filter sterilized 0.01% Tween 20 was used as a negative control. Visual assessment of the disease severity was performed using a 0–7 scale based on the development of infected lesions and chlorosis, by cross-referencing to selected diseased leaf photos corresponding to different levels of disease severity (Figure 4F). The disease index and preventive effect were calculated using the following equations:
Detached Whole Leaf Bioassay
Adapting a previous protocol (Sun et al., 2013), whole cucumber leaves from the 2nd – 5th node were selected, and each leaf was placed on a damp filter paper wetted with the same nutrient solution used in leaf disk bioassay and placed in a 150 mm diameter Petri dish. The petiole of each leaf was wrapped in an autoclaved, wetted (with cucumber nutrient solution) cotton puff. Overnight endophytic bacterial cultures were harvested and re-suspended in LB broth supplemented with 0.01% Tween 20 and adjusted to an OD600 ∼0.3–0.5. Each leaf was sprayed with a ∼5 ml bacterial suspension using a hand sprayer. Petri dishes were incubated at 30°C in darkness. After 24 h, the leaves were sprayed with ∼0.5 ml of conidial suspension (1 × 105 CFU/ml) using a hand sprayer. The Petri dishes were sealed with breathable tape and incubated in a growth chamber for 7 days (under the same conditions used for pathogen maintenance). The same positive and negative controls were used. For each treatment including controls there were five leaves representing five replicates. The disease severity was performed visually using the same 0–7 scale as noted above. The disease index and preventive effects were calculated using the same equations utilized in the leaf disk bioassay.
Statistical Analysis
Data of leaf disk bioassay were analyzed using GraphPad Prism 7. One-way analysis of variance (ANOVA) was applied, and mean values of the treatments were compared to controls using Dunnett’s multiple comparison test. Data from the detached whole leaf bioassay were analyzed using t-tests of visual disease scores (one-tailed and unequal variance).
Author Contributions
MR designed the research and helped to write the manuscript. EK performed all the lab work, data analysis and wrote the manuscript. All authors read and approved the final manuscript.
Funding
Funding was provided by a generous fellowship to EK from the Government of Egypt, and grants to MR from the Ontario Ministry of Agriculture, Food and Rural Affairs (030218) and NSERC (401036).
Conflict of Interest Statement
The authors declare that the research was conducted in the absence of any commercial or financial relationships that could be construed as a potential conflict of interest.
Acknowledgments
The authors thank Sara Wyngaarden (University of Guelph) for assistance. They thank Cara McCreary (Greenhouse Vegetable IPM Specialist with the Ontario Ministry of Agriculture, Food and Rural Affairs) for helpful advice and for supplying cucumber leaves infected with powdery mildew. They also thank Cheryl Trueman (Ridgetown College, University of Guelph) for expert advice.
Supplementary Material
The Supplementary Material for this article can be found online at: https://www.frontiersin.org/articles/10.3389/fmicb.2018.00042/full#supplementary-material
FIGURE S1 | Selected powdery mildew disease leaf photos corresponding to different levels of disease severity used as references to guide visual disease assessments of the detached whole leaf bioassay. (A–E) Shown are representative photos of tested cucumber leaves: (A) chemical fungicide positive control (Prothioconazole), (B) commercial biocontrol agent positive control (Bacillus subtilis strain QST 713), (C) example of a typical promising endophytic bacteria; Pantoea EKM103V, (D) negative control (LB broth amended with filter sterilized 0.01% Tween 20), (E) example of an inoculant Bacillus_EKM502B with adverse effect. R (1–5) denotes replicate number.
TABLE S1 | Detailed information of in vitro phenotypic traits and antimicrobial activity of cucurbit seed bacterial endophytes tested in this study.
TABLE S2 | Detailed information of the effect of the cucurbit seed bacterial endophytes on powdery mildew disease using the leaf disk bioassay and detached whole leaf bioassay.
TABLE S2A | Summary of the leaf disk bioassay results for all endophytes tested against powdery mildew, with their corresponding Genbank accession numbers, organized by bacterial phylum, genus and original host seed source. A greater Disease Index indicates increased disease symptoms, whereas a greater Preventative Effect denotes improved control of the disease.
TABLE S2B | Raw data and calculations for Disease Index, corresponding to Supplementary Table S2A (leaf disk bioassay).
TABLE S2C | Raw data and calculations for Preventative Effect, corresponding to Supplementary Table S2A (leaf disk bioassay).
TABLE S2D | Detailed information of the detached whole leaf bioassay results for all endophytes tested against powdery mildew, with their corresponding Genbank accession numbers, organized by bacterial phylum, genus and original host seed source. A greater Disease Index indicates increased disease symptoms, whereas a greater Preventative Effect denotes improved control of the disease.
TABLE S2E | Molecular identification (ITS rDNA) of the powdery mildew isolate used in this study.
TABLE S3 | Complete list of endophytic strains displaying a positive preventative effect (PE) against powdery mildew in the leaf disk bioassay, organized by bacterial phylum, genus and original seed source. Statistical analysis (Dunnett’s Multiple Comparison Test) of the disease index percentages (DI%) per treatment (n = 3) compared to the negative control is represented as calculated p values. A greater Disease Index indicates increased disease symptoms, whereas a greater Preventative Effect denotes improved control of the disease. The circumflex accent (ˆ) denotes that fresh fruits were used as the seed source. NS, non-significant, ∗p < 0.05, ∗∗p < 0.01, ∗∗∗p < 0.001, ∗∗∗∗p < 0.0001.
TABLE S4 | Raw data and graphical representation of calculated means of the disease index percentage (DI%) displayed by endophytic bacteria that exhibited significant suppression of powdery mildew (p < 0.05) in the leaf disk bioassay along with their corresponding standard deviation and standard error of mean (SEM). Error bars show the SEM.
References
Akbar, A., Ali, I., and Anal, A. K. (2016). Industrial perspectives of lactic acid bacteria for biopreservation and food safety. J. Anim. Plant Sci. 26, 938–948.
Amend, A. S., Seifert, K. A., Samson, R., and Bruns, T. D. (2010). Indoor fungal composition is geographically patterned and more diverse in temperate zones than in the tropics. Proc. Natl. Acad. Sci. U.S.A. 107, 13748–13753. doi: 10.1073/pnas.1000454107
Asari, S., Matzén, S., Petersen, M. A., and Bejai, S. (2016). Multiple effects of Bacillus amyloliquefaciens volatile compounds: plant growth promotion and growth inhibition of phytopathogens. FEMS Microbiol. Ecol. 92:fiw070. doi: 10.1093/femsec/fiw070
Bacon, C. W., and White, J. F. (2016). Functions, mechanisms and regulation of endophytic and epiphytic microbial communities of plants. Symbiosis 68, 87–98. doi: 10.1007/s13199-015-0350-2
Berg, G. (2009). Plant-microbe interactions promoting plant growth and health: perspectives for controlled use of microorganisms in agriculture. Appl. Microbiol. Biotechnol. 84, 11–18. doi: 10.1007/s00253-009-2092-7
Berg, G., Köberl, M., Rybakova, D., Müller, H., Grosch, R., and Smalla, K. (2017). Plant microbial diversity is suggested as the key to future biocontrol and health trends. FEMS Microbiol. Ecol. 93:fix050. doi: 10.1093/femsec/fix050
Bharti, N., Sharma, S. K., Saini, S., Verma, A., Nimonkar, Y., and Prakash, O. (2017). “Microbial plant probiotics: problems in application and formulation,” in Probiotics and Plant Health, eds V. Kumar, M. Kumar, S. Sharma, and R. Prasad (Singapore: Springer Nature). doi: 10.1007/978-981-10-3473-2
Bitas, V., Kim, H.-S., Bennett, J. W., and Kang, S. (2013). Sniffing on microbes: diverse roles of microbial volatile organic compounds in plant health. Mol. Plant Microbe Interact. 26, 835–843. doi: 10.1094/MPMI-10-12-0249-CR
Borneman, J., Skroch, P. W., O’Sullivan, K. M., Palus, J. A., Rumjanek, N. G., Jansen, J. L., et al. (1996). Molecular microbial diversity of an agricultural soil in Wisconsin. Appl. Environ. Microbiol. 62, 1935–1943.
Bosmans, L., De Bruijn, I., De Mot, R., Rediers, H., and Lievens, B. (2016). Agar composition affects in vitro screening of biocontrol activity of antagonistic microorganisms. J. Microbiol. Methods 127, 7–9. doi: 10.1016/j.mimet.2016.05.004
Brader, G., Compant, S., Vescio, K., Mitter, B., Trognitz, F., Ma, L.-J., et al. (2017). Ecology and genomic insights on plant-pathogenic and -nonpathogenic endophytes. Annu. Rev. Phytopathol. 55, 61–83. doi: 10.1146/annurev-phyto-080516-035641
Braun, U., and Cook, R. T. A. (eds). (2012). Taxonomic Manual of the Erysiphales. Utrecht: CBS-KNAW Fungal Biodiversity Centre.
Böhme, M. H., Pinker, I., and Junge, H. (2016). “Effect of organic biostimulators with Bacillus amyloliquefaciens ssp. Plantarum (former Bacillus subtilis) as the main agent in vegetable cultivation,” in Bacilli and Agrobiotechnology, eds M. T. Islam, M. Rahman, P. Pandey, C. K. Jha, and A. Aeron (Cham: Springer). doi: 10.1007/978-3-319-44409-3
Chen, R. S., Chu, C., Cheng, C. W., Chen, W. Y., and Tsay, J. G. (2008). Differentiation of two powdery mildews of sunflower (Helianthus annuus) by a PCR-mediated method based on ITS sequences. Eur. J. Plant Pathol. 121, 1–8. doi: 10.1007/s10658-007-9234-5
Choudhary, D. K., and Johri, B. N. (2009). Interactions of Bacillus spp. and plants - With special reference to induced systemic resistance (ISR). Microbiol. Res. 164, 493–513. doi: 10.1016/j.micres.2008.08.007
Chung, J., Song, G. C., and Ryu, C.-M. (2016). Sweet scents from good bacteria: case studies on bacterial volatile compounds for plant growth and immunity. Plant Mol. Biol. 90, 677–687. doi: 10.1007/s11103-015-0344-8
Cohen, R. (1993). A leaf disk assay for detection of resistance of melons to Sphaerotheca fuliginea race 1. Plant Dis. 77, 513–517.
Cottyn, B., Regalado, E., Lanoot, B., De Cleene, M., Mew, T. W., and Swings, J. (2001). Bacterial populations associated with rice seed in the tropical environment. Phytopathology 91, 282–292. doi: 10.1094/PHYTO.2001.91.3.282
Dean, R., Van Kan, J. A. L., Pretorus, Z. A., Hammond-Kosack, K. E., Di Pietro, A., Spanu, P. D., et al. (2012). The Top 10 fungal pathogens in molecular plant pathology. Mol. Plant Pathol. 13, 414–430. doi: 10.1111/j.1364-3703.2011.00783.x
Díaz Herrera, S., Grossi, C., Zawoznik, M., and Groppa, M. D. (2016). Wheat seeds harbour bacterial endophytes with potential as plant growth promoters and biocontrol agents of Fusarium graminearum. Microbiol. Res. 186–187, 37–43. doi: 10.1016/j.micres.2016.03.002
Douriet-Gámez, N. R., Maldonado-Mendoza, I. E., Ibarra-Laclette, E., Blom, J., and Calderón-Vázquez, C. L. (2017). Genomic analysis of Bacillus sp. Strain B25, a biocontrol agent of maize pathogen Fusarium verticillioides. Curr. Microbiol. doi: 10.1007/s00284-017-1372-1 [Epub ahead of print]
Duran-Flores, D., and Heil, M. (2016). Sources of specificity in plant damaged-self recognition. Curr. Opin. Plant Biol. 32, 77–87. doi: 10.1016/j.pbi.2016.06.019
Eljounaidi, K., Lee, S. K., and Bae, H. (2016). Bacterial endophytes as potential biocontrol agents of vascular wilt diseases – Review and future prospects. Biol. Control 103, 62–68. doi: 10.1016/j.biocontrol.2016.07.013
Emmert, E. A., and Handelsman, J. (1999). Biocontrol of plant disease: a (gram-) positive perspective. FEMS Microbiol. Lett. 171, 1–9. doi: 10.1111/j.1574-6968.1999.tb13405.x
Filipenko, E. A., Kochetov, A. V., Kanayama, Y., Malinovsky, V. I., and Shumny, V. K. (2013). PR-proteins with ribonuclease activity and plant resistance against pathogenic fungi. Russ. J. Genet. Appl. Res. 3, 474–480. doi: 10.1134/S2079059713060026
Fürnkranz, M., Adam, E., Müller, H., Grube, M., Huss, H., Winkler, J., et al. (2012). Promotion of growth, health and stress tolerance of Styrian oil pumpkins by bacterial endophytes. Eur. J. Plant Pathol. 134, 509–519. doi: 10.1007/s10658-012-0033-2
Gagne-Bourgue, F., Aliferis, K. A., Seguin, P., Rani, M., Samson, R., and Jabaji, S. (2013). Isolation and characterization of indigenous endophytic bacteria associated with leaves of switchgrass (Panicum virgatum L.) cultivars. J. Appl. Microbiol. 114, 836–853. doi: 10.1111/jam.12088
Gajbhiye, M. H., and Kapadnis, B. P. (2016). Antifungal-activity-producing lactic acid bacteria as biocontrol agents in plants. Biocontrol Sci. Technol. 26, 1451–1470. doi: 10.1080/09583157.2016.1213793
Garbeva, P., Van Veen, J. A., and Van Elsas, J. D. (2003). Predominant Bacillus spp. in agricultural soil under different management regimes detected via PCR-DGGE. Microb. Ecol. 45, 302–316. doi: 10.1007/s00248-002-2034-8
García-Gutiérrez, L., Romero, D., Zeriouh, H., Cazorla, F. M., Torés, J. A., de Vicente, A., et al. (2012). Isolation and selection of plant growth-promoting rhizobacteria as inducers of systemic resistance in melon. Plant Soil 358, 201–212. doi: 10.1007/s11104-012-1173-z
García-Gutiérrez, L., Zeriouh, H., Romero, D., Cubero, J., de Vicente, A., and Pérez-García, A. (2013). The antagonistic strain Bacillus subtilis UMAF6639 also confers protection to melon plants against cucurbit powdery mildew by activation of jasmonate- and salicylic acid-dependent defence responses. Microb. Biotechnol. 6, 264–274. doi: 10.1111/1751-7915.12028
Glassner, H., Zchori-Fein, E., Compant, S., Sessitsch, A., Katzir, N., Portnoy, V., et al. (2015). Characterization of endophytic bacteria from cucurbit fruits with potential benefits to agriculture in melons (Cucumis melo L.). FEMS Microbiol. Ecol. 91, 1–13. doi: 10.1093/femsec/fiv074
Glawe, D. A. (2008). The powdery mildews: a review of the world’s most familiar (yet poorly known) plant pathogens. Annu. Rev. Phytopathol. 46, 27–51. doi: 10.1146/annurev.phyto.46.081407.104740
Hardoim, P. R., van Overbeek, L. S., Berg, G., Pirttilä, A. M., Compant, S., Campisano, A., et al. (2015). The hidden world within plants: ecological and evolutionary considerations for defining functioning of microbial endophytes. Microbiol. Mol. Biol. Rev. 79, 293–320. doi: 10.1128/MMBR.00050-14
Hausbeck, M. K., and Lamour, K. H. (2004). Phytophthora capsici on vegetable crops: research progress and management challenges. Plant Dis. 88, 1292–1303. doi: 10.1094/PDIS.2004.88.12.1292
Heneidak, S., and Khalik, K. A. (2015). Seed coat diversity in some tribes of Cucurbitaceae: implications for taxonomy and species identification. Acta Bot. Bras. 29, 129–142. doi: 10.1590/0102-33062014abb3705
Heydari, A., and Pessarakli, M. (2010). A review on biological control of fungal plant pathogens using microbial antagonists. J. Biol. Sci. 10, 273–290.
Hole, R. C., Singhal, R. S., Melo, J. S., and D’Souza, S. F. (2004). A rapid plate screening technique for extracellular ribonuclease producing strains. BARC Newsl. 249, 91–97.
Huang, Y., Kuang, Z., Wang, W., and Cao, L. (2016). Exploring potential bacterial and fungal biocontrol agents transmitted from seeds to sprouts of wheat. Biol. Control 98, 27–33. doi: 10.1016/j.biocontrol.2016.02.013
Jeffrey, C. (1980). A review of the Cucurbitaceae. Biol. J. Linn. Soc. 81, 233–247. doi: 10.1111/j.1095-8339.1980.tb01676.x
Johnson, K. B., and Stockwell, V. O. (1998). Management of fire blight: a case study in microbial ecology. Annu. Rev. Phytopathol. 36, 227–248.
Johnston-Monje, D., Lundberg, D. S., Lazarovits, G., Reis, V. M., and Raizada, M. N. (2016). Bacterial populations in juvenile maize rhizospheres originate from both seed and soil. Plant Soil 405, 337–355. doi: 10.1007/s11104-016-2826-0
Johnston-Monje, D., and Raizada, M. N. (2011). Conservation and diversity of seed associated endophytes in Zea across boundaries of evolution, ethnography and ecology. PLOS ONE 6:e20396. doi: 10.1371/journal.pone.0020396
Justyna, N., Magdalena, S., and Urszula, M. (2017). Trichoderma atroviride enhances phenolic synthesis and cucumber protection against Rhizoctonia solani. Plant Prot. Sci. 53, 2007–2013. doi: 10.17221/126/2016-PPS
Kandel, S. L., Firrincieli, A., Joubert, P. M., Okubara, P., Natalie, D., Mcgeorge, K. M., et al. (2017). An in vitro study of bio-control and plant growth promotion potential of Salicaceae endophytes. Front. Microbiol. 8:386. doi: 10.3389/fmicb.2017.00386
Karimi, E., Safaie, N., Shams-Baksh, M., and Mahmoudi, B. (2016). Bacillus amyloliquefaciens SB14 from rhizosphere alleviates Rhizoctonia damping-off disease on sugar beet. Microbiol. Res. 192, 221–230. doi: 10.1016/j.micres.2016.06.011
Kates, H. R., Soltis, P. S., and Soltis, D. E. (2017). Evolutionary and domestication history of Cucurbita (pumpkin and squash) species inferred from 44 nuclear loci. Mol. Phylogenet. Evol. 111, 98–109. doi: 10.1016/j.ympev.2017.03.002
Khalaf, E. M., and Raizada, M. N. (2016). Taxonomic and functional diversity of cultured seed associated microbes of the cucurbit family. BMC Microbiol. 16:131. doi: 10.1186/s12866-016-0743-2
Khan, A., Doshi, H. V., and Thakur, M. C. (2016). “A profilic siderophore producer,” in Bacilli and Agrobiotechnology, eds M. T. Islam, M. Rahman, P. Pandey, C. K. Jha, and A. Aeron (Cham: Springer). doi: 10.1007/978-3-319-44409-3
Kim, J. J., Goettel, M. S., and Gillespie, D. R. (2007). Potential of Lecanicillium species for dual microbial control of aphids and the cucumber powdery mildew fungus, Sphaerotheca fuliginea. Biol. Control 40, 327–332. doi: 10.1016/j.biocontrol.2006.12.002
Lamichhane, J. R., Dürr, C., Schwanck, A. A., Robin, M. H., Sarthou, J. P., Cellier, V., et al. (2017). Integrated management of damping-off diseases. A review. Agron. Sustain. Dev. 37:10. doi: 10.1007/s13593-017-0417-y
Larousse, M., and Galiana, E. (2017). Microbial partnerships of pathogenic oomycetes. PLOS Pathog. 13:e1006028. doi: 10.1371/journal.ppat.1006028
Lebeda, A., Køístková, E., Sedláková, B., McCreight, J. D., and Coffey, M. D. (2016). Cucurbit powdery mildews: methodology for objective determination and denomination of races. Eur. J. Plant Pathol. 144, 399–410. doi: 10.1007/s10658-015-0776-7
Lee, B., Farag, M. A., Park, H. B., Kloepper, J. W., Lee, S. H., and Ryu, C. M. (2012). Induced resistance by a long-chain bacterial volatile: elicitation of plant systemic defense by a C13 volatile produced by Paenibacillus polymyxa. PLOS ONE 7:e48744. doi: 10.1371/journal.pone.0048744
Liu, Y., Bai, F., Li, N., Wang, W., and Cheng, C. (2017). Identification of endophytic bacterial strain RSE1 from seeds of super hybrid rice Shenliangyou 5814 (Oryza sativa L.,) and evaluation of its antagonistic activity. Plant Growth Regul. 82, 403–408. doi: 10.1007/s10725-017-0265-4
Loper, J. E., and Henkels, M. D. (1999). Utilization of heterologous siderophores enhances levels of iron available to Pseudomonas putida in the rhizosphere. Appl. Environ. Microbiol. 65, 5357–5363.
Mahaffee, W. F., and Kloepper, J. W. (1997). Temporal changes in the bacterial communities of soil, rhizosphere, and endorhiza associated with field-grown cucumber (Cucumis sativus L.). Microb. Ecol. 34, 210–223. doi: 10.1007/s002489900050
Mandal, V., Sen, S. K., and Mandal, N. C. (2007). Detection, isolation and partial characterization of antifungal compound(s) produced by Pediococcus acidilactici LAB 5. Nat. Prod. Commun. 2, 671–674.
McSpadden Gardener, B. B. (2004). Ecology of Bacillus and Paenibacillus spp. in agricultural systems. Phytopathology 94, 1252–1258. doi: 10.1094/PHYTO.2004.94.11.1252
McSpadden Gardener, B. B., and Fravel, D. R. (2002). Biological control of plant pathogens: research, commercialization, and application in the USA. Plant Health Prog. doi: 10.1094/PHP-2002-0510-01-RV
Miranda, V., de, J., Porto, W. F., Nolasco, D. O., Claudia, A., Araujo, G., et al. (2017). Comparative transcriptomic analysis indicates genes associated with local and systemic resistance to Colletotrichum graminicola in maize. Sci. Rep. 7:2483. doi: 10.1038/s41598-017-02298-8
Mitter, B., Pfaffenbichler, N., and Sessitsch, A. (2016). Plant-microbe partnerships in 2020. Microb. Biotechnol. 9, 635–640. doi: 10.1111/1751-7915.12382
Moosa, A., Farzand, A., Sahi, S. T., and Khan, S. A. (2017). Transgenic expression of antifungal pathogenesis-related proteins against phytopathogenic fungi – 15 years of success. Isr. J. Plant Sci. doi: 10.1080/07929978.2017.1288407
Mousa, W. K., Shearer, C., Limay-Rios, V., Ettinger, C. L., Eisen, J. A., and Raizada, M. N. (2016). Root-hair endophyte stacking in finger millet creates a physicochemical barrier to trap the fungal pathogen Fusarium graminearum. Nat. Microbiol. 1:16167. doi: 10.1038/nmicrobiol.2016.167
Mousa, W. K., Shearer, C. R., Limay-Rios, V., Zhou, T., and Raizada, M. N. (2015). Bacterial endophytes from wild maize suppress Fusarium graminearum in modern maize and inhibit mycotoxin accumulation. Front. Plant Sci. 6:805. doi: 10.3389/fpls.2015.00805
Mukhopadhyay, K., Garrison, N. K., Hinton, D. M., Bacon, C. W., Khush, G. S., Peck, H. D., et al. (1996). Identification and characterization of bacterial endophytes of rice. Mycopathologia 134, 151–159. doi: 10.1007/BF00436723
Muraschi, T. F., Friend, M., and Bolles, D. (1965). Erwinia-like microorganisms isolated from animal and human hosts. Appl. Microbiol. 13, 128–131.
Nadarasah, G., and Stavrinides, J. (2014). Quantitative evaluation of the host-colonizing capabilities of the enteric bacterium Pantoea using plant and insect hosts. Microbiology 160, 602–615. doi: 10.1099/mic.0.073452-0
Nagórska, K., Bikowski, M., and Obuchowski, M. (2007). Multicellular behaviour and production of a wide variety of toxic substances support usage of Bacillus subtilis as a powerful biocontrol agent. Acta Biochim. Pol. 54, 495–508.
Nelson, E. B. (2017). The seed microbiome: origins, interactions, and impacts. Plant Soil doi: 10.1007/s11104-017-3289-7
Ongena, M., and Jacques, P. (2008). Bacillus lipopeptides: versatile weapons for plant disease biocontrol. Trends Microbiol. 16, 115–125. doi: 10.1016/j.tim.2007.12.009
Paris, H. S. (2016a). Germplasm enhancement of Cucurbita pepo (pumpkin, squash, gourd: Cucurbitaceae): progress and challenges. Euphytica 208, 415–438. doi: 10.1007/s10681-015-1605-y
Paris, H. S. (2016b). Overview of the origins and history of the five major cucurbit crops: issues for ancient DNA analysis of archaeological specimens. Veg. Hist. Archaeobot. 25, 1–10. doi: 10.1007/s00334-016-0555-1
Pessarakli, M. (ed.). (2016). Handbook of Cucurbits: Growth, Cultural Practices, and Physiology. Boca Raton, FL: CRC Press.
Pető,Á., Kenéz,Á., Lisztes-Szabó, Z., Sramkó, G., Laczkó, L., Molnár, M., et al. (2016). The first archaeobotanical evidence of Lagenaria siceraria from the territory of Hungary: histology, phytoliths and (a)DNA. Veg. Hist. Archaeobot. 26, 125–142. doi: 10.1007/s00334-016-0566-y
Phalip, V., Schmitt, P., and Divies, C. (1994). A method for screening diacetyl and acetoin-producing bacteria on agar plates. J. Basic Microbiol. 34, 277–280.
Pliego, C., Ramos, C., de Vicente, A., and Cazorla, F. M. (2011). Screening for candidate bacterial biocontrol agents against soilborne fungal plant pathogens. Plant Soil 340, 505–520. doi: 10.1007/s11104-010-0615-8
Porto, M. C. W., Kuniyoshi, T. M., Azevedo, P. O., Vitolo, M., and de Souza Oliveira, R. P. (2017). Pediococcus spp.: an important genus of lactic acid bacteria and pediocin producers. Biotechnol. Adv. 35, 361–374. doi: 10.1016/j.biotechadv.2017.03.004
Pramanik, M. H. R., Nagai, M., Asao, T., and Matsui, Y. (2000). Effects of temperature and photoperiod on phytotoxic root exudates of cucumber (Cucumis sativus) in hydroponic culture. J. Chem. Ecol. 26, 1953–1967.
Punja, Z. K., and Yip, R. (2003). Biological control of damping-off and root rot caused by Pythium aphanidermatum on greenhouse cucumbers. Can. J. Plant Pathol. 25, 411–417. doi: 10.1080/07060660309507098
Pusey, P. L. (2002). Biological control agents for fire blight of apple compared under conditions limiting natural dispersal. Plant Dis. 86, 639–644. doi: 10.1094/PDIS.2002.86.6.639
Raza, W., Ling, N., Zhang, R., Huang, Q., Xu, Y., and Shen, Q. (2017). Success evaluation of the biological control of Fusarium wilts of cucumber, banana, and tomato since 2000 and future research strategies. Crit. Rev. Biotechnol. 37, 202–212. doi: 10.3109/07388551.2015.1130683
Renner, S. S., Schaefer, H., and Kocyan, A. (2007). Phylogenetics of Cucumis (Cucurbitaceae): Cucumber (C. sativus) belongs in an Asian/Australian clade far from melon (C. melo). BMC Evol. Biol. 7:58. doi: 10.1186/1471-2148-7-58
Robinson, R. W., and Decker-Walters, D. S. (1997). Cucurbits (Crop Production Science in Horticulture), 1st Edn. Wallingford: CAB international.
Romero, D., Pérez-García, A., Rivera, M. E., Cazorla, F. M., and De Vicente, A. (2004). Isolation and evaluation of antagonistic bacteria towards the cucurbit powdery mildew fungus Podosphaera fusca. Appl. Microbiol. Biotechnol. 64, 263–269. doi: 10.1007/s00253-003-1439-8
Ruiza, D., Agaras, B., de Werrab, P., Wall, L. G., and Valverde, C. (2011). Characterization and screening of plant probiotic traits of bacteria isolated from rice seeds cultivated in Argentina. J. Microbiol. 49, 902–912. doi: 10.1007/s12275-011-1073-6
Rybakova, D., Cernava, T., Köberl, M., Liebminger, S., Etemadi, M., and Berg, G. (2016). Endophytes-assisted biocontrol: novel insights in ecology and the mode of action of Paenibacillus. Plant Soil 405, 125–140. doi: 10.1007/s11104-015-2526-1
Ryu, C.-M., Farag, M. A., Hu, C.-H., Reddy, M. S., Kloepper, J. W., and Paré, P. W. (2004). Bacterial volatiles induce systemic resistance in Arabidopsis. Plant Physiol. 134, 1017–1026. doi: 10.1104/pp.103.026583
Ryu, C.-M., Farag, M. A., Hu, C.-H., Reddy, M. S., Wei, H.-X., Pare, P. W., et al. (2003). Bacterial volatiles promote growth in Arapidopsis. Proc. Natl. Acad. Sci. U.S.A. 100, 4927–4932.
Sandhya, V., Shrivastava, M., Ali, S. Z., and Sai Shiva Krishna Prasad, V. (2017). Endophytes from maize with plant growth promotion and biocontrol activity under drought stress. Russ. Agric. Sci. 43, 22–34. doi: 10.3103/S1068367417010165
Schaefer, H., Heibl, C., and Renner, S. S. (2009). Gourds afloat: a dated phylogeny reveals an Asian origin of the gourd family (Cucurbitaceae) and numerous oversea dispersal events. Proc. R. Soc. B Biol. Sci. 276, 843–851. doi: 10.1098/rspb.2008.1447
Sebastian, P., Schaefer, H., Telford, I. R. H., and Renner, S. S. (2010). Cucumber (Cucumis sativus) and melon (C. melo) have numerous wild relatives in Asia and Australia, and the sister species of melon is from Australia. Proc. Natl. Acad. Sci. U.S.A. 107, 14269–14273. doi: 10.1073/pnas.1005338107
Sellamani, M., Kalagatur, N. K., Siddaiah, C., Mudili, V., Krishna, K., Natarajan, G., et al. (2016). Antifungal and zearalenone inhibitory activity of Pediococcus pentosaceus isolated from dairy products on Fusarium graminearum. Front. Microbiol. 7:890. doi: 10.3389/fmicb.2016.00890
Shehata, H. R., Lyons, E. M., Jordan, K. S., and Raizada, M. N. (2016). Relevance of in vitro agar based screens to characterize the anti-fungal activities of bacterial endophyte communities. BMC Microbiol. 16:7. doi: 10.1186/s12866-016-0623-9
Shi, L., Du, N., Shu, S., Sun, J., Li, S., and Guo, S. (2017). Paenibacillus polymyxa NSY50 suppresses Fusarium wilt in cucumbers by regulating the rhizospheric microbial community. Sci. Rep. 7:41234. doi: 10.1038/srep41234
Shishkoff, N. (2000). The name of the cucurbit powdery mildew: Podosphaera (sect. Sphaerotheca) xanthii (Castag.) U. Braun & N. Shish. comb. nov. Phytopathology 90, 1077–1093.
Specht, K., Siebert, R., Hartmann, I., Freisinger, U. B., Sawicka, M., Werner, A., et al. (2014). Urban agriculture of the future: an overview of sustainability aspects of food production in and on buildings. Agric. Hum. Values 31, 33–51. doi: 10.1007/s10460-013-9448-4
Strange, R. N., and Scott, P. R. (2005). Plant disease: a threat to global food security. Annu. Rev. Phytopathol. 43, 83–116. doi: 10.1146/annurev.phyto.43.113004.133839
Strausbaugh, C. A. (2015). Leuconostoc spp. Associated with root rot in sugar beet and their interaction with Rhizoctonia solani. Phytopathology 106, 432–441.
Sun, Z. B., Yuan, X. F., Zhang, H., Wu, L. F., Liang, C., and Feng, Y. J. (2013). Isolation, screening and identification of antagonistic downy mildew endophytic bacteria from cucumber. Eur. J. Plant Pathol. 137, 847–857. doi: 10.1007/s10658-013-0293-5
Truyens, S., Weyens, N., Cuypers, A., and Vangronsveld, J. (2015). Bacterial seed endophytes: genera, vertical transmission and interaction with plants. Environ. Microbiol. Rep. 7, 40–50. doi: 10.1111/1758-2229.12181
Val-Moraes, S. P. (2015). Organic Amendments and Soil Suppressiveness in Plant Disease Management: Soil Biology, eds M. K. Meghvansi and A. Varma. Cham: Springer, 175–184. doi: 10.1007/978-3-319-23075-7
Verma, S. K., Kingsley, K., Irizarry, I., Bergen, M., Kharwar, R. N., and White, J. F. (2017). Seed-vectored endophytic bacteria modulate development of rice seedlings. J. Appl. Microbiol. 122, 1680–1691. doi: 10.1111/jam.13463
Vespermann, A., Kai, M., and Piechulla, B. (2007). Rhizobacterial volatiles affect the growth of fungi and Arabidopsis thaliana. Appl. Environ. Microbiol. 73, 5639–5641. doi: 10.1128/AEM.01078-07
Walterson, A. M., and Stavrinides, J. (2015). Pantoea: insights into a highly versatile and diverse genus within the Enterobacteriaceae. FEMS Microbiol. Rev. 39, 968–984. doi: 10.1093/femsre/fuv027
Whipps, J. M. (2001). Microbial interactions and biocontrol in the rhizosphere. J. Exp. Bot. 52, 487–511. doi: 10.1093/jexbot/52.suppl_1.487
Keywords: seed endophytes, cucurbit, biocontrol, Phytophthora capsici, Pythium aphanidermatum, Rhizoctonia solani, Fusarium graminearum, powdery mildew
Citation: Khalaf EM and Raizada MN (2018) Bacterial Seed Endophytes of Domesticated Cucurbits Antagonize Fungal and Oomycete Pathogens Including Powdery Mildew. Front. Microbiol. 9:42. doi: 10.3389/fmicb.2018.00042
Received: 15 July 2017; Accepted: 09 January 2018;
Published: 05 February 2018.
Edited by:
Pilar Martínez-Hidalgo, Universidad de Salamanca, SpainReviewed by:
Shyam Lal Kandel, University of Washington, United StatesJuan F. Jimenez, Institute for Scientific and Technological Research, Mexico
Copyright © 2018 Khalaf and Raizada. This is an open-access article distributed under the terms of the Creative Commons Attribution License (CC BY). The use, distribution or reproduction in other forums is permitted, provided the original author(s) and the copyright owner are credited and that the original publication in this journal is cited, in accordance with accepted academic practice. No use, distribution or reproduction is permitted which does not comply with these terms.
*Correspondence: Manish N. Raizada, cmFpemFkYUB1b2d1ZWxwaC5jYQ==