- 1Leptospirosis Research and Expertise Unit, Institut Pasteur in New Caledonia, Institut Pasteur International Network, Noumea, New Caledonia
- 2Bacteriology and Mycology, Institute for Infectious Diseases and Zoonoses, Department of Veterinary Sciences, Faculty of Veterinary Medicine, Ludwig Maximilian University of Munich, Munich, Germany
- 3Institut des Sciences Exactes et Appliquées, Plateau MET/MEB, Université de la Nouvelle-Calédonie, Noumea, New Caledonia
- 4Bioinformatics Unit, Institut Pasteur Montevideo, Montevideo, Uruguay
- 5Biology of Spirochetes Unit, Institut Pasteur, National Reference Centre and WHO Collaborating Center for Leptospirosis, Paris, France
Leptospirosis is an important environmental disease and a major threat to human health causing at least 1 million clinical infections annually. There has recently been a growing interest in understanding the environmental lifestyle of Leptospira. However, Leptospira isolation from complex environmental samples is difficult and time-consuming and few tools are available to identify Leptospira isolates at the species level. Here, we propose a polyphasic isolation and identification scheme, which might prove useful to recover and identify environmental isolates and select those to be submitted to whole-genome sequencing. Using this approach, we recently described 12 novel Leptospira species for which we propose names. We also show that MALDI-ToF MS allows rapid and reliable identification and provide an extensive database of Leptospira MALDI-ToF mass spectra, which will be valuable to researchers in the leptospirosis community for species identification. Lastly, we also re-evaluate some of the current techniques for the molecular diagnosis of leptospirosis taking into account the extensive and recently revealed biodiversity of Leptospira in the environment. In conclusion, we describe our method for isolating Leptospira from the environment, confirm the usefulness of mass spectrometry for species identification and propose names for 12 novel species. This also offers the opportunity to refine current molecular diagnostic tools.
Introduction
Leptospirosis is a bacterial disease that affects human and animals that are exposed to a contaminated environment; usually water or soils contaminated by the urine of reservoir animals. The global burden of leptospirosis is estimated to more than 1 million cases and 60,000 fatalities annually, mostly impacting rural or suburban impoverished populations in tropical and subtropical regions worldwide (Costa et al., 2015).
Leptospires are thin helical-shaped bacteria from the phylum of Spirochaetes. One century ago, freshwater saprophytic leptospires and pathogenic leptospires, the etiological agents of leptospirosis, had been first described and rats had been identified as the main animal reservoirs of virulent strains (Ido et al., 1917; Noguchi, 1917). Prophetically, in the same issue of The Journal of Experimental Medicine, Noguchi questioned the survival of leptospires in nature, including in water and soils, and studied the interactions of Leptospira with other bacteria (Noguchi, 1918a). The foundations of the epidemiology and taxonomy of Leptospira were therefore clearly established in a very short period of time one century ago (Noguchi, 1918b).
Pathogenic Leptospira have then been classified as L. interrogans sensu lato while saprophytes were named L. biflexa sensu lato for decades (both denominations being now inappropriate in modern taxonomy), until the 1980's and the description of Leptospira inadai (Schmid et al., 1986). This species later proved to be the first representative of the so-called “intermediate” cluster (Hookey et al., 1993). At the end of the twentieth century, there were 16 validly described Leptospira species, with the description of a second intermediate species Leptospira fainei (Perolat et al., 1998) and further molecular reclassification of collection strains (Brenner et al., 1999).
In the recent years, a pan-Leptospira genome project supported by the National Institute of Allergy and Infectious Disease (NIAID) Genome Sequencing Center has provided the scientific community with hundreds of whole-genome sequences allowing large comparative genomic analyses (Lehmann et al., 2013, 2014; Fouts et al., 2016). This has offered new insights into the phylogeny of the genus Leptospira, notably confirming the discrimination of the three major clades (“pathogens,” “intermediates,” and “saprophytes”) by whole-genome analysis. Within the pathogens, this has also allowed to identify sub-clusters of varying pathogenicity (Xu et al., 2016). Our recent genomic study of environmental isolates from New Caledonia, a tropical island where leptospirosis is endemic, suggested that more heterogeneity in virulence exists in the cluster named “pathogens” and that virulence was acquired independently in “pathogens” and “intermediates” (Thibeaux et al., 2018). However, major questions are still open about the comparative virulence (Picardeau, 2017) as some species from the cluster “intermediates” are increasingly recognized in human disease (Tsuboi et al., 2017; Puche et al., 2018).
In this study, we isolated Leptospira from soils suspected to be contamination sources in New Caledonia. Isolates were submitted to a polyphasic approach for identification, with three sequential steps. First, a melting curve analysis of the 16S ribosomal RNA gene achieved a fast presumptive identification of the cluster (i.e., pathogens, intermediates, or saprophytes). A proteomic analysis based on matrix-assisted laser desorption ionization time-of-flight (MALDI-ToF) mass spectrometry using the MALDI Biotyper Systems allowed species identification through pairwise comparison with reference spectra of the 23 validly described Leptospira species. Whole-genome sequencing (WGS) for formal identification of all 26 isolates provided unambiguous identification of clusters and species, thus allowing to evaluate the relevance of the identification scheme. In total, whole-genome sequencing confirmed the relevance of this approach and showed that MALDI-ToF spectra-based identification is a powerful tool to identify Leptospira at the species level. In parallel, the biodiversity revealed from these environmental isolates also questions molecular diagnosis. Therefore, we evaluated two real time PCR assays for leptospirosis diagnosis (Merien et al., 2005; Stoddard et al., 2009) and discuss possible improvement as well as their applicability in different contexts.
Materials and Methods
Leptospira Reference Strains and Environmental Isolates
Leptospira strains used in this study (Supplementary Table 1) were from the bacterial collections of the Institut Pasteur in New Caledonia and in Paris.
Environmental isolates as well as isolation procedure have been described previously (Thibeaux et al., 2018). Briefly, 5 g of topsoil were mixed with 5 mL of PBS (phosphate buffered saline) and 2 mL of supernatant were filtered through a sterile 0.45 μm filter into a tube filled with 2.5 mL of Ellinghausen-McCullough-Johnson-Harris (EMJH) medium 2x and 0.5 mL of 10X concentrated STAFF (sulfamethoxazole, trimethoprim, amphotericin B, fosfomycin, and 5-fluorouracil) (Chakraborty et al., 2011). Cultures were incubated at 30°C and when spirochetes were observed, 100-μL of the diluted culture (1:100 and 1:1,000) was plated onto solid EMJH agar (12 g/L) using sterile glass beads and incubated at 30°C until individual subsurface colonies were visible. One to five characteristic subsurface individual colonies from each plate were collected with a sterile tip, for confirmation of a Leptospira morphology and motility by dark field microscopy before clonal subculture in liquid EMJH. The isolation procedure is summarized in Figure 1A.
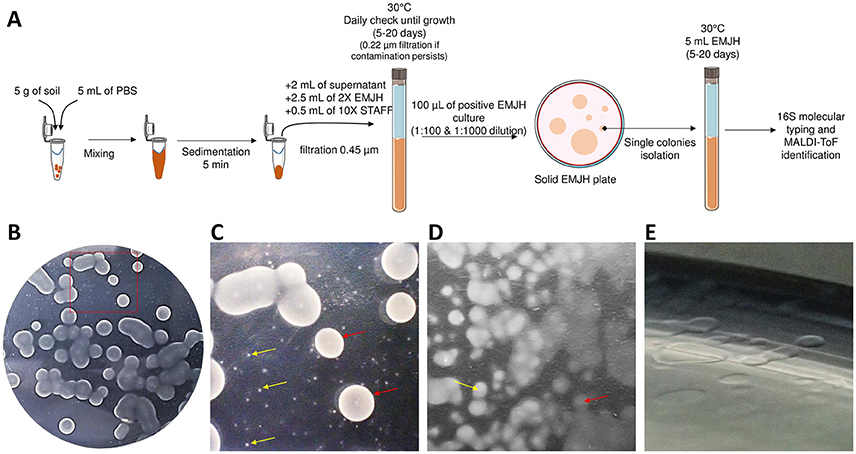
Figure 1. Leptospira isolation on EMJH agar plates. (A) Global isolation strategy. (B) Macroscopic aspect of colonies. (C) Closer view from (A) showing colonies of different sizes. (D) Opaque (yellow arrow) and transparent (red arrow) colonies. (E) Translucent colonies.
DNA Extraction and 16S RNA Molecular Typing
Genomic DNA was extracted from 200 μL of EMJH liquid clonal cultures. Extraction was performed using a commercial kit (QIAamp® DNA Mini Kit, Qiagen, Australia) according to the manufacturer's instructions. DNA elution was performed with 50 μL of buffer AE. The quantity of DNA was measured with NanoDrop™ (Thermo Fisher Scientific, Waltham, MA USA) and adjusted to 0.1 ng/μL.
Amplification of a fragment of the 16S rRNA gene was performed in a total volume of 10 μL on a LightCycler 480 II. The reaction mixture consisted in 2 μL PCR grade water, 5 μL of 2X reaction SYBR Green Mix (LC480 SYBR Green I Master kit, Roche, New Zealand), 0.5 μL of forward primer (5′-GGCGGCGCGTCTTAAACATG-3′), 0.5 μL of reverse primer (5′-CTTAACTGCTGCCTCCCGTA-3′), and 2 μL (0.2 ng) of DNA (Mérien et al., 1992). After amplification, the melting temperature of the amplified product was analyzed using LightCycler 480 Software.
MALDI-ToF Mass Spectrum Analysis: Sample Preparation and Construction of a Database
Samples were prepared as described in an earlier study (Rettinger et al., 2012) with minor changes. Briefly, Leptospira cells from liquid EMJH culture were harvested by centrifugation (18,000 g for 10 min) of 1 mL of an exponential 8-day old culture in EMJH culture medium. The pellet was resuspended in 200 μL PBS to wash cells and remove the excess of protein coming from the EMJH culture medium. Bacterial cells were pelleted again by identical centrifugation (18,000 g for 10 min). The pellet was resuspended and homogenized in 300 μL sterile distilled water before addition of 900 μL absolute ethanol. At this step, the sample can be stored up to 6 months at −20°C. The cells were pelleted again (18,000 g for 10 min), the pellet was dried under a hood. Finally, the dry pellet was thoroughly homogenized in 30 μL 70% formic acid before addition of 30 μL pure acetonitrile.
The homogeneous mixture was centrifuged 2 min at 15,000 g and 1 μL of the supernatant was spotted on a MSP-96 polished steel target plate (Bruker Daltonics, Wissembourg, France) and allowed to dry at room temperature. The sample was overlaid with 1.2 μL matrix (10 mg α-cyano-4-hydroxy-cinnamic acid / mL in 50% acetonitrile/2.5% trifluoroacetic acid). Mass spectra (MS) were acquired using a Microflex mass spectrometer (Bruker Daltonics, Wissembourg, France) in the linear mode and a mass range of 2–20 kDa using the automated functionality of flexControl 3.0 software (Bruker Daltonics, Wissembourg, France). To create reference spectra, at least 20 raw spectra (8 spots read 3 times each) were used to generate a main spectrum. A database was constructed with the reference MS of 43 strains (30 pathogenic, 6 intermediates and 7 saprophytes, Supplementary Table 1) encompassing representative of all 23 validly described species.
To identify isolates, samples were prepared similarly. The MS database was loaded into MALDI Biotyper 3.0 software (Bruker Daltonics) and used to compare the MS of the isolate with the MS database. The software MALDI Biotyper calculates a similarity score considering the proportion of matching peaks between the analyzed MS and all MS in the database. The similarity score values range from 0 (no similarity) to 3 (absolute identity). An identification score ≥2.3 was considered valid for identification at the species level as recommended by the manufacturer and confirmed in a previous study (Rettinger et al., 2012). We first loaded reference MS created in another laboratory (Rettinger et al., 2012) to evaluate the inter-laboratory portability of reference MS. Using this imported database, we submitted our routine strains and isolates (bold lines in Supplementary Table 1) to identification. We considered that an identification score in the range 2.0–2.3 could reveal “possible” novel species whereas a score below 2.0 was regarded as a “probable” novel species (Table 1). The main spectra library (MSP) dendrogram was created by the standard MALDI Biotyper MSP creation method (Bruker Daltonics, Bremen, Germany), where distance values are relative and are always normalized to a maximum value of 1,000.
Whole-Genome Sequencing and Phylogenetic Analysis
The methods for DNA extraction, Whole-genome sequencing, assembly and species identification have been described in an earlier study (Thibeaux et al., 2018). Briefly, genomic DNA was prepared by collection of cells by centrifugation from an exponential-phase liquid culture and extraction with MagNA Pure 96 Instrument (Roche Life Science, Auckland, New Zealand). Next-generation sequencing was performed by the Mutualized Platform for Microbiology (P2M) at Institut Pasteur, using the Nextera XT DNA Library Preparation kit (Illumina), the NextSeq 500 sequencing systems (Illumina), and the CLC Genomics Workbench 9 software (Qiagen) for analysis. The whole genome sequences were studied as described previously and novel species were identified by pairwise comparisons of overall genomic relatedness indices ANI and AAI (Thibeaux et al., 2018).
Ability of Routine Diagnostic PCR to Detect the Pathogenic Species
The biological diagnosis of leptospirosis increasingly uses real time PCR. Here, we tested both a Taq-Man based PCR targeting lipL32 for detection (Stoddard et al., 2009) and a lfb1 SYBR Green I real time PCR that is used for both detection and genotyping (Merien et al., 2005; Perez and Goarant, 2010). Both techniques are used in a number of diagnostic or reference laboratories globally. The lfb1 and lipL32 sequences were retrieved from whole-genomes by blastn (Altschul et al., 1990), aligned and compared to the primers and probe described before (Supplementary Figure 1). Degenerate primers were designed to evaluate a possible improved detection of lipL32 from all pathogens: lipL32-47Fd (GCATTACMGCTTGTGGTG) and lipL32-301Rd (CCGATTTCGCCWGTTGG) were used together with lipL32-189P (Stoddard et al., 2009) using the same PCR cycles. Using both qPCR designs, 0.2 ng pure DNA were amplified in a LightCycler 480 (lipL32) or in a LightCycler 2.0 (lfb1). Ct values were compared with the Ct values obtained from the same concentrations of pure DNA for each species with the original and the degenerate primers.
Scanning Electron Microscopy Imaging and Morphology
Glass coverslips (12 × 12 mm, Menzel-Glaser) were placed into 24-well polystyrene plates (Corning) with 1 mL of a bacterial suspension at 106 bacteria / mL and were incubated for 2 days. Coverslips were removed and rinsed once in PBS, then fixed in 4% paraformaldehyde / 1% glutaraldehyde for 15 min. Coverslips were rinse again in PBS and stained with 1% osmium tetroxide (OsO4 in PBS, Acros Organics) for 1 h. After staining, samples were dehydrated through a series of ethanol concentrations (25, 50, 70, 90, and 100% for 10 min each) before being desiccated using hexamethyldisilazane (HMDS, Acros Organics). Before visualization, samples were submitted to carbon sputtering to increase their electrical conductance (Em ACE600, Carbon Coater, Leica) and examined under a Jeol JSM IT-300 IntouchScope microscope (SED detector, 10 kV). Length, width and helix pitch were measured on 6–10 representative isolated cells to calculate mean dimensions.
Results
Environmental Strain Isolation
The major difficulty for isolating environmental leptospires resides in the ability to recover a clonal pure Leptospira culture from a complex polymicrobial arrangement. This procedure has been greatly facilitated by using the STAFF combination of antimicrobial agents (Chakraborty et al., 2011) which allows selective isolation of leptospires from contaminated samples. Earlier work has used quite similar combinations of agents to grow leptospires selectively with some success (Cousineau and McKiel, 1961; Jones et al., 1981). Although very effective, this is not sufficient to recover pure cultures as multiple Leptospira species can be present alongside in the initial sample. An additional step of clonal isolation from an individual isolated colony on an agar plate was necessary to recover clonal Leptospira cultures. We applied this procedure to attempt Leptospira isolation in 27 soil samples collected from 7 different sites throughout the mainland of New Caledonia (Thibeaux et al., 2018). After 5–10 days of liquid culture with STAFF, 23 cultures displayed the growth of Leptospira-like motile helical-shaped bacteria by dark-field microscopy. Positive liquid cultures were plated on solid agar plates and 26 individual colonies were sub-cloned in liquid EMJH according to their size, color and opacity (Figure 1).
16S rRNA Tm Analysis For Rapid Identification of Leptospira at the Clade-Level
The melting temperature analysis of the amplicon of a region of the 16S rRNA gene (Mérien et al., 1992) conserved in the entire Leptospira genus allowed a rapid putative identification of the clade. Melting temperature values ranged from 85.26 to 86.65 and could separate the three clades within the Leptospira genus. Tm values of the saprophytic species were the lowest, ranging from 85.26 to 85.76 without overlapping with Tm values of the other species. Intermediate species had the highest melting temperature (86.45–86.54) while pathogenic species had Tm values ranging from 85.84 to 86.20, with the remarkable exception of Leptospira weilii, belonging to the pathogenic cluster but had the highest Tm value: 86.65°C, making this particular species the only outlier reported in this study (Figure 2.). Using these cluster-specific Tm ranges, we presumptively identified 10 saprophytic, 9 intermediate and 7 pathogenic Leptospira strains from pure cultures (Table 1, Step I).
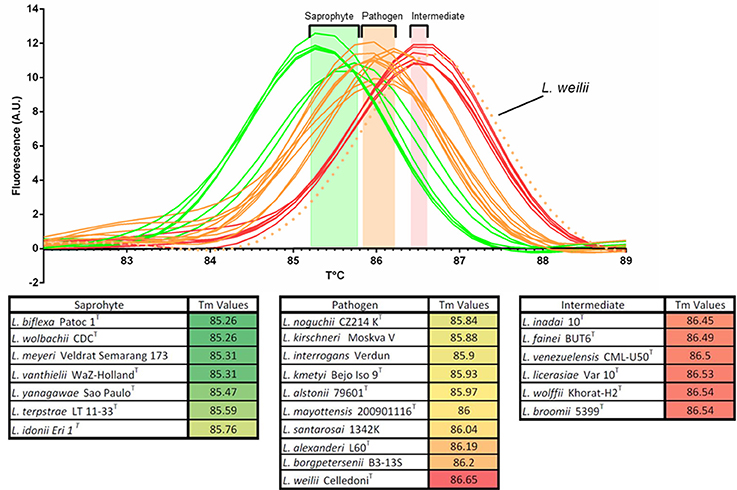
Figure 2. Melting peaks and melting temperatures (Tm) of a 16S rRNA PCR product for representative strains from all 23 validly described Leptospira species, evidencing the clustering of Tm values with the notable exception of Leptospira weilii.
MALDI-ToF Analysis for Rapid Identification of Leptospira Species
Using the imported MS database on our MALDI-ToF apparatus with our strain collection, culture medium and preparation reagents, we correctly identified all 27 reference strains at the species level with identification scores ≥2.30 and not significantly different from scores obtained with our in-house database (data not shown). These results confirm the portability of MS databases. MALDI-ToF MS was then used to attempt identification of our 26 isolates. Pairwise comparison with reference spectra representative of the 23 known Leptospira species was performed to identify isolates at the species level. Referring to the most complete database of reference spectra is a prerequisite to make use of the MALDI-ToF as an identification strategy of unknown species. In this case, an identification that fail to be matched with reference species MS with a valid score ≥2.3 would possibly point to a novel species deserving WGS analysis. Applying this identification strategy to our environmental isolates, 5 isolates had a high-confidence identification score (≥2.3) and were identified as L. kmetyi (n = 2), and L. meyeri (n = 3) (Table 1, Step II). The other 21 isolates failed to be matched with known species, suggesting possible novel species. Interestingly, we noticed for these isolates that even though the score was below the identification threshold, the proposed closest hit was always in good agreement with the proposed clade putatively assigned with the Tm of the 16S rRNA PCR product (Table 1, Step II).
Furthermore, we have refined the identification of these 21 unidentified cultures by performing pairwise comparison with each other to identify isolates belonging to the same species, if any. Some cultures were identified as identical putative novel species (comparison score ≥2.3), pointing to 13 possible or putative novel species (Table 1 and Figure 3).
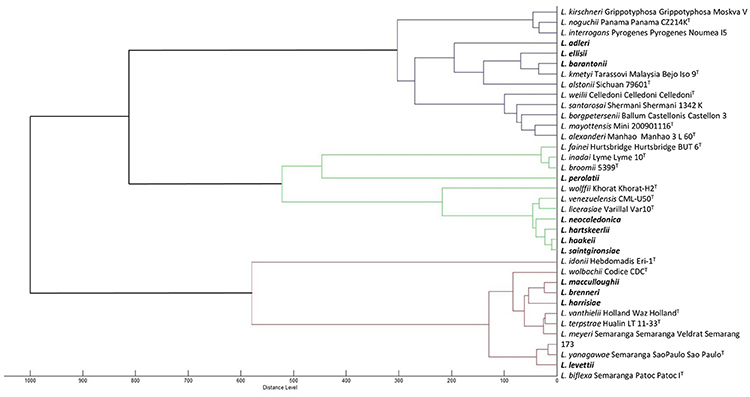
Figure 3. Dendrogram based on the comparison of MALDI-ToF mass spectra of the 35 Leptospira species. Novel species are indicated in bold.
Whole-Genome Sequencing
As the last step of the stepwise identification, the whole-genome sequence of all 26 isolates were determined. The 5 isolates identified by MALDI-ToF MS as L. meyeri (n = 3) or L. kmetyi (n = 2) were confirmed members of these species by ad-hoc analysis of whole-genome sequences (Thibeaux et al., 2018). Of 21 unknown isolates possibly representing novel species, one isolate (FH2-C-A2), assigned to a possible novel intermediate species, was formally identified as L. wolffii. Of note, the MALDI-ToF identification score with L. wolffii Khorat-H2T was 2.07, in the range of the species uncertainty suggested in a former study (Rettinger et al., 2012) and recommended by the manufacturer. In addition, 20 isolates were confirmed to belong to 12 novel Leptospira species (3 pathogens, 5 intermediates and 4 saprophytes) by WGS and genome analysis (Thibeaux et al., 2018) (Table 1, step III).
Description of Novel Species
Figure 4 shows the morphology of novel species obtained by scanning electron microscopy. The position of novel species in a 16S rRNA phylogenetic tree is shown in Supplementary Figure 2. We propose the following names and descriptions for the novel species:
Description of Leptospira ellisii sp. nov
Leptospira ellisii (e.li'si.i N.L gen. masc. n. ellisii of Ellis, named after Dr William A. Ellis, an Irish veterinary microbiologist who made significant contributions to the study of Leptospira and leptospirosis in animals). Morphology observed under scanning electron microscopy is consistent with those for the genus. Cells have helical morphology, finely thin and usually curved at each end, forming a semicircular hook. Cells are 11.13 ± 1 μm long and 221 ± 18 nm in diameter with a pitch of 396 ± 44 nm. Motile and aerobic. Cells grow well in liquid and solid EMJH medium (1.2% agar) at 30°C. Growth in the presence of antibiotics, such as 5-fluorouracil, 1 mg/mL; Amphotericin B, 50 μg/mL; Sulfamethoxazole, 400 μg/mL; Trimethoprim, 200 μg/mL and Fosmomycin, 4 mg/mL was also observed. The genomic G+C content of the type strain is 47.8%. This strain could neither induce signs or symptoms of infection in the hamster model nor be recovered from hamster blood. Similarly, it could not be detected in mouse urine or kidney after inoculation. The type strain isolated to date is ATI7-C-A5T, isolated from a soil sample in the South Province of New Caledonia. Genome Accession Number is NPEF00000000. Belonging to the cluster of “pathogens,” this species was formerly described as Leptospira sp. nov. patho 1 (Thibeaux et al., 2018).
Description of Leptospira barantonii sp. nov
Leptospira barantonii (ba.ran.to'ni.i. N.L gen. masc. n. barantonii of Baranton, named after Pr Guy Baranton, a French microbiologist who made significant contributions to the study of Spirochaetes). Morphology observed under scanning electron microscopy is consistent with those for the genus. Cells have helical morphology, finely thin and usually curved at each end, forming a semicircular hook. Cells are 13.26 ± 2.16 μm long and 208 ± 33 nm in diameter with a pitch of 448 ± 31 nm. Motile and aerobic. Cells grow well in liquid and solid EMJH medium (1.2% agar) at 30°C. Growth in the presence of antibiotics, such as 5-fluorouracil, 1 mg/mL; Amphotericin B, 50 μg/mL; Sulfamethoxazole, 400 μg/mL; Trimethoprim, 200 μg/mL and Fosmomycin, 4 mg/mL was also observed. The genomic G+C content of the type strain is 43.96%. This strain could neither induce signs or symptoms of infection in the hamster model nor be recovered from hamster blood. Similarly, it could not be detected in mouse urine or kidney after inoculation. The type and only strain isolated to date is FH4-C-A1T, isolated from a soil sample in the North Province of New Caledonia. Genome Accession Number is NPDS00000000. Belonging to the cluster of “pathogens,” this species was formerly described as Leptospira sp. nov. patho 2 (Thibeaux et al., 2018).
Description of Leptospira adleri sp. nov
Leptospira adler (a.dle'ri. N.L gen. masc. n. adleri of Adler, named after Pr Ben Adler, an Australian microbiologist who made significant research contributions in many aspects of Leptospira and leptospirosis). Morphology observed under scanning electron microscopy is consistent with those for the genus. Cells have helical morphology, finely thin and usually curved at each end, forming a semicircular hook. Cells are 11.52 ± 1.73 μm long and 178 ± 17 nm in diameter with a pitch of 454 ± 31 nm. Motile and aerobic. Cells grow well in liquid and solid EMJH medium (1.2% agar) at 30°C. Growth in the presence of antibiotics, such as 5-fluorouracil, 1 mg/mL; Amphotericin B, 50 μg/mL; Sulfamethoxazole, 400 μg/mL; Trimethoprim, 200 μg/mL and Fosmomycin, 4 mg/mL was also observed. The genomic G+C content of the type strain is 43.55%. This strain could neither induce signs or symptoms of infection in the hamster model nor be recovered from hamster blood. Similarly, it could not be detected in mouse urine or kidney after inoculation. The type strain isolated to date is FH2-B-D1T, isolated from a soil sample in the North Province of New Caledonia. Genome Accession Number is NPDU00000000. Belonging to the cluster of “pathogens,” this species was formerly described as Leptospira sp. nov. patho 3 (Thibeaux et al., 2018).
Description of Leptospira perolatii sp. nov
Leptospira perolatii (pe.ro.la'ti.i. N.L gen. masc. n. perolatii of Perolat, named after Dr Philippe Perolat, a French microbiologist who made significant contributions to the study of Leptospira). Morphology observed under scanning electron microscopy is consistent with those for the genus. Cells have helical morphology, finely thin and usually curved at each end, forming a semicircular hook but spiralstructure of this isolate appear more relaxed. Cells are 11.35 ± 1.13 μm long and 153 ± 20 nm in diameter with a pitch of 596 ± 58 nm. Motile and aerobic. Cells grow well in liquid and solid EMJH medium (1.2% agar) at 30°C. Growth in the presence of antibiotics, such as 5-fluorouracil, 1 mg/mL; Amphotericin B, 50 μg/mL; Sulfamethoxazole, 400 μg/mL; Trimethoprim, 200 μg/mL and Fosmomycin, 4 mg/mL was also observed. The genomic G+C content of the type strain is 42.36%. The type strain isolated to date is FH1-B-B1T, isolated from a soil sample in the North Province of New Caledonia. Genome Accession Number is NPDZ00000000. Belonging to the cluster of “intermediates,” this species was formerly described as Leptospira sp. nov. inter 1 (Thibeaux et al., 2018).
Description of Leptospira neocaledonica sp. nov
Leptospira neocaledonica (neo.ca.le.do.ni'ca. N.L gen. fem. adj. neocaledonica reflecting its geographic origin in New Caledonia). Morphology observed under scanning electron microscopy is consistent with those for the genus. Cells have helical morphology, finely thin and usually curved at each end, forming a semicircular hook. Cells are 11.82 ± 1.13 μm long and 152 ± 20 nm in diameter with a pitch of 498 ± 49 nm. Motile and aerobic. Cells grow well in liquid and solid EMJH medium (1.2% agar) at 30°C. Growth in the presence of antibiotics, such as 5-fluorouracil, 1 mg/mL; Amphotericin B, 50 μg/mL; Sulfamethoxazole, 400 μg/mL; Trimethoprim, 200 μg/mL and Fosmomycin, 4 mg/mL was also observed. The genomic G+C content of the type strain is 40.17%. The type and only strain isolated to date is ES4-C-A1T, isolated from a soil sample in the North Province of New Caledonia. Genome Accession Number is NPEA00000000. Belonging to the cluster of “intermediates,” this species was formerly described as Leptospira sp. nov. inter 2 (Thibeaux et al., 2018).
Description of Leptospira saintgironsiae sp. nov
Leptospira saintgironsiae (saint.gi.ron'si.ae. N.L gen. fem. n. saintgironsiae of Saint-Girons, named after Dr Isabelle Saint-Girons, a French microbiologist who made significant contributions to the study of Spirochaetes). Morphology observed under scanning electron microscopy is consistent with those for the genus. Cells have helical morphology, finely thin and usually curved at each end, forming a semicircular hook. Cells are 13.06 ± 1.27 μm long and 152 ± 14 nm in diameter with a pitch of 469 ± 74 nm. Motile and aerobic. Cells grow well in liquid and solid EMJH medium (1.2% agar) at 30°C. Growth in the presence of antibiotics, such as 5-fluorouracil, 1 mg/mL; Amphotericin B, 50 μg/mL; Sulfamethoxazole, 400 μg/mL; Trimethoprim, 200 μg/mL and Fosmomycin, 4 mg/mL was also observed. The genomic G+C content of the type strain is 45.82%. The type and only strain isolated to date is FH4-C-A2T, isolated from a soil sample in the North Province of New Caledonia. Genome Accession Number is NPDR00000000. Belonging to the cluster of “intermediates,” this species was formerly described as Leptospira sp. nov. inter 3 (Thibeaux et al., 2018).
Description of Leptospira haakeii sp. nov
Leptospira haakeii (ha.a'kei.i. N.L gen. masc. n. haakeii of Haake, named after Pr David Haake, an American microbiologist who made significant contributions to the study of Leptospira, notably on the role of outer membrane proteins in the pathophysiology of leptospirosis). Morphology observed under scanning electron microscopy is consistent with those for the genus. Cells have helical morphology, finely thin and usually curved at each end, forming a semicircular hook. Cells are 13.89 ± 1.06 μm long and 183 ± 5 nm in diameter with a pitch of 444 ± 58 nm. Motile and aerobic. Cells grow well in liquid and solid EMJH medium (1.2% agar) at 30°C. Growth in the presence of antibiotics, such as 5-fluorouracil, 1 mg/mL; Amphotericin B, 50 μg/mL; Sulfamethoxazole, 400 μg/mL; Trimethoprim, 200 μg/mL and Fosmomycin, 4 mg/mL was also observed. The genomic G+C content of the type strain is 39.8%.The type strain isolated to date is ATI7-C-A4T, isolated from a soil sample in the South Province of New Caledonia. Genome Accession Number is NPEG00000000. Belonging to the cluster of “intermediates,” this species was formerly described as Leptospira sp. nov. inter 4 (Thibeaux et al., 2018).
Description of Leptospira hartskeerlii sp. nov
Leptospira hartskeerlii (hart.skeer'li.i. N.L gen. masc. n. hartskeerlii of Harskeerl named after Dr Rudy Harskeerl, an Dutch microbiologist who made significant contributions to the study of all aspects of leptospirosis, notably diagnosis and epidemiology). Morphology observed under scanning electron microscopy is consistent with those for the genus. Cells have helical morphology, finely thin and usually curved at each end, forming a semicircular hook. Cells are 11.41 ± 2.51 μm long and 147 ± 7 nm in diameter with a pitch of 345 ± 45 nm. Motile and aerobic. Cells grow well in liquid and solid EMJH medium (1.2% agar) at 30°C. Growth in the presence of antibiotics, such as 5-fluorouracil, 1 mg/mL; Amphotericin B, 50 μg/mL; Sulfamethoxazole, 400 μg/mL; Trimethoprim, 200 μg/mL and Fosmomycin, 4 mg/mL was also observed. The genomic G+C content of the type strain is 40.47%. The type strain isolated to date is MCA2-B-A3T, isolated from a soil sample in the North Province of New Caledonia. Genome Accession Number is NPDL00000000. Belonging to the cluster of “intermediates,” this species was formerly described as Leptospira sp. nov. inter 5 (Thibeaux et al., 2018).
Description of Leptospira harrisiae sp. nov
Leptospira harrisiae (ha.rri'si.ae. N.L gen. fem. n. harrisiae of Harris named after Dr Virginia G. Harris, an American microbiologist who made significant contributions to the study of nutrition and physiology of Leptospira). Morphology observed under scanning electron microscopy is consistent with those for the genus. Cells have helical morphology, finely thin and usually curved at each end, forming a semicircular hook. Cells are 10.31 ± 1.38 μm long and 202 ± 8 nm in diameter with a pitch of 481 ± 58 nm. Motile and aerobic. Cells grow well in liquid and solid EMJH medium (1.2% agar) at 30°C. Growth in the presence of antibiotics, such as 5-fluorouracil, 1 mg/mL; Amphotericin B, 50 μg/mL; Sulfamethoxazole, 400 μg/mL; Trimethoprim, 200 μg/mL and Fosmomycin, 4 mg/mL was also observed. The genomic G+C content of the type strain is 37.86%. The type strain isolated to date is FH2-B-A1T, isolated from a soil sample in the North Province of New Caledonia. Genome Accession Number is NPDX00000000. Belonging to the cluster of “saprophytes,” this species was formerly described as Leptospira sp. nov. sapro 1 (Thibeaux et al., 2018).
Description of Leptospira levettii sp. nov
Leptospira levettii (le'vet.ti.i. N.L gen. masc. n. levettii of Levett named after Dr Paul N. Levett, a Canadian microbiologist who made significant contributions to the study of Leptospira and leptospirosis). Morphology observed under scanning electron microscopy is consistent with those for the genus. Cells have helical morphology, finely thin and usually curved at each end, forming a semicircular hook. Cells are 12.88 ± 1.45 μm long and 184 ± 14 nm in diameter with a pitch of 563 ± 14 nm. Motile and aerobic. Cells grow well in liquid and solid EMJH medium (1.2% agar) at 30°C. Growth in the presence of antibiotics, such as 5-fluorouracil, 1 mg/mL; Amphotericin B, 50 μg/mL; Sulfamethoxazole, 400 μg/mL; Trimethoprim, 200 μg/mL and Fosmomycin, 4 mg/mL was also observed. The genomic G+C content of the type strain is 37.61%. The type strain isolated to date is MCA2-B-A1T, isolated from a soil sample in the North Province of New Caledonia. Genome Accession Number is NPDM00000000. Belonging to the cluster of “saprophytes,” this species was formerly described as Leptospira sp. nov. sapro 2 (Thibeaux et al., 2018).
Description of Leptospira brenneri sp. nov
Leptospira brenneri (bre.nne.ri. N.L gen. masc. n. brenneri of Brenner named after Dr Don J. Brenner, an American microbiologist who made significant contributions to the study of Leptospira, notably pioneered the field of molecular taxonomy). Morphology observed under scanning electron microscopy is consistent with those for the genus. Cells have helical morphology, finely thin and usually curved at each end, forming a semicircular hook. Cells are 11.17 ± 2.12 μm long and 175 ± 7 nm in diameter with a pitch of 538 ± 30 nm. Motile and aerobic. Cells grow well in liquid and solid EMJH medium (1.2% agar) at 30°C. Growth in the presence of antibiotics, such as 5-fluorouracil, 1 mg/mL; Amphotericin B, 50 μg/mL; Sulfamethoxazole, 400 μg/mL; Trimethoprim, 200 μg/mL and Fosmomycin, 4 mg/mL was also observed. The genomic G+C content of the type strain is 38.34%. The type and only strain isolated to date is JW2-C-A2T, isolated from a soil sample in the North Province of New Caledonia. Genome Accession Number is NPDQ00000000. Belonging to the cluster of “saprophytes,” this species was formerly described as Leptospira sp. nov. sapro 3 (Thibeaux et al., 2018).
Description of Leptospira macculloughii sp. nov
Leptospira macculloughii (mac.cu.llou'ghi.i. N.L gen. masc. n. macculloughii of McCullough named after Dr. Willard G. McCullough, an American microbiologist who made significant contributions to the study of the nutritional requirements of Leptospira). Morphology observed under scanning electron microscopy is consistent with those for the genus. Cells have helical morphology, finely thin and usually curved at each end, forming a semicircular hook. Cells are 11.11 ± 1.63 μm long and 190 ± 8 nm in diameter with a pitch of 514 ± 46 nm. Motile and aerobic. Cells grow well in liquid and solid EMJH medium (1.2% agar) at 30 °C. Growth in the presence of antibiotics, such as 5-fluorouracil, 1 mg/mL; Amphotericin B, 50 μg/mL; Sulfamethoxazole, 400 μg/mL; Trimethoprim, 200 μg/mL and Fosmomycin, 4 mg/mL was also observed. The genomic G+C content of the type strain is 37.93%. The type and only strain isolated to date is ATI2-C-A1T, isolated from a soil sample in the North Province of New Caledonia. Genome Accession Number is NPEK00000000. Belonging to the cluster of “saprophytes,” this species was formerly described as Leptospira sp. nov. sapro 4 (Thibeaux et al., 2018).
Evaluation and Optimization of qPCR Targeting the Pathogenic Species
Alignments of lipL32 and lfb1 gene sequences from previously described and novel pathogenic species revealed polymorphisms resulting in mismatches in the primers and probe of the diagnostic qPCR designs tested. Using routine protocols, the novel species were still amplified and detected, but with much higher Cycle threshold (Ct) values, pointing to a low sensitivity of these diagnostic PCR for these novel species as well as others. Figure 5 shows the real-time PCR amplification curves and Ct values for qPCR using lipL32 as the target. The degenerate lipL32 primers lipL32-47Fd and lipL32-301Rd (Supplementary Figure 1) used in conjunction with the probe lipL32-189P described in the original design (Stoddard et al., 2009) demonstrated a higher efficacy to detect all species from the cluster “pathogens,” Indeed, using these degenerate lipL32 primers, an astonishing Ct gain (showing improved sensitivity) of 16.94 cycles was observed for L. alexanderi. PCR sensitivity was also greatly increased for L. mayottensis (a 12.34-cycle gain), L. alstonii (7.06 cycles), L. kmetyi (6.72 cycles), L. adleri sp. nov. (3.6 cycles), L. ellisii sp. nov. (1.82 cycles), L. weilii (1.58 cycles) and L. barantonii sp. nov. (1.22 cycles). For L. borgpetersenii, L. noguchii, L. kirschneri, L. interrogans and L. santarosai, PCR sensitivity was barely affected with respectively + 0.17 cycle, −0.26 cycle, −0.08 cycle, −0.17 cycle, +0.16 cycle. All these results are summarized in Supplementary Table 2. A proper evaluation of this new qPCR design in terms of sensitivity and specificity would still be needed before it could be used for diagnostic purpose.
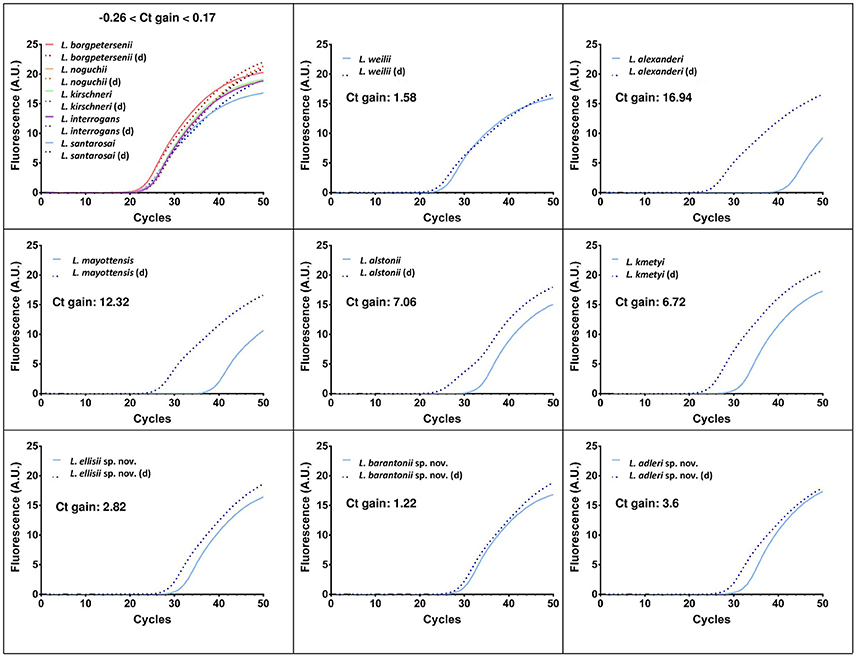
Figure 5. Real-time PCR amplification curves and Ct gains using the original (Merien et al., 2005) and a modified qPCR using degenerate primers (d) described in this paper targeting lipL32 to detect pathogenic Leptospira.
Concerning Ct values and amplification curves for qPCR using lfb1 as a target, important discrepancies were also observed with Ct values ranging from 23.43 to 47.48 also resulting from important sequence polymorphisms affecting primer match. Comparative Ct values and Melting temperatures obtained with the lfb1 qPCR (Merien et al., 2005) are presented in Supplementary Table 3.
Discussion
In the last decade, the size of the genus Leptospira has increased through the description of novel species from clinical specimens, either belonging to the intermediate cluster like Leptospira broomii (Levett et al., 2006), Leptospira licerasiae (Matthias et al., 2008), Leptospira wolffii (Slack et al., 2008), Leptospira venezuelensis (Puche et al., 2018) or to the pathogenic cluster with Leptospira mayottensis (Bourhy et al., 2014) and novel species from soils (Thibeaux et al., 2018). Simultaneously, concern is increasing about intermediate species being etiological agents of human disease (Tsuboi et al., 2017). This highlights both the need to continue Leptospira isolation to better evaluate the diversity of this genus (Girault et al., 2017) and a growing need for a universal PCR assay for the diagnostic of both intermediate and pathogenic Leptospira from clinical specimens.
In this study, we first report the stepwise identification of 26 Leptospira isolates from tropical freshwater soils in New Caledonia. Studying Leptospira in water and soil has first revealed (Ganoza et al., 2006; Viau and Boehm, 2011), then confirmed an overlooked biodiversity within the genus Leptospira (Thibeaux et al., 2018). These studies support the concept that leptospires are primarily telluric saprophytic bacteria and that only some members have evolved toward pathogenicity. From an epidemiological viewpoint, this illustrates the importance of soils in the infection cycle by virulent strains, pointing to the notion that ecosystems that support the survival of virulent leptospires should be considered as a passive, yet major reservoir in the epidemiology of the disease. Not only water content and pH, but a number of soil parameters may determine the suitability of a soil to leptospires and condition the risk to human and animal health.
To progress in this understanding, the discovery of this high biodiversity also reinforces the need of accurate isolation and identification techniques as well as relevant diagnostic tools. For isolation, an essential technical step was to plate positive liquid cultures on EMJH-agar to pick each isolate from a single colony, as shown by the diversity on plates from a single liquid culture (Figure 1). For identification, we used a polyphasic approach based on three sequential steps: (i) melting temperature analysis of a PCR product within the 16S rRNA gene, (ii) MALDI-ToF MS pairwise comparisons to a broad MS database and (iii) WGS analyses.
The recovery of isolates from the cluster “pathogens” from the environment is fastidious and challenging (Henry et al., 1971). Pathogenic leptospires have been historically isolated from soils in Malaysia (Baker and Baker, 1970; Alexander et al., 1975), using inoculation to a large number of susceptible animals, a technique nowadays ethically unacceptable. In addition, this animal-based strategy is biased since only strains leading to hamster disease could be recovered. Only virulent strains at a concentration above the infective dose can be recovered with this technique. Attempts to isolate Leptospira from the environment has led to the description of L. kmetyi from soil and other successful isolation of pathogenic leptospires from the environment in Malaysia (Slack et al., 2009; Ridzlan et al., 2010; Benacer et al., 2013). The use of selective agents to isolate leptospires from complex samples was imagined in the early 1960s (Cousineau and McKiel, 1961), successfully used later (Jones et al., 1981), and a revised combination was successfully used recently (Chakraborty et al., 2011).
Comparative analysis of the small ribosomal 16S rRNA subunit gene has been regarded as a standard for bacterial species identification for decades. In Leptospira, this gene was also largely used as a target for diagnostics (Mérien et al., 1992; Smythe et al., 2002) and typing (Ahmed et al., 2006; Guernier et al., 2018). Sequences of partial 16S rRNA gene are also widely used to identify Leptospira at the species level, either by direct sequencing (Benacer et al., 2013; Saito et al., 2014; Chaiwattanarungruengpaisan et al., 2018) or using High Resolution Melting (Pelaez Sanchez et al., 2017). However, partial sequences of the 16S rRNA gene might not be able to differentiate between closely-related species (Guernier et al., 2018), possibly leading to inconclusive or even improper identification at the species level. In recent studies, many isolates were putatively identified as L. alstonii, L. wolffii, or L. licerasiae based on their 16S rRNA gene sequence (Chakraborty et al., 2011; Benacer et al., 2013; Saito et al., 2013; Azali et al., 2016). Here, we suggest that these isolates would deserve further characterization. Hence, we propose that the simple melting temperature after real time PCR of a short portion of the 16S rRNA gene can presumptively classify an isolate in one of the 3 Leptospira clusters (pathogens, intermediates and saprophytes), with the notable exception of L. weilii (Table 1 and Figure 2).
We then used MALDI-ToF to identify pure cultures by creating a broad MS database allowing automated pairwise comparison to all validly described species. This technology is emerging in the field of medical microbiology and already used in the genus Leptospira (Djelouadji et al., 2012; Rettinger et al., 2012; Calderaro et al., 2014). As expected from previous work, we were able to identify some environmental isolates as members of species already described in all three Leptospira clusters. The MSP dendrogram deduced from the MALDI-ToF MS fingerprints clearly separated the three main clusters within the genus Leptospira and successfully distinguished all previously described species (Figure 3). Lastly, we used whole-genome sequence analyses to provide an identification of species (Thibeaux et al., 2018). This unambiguous identification of species confirmed the validity of the MALDI-ToF identifications, with correct species assignment for identification scores ≥2.3. WGS analyses also identified one isolate as L. wolffii; pairwise comparisons of MS to L. wolffii Khorat KH2T had an identification score of 2.07, in the “uncertain identification” range. Within this range, all other isolates corresponded to novel species not included in the MS database. Lastly, isolates with an identification score below 2.0 all corresponded to novel species. After WGS analyses and phylogenetic assignment of novel species, we confirmed the correct identification of the cluster based on 16S rRNA melting peaks (Figure 2). Compared to MALDI-ToF identifications, we also noted that the closest match was always a species from the same Leptospira cluster (Figure 3). Taken together, we propose a sequential approach to isolate and identify environmental Leptospira. Although MALDI-ToF bacterial identification is not readily available in every research laboratory, the possibility to halt the identification process by freezing the protein extract (see section Materials and Methods) offers the possibility to refer specimens to distant laboratories. MALDI-ToF-based species identification requires a broad MS database, which is provided for research use as part of our article (Supplementary: MspExport_BDD-UREL.btmsp file available online).
The diagnostic and surveillance of leptospirosis has mostly used serology and culture for decades. Since the advent of molecular techniques, PCR and real time PCR have been most widely used, frequently leading to abandon culture isolation (Perez and Goarant, 2010; Girault et al., 2017). However, most of the current molecular techniques specifically target the pathogenic cluster of Leptospira, or take L. interrogans sequences as the model target. The real time PCR primers (and eventually probe) may not be fitted to all relevant species. We tested two real time PCR assays widely used for diagnosis over the 10 validly described pathogenic species as well as the 3 novel pathogens. Surprisingly, we found that a TaqMan-based real time PCR targeting lipL32 that is widely used (Stoddard et al., 2009) was poorly sensitive for the detection of several Leptospira from the pathogenic cluster, including L. alexanderi and L. mayottensis (Supplementary Table 2). Using a new set of degenerate primers with the original probe of a TaqMan assay used widely, we significantly improved the detection of these species (Figure 5 and Supplementary Table 2). Similarly, a SYBR-Green I technique targeting lfb1 that is used for diagnostic purpose (Merien et al., 2005) was poorly sensitive for the detection of L. alstonii, L. kmetyi and the novel pathogenic species (Supplementary Table 3). This suggests that the high specificity of diagnostic PCR might lead to miss the detection of several rare or atypical Leptospira strains. The modified design proposed here should be tested further for validation of sensitivity and specificity, but may already be useful for exploratory studies.
LipL32 is known to be present in all species from both “pathogens” and “intermediate” clusters. However, this gene is not necessary for virulence (Murray et al., 2009) and is also present in low-virulence species described recently (Thibeaux et al., 2018). In addition and because of gene sequence polymorphisms, the most used real time PCR techniques targeting lipL32 do not detect intermediate species (Levett et al., 2005; Stoddard et al., 2009). Genus-specific targets might be used in clinical specimens, where only virulent leptospires are expected to be present. However and because recent work strongly suggests very distinct virulence levels within the pathogenic cluster (Lehmann et al., 2014; Xu et al., 2016; Thibeaux et al., 2018), molecular detection in environmental samples should either be followed by typing (Thibeaux et al., 2017) or interpreted with caution until validated virulence-specific detection methods are available. The identification of relevant target genes by comparative genomics will help improve the relevance of future diagnostic tools.
Author Contributions
CG conceived and designed the study. CG, RT, DG, EB, and MESG designed isolation strategy and isolated leptospires. CG, RT, EB, and MESG developed and implemented molecular strategy. DG, AR, and CG developed MALDI-ToF techniques and constructed MSP database. GI, MP, and CG WGS and analysis. RT, AD, and MM did the Electron Microscopy. CG, RT, DG, GI, and MP analyzed the data. CG, RT, and DG wrote the manuscript. MP, GI, and AR revised and edited the manuscript. All authors validated the manuscript.
Conflict of Interest Statement
The authors declare that the research was conducted in the absence of any commercial or financial relationships that could be construed as a potential conflict of interest.
Acknowledgments
This work was supported by a PTR (grant 30-17) from the Institut Pasteur (Paris). The researcher position of RT was supported in 2015-2017 by an AXA Research Funds grant AXA Postdoctoral Fellowship 15-AXA-PDOC-037 and by the Government of New Caledonia since 2018. EB has a doctoral grant from the Institut Pasteur International Network. GI is supported by the Fondo de Convergencia Estructural del Mercosur (FOCEM) grant COF 04/11. Thanks are due to Emilie Barsac, Benjamin De Georges De Ledenon and Julien Colot, for technical assistance with the MALDI-ToF and to Vincent Enouf and his platform team [Institut Pasteur, Pasteur International Bioresources network (PIBnet), Mutualized Platform for Microbiology (P2M)] for the next-generation sequencing analysis. Thanks are also due to the CRESICA [Consortium for Research, Higher Education and Innovation in New Caledonia] for funding the SEM platform. Leptospira collection permits were obtained from the North (# 60912-2002-2017/JJC) and South (Arrêté 1689-2017/ARR/DENV) Provinces of New Caledonia.
Supplementary Material
The Supplementary Material for this article can be found online at: https://www.frontiersin.org/articles/10.3389/fmicb.2018.00816/full#supplementary-material
MALDI-ToF database with mass spectra of 35 Leptospira species (btmsp file).
References
Ahmed, N., Devi, S. M., Valverde Mde, L., Vijayachari, P., Machang'u, R. S., Ellis, W. A., et al. (2006). Multilocus sequence typing method for identification and genotypic classification of pathogenic Leptospira species. Ann. Clin. Microbiol. Antimicrob. 5:28. doi: 10.1186/1476-0711-5-28
Alexander, A. D., Evans, L. B., Baker, M. F., Ellison, D., and Marriapan, M. (1975). Pathogenic Leptospiras isolated from Malaysian surface waters. Appl. Microbiol. 29, 30–33.
Altschul, S. F., Gish, W., Miller, W., Myers, E. W., and Lipman, D. J. (1990). Basic local alignment search tool. J. Mol. Biol. 215, 403–410. doi: 10.1016/S0022-2836(05)80360-2
Azali, M. A., Yean Yean, C., Harun, A., Aminuddin Baki, N. N., and Ismail, N. (2016). Molecular characterization of Leptospira spp. in environmental samples from North-Eastern Malaysia revealed a pathogenic strain, Leptospira alstonii. J. Trop. Med. 2016:2060241. doi: 10.1155/2016/2060241
Baker, M. F., and Baker, H. J. (1970). Pathogenic Leptospira in Malaysian surface waters. I. a method of survey for Leptospira in natural waters and soils. Am. J. Trop. Med. Hyg. 19, 485–492. doi: 10.4269/ajtmh.1970.19.485
Benacer, D., Woh, P. Y., Mohd Zain, S. N., Amran, F., and Thong, K. L. (2013). Pathogenic and Saprophytic Leptospira species in water and soils from selected urban sites in Peninsular Malaysia. Microbes Environ. JSME 28, 135–140. doi: 10.1264/jsme2.ME12154
Bourhy, P., Collet, L., Brisse, S., and Picardeau, M. (2014). Leptospira mayottensis sp., nov., a pathogenic Leptospira species isolated from humans. Int. J. Syst. Evol. Microbiol. 64, 4061–4067. doi: 10.1099/ijs.0.066597-0
Brenner, D. J., Kaufmann, A. F., Sulzer, K. R., Steigerwalt, A. G., Rogers, F. C., and Weyant, R. S. (1999). Further determination of DNA relatedness between serogroups and serovars in the family Leptospiraceae with a proposal for Leptospira alexanderi sp. nov. and four new Leptospira genomospecies. Int. J. Syst. Bacteriol. 49, 839–858. doi: 10.1099/00207713-49-2-839
Calderaro, A., Piccolo, G., Gorrini, C., Montecchini, S., Buttrini, M., Rossi, S., et al. (2014). Leptospira species and serovars identified by MALDI-TOF mass spectrometry after database implementation. BMC Res. Notes 7:330. doi: 10.1186/1756-0500-7-330
Chaiwattanarungruengpaisan, S., Suwanpakdee, S., Sangkachai, N., Chamsai, T., Taruyanon, K., and Thongdee, M. (2018). Potential pathogenic Leptospira species isolated from waterfall in Thailand. Jpn. J. Infect. Dis. 71, 65–67. doi: 10.7883/yoken.JJID.2017.363
Chakraborty, A., Miyahara, S., Villanueva, S. Y., Saito, M., Gloriani, N. G., and Yoshida, S. I. (2011). A novel combination of selective agents for isolation of Leptospira species. Microbiol. Immunol. 55, 494–501. doi: 10.1111/j.1348-0421.2011.00347.x
Costa, F., Hagan, J. E., Calcagno, J., Kane, M., Torgerson, P., Martinez-Silveira, M. S., et al. (2015). Global morbidity and mortality of leptospirosis: a systematic review. PLoS Negl. Trop. Dis. 9:e0003898. doi: 10.1371/journal.pntd.0003898
Cousineau, J. G., and McKiel, J. A. (1961). In vitro sensitivity of Leptospira to various antimicrobial agents. Can. J. Microbiol. 7, 751–758. doi: 10.1139/m61-089
Djelouadji, Z., Roux, V., Raoult, D., Kodjo, A., and Drancourt, M. (2012). Rapid MALDI-TOF mass spectrometry identification of Leptospira organisms. Vet. Microbiol. 158, 142–146. doi: 10.1016/j.vetmic.2012.01.028
Fouts, D. E., Matthias, M. A., Adhikarla, H., Adler, B., Amorim-Santos, L., Berg, D. E., et al. (2016). What makes a bacterial species pathogenic?:comparative genomic analysis of the genus Leptospira. PLoS Negl. Trop. Dis. 10:e0004403. doi: 10.1371/journal.pntd.0004403
Ganoza, C. A., Matthias, M. A., Collins-Richards, D., Brouwer, K. C., Cunningham, C. B., Segura, E. R., et al. (2006). Determining risk for severe leptospirosis by molecular analysis of environmental surface waters for pathogenic Leptospira. PLoS Med. 3:e308. doi: 10.1371/journal.pmed.0030308
Girault, D., Soupé-Gilbert, M. E., Geroult, S., Colot, J., and Goarant, C. (2017). Isolation of Leptospira from blood culture bottles. Diagn. Microbiol. Infect. Dis. 88, 17–19. doi: 10.1016/j.diagmicrobio.2017.01.014
Guernier, V., Allan, K. J., and Goarant, C. (2018). Advances and challenges in barcoding pathogenic and environmental Leptospira. Parasitology doi: 10.1017/S0031182017001147. [Epub ahead of print].
Henry, R. A., Johnson, R. C., Bohlool, B. B., and Schmidt, E. L. (1971). Detection of Leptospira in soil and water by immunofluorescence staining. Appl. Microbiol. 21, 953–956.
Hookey, J. V., Bryden, J., and Gatehouse, L. (1993). The use of 16S rDNA sequence analysis to investigate the phylogeny of Leptospiraceae and related spirochaetes. J. Gen. Microbiol. 139, 2585–2590. doi: 10.1099/00221287-139-11-2585
Ido, Y., Hoki, R., Ito, H., and Wani, H. (1917). The rat as a carrier of Spirochaeta Icterohaemorrhaguae, the causative agent of Weil's disease (Spirochaetosis Icterohaemorrhagica). J. Exp. Med. 26, 341–353. doi: 10.1084/jem.26.3.341
Jones, P. W., Rennison, L. M., Matthews, P. R., Collins, P., and Brown, A. (1981). The occurrence and significance to animal health of Leptospira, Mycobacterium, Escherichia coli, Brucella abortus and Bacillus anthracis in sewage and sewage sludges. J. Hyg. 86, 129–137. doi: 10.1017/S0022172400068820
Lehmann, J. S., Fouts, D. E., Haft, D. H., Cannella, A. P., Ricaldi, J. N., Brinkac, L., et al. (2013). Pathogenomic inference of virulence-associated genes in Leptospira interrogans. PLoS Negl. Trop. Dis. 7:e2468. doi: 10.1371/journal.pntd.0002468
Lehmann, J. S., Matthias, M. A., Vinetz, J. M., and Fouts, D. E. (2014). Leptospiral pathogenomics. Pathogens 3, 280–308. doi: 10.3390/pathogens3020280
Levett, P. N., Morey, R. E., Galloway, R. L., and Steigerwalt, A. G. (2006). Leptospira broomii sp. nov., isolated from humans with leptospirosis. Int. J. Syst. Evol. Microbiol. 56(Pt 3), 671–673. doi: 10.1099/ijs.0.63783-0
Levett, P. N., Morey, R. E., Galloway, R. L., Turner, D. E., Steigerwalt, A. G., and Mayer, L. W. (2005). Detection of pathogenic leptospires by real-time quantitative PCR. J. Med. Microbiol. 54, 45–49. doi: 10.1099/jmm.0.45860-0
Matthias, M. A., Ricaldi, J. N., Cespedes, M., Diaz, M. M., Galloway, R. L., Saito, M., et al. (2008). Human leptospirosis caused by a new, antigenically unique leptospira associated with a rattus species reservoir in the peruvian Amazon. PLoS Negl. Trop. Dis. 2:e213. doi: 10.1371/journal.pntd.0000213
Mérien, F., Amouriaux, P., Perolat, P., Baranton, G., and Saint Girons, I. (1992). Polymerase chain reaction for detection of Leptospira spp. in clinical samples. J. Clin. Microbiol. 30, 2219–2224.
Merien, F., Portnoi, D., Bourhy, P., Charavay, F., Berlioz-Arthaud, A., and Baranton, G. (2005). A rapid and quantitative method for the detection of Leptospira species in human leptospirosis. FEMS Microbiol. Lett. 249, 139–147. doi: 10.1016/j.femsle.2005.06.011
Murray, G. L., Srikram, A., Hoke, D. E., Wunder, E. A. Jr., Henry, R., Lo, M., et al. (2009). Major surface protein LipL32 is not required for either acute or chronic infection with Leptospira interrogans. Infect. Immun. 77, 952–958. doi: 10.1128/IAI.01370-08
Noguchi, H. (1917). Spirochaeta icterohaemorrhagiae in American wild rats and its relation to the Japanese and European strains: first paper. J. Exp. Med. 25, 755–763. doi: 10.1084/jem.25.5.755
Noguchi, H. (1918a). The survival of Leptospira (Spirochaeta) Icterohaemorrhagiae in nature; observations concerning microchemical reactions and intermediary hosts. J. Exp. Med. 27, 609–625. doi: 10.1084/jem.27.5.609
Noguchi, H. (1918b). Morphological characteristics and nomenclature of Leptospira (Spirochaeta) ictero-hemorrhagiae (Inada and Ido). J. Exp. Med. 27, 575–592. doi: 10.1084/jem.27.5.575
Peláez Sánchez, R. G., Quintero, J. A. L., Pereira, M. M., and Agudelo-Florez, P. (2017). High-resolution melting curve analysis of the 16s ribosomal gene to detect and identify pathogenic and saprophytic Leptospira Species in Colombian isolates. Am. J. Trop. Med. Hyg. 96, 1031–1038. doi: 10.4269/ajtmh.16-0312
Perez, J., and Goarant, C. (2010). Rapid Leptospira identification by direct sequencing of the diagnostic PCR products in New Caledonia. BMC Microbiol. 10:325. doi: 10.1186/1471-2180-10-325
Perolat, P., Chappel, R. J., Adler, B., Baranton, G., Bulach, D. M., Billinghurst, M. L., et al. (1998). Leptospira fainei sp. nov., isolated from pigs in Australia. Int. J. Syst. Bacteriol. 48, 851–858. doi: 10.1099/00207713-48-3-851
Picardeau, M. (2017). Virulence of the zoonotic agent of leptospirosis: still terra incognita? Nat. Rev. 15, 297–307. doi: 10.1038/nrmicro.2017.5
Puche, R., Ferrès, I., Caraballo, L., Rangel, Y., Picardeau, M., Takiff, H., et al. (2018). Leptospira venezuelensis sp. nov., a new member of the intermediates group isolated from rodents, cattle and humans. Int. J. Syst. Evol. Microbiol. 68, 513–517. doi: 10.1099/ijsem.0.002528
Rettinger, A., Krupka, I., Grunwald, K., Dyachenko, V., Fingerle, V., Konrad, R., et al. (2012). Leptospira spp. strain identification by MALDI TOF MS is an equivalent tool to 16S rRNA gene sequencing and multi locus sequence typing (MLST). BMC Microbiol. 12:185. doi: 10.1186/1471-2180-12-185
Ridzlan, F. R., Bahaman, A. R., Khairani-Bejo, S., and Mutalib, A. R. (2010). Detection of pathogenic Leptospira from selected environment in Kelantan and Terengganu, Malaysia. Trop. Biomed. 27, 632–638.
Saito, M., Miyahara, S., Villanueva, S. Y., Aramaki, N., Ikejiri, M., Kobayashi, Y., et al. (2014). PCR and culture identification of pathogenic Leptospira from coastal soil in Leyte, Philippines after a storm surge during Super Typhoon Haiyan (Yolanda). Appl. Environ. Microbiol. 80, 6926–6932. doi: 10.1128/AEM.02568-14
Saito, M., Villanueva, S. Y., Chakraborty, A., Miyahara, S., Segawa, T., Asoh, T., et al. (2013). Comparative analysis of Leptospira strains isolated from environmental soil and water in the Philippines and Japan. Appl. Environ. Microbiol. 79, 601–609. doi: 10.1128/AEM.02728-12
Schmid, G. P., Steere, A. C., Kornblatt, A. N., Kaufmann, A. F., Moss, C. W., Johnson, R. C., et al. (1986). Newly recognized Leptospira species (“Leptospira inadai” serovar lyme) isolated from human skin. J. Clin. Microbiol. 24, 484–486
Slack, A. T., Kalambaheti, T., Symonds, M. L., Dohnt, M. F., Galloway, R. L., Steigerwalt, A. G., et al. (2008). Leptospira wolffii sp. nov., isolated from a human with suspected leptospirosis in Thailand. Int. J. Syst. Evol. Microbiol. 58(Pt 10), 2305–2308. doi: 10.1099/ijs.0.64947-0
Slack, A. T., Khairani-Bejo, S., Symonds, M. L., Dohnt, M. F., Galloway, R. L., Steigerwalt, A. G., et al. (2009). Leptospira kmetyi sp. nov., isolated from an environmental source in Malaysia. Int. J. Syst. Evol. Microbiol. 59(Pt 4), 705–708. doi: 10.1099/ijs.0.002766-0
Smythe, L. D., Smith, I. L., Smith, G. A., Dohnt, M. F., Symonds, M. L., Barnett, L. J., et al. (2002). A quantitative PCR (TaqMan) assay for pathogenic Leptospira spp. BMC Infect. Dis. 2:13. doi: 10.1186/1471-2334-2-13
Stoddard, R. A., Gee, J. E., Wilkins, P. P., McCaustland, K., and Hoffmaster, A. R. (2009). Detection of pathogenic Leptospira spp. through TaqMan polymerase chain reaction targeting the LipL32 gene. Diagn. Microbiol. Infect. Dis. 64, 247–55. doi: 10.1016/j.diagmicrobio.2009.03.014
Thibeaux, R., Geroult, S., Benezech, C., Chabaud, S., Soupé-Gilbert, M. E., Girault, D., et al. (2017). Seeking the environmental source of Leptospirosis reveals durable bacterial viability in river soils. PLoS Negl. Trop. Dis. 11:e0005414. doi: 10.1371/journal.pntd.0005414
Thibeaux, R., Iraola, G., Ferrés, I., Bierque, E., Girault, D., Soupé-Gilbert, M. E., et al. (2018). Deciphering the unexplored Leptospira diversity from soils uncovers genomic evolution to virulence. Microb. Genomics 4:000144. doi: 10.1099/mgen.0.000144
Tsuboi, M., Koizumi, N., Hayakawa, K., Kanagawa, S., Ohmagari, N., and Kato, Y. (2017). Imported Leptospira licerasiae infection in traveler returning to Japan from Brazil. Emerg. Infect. Dis. 23, 548–549. doi: 10.3201/eid2303.161262
Viau, E. J., and Boehm, A. B. (2011). Quantitative PCR-based detection of pathogenic Leptospira in Hawai'ian coastal streams. J. Water Health 9, 637–646. doi: 10.2166/wh.2011.064
Keywords: soil microbiology, MALDI-TOF MS, WGS comparisons, novel species, isolation
Citation: Thibeaux R, Girault D, Bierque E, Soupé-Gilbert M-E, Rettinger A, Douyère A, Meyer M, Iraola G, Picardeau M and Goarant C (2018) Biodiversity of Environmental Leptospira: Improving Identification and Revisiting the Diagnosis. Front. Microbiol. 9:816. doi: 10.3389/fmicb.2018.00816
Received: 06 February 2018; Accepted: 10 April 2018;
Published: 01 May 2018.
Edited by:
Paolina Garbeva, Netherlands Institute of Ecology (NIOO-KNAW), NetherlandsReviewed by:
Jason Sahl, Northern Arizona University, United StatesUlisses Padua Pereira, Universidade Estadual de Londrina, Brazil
Copyright © 2018 Thibeaux, Girault, Bierque, Soupé-Gilbert, Rettinger, Douyère, Meyer, Iraola, Picardeau and Goarant. This is an open-access article distributed under the terms of the Creative Commons Attribution License (CC BY). The use, distribution or reproduction in other forums is permitted, provided the original author(s) and the copyright owner are credited and that the original publication in this journal is cited, in accordance with accepted academic practice. No use, distribution or reproduction is permitted which does not comply with these terms.
*Correspondence: Cyrille Goarant, Y2dvYXJhbnRAcGFzdGV1ci5uYw==
†These authors have contributed equally to this work.
‡Present Address: Anna Rettinger, Bavarian Health and Food Safety Authority, Erlangen, Germany