- 1Department of Clinical Laboratory, The Second Affiliated Hospital of Soochow University, Suzhou, Jiangsu, China
- 2Department of Clinical Laboratory, The First Affiliated Hospital of Kunming Medical University, Kunming, China
- 3Center for Clinical Laboratory, 302 Hospital of PLA, Beijing, China
- 4Aviation General Hospital, China Medical University, Beijing, China
- 5Medical Laboratory Center, General Hospital of Ningxia Medical University, Yinchuan, China
- 6Department of Laboratory Medicine, The First Affiliated Hospital, Sun Yat-sen University, Guangzhou, China
- 7Sichuan Academy of Medical Sciences Sichuan Provincial People’s Hospital, Chengdu, China
- 8Department of Laboratory Medicine, Memorial Sloan Kettering Cancer Center, New York, NY, United States
- 9Department of Pathology and Laboratory Medicine, Weill Cornell Medicine, Cornell University, New York, NY, United States
- 10Cepheid, Shanghai, China
- 11Center for Discovery and Innovation, Hackensack Meridian Health, Nutley, NJ, United States
- 12Hackensack Meridian School of Medicine at Seton Hall University, Nutley, NJ, United States
KPC-producing Klebsiella pneumoniae (KPC-KP) is the most widely spread carbapenem-resistant Enterobacteriaceae (CRE) in China. Avibactam is a novel non-β-lactam β-lactamase inhibitor which is highly active against KPC. Recently, ceftazidime-avibactam (CAZ-AVI) was approved for clinical treatment in China. Here we conducted a retrospective study to examine the antimicrobial susceptibility of CAZ-AVI prior to its usage in China, and evaluated the potential to develop resistance in KPC-KP. CAZ-AVI MICs were tested in 347 KPC-KP isolates collected from patients with no prior treatment with this combination from six medical centers in China. Almost all isolates (n = 346; 99.7%) were CAZ-AVI-susceptible, with only 12 (3.5%) which showed reduced susceptibility (MIC ≥ 4/4 μg/ml) or resistance. The 12 isolates belong to ST11 and half of them carry virulence genes. In comparison to susceptible isolates, these isolates demonstrated higher blaKPC–2 copy numbers and expressions, and demonstrated higher frequency of developing CAZ-AVI resistance.
Introduction
With the widely use of carbapenem antibiotics, carbapenem-resistant Enterobacteriaceae (CRE) have been increasingly detected worldwide. The production of carbapenemases is the leading cause of carbapenem resistance in CRE. Klebsiella pneumoniae carbapenemase (KPC) is currently the most widely spread carbapenemase in the world, including China (Nordmann and Poirel, 2014), while K. pneumoniae is the main clinical species producing KPC (Zhang et al., 2017). KPC-type β-lactamases could hydrolyze carbapenems and almost all β-lactam antibiotics, and traditional β-lactamase inhibitors have limited effects on KPC (Papp-Wallace et al., 2010). In addition, isolates producing KPC are commonly resistant to many other clinical agents (Pollett et al., 2014) due to the co-expression of several resistant determinants. Novel treatments for infections caused by KPC-producing K. pneumoniae (KPC-KP) are in urgent need.
Avibactam, a novel non-β-lactam β-lactamase inhibitor, had a spectrum of activity against β-lactamase of classes A (e.g., KPC), C (AmpC), and selected D (e.g., OXA-48) enzymes (van Duin and Bonomo, 2016). The combination of ceftazidime-avibactam (CAZ-AVI) has been approved in clinical treatment for KPC-producing Enterobacteriaceae by the United States Food and Drug Administration (FDA) in 2015 (FDA, 2015). In China, injectable CAZ-AVI (ZAVICEFTA®) was recently (May 2019) approved for the treatment of complicated intra-abdominal infections (cIAI) and hospital-acquired pneumonia (HAP), including ventilator associated pneumonia (VAP), caused by multidrug resistant Gram-negative bacteria.
In this study, we examined the CAZ-AVI susceptibility of KPC-KP from patients with no prior CAZ-AVI treatment history in China and evaluated the potential of isolates with reduced susceptibility to this combination to develop resistance through in vitro selection experiments.
Materials and Methods
Collection and Identification of Carbapenem-Resistant K. pneumoniae
A total of 616 unique clinical K. pneumoniae displaying carbapenem resistance which were defined as any isolate displaying imipenem and/or meropenem MIC values of >2 μg/ml based on CLSI guideline (Clinical and Laboratory Standards Institute, 2018) were collected from September 2016 to June 2018 from six tertiary hospitals in six different cities in China including Chengdu (Southwest China), Kunming (Southwest China), Guangzhou (Southern China), Yinchuan (Northwest China), Suzhou (Eastern China), and Beijing (Northern China). Species identification was performed by matrix-assisted laser desorption ionization-time of flight mass spectrometry (MALDI-TOF MS) method using the Bruker Daltonics MALDI Biotyper according to the instructions of manufacturer, and confirmed by 16S rRNA sequencing (Weisburg et al., 1991).
Detection of Carbapenemases, ESBLs and Analysis of Porin Encoding Genes
Polymerase chain reaction (PCR) was performed to detect the presence of carbapenemase-encoding genes (blaKPC, blaNDM, blaIMP, blaVIM, blaOXA–48) (Candan and Aksoz, 2015), the extended-spectrum beta-lactamases (ESBLs) genes (blaCTX–M, blaSHV and blaTEM) (Bokaeian et al., 2015) and to amplify porin encoding genes (ompK35 and ompK36) (Clancy et al., 2013) in the carbapenem-resistant K. pneumoniae. The entire coding sequences for these genes were then amplified using previously published primers and conditions (Costa et al., 2009; Schechner et al., 2009) and subjected to Sanger sequencing.
Determination of CAZ-AVI Minimal Inhibitory Concentrations
The CAZ-AVI minimal inhibitory concentrations (MICs) were determined using the broth microdilution method recommended by CLSI (Clinical and Laboratory Standards Institute, 2018). Escherichia coli ATCC 25922 was used as quality control strain (Clinical and Laboratory Standards Institute, 2018). MICs were interpreted according to CLSI breakpoints (Clinical and Laboratory Standards Institute, 2018). A CAZ-AVI MIC of ≥4/4 μg/ml was used as the cut-off of reduced susceptibility to CAZ-AVI for this study (Shen et al., 2017).
Antimicrobial Susceptibility Testing
In vitro susceptibility of the isolates with reduced CAZ-AVI susceptibility against the commonly used clinical antimicrobials was evaluated by Phoenix 100 Automated Microbiology System and interpreted using CLSI (Clinical and Laboratory Standards Institute, 2018) guidelines or European Committee on Antimicrobial Susceptibility Testing (EUCAST) (for moxifloxacin and colistin). A total of 20 antibiotics belonging to 11 classes of antimicrobials were tested, including carbapenems (imipenem and meropenem), ureidopenicillin (piperacillin), β-lactam/β-lactamase inhibitor complexes (amoxicillin-clavulanate, ampicillin-sulbactam, and piperacillin-tazobactam), cephalosporins (cefazolin, cefotaxime, ceftazidime, and cefepime), monocyclic β-lactams (aztreonam), aminoglycosides (gentamicin and amikacin), fluoroquinolones (ciprofloxacin, moxifloxacin and levofloxacin), folate metabolic pathway inhibitors (trimethoprim-sulfamethoxazole), chloramphenicol, colistin, and tetracycline.
Collection of Clinical Information
The clinical information including city, age range, isolation date, clinical department, sample, medical condition and outcome of the patients from whom the carbapenem-resistant K. pneumoniae was isolated was collected using EPIINFO software based on the medical records.
Multilocus Sequence Typing (MLST)
Multilocus sequence typing (MLST) was conducted to investigate the genetic relationships of the isolates with reduced CAZ-AVI susceptibility. PCR followed by Sanger sequencing was used to detect conserved housekeeping including gapA, infB, mdh, pgi, phoE, rpoB, and tonB (Diancourt et al., 2005). Allelic profiles and sequence types (STs) were determined using the K. pneumoniae MLST database1.
Pulsed-Field Gel Electrophoresis (PFGE)
The clonal relatedness between the isolates with reduced CAZ-AVI susceptibility was investigated by pulsed-field gel electrophoresis (PFGE) with XbaI-digested DNA using a CHEF Mapper Power Module instrument (Bio-Rad, United States). Conditions of electrophoresis were as follow: voltage 6V/cm, running time 20 h, temperature 14°C, and pulse times of 1–40 s. Salmonella enterica serotype Braenderup H9812 was used as size marker. The results were evaluated using GelJ v.2.0 analyzing software.
Investigation of the Capsular Types and Virulence Genes
Multiplex PCR-II analysis was applied to investigate the capsular types including K1, K2, KL64, KL47 (Yu et al., 2018), and multiplex PCR-III analysis was performed to detect four virulence genes previously described on the pLVPK virulence plasmid namely rmpA, rmpA2, iroN, and iutA (Yu et al., 2018) in the isolates with reduced CAZ-AVI susceptibility.
Quantitative Real-Time PCR (qRT-PCR) and blaKPC Promoter Region Sequencing
A total of 16 isolates, including 8 isolates with reduced susceptibility and 8 randomly selected susceptible isolates, were examined by quantitative real-time PCR (qRT-PCR) to assess the blaKPC–2 copy numbers relative to an internal K. pneumoniae housekeeping gene, rpoB, as previously described (Kitchel et al., 2010). The expression of blaKPC–2 in the same isolate set was measured using DNA-free RNA preparations by qRT-PCR as described previously (Ruzin et al., 2005; Doumith et al., 2009). The statistical software used in this study was Prism 5 (Graph Pad Software). In addition, the blaKPC promoter regions from 12 isolates with reduced CAZ-AVI susceptibility and the 8 randomly selected susceptible isolates were amplified and sequenced using the primers (KPCpro-F, 5′-AACGGTCGTATCAGCGACAT-3′ and KPCpro-R, 5′-CGAGTTTAGCGAATGGTTCC-3′), covering the previously described promoter regions in KPC-KP isolates from China (Huang et al., 2019).
In vitro Selection Testing
All isolates underwent qRT-PCR detection, except for the CAZ-AVI (MIC 16/4 μg/ml) resistant isolate (n = 15), were subject to in vitro CAZ-AVI selective pressure testing, using a previously described method (Rodriguez-Villodres et al., 2020). The 15 strains included 7 isolates with reduced susceptibility (MIC ≥ 4/4 μg/ml) and 8 randomly selected susceptible isolates (see above). In brief, in vitro selection was performed by inoculation of ∼108 cfu in 2-ml LB broth containing CAZ-AVI at the 0.5 × MICs followed by incubation for 24 h. This procedure was repeated daily, each time doubling the CAZ-AVI concentration up to a maximum of 8/4 μg/ml. Selected colonies collected at different in vitro selection antibiotic levels were subject to CAZ-AVI susceptibility testing. The isolates with increased MICs ≥ 16/4 μg/ml after CAZ-AVI selection were determined as selected CAZ-AVI resistance.
Results
Distribution of the KPC-KP
The 616 non-duplicate clinical K. pneumoniae collected from September 2016 to June 2018 were from Chengdu (226, 36.7%, Southwest China), Kunming (148, 24.0%, Southwest China), Guangzhou (90, 14.6%, Southern China), Yinchuan (62, 10.1%, Northwest China), Suzhou (47, 7.6%, Eastern China), and Beijing (43, 7.0%, Northern China). In this collection 347 (56.3%) K. pneumoniae contained blaKPC, without coexistence of metallo-β-lactamases genes, the majority of which were from Kunming (119, 34.3%) followed by Chengdu (97, 28.0%), Guangzhou (49, 14.1%), Beijing (34, 9.8%), Suzhou (25, 7.2%), and Yinchuan (23, 6.6%) (Table 1).
Antimicrobial Susceptibility of KPC-KP
Minimal inhibitory concentrations of CAZ-AVI inhibiting KPC-KP ranged from ≤0.125/4 to 16/4 μg/ml, with only one strain which was resistant (16/4 μg/ml) according to the CLSI breakpoint (Clinical and Laboratory Standards Institute, 2018). The MIC50 was 1/4 μg/ml and MIC90 was 2/4 μg/ml (Table 1). We then used CAZ-AVI MIC of ≥4/4 μg/ml as the cut-off of reduced susceptibility to CAZ-AVI for this study (Shen et al., 2017). A total of 12 KPC-KP were found to have a CAZ-AVI MIC value ≥ 4/4 μg/ml with high level resistance to carbapenems (meropenem MICs ≥ 256 μg/ml) (Table 1).
These 12 isolates showed resistance to nearly all tested antimicrobials except trimethoprim-sulfamethoxazole (41.7%), chloramphenicol (16.7%), colistin (0%), and tetracycline (41.7%) (Table 2).
Clinical Information
The 12 isolates were obtained from distinct patients aged between 0 and 65 years old. They were from Beijing (n = 6), Kunming (n = 3), Guangzhou (n = 2), and Suzhou (n = 1). Four out of six Beijing isolates were collected from patients in ICU wards within the same month. In addition, most of these patients received empirical antibiotic treatments before these isolation of strains. Among them, carbapenems were the most commonly used antibiotic, followed by piperacillin-tazobactam (Table 3).
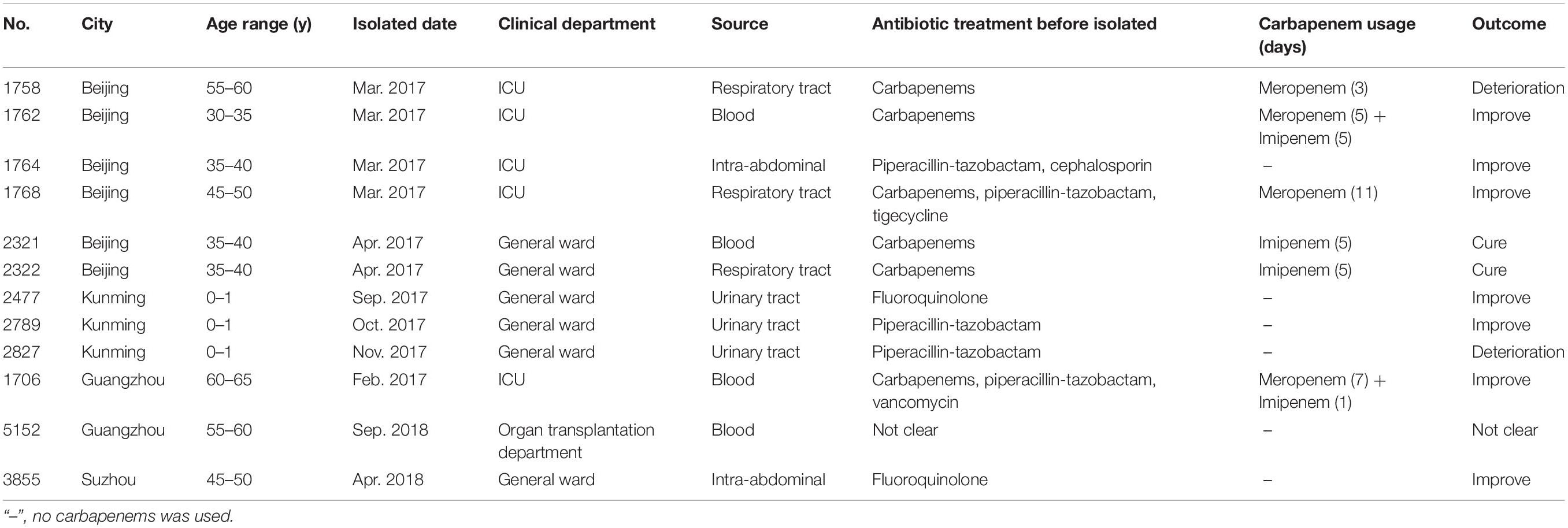
Table 3. Clinical information of the 12 patients connected with the isolates with reduced susceptibility to ceftazidime-avibactam.
Detection of ESBL and Virulence Genes and Capsular Genotyping
These 12 isolates contain ESBLs genes including blaCTX–M–65 (n = 9), blaCTX–M–14 (n = 3) and blaSHV–12 (n = 8). In addition, the results of multiplex PCR showed that 6 isolates from Beijing were positive for the four virulence genes, namely rmpA, rmpA2, iroN, and iutA. Eight isolates belonged to capsular type KL64 and the other 4 isolates were from KL47 (Table 4).
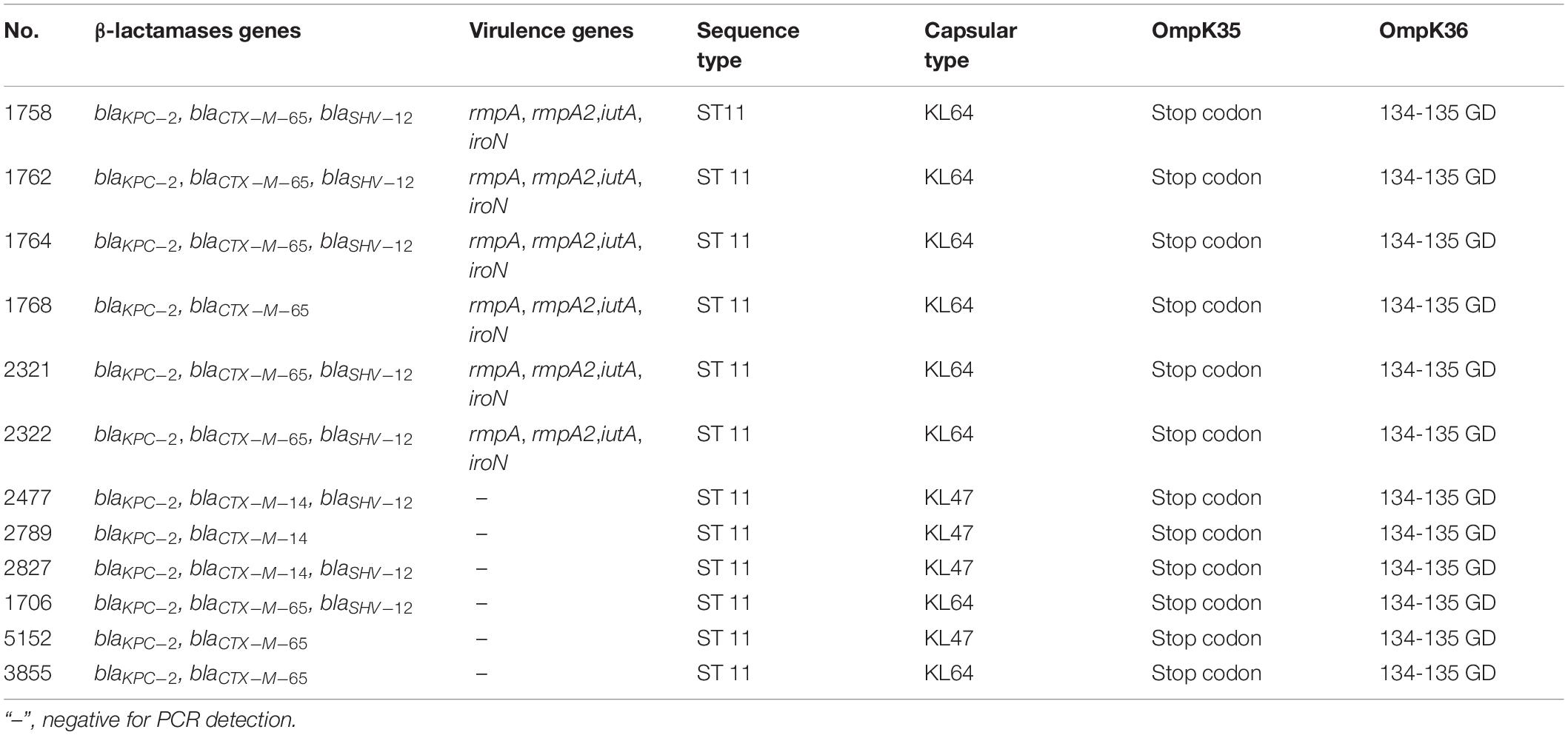
Table 4. Molecular characteristics of the 12 KPC-KP with reduced susceptibility to ceftazidime-avibactam.
MLST Sequence Types and PFGE Patterns
Multilocus sequence typing results showed that all 12 isolates belonged to ST11. Furthermore, these 12 isolates were investigated by PFGE. As shown in Figure 1, the 6 isolates from Beijing shared the same PFGE pattern. In addition, 2 of the 3 isolates from Kunming were highly homologous (>90%, dice similarity coefficient). The other isolates demonstrated different pulsotypes.
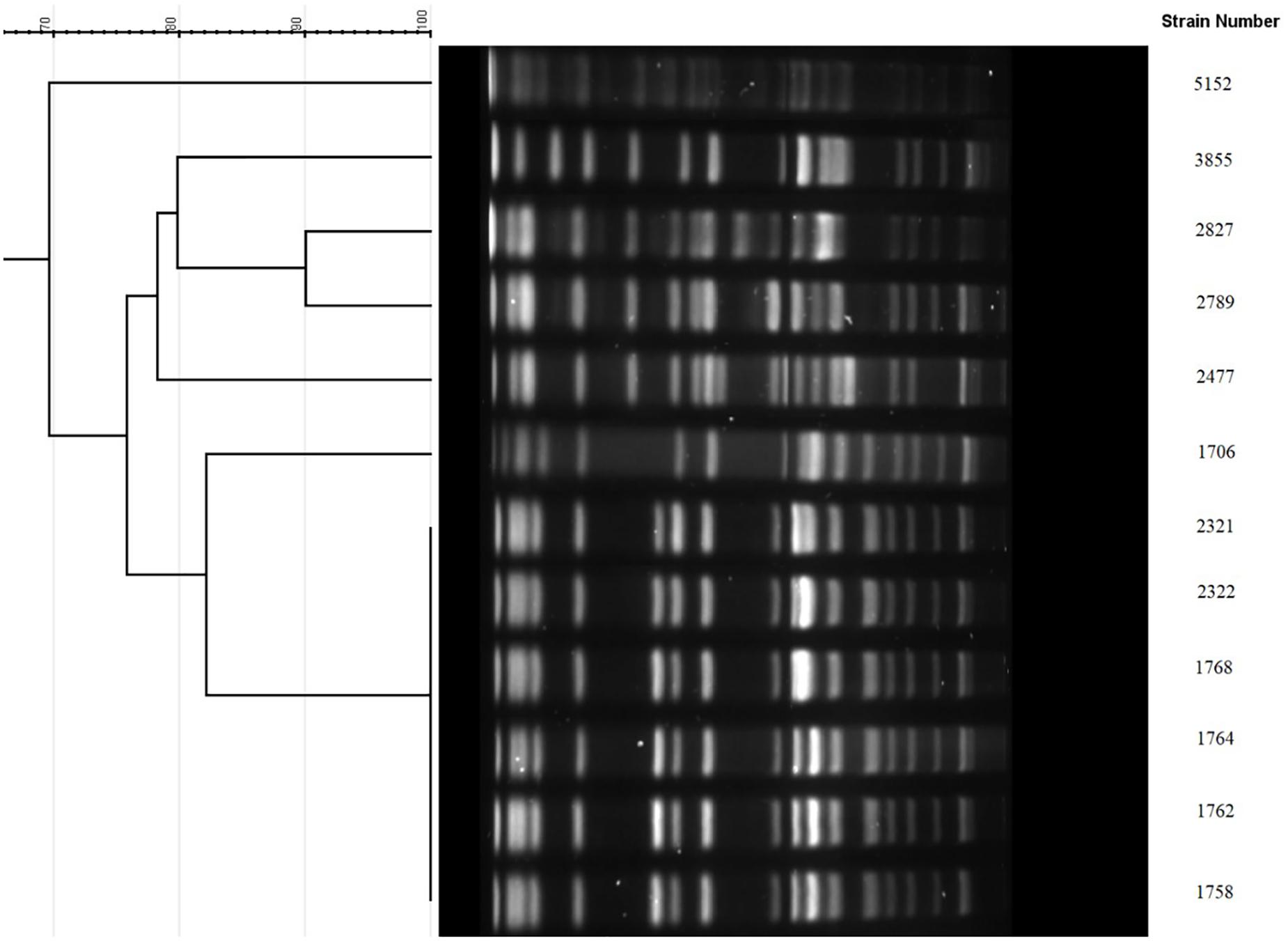
Figure 1. PFGE cluster analysis of the 12 KPC-KP with reduced susceptibility to ceftazidime-avibactam. 1758, 1762, 1764, 1768, 2321, and 2322 were from Beijing sharing the same PFGE pattern. 2477, 2789, and 2827 were from Kunming, among which 2789 and 2827 were highly homologous (>90%). 1706 and 5152 were from Guangzhou. 3855 was from Suzhou.
Outer Membrance Porin Gene Sequence Analysis
Sequencing of the outer membrane porin genes ompK35 and ompK36 showed that all 12 isolates contain a mutant OmpK35, with a premature stop codon at amino acid position 63, as well as a mutant OmpK36, due to the glycine and aspartic acid duplication at amino acid 134 (134–135 GD insertion). However, the OmpK35 and OmpK36 gene sequences of 8 randomly selected susceptible K. pneumoniae ST11 isolates showed the same genotypes (OmpK35 stop codon and OmpK36 134–135 GD insertion) as those of the 12 isolates with reduced CAZ/AVI susceptibility.
Mechanism of Reduced CAZ-AVI Susceptibility
Polymerase chain reaction detection and Sanger sequencing showed that the 12 isolates all harbored wild-type blaKPC–2. Examination of the blaKPC–2 promoter regions failed to identify any mutations in comparison to the sequences from the susceptible strains. The results of qRT-PCR showed that the relative blaKPC–2 copy numbers in the reduced susceptibility group were significantly higher than those in the susceptibility group (2.6-fold, P = 0.0004) (Figure 2A). In addition, the relative expressions of blaKPC–2 in the reduced susceptibility group were 3.9-fold higher than those in the susceptibility group (P = 0.0034) (Figure 2B).
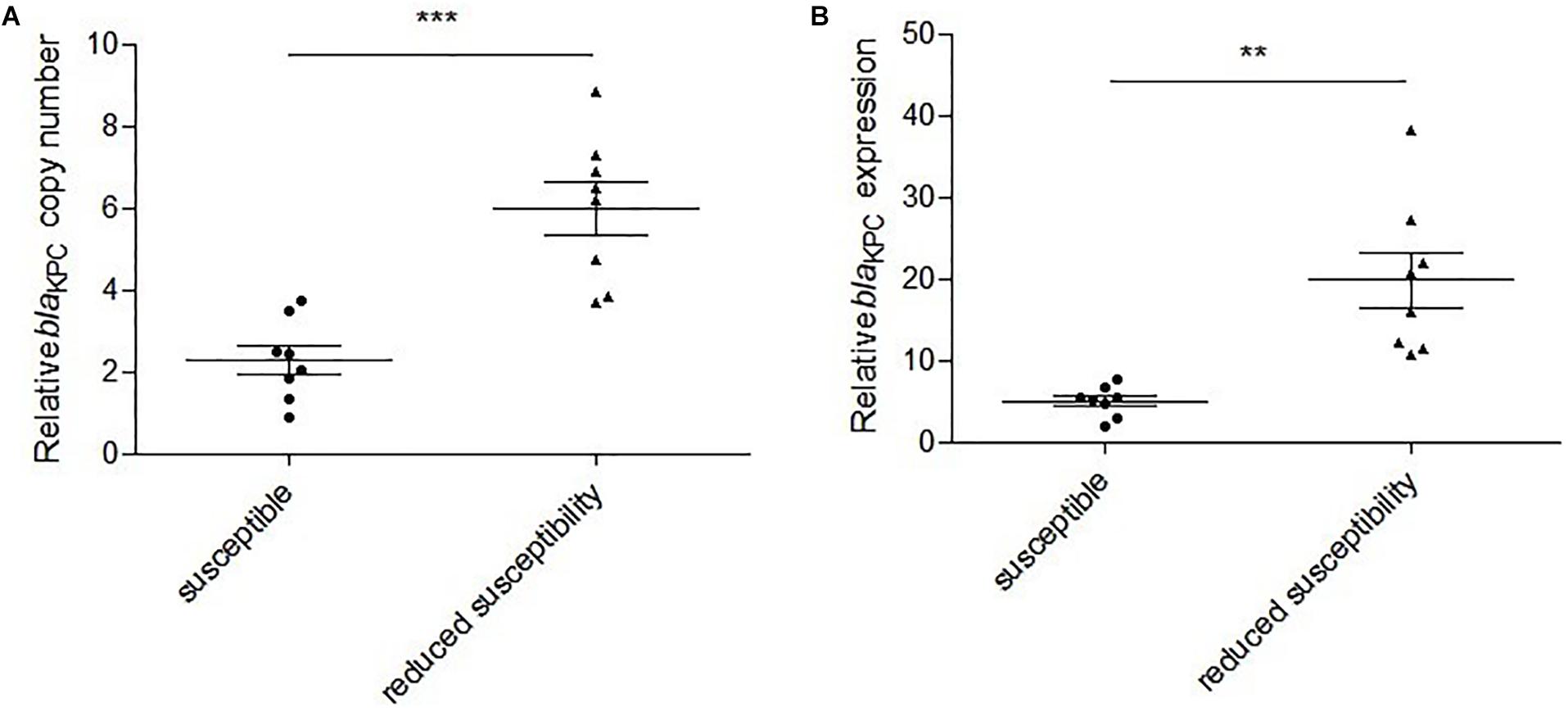
Figure 2. Relative blaKPC–2 copy numbers and expressions in susceptible and reduced susceptibility isolates. (A) Relative blaKPC–2 copy numbers in selected isolates. ***P = 0.0004, Unpaired t test with Welch’s correction. (B) Relative blaKPC–2 expressions in selected isolates. **P = 0.0034, Unpaired t test with Welch’s correction.
In vitro Selection Testing
The in vitro selection experiments showed that 4 of the 7 isolates with reduced susceptibility developed resistance to CAZ-AVI at the selection concentration of 2/4 μg/ml, and all 7 isolates developed resistance to CAZ-AVI with MICs ranging from 32/4 to 256/4 μg/ml when the selection concentration reached 4/4 μg/ml. By contrast, no isolates were developed to be resistance to CAZ-AVI in the susceptibility group, and all eight isolates stopped growing when the selection concentration reached 8/4 μg/ml.
Discussion
At present time, KPCs are the most common carbapenemases identified worldwide especially in K. pneumoniae from China (Nordmann and Poirel, 2014). Due to the limited therapies, the detection rate of KPC-KP in China demonstrated a continuous upward trend (Hu et al., 2016). In this study, CAZ-AVI showed potent in vitro activities against KPC-KP, which agreed with previous studies (de Jonge et al., 2016; Spiliopoulou et al., 2020). However, ∼3% isolates displayed reduced susceptibility to CAZ-AVI (MIC ≥ 4/4 μg/ml) despite they were obtained from patients without previous CAZ-AVI treatment history. Notably, these isolates also showed high level resistance to carbapenems.
In 2018, a fatal outbreak caused by ST11 KPC-KP with acquisition of a pLVPK-like virulence plasmid that increased the virulence of these isolates was reported (Gu et al., 2018). In our study, half of the isolates with reduced CAZ-AVI susceptibility contained several known virulence genes, suggesting that these isolates may have increased virulence, which should be closely monitored.
Since the previously reported gene mutations (e.g., D179Y) associated to CAZ-AVI resistance (Giddins et al., 2018) weren’t found in the blaKPC–2 genes, this study suggested that mechanisms other than blaKPC–2 gene mutation were underlying the reduced CAZ-AVI susceptibility among these isolates. OmpK35/36 defects had previously been reported to lead to reduced susceptibility or resistance to CAZ-AVI in KPC-KP (Nelson et al., 2017). In this study, our results showed 12 isolates with reduced CAZ-AVI susceptibility contained OmpK35 and OmpK36 gene mutations, however, the same gene mutations were also found in the susceptible strains, which suggested that OmpK35/36 defects may only partially contribute to the reduced CAZ-AVI susceptibility among those 12 strains, while additional mechanisms may be involved. Our results demonstrated that the blaKPC–2 copy numbers and expressions in the reduced susceptibility group were significantly higher than those in the susceptibility group. We therefore suspected that the reduced CAZ-AVI susceptibility in these strains was likely due to the higher blaKPC–2 copy numbers and gene expressions, in combination to the OmpK35/36 defects. The results were consistent with some previously published studies (Shen et al., 2017; Zhang et al., 2020). The higher copy numbers of blaKPC–2 may potentially result from the high copy numbers of the plasmids carrying blaKPC (Roth et al., 2011) and/or a duplication of mobile genetic elements associated with blaKPC (Coppi et al., 2020). Since the examination of the blaKPC–2 promoter regions failed to identify any mutations, the higher blaKPC gene expression may potentially be affected by the higher copy numbers of blaKPC or other gene regulatory mechanisms. Further studies, including whole genome sequences, are needed to explore the molecular mechanisms underlying the higher blaKPC–2 copy numbers and gene expressions among those strains.
Shields et al. (2017) has reported on the acquisition of CAZ-AVI resistance among ST258 KPC-KP during treatment in the United States. After that, (Raisanen et al., 2019) isolated a ST39 KPC-KP that was resistant to CAZ-AVI after this combination treatment. In China, ST11 KPC-KP is commonly prevalent (Chen et al., 2014; Zhang et al., 2017). In this study, the ST11 KPC-KP with reduced susceptibility were more prone to develop CAZ-AVI resistance compared to susceptible isolates under the pressure of CAZ-AVI exposing.
Taken together, our study demonstrated that CAZ-AVI has potent in vitro activities against KPC-KP in China and highlighted the clinical significance of the isolates with reduced susceptibility to CAZ-AVI isolated from patients without previous CAZ-AVI treatment history. Our results suggested that the optimal clinical usage of CAZ-AVI should be guided by the in vitro susceptibility results in order to prevent selection resistance.
Data Availability Statement
The datasets generated for this study are available on request to the corresponding author.
Ethics Statement
This study was approved by the institutional review board (IRB) of The Second Affiliated Hospital of Soochow University. The clinical isolates were retrospectively collected, and patient data were not included in this study, therefore the need for written informed consent was waived by the IRB.
Author Contributions
XC, BS, and XZ contributed to conducing the study, data analysis, and manuscript preparation. FQ, WJ, BH, HY, and Y-WT analyzed the data and reviewed the manuscript. LC and HD contributed to study design, data analysis, and manuscript preparation. All authors contributed to the article and approved the submitted version.
Funding
This study was supported by the National Natural Science Foundation of China (81572032), the Six Talent Peaks Project in Jiangsu Province (2016-WSN-112), the Key Research and Development Project of Jiangsu Provincial Science and Technology Department (BE2017654), Gusu Key Health Talent of Suzhou, the Jiangsu Youth Medical Talents Program (QN-867), and the Science and Technology Program of Suzhou (SZS201715 and SLT201934).
Conflict of Interest
Y-WT was employed by Cepheid, Shanghai, China.
The remaining authors declare that the research was conducted in the absence of any commercial or financial relationships that could be construed as a potential conflict of interest.
Footnotes
References
Bokaeian, M., Shahraki Zahedani, S., Soltanian Bajgiran, M., and Ansari Moghaddam, A. (2015). Frequency of PER, VEB, SHV, TEM and CTX-M Genes in Resistant Strains of Pseudomonas aeruginosa producing extended spectrum beta-lactamases. Jundishapur J. Microbiol. 8:e13783. doi: 10.5812/jjm.13783
Candan, E. D., and Aksoz, N. (2015). Klebsiella pneumoniae: characteristics of carbapenem resistance and virulence factors. Acta Biochim. Pol. 62, 867–874. doi: 10.18388/abp.2015_1148
Chen, L., Mathema, B., Chavda, K. D., DeLeo, F. R., Bonomo, R. A., and Kreiswirth, B. N. (2014). Carbapenemase-producing Klebsiella pneumoniae: molecular and genetic decoding. Trends Microbiol. 22, 686–696. doi: 10.1016/j.tim.2014.09.003
Clancy, C. J., Chen, L., Hong, J. H., Cheng, S., Hao, B., Shields, R. K., et al. (2013). Mutations of the OmpK36 porin gene and promoter impact responses of sequence type 258, KPC-2-producing Klebsiella pneumoniae strains to doripenem and doripenem-colistin. Antimicrob. Agents Chemother. 57, 5258–5265. doi: 10.1128/AAC.01069-13
Clinical and Laboratory Standards Institute (2018). M100-S28. Performance Standards for Antimicrobial Susceptibility Testing: 28th Informational Supplement. Wayne, PA: Clinical and Laboratory Standards Institute.
Coppi, M., Di Pilato, V., Monaco, F., Giani, T., Conaldi, P. G., and Rossolini, G. M. (2020). Ceftazidime-avibactam resistance associated with increased blaKPC-3 gene copy number mediated by pKpQIL plasmid derivatives in sequence Type 258 Klebsiella pneumoniae. Antimicrob. Agents Chemother. 64:e01816-19. doi: 10.1128/AAC.01816-19
Costa, D., Vinue, L., Poeta, P., Coelho, A. C., Matos, M., Saenz, Y., et al. (2009). Prevalence of extended-spectrum beta-lactamase-producing Escherichia coli isolates in faecal samples of broilers. Vet. Microbiol. 138, 339–344. doi: 10.1016/j.vetmic.2009.03.029
de Jonge, B. L., Karlowsky, J. A., Kazmierczak, K. M., Biedenbach, D. J., Sahm, D. F., and Nichols, W. W. (2016). In vitro susceptibility to ceftazidime-avibactam of carbapenem-nonsusceptible Enterobacteriaceae Isolates collected during the INFORM global surveillance study (2012 to 2014). Antimicrob. Agents Chemother. 60, 3163–3169. doi: 10.1128/AAC.03042-15
Diancourt, L., Passet, V., Verhoef, J., Grimont, P. A., and Brisse, S. (2005). Multilocus sequence typing of Klebsiella pneumoniae nosocomial isolates. J. Clin. Microbiol. 43, 4178–4182. doi: 10.1128/JCM.43.8.4178-4182.2005
Doumith, M., Ellington, M. J., Livermore, D. M., and Woodford, N. (2009). Molecular mechanisms disrupting porin expression in ertapenem-resistant Klebsiella and Enterobacter spp. clinical isolates from the UK. J. Antimicrob. Chemother. 63, 659–667. doi: 10.1093/jac/dkp029
Giddins, M. J., Macesic, N., Annavajhala, M. K., Stump, S., Khan, S., McConville, T. H., et al. (2018). Successive emergence of ceftazidime-avibactam resistance through distinct genomic adaptations in blaKPC-2-Harboring Klebsiella pneumoniae sequence type 307 isolates. Antimicrob. Agents Chemother. 62:e02101-17. doi: 10.1128/AAC.02101-17
Gu, D., Dong, N., Zheng, Z., Lin, D., Huang, M., Wang, L., et al. (2018). A fatal outbreak of ST11 carbapenem-resistant hypervirulent Klebsiella pneumoniae in a Chinese hospital: a molecular epidemiological study. Lancet Infect. Dis. 18, 37–46. doi: 10.1016/S1473-3099(17)30489-9
Hu, F. P., Guo, Y., Zhu, D. M., Wang, F., Jiang, X. F., Xu, Y. C., et al. (2016). Resistance trends among clinical isolates in China reported from CHINET surveillance of bacterial resistance, 2005-2014. Clin. Microbiol. Infect. 22(Suppl. 1), S9–S14. doi: 10.1016/j.cmi.2016.01.001
Huang, J., Hu, X., Zhao, Y., Shi, Y., Ding, H., Wu, R., et al. (2019). Comparative analysis of blaKPC expression in Tn4401 transposons and the Tn3-Tn4401 Chimera. Antimicrob. Agents Chemother. 63:e02434-18. doi: 10.1128/AAC.02434-18
Kitchel, B., Rasheed, J. K., Endimiani, A., Hujer, A. M., Anderson, K. F., Bonomo, R. A., et al. (2010). Genetic factors associated with elevated carbapenem resistance in KPC-producing Klebsiella pneumoniae. Antimicrob. Agents Chemother. 54, 4201–4207. doi: 10.1128/AAC.00008-10
Nelson, K., Hemarajata, P., Sun, D., Rubio-Aparicio, D., Tsivkovski, R., Yang, S., et al. (2017). Resistance to ceftazidime-avibactam is due to transposition of KPC in a Porin-deficient strain of Klebsiella pneumoniae with increased efflux activity. Antimicrob. Agents Chemother. 61:e00989-17. doi: 10.1128/AAC.00989-17
Nordmann, P., and Poirel, L. (2014). The difficult-to-control spread of carbapenemase producers among Enterobacteriaceae worldwide. Clin. Microbiol. Infect. 20, 821–830. doi: 10.1111/1469-0691.12719
Papp-Wallace, K. M., Bethel, C. R., Distler, A. M., Kasuboski, C., Taracila, M., and Bonomo, R. A. (2010). Inhibitor resistance in the KPC-2 beta-lactamase, a preeminent property of this class A beta-lactamase. Antimicrob. Agents Chemother. 54, 890–897. doi: 10.1128/AAC.00693-09
Pollett, S., Miller, S., Hindler, J., Uslan, D., Carvalho, M., and Humphries, R. M. (2014). Phenotypic and molecular characteristics of carbapenem-resistant Enterobacteriaceae in a health care system in Los Angeles, California, from 2011 to 2013. J. Clin. Microbiol. 52, 4003–4009. doi: 10.1128/JCM.01397-14
Raisanen, K., Koivula, I., Ilmavirta, H., Puranen, S., Kallonen, T., Lyytikainen, O., et al. (2019). Emergence of ceftazidime-avibactam-resistant Klebsiella pneumoniae during treatment, Finland, December 2018. Euro. Surveill 24:1900256. doi: 10.2807/1560-7917.ES.2019.24.19.1900256
Rodriguez-Villodres, A., Gil-Marques, M. L., Alvarez-Marin, R., Bonnin, R. A., Pachon-Ibanez, M. E., Aguilar-Guisado, M., et al. (2020). Extended-spectrum resistance to beta-lactams/beta-lactamase inhibitors (ESRI) evolved from low-level resistant Escherichia coli. J Antimicrob Chemother. 75, 77–85. doi: 10.1093/jac/dkz393
Roth, A. L., Kurpiel, P. M., Lister, P. D., and Hanson, N. D. (2011). bla(KPC) RNA expression correlates with two transcriptional start sites but not always with gene copy number in four genera of Gram-negative pathogens. Antimicrob. Agents Chemother. 55, 3936–3938. doi: 10.1128/AAC.01509-10
Ruzin, A., Visalli, M. A., Keeney, D., and Bradford, P. A. (2005). Influence of transcriptional activator RamA on expression of multidrug efflux pump AcrAB and tigecycline susceptibility in Klebsiella pneumoniae. Antimicrob. Agents Chemother. 49, 1017–1022. doi: 10.1128/AAC.49.3.1017-1022.2005
Schechner, V., Straus-Robinson, K., Schwartz, D., Pfeffer, I., Tarabeia, J., Moskovich, R., et al. (2009). Evaluation of PCR-based testing for surveillance of KPC-producing carbapenem-resistant members of the Enterobacteriaceae family. J. Clin. Microbiol. 47, 3261–3265. doi: 10.1128/JCM.02368-08
Shen, Z., Ding, B., Ye, M., Wang, P., Bi, Y., Wu, S., et al. (2017). High ceftazidime hydrolysis activity and porin OmpK35 deficiency contribute to the decreased susceptibility to ceftazidime/avibactam in KPC-producing Klebsiella pneumoniae. J Antimicrob Chemother. 72, 1930–1936. doi: 10.1093/jac/dkx066
Shields, R. K., Chen, L., Cheng, S., Chavda, K. D., Press, E. G., Snyder, A., et al. (2017). Emergence of ceftazidime-avibactam resistance due to plasmid-borne blaKPC-3 mutations during treatment of carbapenem-resistant klebsiella pneumoniae infections. Antimicrob. Agents Chemother 61:e02097-16. doi: 10.1128/AAC.02097-16
Spiliopoulou, I., Kazmierczak, K., and Stone, G. G. (2020). In vitro activity of ceftazidime/avibactam against isolates of carbapenem-non-susceptible Enterobacteriaceae collected during the INFORM global surveillance programme (2015-17). J Antimicrob Chemother. 75, 384–391. doi: 10.1093/jac/dkz456
van Duin, D., and Bonomo, R. A. (2016). Ceftazidime/Avibactam and ceftolozane/tazobactam: second-generation beta-lactam/beta-lactamase inhibitor combinations. Clin. Infect. Dis. 63, 234–241. doi: 10.1093/cid/ciw243
Weisburg, W. G., Barns, S. M., Pelletier, D. A., and Lane, D. J. (1991). 16S ribosomal DNA amplification for phylogenetic study. J. Bacteriol. 173, 697–703. doi: 10.1128/jb.173.2.697-703.1991
Yu, F., Lv, J., Niu, S., Du, H., Tang, Y. W., Pitout, J. D. D., et al. (2018). Multiplex PCR analysis for rapid detection of Klebsiella pneumoniae carbapenem-resistant (Sequence Type 258 [ST258] and ST11) and hypervirulent (ST23, ST65, ST86, and ST375) strains. J. Clin. Microbiol. 56:e00731-18. doi: 10.1128/JCM.00731-18
Zhang, P., Shi, Q., Hu, H., Hong, B., Wu, X., Du, X., et al. (2020). Emergence of ceftazidime/avibactam resistance in carbapenem-resistant Klebsiella pneumoniae in China. Clin. Microbiol. Infect. 26, 124.e1–124.e4. doi: 10.1016/j.cmi.2019.08.020
Keywords: KPC, Klebsiella pneumoniae, ceftazidime-avibactam, antimicrobial susceptibility, antimicrobial resistance
Citation: Cui X, Shan B, Zhang X, Qu F, Jia W, Huang B, Yu H, Tang Y-W, Chen L and Du H (2020) Reduced Ceftazidime-Avibactam Susceptibility in KPC-Producing Klebsiella pneumoniae From Patients Without Ceftazidime-Avibactam Use History – A Multicenter Study in China. Front. Microbiol. 11:1365. doi: 10.3389/fmicb.2020.01365
Received: 21 February 2020; Accepted: 27 May 2020;
Published: 23 June 2020.
Edited by:
Constantinos Tsioutis, European University Cyprus, CyprusReviewed by:
Marco Maria D’Andrea, University of Rome Tor Vergata, ItalyVincenzo Di Pilato, University of Genoa, Italy
Copyright © 2020 Cui, Shan, Zhang, Qu, Jia, Huang, Yu, Tang, Chen and Du. This is an open-access article distributed under the terms of the Creative Commons Attribution License (CC BY). The use, distribution or reproduction in other forums is permitted, provided the original author(s) and the copyright owner(s) are credited and that the original publication in this journal is cited, in accordance with accepted academic practice. No use, distribution or reproduction is permitted which does not comply with these terms.
*Correspondence: Hong Du, hongdu@suda.edu.cn; hong_du@126.com
†These authors have contributed equally to this work