- 1Laboratorio 5: LEDEFAR, Unidad de Investigacion Multidisciplinaria, Facultad de Estudios Superiores Cuautitlan, Universidad Nacional Autonoma de México, Cuautitlan Izcalli, Mexico
- 2Departamento de Medicina Veterinária Preventiva, Centro de Diagnóstico e Pesquisa em Patologia Aviária, Universidade Federal do Rio Grande do Sul, Porto Alegre, Brazil
- 3Department of Poultry Science, University of Arkansas, Fayetteville, AR, United States
- 4Departamento de Medicina y Zootecnia de Aves, Facultad de Medicina Veterinaria y Zootecnia, Universidad Nacional Autónoma de México, Mexico City, Mexico
- 5Laboratorio 14: Alimentos, Micotoxinas y Micotoxicosis, Unidad de Investigacion Multidisciplinaria, Facultad de Estudios Superiores Cuautitlan, Universidad Nacional Autonoma de México, Cuautitlan Izcalli, Mexico
In the present study, in vitro assays were conducted to evaluate the solubility of curcumin (CUR) alone or with polyvinylpyrrolidone (PVP) at different pH, as well as its permeability in Caco-2 cells. Results confirmed that the solid dispersion of CUR with PVP (CUR/PVP) at a 1:9 ratio, significantly increased (P < 0.05) solubility and permeability compared to CUR alone. Then, the antimicrobial activity of CUR/PVP, boric acid (BA), and a combination of 0.5% CUR/PVP and 0.5% BA (CUR/PVP-BA) against Salmonella Enteritidis (SE) was determined using an in vitro digestion model that simulates crop, proventriculus, and intestine. The results revealed that in the proventriculus and intestinal compartments significant reductions of SE were observed in all the experimental treatments, but 1% BA eliminated SE in the intestinal compartment and CUR/PVP-BA showed a synergistic effect on antimicrobial activity against SE. To complement these findings, two independent in vivo trials were conducted to determine the effect of 0.1% CUR/PVP; 0.1% BA; or the combination of 0.05% CUR/PVP (1:9 ratio) and 0.05% BA (CUR/PVP-BA) on the antimicrobial activity against SE, intestinal permeability and inflammatory responses in broiler chickens. BA at 0.1% had no significant in vivo effects against SE. However, the combination of 0.05% BA and 0.05% CUR/PVP and 0.05% BA was sufficient to reduce crop and intestinal SE colonization in broiler chickens in two independent trials, confirming the synergic effect between them. A similar antimicrobial impact against SE intestinal colonization was observed in chickens treated with 0.1% CUR/PVP at a 1:9 ratio, which could be due to the increase in solubility of CUR by PVP. Furthermore, 0.1% CUR/PVP reduced the intestinal permeability of FITC-d and total intestinal IgA, as well as increase the activity of SOD when compared to control, while, CUR/PVP-BA only decreased SOD activity. Further studies to confirm and expand the in vivo results obtained in this pilot study, adding intestinal microbial commensal groups and more inflammatory biomarkers to get a complete description of the effects of BA and CUR deserves further investigation.
Introduction
Salmonella enterica serovar Enteritidis is a pathogen frequently found in the gastrointestinal tract of poultry which can also cause foodborne illness in humans. The primary route of transmission from animals to humans is through contaminated food or foodstuffs such as eggs, egg products and poultry meat (Mani-López et al., 2012; Bertelloni et al., 2017). It is estimated that the consequences of foodborne diseases are substantial (WHO, 2018). Furthermore, the use of antibiotics as growth promoters in animal production have stressed the need for alternatives that can offer similar performance benefits. These alternatives include probiotics, prebiotics and plant extracts (Huyghebaert et al., 2011); bacteriophages (Andreatti Filho et al., 2007); and organic acids (Menconi et al., 2011). Boron is an essential nutrient for animals, plants, and fungi.
Boron is a weak acid pKa = 9.1) and, at physiological pH, is present mostly as BA (H3BO3) (Hunt, 1994, 2003). BA is used as antibacterial, pesticide, fire retardant, and a precursor to other chemicals (Kabu and Akosman, 2013). On the other hand, CUR, is a curcuminoid of turmeric (Curcuma longa), a member of the ginger family, Zingiberaceae, used as an herbal food supplement, cosmetic and food coloring. CUR is a diarylheptanoid (natural phenols) with antioxidant and anti-inflammatory properties (Bereswill et al., 2010; Wanninger et al., 2015). In poultry production, CUR has been used as a growth promoter (Khan et al., 2012; Rajput et al., 2013) and antimicrobial (Lawhavinit et al., 2010; Moghadamtousi et al., 2014) compound. In a recent in vitro study published by our laboratories, we showed that 1% CUR, had no antimicrobial effect against SE; however, the combination of BA and CUR have a synergistic bactericidal impact against SE (Hernandez-Patlan et al., 2017). However, one major limitation of CUR is its low solubility and bioavailability. PVP is a water-soluble synthetic polymer used as a binder and as a lubricant for eye drops (Haaf et al., 1985). Furthermore, it has been reported that PVP can improve the solubility and dissolution of many poorly water-soluble drugs as CUR (Kaewnopparat et al., 2009).
The objectives of the present study were, first to evaluate the solubility and permeability of CUR combined with PVP in vitro. Second, to evaluate the in vitro antimicrobial effect of a solid CUR/PVP at a 1:9 ratio, BA and the combination of 0.5% CUR/PVP and 0.5% BA (CUR/PVP-BA) against SE since some interactions between them have been reported. Third, to evaluate the in vivo effects of these feed additive candidates on SE colonization to confirm the interactions between CUR/PVP and BA, and their effects in some inflammation parameters in broiler chickens.
Materials and Methods
Solubility Studies
The solubility of CUR was assessed by adding an excess amount of CUR (200 mg) (≈50%, Mixim Laboratories, Naucalpan, Mexico) to 20 mL of aqueous buffer solutions at pH 1.2, 5.2, and 6.4, containing or not PVP (Agrimer K-30, Ashland, Columbus, OH, United States) in 1:6 and 1:9 ratios (CUR/PVP). The suspensions formed were equilibrated under continuous agitation for 24 h at room temperature. Then centrifuged (Beckman Coulter Microfuge 20R, United States) at 2292 × g for 10 min at 4°C and the supernatants were filtered through a 0.45 μm pore size Millipore membrane filter. The filtrates were analyzed with a UV/VIS spectrophotometer (Varian Cary 100, United States) at 421 nm. Solubility studies were performed in triplicate. The concentration of soluble CUR was determined using a calibration curve with a concentration range of 1.36–13.6 μg/mL.
Caco-2 Cell Culture
The Caco-2 human colon cancer cells from the American Type Culture Collection (ATCC, Rockville, MD, United States) at passage 16 were cultured in T-75 cm2 flasks containing Dulbecco’s modified eagle medium (DMEM, Gibco-Invitrogen, Thermo Fisher Scientific, Illkirch-Graffenstaden, France) supplemented with glucose (4.5 g/L), FBS (15%; v/v), vitamins (2%; v/v), nonessential amino acid solution (2%; v/v), L-glutamine (2%; v/v), and antibiotic solution (2%; v/v), at 37°C in a humidified atmosphere of 5% CO2/95% air. When the cell culture reached 80–90% confluence, cells were dispersed with 0.025 M trypsin-EDTA and reseeded in a new flask. Medium was replaced every 3 days.
Permeability Studies
Caco-2 cells were seeded on the apical side of Transwell® permeable supports (Transwell Corning®, Kennebunk, EU, 0.4 μm polycarbonate membrane, 1.12 cm2 surface and a volume of 0.5 mL in the apical compartment and 1.5 mL in the basolateral compartment) at a density of 2 × 104 cells per well for 21 days. The culture medium was changed every 3 days.
Permeability studies were performed after complete cell differentiation and only wells that exhibited transepithelial electrical resistance (TEER, MERSSTX01 electrode, Millicell ERS-2, Millipore, Billerica, MA, United States) greater than 600 Ω cm2 were used. Suspensions of CUR alone or with PVP in 1:6 and 1:9 ratios were diluted in DMEM to reach a concentration of 250 μg CUR/mL (Equivalent to 125 μg of CUR per well). Before starting the experiment, the culture medium was removed, and cell monolayers were washed twice with 1 mL of PBS buffer. Then, 0.5 mL of each diluted CUR suspension was added to the apical side of the cell monolayers, while on the basolateral side 1.5 mL of DMEM was placed. Cells were incubated at 37°C for 120 min. Passage of CUR to the basolateral side was determined by UPLC-TQ-ESI-MS/MS (Waters ACQUITY UPLC system, Milford, MA, United States). Chromatographic analysis was done using a Waters ACQUITY BEH Shield RP 18 column (2.1 mm × 100 mm, 1.7 μm). The mobile phase was composed of 60% A (acetonitrile and 0.1% formic acid) and 40% B (0.1% formic acid solution). The flow rate of the mobile phase was 0.25 mL/min, and the injection volume was 7.5 μL. The column temperature was conditioned at 30°C, and the autosampler was maintained at 4°C.
Results are expressed as apparent permeability coefficient (Papp) and absorption enhancement ratio (R), according to the following equations:
Where dQ/dt is the steady-state flux, A is the surface area of the filter and C0 is the initial concentration in the donor chamber (Apical side).
Where PappCtrl is the average of the apparent permeability coefficient of CUR alone suspended in DMEM and PappTest is the average of the apparent permeability coefficient of CUR/PVP suspensions in 1:6 and 1:9 ratios.
Bacterial Strain and Culture Conditions
The organism used was a poultry isolate of Salmonella enterica serovar Enteritidis (SE), bacteriophage type 13A, obtained from the USDA National Veterinary Services Laboratory (Ames, IA, United States). The isolate is resistant to 25 μg/mL of novobiocin (NO, catalog no.N-1628, Sigma) and was selected for resistance to 20 μg/mL of nalidixic acid (NA, catalog no.N-4382, Sigma) in our laboratory. In this study, 100 μL of SE from a frozen aliquot was added to 10 mL of tryptic soy broth (Catalog No. 22092, Sigma) and incubated at 37°C for 8 h, and passed three times every 8 h to ensure that all bacteria were in log phase as previously described (Lin et al., 1995). Post-incubation, bacterial cells were washed three times with sterile 0.9% saline by centrifugation at 1864 × g for 10 min, reconstituted in saline, quantified by densitometry with a spectrophotometer (Spectronic 20D+, Spectronic Instruments Thermo Scientific, Rochester, NY, United States), and diluted to an approximate concentration of 4 × 107 cfu/mL. Concentrations of SE were further verified by serial dilution and plated on brilliant green agar (BGA, Catalog No. 70134, Sigma) with NO and NA for enumeration of actual cfu used in the experiment.
Preparation of Treatments and Diets
In Vitro Experiments
The treatments for the in vitro experiments were: (1) a control non-supplemented diet; (2) basal diet plus 1% BA (99–100%, USP grade, Drogueria Cosmopolita, Naucalpan, Mexico City, Mexico); (3) basal diet plus 1% CUR/PVP; and (4) basal diet plus a BA-CUR/PVP.
In Vivo Experiments
In the case of the in vivo experiments the treatments were: (1) a control non-supplemented diet; (2) basal diet plus 0.1% BA; (3) basal diet plus 0.1% CUR/PVP; and 4) basal diet plus BA-CUR/PVP.
In all the treatments, the particle size was homogenized using a No. 25 mesh sieve to obtain particles of around 700 μm. In both in vitro and in vivo experiments, treatment 3 was prepared by dissolving 10 g of CUR in a solution containing 90 g of PVP (CUR/PVP in a 1: 9 ratio) followed by the solvent evaporation at 40°C for 48 h and subsequently sieving. For treatment 4, in both in vitro and in vivo experiments, 50 g of BA and 50 g of CUR/PVP were combined, granulated, dried and sieved. Once prepared, each of the treatments was added to the feed in a concentration of 1% (10 g/kg of feed) to obtain the diets used in the in vitro studies and 0.1% (1 g/kg of feed) for in vivo studies. Starter feed used in this experiment was formulated to approximate the nutritional requirements for broiler chickens as recommended by the National Research Council (1994), and adjusted to breeder’s recommendations (Cobb-Vantress, Inc., 2015). No antibiotics, coccidiostats, or enzymes were added to the feed (Table 1).
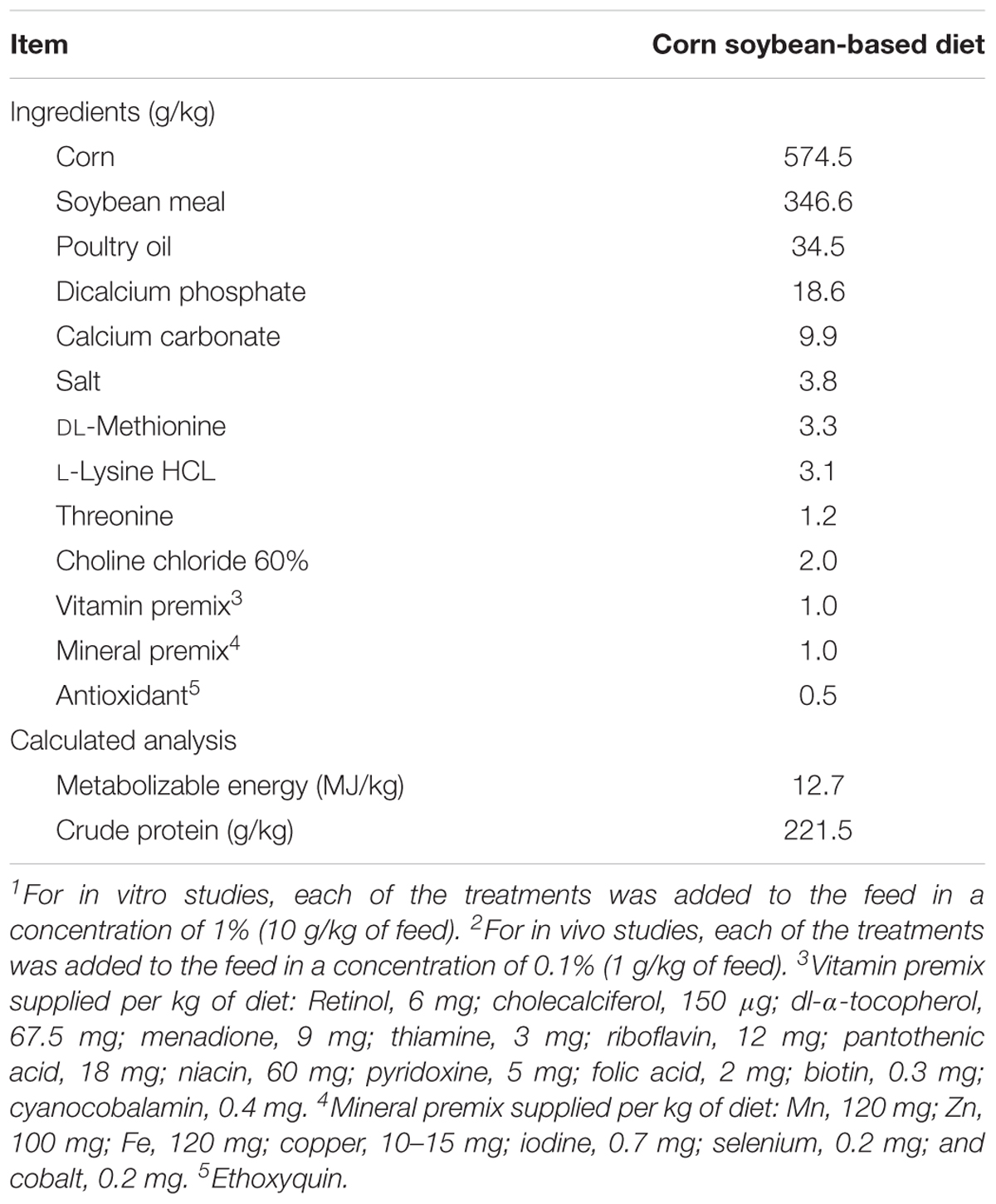
TABLE 1. Ingredient composition and nutrient content of a basal starter diet used for both in vitro1 and in vivo2 studies.
Determination of Antimicrobial Activity Using an in Vitro Digestion Model
An in vitro digestive assay previously described (Latorre et al., 2015; Hernandez-Patlan et al., 2017) was used to determine the antimicrobial activity of CUR/PVP in a 1:9 ratio, BA and CUR/PVP-BA at 1% (w/w). Experiments were run in triplicate. Briefly, the digestive model used allows simulating three main compartments of the chicken gastrointestinal tract. The first gastrointestinal compartment simulated was the crop, where 5 g of feed and 10 mL of 0.03 M hydrochloric acid (HCl; EMD Millipore Corporation, Billerica, MA, United States) were placed in 50 mL polypropylene centrifuge tubes, reaching a pH value around 5.2. In this compartment, 1 mL of SE (108 cfu/mL) was added. Tubes were then incubated for 30 min and mixed with a standard orbital shaker (19 rpm; VWR). To simulate the proventriculus as the next gastrointestinal compartment, 3000 U of pepsin per g of feed (Sigma-Aldrich, St Louis, MO, United States) and 2.5 mL of 1.5 M HCl was added to each tube, reaching a pH of 1.4–2.0. Tubes were incubated for additional 45 min. The third and final step was intended to simulate the intestinal section of the gastrointestinal tract. For that, 6.84 mg of 8× pancreatin (Sigma-Aldrich) in 6.5 mL of 1.0 M sodium bicarbonate (Sigma-Aldrich) was added, reaching a pH value between 6.4 and 6.8. Samples were further incubated for 2 h (total incubation time 3.25 h). In each compartment, a sample of 1 mL was taken and centrifuged for 30 min at 1864 × g. After that, the supernatants were discarded, and the pellets were reconstituted with 988 μL of 0.9% sterile saline and 12 μL of PrestoBlue®. These samples were incubated for 60 min at 40°C and centrifuged again for 30 min at 1864 × g. Finally, the supernatants were recovered and a sample of 100 μL was taken and placed in 96-well flat bottom Bacti plates (Catalog No. 269787; Nalgene Nunc International, Rochester, NY, United States) to measure the fluorescence intensity, which was measured a at an excitation wavelength of 530 nm and an emission wavelength of 590 nm (Synergy HT, multimode microplate reader; BioTek Instruments, Inc., Winooski, VT, United States). The fluorescence units of the samples were converted to concentration and reported as cfu/mL of SE using a calibration curve as previously described (Hernandez-Patlan et al., 2017).
Animal Source, Diets, and Experimental Design
In the present study, two independent trials were conducted. In each experiment, 60 day-of-hatch male Cobb-Vantress broiler chickens (Fayetteville, AR, United States) were randomly allocated to one of four groups (n = 15 chickens). Chicks were housed in brooder battery cages, provided with their respective diet and water ad libitum, and maintained at an age-appropriate temperature during the 7 days of the experiments. All animal handling procedures complied with Institutional Animal Care and Use Committee (IACUC) at the University of Arkansas, Fayetteville (protocol #15006). At day 6, all chicks were orally challenged with 107 cfu of SE per bird. The concentration of FITC-d was calculated based on group body weight; therefore, groups were weighed at 6 d of age. All chickens were given an appropriate dose of FITC-d by oral gavage at 7 days of age. One-hour post-FITC-d gavage, all chickens were euthanized by CO2 inhalation, and blood samples were collected from the femoral vein and centrifuged (1000 × g for 15 min) to separate the serum from the red blood cells for FITC-d and SOD determination. Furthermore, intestinal samples for total IgA levels, as well as crop and CCT to evaluate SE recovery were also collected.
Salmonella Recovery
In this experiment, crop and CCT were homogenized and diluted with saline (1:4 by w/v), and 10-fold dilutions were plated on BGA with NO and NA, incubated at 37°C for 24 h to enumerate total SE colony forming units. Subsequently, the crop and CCT samples were enriched in 2× concentrated tetrathionate enrichment broth and further incubated at 37°C for 24 h. Following this, enrichment samples were confirmed negative for Salmonella by streak plating on Xylose Lysine Tergitol-4 (XLT-4, Catalog No. 223410, BD DifcoTM) selective media.
Serum Determination of FITC-d Leakage
Intestinal leakage of FITC-d (MW 3–5 kDa; Sigma-Aldrich Co., St. Louis, MO, United States) and the measurement of its serum concentration were determined since FITC-d is a marker of paracellular transport and mucosal barrier dysfunction (Yan et al., 2009; Kuttappan et al., 2015; Vicuña et al., 2015a,b). One hour before the chicks were humanely killed by CO2 inhalation, 12 chicks of each group were given an oral gavage dose of FITC-d (8.32 mg/kg), and the rest of chickens were used as controls. FITC-d levels of diluted sera were measured at excitation wavelength of 485 nm and an emission wavelength of 528 nm (Synergy HT, Multi-mode microplate reader, BioTek Instruments, Inc., VT, United States) (Baxter et al., 2017).
Superoxide Dismutase Activity
Superoxide dismutase activity was measured in serum samples using a commercial assay kit (Cayman chemical company, Item No. 706002, Ann Arbor, MI, United States) following the manufacturer’s instructions. Three types of SOD (Cu/Zn, Mn, and FeSOD) were determined and the optimal dilution to quantify the SOD activity was 1:5. Samples were measured at 450 nm using an ELISA plate reader (Synergy HT, multi-mode microplate reader, BioTek Instruments, Inc., Winooski, VT, United States).
Intestinal Total Immunoglobulin A (IgA) Levels
Total IgA levels were determined in gut rinse samples as previously described (Merino-Guzmán et al., 2017). Briefly, an intestinal section of 5 cm from Meckel’s diverticulum was taken and rinsed three times with 5 mL 0.9% saline; then the rinse was collected in a tube and centrifuged at 1864 × g at 4°C for 10 min. The supernatant was poured into a 96-microwell plate and stored at -20°C until tested. A commercial indirect ELISA set was used to quantify IgA according to the manufacturer’s instructions (Catalog No. E30-103, Bethyl Laboratories, Inc., Montgomery, TX, United States). 96-well plates (Catalog No. 439454, Nunc MaxiSorp, Thermo Fisher Scientific, Rochester, NY) were used, and samples were measured at 450 nm using an ELISA plate reader (Synergy HT, multi-mode microplate reader, BioTek Instruments, Inc., Winooski, VT, United States). The obtained chicken IgA concentration was multiplied by the dilution factor (1:100) to determine the amount of chicken IgA in the undiluted samples.
Statistical Analysis
Data from bacterial counts (SE Log10 cfu/g) in crop and CCT, SOD activity, total IgA levels and serum FITC-d concentration were subjected to analysis of variance as a completely randomized design, using the General Linear Models procedure of SAS (SAS Institute, 2002). Significant differences among the means were determined by Duncan’s multiple-range test at P < 0.05. Enrichment data were expressed as positive/total chickens (%), and the percent recovery of SE was compared by chi-squared analysis (Zar, 1984), testing all possible combinations to determine the significance (P < 0.05).
Results
The results of the solubility studies of CUR alone or with PVP at different ratios and pH conditions under room temperature are summarized in Table 2. At pH 1.2 and 5.2, CUR showed a very low solubility, and at pH 6.4 the solubility was not determined due to CUR degradation. However, when CUR was mixed with PVP, the solubility of CUR was significantly improved (P < 0.05), particularly at a CUR-PVP ratio of 1:9.
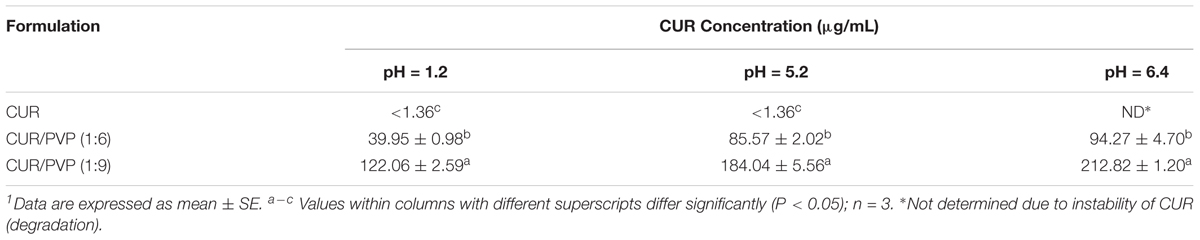
TABLE 2. Solubility of curcumin (CUR) alone or with polyvinylpyrrolidone (PVP) in different proportions at pH 1.2, 5.2, and 6.4 under room temperature1.
Table 3 shows the results of the evaluation of mean apparent permeability (Papp) and the absorption enhancement ratio (R) of CUR with PVP at different ratios across Caco-2 cell monolayers after two h incubation. The permeability of CUR in the Caco-2 cells was significantly improved by CUR-PVP ratio 1:9, followed by CUR-PVP ratio 1:6. The lowest permeability value was for CUR alone (Table 3).

TABLE 3. Evaluation of mean apparent permeability (Papp) and the absorption enhancement ratio (R) of curcumin (CUR) with polyvinylpyrrolidone (PVP) at different ratios across Caco-2 cell monolayers after 2 h incubation1.
The results of the evaluation of the antimicrobial activity of CUR/PVP, BA or CUR/PVP-BA on SE using the in vitro digestion model and a fluorometric method with PrestoBlue® are summarized in Table 4. None of the experimental groups reduced SE in the crop compartment. However, significant reductions in SE were observed in all the experimental treatments in the compartments that simulate the proventriculus and intestine. It was remarkable to observe that in just 3.25 h, 1% BA eliminated SE in the intestinal compartment (Table 4).
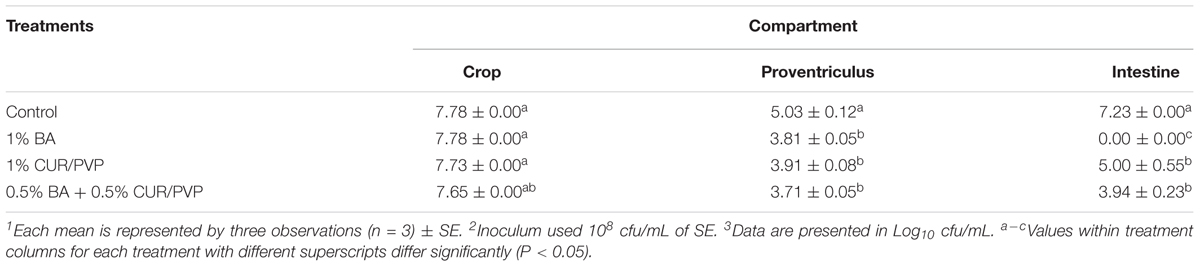
TABLE 4. Evaluation of the antimicrobial activity of curcumin with polyvinylpyrrolidone (CUR/PVP, 1:9 ratio), boric acid (BA), and CUR/PVP-BA1 on Salmonella Enteritidis (SE)2 using an in vitro digestion model and a fluorometric method with PrestoBlue®3.
Table 5 summarizes the in vivo results of CUR/PVP, BA or CUR/PVP-BA administration on SE colonization in the crop and CCT of broiler chickens in trial 1 and trial 2. There was no reduction of SE colonization in the crop in chicks receiving 0.1% CUR/PVP or 0.1% BA in both trials. However, when products were used in combination, there was a significant reduction in SE colonization in the crop, which was consistent in both trials. This synergistic effect was even more apparent after enrichment of the samples. Chickens receiving 0.1% of CUR/PVP or 0.1% CUR/PVP-BA (0.05% CUR/PVP and 0.05% BA) had a significant reduction in the number of positive SE samples in the crop and CCT compared to the control non-treated chickens (Table 5). The results of the dietary administration of CUR/PVP, BA or CUR/PVP-BA on serum FITC-d concentration, SOD activity and IgA levels in broiler chickens are shown in Table 6. In the present study, no significant difference was observed in body weight and body weight gain between treatments (Data not shown).
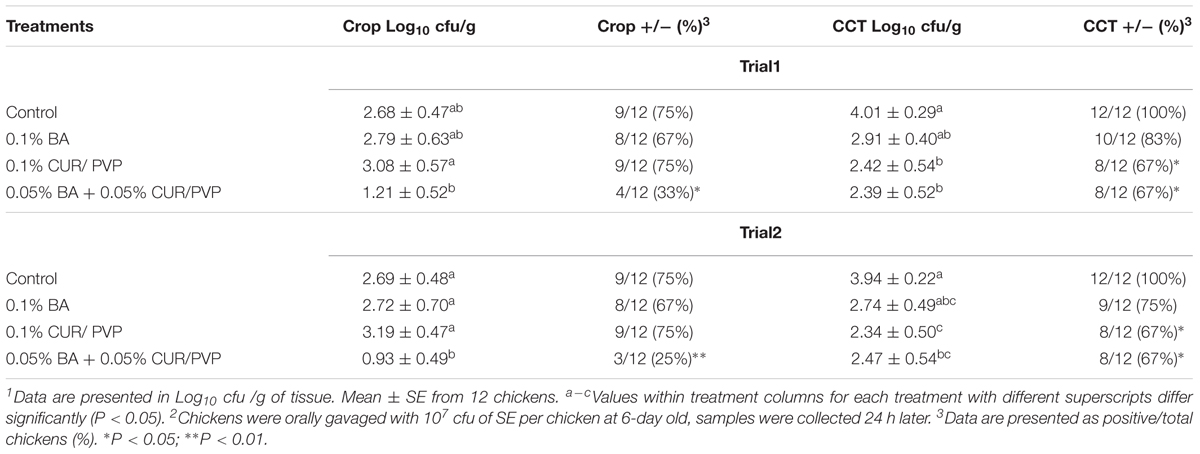
TABLE 5. Evaluation of curcumin with polyvinylpyrrolidone (CUR/PVP, 1:9 ratio), boric acid (BA), and CUR/PVP-BA on crop and ceca-cecal tonsils (CCT) colonization of Salmonella Enteritidis (SE)1 in broiler chickens in trial 1 and trial 22.

TABLE 6. Evaluation of curcumin with polyvinylpyrrolidone (CUR/PVP, 1:9 ratio), boric acid (BA), and CUR/PVP-BA on serum concentration of fluorescein isothiocyanate–dextran (FITC-d), superoxide dismutase (SOD) activity and immunoglobulin A (IgA) levels in broiler chickens1.
In the present study, only the administration of 0.1% CUR/PVP was able to significantly reduce the intestinal permeability of FITC-d when compared to the control non-treated chickens. However, only chickens that received CUR/PVP-BA showed significant reduction of serum SOD, while those that received CUR/PVP significantly increased serum SOD compared to the other experimental groups. Interestingly, once again, chickens that received 0.1% CUR/PVP showed a significant reduction on total intestinal IgA when compared to the control non-treated chickens (Table 6).
Discussion
In the United States, foodborne infections cause more than seventy million infections and an average of five thousand loss of life each year (Allos et al., 2004) with an annual estimated cost of 51 billion dollars (Mead et al., 1999; Scharff, 2012). In addition, concerns about drug-resistant bacteria and the appearance of antimicrobial residues in animal products have driven the industry to find alternatives to antibiotic growth promoters (Subbiah, 2007; Hailu et al., 2009; Skjånes et al., 2013; Samad et al., 2016).
The in vitro antimicrobial activity of 1% BA against SE (Table 4) was similar to previously published work (Hernandez-Patlan et al., 2017). The antimicrobial activity of BA is associated with its ability to diffuse through the cell membrane of microorganisms since it is non-dissociated in the different pH of the gastrointestinal tract due to its pKa (9.1). Once inside the microorganism, BA can affect enzymatic and non-enzymatic processes such as the suppression of NADH oxidation, which decreases metabolism and leads to the subsequent death of the microorganism (Schmidt et al., 2010; Kabu and Akosman, 2013).
In the present study, the solid CUR/PVP at a 1:9 ratio (CUR/PVP) prepared and tested at 1% (10 g/Kg of feed) showed a significant reduction in SE by a 2.25 log10 when compared to the control non-treated group (Table 4). This result is in contrast with our previous results, where 1% CUR without PVP had no in vitro antimicrobial activity against SE. These results are justified given the increase in solubility and permeability of CUR when it is associated with PVP as displayed in the in vitro studies (Tables 2, 3). The increase in solubility might be due to the formation of soluble complexes between CUR and PVP (Kaewnopparat et al., 2009). Furthermore, the combination of 0.5% BA with 0.5% CUR/PVP confirmed the synergistic antimicrobial effect described in previous publications (Hernandez-Patlan et al., 2017).
This synergistic effect was more evident in the in vivo trials where administration of 0.05% CUR/PVP with 0.05% BA significantly reduced the colonization of SE in both the crop and CCT compared to the control non-treated chickens (Table 5). Previous studies had demonstrated the synergistic effect between CUR and BA given their interaction to form more stable complexes such as rosocyanine and rubrocurcumin (Halim et al., 2012; Wanninger et al., 2015; Hernandez-Patlan et al., 2017). These types of complexes modify the properties of CUR, which could be related to the improvement in antimicrobial activity (Priyadarsini, 2014). Interestingly, 0.1% CUR/PVP was also effective in reducing SE colonization, but this reduction occurred only in CCT in both in vivo experiments (Table 5). This is likely due to the solubility of CUR/PVP (1: 9 ratio), which is almost two times higher at pH 6.4 than at 1.2 (Table 2) and also due to the longer transit time in the intestine than in the crop. Several studies have shown that the solubility of CUR increases more than 25-fold at pH 6.8 when it is compared with its solubility at pH 1.2 (Song et al., 2016). It is known that at physiological pH, CUR alone rapidly degrades forming products such as bicyclopentadione, vanillin, and ferulic acid (Kharat et al., 2017).
According to our results, it is likely that the use of a solid CUR/PVP (1:9 ratio) improves the stability of CUR at alkaline pH and thus avoid its degradation. It is known that one of the most critical properties of CUR besides the antioxidant effect is its antimicrobial effect (Samarasinghe et al., 2003; Khan et al., 2012; Moghadamtousi et al., 2014). Some studies have demonstrated that the antibacterial activity of CUR is due to the inhibition of bacterial cell proliferation (Rai et al., 2008) or affecting virulence, quorum sensing, and biofilm initiation (Rudrappa and Bais, 2008). Nevertheless, it is clear that the antimicrobial mechanism of action of CUR is related to the membrane damage of prokaryotes (Tyagi et al., 2015). In contrast, in both in vivo trials, BA failed to significantly reduce SE colonization in crop and CCT (Table 5).
Gut integrity involves a close relationship between the microbiome, the enteric nervous system and the mucosal immunity (Gilani et al., 2016). Biological, chemical, physical, or environmental stresses can alter the gut microbiome (dysbiosis), increasing the permeability to toxins and stimulate an inflammatory response (Oz, 2017). Under healthy conditions, pathogens cannot cross the epithelial barrier, but when microbial infection occurs, pathogens can interact and damage the epithelial barrier, causing an increase in intestinal permeability (Gilani et al., 2016). Gut leakage can be measured using FITC-d as a marker of intestinal integrity (Vicuña et al., 2015b). In the present study, the group treated with CUR/PVP significantly decreased serum FITC-d levels when compared to the control non-treated group. The reduction in serum FITC-d levels could be due to the control of SE by 0.1% CUR/PVP and the capacity of CUR to increase the intestinal epithelial cell proliferation/regeneration (Bereswill et al., 2010). However, there was no reduction of serum FITC-d when 0.05% CUR/PVP is associated with 0.05% BA (CUR/PVP-BA), indicating that CUR/PVP should not be associated with BA to allow for cell regeneration.
The intestinal mucosa releases anti-microbial proteins and IgA. IgA is an antibody isotype specialized in protecting the intestinal mucosa, as well as inhibiting inflammatory processes, neutralizing bacterial toxins, and enhancing nonspecific defense mechanisms (e.g., lactoperoxidase and lactoferrin) (Gutzeit et al., 2014; Merino-Guzmán et al., 2017). Since the secretory IgA provides the first line of defense by preventing pathogen entry into the mucosa (Neutra and Kozlowski, 2006), its concentration was measured in a segment of intestine (Table 6). In the present study, control non-treated chickens showed the highest IgA levels. This is likely due to SE ability to induce a strong local inflammatory response in an intestinal tissue, leading to increase of pro-inflammatory cytokines secretion (Steinberg et al., 2014) which stimulates the production of IgA, antimicrobial peptides, and promote epithelial repair (Patel and McCormick, 2014). The decrease of IgA levels in the group treated with CUR/PVP is related to the anti-inflammatory properties of CUR (Gupta et al., 2012). Therefore, these results can be correlated with the low serum FITC-d levels since the mucosal inflammation process was less severe. Although there are no significant differences in the levels of IgA in the groups treated with BA, and CUR/PVP-BA, the slight decrease observed could have been because these treatments decreased the concentration of SE, as well as, the inflammatory response by regulation of enzymes (Hunt, 1996). SOD is an enzyme involved in shielding cells from the harmful reactive oxygen species (ROS) produced in response to an inflammatory process. It is known that CUR can induce the expression of cytoprotective proteins such as SOD. Furthermore, CUR is also able to react directly with ROS and reactive nitrogen species (RNS) to scavenge superoxide anions, hydroxyl radicals, H2O2, singlet oxygen, nitric oxide, peroxynitrite and peroxyl radicals (Trujillo et al., 2013; Kant et al., 2014). Chickens that received 0.1% CUR/PVP had significantly higher SOD activity. However, in the group treated with CUR/PVP-BA the activity of SOD decreased compared to the control non-treated chickens. Probably, the complexes formed between CUR/PVP and BA were not able to stimulate the expression of SOD, and it is evident that the decrease in SOD activity in this group could be due to the control of SE.
In the present study, no significant differences in SOD activity between the 0.1% BA group and control non-treated chickens were observed, although BA decreased the concentration of SE more than 1 Log10. Some studies have shown that boron supplementation increases the activity of SOD (Pizzorno, 2015; Bhasker et al., 2016). However, the mechanism by which the enzymatic activity is increased is not clear. Boron may also be promoting thymocyte proliferation while inhibiting apoptosis via enhancing the antioxidative function of the thymus (Jin et al., 2017).
The results suggest that the increase in the solubility and stability of CUR when it is associated with PVP could be involved in the improvement of the antimicrobial activity of CUR against SE both in the in vitro digestion model and in the two independent in vivo trials. In addition, it was confirmed in the in vivo trials that the combination between CUR/PVP and BA have a synergistic effect since the antimicrobial effect against SE intestinal colonization was similar to CUR/PVP but better than BA, considering that the proportion of each of them was 50% (0.05% CUR/PVP and 0.05% BA). On the other hand, 0.1% CUR/PVP was able to reduce the intestinal permeability of FITC-d and total intestinal IgA, as well as increase the activity of SOD when compared to control, while, BA-CUR/PVP only decreased SOD activity. Further studies to confirm and expand the in vivo results obtained in this pilot study, adding intestinal microbial commensal groups and more inflammatory biomarkers to get a complete description of the effects of BA and CUR deserves further investigation.
Author Contributions
DH-P and GT conceived, designed, and drafted the manuscript. JL, XH-V, and GT drafted the article or revised it critically for valuable intellectual content. DH-P, BS-C, KP, JL, MB, RM-G, and AM-A acquired the data. BH, RL-A, DH-P, and GT analyzed and interpreted the data.
Funding
This research was supported by the Arkansas Bioscience Institute under the project: “Development of an Avian Model for Evaluation Early Enteric Microbial Colonization on the Gastrointestinal Tract and Immune Function.”
Conflict of Interest Statement
The authors declare that the research was conducted in the absence of any commercial or financial relationships that could be construed as a potential conflict of interest.
Acknowledgments
The authors thank the CONACyT for the doctoral scholarship number 447447 and the financial support obtained through the program PAPIIT IN218115 of DGAPA-UNAM.
Abbreviations
BA, boric acid; BA-CUR/PVP, combination of 0.5% BA and 0.5% CUR/PVP; CCT, ceca-cecal tonsils; CUR, curcumin; CUR/PVP, dispersion of CUR with PVP; FITC-d, fluorescein isothiocyanate–dextran; PVP, polyvinylpyrrolidone; SE, solid Salmonella enterica serovar Enteritidis; SOD, superoxide dismutase.
References
Allos, B. M., Moore, M. R., Griffin, P. M., and Tauxe, R. V. (2004). Surveillance for sporadic foodborne disease in the 21st century: the FoodNet perspective. Clin. Infect. Dis. 38(Suppl. 3), S115–S120. doi: 10.1086/381577
Andreatti Filho, R. L., Higgins, J. P., Higgins, S. E., Gaona, G., Wolfenden, A. D., Tellez, G., et al. (2007). Ability of bacteriophages isolated from different sources to reduce Salmonella enterica serovar Enteritidis in vitro and in vivo. Poult. Sci. 86, 1904–1909. doi: 10.1093/ps/86.9.1904
Baxter, M. F. A., Merino-Guzman, R., Latorre, J. D., Mahaffey, B. D., Yang, Y., Teague, K. D., et al. (2017). Optimizing fluorescein isothiocyanate dextran measurement as a biomarker in a 24-h feed restriction model to induce gut permeability in broiler chickens. Front. Vet. Sci. 4:56. doi: 10.3389/fvets.2017.00056
Bereswill, S., Muñoz, M., Fischer, A., Plickert, R., Haag, L. M., Otto, B., et al. (2010). Anti-Inflammatory effects of resveratrol, curcumin and simvastatin in acute small intestinal inflammation. PLoS One 5:e15099. doi: 10.1371/journal.pone.0015099
Bertelloni, F., Tosi, G., Massi, P., Fiorentini, L., Parigi, M., Cerri, D., et al. (2017). Some pathogenic characters of paratyphoid Salmonella enterica strains isolated from poultry. Asian Pac. J. Trop. Med. 10, 1161–1166. doi: 10.1016/j.apjtm.2017.10.023
Bhasker, T. V., Gowda, N. K. S., Mondal, S., Krishnamoorthy, P., Pal, D. T., Mor, A., et al. (2016). Boron influences immune and antioxidant responses by modulating hepatic superoxide dismutase activity under calcium deficit abiotic stress in Wistar rats. J. Trace Elem. Med. Biol. 36, 73–79. doi: 10.1016/j.jtemb.2016.04.007
Cobb-Vantress, Inc. (2015). Cobb 500 Broiler Performance and Nutrition Supplement. Available at: https://cobbstorage.blob.core.windows.net/guides/3914ccf0-6500-11e8-9602-256ac3ce03b1
Gilani, S., Howarth, G. S., Kitessa, S. M., Forder, R. E. A., Tran, C. D., and Hughes, R. J. (2016). New biomarkers for intestinal permeability induced by lipopolysaccharide in chickens. Anim. Prod. Sci. 56, 1984–1997. doi: 10.1071/AN15725
Gupta, S. C., Patchva, S., Koh, W., and Aggarwal, B. B. (2012). Discovery of curcumin, a component of golden spice, and its miraculous biological activities. Clin. Exp. Pharmacol. Physiol. 39, 283–299. doi: 10.1111/j.1440-1681.2011.05648.x
Gutzeit, C., Magri, G., and Cerutti, A. (2014). Intestinal IgA production and its role in host-microbe interaction. Immunol. Rev. 260, 76–85. doi: 10.1111/imr.12189
Haaf, F., Sanner, A., and Straub, F. (1985). Polymers of N-vinylpyrrolidone: synthesis, characterization and uses. Polym. J. 17, 143–152. doi: 10.1295/polymj.17.143
Hailu, G., Boecker, A., Henson, S., and Cranfield, J. (2009). Consumer valuation of functional foods and nutraceuticals in Canada. A conjoint study using probiotics. Appetite 52, 257–265. doi: 10.1016/j.appet.2008.10.002
Halim, A. A., Bakar, A. F. A., Hanafiah, M. A. K. M., and Zakaria, H. (2012). Boron removal from aqueous solutions using curcumin-aided electrocoagulation. Middle East J. Sci. Res. 11, 583–588.
Hernandez-Patlan, D., Solis-Cruz, B., Méndez-Albores, A., Latorre, J. D., Hernandez-Velasco, X., Tellez, G., et al. (2017). Comparison of PrestoBlue® and plating method to evaluate antimicrobial activity of ascorbic acid, boric acid and curcumin in an in vitro gastrointestinal model. J. Appl. Microbiol. 124, 423–430. doi: 10.1111/jam.13659
Hunt, C. D. (1994). The biochemical effects of physiologic amounts of dietary boron in animal nutrition models. Environ. Health Perspect. 102, 35–43. doi: 10.1289/ehp.94102s735
Hunt, C. D. (1996). Main content area Biochemical effects of physiological amounts of dietary boron. J. Trace Elem. Exp. Med. 9, 185–213. doi: 10.1002/(SICI)1520-670X(1996)9:4<185::AID-JTRA6>3.0.CO;2-Q
Hunt, C. D. (2003). Dietary boron: an overview of the evidence for its role in immune function. J. Trace Elem. Exp. Med. 16, 291–306. doi: 10.1002/jtra.10041
Huyghebaert, G., Ducatelle, R., and Van Immerseel, F. (2011). An update on alternatives to antimicrobial growth promoters for broilers. Vet. J. 187, 182–188. doi: 10.1016/j.tvjl.2010.03.003
Jin, E., Ren, M., Liu, W., Liang, S., Hu, Q., Gu, Y., et al. (2017). Effect of Boron on thymic cytokine expression, hormone secretion, antioxidant functions, cell proliferation, and apoptosis potential via the extracellular signal-regulated kinases 1 and 2 signaling pathway. J. Agric. Food Chem. 65, 11280–11291. doi: 10.1021/acs.jafc.7b04069
Kabu, M., and Akosman, M. S. (2013). “Biological effects of Boron BT,” in Reviews of Environmental Contamination and Toxicology, ed. D. M. Whitacre (New York, NY: Springer), 57–75. doi: 10.1007/978-1-4614-6470-9_2
Kaewnopparat, N., Kaewnopparat, S., Jangwang, A., Maneenaun, D., Chuchome, T., and Panichayupakaranant, P. (2009). Increased solubility, dissolution and physicochemical studies of curcumin-polyvinylpyrrolidone K-30 solid dispersions. World Acad. Sci. Eng. Technol. 55, 229–234.
Kant, V., Gopal, A., Pathak, N. N., Kumar, P., Tandan, S. K., and Kumar, D. (2014). Antioxidant and anti-inflammatory potential of curcumin accelerated the cutaneous wound healing in streptozotocin-induced diabetic rats. Int. Immunopharmacol. 20, 322–330. doi: 10.1016/j.intimp.2014.03.009
Khan, R. U., Naz, S., Javdani, M., Nikousefat, Z., Selvaggi, M., Tufarelli, V., et al. (2012). The use of Turmeric (Curcuma longa) in poultry feed. Worlds Poult. Sci. J. 68, 97–103. doi: 10.1017/S0043933912000104
Kharat, M., Du, Z., Zhang, G., and McClements, D. J. (2017). Physical and chemical stability of curcumin in aqueous solutions and emulsions: impact of pH, temperature, and molecular environment. J. Agric. Food Chem. 65, 1525–1532. doi: 10.1021/acs.jafc.6b04815
Kuttappan, V. A., Berghman, L. R., Vicuña, E. A., Latorre, J. D., Menconi, A., Wolchok, J. D., et al. (2015). Poultry enteric inflammation model with dextran sodium sulfate mediated chemical induction and feed restriction in broilers. Poult. Sci. 94, 1220–1226. doi: 10.3382/ps/pev114
Latorre, J. D., Hernandez-Velasco, X., Kuttappan, V. A., Wolfenden, R. E., Vicente, J. L., Wolfenden, A. D., et al. (2015). Selection of Bacillus spp. for cellulase and xylanase production as direct-fed microbials to reduce digesta viscosity and Clostridium perfringens proliferation using an in vitro digestive model in different poultry diets. Front. Vet. Sci. 2:25. doi: 10.3389/fvets.2015.00025
Lawhavinit, O., Kongkathip, N., and Kongkathip, B. (2010). Antimicrobial activity of curcuminoids from Curcuma longa L. on pathogenic bacteria of shrimp and chicken. Kasetsart J. (Nat. Sci.) 44, 364–371.
Lin, J., Lee, I. S., Frey, J., Slonczewski, J. L., and Foster, J. W. (1995). Comparative analysis of extreme acid survival in Salmonella typhimurium, Shigella flexneri, and Escherichia coli. J. Bacteriol. 177, 4097–4104. doi: 10.1128/jb.177.14.4097-4104.1995
Mani-López, E., García, H. S., and López-Malo, A. (2012). Organic acids as antimicrobials to control Salmonella in meat and poultry products. Food Res. Int. 45, 713–721. doi: 10.1016/j.foodres.2011.04.043
Mead, P. S., Slutsker, L., Dietz, V., McCaig, L. F., Bresee, J. S., Shapiro, C., et al. (1999). Food-related illness and death in the United States. Emerg. Infect. Dis. 5, 607–625. doi: 10.3201/eid0505.990502
Menconi, A., Wolfenden, A. D., Shivaramaiah, S., Terraes, J. C., Urbano, T., Kuttel, J., et al. (2011). Effect of lactic acid bacteria probiotic culture for the treatment of Salmonella enterica serovar Heidelberg in neonatal broiler chickens and turkey poults. Poult. Sci. 90, 561–565. doi: 10.3382/ps.2010-01220
Merino-Guzmán, R., Latorre, J. D., Delgado, R., Hernandez-Velasco, X., Wolfenden, A. D., Teague, K. D., et al. (2017). Comparison of total immunoglobulin A levels in different samples in Leghorn and broiler chickens. Asian Pac. J. Trop. Biomed. 7, 116–120. doi: 10.1016/j.apjtb.2016.11.021
Moghadamtousi, S. Z., Kadir, H. A., Hassandarvish, P., Tajik, H., Abubakar, S., and Zandi, K. (2014). A review on antibacterial, antiviral, and antifungal activity of curcumin. Biomed Res. Int. 2014:186864. doi: 10.1155/2014/186864
National Research Council (1994). Nutrient Requirements of Poultry, 9th Rev. Edn. Washington, DC: National Academy Press.
Neutra, M. R., and Kozlowski, P. A. (2006). Mucosal vaccines: the promise and the challenge. Nat. Rev. Immunol. 6, 148–158. doi: 10.1038/nri1777
Oz, H. S. (2017). Nutrients, infectious and inflammatory diseases. Nutrients 9:E1085. doi: 10.3390/nu9101085
Patel, S., and McCormick, B. A. (2014). Mucosal inflammatory response to Salmonella typhimurium infection. Front. Immunol. 5:311. doi: 10.3389/fimmu.2014.00311
Priyadarsini, I. K. (2014). The chemistry of curcumin: from extraction to therapeutic agent. Molecules 19, 20091–20112. doi: 10.3390/molecules191220091
Rai, D., Singh, J. K., Roy, N., and Panda, D. (2008). Curcumin inhibits FtsZ assembly: an attractive mechanism for its antibacterial activity. Biochem. J. 410, 147–155. doi: 10.1042/BJ20070891
Rajput, N., Muhammad, N., Yan, R., Zhong, X., and Wang, T. (2013). Effect of dietary supplementation of curcumin on growth performance, intestinal morphology and nutrients utilization of broiler chicks. J. Poult. Sci. 50, 44–52. doi: 10.2141/jpsa.0120065
Rudrappa, T., and Bais, H. P. (2008). Curcumin, a known phenolic from Curcuma longa, attenuates the virulence of Pseudomonas aeruginosa PAO1 in whole plant and animal pathogenicity models. J. Agric. Food Chem. 56, 1955–1962. doi: 10.1021/jf072591j
Samad, M. A., Hashim, S. H., Simarani, K., and Yaacob, J. S. (2016). Antibacterial properties and effects of fruit chilling and extract storage on antioxidant activity, total phenolic and anthocyanin content of four date palm (Phoenix dactylifera) cultivars. Molecules 21:419. doi: 10.3390/molecules21040419
Samarasinghe, K., Wenk, C., Silva, K., and Gunasekera, J. (2003). Turmeric (Curcuma longa) root powder and mannanoligosaccharides as alternatives to antibiotics in broiler chicken diets. ASIAN Australas. J. Anim. Sci. 16, 1495–1500. doi: 10.5713/ajas.2003.1495
Scharff, R. L. (2012). Economic burden from health losses due to foodborne illness in the United States. J. Food Prot. 75, 123–131. doi: 10.4315/0362-028X.JFP-11-058
Schmidt, M., Schaumberg, J. Z., Steen, C. M., and Boyer, M. P. (2010). Boric acid disturbs cell wall synthesis in Saccharomyces cerevisiae. Int. J. Microbiol. 2010:930465. doi: 10.1155/2010/930465
Skjånes, K., Rebours, C., and Lindblad, P. (2013). Potential for green microalgae to produce hydrogen, pharmaceuticals and other high value products in a combined process. Crit. Rev. Biotechnol. 33, 172–215. doi: 10.3109/07388551.2012.681625
Song, I. S., Cha, J. S., and Choi, M. K. (2016). Characterization, in vivo and in vitro evaluation of solid dispersion of curcumin containing d-α-Tocopheryl polyethylene glycol 1000 succinate and mannitol. Molecules 21:E1386. doi: 10.3390/molecules21101386
Steinberg, R. S., Silva, L. C. S., Souza, T. C., Lima, M. T., de Oliveira, N. L. G., Vieira, L. Q., et al. (2014). Safety and Protective effectiveness of two strains of Lactobacillus with probiotic features in an experimental model of salmonellosis. Int. J. Environ. Res. Public Health 11, 8755–8776. doi: 10.3390/ijerph110908755
Subbiah, M. T. R. (2007). Nutrigenetics and nutraceuticals: the next wave riding on personalized medicine. Transl. Res. 149, 55–61. doi: 10.1016/j.trsl.2006.09.003
Trujillo, J., Chirino, Y. I., Molina-Jijón, E., Andérica-Romero, A. C., Tapia, E., and Pedraza-Chaverrí, J. (2013). Renoprotective effect of the antioxidant curcumin: recent findings. Redox Biol. 1, 448–456. doi: 10.1016/j.redox.2013.09.003
Tyagi, P., Singh, M., Kumari, H., Kumari, A., and Mukhopadhyay, K. (2015). Bactericidal activity of curcumin I is associated with damaging of bacterial membrane. PLoS One 10:e0121313. doi: 10.1371/journal.pone.0121313
Vicuña, E. A., Kuttappan, V. A., Galarza-Seeber, R., Latorre, J. D., Faulkner, O. B., Hargis, B. M., et al. (2015a). Effect of dexamethasone in feed on intestinal permeability, differential white blood cell counts, and immune organs in broiler chicks. Poult. Sci. 94, 2075–2080. doi: 10.3382/ps/pev211
Vicuña, E. A., Kuttappan, V. A., Tellez, G., Hernandez-Velasco, X., Seeber-Galarza, R., Latorre, J. D., et al. (2015b). Dose titration of FITC-d for optimal measurement of enteric inflammation in broiler chicks. Poult. Sci. 94, 1353–1359. doi: 10.3382/ps/pev111
Wanninger, S., Lorenz, V., Subhan, A., and Edelmann, F. T. (2015). Metal complexes of curcumin – synthetic strategies, structures and medicinal applications. Chem. Soc. Rev. 44, 4986–5002. doi: 10.1039/C5CS00088B
WHO (2018). Salmonella (Non-typhoidal). Fact Sheets. Reviewed January 2018. Food Safety Department. Geneva: World Health Organization.
Yan, Y., Kolachala, V., Dalmasso, G., Nguyen, H., Laroui, H., Sitaraman, S. V., et al. (2009). Temporal and spatial analysis of clinical and molecular parameters in dextran sodium sulfate induced colitis. PLoS One 4:e6073. doi: 10.1371/journal.pone.0006073
Keywords: boric acid, chickens, curcumin, polyvinylpyrrolidone, Salmonella
Citation: Hernandez-Patlan D, Solis-Cruz B, Pontin KP, Latorre JD, Baxter MFA, Hernandez-Velasco X, Merino-Guzman R, Méndez-Albores A, Hargis BM, Lopez-Arellano R and Tellez G (2018) Evaluation of a Solid Dispersion of Curcumin With Polyvinylpyrrolidone and Boric Acid Against Salmonella Enteritidis Infection and Intestinal Permeability in Broiler Chickens: A Pilot Study. Front. Microbiol. 9:1289. doi: 10.3389/fmicb.2018.01289
Received: 03 April 2018; Accepted: 28 May 2018;
Published: 15 June 2018.
Edited by:
George Grant, University of Aberdeen, United KingdomReviewed by:
Luis A. Rubio, Estación Experimental del Zaidín (EEZ), SpainAlaeldein M. Abudabos, King Saud University, Saudi Arabia
Copyright © 2018 Hernandez-Patlan, Solis-Cruz, Pontin, Latorre, Baxter, Hernandez-Velasco, Merino-Guzman, Méndez-Albores, Hargis, Lopez-Arellano and Tellez. This is an open-access article distributed under the terms of the Creative Commons Attribution License (CC BY). The use, distribution or reproduction in other forums is permitted, provided the original author(s) and the copyright owner are credited and that the original publication in this journal is cited, in accordance with accepted academic practice. No use, distribution or reproduction is permitted which does not comply with these terms.
*Correspondence: Guillermo Tellez, Z3RlbGxlekB1YXJrLmVkdQ==