- 1Department of Clinical Analysis, School of Pharmaceutical Sciences, São Paulo State University-UNESP, Araraquara, Brazil
- 2Laboratory of Mycology and Environmental Diagnosis, University of Franca, Franca, Brazil
- 3Department of Preventive and Community Dentistry, Dental School, Federal University of Rio Grande do Sul, Porto Alegre, Brazil
The oral cavity is a highly diverse microbial environment in which microorganisms interact with each other, growing as biofilms on biotic and abiotic surfaces. Understanding the interaction among oral microbiota counterparts is pivotal for clarifying the pathogenesis of oral diseases. Candida spp. is one of the most abundant fungi in the oral mycobiome with the ability to cause severe soft tissue lesions under certain conditions. Paracoccidioides spp., the causative agent of paracoccidioidomycosis, may also colonize the oral cavity leading to soft tissue damage. It was hypothesized that both fungi can interact with each other, increasing the growth of the biofilm and its virulence, which in turn can lead to a more aggressive infectivity. Therefore, this study aimed to evaluate the dynamics of mono- and dual-species biofilm growth of Paracoccidioides brasiliensis and Candida albicans and their infectivity using the Galleria mellonella model. Biomass and fungi metabolic activity were determined by the crystal violet and the tetrazolium salt reduction tests (XTT), respectively, and the colony-forming unit (CFU) was obtained by plating. Biofilm structure was characterized by both scanning electronic- and confocal laser scanning- microscopy techniques. Survival analysis of G. mellonella was evaluated to assess infectivity. Our results showed that dual-species biofilm with P. brasiliensis plus C. albicans presented a higher biomass, higher metabolic activity and CFU than their mono-species biofilms. Furthermore, G. mellonella larvae infected with P. brasiliensis plus C. albicans presented a decrease in the survival rate compared to those infected with P. brasiliensis or C. albicans, mainly in the form of biofilms. Our data indicate that P. brasiliensis and C. albicans co-existence is likely to occur on oral mucosal biofilms, as per in vitro and in vivo analysis. These data further widen the knowledge associated with the dynamics of fungal biofilm growth that can potentially lead to the discovery of new therapeutic strategies for these infections.
Introduction
An important step in the development of infectious diseases involves the ability of microorganisms to adhere to host surfaces. Adherence is a widely distributed biological phenomenon and is also the first step in the process of biofilm formation (Verstrepen and Klis, 2006; Pitangui et al., 2012; Martinez and Casadevall, 2015). Biofilm arrangement can protect fungal pathogens from host defenses and reduce the diffusion of antifungal drugs (Martinez and Casadevall, 2006; Kernien et al., 2017; Tulasidas et al., 2018; Thomaz et al., 2020). Clinically, fungal biofilms can adhere to both abiotic surfaces, comprised of medical and dental devices, as well as to mucous membranes (Kernien et al., 2017). Many clinically relevant fungi can grow as biofilms, including Candida spp. (Hawser and Douglas, 1994) and Paracoccidioides spp. (Sardi Jde et al., 2015; Cattana et al., 2017).
Paracoccidioidomycosis (PCM) is a human systemic mycosis which assumes increasing clinical importance due to the increase in their frequency and mortality rates in South and Central America (Coutinho et al., 2002). Paracoccidioides spp. are dimorphic fungi that change their morphology according to the environmental temperature, with a transition from mold at 25°C to yeast at 37°C in human lungs (San-Blas and Vernet, 1977; Gauthier, 2017). Although the primary route of PCM infection is pulmonary, the disease is frequently diagnosed by extensive, ulcerative and painful buccal manifestations corresponding to the clinical form of the multifocal type (Franco et al., 1987; Mendes et al., 2017; de Arruda et al., 2018).
Candida spp. is a commensal fungus found in the gastrointestinal tract, on oropharyngeal and vaginal mucosa of humans, with the ability to cause mucosal infections under certain conditions, such as immunosuppression, radiotherapy, antibiotics, and corticosteroids (Fotos et al., 1992; Akpan and Morgan, 2002; Epstein et al., 2002; Fangtham et al., 2014). Candida albicans is one of the most abundant fungi in the oral mycobiome (Ramage et al., 2004; Dongari-Bagtzoglou et al., 2009; Millsop and Fazel, 2016). Increasing evidence indicate that the coexistence of C. albicans and oral bacteria as well as other interactions with different kingdoms affect both the dynamics of biofilm growth and the course and severity of mucosal lesions, as reviewed by Negrini et al. (2019).
Considering that both Candida and Paracoccidioides may co-exist in the oral cavity, the impact of such fungal-fungal interaction on biofilm formation and its pathogenic potential are still unknown. Therefore, this study aimed to evaluate the dynamics of mono- and dual-species biofilm formed by P. brasiliensis plus C. albicans and their infectivity using the Galleria mellonella model. Our hypothesis is that both fungi interact with each other boosting the biofilm growth, that in turn could lead to a more aggressive infectivity.
Materials and Methods
Fungal Strains and Growth Conditions
Paracoccidioides strains are currently classified as a complex of five cryptic species (P. brasiliensis, P. americana, P. restrepiensis, P. venezuelensis, and P. lutzii) distinguished by the phylogeny findings and studies using molecular techniques (Matute et al., 2006; Carrero et al., 2008; Turissini et al., 2017; De Macedo et al., 2019). We used the P. brasiliensis strain (former S1 phylogenetic group, Pb 18 strain, De Macedo et al., 2019), originally isolated from a case of pulmonary paracoccidioidomycosis in São Paulo, SP, Brazil. This strain belongs to the collection of the Clinical Mycology Laboratory, Department of Clinical Analysis, School of Pharmaceutical Sciences, UNESP. C. albicans ATCC 90028 was also used and it was cultured on Sabouraud dextrose agar (Difco—BD Biosciences, Sparks, MD, United States) and incubated at 37°C for 48 h. P. brasiliensis (Pb18) was cultured in Fava-Netto standard medium (Fava Netto et al., 1969) and incubated at 37°C for 5 days.
In vitro Biofilm Growth
The inocula were prepared as previously published, as per the C. albicans biofilm formation protocol (Jin et al., 2004). Briefly, a suspension of each fungus was standardized in Brain Heart Infusion (BHI) broth at 1 × 106 cells/mL using a hemocytometer. In all experiments, the fungal cell viability was evaluated using trypan blue (do Carmo Silva et al., 2015). Monospecies biofilms were developed on a polystyrene 96-well microtiter plate by adding 200 μL of C. albicans or P. brasiliensis standard inoculum to six wells. The plates were incubated at 37°C for 168 h with both fungi. The medium was changed during the total incubation time every 24 h. To compare the growth rates, both the biofilms were incubated for 168 h according to the kinetics for the biofilm of P. brasiliensis previously determined by our group (Sardi Jde et al., 2015).
Dual-species biofilms were developed on a 96-well microtiter plate by adding 100 μL of P. brasiliensis and 100 μL of C. albicans standard inoculum to six wells and by incubating them for 168 h at 37°C. Based on the assumption that C. albicans already exists in the oral microenvironment, we developed a second group of dual-species biofilms. In this group, we inoculated 100 μL of P. brasiliensis standard inoculum to a preformed 12 h C. albicans biofilm at 37°C. This was followed by incubation for a total period of 168 h at 37°C. In both dual-species biofilm groups, the medium was changed every 24 h of incubation.
Quantitative Biofilm Analysis
Biomass Quantification
Biofilm biomass was quantified based on the crystal violet (CV) assay (Peeters et al., 2008) with modifications. One microplate containing mono-species and dual-species biofilms was subjected to the CV methodology every 24 h until a 168 h total period. After each 24 h of incubation, the plates were washed with PBS to remove non-adherent cells. The biofilms were fixed with 100 μL of 99% methanol (Sigma-Aldrich, São Paulo, Brazil). After 15 min, the supernatants were removed, and the plates were air-dried. Then, 100 μL of 0.1% CV solution was added to all wells. After 20 min, the excess CV was removed by washing with PBS. Finally, the bound CV was released by adding 150 μL of 33% acetic acid (Sigma-Aldrich, São Paulo, Brazil). The absorbance was measured at 570 nm using a microplate reader (Epoch, Biotek). All steps were carried out at room temperature. Three independent experiments were performed with six replicates each.
Biofilm Metabolic Activity Assessment
The biofilm metabolic activity was assessed through the XTT (2.3-bis (2-methoxy-4-nitro-5-sulfophenyl)-5-[carbonyl (phenylamino)]-2H-tetrazolium hydroxide) reduction assay. About 50 μL of XTT salt solution (1 mg/mL in PBS) and 4 μL of menadione solution (1 mM in ethanol; Sigma-Aldrich, São Paulo, SP., Brazil) were added to each well of the 96-well plate and incubated at 37°C for 3 h. The test was carried out every 24 h until a total period of 168 h for both mono-species and dual-species biofilms. The absorbance was measured using a microplate reader (Epoch, Biotek) at 490 nm (Martinez and Casadevall, 2006; Silva et al., 2010). In all assays, the culture media were included as negative control. Three independent experiments were performed with six replicates each.
Counts of Fungal Viable Cells
After biofilm formation, at different point times (0, 24, 48, 72, 96, 120, 144, and 168 h), the wells were washed thrice with PBS to remove loosely adhered cells. The biofilms were then mechanically disrupted, filling the wells with 100 μL of BHI broth and then vigorously mixed. Each individual well content was transferred to microtubes containing 900 μL of BHI broth. The suspensions were serially diluted and plated onto BHI agar supplemented with 1% glucose, 5% of P. brasiliensis 339 culture filtrate and 4% fetal bovine serum and caspofungin 0.25 μg/mL (for P. brasiliensis counts) and on Sabouraud dextrose agar (for C. albicans counts) that were incubated at 37°C for 10 days and 48 h, respectively. The number of viable fungal colony-forming units (CFU) was determined under a stereomicroscope and the results were expressed as log10 CFU/mL. Three independent experiments were performed with six replicates each.
Structural Biofilm Analysis
Confocal Laser Scanning Microscopy (CLSM)
The standardized P. brasiliensis inoculum was treated with carboxyfluorescein diacetate succinimidyl ester dye (CFSE, 100 μM/mL, BioChemika), kindly provided by Dr Paula Aboud Barbugli from Araraquara School of Dentistry (São Paulo State University - UNESP) for 30 min at 37°C. The fungi cells were washed with PBS and resuspended in BHI medium by restoring the initial volume. This inoculum was then incubated with 12 h preformed C. albicans biofilms in 24-well plates. Mono-species biofilms of P. brasiliensis or C. albicans grown for a time that allowed their maturation (144 and 48 h, respectively) were used as controls (Sardi Jde et al., 2015). For the formation of dual-species biofilms of P. brasiliensis and C. albicans, equitable volumes of the standardized inoculum of the two organisms were co-cultivated and grown for 120 h, the time of greatest metabolic activity determined by the XTT test (section “Biofilm Metabolic Activity Assessment”). Next, the biofilms were washed carefully with sterile PBS to remove non-adherent cells. The biofilms were stained with calcofluor white (1 g/L; Sigma-Aldrich St. Louis, MO, United States) for 30 min at room temperature and washed again with PBS. The biofilms were photographed under a 20× dry immersion objective (numerical aperture 1.0) using a Zeiss LSM 800 confocal microscope. Each field of view was photographed using a 405 nm laser to capture calcofluor with an emission range up to 450 and 488 nm for CFSE detection with an emission range up to 540 nm.
Scanning Electron Microscopy (SEM)
To access the morphology, the biofilms were formed in 24-well plates according to previously published protocols (Morris et al., 1997; Ells and Truelstrup Hansen, 2006) with modifications. Mono-species biofilms of P. brasiliensis or C. albicans grown at 37 C for 144 and 48 h, respectively, were used as controls. Dual-species biofilms were formed over a period of 120 h. Next, the plates were washed with PBS to remove non-adherent cells. The biofilms were fixed with 1,000 μL of 2.5% glutaraldehyde solution (Sigma-Aldrich, St Louis, MO, United States) in sterile distilled water overnight (for both P. brasiliensis mono-species and dual-species biofilms) or for 3 h (C. albicans mono-species biofilms), washed thrice with PBS and sequentially dehydrated with ethanol (50–100%) at room temperature. All samples were dried in a 780A Samdri desiccator (Rockville, MD, United States). Subsequently, the samples were mounted on aluminum and silver cylinders and disposed in a high vacuum evaporator (Denton Vacuum Desk V, Jeol, Moorestown, NJ, United States) for gold coating. The topographic images of biofilms were captured under a scanning electron microscope (Jeol JSM- 6610LV, Peabody, MA, United States).
Survival Assay Using G. mellonella Model
Survival assay was performed according to the previous protocol (Scorzoni et al., 2015), with some modifications. G. mellonella larvae (School of Pharmaceutical Sciences, São Paulo State University–UNESP) with a body weight ranging from 150 to 200 mg were randomly chosen for the experiments. The larvae were separated into six infection groups, each group consisting of 9–10 larvae which were kept in Petri plates at 37°C overnight, prior to use. To obtain planktonic cells, C. albicans was grown in Sabouraud agar at 25°C for 48 h and P. brasiliensis was grown in BHI broth supplemented with 1% glucose at 37°C and 150 rpm for 72 h. Pre-formed biofilms of C. albicans for 12 h and P. brasiliensis for 120 h in a 24-well plate were scrapped using a sterile tip and utilized for inoculum preparation. The inoculum of fungi for planktonic cells or cells from biofilms were prepared in PBS and counted using a hemocytometer. For each group, the larvae were injected at a concentration of 1 × 106 cells/larvae of C. albicans or P. brasiliensis (2 × 106 cells/larvae total for co-infection) into the last pro-leg using a 10 μL Hamilton syringe (Chibebe Junior et al., 2013; Scorzoni et al., 2015; de Barros et al., 2018). Survival larvae were monitored daily for up to 7 days of infection. Larvae were considered dead when they displayed no movement in response to touch. Larvae inoculated once or twice with sterile PBS were used as controls. Three independent experiments were performed, resulting in an n = 27–30 larvae/group.
Statistical Analysis
The statistical analysis was conducted using the GraphPad Prism 5.0 software (GraphPad Software, Inc., San Diego, CA). The results were presented as mean ± standard deviation and compared by analysis of variance (ANOVA) followed by Bonferroni test. The data from the survival of G. mellonella larvae were plotted as Kaplan–Meier survival curves and compared using log-rank tests. Statistical significance was considered when p < 0.05.
Results
Biomass Quantification
Overall, a biomass increase of all tested biofilms was observed in the period from 24 to 96 h growth, and this increase extended to up to 144 h in the case of P. brasiliensis mono-species biofilm (Figure 1). Comparison of mono-species with dual-species biofilms was performed using the analysis of variance (ANOVA) followed by Bonferroni test. Two different approaches have been done in relation to the dual-species genera interaction: (1) P. brasiliensis and C. albicans incubated simultaneously; (2) P. brasiliensis incubated after C. albicans pre-formed biofilm. The biomass of dual-species biofilms formed after P. brasiliensis was added to pre-formed 12 h C. albicans biofilms was statistically higher than the biomass of simultaneously formed dual-species biofilms at 48 h growth (p < 0.05). The biomass of P. brasiliensis mono-species biofilms was statistically lower than the biomass of simultaneously formed dual-species biofilms at 48 h (p < 0.01), 72 h (p < 0.05), and 96 h (p < 0.001) of growth and lower than the biomass of dual-species biofilms grown from P. brasiliensis added to 12 h pre-formed C. albicans biofilms at 48, 72, and 96 h (p < 0.001) and at 120 and 168 h (p < 0.01) of growth. No difference was found in the biomass of C. albicans as a mono-species biofilm compared to simultaneously formed dual-species biofilms in all periods analyzed, except the biomass at 48 h that was statistically lower than the biomass of dual-species biofilms grown from P. brasiliensis added to 12 h preformed C. albicans biofilms (p < 0.01).
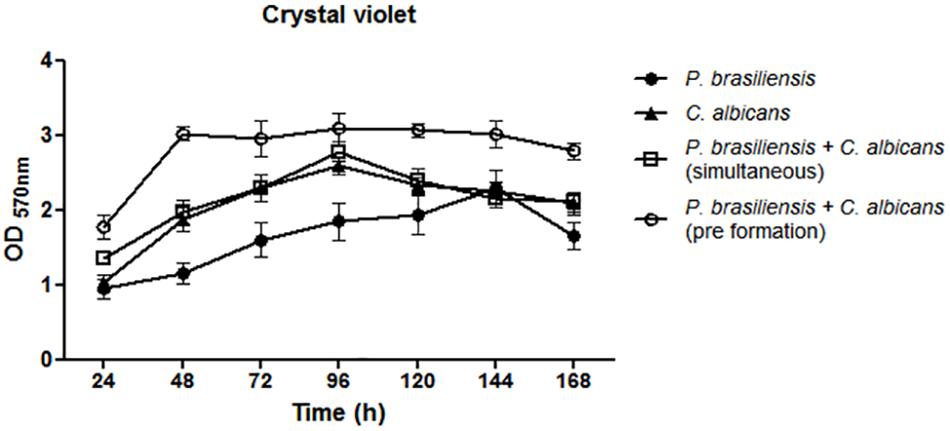
Figure 1. Quantification of mono and dual-species biofilm biomass by crystal violet methodology. Co-cultivated dual-species biofilms and dual-species biofilms formed by the addition of P. brasiliensis inoculum to preformed 12 h C. albicans biofilms. Three independent experiments with six replicates each were performed. Error bars indicate the standard error. Statistical significances were calculated by using analysis of variance (ANOVA) followed by Bonferroni test.
Biofilm Metabolic Activity
P. brasiliensis biofilm presents an increase in the metabolic activity from 24 h to up to 120 h of growth, whereas the C. albicans biofilm exhibits an increase in metabolic activity up to 48 h. Dual-species biofilms showed higher metabolic activity, mainly when P. brasiliensis was added to preformed 12 h C. albicans biofilms and there was a slight reduction after 72 h. Comparison of mono-species biofilms with dual-species was performed using analysis of variance (ANOVA) followed by Bonferroni test. The metabolic activity of P. brasiliensis biofilms was statistically lower than simultaneously formed dual-species biofilms at all time points (p < 0.001 for 24, 48, 72, 96, and 168 h and p < 0.05 for 120 and 144 h) and statistically lower than dual-species biofilms grown from P. brasiliensis added to preformed 12 h C. albicans biofilms at all time points (p < 0.001) except at 120 h of growth. Metabolic activities of P. brasiliensis and C. albicans biofilms were statistically lower than simultaneously formed dual-species biofilms only at 120 h of growth (p < 0.01) and at 24 h (p < 0.05), 48 and 72 h (p < 0.001) in comparison to dual-species biofilms when P. brasiliensis was added to preformed 12 h C. albicans biofilms. The metabolic activity of simultaneously formed dual-species biofilms was statistically lower than those in other conditions at 48 and 72 h of growth (p < 0.01) (Figure 2).
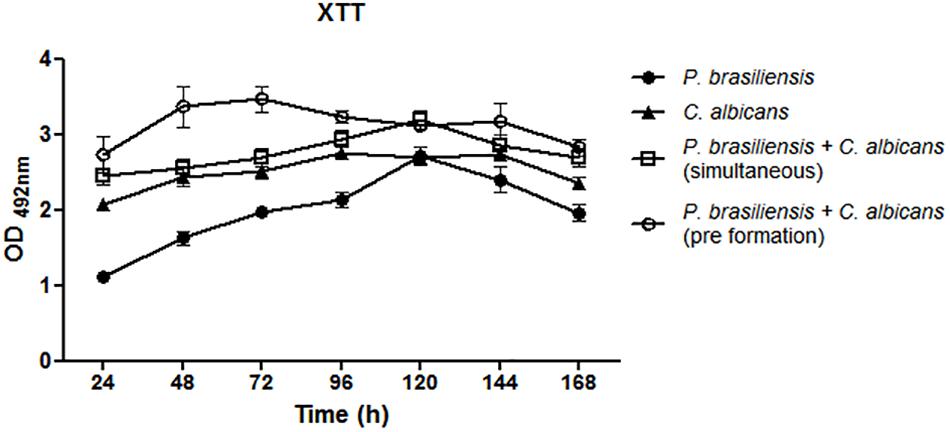
Figure 2. Metabolic activity of mono- and dual-species biofilms. Three independent experiments with six replicates each were performed. Co-cultivated dual-species biofilms and dual-species biofilms formed by the addition of P. brasiliensis inoculum to preformed 12 h C. albicans biofilms. Error bars indicate the standard error. Statistical significances were calculated by using analysis of variance (ANOVA) followed by Bonferroni test.
Determination of Viable Cells as CFU/mL
Comparison between the C. albicans CFU values were similar in mono or dual-species biofilms (ANOVA followed by Bonferroni test). However, the values of dual-species biofilms when P. brasiliensis was added to 12 h preformed C. albicans biofilms were statistically significant and higher than C. albicans biofilm at 144 h (p < 0.001) (Figure 3A). Similarly, the comparison between the CFU values of P. brasiliensis was similar in mono or dual-species biofilms (Figure 3B). There was no significant difference in the evaluated times, suggesting that both fungi are able to co-exist in dual-species biofilms resembling those formed on oral mucosal surfaces.
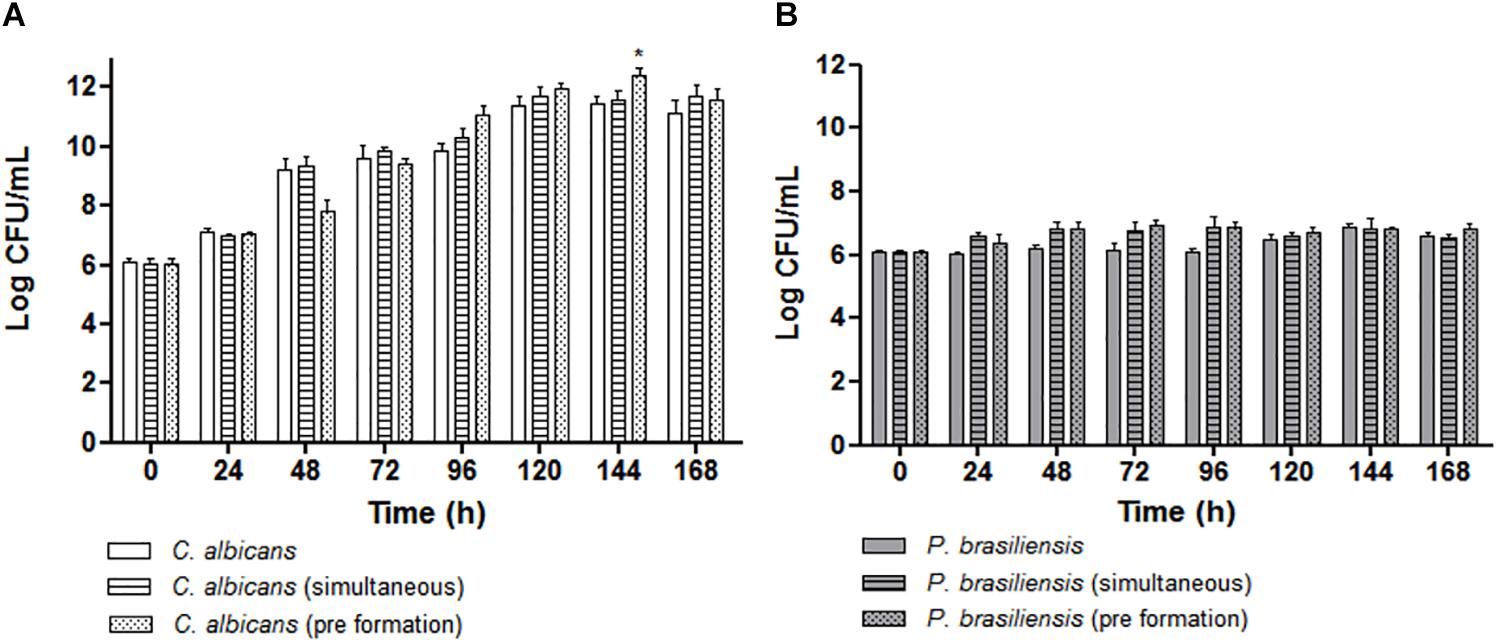
Figure 3. Quantitative analysis of biofilm formation in vitro by CFU/mL (Log10) count for the following groups: (A) Mono-species biofilms formed by C. albicans, co-cultivated C. albicans plus P. brasiliensis biofilms or dual-species biofilms formed by the addition of P. brasiliensis inoculum to preformed 12 h C. albicans biofilms (*p < 0.001). (B) Mono-species biofilms formed by P. brasiliensis, co-cultivated C. albicans plus P. brasiliensis biofilms or dual-species biofilms formed by the addition of P. brasiliensis inoculum to preformed 12 h C. albicans biofilms. Statistical significances were calculated by using analysis of variance (ANOVA) followed by Bonferroni test.
Biofilm Structure
Confocal images showed C. albicans as a carpet-like biofilm (either as mono or as dual-species biofilms) whereas in dual-species biofilms, P. brasiliensis presented as organized clusters surrounded by C. albicans cells (Figure 4). The SEM images showed homogeneous and organized biofilms which were interspaced by an extracellular polysaccharide matrix (Figure 5). C. albicans and P. brasiliensis mono-species biofilms presented mainly yeasts forms (A) whilst (C) dual-species biofilms grown from P. brasiliensis added to preformed 12 h C. albicans biofilms presented a dense colonization by C. albicans (E). It appears that the interaction between C. albicans and P. brasiliensis, in addition to the direct physical contact, is also mediated by extracellular polysaccharides (F).
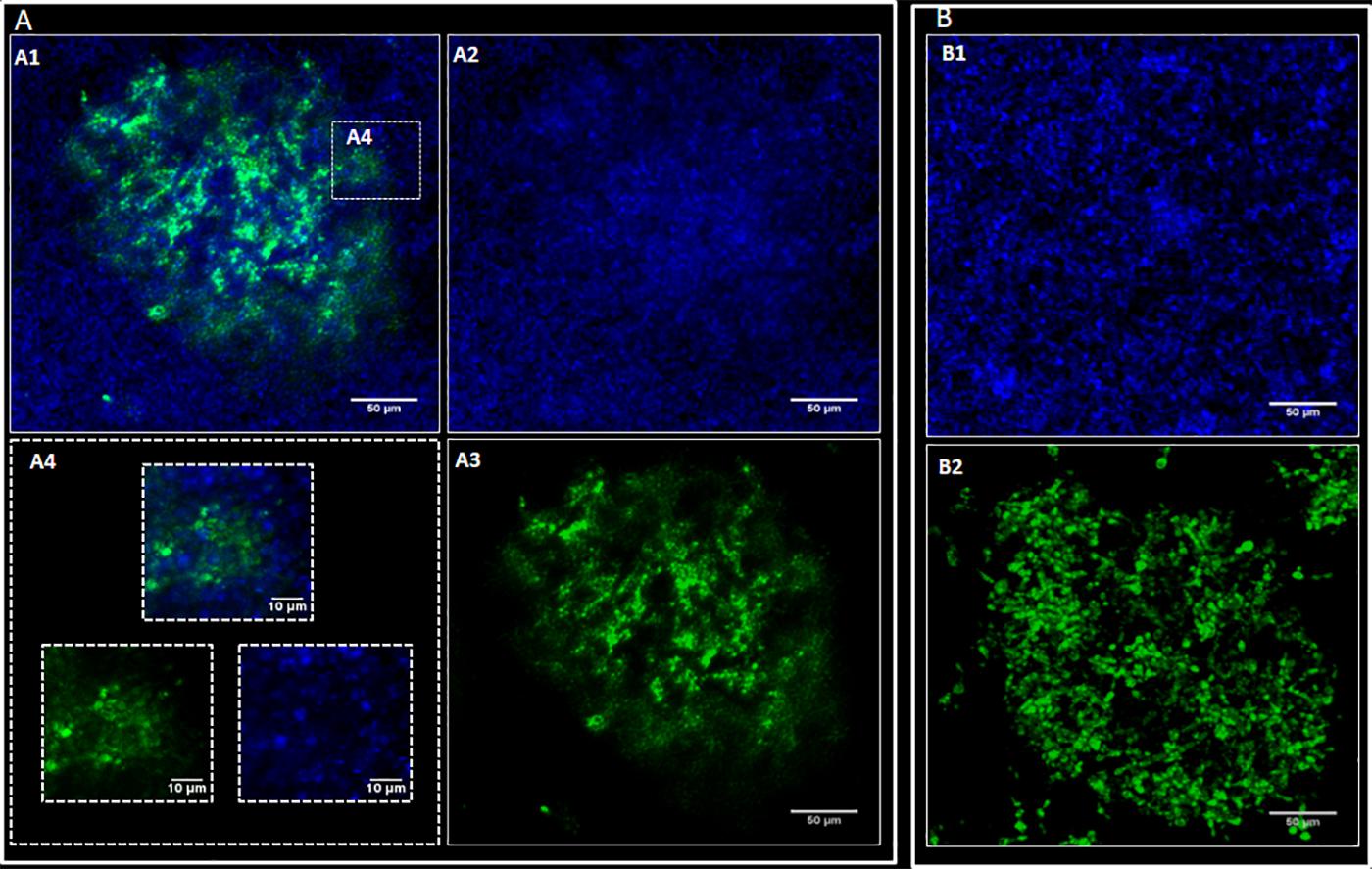
Figure 4. Representative confocal microscopy images. P. brasiliensis was marked with CFSE (green) and C. albicans with Calcofluor white (blue). C. albicans (CA) and P. brasiliensis (PB) mono-species biofilms formed for 48 and 144 h, respectively. For dual-species biofilms, P. brasiliensis previously treated with CFSE was added to 12 h preformed C. albicans biofilm, and incubated for 120 h. (A) Dual-species biofilms: overlay of CA and PB (A1), CA (A2), PB (A3) and zoom in image showing dual-species interaction (A4); (B) Mono-species biofilms: CA biofilm (B1) and PB biofilm (B2).
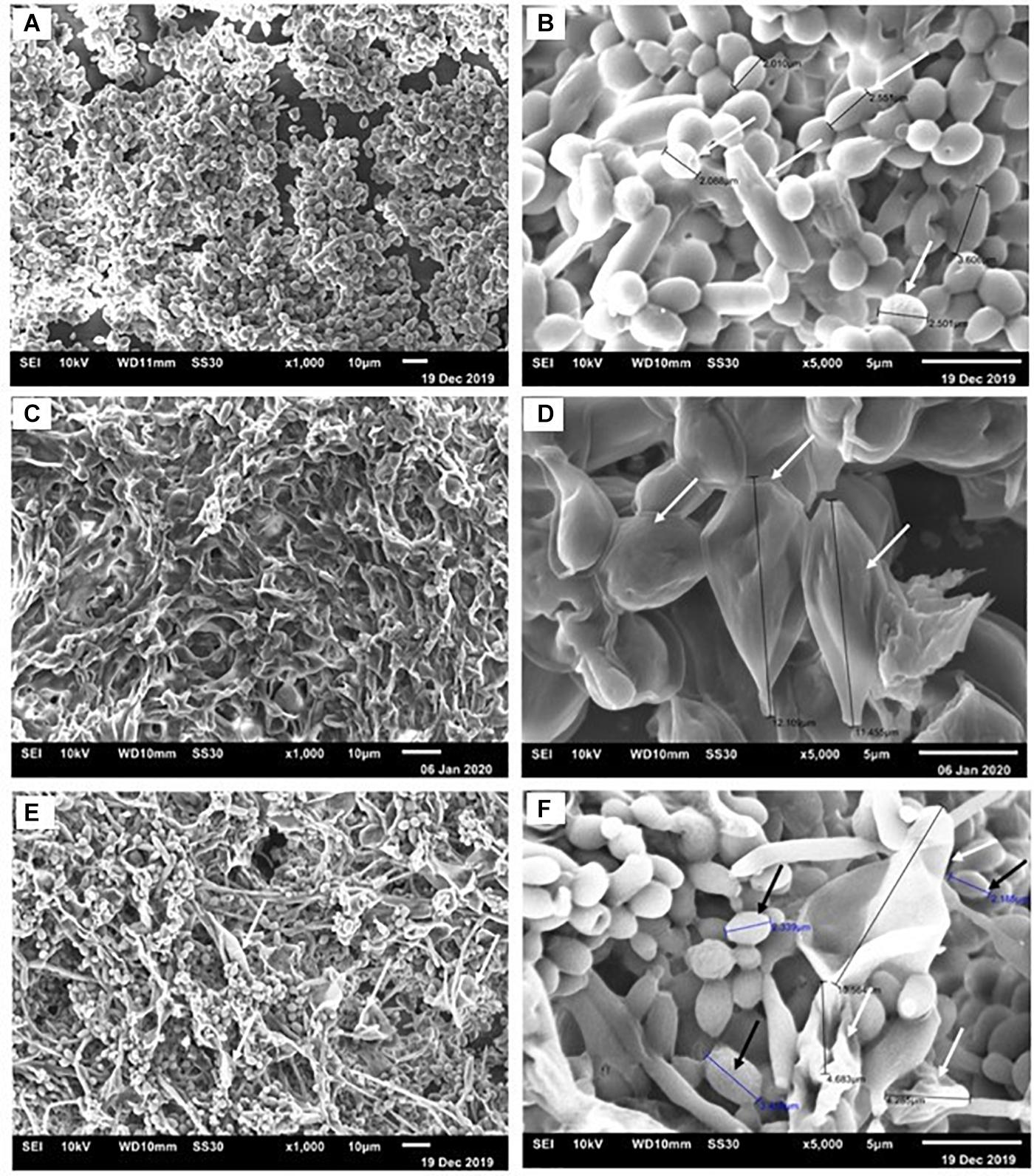
Figure 5. Representative scanning electron micrographs of C. albicans and P. brasiliensis mono-species biofilms formed for 48 and 144 h, respectively. For dual-species biofilms, P. brasiliensis was added to 12 h preformed C. albicans biofilm for 120 h. Biofilms formed by C. albicans (A) 1,000× and (B) 5,000× magnification. The white arrows indicate the yeast size (B). Biofilms formed by P. brasiliensis (C) 1,000× and (D) 5,000×. The black arrows denote the measure of yeast (D). Dual-species biofilms grown from P. brasiliensis added to 12 h preformed C. albicans biofilms (E) 1,000× and (F) 5,000×.
Microbial Interaction in the G. mellonella Model
The pathogenicity of cells released from the disrupted biofilms of P. brasiliensis and C. albicans and its co-infection were tested in the G. mellonella model (Figure 6). The data from larvae survival were plotted as Kaplan–Meier survival curves and compared using log-rank tests. Firstly, we observed a decrease in the survival rate of the larvae when infected with the mono- and dual-species biofilm cells compared to their planktonic cells. About 55% of the larvae were alive on the 7th day when infected with P. brasiliensis planktonic cells, but up to 38% were alive for its biofilm form. Likewise, infections by the two fungi in planktonic form led to the death of the larvae in the first 24 h, while larvae death was observed after 12 h of infection by the biofillm form. No larvae died with the single or double injection of PBS.
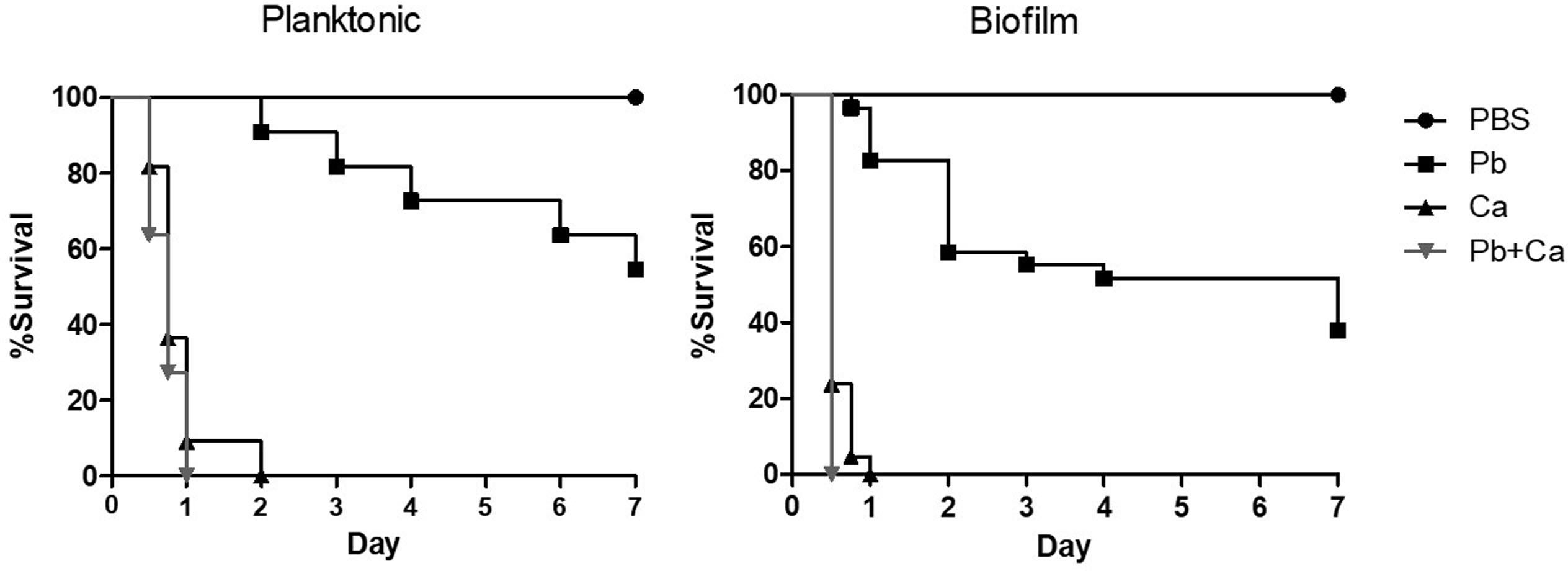
Figure 6. Survival curve of Galleria mellonella larvae infected with 1 × 106 cells/larvae of planktonic cells, mono-, and dual-species biofilms grown after P. brasiliensis (Pb) was added to preformed C. albicans (Ca) biofilms. Larvae inoculated with PBS was used as control. The values are plotted as Kaplan–Meier survival curves and compared using log-rank tests.
Secondly, when we compared the profile curve of larvae infected with mono- and dual-species biofilms with planktonic cells, there was a significant difference between P. brasiliensis and P. brasiliensis plus C. albicans (p < 0.05). On the other hand, the profile curve between C. albicans and P. brasiliensis plus C. albicans did not show a statistical difference, although the dual-species infection led to faster larvae mortality compared to C. albicans (1 vs. 2 days).
Thirdly, the comparison of larvae infected with mono- and dual-species biofilms cells showed a significant difference between P. brasiliensis or C. albicans and P. brasiliensis + C. albicans (p < 0.05).
Discussion
Candida species, the most representative oral mycobiome microorganism (Bertolini and Dongari-Bagtzoglou, 2019), is normally found in the oral cavity at levels compatible to health. However, under certain conditions, especially those related to immune suppression or even in neonates or elderly, there is an increase in Candida carriage in the oral cavity that ultimately leads to the development of opportunistic mucosal infections (Nicolatou-Galitis et al., 2001; Bertolini and Dongari-Bagtzoglou, 2019). In this context, many studies have reported inter-kingdom Candida-bacterial interactions in the oral cavity, as well as Candida-Candida interactions and their role in oral diseases, especially at the mucosal sites (Baena-Monroy et al., 2005; Thein et al., 2009; Martins et al., 2016; Negrini et al., 2019; Pathirana et al., 2019; Rodrigues et al., 2019). Moreover, Paracoccidioides spp. can also be found in the oral mucosal lesions as loose or dense granulomas (de Carli et al., 2015). Thus, both fungi can be found in a suitable niche in the oral cavity to exert their pathogenicity.
Both fungi may grow as in vitro or in vivo mono-species biofilms (Hawser and Douglas, 1994; Douglas, 2003; Sardi Jde et al., 2015; Cattana et al., 2017). Therefore, evaluating the dynamics of mono- and dual-species biofilm formed by P. brasiliensis and C. albicans can widen the knowledge about the PCM infection mechanism, the most important mycotic disease in Latin America. To our knowledge, this is the first time that such dual-species biofilm has been developed and reported.
Despite the disadvantages of reproducing some biological conditions (Diaz-Garcia et al., 2019), polystyrene microtiter plates are commonly used as the standard system to study adhesion and biofilm formation by bacteria and fungi (Simoes et al., 2010; Pemmaraju et al., 2013; Sardi Jde et al., 2015).
Both biomass and biofilm metabolic activity were used in this study to characterize the dynamics of biofilm growth. Based on the quantification of biomass, we found that C. albicans mono-species biofilms reached a mature stage from 72 to 96 h in agreement with previous data (Pierce et al., 2010; Cavalheiro and Teixeira, 2018). P. brasiliensis needed 144 h to reach its highest biomass, which corroborates with the previous studies carried out by Sardi Jde et al. (2015). In addition, the C. albicans mono-species biofilm showed higher values of biomass, metabolic activity and CFU than that found in the P. brasiliensis mono-species biofilm in most of the evaluated times.
Interestingly, the growth dynamics of dual-species biofilms was different. The mature stage (in terms of biomass) of co-cultivated dual-species biofilms was reached after 96 h of growth, while the dual-species biofilm formed after P. brasiliensis was added to 12 h preformed C. albicans biofilm reached its highest biomass in 48 h. In line with the fast growth of dual-species biofilms, their metabolic activity was also higher than the mono-species biofilms, almost at all time points, mainly in 72 h. In agreement with the biomass and metabolic activity results, the C. albicans CFU values in dual-species biofilms were higher compared to the CFU values of mono-species biofilms, specially from 96 h. The increase in metabolic activity, biomass and CFU, primarily in the dual-species biofilms grown after P. brasiliensis was added to preformed 12 h C. albicans biofilms, compared to P. brasiliensis mono-species biofilms, indicate that there were no loss to P. brasiliensis and that can coexist in the same environment as C. albicans.
There are common functional organization principles that occur at sites of polymicrobial infection, including exploration of metabolites, immunological modulation, niche optimization and induction of virulence. Thus, the presence of a microorganism can generate a niche for other pathogenic microorganisms or predispose the host to colonization by other microorganisms, or two or more non-pathogenic microorganisms together can cause disease (Brogden et al., 2005; Peters et al., 2012). Clinically, we could hypothesize that oral cavity lesions caused by P. brasiliensis would be more aggressive when both fungi (C. albicans and P. brasiliensis) are present at both the same time and site, once we observe an improvement in the biofilm features. The surface first settled by C. albicans cells has already been related to increased adhesion, accompanied by a significant increase in cell viability with bacterial biofilms (Bartnicka et al., 2019). Interactions in mixed biofilms are essential to produce a rich exopolysaccharide matrix. In a previous study, the synergism between C. albicans and Streptococcus mutans increased the virulence of mixed biofilms formed on the surfaces of teeth, thus contributing to the severity of caries (Falsetta et al., 2014; Sztajer et al., 2014). Here, the enhanced growth through intergeneric cooperation may be taking place and the damage to oral mucosa tissues caused by Candida may be influence the PCM pathogenesis.
The SEM images of mono-species biofilms showed C. albicans and P. brasiliensis yeasts embedded in extracellular polysaccharide matrix and presenting an average size from 2 to 4 μm and from 11 to 12 μm, respectively. Dual-species biofilms also showed similar features of mono species biofilms, however, presenting a higher proportion of C. albicans yeast cells. This higher proportion of C. albicans may be due to the slower growth of P. brasiliensis. Interestingly, the interaction between C. albicans and P. brasilensis in dual-species biofilms seem to happen both by direct physical contact and mediated by the extracellular polysaccharide matrix. It is known that some Candida surface adhesins, such as Als3, exert an important role on Candida biofilm formation as well as mediating the co-aggregation between Candida and other microorganisms (Verstrepen and Klis, 2006; Cavalheiro and Teixeira, 2018; Negrini et al., 2019). Moreover, Candida cell-wall mannan-residues might also act as an adhesion element for extracellular polysaccharides (Negrini et al., 2019). In addition, several Paracoccidioides adhesin proteins have been shown to be extracellular matrix ligands, such as 43 kDa glycoprotein (gp43), enolase and glyceraldehyde-3-phosphate dehydrogenase (GAPDH) (Vicentini et al., 1994; Mendes-Giannini et al., 2004; Barbosa et al., 2006; Donofrio et al., 2009). These adhesins may be used in the adhesion process, the initial phase of biofilm formation. In recent studies, GP43, GAPDH, and proteinase genes were considered to be overexpressed in the biofilm (Sardi Jde et al., 2015). Then, we hypothesize that agglutinin-like sequence (Als) surface proteins optimize the physical interaction and co-aggregation between C. albicans and P. brasiliensis and the cell-wall mannan-residues might act as a receptor to extracellular polysaccharides, which in turn act as a bridge, facilitating the adhesion between both microorganisms. It is unclear though, at this moment, which of those adhesion mechanisms are the most predominant and whether other adhesion mechanisms might be involved in such interactions. These interactions between two organisms can induce additional responses among themselves and modify the immediate environment to influence pathogenesis. Although we have begun to investigate the consequences of these interactions, much is still unknown. This highlights the need for future mechanistic studies to be properly designed to address those questions.
Galleria mellonella has been presented as a useful invertebrate model to assess the fungi virulence, innate immune response and antifungal efficacy due to the ease of breeding it in the laboratory as well as in carrying out experiments with the larvae (Fuchs and Mylonakis, 2006). The model is especially advantageous in the study of dimorphic fungi due to the possibility that the larvae are kept at 37°C during survival assays, which represents the conditions of human physiology and contributes to maintaining the yeast phase of fungi such as Paracoccidioides spp. (Singulani et al., 2018). More recently, G. mellonella has been used to evaluate co-infection between fungi (Alcazar-Fuoli et al., 2015) or fungus and bacteria (Kean et al., 2017; Rossoni et al., 2017; Reece et al., 2018; Sheehan et al., 2020). Furthermore, some results are similar to those found in mammal models (Sheehan et al., 2020). Here, we used the G. mellonella model to evaluate the virulence of mono-species and dual-species P. brasiliensis and C. albicans biofilm formation. Cells from biofilms usually exhibit different phenotypes compared to planktonic cells and are associated with a higher pathogenicity of the microorganisms, including resistance to host immune mechanisms (Martinez and Fries, 2010). In this aspect, our results showed that cells released from the disrupted biofilms increased the virulence of both fungi compared to their planktonic cells and consequently, it decreased the survival rate of the larvae. Similar results were presented for Cryptococcus neorfomans in a previous study (Benaducci et al., 2016). Furthermore, the comparison of larvae infected with mono-species and dual-species biofilms presented the difference between P. brasiliensis or C. albicans and P. brasiliensis plus C. albicans, especially for biofilm formation. This means that the larvae survival on dual-species biofilms was lower when compared to survival with mono-species biofilms. Thus, our results from G. mellonella are based on in vitro assays since dual-species biofilms showed greater biomass and metabolic activity, and therefore were more virulent than mono-species biofilms. Finally, our data may indicate that P. brasiliensis and C. albicans co-existence is likely to occur on oral mucosal as biofilms, using an in vivo model. In the same way, when G. mellonella larvae were infected with C. albicans and Staphylococcus aureus, there was an increase in the virulence of these microorganisms and the antifungal resistance, which indicated a synergic interaction (Kean et al., 2017).
One may argue that the dynamics of dual-species biofilm growth could be affected by the different growth kinetics of P. brasiliensis and C. albicans and that both strains should ideally present similar growth curves. We acknowledge that the maturity of P. brasiliensis mono-species biofilms was reached later compared with C. albicans biofilms. This way, we might consider the contribution of C. albicans to dual-species biofilms (in terms of biofilm dry weight, biofilm metabolic activity and infectivity on the G. mellonella model) to be greater than P. brasiliensis. Paracoccidioides and Candida are often co-isolated in the oral mucosa. This presence may represent a polymicrobial association with mutual collaboration or advantage for one of the agents, that can lead to more exacerbated clinical and pathological manifestations. Moreover, the comparison of larvae infected with mono- and dual-species biofilms cells showed a significant difference between C. albicans and P. brasiliensis plus C. albicans (p < 0.05), which can be a consequence of virulence factors and quorum sensing mechanisms of biofilms.
Irrespective of any difference in growth kinetics, both the strains were able to co-exist on the studied dual-species biofilms, suggesting that a fungal-fungal interaction is likely to occur on oral mucosal sites. In fact, an in vivo rodent model should be adopted in further studies to assess both the infectivity of this dual-species biofilms on oral mucosal surfaces and the elicited immune-response against those fungal biofilms. Thus, a deeper understanding of the adhesion and signaling mechanisms involved in the interactions between both fungi will provide a new perspective on the role of known virulence determinants and the relevant factors for both diseases.
Conclusion
This study suggested that the C. albicans and P. brasiliensis can coexist in the same environment and that a fungal-fungal interaction is likely to occur on oral mucosal sites. These data widen the knowledge associated with the dynamics of fungal biofilm growth that can potentially lead to the discovery of new therapeutic strategies for fungal infections.
Data Availability Statement
All datasets generated for this study are included in the article/supplementary material.
Author Contributions
LO and MMG contributed to the research idea and experimental design. LO, KM-A, and NF performed the experiments. LO, KM-A, JS, NF, and RA analyzed the data, prepared, and wrote the manuscript. RA, AF-A, RP, and MMG revised the final draft of the manuscript. All authors contributed to the article and approved the submitted version.
Funding
This study was supported by the Fundação de Amparo à Pesquisa do Estado de São Paulo (FAPESP Grants Nos. 2017/06658-9; 2018/15877-9), the Coordenação de Aperfeiçoamento de Pessoal de Nível Superior–Brasil (PROEX-CAPES), the Conselho Nacional de Desenvolvimento Científico e Tecnológico (CNPq Grants Nos. 431455/2018-0; 310524/2018-2; 134009/2018-5), and the PADC-FCF.
Conflict of Interest
The authors declare that the research was conducted in the absence of any commercial or financial relationships that could be construed as a potential conflict of interest.
References
Alcazar-Fuoli, L., Buitrago, M., Gomez-Lopez, A., and Mellado, E. (2015). An alternative host model of a mixed fungal infection by azole susceptible and resistant Aspergillus spp strains. Virulence 6, 376–384. doi: 10.1080/21505594.2015.1025192
Baena-Monroy, T., Moreno-Maldonado, V., Franco-Martinez, F., Aldape-Barrios, B., Quindos, G., and Sanchez-Vargas, L. O. (2005). Candida albicans, Staphylococcus aureus and Streptococcus mutans colonization in patients wearing dental prosthesis. Med. Oral Patol. Oral Cir. Bucal. 10(Suppl. 1), E27–E39.
Barbosa, M. S., Báo, S. N., Andreotti, P. F., Faria, F. P., Felipe, M. S. S., Feitosa, L. S., et al. (2006). Glyceraldehyde-3-phosphate dehydrogenase of Paracoccidioides brasiliensis is a cell surface protein involved in fungal adhesion to extracellular matrix proteins and interaction with cells. Infect. Immun. 74, 382–389. doi: 10.1128/IAI.74.1.382-389.2006
Bartnicka, D., Karkowska-Kuleta, J., Zawrotniak, M., Satala, D., Michalik, K., Zielinska, G., et al. (2019). Adhesive protein-mediated cross-talk between Candida albicans and Porphyromonas gingivalis in dual species biofilm protects the anaerobic bacterium in unfavorable oxic environment. Sci. Rep. 9:4376.
Benaducci, T., Sardi Jde, C., Lourencetti, N. M., Scorzoni, L., Gullo, F. P., Rossi, S. A., et al. (2016). Virulence of Cryptococcus spp. biofilms in vitro and in vivo using Galleria mellonella as an alternative model. Front. Microbiol. 7:290. doi: 10.3389/fmicb.2016.00290
Bertolini, M., and Dongari-Bagtzoglou, A. (2019). The relationship of Candida albicans with the oral bacterial microbiome in health and disease. Adv. Exp. Med. Biol. 1197, 69–78. doi: 10.1007/978-3-030-28524-1_6
Brogden, K. A., Guthmiller, J. M., and Taylor, C. E. (2005). Human polymicrobial infections. Lancet 365, 253–255. doi: 10.1016/s0140-6736(05)70155-0
Carrero, L. L., Niño-Vega, J., Teixeira, M. M., Carvalho, M. J. A., Soares, C. M. A., Pereira, M., et al. (2008). New Paracoccidioides brasiliensis isolate reveals unexpected genomic variability in this human pathogen. Fungal Genet. Biol. 45, 605–612. doi: 10.1016/j.fgb.2008.02.002
Cattana, M. E., Tracogna, M. F., Marques, I., Rojas, F., Fernandez, M., De Los Angeles Sosa, M., et al. (2017). In Vivo Paracoccidioides spp. biofilm on vascular prosthesis. Mycopathologia 182, 747–749. doi: 10.1007/s11046-017-0126-8
Cavalheiro, M., and Teixeira, M. C. (2018). Candida biofilms: threats, challenges, and promising strategies. Front. Med. 5:28. doi: 10.3389/fmed.2018.00028
Chibebe Junior, J., Sabino, C. P., Tan, X., Junqueira, J. C., Wang, Y., Fuchs, B. B., et al. (2013). Selective photoinactivation of Candida albicans in the non-vertebrate host infection model Galleria mellonella. BMC Microbiol. 13:217. doi: 10.1186/1471-2180-13-217
Coutinho, Z. F., Silva, D., Lazera, M., Petri, V., Oliveira, R. M., Sabroza, P. C., et al. (2002). Paracoccidioidomycosis mortality in Brazil (1980-1995). Cad. Saude. Publica 18, 1441–1454. doi: 10.1590/s0102-311x2002000500037
de Arruda, J. A. A., Schuch, L. F., Abreu, L. G., Silva, L. V. O., Mosconi, C., Monteiro, J., et al. (2018). A multicentre study of oral paracoccidioidomycosis: Analysis of 320 cases and literature review. Oral Dis. 24, 1492–1502. doi: 10.1111/odi.12925
de Barros, P. P., Rossoni, R. D., Freire, F., de Camargo Ribeiro, F., Lopes, L. A. D. C., Junqueira, J. C., et al. (2018). Candida tropicalis affects the virulence profile of Candida albicans: an in vitro and in vivo study. Pathog. Dis. 76:10.
de Carli, M. L., Cardoso, B. C., Malaquias, L. C., Nonogaki, S., Pereira, A. A., Sperandio, F. F., et al. (2015). Serum antibody levels correlate with oral fungal cell numbers and influence the patients’ response to chronic paracoccidioidomycosis. Mycoses 58, 356–361. doi: 10.1111/myc.12325
De Macedo, P. M., Teixeira, M. M., Barker, B. M., Zancopé-Oliveira, R. M., Almeida-Paes, R., and Francesconi do Valle, A. C. (2019). Clinical features and genetic background of the sympatric species Paracoccidioides brasiliensis and Paracoccidioides americana. PLoS Negl. Trop. Dis. 13:4. doi: 10.1371/journal.pntd.0007309
Diaz-Garcia, J., Marcos-Zambrano, L. J., Munoz, P., Guinea, J., and Escribano, P. (2019). Does the composition of polystyrene trays affect Candida spp. biofilm formation? Med. Mycol. 57, 504–509. doi: 10.1093/mmy/myy064
do Carmo Silva, L., Tamayo Ossa, D. P., Castro, S. V., Bringel Pires, L., Alves De Oliveira, C. M., Conceição Da Silva, C., et al. (2015). Transcriptome profile of the response of Paracoccidioides spp. to a camphene thiosemicarbazide derivative. PLoS One 10:e0130703. doi: 10.1371/journal.pone.0130703
Dongari-Bagtzoglou, A., Kashleva, H., Dwivedi, P., Diaz, P., and Vasilakos, J. (2009). Characterization of mucosal Candida albicans biofilms. PLoS One 4:e7967. doi: 10.1371/journal.pone.0007967
Donofrio, F. C., Calil, A. C. A., Miranda, E. T., Almeida, A. M. F., Benard, G., Soares, C. P., et al. (2009). Enolase from Paracoccidioides brasiliensis: isolation and identification as a fibronectin-binding protein. J. Med. Microbiol. 58, 706–713. doi: 10.1099/jmm.0.003830-0
Douglas, L. J. (2003). Candida biofilms and their role in infection. Trends Microbiol. 11, 30–36. doi: 10.1016/s0966-842x(02)00002-1
Ells, T. C., and Truelstrup Hansen, L. (2006). Strain and growth temperature influence Listeria spp. attachment to intact and cut cabbage. Int. J. Food Microbiol. 111, 34–42. doi: 10.1016/j.ijfoodmicro.2006.04.033
Epstein, J. B., Gorsky, M., and Caldwell, J. (2002). Fluconazole mouthrinses for oral candidiasis in postirradiation, transplant, and other patients. Oral Surg. Oral Med. Oral Pathol. Oral Radiol. Endod. 93, 671–675. doi: 10.1067/moe.2002.122728
Falsetta, M. L., Klein, M. I., Colonne, P. M., Scott-Anne, K., Gregoire, S., Pai, C. H., et al. (2014). Symbiotic relationship between Streptococcus mutans and Candida albicans synergizes virulence of plaque biofilms in vivo. Infect. Immun. 82, 1968–1981. doi: 10.1128/iai.00087-14
Fangtham, M., Magder, L. S., and Petri, M. A. (2014). Oral candidiasis in systemic lupus erythematosus. Lupus 23, 684–690. doi: 10.1177/0961203314525247
Fava Netto, C., Vegas, V. S., Sciannamea, I. M., and Guarnieri, D. B. (1969). Antígeno polissacarídico do Paracoccidioides brasiliensis. Estudo do tempo de cultivo do P. brasiliensis necessário ao preparo do antígeno. Rev. Inst. Med. Trop. Sao Paulo 11, 177–181.
Fotos, P. G., Vincent, S. D., and Hellstein, J. W. (1992). Oral candidosis. Clinical, historical, and therapeutic features of 100 cases. Oral Surg. Oral Med. Oral Pathol. 74, 41–49.
Franco, M., Montenegro, M. R., Mendes, R. P., Marques, S. A., Dillon, N. L., and Mota, N. G. (1987). Paracoccidioidomycosis: a recently proposed classification of its clinical forms. Rev. Soc. Bras. Med. Trop. 20, 129–132. doi: 10.1590/s0037-86821987000200012
Fuchs, B. B., and Mylonakis, E. (2006). Using non-mammalian hosts to study fungal virulence and host defense. Curr. Opin. Microbiol. 9, 346–351. doi: 10.1016/j.mib.2006.06.004
Gauthier, G. M. (2017). Fungal dimorphism and virulence: molecular mechanisms for temperature adaptation, immune evasion, and in vivo survival. Mediat. Inflamm. 2017:8491383.
Hawser, S. P., and Douglas, L. J. (1994). Biofilm formation by Candida species on the surface of catheter materials in vitro. Infect. Immun. 62, 915–921. doi: 10.1128/iai.62.3.915-921.1994
Jin, Y., Samaranayake, L. P., Samaranayake, Y., and Yip, H. K. (2004). Biofilm formation of Candida albicans is variably affected by saliva and dietary sugars. Arch. Oral. Biol. 49, 789–798. doi: 10.1016/j.archoralbio.2004.04.011
Kean, R., Rajendran, R., Haggarty, J., Townsend, E. M., Short, B., Burgess, K. E., et al. (2017). Candida albicans mycofilms support Staphylococcus aureus colonization and enhances miconazole resistance in dual-species interactions. Front. Microbiol. 8:258. doi: 10.3389/fmicb.2017.00258
Kernien, J. F., Snarr, B. D., Sheppard, D. C., and Nett, J. E. (2017). The interface between fungal biofilms and innate immunity. Front. Immunol. 8:1968. doi: 10.3389/fimmu.2017.01968
Martinez, L. R., and Casadevall, A. (2006). Susceptibility of Cryptococcus neoformans biofilms to antifungal agents in vitro. Antimicrob. Agents Chemother. 50, 1021–1033. doi: 10.1128/aac.50.3.1021-1033.2006
Martinez, L. R., and Casadevall, A. (2015). Biofilm formation by Cryptococcus neoformans. Microbiol. Spectr. 3:6.
Martinez, L. R., and Fries, B. C. (2010). Fungal biofilms: relevance in the setting of human disease. Curr. Fungal Infect. Rep. 4, 266–275. doi: 10.1007/s12281-010-0035-5
Martins, C. H., Pires, R., Cunha, A., Pereira, C., Lacorte Singulani, J., Abrão, F., et al. (2016). Candida/Candida biofilms. First description of dual-species Candida albicans/C. rugosa biofilm. Fungal Biol. 120, 530–537. doi: 10.1016/j.funbio.2016.01.013
Matute, D. R., Sepúlveda, V. E., Quesada, L. M., Goldman, G. H., Taylor, J. W., Restrepo, A., et al. (2006). Microsatellite analysis of three phylogenetic species of Paracoccidioides brasiliensis. J. Clin. Microbiol. 44, 2153–2157. doi: 10.1128/jcm.02540-05
Mendes, R. P., Cavalcante, R. S., Marques, S. A., Marques, M. E. A., Venturini, J., Sylvestre, T. F., et al. (2017). Paracoccidioidomycosis: current perspectives from Brazil. Open Microbiol J 11, 224–282. doi: 10.2174/1874285801711010224
Mendes-Giannini, M. J., Hanna, S. A., Da Silva, J. L., Andreotti, P. F., Vincenzi, L. R., Benard, G., et al. (2004). Invasion of epithelial mammalian cells by Paracoccidioides brasiliensis leads to cytoskeletal rearrangement and apoptosis of the host cell. Microbes Infect. 6, 882–891. doi: 10.1016/j.micinf.2004.05.005
Morris, C. E., Monier, J., and Jacques, M. (1997). Methods for observing microbial biofilms directly on leaf surfaces and recovering them for isolation of culturable microorganisms. Appl. Environ. Microbiol. 63, 1570–1576. doi: 10.1128/aem.63.4.1570-1576.1997
Negrini, T. C., Koo, H., and Arthur, R. A. (2019). Candida-bacterial biofilms and host-microbe interactions in oral diseases. Adv. Exp. Med. Biol. 1197, 119–141. doi: 10.1007/978-3-030-28524-1_10
Nicolatou-Galitis, O., Dardoufas, K., Markoulatos, P., Sotiropoulou-Lontou, A., Kyprianou, K., Kolitsi, G., et al. (2001). Oral pseudomembranous candidiasis, herpes simplex virus-1 infection, and oral mucositis in head and neck cancer patients receiving radiotherapy and granulocyte-macrophage colony-stimulating factor (GM-CSF) mouthwash. J. Oral Pathol. Med. 30, 471–480. doi: 10.1034/j.1600-0714.2001.030008471.x
Pathirana, R. U., McCall, A., Norris, R., and Edgerton, M. (2019). Filamentous non-albicans Candida species adhere to Candida albicans and benefit from dual biofilm growth. Front. Microbiol. 10:1188. doi: 10.3389/fmicb.2019.01188
Peeters, E., Nelis, H. J., and Coenye, T. (2008). Comparison of multiple methods for quantification of microbial biofilms grown in microtiter plates. J. Microbiol. Methods 72, 157–165. doi: 10.1016/j.mimet.2007.11.010
Pemmaraju, S. C., Pruthi, P. A., Prasad, R., and Pruthi, V. (2013). Candida albicans biofilm inhibition by synergistic action of terpenes and fluconazole. Indian J. Exp. Biol. 51, 1032–1037.
Peters, B. M., Jabra-Rizk, M. A., O’May, G. A., Costerton, W., and Shirtliff, M. (2012). Polymicrobial interactions: impact on pathogenesis and human disease. Clin. Microbiol. Rev. 25, 193–213. doi: 10.1128/cmr.00013-11
Pierce, C. G., Uppuluri, P., Tummala, S., and Lopez-Ribot, J. L. (2010). A 96 well microtiter plate-based method for monitoring formation and antifungal susceptibility testing of Candida albicans biofilms. J. Vis. Exp. 21:2287.
Pitangui, N. S., Sardi, J. C., Silva, J. F., Benaducci, T., Moraes Da Silva, R. A., Rodríguez-Arellanes, G., et al. (2012). Adhesion of Histoplasma capsulatum to pneumocytes and biofilm formation on an abiotic surface. Biofouling 28, 711–718. doi: 10.1080/08927014.2012.703659
Ramage, G., Tomsett, K., Wickes, B. L., Lopez-Ribot, J. L., and Redding, S. W. (2004). Denture stomatitis: a role for Candida biofilms. Oral Surg. Oral Med. Oral Pathol. Oral Radiol. Endod. 98, 53–59. doi: 10.1016/j.tripleo.2003.04.002
Reece, E., Doyle, S., Greally, P., Renwick, J., and Mcclean, S. (2018). Aspergillus fumigatus inhibits Pseudomonas aeruginosa in co-culture: implications of a mutually antagonistic relationship on virulence and inflammation in the CF airway. Front. Microbiol. 9:1205. doi: 10.3389/fmicb.2018.01205
Rodrigues, M. E., Gomes, F., and Rodrigues, C. F. (2019). Candida spp./bacteria mixed biofilms. J. Fungi 6:5. doi: 10.3390/jof6010005
Rossoni, R. D., Fuchs, B. B., De Barros, P. P., Velloso, M. D., Jorge, A. O., Junqueira, J. C., et al. (2017). Lactobacillus paracasei modulates the immune system of Galleria mellonella and protects against Candida albicans infection. PLoS One 12:e0173332. doi: 10.1371/journal.pone.0173332
San-Blas, G., and Vernet, D. (1977). Induction of the synthesis of cell wall alpha-1,3-glucan in the yeastlike form of Paracoccidioides brasiliensis strain IVIC Pb9 by fetal calf serum. Infect. Immun. 15, 897–902. doi: 10.1128/iai.15.3.897-902.1977
Sardi Jde, C., Pitangui Nde, S., Voltan, A. R., Braz, J. D., Machado, M. P., Fusco Almeida, A. M., et al. (2015). In vitro Paracoccidioides brasiliensis biofilm and gene expression of adhesins and hydrolytic enzymes. Virulence 6, 642–651. doi: 10.1080/21505594.2015.1031437
Scorzoni, L., De Paula, E., Silva, A. C., Singulani Jde, L., Leite, F. S., De Oliveira, H. C., et al. (2015). Comparison of virulence between Paracoccidioides brasiliensis and Paracoccidioides lutzii using Galleria mellonella as a host model. Virulence 6, 766–776.
Sheehan, G., Tully, L., and Kavanagh, K. A. (2020). Candida albicans increases the pathogenicity of Staphylococcus aureus during polymicrobial infection of Galleria mellonella larvae. Microbiology 166, 375–385. doi: 10.1099/mic.0.000892
Silva, S., Henriques, M., Oliveira, R., Williams, D., and Azeredo, J. (2010). In vitro biofilm activity of non-Candida albicans Candida species. Curr. Microbiol. 61, 534–540. doi: 10.1007/s00284-010-9649-7
Simoes, L. C., Simoes, M., and Vieira, M. J. (2010). Adhesion and biofilm formation on polystyrene by drinking water-isolated bacteria. Antonie Van Leeuwenhoek 98, 317–329. doi: 10.1007/s10482-010-9444-2
Singulani, J. L., Scorzoni, L., De Oliveira, H. C., Marcos, C. M., Assato, P. A., Fusco-Almeida, A. M., et al. (2018). Applications of invertebrate animal models to dimorphic fungal infections. J. Fungi 4:118. doi: 10.3390/jof4040118
Sztajer, H., Szafranski, S. P., Tomasch, J., Reck, M., Nimtz, M., Rohde, M., et al. (2014). Cross-feeding and interkingdom communication in dual-species biofilms of Streptococcus mutans and Candida albicans. ISME J. 8, 2256–2271. doi: 10.1038/ismej.2014.73
Thein, Z. M., Seneviratne, C. J., Samaranayake, Y. H., and Samaranayake, L. P. (2009). Community lifestyle of Candida in mixed biofilms: a mini review. Mycoses 52, 467–475. doi: 10.1111/j.1439-0507.2009.01719.x
Thomaz, D. Y., Melhem, M. S. C., De Almeida Junior, J. N., Benard, G., and Del Negro, G. M. B. (2020). Lack of efficacy of echinocandins against high metabolic activity biofilms of Candida parapsilosis clinical isolates. Braz. J. Microbiol. 51, 1129–1133. doi: 10.1007/s42770-019-00219-7
Tulasidas, S., Rao, P., Bhat, S., and Manipura, R. (2018). A study on biofilm production and antifungal drug resistance among Candida species from vulvovaginal and bloodstream infections. Infect. Drug Resist. 11, 2443–2448. doi: 10.2147/idr.s179462
Turissini, D. A., Gomez, O. M., Teixeira, M. M., Mcewen, J. G., and Matute, D. R. (2017). Species boundaries in the human pathogen Paracoccidioides. Fungal Genet. Biol. 106, 9–25. doi: 10.1016/j.fgb.2017.05.007
Verstrepen, K. J., and Klis, F. M. (2006). Flocculation, adhesion and biofilm formation in yeasts. Mol. Microbiol. 60, 5–15. doi: 10.1111/j.1365-2958.2006.05072.x
Vicentini, A. P., Gesztesi, J. L., Franco, M. F., De Souza, W., De Moraes, J. Z., Travassos, L. R., et al. (1994). Binding of Paracoccidioides brasiliensis to laminin through surface glycoprotein gp43 leads to enhancement of fungal pathogenesis. Infect. Immun. 62, 1465–1469. doi: 10.1128/iai.62.4.1465-1469.1994
Keywords: Candida albicans, Paracoccidioides brasiliensis, dual-species biofilm, oral cavity, Galleria mellonella
Citation: Oliveira LT, Medina-Alarcón KP, Singulani JL, Fregonezi NF, Pires RH, Arthur RA, Fusco-Almeida AM and Mendes Giannini MJS (2020) Dynamics of Mono- and Dual-Species Biofilm Formation and Interactions Between Paracoccidioides brasiliensis and Candida albicans. Front. Microbiol. 11:551256. doi: 10.3389/fmicb.2020.551256
Received: 12 April 2020; Accepted: 15 September 2020;
Published: 14 October 2020.
Edited by:
Eleftherios Mylonakis, Alpert Medical School of Brown University, United StatesReviewed by:
Renátó Kovács, University of Debrecen, HungaryElisa Borghi, University of Milan, Italy
Sónia Silva, University of Minho, Portugal
Copyright © 2020 Oliveira, Medina-Alarcón, Singulani, Fregonezi, Pires, Arthur, Fusco-Almeida and Mendes Giannini. This is an open-access article distributed under the terms of the Creative Commons Attribution License (CC BY). The use, distribution or reproduction in other forums is permitted, provided the original author(s) and the copyright owner(s) are credited and that the original publication in this journal is cited, in accordance with accepted academic practice. No use, distribution or reproduction is permitted which does not comply with these terms.
*Correspondence: Maria José Soares Mendes Giannini, bWFyaWEuZ2lhbm5pbmlAdW5lc3AuYnI=