- 1Department of Public Health, University of Copenhagen, Copenhagen, Denmark
- 2Department of Science and Environment, Roskilde University, Roskilde, Denmark
The global malaria burden, including falciparum malaria, has been reduced by 50% since 2000, though less so in Sub-Saharan Africa. Regional malaria elimination campaigns beginning in the 1940s, up-scaled in the 1950s, succeeded in the 1970s in eliminating malaria from Europe, North America, the Caribbean (except Haiti), and parts of Asia and South- and Central America. Dengue has grown dramatically throughout the pantropical regions since the 1950s, first in Southeast Asia in the form of large-scale epidemics including severe dengue, though mostly sparing Sub-Saharan Africa. Globally, the WHO estimates 50 million dengue infections every year, while others estimate almost 400 million infections, including 100 million clinical cases. Curiously, despite wide geographic overlap between malaria and dengue-endemic areas, published reports of co-infections have been scarce until recently. Superimposed acute dengue infection might be expected to result in more severe combined disease because both pathogens can induce shock and hemorrhage. However, a recent review found no reports on more severe morbidity or higher mortality associated with co-infections. Cases of severe dual infections have almost exclusively been reported from South America, and predominantly in persons infected by Plasmodium vivax. We hypothesize that malaria infection may partially protect against dengue – in particular falciparum malaria against severe dengue – and that this inter-species cross-protection may explain the near absence of severe dengue from the Sub-Saharan region and parts of South Asia until recently. We speculate that malaria infection elicits cross-reactive antibodies or other immune responses that infer cross-protection, or at least partial cross-protection, against symptomatic and severe dengue. Plasmodia have been shown to give rise to polyclonal B-cell activation and to heterophilic antibodies, while some anti-dengue IgM tests have high degree of cross-reactivity with sera from malaria patients. In the following, the historical evolution of falciparum malaria and dengue is briefly reviewed, and we explore early evidence of subclinical dengue in high-transmission malaria areas as well as conflicting reports on severity of co-morbidity. We also discuss examples of other interspecies interactions.
Present and Past Prevalence of Malaria, Dengue, and Population-Level Protection Against Each Disease
Origin, Spread, and Burden of Falciparum Malaria
Plasmodium falciparum malaria parasites probably emerged as a human pathogen from its enzootic origin in West Africa about 5,000–10,000 years ago (Carter and Mendis, 2002). This coincides with the point in time when humans began living in large agricultural communities, and when African Anopheles mosquitoes adapted to the novel agrarian environment breeding in man-made water collections and feeding almost exclusively on humans. The parasite accompanied man to Asia and Melanesia around 4,000 years ago and to the Americas with the slave trade 500 years ago (Curtin, 1993).
According to Carter and Mendis (2002), malaria reached its apex during the 19th century, when more than half of the global population was at risk of infection, and case fatality rates reached above 10%. Declines in malaria incidence were noted by the turn of the 20th century. The halving of the global malaria mortality burden since 2000 has been attributed to a combination of malaria interventions such as the use of insecticide-treated nets (Beiersmann et al., 2011; Kramer et al., 2017), indoor residual spraying, and artemisinin combination therapy (Streatfield et al., 2014), as well as the effect of development, such as increasing urbanization (Tatem et al., 2013) and poverty reduction (Teklehaimanot and Mejia, 2008). Still, each year more than 200 million cases and almost 0.5 million deaths occur due to malaria; these deaths are mainly in children in Sub-Saharan Africa and largely from Plasmodium falciparum transmitted by nocturnal Anopheles species (Streatfield et al., 2014). Transmission in urban areas is generally of lower intensity than in rural areas but can be surprisingly elevated in some urban environments (Wilson et al., 2015).
No effective vaccine exists for malaria, and natural immunity takes years of repeated exposure to develop. Residents of malaria-endemic areas eventually develop some immunity to the infection (Tomson, 1933), but immunity wanes after a single year without exposure (Hoffman, 1986). The difficulties in developing long lasting immunity, including the mechanism by which partial protection is finally achieved, remain largely unexplained. Two main hypotheses, not mutually exclusive, have been put forward (Greenwood and Targett, 2011). The first suggests that adaptive immunity is especially challenged in the case of P. falciparum malaria, as the key parasite protein family, PfEMP1, has more than 60 variants (Gardner et al., 2002). The second postulates that repeated exposure to malaria antigens is needed to drive an effective immune response, perhaps accompanied by a maturation of the immune system with increasing age. Studying inflammatory cytokine responses in individuals with malaria from areas of different transmission intensities have shown that levels of pro-inflammatory cytokines decreased with increasing transmission intensity (Ademolue et al., 2017).
Origin, Spread, and Burden of Dengue
There is no consensus regarding the enzootic origin of dengue virus (DENV); some suggest an African origin, others an Asian, given the presence of sylvatic (“jungle”) viruses in both regions (Rudnick et al., 1967; Gubler, 1987; Halstead, 1990). Irrespective, the virus probably evolved into four distinct serotypes about 1,000 years ago within sylvatic cycles involving mosquitoes and primates. Phylogenetic evidence suggests that each of the four serotypes entered a cycle of stable transmission between humans and mosquito vectors only 125–320 years ago, coinciding with the out-of-Africa migration of the anthropophilic vector Aedes aegypti (Crawford et al., 2017) and historical accounts of local outbreaks and general pandemics of dengue-like disease from the 17th seventeenth century and onwards (Gubler, 1998; Twiddy et al., 2003).
The global pattern of spread of the four dengue serotypes since the early 1940s – when DENV was first isolated – has been highlighted by Messina et al. (2014). These show consistent dispersal of each serotype from South Asia over Southeast Asia to the Pacific and the Americas, with relatively few reported occurrences in sub-Saharan Africa, tribal areas of Papua New-Guinea, North-East India and inner parts of South America. This recently intensified transmission and spread of the DENVs is largely attributed to massive urbanization and globalized travel and trade that characterize the latter half of the 20th century. Importantly, the urban ecology in the tropics has enabled the proliferation of the “domestic” vector Ae. aegypti, while travel and trade have helped widen the global range of both vector and virus (Gubler, 1998; Li et al., 2014).
Today, an estimated 3.9 billion people live at risk of infection in at least 128 countries (Brady et al., 2012). Dengue presents a continuously expanding global disease burden, with a suggested doubling or more in the number of symptomatic infections, every 10 years – between 1990 and 2013 (Stanaway et al., 2016). There is, however, wide variation and substantial uncertainty in estimates of the dengue disease burden (Shepard et al., 2014) given both under-recognition and under-reporting of dengue, in general. Notably, only three WHO regions provide regular reports of annual case numbers (WHO, 2017a). In 2015, WHO recoded 3.2 million illness episodes (WHO, 2017a), yet the true figure is probably far higher, with current estimates based on comprehensive risk models ranging between 96 million (Bhatt et al., 2013) in 2010 and 58.4 million in 2013 (Stanaway et al., 2016).
Major challenges for understanding and controlling dengue through the use of preventive or therapeutic interventions include lack of a laboratory correlate and animal models to investigate protective immunity; continued DENV evolution due to major clade replacements and genetic shifts (Holmes and Twiddy, 2003; Rico-Hesse, 2003; Endy, 2014); and not least the complex viral-host pathogenesis that ranges from mild unspecific manifestations to severe and potentially fatal disease (Endy, 2014). A tetravalent vaccine (Capeding et al., 2014; Hadinegoro et al., 2015; Villar et al., 2015) has been licensed for use in several endemic countries in South America and Asia. However, recent data indicate increased risk of severe dengue among vaccinees without previous exposure to DENV (WHO, 2017b) suggesting that – in spite of its tetravalent properties – the vaccine may induce severe manifestations on secondary exposure, through mechanisms of immuno-enhancement (Deen, 2016). Current recommendations by the WHO/GACVS are that individuals who have not been previously infected with wild DENV should not be administered the vaccine (WHO, 2016, 2017b). In addition, the long term effectiveness of this vaccine remains uncertain (López-Gatell et al., 2016). As such, vector control remains the principal dengue prevention option. The primary vectors of DENV, Ae. aegypti and Aedes albopictus, are both diurnal, rendering insecticide-treated nets of less use for dengue than for malaria control. Public health efforts currently focus on environmental management for elimination of vector breeding sites and on various insecticide applications targeting either larvae or adult stages in the peri-domestic environment (Chu et al., 2013).
The Emergence of Severe Dengue as an Epidemic Entity
The global emergence of severe DENV infection in the latter half of the 20th century is closely aligned with the intensified transmission and spread of the four serotypes, and the occurrence of sequential transmission or actual co-circulation of two or more serotypes (hyperendemicity) in a given population. Dengue epidemics involving severe disease with mortality rates as high as 10% were first noted in Manila and Bangkok in 1954 (Hammon et al., 1960; Hammon and Sather, 1964), but were soon followed by similar epidemics in several other Southeast Asian cities (Rudnick, 1967; Chambers et al., 1990; Endy, 2014). Urban Southeast Asia remained the focus of intense epidemics of severe dengue throughout the 1960s and 1970s, and by the 1970s severe dengue was recorded as a leading cause of pediatric hospitalization and death in many Southeast Asian countries (WHO, 1997).
Whereas the Pacific islands experienced their first outbreaks with severe dengue in the 1970s, the phenomenon was not reported in the Americas before 1981, when Cuba reported a large-scale epidemic with tens of thousands of severe dengue cases (Guzman and Kouri, 2003). The subsequent pattern of intensified transmission with frequent large-scale epidemics in the urban centers of the Pacific and Americas has largely mimicked that of Southeast Asia, albeit two to three decades later. Thus, in the Americas, dengue has doubled every decade, with increasing severity and mortality particularly in children and young adults (San Martín et al., 2010). Interestingly, Halstead et al. (2001) noted the absence of severe dengue despite hyperendemic DENV transmission in Haiti.
For Sub-Saharan Africa, evidence of dengue disease – not least that of severe disease – has been relatively sparse, even though the disease is likely to have been present for centuries (Amarasinghe et al., 2011). Notably, in 1970, Geser et al. (1970) reported the presence of Ae. aegypti in the coastal area of Kenya. In addition, 47% of the population presented with hemagglutinin inhibiting (HI) dengue antibodies, although no outbreaks of dengue had been reported. In 1977, Fagbami et al. found that 45% of tested adults in Nigeria were seropositive for anti-DENV-2 antibodies, as evidenced by neutralizing antibodies (Fagbami et al., 1977). In several African countries DENV transmission has been recognized primarily through diagnosis of travelers or stationed military personnel or through sero-prevalence population studies (de Araújo Lobo et al., 2016; Onoja et al., 2016). It is mainly on the basis of such evidence that dengue is considered endemic to both East and West Africa (Brady et al., 2012).
Recent reviews have cataloged multiple small-scale outbreaks of the 1980s and 1990s, across Sub-Saharan Africa (Messina et al., 2014; Baba et al., 2016). Interestingly, the frequency and scale of outbreaks appear to have increased – as have the numbers of deaths – since the turn of this century (Massangaie et al., 2016). Most notable are: the 2009 outbreak in Cape Verde, with 21,304 reported cases and four deaths; the 2010 outbreak in Sudan involving 3,765 cases and 12 deaths; and the 2011 outbreak in Kenya with 5,000 reported cases and four deaths (Baba et al., 2016). Tanzania has experienced no less than four recurrent outbreaks in 2010, 2012, 2013, and 2014, the latter of which included more than 3,000 reported cases and four deaths (Mboera et al., 2016) (Figure 1D).
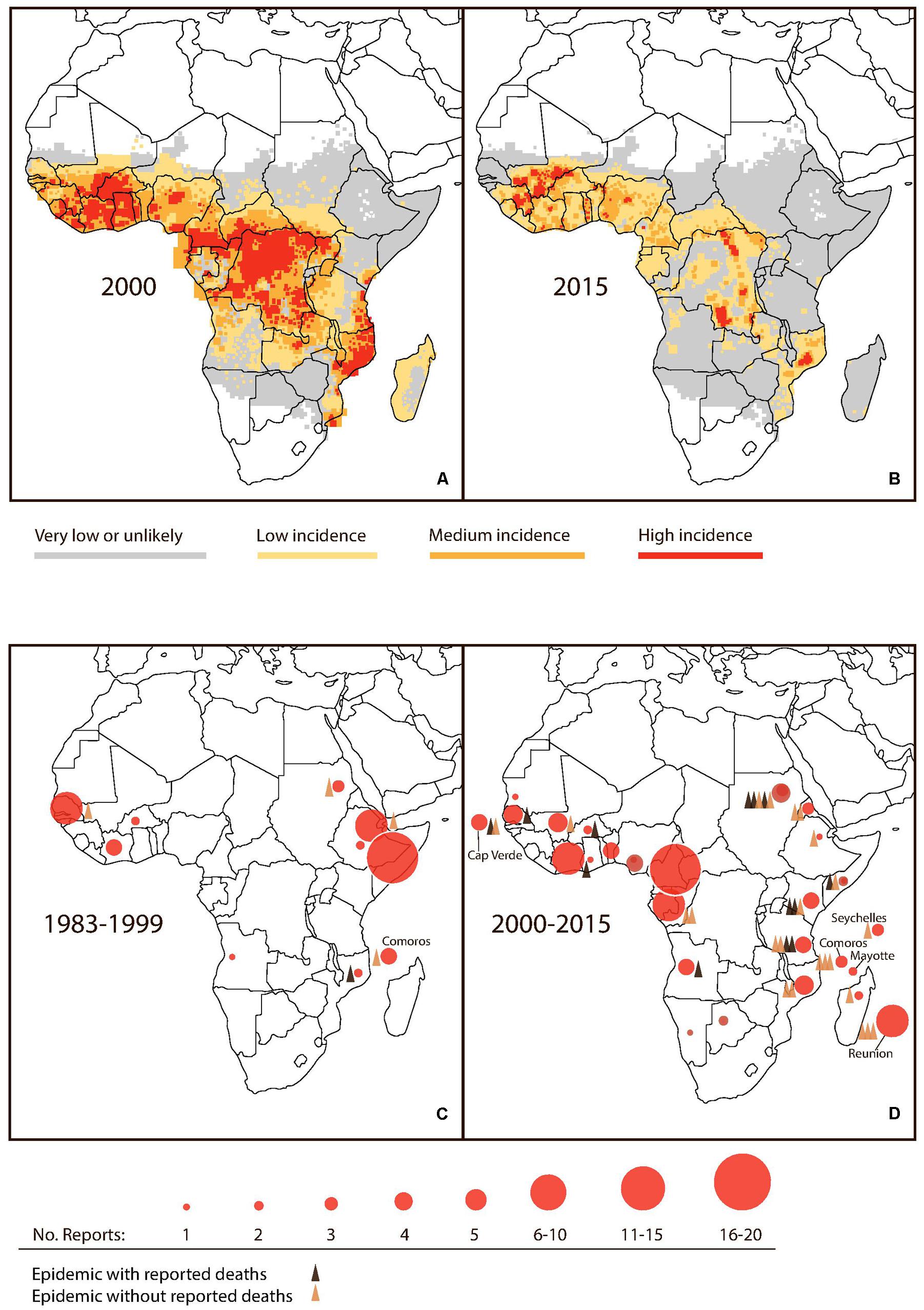
FIGURE 1. Noted changes in malaria and dengue activity in Sub-Saharan Africa. (A,B) Estimated Plasmodium falciparum incidence (cases per 100,000) in 2000 and 2015. Modified from original MAP (Source: MAP, 2018). (C,D) Accumulated reports of dengue activity and reported dengue epidemics for the periods 1983–1999 and 2000–2015. (Source: Messina et al., 2014; Gideon, 2018).
It is generally argued that dengue and severe dengue have been subject to under-diagnosis in Africa given a lack of index of suspicion and little access to laboratory diagnostics (Amarasinghe et al., 2011). However, awareness is typically high in this region regarding hemorrhagic fevers and shock syndromes due to the recurrent outbreaks of yellow fever, Lassa, Marburg, Ebola and Crimean-Congo hemorrhagic fevers (Parkes-Ratanshi et al., 2014). As such, one would expect that outbreaks involving severe dengue would be subject to substantial levels of scrutiny. In spite of the potential under-diagnosis of dengue, the apparent escalation in dengue epidemic activity in Sub-Saharan Africa (Stanaway et al., 2016) might suggest that the region is on the verge of experiencing the emergence of severe dengue as an epidemic entity, similar to the experience of South and Southeast Asia in the 1950s, the Pacific in the 1970s, and the Americas in the 1980s.
Risk Factors for Severe Dengue
Several factors have been shown to modulate the clinical response to DENV infection. Notable risk factors for severe manifestations include previous heterotypic DENV infection; viral virulence, co-morbidities such as diabetes and hypertension; as well as host genetic factors (Carabali et al., 2015; Halstead, 2015).
Immuno Pathogenesis
The groups of (Halstead, 1980, 1988, 2012), Rothman and Ennis (1999), and Rothman et al. (2014) have both been crucial in detailing different aspects of the immuno-pathological mechanisms of severe disease that arise in response to sequential heterotypic DENV infections. These mechanisms implicate the development of heterotypic, cross-reactive but non-neutralizing antibodies and T cells during primary infection and their subsequent activation upon secondary infections with a heterotypic serotype (Endy, 2014). Cross reactive IgG DENV-antibodies have been shown (in vitro) to enhance viral uptake and proliferation in target cells, a mechanism known as antibody dependent enhancement (ADE) of infection with a second dengue serotype. Notably, recent studies suggest that IgG DENV-antibodies may also be linked to thrombocytopenia as observed in severe dengue (Wang et al., 2017). In addition, cross reactive T-cells may unleash a cascade of cytokines and chemical mediators linked to various severity outcomes including endothelial permeability (Pang et al., 2007). New insights in the immunopathology of dengue were recently reviewed by Screaton et al. (2015).
A key point is that the risk of experiencing immune-mediated pathogenesis increases if a population is subject to consecutive infections by heterotypic serotypes. The emergence of severe dengue throughout large parts of the world is thus aligned with the increased global spread of each serotype. Co-circulation of DENV types has been increasingly documented, even in malarious areas of Sub-Saharan Africa (Messina et al., 2014). Although the true extent of DENV co-circulation in Sub-Saharan Africa remains unclear, it is notable that recent outbreaks, as detailed above, have occurred predominantly in areas with reported co-circulation of DENVs (Saluzzo et al., 1986; Caron et al., 2013; Ridde et al., 2016).
Virulence
Rapid molecular evolution and genetic diversity is a common feature among RNA viruses and may explain why certain strains and genotypes of the four DENVs display greater epidemic potential and pathogenicity than others. Gubler and Trent (1994) were among the first to argue that increased circulation of DENVs as observed in hyperendemic areas, increases the likelihood of virulent strains, as viral competition intensifies the evolutionary pressure on each serotype.
Co-morbidity With Other Than Malaria
A recent review of comorbidity with dengue identified – in addition to old age – several non-communicable diseases of importance for the development of severe manifestations including: cardiovascular disease, diabetes, respiratory disease, and renal disease (Toledo et al., 2016). However, due to heterogeneity among studies, the real estimate effect of comorbidities as modifiers of dengue severity could not be established. Bacterial co-infection with dengue is also increasingly described (Trunfio et al., 2017).
Host Genetic Factors
It has been reported that people of African descent are less prone to suffer severe dengue than other ethnic groups, such as Hispanics and Caucasians in Cuba (Guzman and Kouri, 2003), Haiti (Halstead et al., 2001), Brazil (Blanton et al., 2008), and Colombia (Palacios et al., 2016). This observation has offered a plausible explanation for the absence of severe disease from Sub-Saharan Africa – at least up until the recent outbreaks, where severe dengue apparently has increased also among native Africans, as indicated in the following. Furthermore, the study by Moraes et al. (2013) did not confirm that Afro-Americans have lower risks of severe dengue; on the contrary the risk was higher, except when corrected for socio-economic risk factors. HLA and other gene associations have also been linked with dengue disease severity (Stephens, 2010).
Evidence that Elimination of Falciparum Malaria Coincides with the Emergence of Severe Dengue
In a review of the malaria elimination history, Tanner and de Savigny (2008) noticed how regional malaria elimination campaigns conducted in the late 1940s paved the way for the Global Malaria Eradication Program in 1955. As part of this campaign malaria was eliminated from Europe, North America, the Caribbean (except Haiti) (Agarwal et al., 2012) and parts of Asia and South and Central America during the 1960s and by the early 1970s (Bruce-Chwatt, 1986).
After a temporary setback, in part due to the development of insecticide- and drug-resistance, reduction of malaria by 50–75% was eventually obtained in most of Asia and South and Central America (WHO, 2013). However, no major success was obtained in Sub-Saharan Africa until recent decades. As indicated by Figures 1A,B, malaria is now on the retreat in several parts of sub-Saharan Africa. Since 2000, a historically unprecedented decline has been observed (Snow et al., 2017, the Malaria Atlas Project - MAP). For example, malaria has virtually disappeared even in children in Dar es Salaam, Tanzania (Strøm et al., 2013). At the same time, major outbreaks of dengue have been reported in Dar es Salaam, including the 2014 DENV2 outbreak registering a case fatality rate of 4% (Vairo et al., 2016). Dengue epidemics have also been reported from other parts of Tanzania (Mboera et al., 2016), neighboring Kenya (Brady et al., 2012), Mozambique (Massangaie et al., 2016), as well as Angola (Sharp et al., 2015), Sierra Leone (de Araújo Lobo et al., 2016), Burkina Faso (Ridde et al., 2016), and Nigeria (Onoja et al., 2016) and several other parts of Sub-Saharan Africa (L’Azou et al., 2015). Notably, in 2017, dengue outbreaks were reported from eight Sub-Saharan countries reaching an excess of 21,000 total cases. Burkina Faso recorded more than half of these cases (13,135) including 28 deaths (Gideon, 2018). Details for accumulated reports of dengue including epidemic activity in Sub-Saharan Africa from 1983 to 2015 are provided in Figures 1B,C.
Co-Morbidity of Dengue and Malaria
So far, we have discussed concurrent phenomena of dengue emergence and malaria decline at population-level. Another way to consider the hypothesis of mutual exclusion or interdependence of the two pathogens comes from evidence of co-infections at the patient-level. Clinical diagnosis and distinction of malaria and dengue is difficult due to overlapping symptoms. Either disease may induce similar general symptoms as well as severe manifestations in terms of thrombocytopenia, central nervous symptoms, “cytokine-storm” (Clark, 2007), shock and even bleeding. Manifestations of concurrent infection in a patient would be expected to have severe consequences (Hati et al., 2012). However, as noticed by Mohapatra et al. (2012) reports on co-infection are relatively scarce in literature and those of severe manifestations even more so.
The first patient with falciparum malaria and dengue co-infection was reported by Charrel et al. (2005). Our own search from 2005 to 2017 revealed 55 reports of co-infections, including more than 360 cases of acute co-infections as confirmed by malaria RDT and/or microcopy and DENV virology, antigen tests and/or seroconversion (for details please refer to Supplementary Material). The malaria-immune status was not reported for most co-infections neither were parasitaemia levels which could otherwise be used as a proxy for immunity (with high parasitaemia indicating low immunity). Instead, we suggest that malaria incidence in the countries from where co-infections were reported may be used to assume the general level of malaria immunity. With the exception of Sub-Saharan Africa, most areas reported low or medium transmission of P. falciparum malaria during the period of interest.
Table 1 includes only cases of P. falciparum in acute dengue confirmed by DENV RT-PCR, NS1 test, or seroconversion. Of 74 identified co-infections, severe symptoms were only recorded in three cases of which one was acquired in West Africa as reported by Charrel et al. (2005). This case involved a traveler, assumed to be non-malaria immune, who after returning to France developed disseminated intravascular coagulation, which may be a manifestation of either disease (ibid). Alam and Dm (2013) reported a single case of cerebral malaria and dengue co-infection in a military personnel located in Patna, India. Again, the level of parasitaemia was not reported; however, cerebral malaria would indicate low or absence of malaria immunity, in line with the low incidence level reported for the area. Finally, Yong et al. (2012) reported severe manifestations in a local Indonesian adult male with 4% falciparum parasitaemia and dengue co-infection. This case was complicated by rhabdomyolysis and acute renal insufficiency, which may both be induced by malaria and dengue.
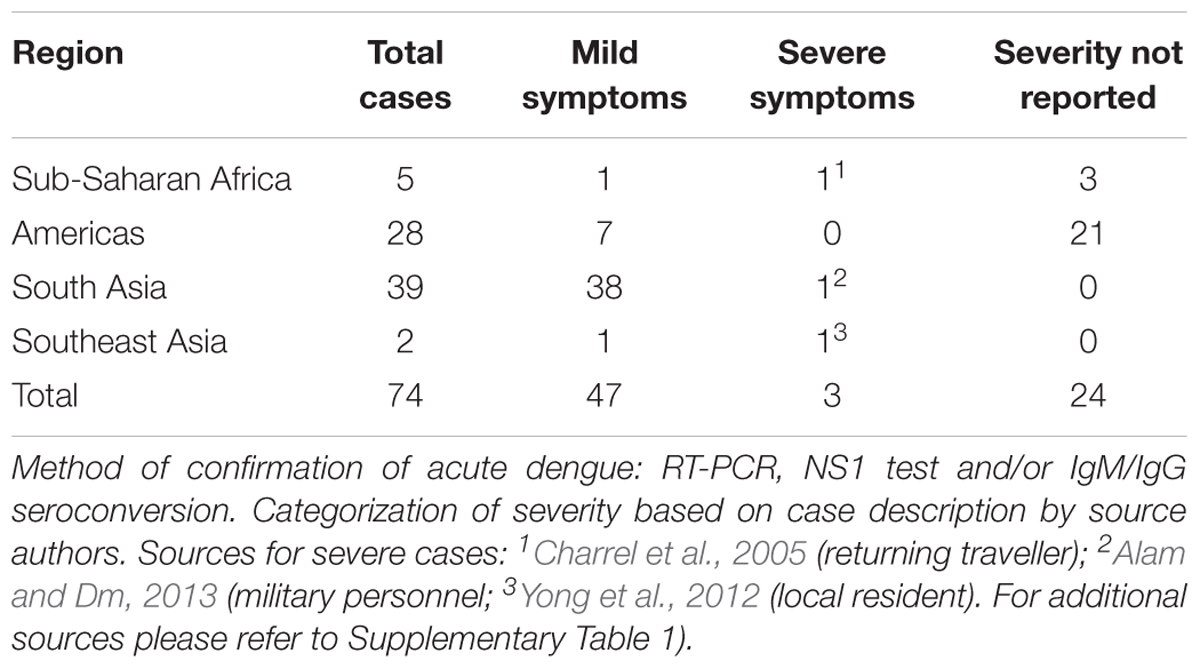
TABLE 1. Reported cases of acute dengue virus and P. falciparum co-infections by global region, 2005–2017.
Lack of reporting of dual infection in the field could result from testing positive for either malaria or dengue infection with no further testing being done, given low index of suspicion for co-infections (i.e., case considered diagnosed after one positive test). Yet, some argue that severity could also prompt testing for dual disease, and thereby potentially bias reporting. Notably, studies that have actively investigated the clinical picture of mono-infections as compared to that of dengue and malaria co-infections disagree with respect to observed differences in severity levels. Epelboin et al. (2012) reported that among local resident in French Guyana, the clinical picture of dual infections was more severe than in either dengue or malaria alone. It is notable that P. vivax was identified in the majority of cases (∼80%) and that only 50% of the dual infected reported previous malaria. Unfortunately, the report did not stratify between P. vivax or P. falciparum co-infections, nor did it report on parasitaemia levels.
In contrast, Halsey et al. (2016) found similar severity levels between mono and co-infected individuals among local populations in Peru. As for Epelboin et al. (2012) the majority of cases were reported as P. vivax. In India, Mohapatra et al. (2012) reported generally benign manifestations among co-infections as compared with malaria mono-infections. In this study the majority of cases (∼90%) were reported as P. falciparum infections. Notably, lower parasitic counts were observed among co-infected than for mono-infected patient, although reported as non-significant. In line with these findings, Ahmad reported that the presentation and severity of co-infections appeared “to be milder and outcomes comparable (if not better) to mono-infections” during a dengue outbreak in Uttarakhand, India. While co-infections occurred with both P. falciparum and P. vivax these were not clearly distinguished in the report.
Stoler et al. (2015) reported dengue-specific IgG antibodies in 22% of urban Ghanaian children with P. falciparum parasitaemia, suggesting previous exposure, and dengue IgM antibodies in 3%, indicating probable acute co-infection. While co-infection was indicated, co-morbidity or increased severity was not. None of the children appeared to have severe disease; but IgM dengue positive children had (non-significantly) higher levels of P. falciparum parasitaemia, which could indicate they were only partially immune to falciparum malaria at the time of DENV infection. Unfortunately, levels of malaria antibodies were not reported. Specificity of the anti-dengue Ig tests used was 97% for multisite pooled sera, though cross-reactivity with other flavivira was high (30% and 60% for IgM and IgG, respectively).
Cross-Reactivity and “False-Positivity” in Tests for Dengue and Malaria
Malaria infection gives rise to polyclonal B-cell activation (Greenwood, 1974) and to heterophilic antibodies (Houba et al., 1974), which may react to host antigens as well as to other pathogens (Ribeiro, 1988). Some anti-dengue IgM tests have shown a high degree of cross-reactivity (10–70%) with sera from malaria patients, and with other flavivira (Hunsperger et al., 2009; WHO, 2009). Hunsperger et al. (2009), when evaluating four anti-DENV IgM kits and five micro-plate ELISAs found between 5 and 70% false-positive results for patients with malaria or past dengue infections. Knowing the true population prevalence of dengue as well as malaria infections is hampered by the lack of representative population-based studies, and also by false-positive test results or cross-reactivity that are a problem in many serological assays including rapid diagnostic tests (RDTs) for dengue and malaria. In other words, a positive DENV IgM test may signify current or recent primary infection, secondary or tertiary infection (often lower titers, though primary infection responses also vary), or cross-reactivity with other virus or even malaria (current or past infection). Thus, with current laboratory assays available in countries with limited resources, it is very difficult to tease apart the possibility of malaria and dengue together in an area or a patient, wherefore low-cost antigen tests and viral and plasmodia gene detection kits are needed.
Possible Co-protection of Malaria Infection Against Severe Dengue?
Repeated exposure to falciparum malaria is followed by development of protective antibodies, decline of pro-inflammatory host-responses (Ademolue et al., 2017) and decreasing severity (Reyburn et al., 2005), whereas sequential exposure to heterogeneous DENVs – and related viruses, e.g., Zika virus – may lead to enhanced host immune responses (Cohen, 2017). However, on the basis of the presented clinical, epidemiological, immunological and laboratory-based reports, we speculate whether cross-reactivity observed between tests for dengue and malaria pathogens could also be an indicator of some level of cross-protection between two potentially severe diseases. In other words, could dengue-induced antibody-dependent enhancement of infection (ADE) (de Alwis et al., 2014) be “de-hanced” by malaria induced antibodies? Or could alleviated cytokine responses among clinically malaria immune individuals (Ademolue et al., 2017) similarly reduce “over”-reaction to dengue (re-)infection?
Proof of concept of cross-protection from malaria to dengue in man would require exposing malaria immune humans to dengue in controlled experiments. As reviewed by Sabin (1952), research on dengue during World War II did include research on human volunteers exposed to DENV and the development of immunity to the homologues strain. Unfortunately, the level of immunity to malaria was not reported in these volunteers. A human model of dengue exposure is currently being re-introduced (Larsen et al., 2015; Royer, 2015).
Examples of Interspecies Interactions
In the literature, there are certainly important examples of interaction between infections of unrelated pathogens leading to more severe outcomes, such as HIV infection leading to latent tuberculosis reactivation (Pitchenik et al., 1983), and severe outcomes of pneumococcal disease following influenza infection, the so-called lethal synergy (McCullers, 2014). And most recently, Zika virus infection has been found to be enhanced in recent dengue cases, much like ADE among the four DENV types (Kawiecki and Christofferson, 2016). But examples in which co- or sequential infection may decrease severity of either or both diseases have been less investigated. An interesting, historical example is the now obsolete treatment of syphilis with malaria (Moore, 1930), and the possible sharp reduction in tuberculosis in the US population immediately following the 1918 severe pandemic influenza outbreak (Noymer, 2011). More recently, it has been shown that P. falciparum infection may protect partly against severe Ebola outcomes (Rosenke et al., 2016).
Conclusion
In summary, we conclude that at the population level the evidence of interaction between dengue and malaria is perhaps already there: when comparing time trends in the two diseases at continent level, a suggestive pattern emerges. We have discussed the striking coincidence of the emergence of dengue in recent years (Figure 1D), with the reduction in incidence of malaria (Figure 1B), suggesting that declining malaria exposure due to successful control programs or other environmental factors may have inadvertently led to the cessation of protection against clinically apparent and severe dengue infections, especially in Sub-Saharan Africa. However, individual-level studies would be the best way to determine if co-protection exists between falciparum malaria and dengue. Available individual-level studies reviewed here are only suggestive and interpretation hampered by non-specificity of used tests. Also, they present a risk of bias in favor of our hypothesis, in that more severe patients are more likely to be tested for several pathogens and subsequently reported, and vice versa that lack of reporting of dual infection in the field could result from testing positive for either malaria or dengue infection and then no further testing being done.
Evidently, more research is required to investigate whether there is a causal link between declining falciparum malaria and increased dengue in recent years. We suggest in vitro experiments such as exposing in vitro cultures of DENV to malaria antibodies, and vice versa as a proxy to evidence (or not) of cross-protection. Other modern in vitro technologies including epigenetic silencing or activation of regulatory genes implicated in immunity and susceptibility of disease (Kirchner et al., 2016) or development of animal models may also help, while acknowledging that suitable animal models are still not available for either infection. It would be interesting – if considered ethically acceptable – to include malaria-immune individuals in the ongoing dengue human exposure model. Further studies of the potential role of unspecific sides of acquired immunity (Muraille, 2016), and studies of cross-kingdom interaction (Luckhart et al., 2015) could be rewarding as well. Especially, if combined with integrated epidemiology and surveillance for vector-borne diseases and by integrating layers of data from both malaria and dengue (Wardrop, 2016). These studies could be complemented by large-scale prospective studies e.g. through the INDEPTH-network of health and demographic surveillance systems in low- and middle-income countries (INDEPTH, 2018).
Finally, we believe it is important to broaden considerations across pathogens and diseases in order to explain the striking associations in temporal patterns of malaria and dengue globally observed since the 1950s and in Africa, specifically, after the Millennium. More broadly, we suggest that considering non-specific effects of inter-species interaction and interdependences may be a critical aspect of recognizing possible outcomes beyond those of the target pathogen and to understand the broader consequences of global health intervention programs designed to eliminate a given disease.
Author Contributions
IB conceived the hypothesis of potential interaction between malaria and dengue, and drafted the first version of the manuscript, and wrote the subsequent versions in close collaboration with KS and LS. KS contributed in particular to the sections of dengue and malaria epidemiology mapping, and to Table 1 and to the Supplementary Material, and contributed to all sections and versions of the manuscript. LS contributed in particular to the sections on historic epidemiology and interspecies interaction and to other sections and versions of the manuscript. All authors read and approved the submitted version.
Conflict of Interest Statement
The authors declare that the research was conducted in the absence of any commercial or financial relationships that could be construed as a potential conflict of interest.
Acknowledgments
We wish to thank Mr. Casper Nielsen, COBL University of Copenhagen, and Ms. Ditte Frisk Hansen for graphic support.
Supplementary Material
The Supplementary Material for this article can be found online at: https://www.frontiersin.org/articles/10.3389/fmicb.2018.01120/full#supplementary-material
References
Ademolue, T. W., Aniweh, Y., Kusi, K. A., and Awandare, G. A. (2017). Patterns of inflammatory responses and parasite tolerance vary with malaria transmission intensity. Malar. J. 16:145. doi: 10.1186/s12936-017-1796-x
Agarwal, A., McMorrow, M., Arguin, P. M. (2012). The increase of imported malaria acquired in Haiti among US travelers in 2010. Am. J. Trop. Med. Hyg. 86, 9–10. doi: 10.4269/ajtmh.2012.11-0394
Alam, A., and Dm, M. (2013). A case of cerebral malaria and dengue concurrent infection. Asian Pac. J. Trop. Biomed. 3, 416–417. doi: 10.1016/S2221-1691(13)60087-8
Amarasinghe, A., Kuritsk, J. N., Letson, G. W., and Margolis, H. S. (2011). Dengue virus infection in Africa. Emerg. Infect. Dis. 17, 1349–1354. doi: 10.3201/eid1708.101515
Baba, M., Villinger, J., and Masiga, D. K. (2016). Repetitive dengue outbreaks in East Africa: a proposed phased mitigation approach may reduce its impact. Rev. Med. Virol. 26, 183–196. doi: 10.1002/rmv.1877
Beiersmann, C., Bountogo, M., Tiendrébeogo, J., De Allegri, M., Louis, V. R., Coulibaly, B., et al. (2011). Falciparum malaria in young children of rural Burkina Faso: comparison of survey data in 1999 with 2009. Malar. J. 10:296. doi: 10.1186/1475-2875-10-296
Bhatt, S., Gething, P. W., Brady, O. J., Messina, J. P., Farlow, A. W., et al. (2013). The global distribution and burden of dengue. Nature 496, 504–507. doi: 10.1038/nature12060
Blanton, R. E., Silva, L. K., Morato, V. G., Parrado, A. R., Dias, J. P., Melo, P. R., et al. (2008). Genetic ancestry and income are associated with dengue hemorrhagic fever in a highly admixed population. Eur. J. Hum. Genet. 16, 762–765. doi: 10.1038/ejhg.2008.4
Brady, O. J., Gething, P. W., Bhatt, S., Messina, J. P., Brownstein, J. S., and Hoen, A. G. et al. (2012). Refining the global spatial limits of dengue virus transmission by evidence-based consensus. PLoS Negl. Trop. Dis. 12:e1760. doi: 10.1371/journal.pntd.0001760
Capeding, M. R., Tran, N. H., Hadinegoro, S. R., Ismail, H. I., Chotpitayasunondh, T., and Chua, M. N., et al. (2014). Clinical efficacy and safety of a novel tetravalent dengue vaccine in healthy children in Asia: a phase 3, randomized, observer-masked, placebo-controlled trial. Lancet 384, 1358–1365. doi: 10.1016/S0140-6736(14)61060-6
Carabali, M., Hernandez, L. M., Arauz, M. J., Villar, L. A., and Ridde, V. (2015). Why are people with dengue dying? A scoping review of determinants for dengue mortality. BMC Infect. Dis. 15:301. doi: 10.1186/s12879-015-1058-x
Caron, M., Grard, G., Paupy, C., Mombo, I. M., Bi Nso, B., Kassa Kassa, F. R., Nkoghe, D., et al. (2013). First evidence of simultaneous circulation of three different dengue virus serotypes in Africa. PLoS One 8:e78030. doi: 10.1371/journal.pone.0078030
Carter, R., and Mendis, K. N. (2002). Evolutionary and historical aspects of the burden of malaria. Clin. Microbiol. Rev. 15, 564–594. doi: 10.1128/CMR.15.4.564-594.2002
Chambers, T., Hahn, C., Galle, R., and Rice, C. (1990). Flavivirus genome organization, expression, and replication. Annu. Rev. Microbiol. 44, 649–688. doi: 10.1146/annurev.mi.44.100190.003245
Charrel, R. N., Brouqui, P., Foucault, C., and de Lamballerie, X. (2005). Concurrent dengue and malaria. Emerg. Infect. Dis. 11, 1153–1154. doi: 10.3201/eid1107.041352
Chu, H. J., Chan, T. C., and Jao, F. J. (2013). GIS-aided planning of insecticide spraying to control dengue transmission. Int. J. Health. Geogr. 12:42. doi: 10.1186/1476-072X-12-42
Clark, I. A. (2007). The advent of the cytokine storm. Immunol. Cell Biol. 85, 271–273. doi: 10.1038/sj.icb.7100062
Cohen, J. (2017). Dengue may bring out the worst in Zika. Science 355:1362. doi: 10.1126/science.355.6332.1362
Crawford, J. E., Alves, J. M., Palmer, W. J., Day, J. P., Sylla, M., and Ramasamy, R., et al. (2017). Population genomics reveals that an anthropophilic population of Aedes aegypti mosquitoes in West Africa recently gave rise to American and Asian populations of this major disease vector. BMC Biol. 15:16. doi: 10.1186/s12915-017-0351-0
Curtin, P. D. (1993). Disease exchange across the tropical Atlantic. Hist. Philos. Life Sci. 15, 329–356.
de Alwis, R., Williams, K. L., Schmid, M. A., Lai, C.-Y., Patel, B., and Smith, S. A., et al. (2014). Dengue viruses are enhanced by distinct populations of serotype cross-reactive antibodies in human immune sera. PLoS Pathog. 10:e1004386. doi: 10.1371/journal.ppat.1004386
de Araújo Lobo, J. M., Mores, C. N., Bausch, D. G., and Christofferson, R. C. (2016). Short report: serological evidence of under-reported dengue circulation in Sierra Leone. PLoS Negl. Trop. Dis. 10:e0004613. doi: 10.1371/journal.pntd.0004613
Endy, T. P. (2014). Human immune responses to dengue virus infection: lessons learned from prospective cohort studies. Front. Immunol. 5:183. doi: 10.3389/fimmu.2014.00183
Epelboin, L., Hanf, M., Dussart, P., Ouar-Epelboin, S., Djossou, F., Nacher, M., et al. (2012). Is dengue and malaria co-infection more severe than single infections? A retrospective matched-pair study in French Guiana. Malar. J. 11:142. doi: 10.1186/1475-2875-11-142
Fagbami, A. H., Monath, T. P, and Fabiyi, A. (1977). Dengue virus infections in Nigeria: a survey for antibodies in monkeys and humans. Trans. R. Soc. Trop. Med. Hyg. 71, 60–65. doi: 10.1016/0035-9203(77)90210-3
Gardner, M. J., Hall, N., Fung, E., White, O., Berriman, M., and Hyman, R. W. et al. (2002). Genome sequence of the human malaria parasite Plasmodium falciparum. Nature 419, 498–511. doi: 10.1038/nature01097
Geser, A., Henderson, B. E., and Christensen, S. (1970). A multipurpose serological survey in Kenya. 2. Results of arbovirus serological tests. Bull. World Health Organ. 43, 539–552.
Gideon (2018). The World’s Premier Global Infectious Diseases Database. Available at: www.gideononline.com [accessed May 13, 2018].
Greenwood, B. M. (1974). Possible role of a B-cell mitogen in hypergammaglobulinaemia in malaria and trypanosomiasis. Lancet 16, 435–436. doi: 10.1016/S0140-6736(74)92386-1
Greenwood, B. M., and Targett, G. A. (2011). Malaria vaccines and the new malaria agenda. Clin. Microbiol. Infect. 17, 1600–1607. doi: 10.1111/j.1469-0691.2011.03612.x
Gubler, D. J., and Trent, D. W. (1994). Emergence of epidemic dengue/dengue hemorrhagic fever as a public health problem in the Americas. Infect. Agents Dis. 2, 83–393.
Gubler, D. J. (1987). Dengue and dengue hemorrhagic fever in the Americas. P. R. Health Sci. J. 6, 107–111.
Guzman, M. G., and Kouri, G. (2003). Dengue and dengue hemorrhagic fever in the Americas: lessons and challenges. J. Clin. Virol. 27, 1–13. doi: 10.1016/S1386-6532(03)00010-6
Hadinegoro, S. R., Arredondo-García, J. L., Capeding, M. R., Deseda, C., Chotpitayasunondh, T., Dietze, R., et al. (2015). Efficacy and long-term safety of a dengue vaccine in regions of endemic disease. N. Engl. J. Med. 373, 1195–1206. doi: 10.1056/NEJMoa1506223
Halsey, E. S., Baldeviano, G. C., Edgel, K. A., Vilcarromero, S., Sihuincha, M., and Lescano, A. G. (2016). Symptoms and immune markers in plasmodium/dengue virus co-infection compared with mono-infection with either in Peru. PLoS Negl. Trop. Dis. 10:e0004646. doi: 10.1371/journal.pntd.0004646
Halstead, S. B. (1980). Dengue haemorrhagic fever- a public health problem and a field for research. Bull. World Health Organ. 58, 1–21.
Halstead, S. B. (1988). Pathogenesis of dengue: challenges to molecular biology. Science 239, 476–481. doi: 10.1126/science.239.4839.476
Halstead, S. B. (1990). Global epidemiology of dengue hemorrhagic fever. Southeast Asian J. Trop. Med. Public Health 21, 636–641.
Halstead, S. B. (2012). Controversies in dengue pathogenesis. Paediatr. Int. Child Health 32(Suppl. 1), 5–9. doi: 10.1179/2046904712Z.00000000045
Halstead, S. B. (2015). Pathogenesis of dengue: dawn of a new Era. F1000Res. 4:F1000 Faculty Rev-476. doi: 10.12688/f1000research.7024.1
Halstead, S. B., Streit, T. G., Lafontant, J. G., Putvatana, R., Russell, K., Sun, W., et al. (2001). Haiti: absence of dengue hemorrhagic fever despite hyperendemic dengue virus transmission. Am. J. Trop. Med. Hyg. 65, 180–183. doi: 10.4269/ajtmh.2001.65.180
Hammon, W. M., and Sather, G. E. (1964). Virological findings in the 1960 hemorrhagic fever epidemic (Dengue) in Thailand. Am. J. Trop. Med. Hyg. 13, 629–641. doi: 10.4269/ajtmh.1964.13.629
Hammon, W. M., Rudnic, A., and Sather, G. E. (1960). Viruses associated with epidemic hemorrhagic fevers of the Philippines and Thailand. Science 131, 1102–1103. doi: 10.1126/science.131.3407.1102
Hati, A. K., Bhattacharjee, I., Mukherjee, H., Bandyopadhayay, B., Bandyopadhyay, D., De, R., et al. (2012). Concurrent dengue and malaria in an area in Kolkata. Asian Pac. J. Trop. Med. 5, 315–317. doi: 10.1016/S1995-7645(12)60046-7
Hoffman, S. L. (1986). “Treatment of malaria,” in Malaria Clinics in Tropical Medicine and Communicable Diseases, Vol. 1, ed. G. T. Strickland (London: WB Saunders Company), 214.
Holmes, E. C., and Twiddy, S. (2003). The origin, emergence and evolutionary genetics of dengue virus. Infect. Genet. Evol. 3, 19–28. doi: 10.1016/S1567-1348(03)00004-2
Houba, V., Faulk, W. P., and Matola, Y. G. (1974). Heterophilic antibodies in relation to malarial infection: population and experimental studies. Clin. Exp. Immunol. 18, 89–93.
Hunsperger, E. A., Yoksan, S., Buchy, P., Nguyen, V. C., Sekaran, S. D., Enria, D. A., et al. (2009). Evaluation of commercially available anti-dengue virus immunoglobulin M tests. Emerg. Infect. Dis. 15, 436–440. doi: 10.3201/eid1503.080923
INDEPTH (2018). INDEPTH Network. Available at: http://www.indepth-network.org/ [accessed February 17, 2018].
Kawiecki, A. B. and Christofferson, R. C. (2016). Zika virus-induced antibody response enhances dengue virus serotype 2 replication in vitro. J. Infect. Dis. 214, 1357–1360. doi: 10.1093/infdis/jiw377
Kirchner, S., Power, B. J., and Waters, A. P. (2016). Recent advances in malaria genomics and epigenomics. Genome Med. 8:92. doi: 10.1186/s13073-016-0343-7
Kramer, K., Mandike, R., Nathan, R., Mohamed, A., Lynch, M., Brown, N., et al. (2017). Effectiveness and equity of the Tanzania National Voucher Scheme for mosquito nets over 10 years of implementation. Malar. J. 16:255. doi: 10.1186/s12936-017-1902-0
Larsen, C. P., Whitehead, S. S., and Durbin, A. P. (2015). Dengue human infection models to advance dengue vaccine development. Vaccine 33, 7075–7082. doi: 10.1016/j.vaccine.2015.09.052
L’Azou, M., Succo, T., Kamagaté, M., Ouattara, A., Gilbernair, E., Adjogoua, E., et al. (2015). Dengue: etiology of acute febrile illness in Abidjan, Côte d’Ivoire, in 2011-2012. Trans. R. Soc. Trop. Med. Hyg. 109, 717–722. doi: 10.1093/trstmh/trv076
Li, Y., Kamara, F., Zhou, G., Puthiyakunnon, S., Li, C., Liu, Y., et al. (2014). Urbanization increases Aedes albopictus larval habitats and accelerates mosquito development and survivorship. PLoS Negl. Trop. Dis. 8:e3301. doi: 10.1371/journal.pntd.0003301
López-Gatell, H., Alpuche-Aranda, C. M., Santos-Preciado, J. I., and Hernández-Ávila, M. (2016). Dengue vaccines: local decision, global consequences. Bull. World Health Organ. 94, 850–855. doi: 10.2471/BLT.15.168765
Luckhart, S., Pakpour, N., and Giulivi, C. (2015). Host-pathogen interactions in malaria: cross-kingdom signaling and mitochondrial regulation. Curr. Opin. Immunol. 36, 73–79. doi: 10.1016/j.coi.2015.07.002
MAP (2018). Malaria Atlas Project. Available at: https://map.ox.ac.uk [accessed February 17, 2018].
Massangaie, M., Pinto, G., Padama, F., Chambe, G., da Silva, M., Mate, I., et al. (2016). Clinical and epidemiological characterization of the first recognized outbreak of dengue virus-type 2 in Mozambique, 2014. Am. J. Trop. Med. Hyg. 94, 413–441 doi: 10.4269/ajtmh.15-0543
Mboera, L. E., Mweya, C. N., Rumisha, S. F., Tungu, P. K., Stanley, G., Makange, M. R., et al. (2016). The Risk of Dengue Virus Transmission in Dar es Salaam, Tanzania during an epidemic period of 2014. PLoS Negl. Trop. Dis. 10:e0004313. doi: 10.1371/journal.pntd.0004313
McCullers, J. A. (2014). The co-pathogenesis of influenza viruses with bacteria in the lung. Nat. Rev. Microbiol. 12, 252–262. doi: 10.1038/nrmicro3231
Messina, J. P., Brady, O. J., Scott, T. W., Zou, C., Pigott, D. M., Duda, K. A., et al. (2014). Global spread of dengue virus types: mapping the 70 year history. Trends Microbiol. 22, 138–146. doi: 10.1016/j.tim.2013.12.011
Mohapatra, M. K., Patra, P., and Agrawala, R. (2012). Manifestation and outcome of concurrent malaria and dengue infection. J. Vector Borne Dis. 49, 262–265.
Moore, R. (1930). Late syphilis-treatment with a single strain of malaria: analytical evaluation of therapeutic results in four hundred cases. Cal. West. Med. 33, 796–798.
Moraes, G. H., de Fátima Duarte, E., and Duarte, E. C. (2013). Determinants of mortality from severe dengue in Brazil: a population-based case-control study. Am. J. Trop. Med. Hyg. 88, 670–676. doi: 10.4269/ajtmh.11-0774
Muraille, E. (2016). The unspecific side of acquired immunity against infectious disease: causes and consequences. Front. Microbiol. 6:1525. doi: 10.3389/fmicb.2015.01525
Noymer, A. (2011). The 1918 influenza pandemic hastened the decline of tuberculosis in the United States: an age, period, cohort analysis. Vaccine. 22, 29 Suppl 2:B38-41. doi: 10.1016/j.vaccine.2011.02.053.
Onoja, A. B., and Adeniji, J. A., Olaleye, O. D. (2016). High rate of unrecognized dengue virus infection in parts of the rainforest region of Nigeria. Acta Trop. 160, 39–43. doi: 10.1016/j.actatropica.2016.04.007
Palacios, J. H. R., Alzate, A., Romero H. J. M., Concha-Eastman, A. I. (2016). Afro Colombian ethnicity, a paradoxical protective factor against Dengue. Colomb. Med. 47, 133–141.
Pang, T., Cardosa, M. J., and Guzman, M. G. (2007). Of cascades and perfect storms: the immunopathogenesis of dengue haemorrhagic fever-dengueshock syndrome (DHF/DSS). Immunol. Cell Biol. 85, 43–45. doi: 10.1038/sj.icb.7100008
Parkes-Ratanshi, R., Elbireer, A., Mbambu, B., Mayanja, F., Coutinho, A., and Merry, C. (2014). Ebola outbreak response; experience and development of screening tools for viral haemorrhagic fever (VHF) in a HIV center of excellence near to VHF epicentres. PLoS One 9:e100333. doi: 10.1371/journal.pone.0100333
Pitchenik, A. E., Fischl, M. A., Dickinson, G. M., Becker, D. M., Fournier, A. M., O’Connell, M. T., et al. (1983). Opportunistic infections and Kaposi’s sarcoma among Haitians: evidence of a new acquired immunodeficiency state. Ann. Intern. Med. 98, 277–284. doi: 10.7326/0003-4819-98-3-277
Reyburn, H., Mbatia, R., Drakeley, C., Bruce, J., Carneiro, I., Olomi, R., et al. (2005). Association of transmission intensity and age with clinical manifestations and case fatality of severe Plasmodium falciparum malaria. JAMA 293, 1461–1470. doi: 10.1001/jama.293.12.1461
Ribeiro, C. D. (1988). Polyclonal B-cell activation (PBA). What have we learned from the study of malaria? Mem. Inst. Oswaldo Cruz 83(Suppl. 1), 633–648. doi: 10.1590/S0074-02761988000500076
Rico-Hesse, R. (2003). Microevolution and virulence of dengue viruses. Adv. Virus Res. 59, 315–341. doi: 10.1016/S0065-3527(03)59009-1
Ridde, V., Agier, I., Bonnet, E., Carabali, M., Dabiré, K. R., Fournet, F., et al. (2016). Presence of three dengue serotypes in Ouagadougou (Burkina Faso): research and public health implications. Infect. Dis. Poverty 5:23. doi: 10.1186/s40249-016-0120-2
Rosenke, K., Adjemian, J., Munster, V. J., Marzi, A., Falzarano, D., Onyango, C. O., et al. (2016). Plasmodium Parasitemia associated with increased survival in Ebola virus-infected patients. Clin. Infect. Dis. 63, 1026–1033. doi: 10.1093/cid/ciw452
Rothman, A. L., and Ennis, F. A. (1999). Immunopathogenesis of Dengue hemorrhagic fever. Virology 257, 1–6. doi: 10.1006/viro.1999.9656
Rothman, A. L., Medin, C. L., Friberg, H., and Currier, J. R. (2014). Immunopathogenesis versus protection in dengue virus infections. Curr. Trop. Med. Rep. 1, 13–20. doi: 10.1007/s40475-013-0009-0
Royer, D. (2015). Upstate Medical University. Available at: www.upstate.edu/news/article.php?title=8993
Rudnick, A., Marchette, N. J., Garcia, R. (1967). Possible jungle dengue- recent studies and hypotheses. Jpn. J. Med. Sci. Biol. 20 Suppl, 69–74.
Sabin, A. B. (1952). Research on dengue during World War II. Am. J. Trop. Med. Hyg. 1, 30–50.. doi: 10.4269/ajtmh.1952.1.30
Saluzzo, J. F., Cornet, M., Castagnet, P., Rey, C., Digoutte, J. P. (1986). Isolation of dengue 2 and dengue 4 viruses from patients in Senegal. Trans. R. Soc. Trop. Med. Hyg. 80:5. doi: 10.1016/0035-9203(86)90182-3
San Martín, J. S., Brathwaite, O., Zambrano, B., Solórzano, J. O., Bouckenooghe, A., Dayan, G. H., et al. (2010). The epidemiology of dengue in the Americas over the last three decades: a worrisome reality. Am. J. Trop. Med. Hyg. 82, 128–135. doi: 10.4269/ajtmh.2010.09-0346
Screaton, G., Mongkolsapaya, J., Yacoub, S., and Roberts, C. (2015). New insights into the immunopathology and control of dengue virus infection. Nat. Rev. Immunol. 15, 745–759. doi: 10.1038/nri3916
Sharp, T. M., Moreira, R., Soares, M. J., Miguel da Costa, L., Mann, J., DeLorey, M., et al. (2015). Underrecognition of Dengue during 2013 Epidemic in Luanda, Angola. Emerg. Infect. Dis. 21, 1311–1316. doi: 10.3201/eid2108.150368
Shepard, D. S., Undurraga, E. A., Betancourt-Cravioto, M., Guzmán, M. G., Halstead, S. B., Harris, E., et al. (2014). Approaches to refining estimates of global burden and economics of dengue. PLoS Negl. Trop. Dis. 8:e3306. doi: 10.1371/journal.pntd.0003306
Snow, R. W., Sartorius, B., Kyalo, D., Maina, J., Amratia, P., and Mundia, C. W., et al. (2017). The prevalence of Plasmodium falciparum in sub-Saharan Africa since 1900. Nature 550, 515–518. doi: 10.1038/nature24059
Stanaway, J. D., Shepard, D. S, Undurraga, E. A., Halasa, Y. A., Coffeng, L. E., Brady, O. J., et al. (2016). The global burden of dengue: an analysis from the Global Burden of Diseases Study 2013. Lancet Infect. Dis. 16, 712–723. doi: 10.1016/S1473-3099(16)00026-8
Stephens, H. A. (2010). HLA and other gene associations with dengue disease severity. Curr. Top. Microbiol. Immunol. 338, 99–114. doi: 10.1007/978-3-642-02215-9_8
Stoler, J., Delimini, R. K., Bonney, J. H., Oduro, A. R., Owusu-Agyei, S., Fobil, J. N., Awandare, G. A. (2015). Evidence of recent dengue exposure among malaria parasite-positive children in three urban centers in Ghana. Am. J. Trop. Med. Hyg. 92, 497–500. doi: 10.4269/ajtmh.14-0678
Streatfield, P. K., Khan, W. A., Bhuiya, A., Hanifi, S. M., Alam, N., and Diboulo, E., et al. (2014). Malaria mortality in Africa and Asia: evidence from INDEPTH health and demographic surveillance system sites. Glob. Health Action 7:25369. doi: 10.3402/gha.v7.25369
Strøm, G. E. A., Haanshuus, C. G., Fataki, M., Langeland, N., and Blomberg, B. (2013). Challenges in diagnosing paediatric malaria in Dar es Salaam, Tanzania. Malar. J. 12:228. doi: 10.1186/1475-2875-12-228
Tanner, M., and de Savigny, D. (2008). Malaria eradication back on the table. Bull. World Health Organ. 86:82. doi: 10.2471/BLT.07.050633
Tatem, A. J., Gething, P. W., Smith, D. L., and Hay, S. I. (2013). Urbanization and the global malaria recession. Malar. J. 12:133. doi: 10.1186/1475-2875-12-133
Toledo, J., George, L., Martinez, E., Lazaro, A., Han, W. W., Coelho, G. E., et al. (2016). Relevance of non-communicable comorbidities for the development of the severe forms of dengue: a systematic literature review. PLoS Negl. Trop. Dis. 10:e0004284. doi: 10.1371/journal.pntd.0004284
Tomson, G. (1933). Immunity in malaria. Trans. R. Soc. Trop. Med. Hyg. 26, 483–503. doi: 10.1016/S0035-9203(33)90123-6
Trunfio, M., Savoldi, A., Viganò, O., and d’Arminio Monforte, A. (2017). Bacterial coinfections in dengue virus disease: what we know and what is still obscure about an emerging concern. Infection 45, 1–10. doi: 10.1007/s15010-016-0927-6
Twiddy, S. S., Holmes, E. C., and Rambaut, A. (2003). Inferring the rate and time-scale of dengue virus evolution. Mol. Biol. Evol. 20, 122–129. doi: 10.1093/molbev/msg010
Vairo, F., Mboera, L. E. G., De Nardo, P., Oriyo, N. M., Meschi, S., Rumisha, S. F., et al. (2016). Clinical, virologic, and epidemiologic characteristics of dengue outbreak, Dar es Salaam, Tanzania, 2014. Emerg. Infect. Dis. 22, 895–899. doi: 10.3201/eid2205.151462
Villar, L., Dayab, G. H., Aredono-Garcia, J. L., Rivera, D. M., Cunha, R., Deseda, C., et al. (2015) Efficacy of a tetravalent dengue vaccine in children in Latin America. N. Engl. J. Med. 372, 113–123. doi: 10.1056/NEJMoa1411037
Wang, T. T., Sewatanon, J., Memoli, M. J., Wrammert, J., Bournazos, S., Bhaumik, S. K., et al. (2017). IgG antibodies to dengue enhanced for FcγRIIIA binding determine disease severity. Science 355, 395–398. doi: 10.1126/science.aai8128
Wardrop, N. A. (2016). Integrated epidemiology for vector-borne zoonoses. Trans. R. Soc. Trop. Med. Hyg. 110, 87–89. doi: 10.1093/trstmh/trv115
WHO (2013). Malaria Policy Advisory Committee to the WHO: conclusions and recommendations of September 2013 meeting. Malar. J. 12:456. doi: 10.1186/1475-2875-12-456
WHO (1997). Dengue Haemorrhagic Fever: Diagnosis, Treatment, Prevention and Control, 2nd edn. Geneva: WHO
WHO (2009). Evaluation of Commercially Available Anti-Dengue Virus Immunoglobulin M Tests. Available at: http://www.who.int/tdr/publications/documents/diagnostics-evaluation-3.pdf?ua=1 [accessed February 18, 2017].
WHO (2016). Dengue Vaccine: WHO Position Paper - July 2016. Available at: http://www.who.int/wer/2016/wer9130.pdf?ua=1 [accessed February 18, 2017].
WHO (2017a). Dengue and Severe Dengue. Fact sheet, Updated April 2017. Available at: http://www.who.int/mediacentre/factsheets/fs117/en/ [accessed February 18, 2017].
WHO (2017b). GACVS Statement on Dengvaxia® (CYD-TDV). Available at: http://www.who.int/vaccine_safety/committee/GACVS-StatementonDengvaxia-CYD-TDV/en/ [accessed February 18, 2017].
Wilson, M. L. Krogstad, D. J., Arinaitwe, E., Arevalo-Herrera, M., Chery, L., Marcelo, U. et al. (2015). Urban Malaria: understanding its epidemiology, ecology, and transmission across seven diverse ICEMR network sites. Am. J. Trop. Med. Hyg. 93(3 Suppl), 110–123. doi: 10.4269/ajtmh.14-0834
Keywords: dengue, falciparum malaria, co-morbidity, cross-protection, heterologous immunity, interaction
Citation: Bygbjerg IC, Simonsen L and Schiøler KL (2018) Elimination of Falciparum Malaria and Emergence of Severe Dengue: An Independent or Interdependent Phenomenon? Front. Microbiol. 9:1120. doi: 10.3389/fmicb.2018.01120
Received: 23 January 2018; Accepted: 11 May 2018;
Published: 30 May 2018.
Edited by:
Leonard Peruski, Centers for Disease Control and Prevention, United StatesReviewed by:
V. Ann Stewart, Uniformed Services University of the Health Sciences, United StatesTonya Michelle Colpitts, Boston University, United States
Copyright © 2018 Bygbjerg, Simonsen and Schiøler. This is an open-access article distributed under the terms of the Creative Commons Attribution License (CC BY). The use, distribution or reproduction in other forums is permitted, provided the original author(s) and the copyright owner are credited and that the original publication in this journal is cited, in accordance with accepted academic practice. No use, distribution or reproduction is permitted which does not comply with these terms.
*Correspondence: Ib C. Bygbjerg, SWJ5QHN1bmQua3UuZGs=