- 1Clinical Microbiology and Molecular Pathology, Department of Translational Medicine, Faculty of Medicine, Lund University, Malmö, Sweden
- 2Department of Medical Biochemistry and Biophysics, Umeå University, Umeå, Sweden
Outer membrane vesicles (OMVs) play an important role in the persistence of Helicobacter pylori infection. Helicobacter OMVs carry a plethora of virulence factors, including catalase (KatA), an antioxidant enzyme that counteracts the host respiratory burst. We found KatA to be enriched and surface-associated in OMVs compared to bacterial cells. This conferred OMV-dependent KatA activity resulting in neutralization of H2O2 and NaClO, and rescue of surrounding bacteria from oxidative damage. The antioxidant activity of OMVs was abolished by deletion of KatA. In conclusion, enrichment of antioxidative KatA in OMVs is highly important for efficient immune evasion.
Introduction
Helicobacter pylori is a Gram-negative pathogen that commonly colonizes the gastric mucosa. Infection persists for a lifetime without antibiotic treatment although the pathogen constantly experiences hostile conditions including the acidic ventricle environment and host defense (Roesler et al., 2014). In order to survive against the highly acidic gastric juice (pH 1.0–3.0), H. pylori uses a series of acidic acclimation systems that neutralize the surrounding acid. Other virulence mechanisms include expression of abundant molecules at the surface for attachment and manipulation of host extracellular matrix proteins and serum resistance (Parker and Keenan, 2012; Richter et al., 2016). In addition, H. pylori is equipped with antioxidant molecules such as catalase (KatA), catalase-like protein (KatB), alkyl hydroperoxide reductase (AhpC), and superoxide dismutase (SOD) to detoxify reactive oxygen species (ROS) released from host immune cells during the respiratory burst (Wang et al., 2006). Furthermore, H. pylori constitutively releases outer membrane vesicles (OMVs) from its outer membrane (OM).
Outer membrane vesicles are cargos comprising an OM lipid bilayer enveloping several virulence factors. H. pylori OMVs have been extensively studied with respect to composition, proteome, and virulence functions (Mullaney et al., 2009; Olofsson et al., 2010), and play multiple roles in bacterial pathogenesis including biofilm formation, cancer development, and immune evasion (Parker and Keenan, 2012). Furthermore, OMVs display immunomudulatory effects by inducing IL-8 secretion from epithelial cells, activating phagoctyes, and suppressing immune cells of the adaptive immune system (Mullaney et al., 2009; Olofsson et al., 2010; Ko et al., 2016).
KatA, a 55 kDa catalase, is an essential virulence factor protecting H. pylori against the respiratory burst (Olofsson et al., 2010). In fact, KatA is upregulated during oxidative stress (Huang and Chiou, 2011). It is widely known that KatA detoxifies hydrogen peroxide (H2O2) and hypochlorite (OCl-) (Benoit and Maier, 2016). Additionally, we recently reported that KatA mediates vitronectin acquisition resulting in increased serum resistance (Richter et al., 2016). Interestingly, despite the lack of a signal peptide, Helicobacter KatA is ubiquitous with various topology including the bacterial surface, the cytosol and periplasmic space. KatA has also recently been identified in OMVs (Wang et al., 2006; Mullaney et al., 2009). However, little is known regarding the role of KatA in OMVs since previous studies have mainly focused on KatA in the cell-associated context.
We determined the importance of OMVs to eliminate extracellular ROS-mediated killing via KatA enrichment. Our data suggest a new mechanism of OMV-mediated H. pylori evasion from the attack of the innate immune system.
Results
KatA Catalase Is Enriched in H. pylori-Derived OMVs
Helicobacter pylori KatA has been predicted as one of the periplasmic proteins that accounts for 7.4% of the total OMV proteome (Mullaney et al., 2009; Olofsson et al., 2010). Since most OM proteins are also located at the surface of vesicles (Bonnington and Kuehn, 2014), we wanted to investigate whether KatA localizes at the outer surface of H. pylori OMVs. As visualized by TEM, we found deposition of gold-labeled anti-KatA pAb at the surface of intact bacteria and OMVs of H. pylori wild type (wt) (Figure 1A). However, no KatA was detected on any samples derived from the KatA-deficient H. pylori ΔkatA mutant. This suggested a similar surface exposure of KatA on OMVs as seen on intact bacteria. Further enumeration of antibody deposition revealed that more KatA was detected at the “blebbing areas” of wild type bacteria as compared to the “non-blebbing areas,” and this appearance was almost similar to the OMVs (Figure 1B). This observation prompted our interest to compare the amount of KatA present in the OMVs and OM of H. pylori. Interestingly, we observed that OMVs contained sevenfold more KatA (18.37 ± 6.24 ng/μg sample) than bacterial OM (2.42 ± 0.24 ng/μg sample) (Figure 1C and Supplementary Figure S1).
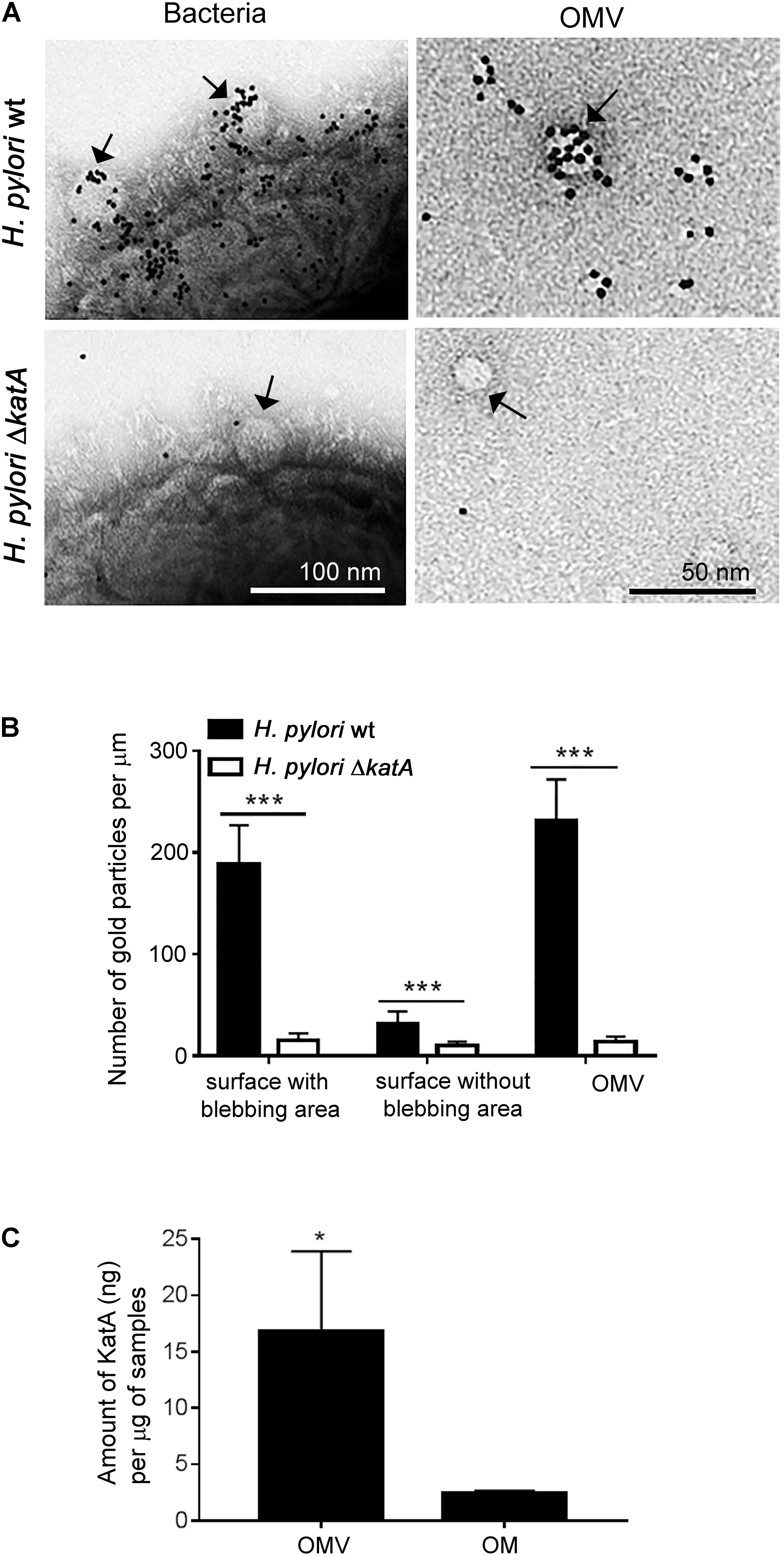
FIGURE 1. Characterization of KatA enrichment and deposition at the surface of Helicobacter outer membrane vesicles (OMVs). (A) Detection of KatA at the surface of OMVs by immunoelectron microscopy using gold conjugated rabbit anti-KatA IgG pAb (black particles) at the outer surface of intact H. pylori 18943 wt (upper panel) or at the outer surface of the KatA-deficient mutant H. pylori 18943ΔkatA (lower panel). Arrows indicate OMVs that are vesiculating from the “blebbing area” at the bacterial surface (left panels) or purified OMVs (right panels). Visualization by Transmission Electron Microscopy (TEM) was performed on a Philips/FEICM 100 TWIN transmission electron microscope, and images were documented with a side-mounted Olympus Veleta camera having a resolution of 2048 × 2048 pixels (2k × 2K) and ITEM acquisitions software. (B) KatA is accumulated in OMVs at the bacterial surface, and in the released OMV fraction. The number of anti-KatA IgG pAb-gold particles per μm from 50 randomly selected TEM image profiles were calculated, and corresponded to at least 1000 different bacteria. (C) A significantly higher concentration of KatA is present in OMVs compared to the OM fraction. Estimation of KatA concentrations in OM and OMVs was done by western blotting as shown in Supplementary Figure S1. For (B) and (C), statistical differences were calculated by two-way ANOVA and two-tailed Student’s t-test, respectively (mean ± SD; n = 3; ∗p < 0.05; ∗∗∗p < 0.001).
KatA Enriched OMVs Exhibit Catalase Activity
Helicobacter KatA of intact bacteria is known to actively hydrolyse H2O2 and detoxify ClO- (Wang et al., 2006; Benoit and Maier, 2016). Interestingly, we found that the H2O2 hydrolysis activity in OMVs was significantly (p < 0.05) higher than the H. pylori wt whole cell lysate (Figure 2A). We further investigated whether KatA could contribute to the antioxidant activity of Helicobacter OMV. As shown in Figure 2B, OMVs isolated from the strain H. pylori 18943 wt exhibited a strong catalase activity based upon hydrolysis of H2O2 compared to OMVs of the H. pylori 18943ΔkatA mutant that had an abolished KatA activity. In parallel, a similar H2O2 hydrolysis activity was also found with OMVs isolated from another H. pylori strain (P12), whereas no activity was observed with the corresponding KatA-deficient H. pylori P12 mutant.
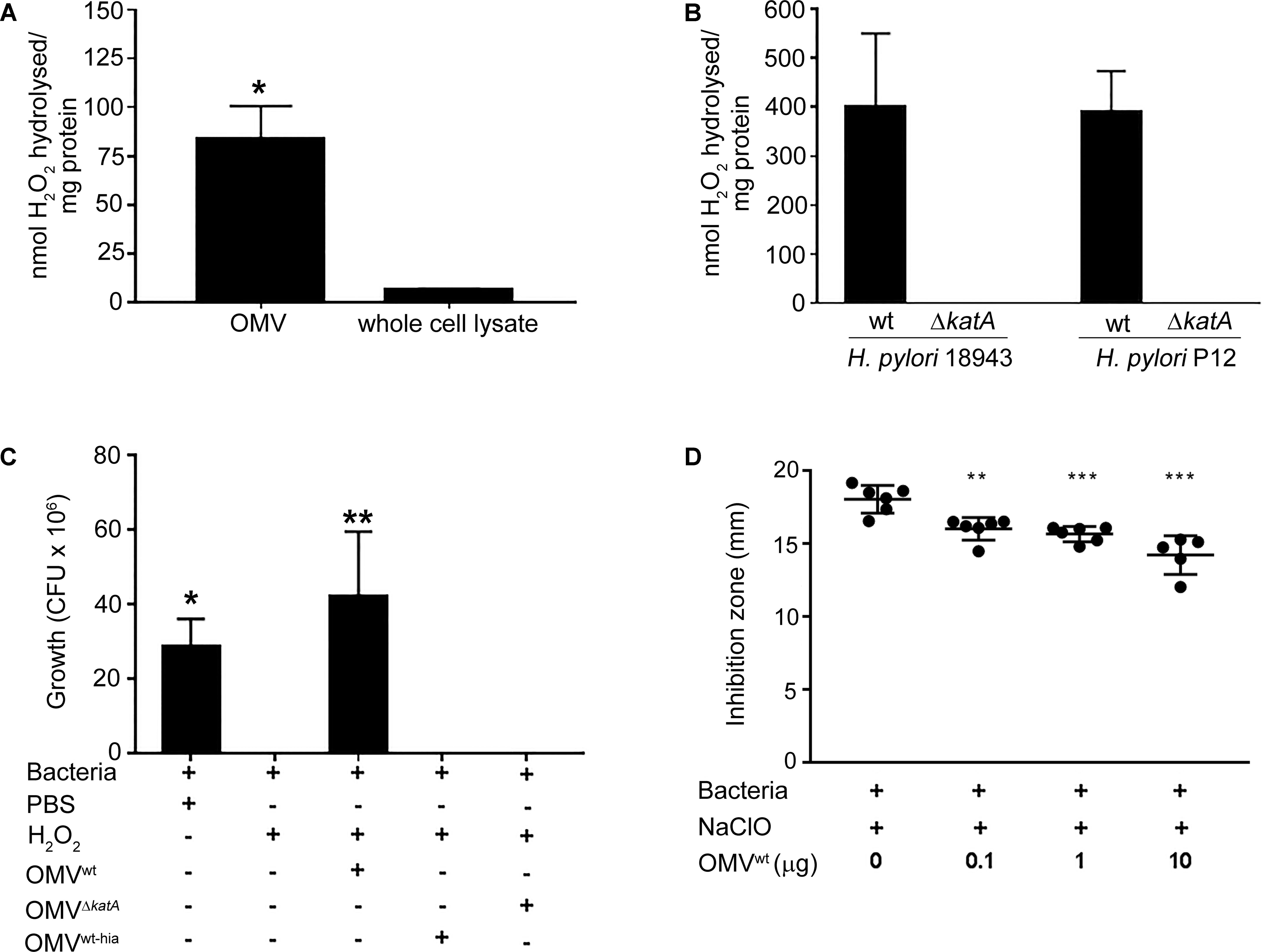
FIGURE 2. OMVs carrying KatA hydrolyse/detoxify ROS and provide protection against ROS-mediated bacterial killing. (A) Comparison of catalase activity between OMVs derived from H. pylori 18943 wt and a bacterial lysate. An increased H2O2 hydrolysis on a weight basis was detected with OMVs compared to bacterial lysate. (B) Determination of KatA-dependent catalase activity in OMVs. Deletion of KatA in two different strains of H. pylori, 18943 and P12 resulted in abolished catalase activity in OMVs from H. pyloriΔkatA mutant strains compared to OMVs derived from the wild type counterparts. In (A) and (B), catalase activity was presented as nmol of H2O2 decomposed per mg of sample tested (mean ± SD; n = 3; ∗p < 0.05; ∗∗p < 0.01). (C) and (D), Helicobacter OMVs reduce the bactericidal activity of H2O2 and NaClO, and thus promoted bacterial survival. In (C), H. pylori P12ΔkatA devoid of catalase activity was challenged with 1 mM H2O2 preincubated with 40 μg/ml of OMV (equivalent to OMVs produced from 109 CFU) (OMVwt, OMVΔkatA or heat-inactivated OMVs; OMVhia). In (D), H. pylori 18943ΔkatA devoid of KatA expression was exposed to 5% NaOCl that had been pre-treated with 0.1–10.0 μg of OMVwt. Pure H2O2 and NaOCl in PBS that were fully bactericidal were included as a positive control. In (C), the viability of bacteria was assessed by plating and counting colony forming units (CFU). Only KatA-containing OMVwt was able to neutralize H2O2 and promote survival of H. pylori P12ΔkatA from peroxidative killing. OMV lacking KatA (OMVΔkatA) or heat-inactived KatA (OMVhia) did not show any protection of whole bacteria (mean ± SD; n = 3; ∗p < 0.05; ∗∗p < 0.01). In (D), the bactericidal activity of NaOCl was decreased by preincubation with increasing amounts of OMVwt (equivalent to OMVs produced from 0.2 to 2 × 108 CFU). The killing of H. pylori 18943ΔkatA by NaOCl was measured by the diameter of inhibition zone, which is the area without bacterial growth (mean ± SD; n = 3; ∗∗p < 0.01; ∗∗∗p < 0.001).
KatA-Enriched OMVs Promote H. pylori Survival Against the ROS of the Oxidative Burst
We also wanted to determine whether OMV loaded with KatA could protect bacteria from the bactericidal activity of ROS (H2O2 and ClO-) (Wang et al., 2006; Benoit and Maier, 2016). First, H. pylori ΔkatA lacking the catalase activity was exposed to H2O2 that had been pre-incubated with OMVs derived from H. pylori wt (OMVwt) or the KatA-deficient mutant (OMVΔkatA). Interestingly, H. pylori ΔkatA survived when OMVwt-pre-treated H2O2 was added, but was completely killed in both H2O2 or H2O2 preincubated with OMVΔkatA (Figure 2C). Since KatA activity is heat sensitive, OMVwt was also heat-inactivated at 60°C to generate OMVwt-hia. We found that H2O2 preincubated with OMVwt-hia remained bactericidal against the mutant H. pylori ΔkatA.
We subsequently performed a disk diffusion assay to examine the capacity of OMVs in protecting H. pylori from the toxicity of NaClO. As shown in Figure 2D, the inhibition zone of H. pylori ΔkatA growth caused by NaClO was gradually reduced in response to increasing amounts (0.1–10 μg) of OMVwt used for preincubation with NaClO. Taken together, our data indicated that H. pylori OMVs exhibiting KatA-dependent catalase activity successfully neutralized both H2O2 and NaClO, and thus promoting bacterial survival when exposed to the bactericidal activity of ROS.
Discussion
Helicobacter pylori has evolved several virulence mechanisms for persistent colonization and infection in the gastric mucosa and this includes release of OMVs (Parker and Keenan, 2012). Here, we deciphered a novel role of OMVs in the pathogenesis of H. pylori; OMVs act as antioxidative particles via enrichment of KatA at the surface of vesicles. To the best of our knowledge, the current study is the first report regarding enrichment of KatA in H. pylori OMVs. Intriguingly, the H. pylori virulence factors OipA and HtrA have also been reported to be enriched in OMVs (Olofsson et al., 2010).
Production of toxic ROS, i.e., superoxide (O2∙-), nitrogen oxide (NO), H2O2, and OCl- by human polymorphonuclear cells (PMNs) during the respiratory burst is an important component of the innate defense to eradicate phagocytosed pathogens (Yang et al., 2013). Despite H. pylori infection induces massive influx of neutrophils into the gastric mucosa and production of ROS, the pathogen expresses KatA to survive at the surface of phagocytes, H. pylori is thus antiphagocytic and resistant against the respiratory burst-dependent killing (Ramarao et al., 2000; Wang et al., 2006). In contrast to bacterial cell-associated KatA, little is known regarding the KatA-dependent ROS resistance of OMVs.
We speculated that the accumulation of KatA in H. pylori OMVs, and thus higher catalase activity compared to bacterial cells (Figure 2A), may confer OMVs as an antioxidant cargo to protect bacteria from extracellular ROS of the respiratory burst. Bacterial interactions with PMNs result in an increase of extracellular H2O2 and ClO- release by neutrophils that is ineffective, however, to efficiently eradicate non-phagocytosed H. pylori (Ramarao et al., 2000; Allen et al., 2005). In addition to KatA, other antioxidant proteins such as KatB and AhpC are also present in the OMV proteome (Mullaney et al., 2009; Olofsson et al., 2010). However, we found that the catalase activity of OMVs is solely attributed to KatA accumulation since the ability to hydrolyse H2O2 was diminished in OMVs lacking KatA (Figure 2B). This could be due to the relatively low amount of KatB and AhpC compared to KatA in the H. pylori OMVs (Mullaney et al., 2009; Olofsson et al., 2010). Importantly, the KatA-dependent catalase activity of H. pylori OMVs is highly conserved among different strains (Figure 2B), further suggesting OMVs as important antioxidant particles.
In this study, we employed the direct H2O2 and NaClO bactericidal assay as an in vitro extracellular ROS respiratory burst model. Of note, H. pylori KatA counteracts the oxidative damage of H2O2 and OCl- via different mechanisms, which are through its catalase hydrolysis activity and oxidation of KatA methionine residues, respectively (Wang et al., 2006; Benoit and Maier, 2016). Our results demonstrated that H. pylori OMVs effectively neutralized ROS and rescued bacteria from lethal oxidative damage (Figures 2C,D).
The strategy to promote bacterial infection by virulence factor enrichment in OMVs has also been reported with other pathogens. Bacteroides spp. escapes from antibiotics by decorating their OMV surface with cephalosporinases (Stentz et al., 2015). Aggregatibacter actinomycetemcomitans utilizes OMVs enriched with leukotoxin to induce immune cell apoptosis (Bonnington and Kuehn, 2014). Our finding pioneered the idea of virulence factor enrichment in OMVs as a novel virulence mechanism of H. pylori. This is exemplified by KatA in OMVs that, in turn, contributes to the novel antioxidative role of H. pylori OMVs, and thus enhanced bacterial defense against host innate immune attacks. We speculate that, during infection in gastric mucosa, H. pylori releases OMVs enriched with KatA to decrease or depelete the surrounding extracellular ROS released from the oxidative burst of influxed PMNs. This will allow H. pylori to escape towards nearby infection sites with lower ROS, thereby facilitating bacterial survival and colonization. In conclusion, we have presented expanded insights on a novel potential virulence mechanism of H. pylori that provide additional knowledge regarding bacterial survival in a hostile PMN-rich environment.
Materials and Methods
Bacterial Strains and Growth Conditions
Bacterial strains and growth conditions are listed in Table 1.
Construction of H. pylori P12 ΔkatA Strain
Kata gene (Genbank Accession Number: CP001217; encodes KatA) deletion was performed as previously described (Richter et al., 2016). A linear katA-knockout construct containing cat (AY219687.1) was inserted between upstream and downstream flanking regions of the katA gene. Upstream flank was amplified from P12 gDNA using primers pair kat1F (5′-TCCCTTGAGCTGGTTGGCAATA-3′) and kat1R (5′-CTTAGCACTTGAGCCTAGAAGAGGCTGAGTACAGCATTG-3′). Primers kat2F (5′-CAATGGTGCCATGAATGGCAAAACCTCTTGGGTCTTTAC-3′) and kat2R (5′-CACCACAAGTAATTGGCCTAGTGTC-3′) were used to amplify downstream flank. Chloramphenicol resistance cassette was amplified from the gDNA of genomic DNA from strain J99sabB::cam using camF (5′-CAATGCTGTACTCAGCCTCTTC TAGGCTCAAGTGCTAAG-3′) and camR (5′-CGGTAAGAGACCCAAGAGGTT TTGCCATTCATGGCACCATTG-3′) primers. The construct containing a chloramphenicol resistance cassette and flanking regions was created by overlap extension PCR. For the overlap reaction of each PCR product were used as templates with primer pair kat1F and kat2R. All of PCR reactions were carried by GoTaq polymerase (Promega) or Phusion Hot start DNA polymerase (Thermo Scientific), and MJPTC-200 thermal cycler (MJ Research). The overlap PCR product was purified by E.Z.N.A Cycle Pure or Gel Extraction kits (OMEGA Bio-Tek, Norcross, GA, United States), prior to transformed into P12 wt. The mutant was verified by sequencing (Eurofin MWG, Ebersberg, Germany).
OM and OMVs Preparation
To isolate OMVs, culture supernatants were concentrated using Vivaflow200 (Sartorius, Goettingen, Germany) and centrifuged at 165,000 ×g (Olofsson et al., 2010). Pellets were separated by Histodenz (20–50%), and centrifugation at 200,000 ×g and resuspended in phosphate buffered saline (PBS). OM was prepared from bacteria as described (Voss et al., 2014).
Transmission Electron Microscopy (TEM)
The localization of KatA at the surface of intact bacteria and OMVs was determined by purified rabbit anti-KatA polyclonal antibodies (pAb) labeled with 5 nm colloidal thiocyanate gold followed by TEM using negative staining (Olofsson et al., 2010).
Estimation of KatA Concentrations and Catalase Enzymatic Assays
Recombinant KatA (rKatA), OM, or OMVs sample were separated by SDS-PAGE followed by immunoblotting using anti-KatA pAb (Supplementary Figure S1) (Richter et al., 2016). Signal intensities generated from known amounts of rKatA were included as a standard curve for KatA estimation. Analysis was done by Image Lab software (Bio-Rad, Copenhagen, Denmark).
Bacterial lysates or OMVs were incubated with 1 mM H2O2 in catalase buffer (50 mM Tris pH 7.4, 0.1% TritonX-100) for 30 min at room temperature. The reaction was terminated with 50 mM sodium azide, and residual H2O2 was detected by OxiRedTM (Biovision, Milpitas, CA, United States) and horseradish peroxidase (Thermoscientific, Waltham, MA, United States) mixture. Plates were read at 570 nm on a FLUOstar Omega microplate reader (BMG Labtech, Ortenberg, Germany).
H2O2 Bactericidal Assay
H2O2 (1 mM) was preincubated with 40 μg/ml of OMV for 1 h at 37°C. Bacteria were resuspended in Brucella broth to an OD600 of 0.1, and added to the OMV-treated H2O2. Mixtures were incubated for 3 h at 37°C, and plated on chocolate agar for 5 days at 37°C to enumerate the bacterial survival based on colony forming units (CFU). Control experiments were performed as described above by using only H2O2 without OMVs.
Hypochlorous Acid-Based Disk Diffusion Sensitivity Assay
A sterilized filter paper (5.4 mm in diameter) was saturated with 20 μl of 5% NaClO that had been pre-incubated for 3 h with OMVs. Bacterial colonies were resuspended in PBS and evenly spread on chocolate agar. Filter papers were placed on top of the agar, and plates were incubated at 37°C for 3 days. The diameter of inhibition zones was measured.
Statistical Analysis
Graph-Pad Prism® 7.0 (La Jolla, CA, United States) was used, and differences between groups or samples were considered statistically significant at p < 0.05.
Author Contributions
AA, SL, Y-CS, and KR designed the study. AV, IO, MB, MA-K, and SL did experiments. KR, SL, and Y-CS wrote the manuscript. All authors have read and approved the submitted version.
Funding
This work was supported by grants from the Alfred Österlund, the Anna and Edwin Berger, Greta and Johan Kock, the Swedish Medical Research Council (Grant No. K2015-57X-03163-43-4, www.vr.se), the Physiographical Society (Forssman’s Foundation), Skåne County Council’s research and development foundation, Cancerfonden [CAN 2015/782 (to AA) and CAN 2016/545 (to KR)] and Lions Cancerforskningsfond Umeå (to AA).
Conflict of Interest Statement
The authors declare that the research was conducted in the absence of any commercial or financial relationships that could be construed as a potential conflict of interest.
Supplementary Material
The Supplementary Material for this article can be found online at: https://www.frontiersin.org/articles/10.3389/fmicb.2018.01837/full#supplementary-material
References
Allen, L. A., Beecher, B. R., Lynch, J. T., Rohner, O. V., and Wittine, L. M. (2005). Helicobacter pylori disrupts NADPH oxidase targeting in human neutrophils to induce extracellular superoxide release. J. Immunol. 174, 3658–3667.
Benoit, S. L., and Maier, R. J. (2016). Helicobacter catalase devoid of catalytic activity protects the bacterium against oxidative stress. J. Biol. Chem. 291, 23366–23373. doi: 10.1074/jbc.M116.747881
Bonnington, K. E., and Kuehn, M. J. (2014). Protein selection and export via outer membrane vesicles. Biochim. Biophys. Acta 1843, 1612–1619. doi: 10.1016/j.bbamcr.2013.12.011
Huang, C. H., and Chiou, S. H. (2011). Proteomic analysis of upregulated proteins in Helicobacter pylori under oxidative stress induced by hydrogen peroxide. Kaohsiung J. Med. Sci. 27, 544–553. doi: 10.1016/j.kjms.2011.06.019
Ko, S. H., Rho, D. J., Jeon, J. I., Kim, Y. J., Woo, H. A., Kim, N., et al. (2016). Crude preparations of Helicobacter pylori outer membrane vesicles induce upregulation of heme oxygenase-1 via activating Akt-Nrf2 and mTOR–IκB kinase–NF-κB pathways in dendritic cells. Infect. Immun. 84, 2162–2174. doi: 10.1128/IAI.00190-116
Mullaney, E., Brown, P. A., Smith, S. M., Botting, C. H., Yamaoka, Y. Y., Terres, A. M., et al. (2009). Proteomic and functional characterization of the outer membrane vesicles from the gastric pathogen Helicobacter pylori. Proteomics Clin. Appl. 3, 785–796. doi: 10.1002/prca.200800192
Olofsson, A., Vallström, A., Petzold, K., Tegtmeyer, N., Schleucher, J., Carlsson, S., et al. (2010). Biochemical and functional characterization of Helicobacter pylori vesicles: H. pylori vesicles and properties for adhesion. Mol. Microbiol. 77, 1539–1555. doi: 10.1111/j.1365-2958.2010.07307.x
Parker, H., and Keenan, J. I. (2012). Composition and function of Helicobacter pylori outer membrane vesicles. Microbes Infect. 14, 9–16. doi: 10.1016/j.micinf.2011.08.007
Ramarao, N., Gray-Owen, S. D., and Meyer, T. F. (2000). Helicobacter pylori induces but survives the extracellular release of oxygen radicals from professional phagocytes using its catalase activity. Mol. Microbiol. 38, 103–113. doi: 10.1046/j.1365-2958.2000.02114.x
Richter, C., Mukherjee, O., Ermert, D., Singh, B., Su, Y. C., Agarwal, V., et al. (2016). Moonlighting of Helicobacter pylori catalase protects against complement-mediated killing by utilising the host molecule vitronectin. Sci. Rep. 6:24391. doi: 10.1038/srep24391.
Roesler, B. M., Rabelo-Gonçalves, E. M. A., and Zeitune, J. M. R. (2014). Virulence factors of Helicobacter pylori: a review. Clin. Med. Insights Gastroenterol. 7, 9–17. doi: 10.4137/CGast.S13760
Stentz, R., Horn, N., Cross, K., Salt, L., Brearley, C., Livermore, D. M., et al. (2015). Cephalosporinases associated with outer membrane vesicles released by Bacteroides spp. protect gut pathogens and commensals against β-lactam antibiotics. J. Antimicrob. Chemother. 70, 701–709. doi: 10.1093/jac/dku466
Voss, B. J., Gaddy, J. A., McDonald, W. H., and Cover, T. L. (2014). Analysis of surface-exposed outer membrane proteins in Helicobacter pylori. J. Bacteriol. 196, 2455–2471. doi: 10.1128/JB.01768-1714
Wang, G., Alamuri, P., and Maier, R. J. (2006). The diverse antioxidant systems of Helicobacter pylori. Mol. Microbiol. 61, 847–860. doi: 10.1111/j.1365-2958.2006.05302.x
Keywords: H. pylori, KatA, outer membrane vesicles, oxidative burst, reactive oxygen species
Citation: Lekmeechai S, Su Y-C, Brant M, Alvarado-Kristensson M, Vallström A, Obi I, Arnqvist A and Riesbeck K (2018) Helicobacter pylori Outer Membrane Vesicles Protect the Pathogen From Reactive Oxygen Species of the Respiratory Burst. Front. Microbiol. 9:1837. doi: 10.3389/fmicb.2018.01837
Received: 16 May 2018; Accepted: 23 July 2018;
Published: 07 September 2018.
Edited by:
Rosana Puccia, Federal University of São Paulo, BrazilReviewed by:
Lee-Ann H. Allen, The University of Iowa, United StatesAlberto Danielli, Università degli Studi di Bologna, Italy
Copyright © 2018 Lekmeechai, Su, Brant, Alvarado-Kristensson, Vallström, Obi, Arnqvist and Riesbeck. This is an open-access article distributed under the terms of the Creative Commons Attribution License (CC BY). The use, distribution or reproduction in other forums is permitted, provided the original author(s) and the copyright owner(s) are credited and that the original publication in this journal is cited, in accordance with accepted academic practice. No use, distribution or reproduction is permitted which does not comply with these terms.
*Correspondence: Kristian Riesbeck, kristian.riesbeck@med.lu.se
†These authors have contributed equally to this work.