- 1Institute of Biological Sciences, Federal University of Minas Gerais (ICB/UFMG), Belo Horizonte, Brazil
- 2STLO, INRA, Agrocampus Ouest, Rennes, France
- 3Institute of Biotechnology, Federal University of Uberlândia, Uberlândia, Brazil
Mucositis is a clinically important gastrointestinal inflammatory infirmity, generated by antineoplastic drugs cytotoxic effects. The inflammatory process caused by this disease frequently leads to derangements in the alimentary tract and great malaise for the patient. Novel strategies are necessary for its prevention or treatment, as currently available treatments of mucositis have several limitations in relieving its symptoms. In this context, several research groups have investigated the use of probiotic bacteria, and in particular dairy bacterial strains. Compelling evidences reveal that milk fermented by certain probiotic bacteria has the capacity to ameliorate intestinal inflammatory disorders. In addition, innovative probiotic delivery strategies, based on probiotics incorporation into protective matrices, such as whey proteins, were able to increase the therapeutic effect of probiotic strains by providing extra protection for bacteria against environmental stresses. Therefore, in this study, we evaluated the role of the whey protein isolate (WPI), when added to skim milk fermented by Lactobacillus casei BL23 (L. casei BL23) or by Propionibacterium freudenreichii CIRM-BIA138 (P. freudenreichii 138), as a protective matrix against in vitro stress challenges. In addition, we investigated the therapeutic effect of these fermented beverages in a murine model of mucositis induced by 5-Fluorouracil (5-FU). Our results demonstrated that milk supplementation with 30% (w/v) of WPI increases the survival rate of both strains when challenged with acid, bile salts, high temperature and cold storage stresses, compared to fermented skim milk without the addition of WPI. Moreover, treatment with the probiotic beverages prevented weight loss and intestinal damages in mice receiving 5-FU. We conclude that the presence of WPI maximizes the anti-inflammatory effects of L. casei BL23, but not for P. freudenreichii 138, suggesting that whey protein enhancement of probiotic activity might be strain-dependent.
Introduction
Mucositis is a severe inflammation that affects the entire extension of the Alimentary Tract (AT) of individuals undergoing malignancy treatment based on chemotherapy or radiotherapy (Sonis, 2004). One of the main drugs associated with this condition is 5-Fluorouracil (5-FU). This is a antimetabolic drug commonly prescribed for the treatment of head, neck and gastrointestinal cancer (Longley et al., 2003). 5-FU unfortunately presents non-specific cytotoxicity toward cells, inhibiting the proliferation of both cancer cells and normal cells with high replication rates, such as the enterocytes of the gastrointestinal tract (GIT) (Carvalho et al., 2017a). A series of clinical symptoms, such as nausea, weight loss, vomiting, severe abdominal pain and diarrhea are commonly reported in patients receiving 5-FU during cancer treatment (Bastos et al., 2016). Moreover, mucositis frequently increases predisposition to local and systemic secondary infections, thus generating additional costs and extending the patient’s hospitalization time (Carvalho et al., 2017a). Mucositis is characterized by pathological changes in the small bowel. These changes include the presence of degenerate enterocytes (Ciorba et al., 2016), submucosal vessel damage, leukocyte infiltrate in the lamina propria, with accumulation of neutrophils and eosinophils (Antunes et al., 2016), increased mucin production and degeneration of goblet cells (Stringer, 2013), atrophy of villi, hypoplasia and apoptosis of intestinal crypts (Chang et al., 2012). Currently, there is no treatment that is completely successful in the prevention and treatment of mucositis. However, there has been a growing interest in the use of probiotics as promising candidates for the treatment of this disease (Carvalho et al., 2017a).
Probiotics are included in a variety of products, including fermented foods, dietary supplements, formulas for newborns and infants, as well as various pharmaceutical formulations (Cousin et al., 2011). Currently, fermented beverages by one or more bacteria, have gained the functional food status, which makes them an important part of our diet as well as our main daily source of beneficial microbes (Leroy and De Vuyst, 2014; Carmo et al., 2017).
Selected strains of lactic acid bacteria (LAB) were reported as probiotic with beneficial effects provided by different mechanisms of action and can be used in functional foods withal (Carvalho et al., 2017b; Eales et al., 2017; Tang et al., 2017). Some studies have shown that the administration of lactobacilli strains can reduced some parameters of mucositis in mice model induced by 5-FU, such as prevent weight loss, attenuate the diarrhea and intestinal damage (Justino et al., 2015). L. casei BL23 has also been considered as a good probiotic strain, according to results obtained in others inflammatory models. Some studies demonstrated that L. casei BL23 was able to alleviates colitis symptoms in a dextran sulfate sodium (DSS) model (Foligne et al., 2007; Rochat et al., 2007) and the ability of these strain to attenuate intestinal inflammation can be enhanced using a protection matrix (Lee et al., 2015b). Other important group of bacteria widely used in the food industry, particularly in Swiss-type cheese manufacture, is propionic acid bacteria (PAB). Propionibacterium freudenreichii represents the main species thereof and is listed in the Qualified Presumption of Safety list (QPS) by the European food safety authority and it has recently been considered a promising probiotic (Rabah et al., 2017). P. freudenreichii produces metabolites are considered as prebiotics, such as 1,4-dihydroxy-2-naphtoic acid (DHNA) and 2-amino-3-carboxy-1,4-naphthoquinone (ACNQ), both associated to bifidogenic effects. Furthermore, P. freudenreichii is the only GRAS bacterial species producing food-grade vitamin B12 at the industrial scale (Rabah et al., 2017). Selected P. freudenreichii strains have been associated with therapeutical effects based on in vitro and in vivo properties to attenuate colitis model induced by trinitrobenzene sulfonic acid (TNBS) (Cousin et al., 2012a, 2016; Plé et al., 2015). A dairy propionibacteria, P. freudenreichii 138, demonstrated a pro-apoptosis capacity, in HTG-1 human gastric cancer cells without toxicity effects in healthy human cells (Cousin et al., 2012a, 2016). Moreover, Cousin et al. (2012b) shown the persistence of P. freudenreichii 138 in piglets colon, withal metabolic activity and producing propionate, which is a SCFA with probiotic properties (Cousin et al., 2012b).
An extremely important factor for the therapeutic effects of probiotics is the ability of the bacteria to survive during transit through the GIT or during industrial processes (Cousin et al., 2012b; Rabah et al., 2017). Digestion indeed imposes harsh conditions including gastric acid and presence of bile salts, which may severely affect bacterial viability (Leroy and De Vuyst, 2014; Huang et al., 2016a). A probiotic microorganism must, however, tolerate these stresses for a long persistence in the host and for an enhanced beneficial effect (Carmo et al., 2017). In the aim to maximize the tolerance of bacteria to stressful environments and thus to increase their probiotic ability, the food matrix used in the manufacture of fermented products plays a key role as a protective medium (Gagnaire et al., 2015; Lee et al., 2015a). As an example, milk proteins, as well as whey protein isolates, constitute very promising protective matrices for probiotics, besides being an efficient delivery vehicles to target protective molecules and microorganisms to digestive epithelium (Livney, 2010; Cousin et al., 2012a; Vargas et al., 2015; Huang et al., 2016a). Whey proteins have been recognized for their various functional and nutritional properties. The functional properties are mainly due to their physical, chemical and structural characteristics and the nutritional value is directly linked to the concentration of essential amino acids (Yadav et al., 2015). Some studies have also demonstrated the potential of whey proteins to enhance the survival and viability of probiotic bacteria during production and storage (Marshall, 2004; Madureira et al., 2007; Almeida et al., 2009; Huang et al., 2016b; Baruzzi et al., 2017; Dąbrowska et al., 2017).
The aims of this work were (i) to evaluate whether whey protein isolate is a good protective matrix for L. casei BL23 and P. freudenreichii CIRM-BIA 138, against adverse environmental conditions and (ii) to investigate the therapeutic effect of administration of whey protein isolate-supplemented beverage, fermented by both strains, in the prevention of mucositis induced by 5-FU.
Materials and Methods
Bacterial Strains and Growth Conditions
Lactobacillus casei BL23 strain was kindly supplied by the UMR1219 Micalis Institute (INRA-AgroParisTech, Jouy-En-Josas, France) and the P. freudenreichii CIRM-BIA138 (alias ITG P9) strain by the Biological Resource Center (International Center of Microbial Resources, INRA, Rennes, France). L. casei BL23 was grown in MRS broth at 37°C for 24 h, without shaking. P. freudenreichii 138 was grown in YEL culture medium at 30°C for 72 h, without agitation (Malik et al., 1968).
Dairy Beverage Formulation and Supplementation With Whey Protein Isolate
The fermented beverage was prepared using skimmed milk (SM) powder 12% w/v (Itambé, Brazil). For cultivation of L. casei BL23 (SMLC), the SM was supplemented with yeast extract (Kasvi Curitiba, Brazil) and glucose (Merck, Germany) (Tharmaraj and Shah, 2003). For cultivation of P. freudenreichii 138 (SMPF), the milk was supplemented with casein peptone (5g/L) (KASVI, Curitiba, Brazil) and sodium lactate (50 m/M) (Sigma, St. Louis, MO, United States) (Cousin et al., 2012b). Both milk were autoclaved at 110°C for 15 min. The SMs was supplemented with whey protein isolate (WPI), natural flavor, 90% protein (Vulgo Supplements, Brazil) at concentrations of 5, 15, and 30% w/v, and strains cultivated in skimmed milk without WPI was used as control. The same growth conditions used to cultivate the strains in MRS or YEL were applied for growth in fermented beverages.
Stress Challenges
Samples of 10 ml from the stationary-phase of L. casei BL23 and P. freudenreichii 138 in culture media or in skim milk, supplemented or not with WPI were subjected to acid, bile salts, and heat challenges (Huang et al., 2016b). For acid stress, the samples were incubated in MRS broth or YEL broth, previously adjusted to pH 2.0 using HCl, at 37°C for 60 min. Briefly, for bile salts stress, the samples were incubated in MRS broth or YEL broth containing 1.0 g/L of bile salts (an equimolar mixture of cholate and deoxycholate, Sigma Chemical, St. Louis, MO, United States) and then, incubated at 37°C for 60 min. Finally, to simulate the pasteurization temperature established by the International Dairy Foods Association (IDFA), the samples were incubated in their specific culture media pre-heated to 63°C for 30 min. After stresses challenges, aliquots of each sample were subjected to 1:10 serial dilutions using peptone water (9 g/L peptone, 5 g/L NaCl) and plated on MRS agar or YEL agar medium. Plates of L. casei BL23 were incubated for 48 h at 37°C. Plates of P. freudenreichii 138 were incubated for 144 h (6 days) at 30°C in jars containing anaerobiosis generator (Anaerocult A®, Merck Millipore). The number of viable bacteria was determined by counting of colony forming unit (CFU) after incubation. The bacteria survival rate (%) through each stress condition was calculated through the following equation (Ferreira et al., 2017):
Where N refers to the number of bacteria population (CFU mL-1) in culture medium after stress challenges, and N0 refers to the number of initial population (CFU mL-1) before the stress challenges.
Bacterial Survival During Storage at 4°C
The long-term survival of L. casei BL23 and P. freudenreichii 138 in dairy beverage supplemented with 30% of WPI was assessed during the storage process at 4°C for 90 days kept away from light (Huang et al., 2016b). For the evaluation of bacterial survival during cold storage, plate seeding was performed on days 0 (pre-storage time), 7, 14, 21, 30, 60, and 90 after storage. The L. casei BL23 and P. freudenreichii 138 plates were incubated according to their specific conditions on agar media (see above). The number of viable bacteria during storage was determined by counting CFU in the culture after incubation. The evolution of acidification of stored samples was also screened in the same days. To evaluate if both fermented beverages could survive GIT stress conditions after being stored at 4°C, we performed acid stress and biliary stress in vitro challenges for the dairy beverage supplemented with 30% of WPI. Acid and bile salts stresses were performed on days 30, 60, and 90 after the storage start.
Evaluation of Therapeutic Effects of Beverages Fermented by L. casei BL23 or P. freudenreichii 138 in a Mice Model of Mucositis
Animals
Conventional female BALB/c mice between 6 and 8 weeks of age were obtained at Federal University of Minas Gerais (UFMG–Belo Horizonte, Brazil). Mice were kept in a temperature-controlled room with ad libitum access to water and standard chow diet. The study was approved by the Ethics Committee on Animal Experimentation of the Federal University of Minas Gerais (CEUA-UFMG, Brazil, protocol 366).
Probiotic Treatment, Mucositis Induction, and Experimental Groups
For probiotic treatment, mice received 0.5 ml of fermented beverages supplemented or not with 30% of WPI via gastric gavage, during 13 days. In order to induce mucositis, mice received a single intraperitoneal injection of 5-FU (Fauldfluor – Libbs) (300 mg/kg) on day 11, and were euthanized 72 h after induction of mucositis, in 14th of experimental day (Carvalho et al., 2017a). An injection of saline (NaCl 0.9%) was used in control groups. After euthanasia, a longitudinal abdominal incision was performed to remove the intestine for further analyses. Body weight of mice was determined throughout the experiment. For in vivo experimentation, BALB/c mice were divided into sixteen groups according to Table 1. All experiments were performed simultaneously therefore, the same control groups were used for all experimental probiotic assays. Each group containing 6–9 animals. All beverages contained 109 CFU mL-1 bacteria.
Histological Analysis
The distal portion of the small bowel (ileum) from the mice was collected and washed with PBS. Afterwards, rolls were prepared for histomorphological analysis. Histological materials were immersed in 4% buffered formaldehyde solution for tissue fixation. Then, the material was embedded in paraffin, and a 4 μm section of each sample was placed on a glass slide and stained with Hematoxylin-Eosin (HE). The histological score was determined using a score that measures the intensity of the infiltrate of mononuclear and polymorphonuclear cells in the lamina propria of the ileum, the presence of ulceration and erosion and changes in mucosal architecture (Soares et al., 2008). For each parameter a classification was given according to the severity of the lesion in the tissues: absent (0), mild (1), moderate (2) and severe (3). For morphometric analysis, 10 images of the ileum of each animal were randomly captured and analyzed using ImageJ software (version 1.8.0). Villi height and the crypt depth were measured vertically from the tip of villi to the base of the adjacent crypt. Additional cuts in the paraffinized samples from the ileum were stained by the Periodic Acid-Schiff (PAS), technique to determine the number of goblet cells in the tissues (Prisciandaro et al., 2011). Ten random field images of each sample were made using the 40× objective and the intact goblet cells were counted using ImageJ software (version 1.8.0) and expressed as the number of cells per high-power field (hpf) (40×, 108.2 μm2).
Measurement of Secretory IgA
Levels of secretory IgA (sIgA) were determined by enzyme-linked immunosorbent assay (ELISA) in small bowel intestinal fluids (Carvalho et al., 2017a). Microtiter plates (Nunc-Immuno Plates, MaxiSorp) were coated with anti-IgA antibodies (Southern Biotechnology, Birmingham, AL, United States) for 18 h at 4°C. The plates were washed with saline (NaCl 0.9%) added with Tween 20 (0.05%) and blocked with 200 μl PBS-casein (0.05%) for 1 h at room temperature. Intestinal fluid samples were diluted in PBS-casein (0.25%) and then added to the plate. After incubation for 1 h at room temperature, the wells were washed and biotin-conjugated anti-mouse IgA antibody (Southern Biotechnology) diluted in PBS-casein (0.25%) (1: 10,000). The plates were incubated for 1 h at 37°C and anti-IgA conjugated to streptavidin peroxidases (1:10,000) were added (Southern Biotechnology). After 1 h of incubation, 100 μl of orthophenylenediamine (OPD) (Sigma, St. Louis, MO, United States) and H2O2 (0.04%) were added to each well. Plates were kept away from light until the coloration developed. The reaction was stopped by addition of 2 N H2SO4. Reading was performed on a plate reader (Bio-Rad Model 450 Microplate Reader) at 492 nm absorbance. The results were measured in concentration of sIgA (μg) per ml of intestinal fluid, according to the standard curve.
Statistical Analyses
The results were reported as the mean ± standard deviation and analyzed using Student’s t-test, Holm–Sidak t-test, One-Way ANOVA or Two-Way ANOVA followed by the Tukey or Sidak post-test. Non-parametric data’s were analyzed using Kruskal-Wallis data followed by the Dunns post-test. Graphs and statistical analyzes were performed in GraphPad Prism version 7.00 for Windows (GraphPad Software, San Diego, CA, United States). P-values under 0.05 were considered significant.
Results
Different Concentrations of Whey Protein Isolate Alter the Growth of Bacteria
The final population of L. casei BL23 and of P. freudenreichii 138 was monitored after growth in skim milk supplemented with different concentrations of WPI (Figure 1). We observed a significant increase in the CFU counting of L. casei BL23 when cultivated in SMLC after 24 h (6.3 × 109 CFU mL-1), in comparison with MRS medium (2.1 × 109 CFU mL-1). Moreover, the largest final population of L. casei BL23 was found in skim milk supplemented with 30% WPI (SMLC + WPI 30%) (9.5 × 109 CFU mL-1). The final population of P. freudenreichii 138 also showed a similar result (Figure 1B). In this case, the highest population (7.2 × 109 CFU mL-1) was obtained in skim milk supplemented with 30% of WPI (SMPF + WPI 30%). This condition allowed the highest growth of the propionibacteria, when compared with all other culture media used.
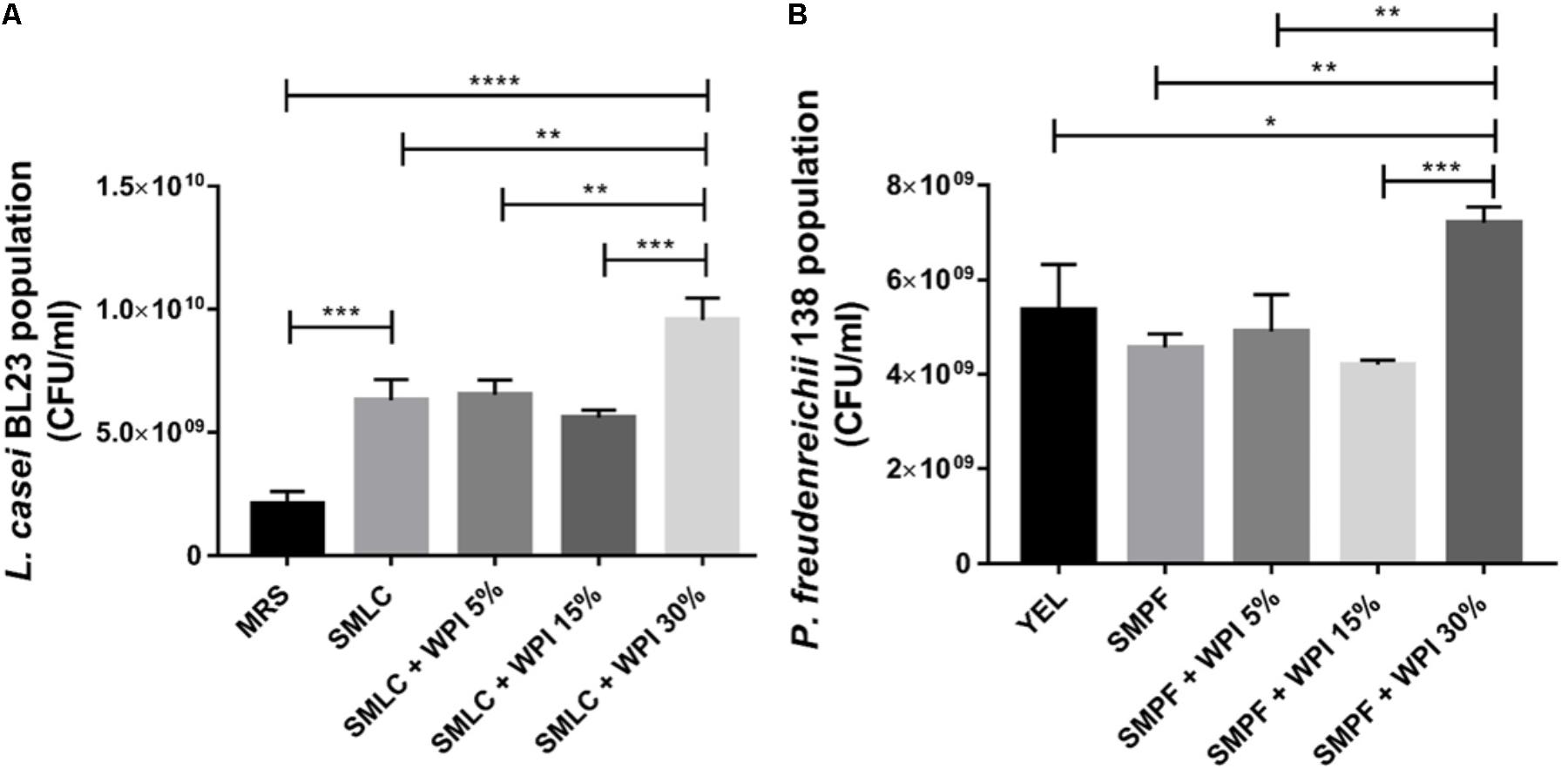
FIGURE 1. Different concentrations of WPI in skim milk impacted on Lactobacillus casei BL23 and Propionibacterium freudenreichii 138 growth. (A) Colony-forming unit (CFU) counting of L. casei BL23, after 24 h of incubation at 37°C and (B) Population of P. freudenreichii 138, after 78 h of anaerobic incubation at 30°C were assessed by CFU counts. These assays were performed in triplicate technique and biological triplicate. Asterisks represent statistically significant differences and were indicated as follows: ∗p < 0.05, ∗∗p < 0.01, ∗∗∗p < 0.001, ∗∗∗∗p < 0.0001.
Whey Protein Isolate Improves the Tolerance of Bacteria Toward Environmental Stresses
The survival rate of L. casei BL23 and P. freudenreichii 138 was evaluated after acid stress, bile salts stress and high-temperature stress (Figure 2). L. casei BL23 showed enhanced survival rate after acid stress, when cultured in SMLC medium (69.2%), compared to the MRS control (55.1%) (Figure 2A). The survival was further increased when SMLC was supplemented with 30% WPI, leading to the highest survival rate of L. casei BL23 (80.6%). This value was significantly higher than skim milk supplemented with 5% and 15% of WPI (data not shown). The population after acid stress in SMLC + WPI 30% was 8.5 × 107 CFU mL-1. A similar result was observed for P. freudenreichii 138 (Figure 2D). After acid stress, the highest tolerance was observed when P. freudenreichii 138 was grown in skim milk supplemented with 30% WPI (SMPF + WPI 30%) (88.6%), compared with skim milk without supplementation (SMPF) (84.3%) or YEL medium (65.3%). In addition, the survival rate of P. freudenreichii 138 grown in milk added with 5% of WPI or 15% are lower than supplementation with 30% (data not shown). The population of P. freudenreichii 138 after acid stress in SMPF + WPI 30% was 7.6 × 108 CFU mL-1. Likewise, our results show enhanced tolerance toward bile salts stress when L. casei BL23 and P. freudenreichii 138 were cultured in beverage containing 30% of WPI (Figures 2B,E). After temperature stress, we observed that both strains presented a high tolerance to 63°C, independent of culture medium. However, the highest survival rate was obtained when bacteria were cultured in milk added with 30% of WPI (Figures 2C,F).
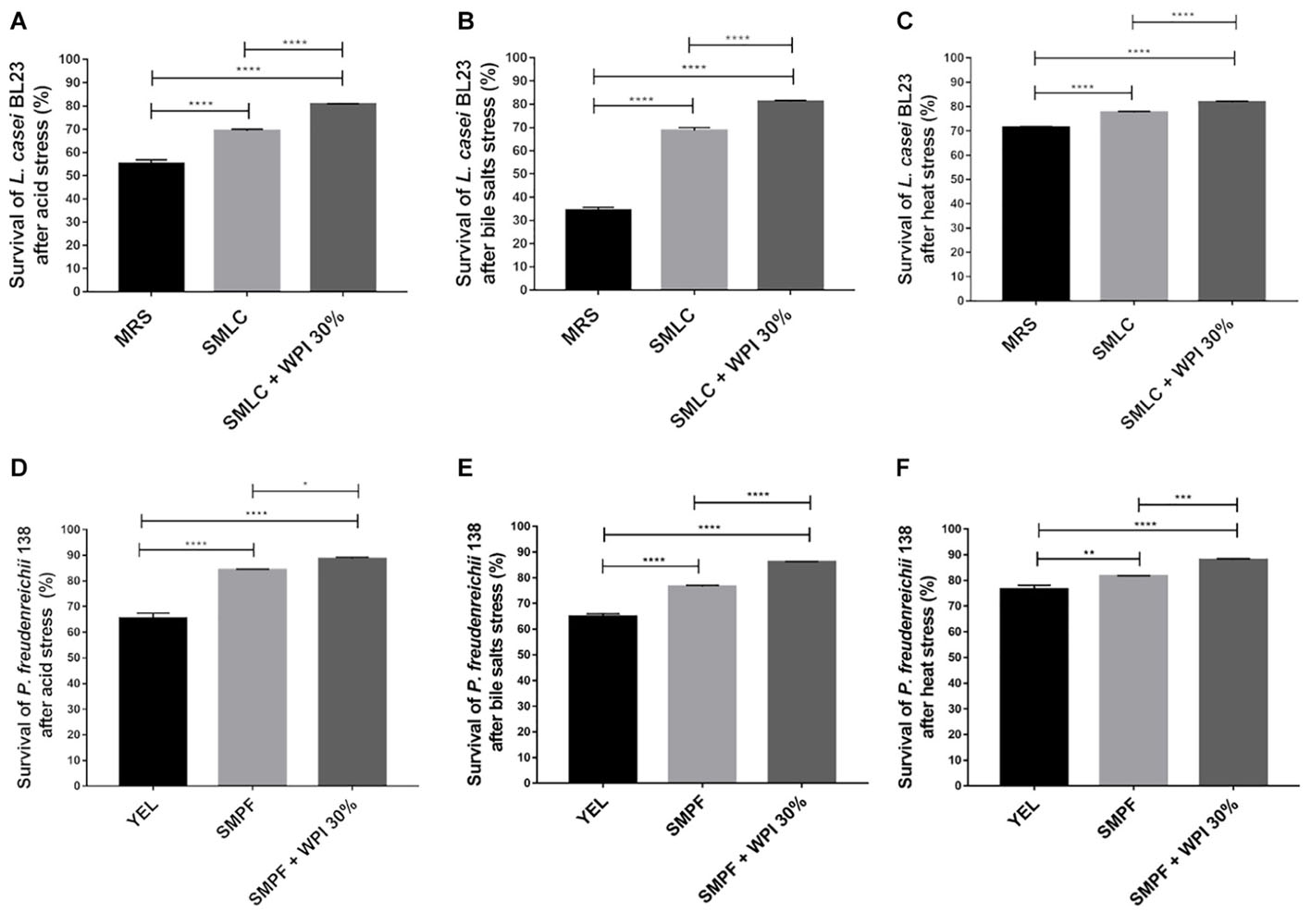
FIGURE 2. Whey protein isolate (WPI) confers stress tolerance on L. casei BL23 and P. freudenreichii 138. L. casei BL23 was cultured for 24 h in the indicated growth media until stationary phase and then subjected to (A) acid stress (pH 2 for 60 min at 37°C); (B) bile salts stress (1 g/liter for 60 min at 37°C) or (C) heat stress (63°C for 30 min). P. freudenreichii 138 was cultured for 72 h in each culture media until stationary phase, and then subjected to (D) acid stress, (E) bile salts stress, or (F) heat stress. Viable bacteria were enumerated by counting colonies in the challenged and control cultures and then, expressed as percent survival (means ± standard deviations). These assays were performed in triplicate technique and biological triplicate. Asterisks represent statistically significant differences and were indicated as follows: ∗p < 0.05, ∗∗p < 0.01, ∗∗∗p < 0.001, ∗∗∗∗p < 0.0001.
Bacteria Remained Viable in the Fermented Milk Supplemented With WPI During Storage at 4°C
Changes in bacterial population in fermented skim milk supplemented with whey protein were monitored during storage at 4°C for 90 days (Figures 3A,B). The viability of both strains, in skim milk contained 30% of WPI, remained practically unchanged, with a small CFU reduction after 90 days. After cold storage, the L. casei BL23 population in the skim milk culture media was maintained at 1.5 × 109 CFU mL-1, while the P. freudenreichii 138 population at 7.4 × 109 CFU mL-1. In contrast, viable L. casei BL23 and P. freudenreichii 138 was significantly decreased when grown in culture media (MRS and YEL, respectively) over the entire storage time, presenting a final population below 2 × 102 CFU mL-1, for L. casei BL23 and 1.0 × 106 CFU mL-1 for P. freudenreichii 138. Figures 3C,D represents the evolution of the pH values in the culture of both strains during the storage. We observed a decrease in pH values in beverages containing WPI. In the order hand, in controls the pH values did not decline over the storage time, remaining constant for L. casei BL23 while a slight increase in the pH value was detected for P. freudenreichii 138. Figures 4A,B represent the survival rates of L. casei BL23 and P. freudenreichii 138, upon acid and bile salt stresses, following storage at 4°C. The survival rate of L. casei remained almost constant between days 0 and 90 of storage (Figure 4A). For P. freudenreichii 138, the acid and bile salts tolerance slightly decreased, between days 0 and 30 (Figure 4B). However, propionibacteria survival remained around 70% after 90 days of storage.
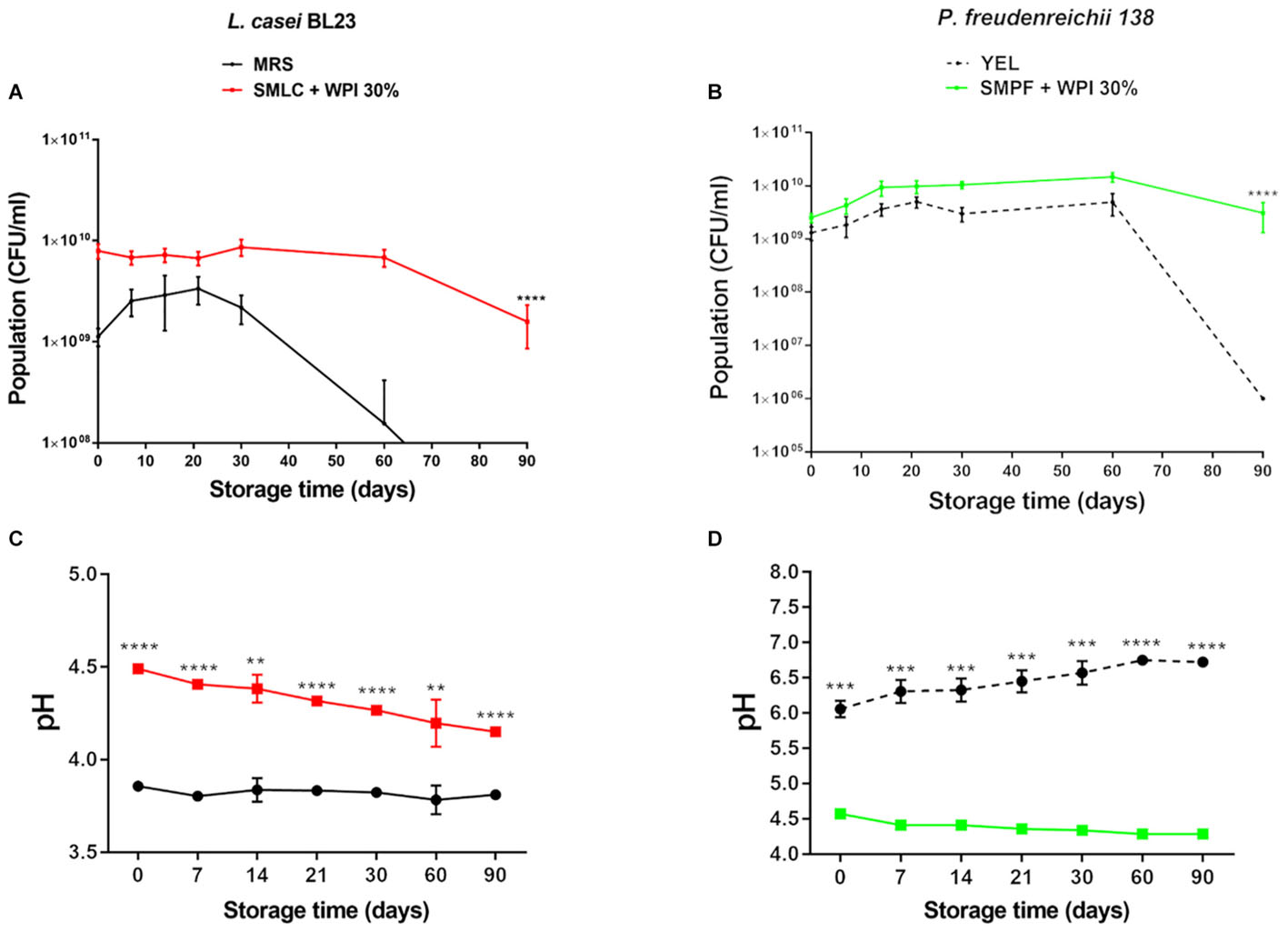
FIGURE 3. Viability of L. casei BL23 and P. freudenreichii 138 are maintained during 90 days of cold storage when are cultured in skim milk supplemented with WPI. Final population (CFU ml-1) of (A) L. casei BL23, and (B) P. freudenreichii 138 in the indicated culture media. Bacteria were culture until the stationary phase and then storage at 4°C for 90 days. Viable bacteria were enumerated by counting the colonies on days 0, 7, 14, 21, 30, 60, and 90 after storage. (C,D) Variation of pH values throughout storage days. Asterisks represent statistically significant differences as follows: ∗p < 0.05, ∗∗p < 0.01, ∗∗∗p < 0.001, ∗∗∗∗p < 0.0001.
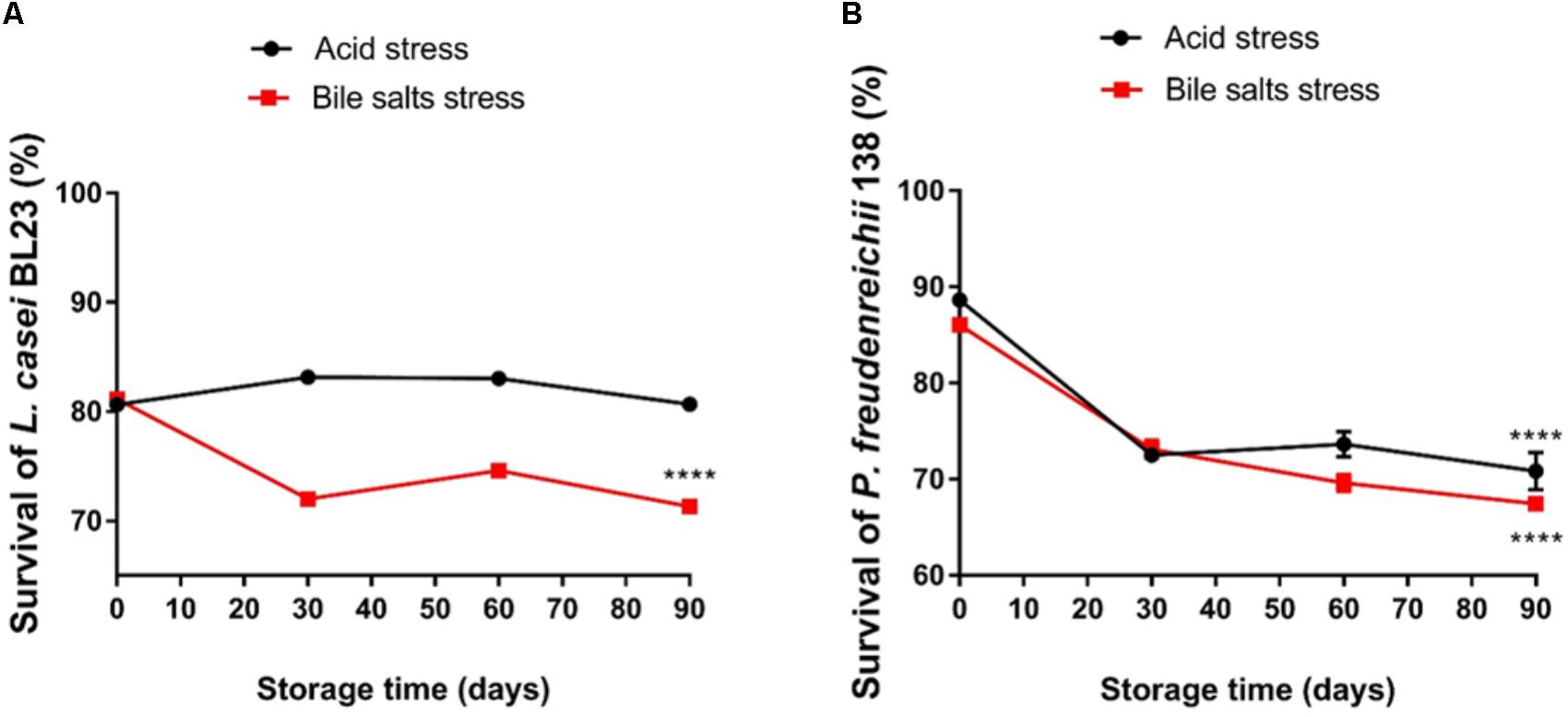
FIGURE 4. Lactobacillus casei BL23 (A) and P. freudenreichii 138 (B) maintain the ability to tolerate acid stress and bile salts stress even during storage when cultured in skim milk supplemented with WPI. Viable bacteria were enumerated by counting colonies on cultures after acid and bile salt stress on days 0, 30, 60, and 90 post-storage and then expressed as percent survival (means ± standard deviation). The assays were performed in triplicate technique and biological triplicate. Asterisks represent statistically significant differences as follows: ∗p < 0.05, ∗∗p < 0.01, ∗∗∗p < 0.001, ∗∗∗∗p < 0.0001.
Probiotic Beverage Fermented by L. casei BL23 or P. freudenreichii 138 Reduces the Weight Loss in Mice With Mucositis
Time-course of the weight of mice during the 14 experimental days is shown in Figure 5. None of the treatments with probiotic beverages was able to significantly alter the weight of mice during the first 11 days of gavage preceding mucositis induction. As expected, mice receiving 5-FU began to lose weight soon after the drug injection on day 11 (Figures 5A,C,E). Interestingly, we observed that the treatment with probiotic beverage fermented by L. casei BL23 (Figure 5B) or fermented by P. freudenreichii 138 (Figure 5D) was able to reduce the weight loss of inflamed mice, in the presence or absence of WPI in the medium. However, the association of both bacterial strains were not efficient to further limit the weight loss, compared to mice that received no probiotic treatment (Figure 5F).
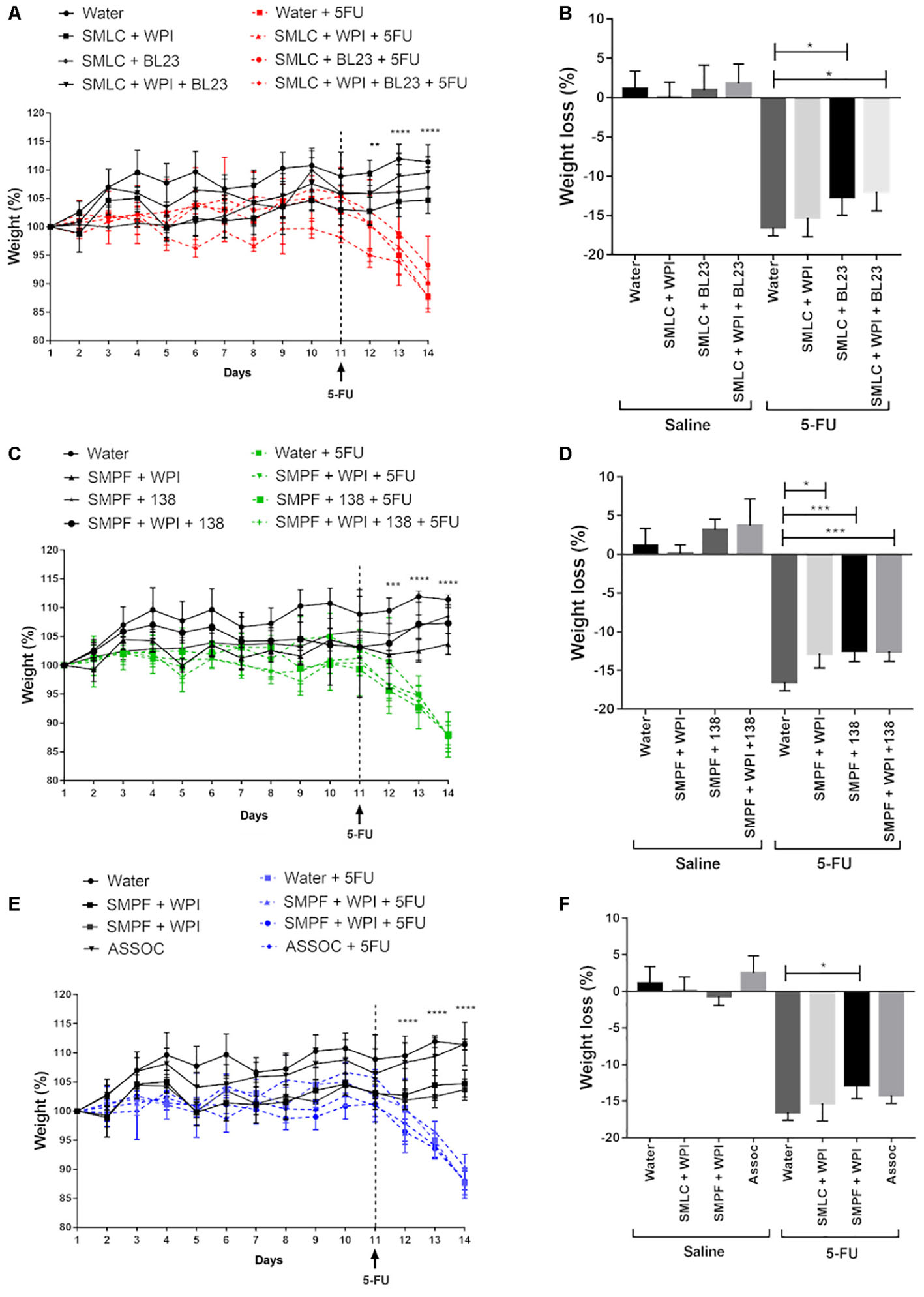
FIGURE 5. Time-course of body weight for mice treated with (A) probiotic beverage fermented by L. casei BL23; (C) probiotic beverage fermented by P. freudenreichii 138 and (E) probiotic beverage fermented by association with L. casei BL23 and P. freudenreichii 138. (B,D,F) Weight loss observed after 5-FU injection and differences across groups. N = 6–9. Mice were weighted daily during 14 days. Asterisks represent statistically significant differences as follows: ∗p < 0.05, ∗∗p < 0.01, ∗∗∗p < 0.001, ∗∗∗∗p < 0.0001.
Beverage Supplemented With Whey Protein Isolate Improves Mucosal Preservation in the Inflamed Mice
Histological analysis revealed a mucosal pattern within normal limits in all groups injected with saline (0.9% NaCl), showing that the probiotics beverages did not alter gut mucosal morphology (Figures 6–8). On the other hand, mice submitted to mucositis demonstrated alterations in the morphological structure of the ileum, which was evidenced by an increase in the histopathological parameters. This reflected mainly inflammatory cell infiltration in the lamina propria, submucosa and muscular layer, and a prominent alteration in villus structure. However, mice treated with probiotic beverages showed decreased mucosal damage, compared to inflamed mice that did not receive any probiotic treatment. Moreover, supplementation with WPI was able to improve the anti-inflammatory effects of L. casei BL23 beverage (Figure 6) but not for P. freudenreichii (Figure 7). Mice treated with the association of L. casei BL23 and P. freudenreichii 138 in skim milk, presented a reduced histopathological score compared to mice receiving only water, however, the histopathological scores obtained are no better than the treatment using the individually fermented milks by L. casei BL23 or P. freudenreichii 138 (Figure 8).
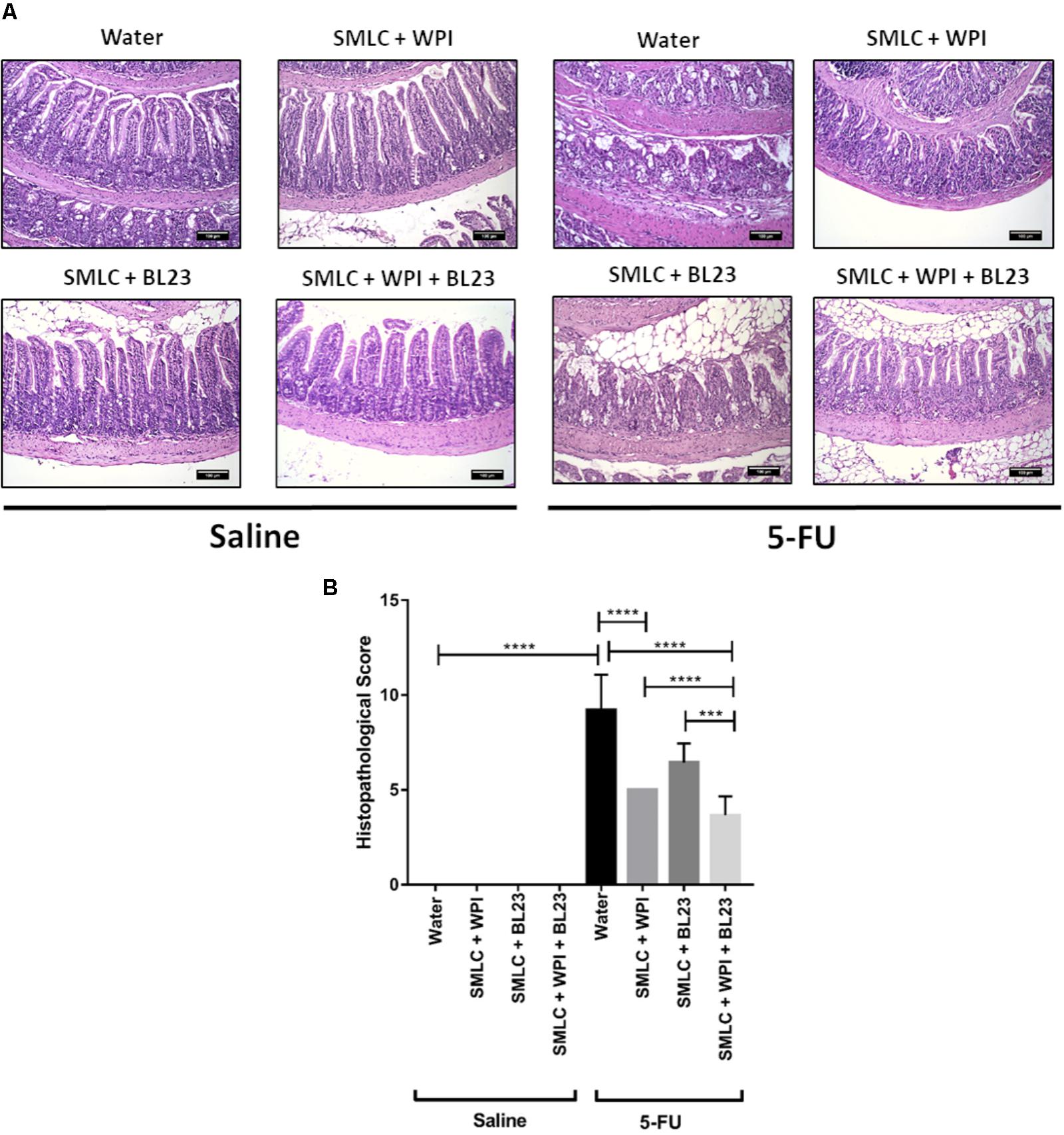
FIGURE 6. Administration of skim milk supplemented with WPI fermented by L. casei BL23 prevents mucosal damage in mice. (A) Representative H&E-stained images from mucosal histopathology and (B) histopathological score obtained in mice treated. The image acquisition was done with a 20× magnification objective. Scale bar = 100 μm. Same control groups were used for all experimental probiotic assays. Asterisks represent statistically significant differences as follows: ∗p < 0.05, ∗∗p < 0.01, ∗∗∗p < 0.001, ∗∗∗∗p < 0.0001.
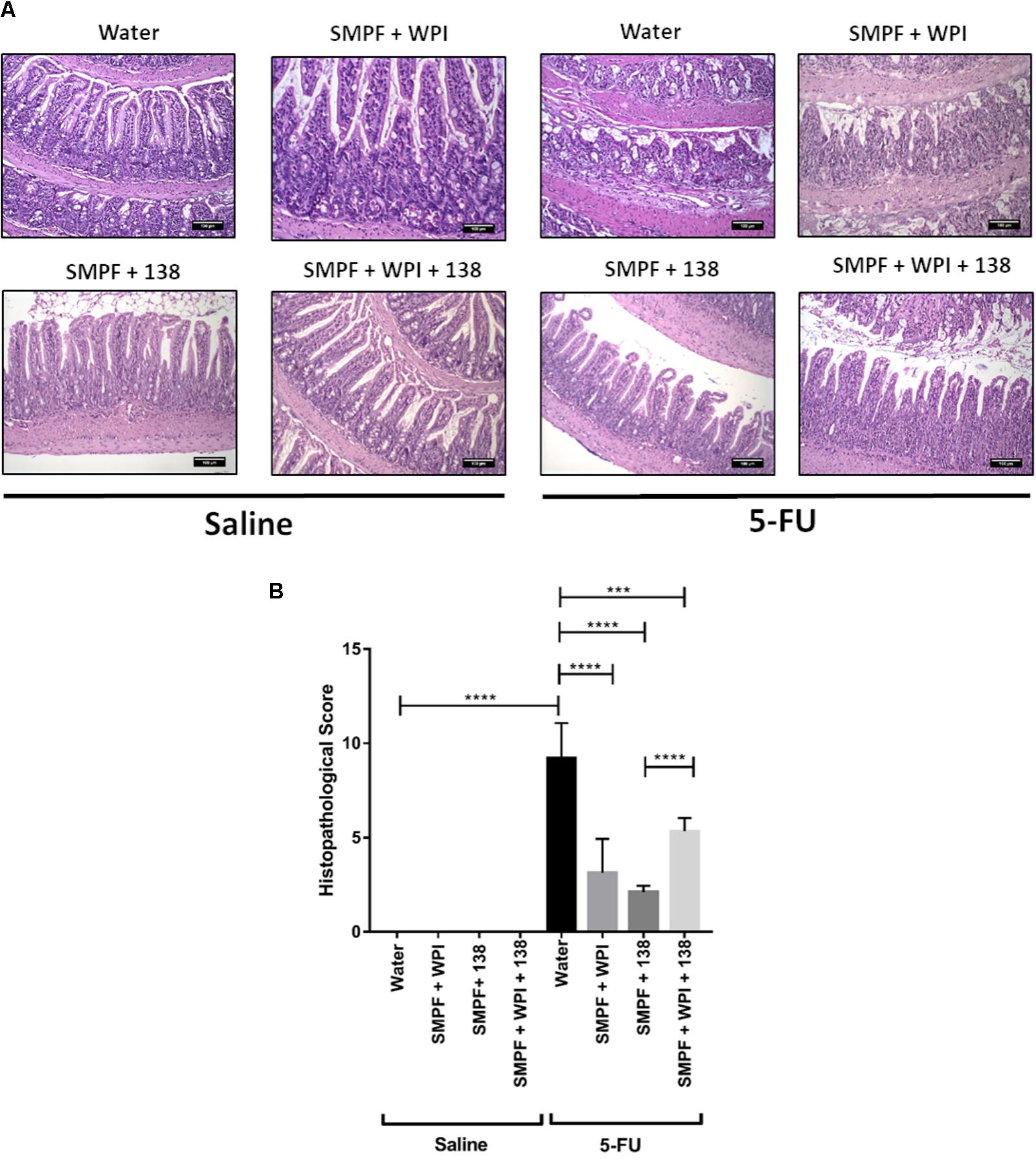
FIGURE 7. Administration of skim milk without supplemented with whey protein isolate and fermented by P. freudenreichii 138 prevents mucosal damage in mice. (A) Representative H&E-stained images from mucosal histopathology and (B) histopathological score obtained in mice. The image acquisition phase was done with a 20× magnification objective. Scale bar = 100 μm. Same control groups were used for all experimental probiotic assays. Asterisks represent statistically significant differences as follows: ∗p < 0.05, ∗∗p < 0.01, ∗∗∗p < 0.001, ∗∗∗∗p < 0.0001.
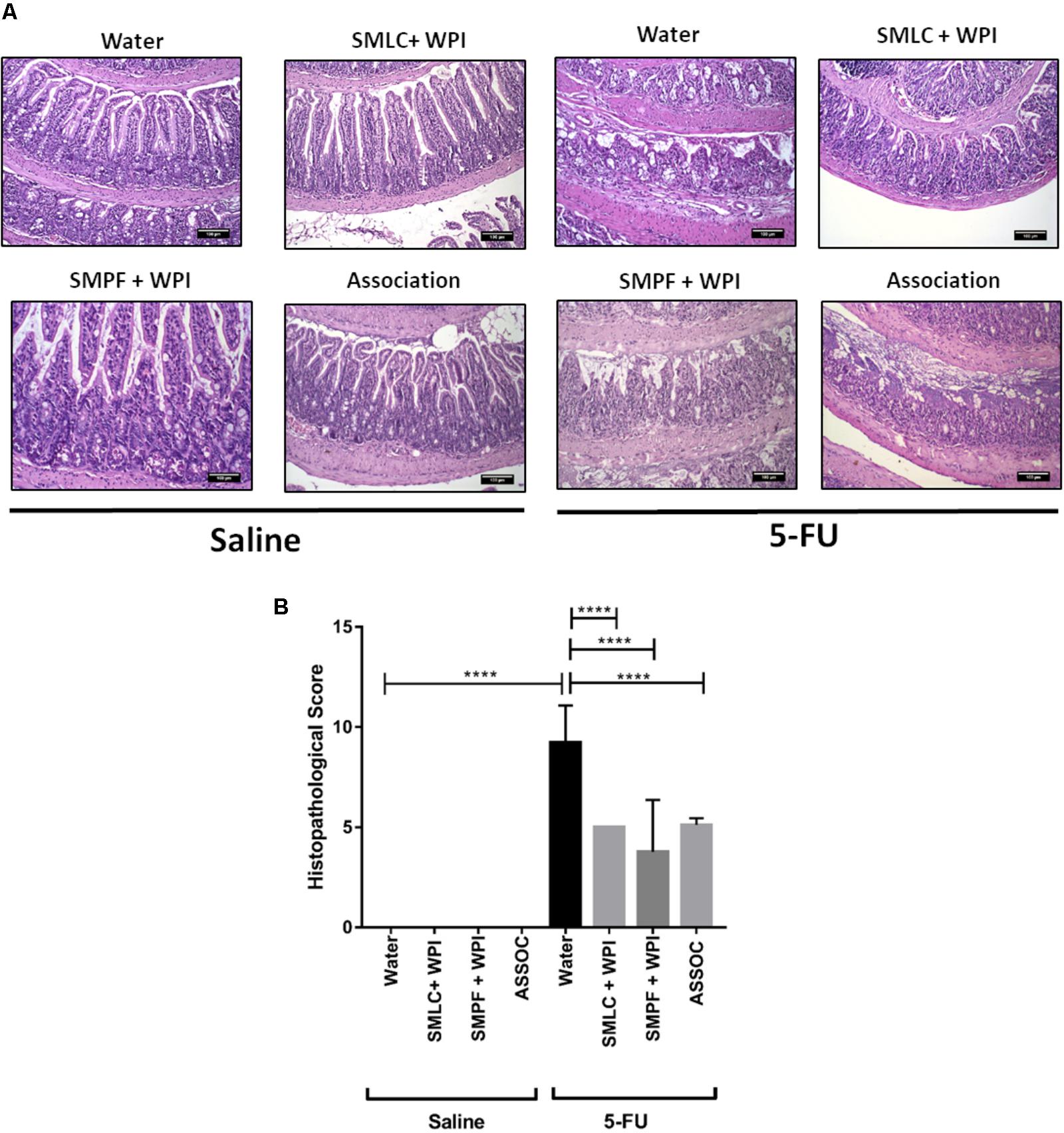
FIGURE 8. Association of skim milk fermented by L. casei BL23 and skim milk fermented by P. freudenreichii 138 does not provide additional effect in prevents mucosal damage. (A) Representative H&E-stained images from mucosal histopathology and (B) histopathological score obtained in the animals treated with different beverages. The image acquisition phase was done with a 20× magnification objective. Scale bar = 100 μm. Same control groups were used for all experimental probiotic assays. Asterisks represent statistically significant differences as follows: ∗p < 0.05, ∗∗p < 0.01, ∗∗∗p < 0.001, ∗∗∗∗p < 0.0001.
Treatment With Probiotic Beverages Prevented Villus Shortening and Degeneration of Goblet Cells
Morphometric analysis was carried out to evaluate epithelial integrity. A decrease in villus height and in crypt depth was observed in mice after 5-FU injection (66 μm) (Figure 9). Treatment with probiotic beverages showed increased villus height, especially in groups treated with SMLC + WPI + BL23 (129.2 μm) (Figure 9A) and SMPF + 138 (176.04 μm) (Figure 9C). No difference was found in crypt depths either in treated or untreated mice. As expected, the mucositis induction resulted in substantial decrease in goblet cells number (9.08 goblet cell/hpf) (Figure 10) when compared to the groups injected with 0.9% saline (51.4 goblet cell/hpf). In the other hand, administration of probiotics beverages prevented the degeneration of goblet cells in the mice ileum. The highest goblet cell count was found in mice treated with SMLC + WPI + BL23 (34.7 goblet cell/hpf) (Figure 10A) and SMPF + 138 (27.9 goblet cell/hpf) (Figure 10B).
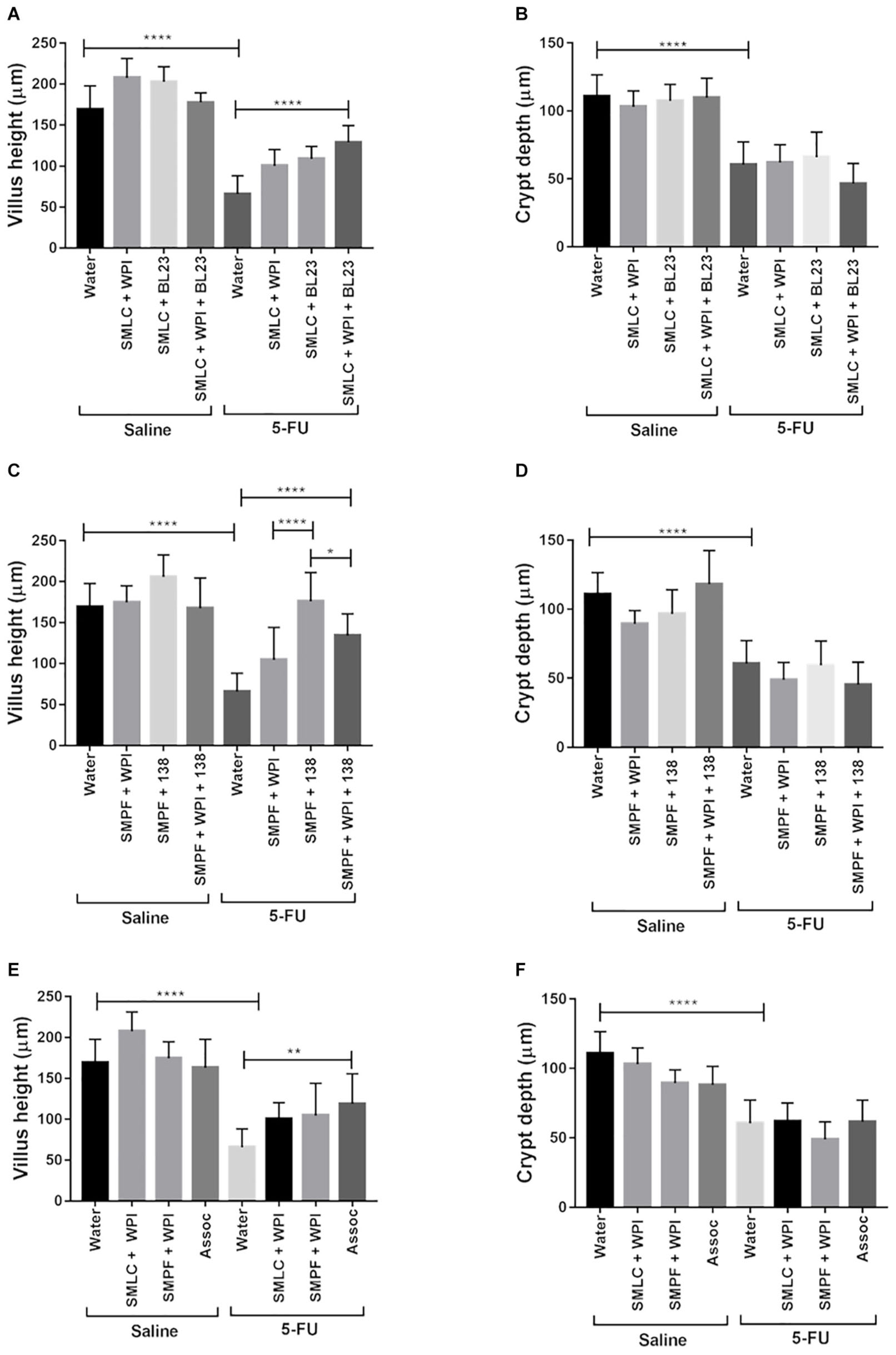
FIGURE 9. Administration of probiotic beverages improves villus architecture. Morphometric analysis of villus height and crypt depth of animals treated with (A,B) beverages fermented by L. casei BL23; (C,D) beverages fermented by P. freudenreichii 138 or (E,F) beverages fermented by the association of both bacteria following 5-FU or saline administration. Values were obtained by measuring ten random images of the ileum of mice. N = 6, 9. Asterisks represent statistically significant differences as follows: ∗p < 0.05, ∗∗p < 0.01, ∗∗∗p < 0.001, ∗∗∗∗p < 0.0001.
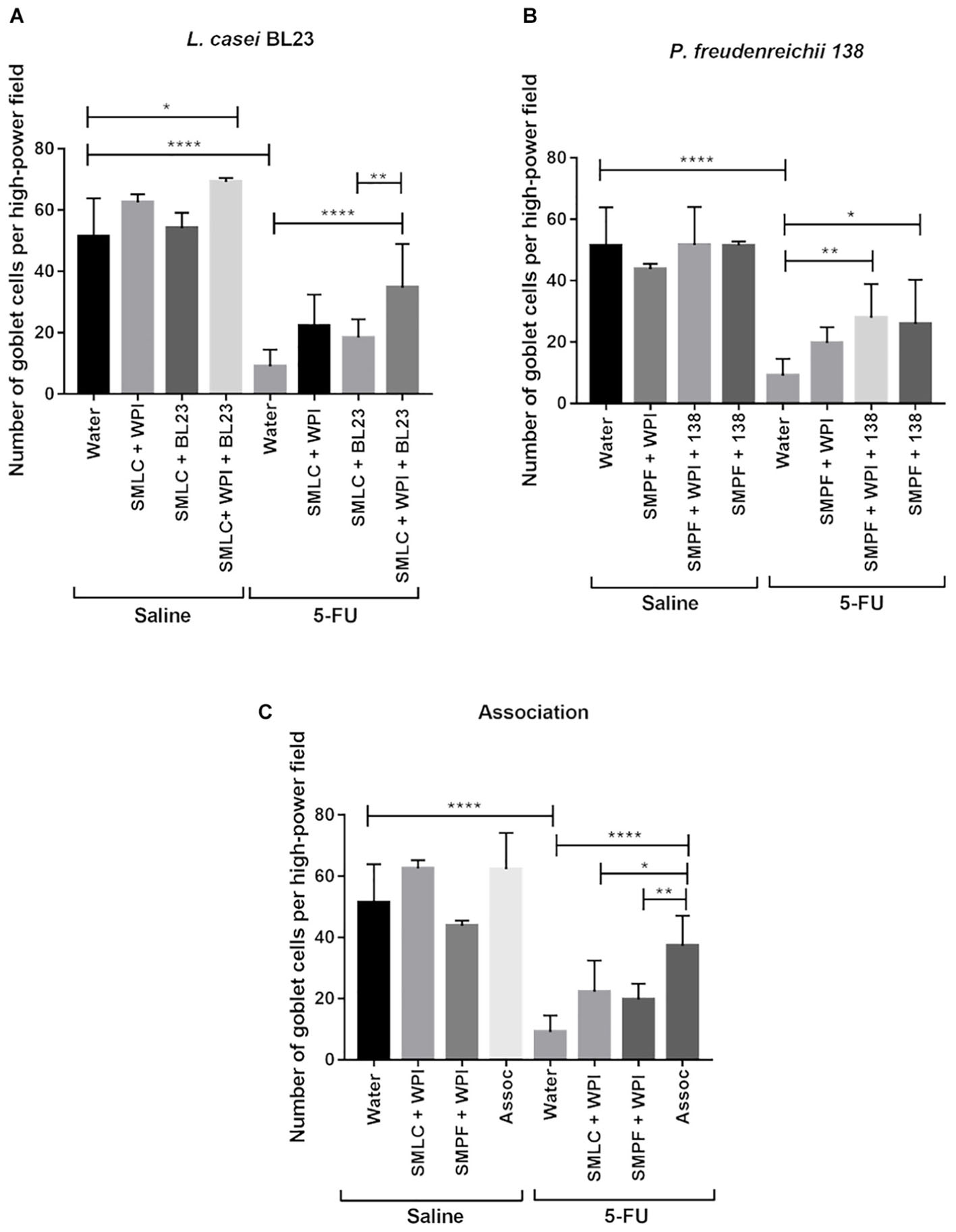
FIGURE 10. Administration of probiotic beverages prevented the marked degeneration of goblet cells in the mice ileum. Quantification of intact goblet cells in the animals ileum treated with (A) beverages fermented by L. casei BL23; (B) beverages fermented by P. freudenreichii 138 or (C) beverages fermented by the association of both bacteria following 5-FU or saline administration. Values were obtained by counting intact cells in ten random field images of mice. Results were expressed as means ± standard deviation. N = 6–9. Asterisks represent statistically significant differences as follows: ∗p < 0.05, ∗∗p < 0.01, ∗∗∗p < 0.001, ∗∗∗∗p < 0.0001.
Administration of Probiotic Beverages Did Not Change the Secretory IgA Production
Figure 11 indicates the concentration of IgA secreted in the small intestine of healthy animals or after induction of 5-FU mucositis, treated or not with L. casei BL23, with P. freudenreichii 138, or with the association of the two strains. Our results showed that there was no significant difference across the groups evaluated in this study.
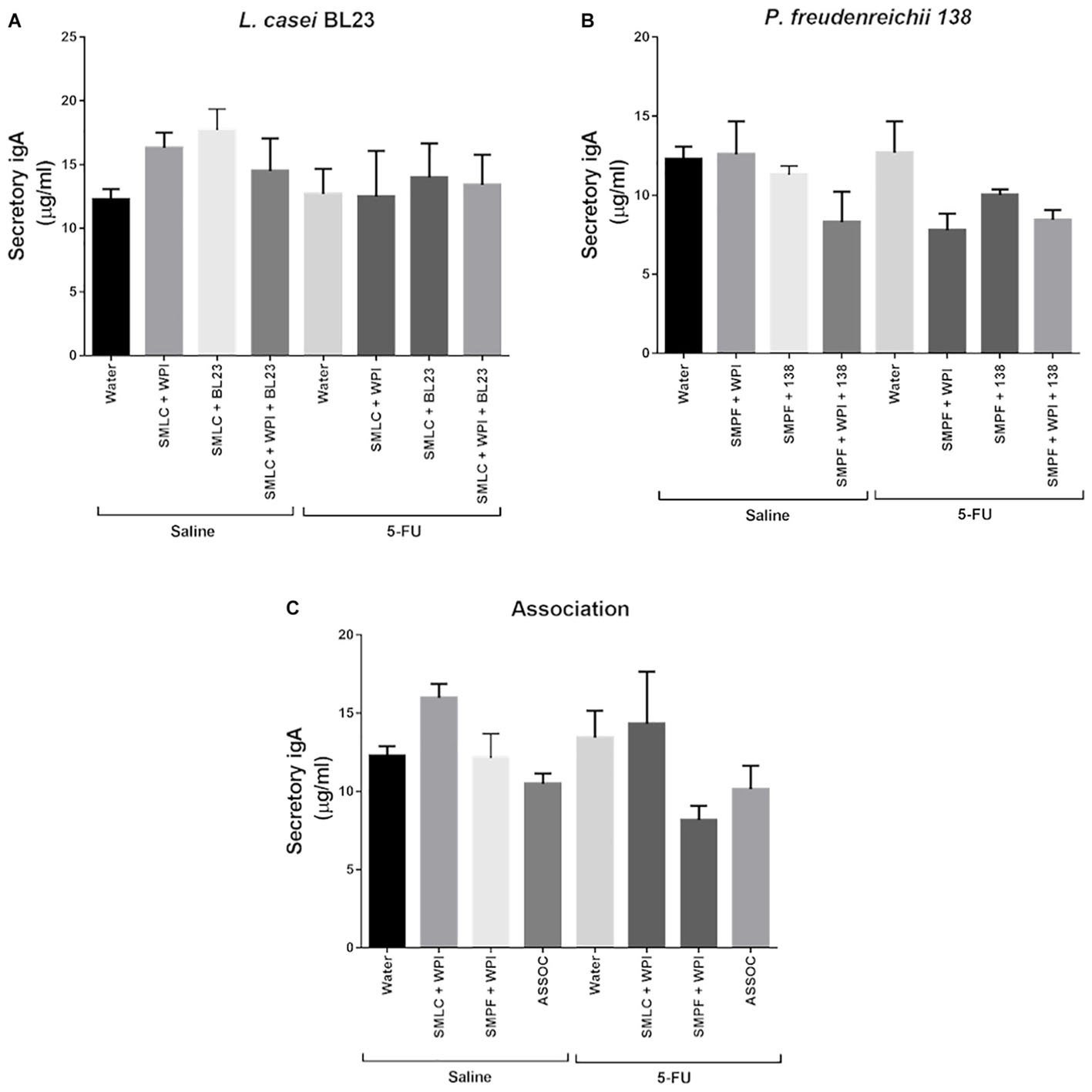
FIGURE 11. Administration of probiotic beverages did not alters the secretory IgA levels. Quantification of immunoglobulin A secretion (sIgA) in the small intestine of healthy or inflammation mice, treated for 14 days with (A) L. casei BL23 (B) P. freudenreichii 138, or (C) association of L. casei BL23 and P. freudenreichii 138. Results were expressed as means ± standard deviation. N = 6–9.
Discussion
Mucositis is a gastrointestinal inflammation that affects the quality of life of patients undergoing malignancy treatments (Sonis, 2004). Currently, classical therapies available for the prevention and treatment of the disease are not very effective and therefore new options have been suggested, such as the use of probiotic bacteria (Carvalho et al., 2017a). Several of these probiotics required the addition of protective matrices, in order to confer a protection via an efficient delivery of probiotic bacteria to the GIT, and enhance the therapeutics effects in the disease context (Carmo et al., 2017). The present study investigated the effects of whey protein isolate as a protective matrix for two bacterial strains and study the probiotic potential of these fermented beverages in a mucositis mice model induced by 5-FU.
Our data show that the addition of whey protein isolate boosted the growth of L. casei BL23 and of P. freudenreichii 138. This result may be due to a larger amount of nutrients provided by milk constituents and by WPI in the beverages, including carbohydrate and nitrogen sources, as they are essential for the energy metabolism of both L. casei BL23 and P. freudenreichii 138 (Cousin et al., 2011; Pessione, 2012). This result corroborates previous studies showing that other bacteria experienced enhanced growth as a result of whey proteins addition (Almeida et al., 2009; Skryplonek and Jasińska, 2015; Huang et al., 2016b; Baruzzi et al., 2017).
Due the fact that the products to be considered as a probiotic must be in sufficient quantities of viable bacteria in your local of action (Cousin et al., 2012a; Rabah et al., 2017), we decided to investigate the efficiency of WPI as a protective matrix for bacteria. For this, we evaluated the survival rate of L. casei BL23 and of P. freudenreichii 138 strains in environments that simulated stomach pH (pH 2), in the presence of bile salts in GIT and at high temperature. Our results demonstrated that the tolerance of L. casei BL23 and of P. freudenreichii 138 to these stressing conditions was significantly higher in the presence of WPI. Therefore, beverages containing WPI also showed a high viable cell counts after these stresses. Thus, skim milk supplemented with WPI is an effective matrix for the two strains used in this work, considering acid, bile salts and high-temperature stresses. Similar results were described by Vargas et al. (2015), who demonstrated that the probiotic strains Streptococcus thermophilus and L. bulgaricus presented a higher tolerance to the same stress conditions in the presence of WPI in the culture medium (Vargas et al., 2015). Cousin et al. (2012b) have also shown that Propionibacterium strains survived better in acid and biliary stresses when included in a dairy matrix (Cousin et al., 2012b). In a study by Huang et al. (2016b) was also demonstrated that the P. freudenreichii CIRM-BIA 129 tolerates simulated GIT stresses when included in hyperconcentrated sweet whey (Huang et al., 2016b). Studies suggest that dairy proteins protect probiotic bacteria via a process called coacervation. In this process, the proteins form microspheres that pack the microorganism inside, thus forming a kind of barrier that protects them from adverse environmental conditions (Silva et al., 2015; Coghetto et al., 2016).
The current definition of probiotic stipulates that microorganisms should be consumed alive (WHO, 2002; Hill et al., 2014). Thus, the survival of bacteria at low temperatures is an important parameter for the development of an effective probiotic product and fermented dairy products are generally stored at 4°C (Cousin et al., 2012b). Both bacteria tested in this work remained viable during at least 90 days at 4°C when cultured in skim milk supplemented with WPI, reaching up to 109 CFU mL-1, after 90 days. Furthermore, it is possible to suggest that these bacteria were also metabolically active as a pH decrease was observed, due to production of lactic acid and propionic acid (main fermentation products of L. casei and P. freudenreichii, respectively) during cold storage. In addition, stress tolerance of L. casei BL23 and of P. freudenreichii 138 was maintained upon cold storage in skim milk plus WPI. Therefore, the amount of nutrients provided by milk plus WPI was sufficient to sustain survival of the bacteria over 90 days of cold storage, in accordance with previous reports (Cousin et al., 2012b; Vargas et al., 2015; Moslemi et al., 2016; Shori, 2016; Baruzzi et al., 2017).
In our probiotic beverages, WPI supplementation increases the bacteria’s survival rate to environmental stresses, which is an essential parameter for therapeutic effects (Rabah et al., 2017). Since, L. casei BL23 is able to reduce inflammation parameters in colitis model (Rochat et al., 2007; Watterlot et al., 2010), and P. freudenreichii 138 has been shown some probiotic effects in vitro and ex vivo model (Cousin et al., 2012a,b), which is interesting to check their potentials in other disease models. In this context, we tested whether the probiotic beverages were able to exert beneficial effects in mice submitted to experimental mucositis and whether the addition of WPI would enhance these probiotic effects. 5-FU treatment caused weight loss, shortening of intestinal villi and an inflammation of mucosa in mice, in accordance with the literature (Carvalho et al., 2017a). Moreover, probiotic beverages fermented by L. casei BL23 and P. freudenreichii 138 were able to decrease 5-FU-induced intestinal inflammation in BALB/c mice, with preservation of the mucosal integrity and reduced weight loss. Same results were observed by oral administration of Simbioflora®, that containing L. paracasei, L. rhamnosus, L. acidophilus and Bifidobacterium lactis plus fructooligosaccharide in a 5-FU-mucositis mice model and in a treatment using a probiotic mixture, named VSL#3, in mucositis model induced by Irinotecan in rats (Bowen et al., 2007; Trindade et al., 2018). Our study also shows that addition of WPI improved L. casei BL23 beneficial effects in the ileum, but not for P. freudenreichii 138.
Another important feature evaluated in this study was the number of goblet cells throughout the tissue. Goblet cells are responsible for producing a layer of mucus that covers the entire surface of the intestinal epithelium and is mainly composed of high molecular weight glycoproteins known as mucins (Johansson et al., 2013). This mucus prevents the direct adhesion of microorganisms to the epithelium and their translocation to the internal layers of the intestine, besides being important for the lubrication of the intestinal walls and for the protection of the epithelium against digestive acidic fluids and toxins (Kim and Khan, 2013). Previous studies have described that the intestine cells need a series of amino acids, mainly threonine, cysteine, and serine, for the synthesis of this mucus in healthy conditions (Faure et al., 2006). However, during inflammatory processes such as mucositis, a superactivation of the goblet cells occurs, aiming to increase the protection of the epithelium damaged by the inflammatory process (Stringer et al., 2007, 2009a). Consequently, the requirement for amino acids by the cells is increased. However, these amino acids are usually insufficient during the inflammation, compromising adequate mucus barrier functioning (Stringer et al., 2009b). The demand for threonine, cysteine, and serine can be adequately supplied by the diet in order to increase the availability of these amino acids (Faure et al., 2006). WPI used in this study is rich in these three amino acids. Our probiotic beverages prevented the degeneration of goblet cells, suggesting that the presence of milk and WPI may have increased the availability of these amino acids, increasing the production of mucus and consequently improving the framework of protection and tissue repair observed in the histological analyses. Similar results were shown in a probiotic treatment with Saccharomyces cerevisiae UFMG A-905 in a murine model of irinotecan-induced mucositis (Bastos et al., 2016), as well as in a mice treated with a mixture of L. acidophilus and Bifidobacterium bifidum in a 5-FU-induced intestinal mucositis model (Yeung et al., 2015). Prisciandaro et al. (2011) also shown that a Escherichia coli Nissle 1917 (EcN) probiotic derived supernatants was able to partially maintained acidic-mucin producing goblet cells in the jejunum and neutral mucin producing goblet cells in the ileum, in 5-FU mucositis model in mice (Prisciandaro et al., 2011). Furthermore, due to the capacity to preserve globet cells and consequently to maintenance of mucin production, this is possible that adhesion of L. casei BL23 and P. freudenreichii 138 strains to the intestinal epithelial cells can be enhanced in mouse GIT, leading to an increase the probiotic therapeutic effect (Ouwehand and Salminen, 2003).
In summary, our results indicate that the developed probiotic beverages have anti-inflammatory effects in mucositis. Thus, we sought to investigate the role of IgA in the regulation of inflammatory conditions in these mice. IgA is the main antibody type found in mucosal secretions (Pabst et al., 2016) and has many important functions such as modulation of intestinal microbiota and mucosal protection against invading pathogens (Lycke and Bemark, 2017). These functions are naturally important in intestinal mucositis because this disease is associated with dysfunctions related to imbalances in the intestinal microbial community (Clemente et al., 2012). Destruction of the physical barrier that covers the GIT facilitates the invasion of pathogens from the lumen (Bischoff et al., 2014). However, none of the probiotic beverages used in this study was able to alter IgA production, ruling out a probiotic effect via stimulation of sIgA. Accordingly, administration of another probiotic species, Lactococcus lactis NCDO, in a DSS-induced colitis model, does not enhance the levels of sIgA (Luerce et al., 2014).
Conclusion
We have demonstrated that the supplementation of skim milk with 30% of whey protein isolate is a good matrix to provide protection for the L. casei BL23 and for P. freudenreichii 138 against environmental stresses. Furthermore, both probiotic beverages developed here were efficient in preventing mucositis induced by 5-Fluorouracil in BALB/c mice. L. casei BL23 protective effect was further enhanced by the addition of WPI. The benefits of adding WPI for the prevention of mucositis thus depends on the bacterial strain used.
Author Contributions
BC performed the in vitro analysis, animal experimentation regarding mucositis pre-treatment with the probiotic strains, interpreted the data regarding the immunological parameters that were assessed, and was a major contributor in the writing of the manuscript. EO and BS were major contributors in the animal experimentation. EF and SS performed, analyzed, and interpreted the histological analysis from ileum slides. JA analyzed and interpreted the morphometric analysis. LA performed the in vitro analysis and data interpretation. LL and HA performed, analyzed, and interpreted the secretory IgA quantification assay. AF, AV, LG, and VA contributed to the data interpretation and were major contributors in the writing of the manuscript. GJ and YLL were responsible for ceding the strains, contributed to the data interpretation, and were major contributors in the writing of the manuscript. RC and FC have contributed equally in the supervision, performing experiments, analysis and interpretation of the immunological data, and were major contributors equally in the writing of the manuscript.
Funding
This work was supported by Fundação de Amparo à Pesquisa do Estado de Minas Gerais (FAPEMIG), Conselho Nacional de Desenvolvimento Científico e Tecnológico (CNPq), and Coordenação de Aperfeiçoamento de Pessoal de Nível Superior (CAPES).
Conflict of Interest Statement
The authors declare that the research was conducted in the absence of any commercial or financial relationships that could be construed as a potential conflict of interest.
Acknowledgments
The authors thank Prof. Simone Vasconcelos Generoso (UFMG), Prof. Aristoteles Goes Neto (UFMG) for expert technical assistance, and useful discussions and advices.
References
Almeida, K. E., Tamime, A. Y., and Oliveira, M. N. (2009). Influence of total solids contents of milk whey on the acidifying profile and viability of various lactic acid bacteria. LWT Food Sci. Technol. 42, 672–678. doi: 10.1016/j.lwt.2008.03.013
Antunes, M. M., Leocádio, P. C. L., Teixeira, L. G., Leonel, A. J., Cara, D. C., Menezes, G. B., et al. (2016). Pretreatment with l-citrulline positively affects the mucosal architecture and permeability of the small intestine in a murine mucositis model. J. Parenter. Enter. Nutr. 40, 279–286. doi: 10.1177/0148607114567508
Baruzzi, F., de Candia, S., Quintieri, L., Caputo, L., and De Leo, F. (2017). Development of a synbiotic beverage enriched with bifidobacteria strains and fortified with whey proteins. Front. Microbiol. 8:640. doi: 10.3389/fmicb.2017.00640
Bastos, R. W., Pedroso, S. H. S. P., Vieira, A. T., Moreira, L. M. C., França, C. S., Cartelle, C. T., et al. (2016). Saccharomyces cerevisiae UFMG A-905 treatment reduces intestinal damage in a murine model of irinotecan-induced mucositis. Benef. Microbes 7, 549–558. doi: 10.3920/BM2015.0190
Bischoff, S. C., Barbara, G., Buurman, W., Ockhuizen, T., Schulzke, J., Serino, M., et al. (2014). Intestinal permeability – a new target for disease prevention and therapy. BMC Gastroenterol. 14:189. doi: 10.1186/s12876-014-0189-7
Bowen, J. M., Stringer, A. M., Gibson, R. J., Yeoh, A. S., Hannam, S., and Keefe, D. M. (2007). VSL#3 probiotic treatment reduces chemotherapy-induced diarrhoea and weight loss. Cancer Biol. Ther. 6, 1445–1450. doi: 10.4161/cbt.6.9.4622
Carmo, F. L. R., Rabah, H., Cordeiro, B., Silva, S., Jan, G., Azevedo, V. A., et al. (2017). “Applications of probiotic bacteria and dairy foods in health,” in Current Research in Microbiology Applications, eds B. Sivasankari, G. Tomazetto, and M. Verma (Wilmington, DE: Open Access eBooks), 1–33.
Carvalho, R. D., Breyner, N., Garcia, Z. M., Rodrigues, N. M., Lemos, L., Maioli, T. U., et al. (2017a). Secretion of biologically active pancreatitis associated protein I ( PAP ) by genetically modified dairy Lactococcus lactis NZ9000 in the prevention of intestinal mucositis. Microb. Cell Fact. 16, 1–11. doi: 10.1186/s12934-017-0624-x
Carvalho, R. D., do Carmo, F. L. R., de Oliveira Junior, A., Langella, P., Chatel, J.-M., Bermúdez-Humarán, L. G., et al. (2017b). Use of wild type or recombinant lactic acid bacteria as an alternative treatment for gastrointestinal inflammatory diseases: a focus on inflammatory bowel diseases and mucositis. Front. Microbiol. 8:800. doi: 10.3389/fmicb.2017.00800
Chang, C. T., Ho, T. Y., Lin, H., Liang, J. A., Huang, H. C., Li, C. C., et al. (2012). 5-fluorouracil induced intestinal mucositis via nuclear factor-kB activation by transcriptomic analysis and in vivo bioluminescence imaging. PLoS One 7:e31808. doi: 10.1371/journal.pone.0031808
Ciorba, M., Hellemeier, C., Stenson, W., and Parikh, P. (2016). Probiotics to prevents gastrointestinal toxicity from cancer therapy: an interpretative review and call to action. Curr. Opin. Support. Palliat. Care 1848, 3047–3054. doi: 10.1016/j.bbamem.2015.02.010.Cationic
Clemente, J. C., Ursell, L. K., Parfrey, L., and Knight, R. (2012). The impact of the gut microbiota on human health: an integrative view. Cell 148, 1258–1270. doi: 10.1016/j.cell.2012.01.035
Coghetto, C. C., Brinques, G. B., and Ayub, M. A. Z. (2016). Probiotics production and alternative encapsulation methodologies to improve their viabilities under adverse environmental conditions. Int. J. Food Sci. Nutr. 67, 929–943. doi: 10.1080/09637486.2016.1211995
Cousin, F. J., Jouan-Lanhouet, S., Dimanche-Boitrel, M. T., Corcos, L., and Jan, G. (2012a). Milk fermented by Propionibacterium freudenreichii induces apoptosis of HGT-1 human gastric cancer cells. PLoS One 7:e31892. doi: 10.1371/journal.pone.0031892
Cousin, F. J., Louesdon, S., Maillard, M. B., Parayre, S., Falentin, H., Deutsch, S. M., et al. (2012b). The first dairy product exclusively fermented by Propionibacterium freudenreichii: a new vector to study probiotic potentialities in vivo. Food Microbiol. 32, 135–146. doi: 10.1016/j.fm.2012.05.003
Cousin, F. J., Jouan-Lanhouet, S., Theret, N., Brenner, C., Jouan, E., Le Moigne-Muller, G., et al. (2016). The probiotic Propionibacterium freudenreichii as a new adjuvant for TRAIL-based therapy in colorectal cancer. Oncotarget 7, 7161–7178. doi: 10.18632/oncotarget.6881
Cousin, F. J., Mater, D. D. G., Foligne, B., and Jan, G. (2011). Dairy propionibacteria as human probiotics: a review of recent evidence. Dairy Sci. Technol. 91, 1–26. doi: 10.1051/dst/2010032
Dąbrowska, A., Babij, K., Marek, S., and Chrzanowska, J. (2017). Viability and growth promotion of starter and probiotic bacteria in yogurt supplemented with whey protein hydrolysate during refrigerated storage. Postep. Hig. Med. Dosw. 71, 952–959. doi: 10.5604/01.3001.0010.5866
Eales, J., Gibson, P., Whorwell, P., Kellow, J., Yellowlees, A., Perry, R. H. J., et al. (2017). Systematic review and meta-analysis: the effects of fermented milk with Bifidobacterium lactis CNCM I-2494 and lactic acid bacteria on gastrointestinal discomfort in the general adult population. Ther. Adv. Gastroenterol. 10, 74–88. doi: 10.1177/1756283X16670075
Faure, M., Mettraux, C., Moennoz, D., Godin, J.-P., Vuichoud, J., Rochat, F., et al. (2006). Specific amino acids increase mucin synthesis and microbiota in dextran sulfate sodium-treated rats. J. Nutr. 136, 1558–1564. doi: 10.1093/jn/136.6.1558
Ferreira, A. A., Huang, S., Perrone,ÍT., Schuck, P., Jan, G., and Carvalho, A. F. (2017). Tracking Amazonian cheese microbial diversity: development of an original, sustainable, and robust starter by freeze drying/spray drying. J. Dairy Sci. 100, 6997–7006. doi: 10.3168/jds.2016-12418
Foligne, B., Nutten, S., Grangette, C., Dennin, V., Goudercourt, D., Poiret, S., et al. (2007). Correlation between in vitro and in vivo immunomodulatory properties of lactic acid bacteria. World J. Gastroenterol. 13, 236–243. doi: 10.3748/wjg.v13.i2.236
Gagnaire, V., Jardin, J., Rabah, H., Briard-Bion, V., and Jan, G. (2015). Emmental cheese environment enhances Propionibacterium freudenreichii stress tolerance. PLoS One 10:e0135780. doi: 10.1371/journal.pone.0135780
Hill, C., Guarner, F., Reid, G., Gibson, G. R., Merenstein, D. J., Pot, B., et al. (2014). Expert consensus document: the international scientific association for probiotics and prebiotics consensus statement on the scope and appropriate use of the term probiotic. Nat. Rev. Gastroenterol. Hepatol. 11, 506–514. doi: 10.1038/nrgastro.2014.66
Huang, S., Cauty, C., Dolivet, A., Le Loir, Y., Chen, X. D., Schuck, P., et al. (2016a). Double use of highly concentrated sweet whey to improve the biomass production and viability of spray-dried probiotic bacteria. J. Funct. Foods 23, 453–463. doi: 10.1016/j.jff.2016.02.050
Huang, S., Rabah, H., Jardin, J., Briard-Bion, V., Parayre, S., Maillard, M. B., et al. (2016b). Hyperconcentrated sweet whey, a new culture medium that enhances Propionibacterium freudenreichii stress tolerance. Appl. Environ. Microbiol. 82, 4641–4651. doi: 10.1128/AEM.00748-16
Johansson, M. E. V., Sjövall, H., and Hansson, G. C. (2013). The gastrointestinal mucus system in health and disease. Nat. Rev. Gastroenterol. Hepatol. 10, 352–361. doi: 10.1038/nrgastro.2013.35
Justino, P. F. C., Melo, L. F. M., Nogueira, A. F., Morais, C. M., Mendes, W. O., Franco, A. X., et al. (2015). Regulatory role of Lactobacillus acidophilus on inflammation and gastric dysmotility in intestinal mucositis induced by 5-fluorouracil in mice. Cancer Chemother. Pharmacol. 75, 559–567. doi: 10.1007/s00280-014-2663-x
Kim, J., and Khan, W. (2013). Goblet cells and mucins: role in innate defense in enteric infections. Pathogens 2, 55–70. doi: 10.3390/pathogens2010055
Lee, B., Tachon, S., Eigenheer, R. A., Phinney, B. S., and Marco, M. L. (2015a). Lactobacillus casei low-temperature, dairy-associated proteome promotes persistence in the mammalian digestive tract. J. Proteome Res. 14, 3136–3147. doi: 10.1021/acs.jproteome.5b00387
Lee, B., Yin, X., Griffey, S. M., and Marco, M. L. (2015b). Attenuation of colitis by Lactobacillus casei BL23 is dependent on the dairy delivery matrix. Appl. Environ. Microbiol. 81, 6425–6435. doi: 10.1128/AEM.01360-15
Leroy, F., and De Vuyst, L. (2014). Fermented food in the context of a healthy diet: how to produce novel functional foods? Curr. Opin. Clin. Nutr. Metab. Care 17, 574–581. doi: 10.1097/MCO.0000000000000108
Livney, Y. D. (2010). Milk proteins as vehicles for bioactives. Curr. Opin. Colloid Interface Sci. 15, 73–83. doi: 10.1016/j.cocis.2009.11.002
Longley, D. B., Harkin, D. P., and Johnston, P. G. (2003). 5-Fluorouracil: mechanisms of action and clinical strategies. Nat. Rev. Cancer 3, 330–338. doi: 10.1038/nrc1074
Luerce, T. D., Gomes-Santos, A. C., Rocha, C. S., Moreira, T. G., Cruz, D. N., Lemos, L., et al. (2014). Anti-inflammatory effects of Lactococcus lactis NCDO 2118 during the remission period of chemically induced colitis. Gut Pathog. 6, 1–11. doi: 10.1186/1757-4749-6-33
Lycke, N. Y., and Bemark, M. (2017). The regulation of gut mucosal IgA B-cell responses: recent developments. Mucosal Immunol. 10, 1361–1374. doi: 10.1038/mi.2017.62
Madureira, A. R., Pereira, C. I., Gomes, A. M. P., Pintado, M. E., and Xavier Malcata, F. (2007). Bovine whey proteins - overview on their main biological properties. Food Res. Int. 40, 1197–1211. doi: 10.1016/j.foodres.2007.07.005
Malik, A. C., Reinbold, G. W., and Vedamuthu, E. R. (1968). An evaluation of the taxonomy of Propionibacterium. Can. J. Microbiol. 14, 1185–1191. doi: 10.1139/m68-199
Moslemi, M., Mazaheri Nezhad Fard, R., Hosseini, S. M., Homayouni-Rad, A., and Mortazavian, A. M. (2016). Incorporation of Propionibacteria in fermented milks as a probiotic. Crit. Rev. Food Sci. Nutr. 56, 1290–1312. doi: 10.1080/10408398.2013.766584
Ouwehand, A. C., and Salminen, S. (2003). In vitro adhesion assays for probiotics and their in vivo relevance: a review. Microb. Ecol. Health Dis. 15, 175–184. doi: 10.1080/08910600310019886
Pabst, O., Cerovic, V., and Hornef, M. (2016). Secretory IgA in the coordination of establishment and maintenance of the microbiota. Trends Immunol. 37, 287–296. doi: 10.1016/j.it.2016.03.002
Pessione, E. (2012). Lactic acid bacteria contribution to gut microbiota complexity: lights and shadows. Front. Cell. Infect. Microbiol. 2:86. doi: 10.3389/fcimb.2012.00086
Plé, C., Richoux, R., Jardin, J., Nurdin, M., Briard-Bion, V., Parayre, S., et al. (2015). Single-strain starter experimental cheese reveals anti-inflammatory effect of Propionibacterium freudenreichii CIRM BIA 129 in TNBS-colitis model. J. Funct. Foods 18, 575–585. doi: 10.1016/j.jff.2015.08.015
Prisciandaro, L. D., Geier, M. S., Butler, R. N., Cummins, A. G., and Howarth, G. S. (2011). Probiotic factors partially improve parameters of 5-fluorouracil-induced intestinal mucositis in rats. Cancer Biol. Ther. 11, 671–677. doi: 10.4161/cbt.11.7.14896
Rabah, H., Rosa do Carmo, F., and Jan, G. (2017). Dairy Propionibacteria: versatile probiotics. Microorganisms 5:E24. doi: 10.3390/microorganisms5020024
Rochat, T., Bermúdez-Humarán, L., Gratadoux, J. J., Fourage, C., Hoebler, C., Corthier, G., et al. (2007). Anti-inflammatory effects of Lactobacillus casei BL23 producing or not a manganese-dependant catalase on DSS-induced colitis in mice. Microb. Cell Fact. 6, 1–10. doi: 10.1186/1475-2859-6-22
Shori, A. B. (2016). Influence of food matrix on the viability of probiotic bacteria: a review based on dairy and non-dairy beverages. Food Biosci. 13, 1–8. doi: 10.1016/j.fbio.2015.11.001
Silva, T. M., Rodrigues, L. Z., Codevilla, C. F., Silva, C., and Menezes, C. R. (2015). Coacervação complexa?: uma técnica para a encapsulação de probióticos. Ciência Nat. 37, 49–55. doi: 10.5902/2179-460X19714
Skryplonek, K., and Jasińska, M. (2015). Fermented probiotic beverages based on acid whey. Acta Sci. Pol. Technol. Aliment. 14, 397–405. doi: 10.17306/J.AFS.2015.4.39
Soares, P. M. G., Mota, J. M. S. C., Gomes, A. S., Oliveira, R. B., Assreuy, A. M. S., Brito, G. A. C., et al. (2008). Gastrointestinal dysmotility in 5-fluorouracil-induced intestinal mucositis outlasts inflammatory process resolution. Cancer Chemother. Pharmacol. 63, 91–98. doi: 10.1007/s00280-008-0715-9
Stringer, A. M. (2013). Interaction between host cells and microbes in chemotherapy-induced mucositis. Nutrients 5, 1488–1499. doi: 10.3390/nu5051488
Stringer, A. M., Gibson, R. J., Bowen, J. M., Logan, R. M., Ashton, K., Yeoh, A. S. J., et al. (2009a). Irinotecan-induced mucositis manifesting as diarrhoea corresponds with an amended intestinal flora and mucin profile. Int. J. Exp. Pathol. 90, 489–499. doi: 10.1111/j.1365-2613.2009.00671.x
Stringer, A. M., Gibson, R. J., Logan, R. M., Bowen, J. M., Yeoh, A. S. J., Hamilton, J., et al. (2009b). Gastrointestinal microflora and mucins may play a critical role in the development of 5-fluorouracil-induced gastrointestinal mucositis. Exp. Biol. Med. 234, 430–441. doi: 10.3181/0810-RM-301
Stringer, A. M., Gibson, R. J., Bowen, J. M., Logan, R. M., Yeoh, A. S.-J., and Keefe, D. M. K. (2007). Chemotherapy-induced mucositis: the role of gastrointestinal microflora and mucins in the luminal environment. J. Support. Oncol. 5, 259–267.
Tang, Y., Wu, Y., Huang, Z., Dong, W., Deng, Y., Wang, F., et al. (2017). Administration of probiotic mixture DM#1 ameliorated 5-fluorouracil induced intestinal mucositis and dysbiosis in rats. Nutrition 33, 96–104. doi: 10.1016/j.nut.2016.05.003
Tharmaraj, N., and Shah, N. P. (2003). Selective enumeration of Lactobacillus delbrueckii ssp. bulgaricus, Streptococcus thermophilus, Lactobacillus acidophilus, bifidobacteria, Lactobacillus casei, Lactobacillus rhamnosus, and propionibacteria. J. Dairy Sci. 86, 2288–2296. doi: 10.3168/jds.S0022-0302(03)73821-1
Trindade, L. M., Martins, V. D., Rodrigues, N. M., Souza, E. L. S., Martins, F. S., Costa, G. M. F., et al. (2018). Oral administration of Simbioflora® (synbiotic) attenuates intestinal damage in a mouse model of 5-fluorouracil-induced mucositis. Benef. Microbes 9, 477–486. doi: 10.3920/BM2017.0082
Vargas, L. A., Olson, D. W., and Aryana, K. J. (2015). Whey protein isolate improves acid and bile tolerances of Streptococcus thermophilus ST-M5 and Lactobacillus delbrueckii ssp. bulgaricus LB-12. J. Dairy Sci. 98, 2215–2221. doi: 10.3168/jds.2014-8869
Watterlot, L., Rochat, T., Sokol, H., Cherbuy, C., Bouloufa, I., Lefèvre, F., et al. (2010). Intragastric administration of a superoxide dismutase-producing recombinant Lactobacillus casei BL23 strain attenuates DSS colitis in mice. Int. J. Food Microbiol. 144, 35–41. doi: 10.1016/j.ijfoodmicro.2010.03.037
WHO (2002). Guidelines for the Evaluation of Probiotics in Food. London: World Health Organization, 1–11. doi: 10.1111/j.1469-0691.2012.03873
Yadav, J. S. S., Yan, S., Pilli, S., Kumar, L., Tyagi, R. D., and Surampalli, R. Y. (2015). Cheese whey: a potential resource to transform into bioprotein, functional/nutritional proteins and bioactive peptides. Biotechnol. Adv. 33, 756–774. doi: 10.1016/j.biotechadv.2015.07.002
Keywords: Lactobacillus, mucositis, probiotics, Propionibacterium, stress tolerance, whey protein isolate
Citation: Cordeiro BF, Oliveira ER, da Silva SH, Savassi BM, Acurcio LB, Lemos L, Alves JL, Assis HC, Vieira AT, Faria AMC, Ferreira E, Le Loir Y, Jan G, Goulart LR, Azevedo V, Carvalho RDO and do Carmo FLR (2018) Whey Protein Isolate-Supplemented Beverage, Fermented by Lactobacillus casei BL23 and Propionibacterium freudenreichii 138, in the Prevention of Mucositis in Mice. Front. Microbiol. 9:2035. doi: 10.3389/fmicb.2018.02035
Received: 08 May 2018; Accepted: 13 August 2018;
Published: 12 September 2018.
Edited by:
Aleš Berlec, Jožef Stefan Institute (IJS), SloveniaReviewed by:
Svetoslav Todorov, Universidade de São Paulo, BrazilVijendra Mishra, National Institute of Food Technology Entrepreneurship and Management, India
Copyright © 2018 Cordeiro, Oliveira, da Silva, Savassi, Acurcio, Lemos, Alves, Assis, Vieira, Faria, Ferreira, Le Loir, Jan, Goulart, Azevedo, Carvalho and do Carmo. This is an open-access article distributed under the terms of the Creative Commons Attribution License (CC BY). The use, distribution or reproduction in other forums is permitted, provided the original author(s) and the copyright owner(s) are credited and that the original publication in this journal is cited, in accordance with accepted academic practice. No use, distribution or reproduction is permitted which does not comply with these terms.
*Correspondence: Vasco Azevedo, vascoariston@gmail.com
†These authors have contributed equally to senior authorship