- 1Department of Psychiatry and Neuropsychology, School for Mental Health and Neuroscience (MHeNs), Maastricht University, Maastricht, Netherlands
- 2Division of Molecular Psychiatry, Laboratory of Translational Neuroscience, Center of Mental Health, Department of Psychiatry, University of Würzburg, Würzburg, Germany
- 3Department of Psychiatry and Psychotherapy, Medical Center – University of Freiburg, Faculty of Medicine, University of Freiburg, Freiburg, Germany
- 4Department of Psychiatry and Psychotherapy, Universitätsmedizin Göttingen, Georg-August-Universität, Göttingen, Germany
- 5Department of Psychiatry, McLean Hospital, Harvard Medical School, Belmont, MA, United States
- 6Departments of Bioinformatics, Psychiatry & Neuro Psychology, NUTRIM School of Nutrition and Translational Research in Metabolism, Maastricht University, Maastricht, Netherlands
- 7Donders Institute for Brain, Cognition and Behaviour, Radboud University, Nijmegen, Netherlands
- 8Department of Biomedical Sciences, University of Veterinary Medicine, Vienna, Austria
- 9Core Unit Systems Medicine, Institute for Molecular Infection Biology, University of Würzburg, Würzburg, Germany
- 10ZB MED – Information Centre for Life Sciences, Cologne, Germany
- 11TH Köln, Faculty of Information Science and Communication Studies, Cologne, Germany
- 12Laboratory of Psychiatric Neurobiology, Institute of Molecular Medicine, I. M. Sechenov First Moscow State Medical University and Institute of General Pathology and Pathophysiology, Moscow, Russia
- 13Center of Mental Health, Department of Psychiatry, Psychosomatics and Psychotherapy, University Hospital of Würzburg, Würzburg, Germany
Converging evidence suggests a role of serotonin (5-hydroxytryptamine, 5-HT) and tryptophan hydroxylase 2 (TPH2), the rate-limiting enzyme of 5-HT synthesis in the brain, in modulating long-term, neurobiological effects of early-life adversity. Here, we aimed at further elucidating the molecular mechanisms underlying this interaction, and its consequences for socio-emotional behaviors, with a focus on anxiety and social interaction. In this study, adult, male Tph2 null mutant (Tph2-/-) and heterozygous (Tph2+/-) mice, and their wildtype littermates (Tph2+/+) were exposed to neonatal, maternal separation (MS) and screened for behavioral changes, followed by genome-wide RNA expression and DNA methylation profiling. In Tph2-/- mice, brain 5-HT deficiency profoundly affected socio-emotional behaviors, i.e., decreased avoidance of the aversive open arms in the elevated plus-maze (EPM) as well as decreased prosocial and increased rule breaking behavior in the resident-intruder test when compared to their wildtype littermates. Tph2+/- mice showed an ambiguous profile with context-dependent, behavioral responses. In the EPM they showed similar avoidance of the open arm but decreased prosocial and increased rule breaking behavior in the resident-intruder test when compared to their wildtype littermates. Notably, MS effects on behavior were subtle and depended on the Tph2 genotype, in particular increasing the observed avoidance of EPM open arms in wildtype and Tph2+/- mice when compared to their Tph2-/- littermates. On the genomic level, the interaction of Tph2 genotype with MS differentially affected the expression of numerous genes, of which a subset showed an overlap with DNA methylation profiles at corresponding loci. Remarkably, changes in methylation nearby and expression of the gene encoding cholecystokinin, which were inversely correlated to each other, were associated with variations in anxiety-related phenotypes. In conclusion, next to various behavioral alterations, we identified gene expression and DNA methylation profiles to be associated with TPH2 inactivation and its interaction with MS, suggesting a gene-by-environment interaction-dependent, modulatory function of brain 5-HT availability.
Introduction
The serotonin (5-hydroxytryptamine; 5-HT) system is one of the brain’s key neuromodulatory systems and as such involved in the regulation of manifold behaviors (Lesch et al., 2012; Paul and Lowry, 2013; Ottenhof et al., 2018). Mice, carrying a constitutive, genetic tryptophan hydroxylase 2 (TPH2) inactivation (Gutknecht et al., 2008), which results in a brain 5-HT reduction of approximately 90% (Gutknecht et al., 2012), were found to display a distinctive behavioral phenotype (Mosienko et al., 2015). Lifelong 5-HT deficiency is associated with altered anxiety-related behaviors, locomotor activity and social behavior. Next to its direct effects on behavior, 5-HT system function is known to modulate the effects of aversive experiences throughout life (Bennett et al., 2002; Caspi et al., 2002, 2003; Champoux et al., 2002; van den Hove et al., 2011; Gutknecht et al., 2015; Sachs et al., 2015; Wong et al., 2015).
Stress throughout development and further along the lifespan has the capacity to affect the stress response and stress-related behaviors later in life by reprogramming the reactivity of limbic brain areas (Lupien et al., 2009). Stress-induced adaption in limbic function is related to alterations in the 5-HT system (Márquez et al., 2013). Accordingly, genetic TPH2 inactivation induces distinct reactivity of various brain regions (Waider et al., 2017; Auth et al., 2018). Complete brain 5-HT deficiency is concomitant with an altered reactivity of basal amygdala, paraventricular nucleus (PVN) and periaqueductal gray (PAG) to diverse challenges. Moreover, stress was found to affect factors of the 5-HT system directly. Exposure to maternal separation (MS) is associated with increased monoamine oxidase a (Mao-a) expression in striatum and brain stem (Wong et al., 2015), decreased 5-HT immunoreactivity in the hypothalamus (Veenema et al., 2006) as well as increased Tph2 expression in the dorsal raphe (DR), in the context of social challenges (Gardner et al., 2009). Thus, while the 5-HT system modulates the effects of stress, stress directly affects 5-HT system function, reflecting an interaction of genetic and environmental factors. At the core of this interaction, the 5-HT system acts as a modulatory interface, regulating the effects of early experiences. 5-HT system function, during the early postnatal period, is vital for the formation and fine tuning of 5-HT projection connectivity in several limbic brain regions. Migliarini et al. (2013) showed that lack of 5-HT reduces the density of serotonergic fibers in the suprachiasmatic nucleus, the hypothalamus and the thalamic PVN, while it increases the density of 5-HT fibers in nucleus accumbens and hippocampus. A suggested potential mechanism for this highly specific connectivity pattern is a 5-HT-dependent regulation of brain derived neurotrophic factor (Bdnf). In support of this view, Bdnf expression was linked to early, 5-HT-induced epigenetic modifications at the Bdnf gene, (Boulle et al., 2016). Another study investigating the involvement of 5-HT in modulating the epigenetic landscape, indicated that 5-HT signaling during early life facilitates the recruitment of chromatin-remodeling complexes, and transcription factors, such as cAMP response element binding protein (CREB) binding protein and early growth response protein 1 (Hellstrom et al., 2012), resulting in an altered epigenetic regulation of stress-relevant genes such as the glucocorticoid receptor and, consequently the activity of the stress axis (Zhang et al., 2013). Of note, most effects of early-life adversity are sex-specific with regard to behavior, stress responses and 5-HT system mediation (McCormick et al., 1995; Veenema et al., 2007; García-Cáceres et al., 2010; van den Hove et al., 2014; Hiroi et al., 2016).
Taken together, an interaction of 5-HT system function and early-life adversity has already been shown in several studies. However, an investigation of the molecular correlates of the altered behavior, with a focus on socio-emotional behavior and in the context of epigenetic regulation, has not yet been performed. In the present study, we aimed to further elucidate the role brain 5-HT plays in the epigenetic mediation of early, postnatal adversity and the effects this interaction exerts on the behavioral phenotype, with a focus on territorial, social behaviors, including aggression, in adult male mice. To reveal molecular mechanisms that are vital for the integration of environmental cues and stress response, we targeted the amygdala, as one of the core components controlling limbic-mediated, emotional processes, which is thoroughly innervated and modulated by 5-HT projections (Asan et al., 2013), for the genome-wide analysis of RNA expression and DNA methylation.
Materials and Methods
Animals and Procedures
All experiments were performed in accordance with the European Parliament and Council Directive (2010/63/EU) and approved by local authorities (Government of Lower Franconia, Würzburg: 55.2-2531.01- 57/12). All efforts were made to minimize numbers and suffering of animals.
Mice of the parental generation were housed in sex-specific groups of 2–7 under a 14 h/10 h light-dark cycle, with lights on at 7 AM–9 PM, in climate-controlled rooms (21 ± 1°C, humidity 45–55%). Standard rodent chow and water were available ad libitum. For breeding purposes, 22 male and 44 female Tph2+/- mice, backcrossed on C57BL/6N for more than 15 generations, and approximately 3 months of age, were put together in standard (267 × 207 × 140 mm) polysulfone cages (Tecniplast Deutschland GmbH, Hohenpeissenberg, Germany) with woodchips and standard nesting material in a 1:2 breeding design. The number of breeding pairs was calculated based on the targeted group size of 12 animals per genotype and condition, and based on the Hardy-Weinberg equilibrium, a 1:1 sex-ratio, and an estimated breeding success rate of 75%. All mating pairs were separated after 5 days and females that had a vaginal plug at least once were housed individually from then onward. Females were weighed before mating and 4, 7 and 10 days after separation. Animals that did not show any weight gain over the first 10 days after separation were mated again, with different males. From 14 days after separation of the breeding pairs, nests were checked for pups once per day. The day of birth was declared postnatal day (P)0. No cage changes were performed until P5. Litter size was not normalized at birth for amount of pups of various genotypes, sex or total litter size, owed to the higher mortality of Tph2 null mutant (Tph2-/-) offspring (Alenina et al., 2009). Furthermore, due to their growth retardation, the determination of sex was more difficult in Tph2-/- animals, in particular before the age of weaning. Thus, to ensure a sufficient amount of Tph2-/- males no adjustments were made pre-weaning. Only litters of 5 or more pups were included. Thus, litter size of included litters ranged from 5 to 11 pups per litter, with an average litter size of 8.1 ± 0.3 pups per litter. At P2, litters were randomly assigned to MS and control condition. 23 litters and their respective nesting material were removed from the maternal cage for 3 h/day P2 through 15, while dams remained in their respective cages. Of 23 MS litters 6 were excluded post-weaning, due to imbalanced sex-ratio and/or to avoid litter effects of isolated genotypes, i.e., for example litters with only Tph2+/- males, leaving 17 MS litters. An equal number of litters (17) had been subjected to standard facility rearing and served as control. Pups were weaned at P24 ± 2. For all following procedures, we used only male offspring. After weaning, mice were housed individually in standard cages under a 12 h light-dark cycle, with lights on 1 AM–1 PM. Physiological parameters of mothers and pups are reported in the Supplementary Information (Data Sheet 1). We found no MS effect on maternal weight, relative pup weight or pup survival and observed a lower weight in Tph2-/- animals throughout adulthood (Supplementary Tables 1, 2; Data Sheet 1; Weidner, 2018).
Adult males of both control and MS litters were subjected to behavioral screening starting at approximately 2 months of age. All tests were conducted during the dark phase. In brief, mice were tested for anxiety-related behavior, using the dark-light box (DLB) test (Crawley and Goodwin, 1980; Onaivi and Martin, 1989) followed by open-field (OF) test under red-light conditions to determine locomotor activity (Post et al., 2011) and elevated plus-maze (EPM) test as another anxiety test (Lister, 1987; Post et al., 2011; Gutknecht et al., 2015). Mice were tracked using infrared light from below the respective apparatus (Post et al., 2011). Trials were recorded from above, using an infrared-sensitive CCD camera. Behavioral analysis was performed, using VideoMot2 tracking software (TSE Systems, Bad Homburg, Germany). Each animal was allowed to rest for at least 6 days between trials. The experimental procedures and measures of DLB, EPM and OF are described in more detail in the Supplementary Methods (Data Sheet 1). Following the anxiety-related behavioral test battery animals were tested in a repeated resident-intruder test (RIT). The RIT is an aggression test, which is based on the naturally occurring territoriality, observed in male mice. The used protocol was adapted from a protocol employed in rats (Koolhaas et al., 2013), as described in the following. In preparation of the test, in order to keep olfactory cues stable and, thus, reinforce natural territoriality in the residents, a small amount of soiled sawdust was carried over at every cage change. From 5 days prior to testing until 1 day after testing, cages were not changed. Intruder males were approximately 2 months old DBA2/N males (Charles River, Sulzfeld, Germany). They were housed in groups of six as described for the parental generation and were allowed to adapt for at least 20 days before testing started. All intruders were weighed prior to the first encounter to allow weight matching them to a respective resident. The heaviest intruder was determined for each cage and excluded from testing. These animals were used prior to the first RIT for instigation. Each instigation lasted 5 min, allowing to stimulate residents by visual and olfactory exposure to an intruder without the possibility of physical contact (de Almeida and Miczek, 2002; de Almeida et al., 2005). 3 min after the instigation ended the actual intruder was introduced into the resident’s home cage. The RIT was performed under red-light. In previous studies it has been reported that the initially observed level in aggression might not be representative of the true innate aggressive potential (Winslow and Miczek, 1984; Miczek et al., 2001; Caramaschi et al., 2008). Therefore, the RIT should be repeated several times, to allow animals to reach a stable level of offensive behavior. In the current study, the RIT was repeated four times with 24 h intervals between each encounter. For each encounter the resident was matched with an unfamiliar intruder. The test time added up to 5 min from the first attack bite (as observed online) or maximum 10 min. Encounters were filmed from a side-on view for subsequent behavioral analysis. An infrared-sensitive high-speed camera (The Imaging Source, Bremen, Germany) was used. Following each encounter, resident and intruder were examined for wounds. Recordings of the first and last of four encounters were analyzed in detail for all animals that were further screened for whole genome RNA expression and DNA methylation (n = 8–10 per group) using the Observer XT software (Noldus, Wageningen, Netherlands). Behaviors were scored in 5 categories, i.e., non-social, behavior independent of the intruder [rearing, digging, self-grooming and walking]; prosocial, affiliative behavior [sniffing, grooming, turning to, following, and looking toward (Miczek et al., 2001; Natarajan and Caramaschi, 2010; Koolhaas et al., 2013)], dominant, behavior to establish a clear hierarchy [grooming, mounting, and following, while the intruder showed clear signs of submission (Natarajan and Caramaschi, 2010; Wang et al., 2014)], threat, display of warning signs [tail rattling, sideway threatening, up-right posturing, boxing, aggressive grooming and fast following (Koolhaas et al., 2013)] and aggression [clinching, keeping down, biting, kicking and chasing (Koolhaas et al., 2013)]. The accumulated amount of time spent for each category was then analyzed relative to the total amount of time the animals spent in the encounter and is reported as percentage. Furthermore, to determine the quality of attacks, bite targets were scored in detail. To gain insight into rule breaking, we distinguished bites aimed at the back, throat, belly, face, paws and tail of the opponents. For further references, bites aimed at the back were declared social, as the targeted areas are non-vulnerable areas. Attacks aimed at the belly, face, paws throat and tail were determined unsocial, as they aimed at body parts, where vital organs are located or that are more prone to infection (Haller et al., 2001, 2005a; Blanchard et al., 2003; Koolhaas et al., 2013; Takahashi and Miczek, 2014). Besides bite targets, the occurrence of tail rattle, grooming, sniffing and mounting were scored.
Measurement of Fecal Corticosterone Metabolites
To investigate functionality of the hypothalamic-pituitary-adrenal (HPA) axis, in a non-invasive manner, fecal boli, accumulated over the 7 day period between cage changes, were collected. This was done at three time-points throughout the experimental timeline: once before behavioral testing, once after EPM exposure and a last time at the day of sacrifice. Following sample collection, fecal boli were stored at -20°C, until further processing. Fecal corticosterone metabolites (FCMs) were extracted (50 mg powdered feces plus 1 ml 80% methanol) and, subsequently, measured with a 5α-pregnane-3ß,11ß,21-triol-20-one enzyme immunoassay (EIA) that was developed and successfully validated for mice (Touma et al., 2003, 2004).
Epigenetic Regulation
For the investigation of epigenetic regulation both RNA and DNA were extracted from amygdala tissue of 8 animals per condition (48 animals in total), as described in more detail elsewhere (van den Hove et al., 2011; Schraut et al., 2014). RNA sequencing was performed by IGA Technologies (Udine, Italy) using the TrueSeq Stranded Total RNA kit and the Illumina HiSeq 2500 platform at a read-length of 125 bp with paired-end reads (60 million reads/sample). Mapping to the mus musculus GRCm38.p5 genome was performed by the Core Unit Systems Medicine at the University of Würzburg. To analyze DNA methylation, DNA capture-based sequencing was performed by Nxt-Dx (Ghent, Belgium). DNA was sheared by sonication followed by DNA capture using the methyl-CpG-binding domain (MBD) of human methyl-CpG binding protein 2 (MeCP2). Paired-end sequencing with 50 bp read-length (20 million reads/sample) was performed on the Illumina HiSeq4000 platform. Reads were mapped to the mus musculus GRCm38.p5 genome using Bowtie2 v2.1.0 software. After mapping, coverage peaks were generated using MACS 14 peak caller v1.4.2 (Zhang et al., 2008). Subsequently, peaks were aligned and sequencing reads within overlapping peak-sets were counted using the DiffBind R-package v2.0.9 (Stark and Brown, 2013). This resulted in count-tables of the methylated loci. Loci were annotated based on the first nearest feature, using ChIPpeakAnno v3.8.9 (Zhu et al., 2010) with the TxDb.Mmusculus.UCSC.mm10.ensGene v3.4.0. (2016) and ensemble-based EnsDb.Mmusculus.v75 v2.1.0 (Rainer, 2016) annotation packages. Sequencing data is deposited in the Gene Expression Omnibus database (identifier: GSE110330) and a more detailed description of RNA and DNA extraction and sequencing can be found in the Supplementary Methods (Data Sheet 1).
Statistical Analysis
For statistical analysis of behavior, SPSS Statistics Version 23 or higher (IBM Deutschland GmbH, Ehningen, Germany) was used. Data was examined for normal distribution and outliers, using the Shapiro-Wilk test and boxplots. As a considerable number of factors did not meet assumptions for parametrical testing, Kruskal-Wallis tests, with grouping variables Tph2 genotype, MS or experimental group (Tph2∗MS), were performed to test for main effects. Main effects were followed-up by Mann-Whitney U tests. For specific aggression parameters, such as target placement of attack bites, we performed Chi-Square test analysis for categorical data, indicating if more animals per grouping variable showed the investigated behavior. P-values were corrected for multiple comparisons per behavioral test, using the false discovery rate (FDR) online calculator1, correcting for measured parameters and applied statistical comparisons. Corrected p < 0.05 was considered significant. A table with all statistical comparisons is displayed in Supplementary Table 3 (Data Sheet 1). The fecal corticosterone metabolite profile was analyzed using repeated, multifactorial analysis of variance (ANOVA; time point, Tph2 genotype and MS) with a Greenhouse-Geisser correction followed by Bonferroni-corrected post hoc analysis. To establish a normal distribution data was square root transformed.
For statistical analysis of RNA expression and DNA methylation, DESeq2 v1.14.1 (Love et al., 2014) was used in the free software environment R v3.3.2 (The R Foundation, Vienna, Austria), using default settings. Contrasts were calculated for Tph2∗MS interactions GE1 [(Tph2+/- MS-Tph2+/- C)-(Tph2+/+ MS-Tph2+/+ C)] and GE2 [(Tph2-/- MS-Tph2-/- C)-(Tph2+/+ MS-Tph2+/+ C)]. For RNA sequencing, this resulted in lists of differentially expressed genes (DEGs), comprising base mean of counts/gene, ratio on a log2 scale (log2 Fold Change; lg2FC), log fold change standard error, Wald statistic, p-value and adjusted p-value (Supplementary Table 4; Data Sheet 1). For this analysis, only genes with a base mean > 0 were taken into account and one gene (i.e., Lars2) was excluded due to an incomparably high base mean. A similar output was obtained for the analysis of the differentially methylated loci (DMLs) comprising, in addition to statistical results and peak loci, a list of annotated genes (Supplementary Table 5; Data Sheet 1). Pre-analysis quality control revealed two outlier samples, both of the Tph2-/- MS condition, with remarkably lower counts, shifted normalized density and additional small peaks as well as an overall shifted peak in the density histogram of signal intensities (for quality control summary see Supplementary Figures 2, 3; Data Sheet 1). Those two samples were excluded from further analysis. Genes of either analysis were determined differential if they showed a nominal p < 0.01 and lg2FC > | 0.2|. Subsequently, the overlap of DEGs and DML-associated genes was determined and the relationship between overlapping DEG and DML raw counts as well as behavior was investigated using Spearman’s Rho (ρ). Correlation analysis was performed over all conditions. For statistically correlated DEGs and DMLs, differences in MS effects were investigated using Mann-Whitney U test. P-value correction was performed as described earlier. Furthermore, pathway enrichment analysis was conducted using the pathway analysis tool PathVisio (van Iersel et al., 2008; Kutmon et al., 2015), which operates based on the Wikipathways platform (Kelder et al., 2012; Kutmon et al., 2016). A detailed description and results of the pathway analysis are reported in Supplementary Methods and Supplementary Table 6 (Data Sheet 1).
Results
TPH2 Inactivation, Maternal Separation and Anxiety-Related Behaviors and Stress Response
In the DLB test, neither Tph2 genotype, MS nor Tph2∗MS interaction affected the latency to enter the light compartment, distance moved (data not shown) or time spent (Figure 1A) in the dark or light compartment. In the EPM test, an effect of Tph2∗MS interaction was observed for time spent on the open arms [χ2(5) = 19.9, p = 0.008] and of Tph2 genotype in the closed arms [χ2(2) = 10.2, p = 0.029], as depicted in Figure 1B. Post hoc analysis revealed that Tph2-/- MS mice spent more time on the open arms than Tph2+/- (U = 21.0, p = 0.010) and Tph2+/+ (U = 9.0, p = 0.004) MS mice. Furthermore, Tph2-/- mice spent less time in the closed arms when compared to Tph2+/- (U = 166.0, p = 0.014) and Tph2+/+ (U = 153.0, p = 0.013) mice. Similarly, the distance covered in the open arms was influenced by the Tph2 genotype, independent of MS [χ2(5) = 26.6, p = 0.001]. Biallelic TPH2 inactivation increased the distance covered on the open arms, when compared to Tph2+/- (C: U = 14.0, p = 0.010, MS: U = 24.0, p = 0.013) and Tph2+/+ (C: U = 19.0, p = 0.013, MS: U = 21.0, p = 0.010) mice. Also, entries into the open arms, time in the center and distance covered in the center were comparable between conditions and genotypes. In neither test did Tph2 genotype, MS or Tph2∗MS interaction affect the total distance covered, while locomotion over 20 min in a non-aversive open-field was affected by the Tph2 genotype (Supplementary Figure 1; Data Sheet 1; Weidner, 2018).
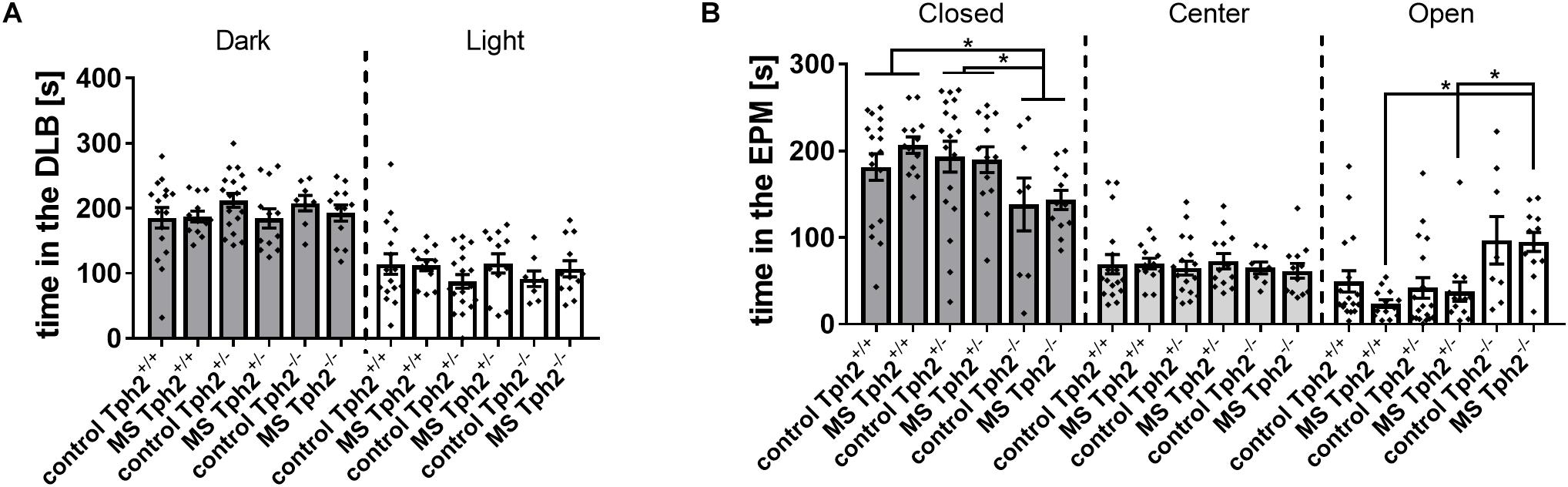
Figure 1. Anxiety-related behaviors in (A) the dark-light box (DLB) and (B) the elevated plus-maze (EPM): DLB performance was unaffected by both tryptophan hydroxylase 2 (Tph2) genotype and maternal separation (MS). In the EPM, full TPH2 inactivation increased the time [s] spent on open arms in offspring exposed to MS and decreased the time spent in closed arms, independent of MS. Bars represent group means ± standard errors (n/group = 8–18). ∗FDR corrected p < 0.050 (Post hoc: Mann-Whitney U).
The FCM profile recorded at three different time points (tp) throughout the experiment showed an effect of Tph2 genotype over time [F(2.9,89.4) = 9.6, p = 2.03E-5] depicted in Figure 2. Over the three time-points (tp1, tp2 and tp3), the profiles of Tph2-/- (tp1-tp2:p = 5.29E-13 tp1-tp3:p = 2.06E-15) and Tph2+/- (tp1-tp2:p = 0.013 tp1-tp3:p = 0.009) mice’s FCM levels changed, while no significant change was observed in Tph2+/+ mice. At different time-points in particular Tph2-/- mice showed a deviating FCM profile. At baseline Tph2-/- mice’s feces showed increased FCM levels compared to the level in Tph2+/- mice’s feces (p = 0.042) and at recovery Tph2-/- mice’s feces showed decreased FCM levels compared to the level in Tph2+/+ mice feces (p = 0.002).
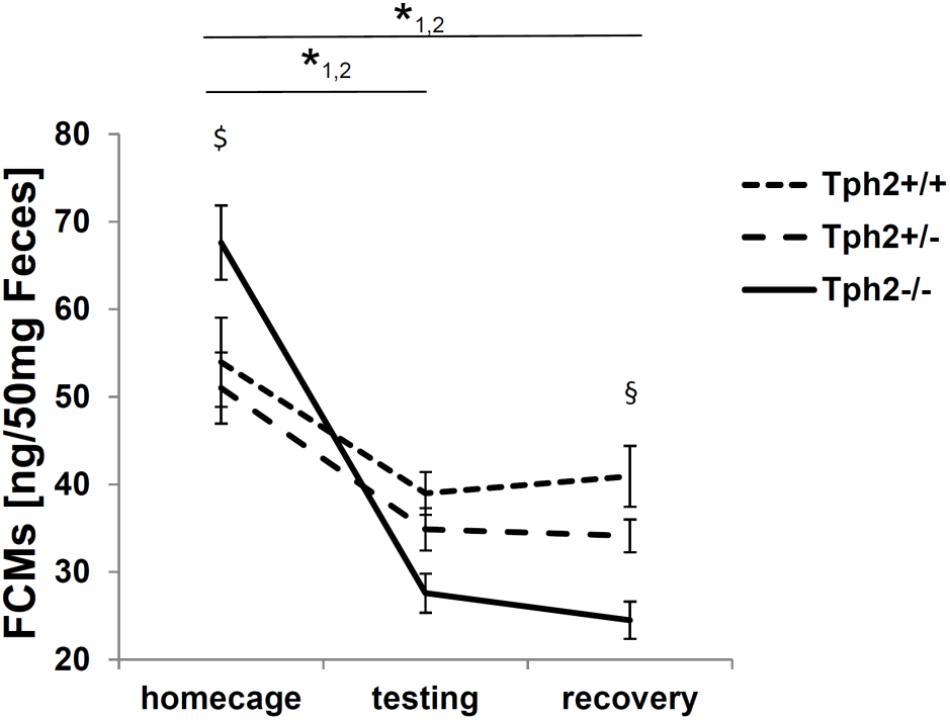
Figure 2. Fecal corticosterone metabolites (FCMs) in 50 mg feces. 1 p < 0.05 for home cage compared to testing and recovery in tryptophan hydroxylase 2 (TPH2)-deficient mice (Tph2-/-). 2 p < 0.05 for home cage compared to testing and recovery in Tph2+/-. $ p < 0.05 Tph2-/- compared to Tph2+/- under home cage conditions. § p < 0.05 Tph2-/- compared to Tph2+/+ after recovery. Data points represent group means ± standard error (n/group = 8–12). Statistical analysis was performed using repeated measures ANOVA followed by Bonferroni corrected t-test.
TPH2 Inactivation, Maternal Separation and Territorial, Social Behaviors
For repeated RIT, the first and the last of four sessions were analyzed. In the first session, none of the classical parameters of RIT, like latency to attack (Figure 3A) or number of attacks (Figure 3B) showed any effect of 5-HT deficiency, MS or a Tph2∗MS interaction. Most prominently, Tph2 genotype was found to affect the total amount of time mice were sniffing the intruder [χ2(2) = 15.8, p = 0.023; Figure 3C], with Tph2-/- mice (U = 38.0, p = 4.42E-4) and Tph2+/- mice (U = 92.0, p = 0.025) spending less time engaged in sniffing in comparison to Tph2+/+ mice.
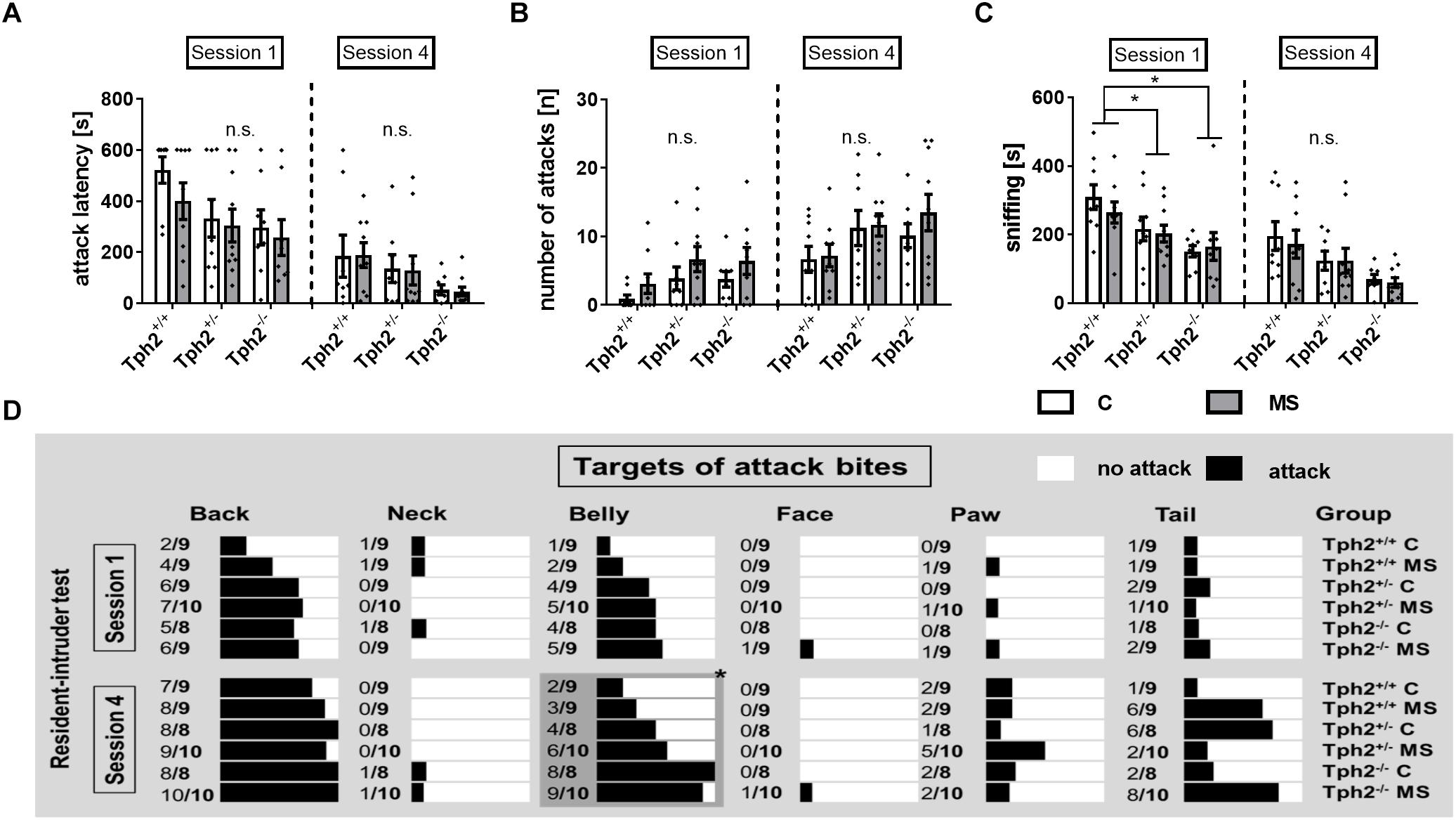
Figure 3. Social behaviors determined using repeated resident-intruder test (RIT). (A) Latency to attack [s] as well as (B) number of attacks [n] were not affected by the animals’ tryptophan hydroxylase 2 (Tph2) genotype, exposure to maternal separation (MS) or Tph2∗MS interaction during the first and fourth session of the RIT. (C) Sniffing [s] during the first session was decreased in mice carrying homo- and heterozygous genetic tryptophan hydroxylase 2 (TPH2) inactivation in both animals of the control (C) and MS condition. (D) During the fourth session targets of attack bites [nominal] of Tph2-/- males were directed more toward vulnerable body parts, i.e., the belly, when compared to Tph2+/- and Tph2+/+ males. (A–C) Bars represent group means ± standard errors (n/group = 8–10). ∗FDR-corrected p < 0.050 (Post hoc: Mann-Whitney U). (D) The relative number of animals per group by attack targets such as back and belly. The black bars represent the % of animals attacking the indicated target. ∗FDR-corrected p < 0.050 (Pearson chi-square test of categorical data, attack vs. no attack, n/group = 8–10).
During the fourth session, neither latency to attack (Figure 3A), nor number of attacks (Figure 3B) were affected by 5-HT deficiency, MS or a Tph2∗MS interaction A significant difference between genotypes, attacking the belly during the fourth session [χ(2) = 16.7, p = 0.023] was observed, with 95% of the Tph2-/- mice targeting this body part at least once, while of Tph2+/- mice 55% and of Tph2+/+ mice 28% attacked the belly (Figure 3D).
TPH2 Inactivation, Maternal Separation and Epigenetic Regulation
Gene expression and DNA methylation profiles were affected by the Tph2∗MS interactions GE1 [(Tph2+/- MS-Tph2+/- C)-(Tph2+/+ MS-Tph2+/+ C)] and GE2 [(Tph2-/- MS-Tph2-/- C)-(Tph2+/+ MS-Tph2+/+ C)] (Weidner, 2018). The results are displayed in Figure 4. For the interaction GE1 309 DEGs (DOWN 84.5%; UP 15.5%) and 1596 DMLs (DOWN 49.8%; UP 50.2%) were identified. The interaction GE2 was associated with 102 DEGs (DOWN 74.5%; UP 25.5%) and 1403 DMLs (DOWN 51.0%; UP 49.0%). Some DMLs were annotated to the same gene.
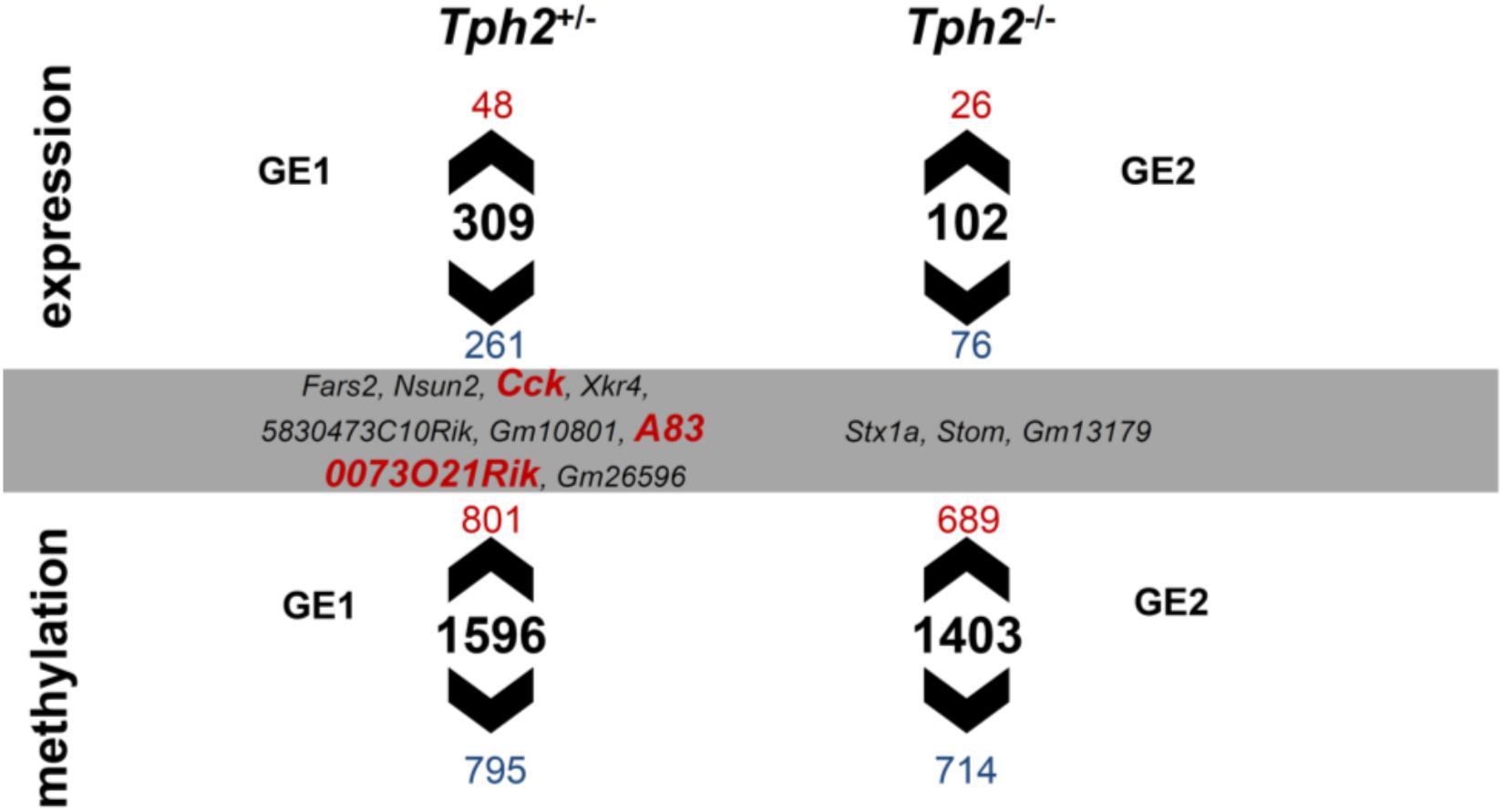
Figure 4. Overlap between differentially expressed genes (DEGs) and differentially methylated loci (DMLs) in the amygdala of mice that were exposed to maternal separation (MS) and are offspring of tryptophan hydroxylase 2-deficient (Tph2+/-) parents. For statistical analysis of RNA expression and DNA methylation, contrasts were calculated for Tph2∗MS interactions GE1 [(Tph2+/- MS-Tph2+/- C)-(Tph2+/+ MS-Tph2+/+ C)] and GE2 [(Tph2-/- MS-Tph2-/- C)-(Tph2+/+ MS-Tph2+/+ C)]. Per group 8 samples (total 48 samples were sequenced for either analysis). For DNA methylation data, pre-analysis quality control revealed two outlier samples, both of the Tph2-/- MS condition, which were excluded from analysis. Genes of either analysis were determined differential if they showed a nominal p < 0.01 and lg2FC > | 0.2|. Fars2, phenylalanine-tRNA synthetase 2 (mitochondrial); Nsun2, NOL1/NOP2/Sun domain family member 2; Cck, cholecystokinin; Xkr4, X-linked Kx blood group related 4; 5830473C10Rik, RIKEN cDNA 5830473C10 gene; Gm10801, predicted gene 10801; A830073O21Rik, RIKEN cDNA A830073O21 gene; Gm26596, predicted gene 26596; Stx1a, syntaxin 1A (brain); Stom, stomatin; Gm13179, predicted gene 13179.
Subsequently, the extent to which DML-associated genes and DEGs overlap was examined (Table 1). Amongst the genes identified in both profiles, only the predicted gene A830073O21Rik (ρ = -0.459, p = 0.002; Figure 5A) and cholecystokinin (Cck) (ρ = -0.343, p = 0.021; Figure 5B), both identified as significantly altered, dependent on the interaction between the Tph2+/+ and Tph2+/- genotype with MS, showed a significant, negative relation between read counts of total RNA and MBD sequencing (Weidner, 2018). Based on these findings we analyzed the effects of Tph2∗MS interaction in detail, and found that, for A830073O21Rik, MS decreased the methylation in Tph2+/+ mice (p = 0.018) as shown in Figure 5C. In addition, Tph2+/- mice of the control condition showed lower A830073O21Rik methylation compared to Tph2+/+ mice of the same condition (p = 0.036) and Tph2+/- mice of the MS condition showed higher A830073O21Rik methylation compared to Tph2+/+ mice of the same condition (p = 0.039). For A830073O21Rik expression the Tph2∗MS interaction could not be attributed to single group comparisons, as none of the post hoc comparisons remained significant after FDR correction, suggesting more subtle effects compared to methylation (Figure 5C). For Cck we observed that MS decreased methylation in Tph2+/+ (p = 0.023) and increased methylation in Tph2+/- mice (p = 0.039) as shown in Figure 5D. In addition, Tph2+/- mice of the MS condition showed a higher Cck methylation compared to Tph2+/+ mice of the same condition (p = 0.009). For Cck expression, similar as observed for A830073O21Rik expression, the Tph2∗MS interaction could not be attributed to single group comparisons (Figure 5D). However, several correlations between the molecular and behavioral readings were observed. We found a positive correlation between A830073O21Rik-related DNA methylation and the distance moved in the light compartment of the DLB (ρ = 0.309, p = 0.034; data not shown). Cck expression positively related to levels of anxiety in the EPM (time open arm: ρ = -0.299, p = 0.039; Figure 6A) and Cck-related DNA methylation showed an opposing relationship with anxiety (time open arm: ρ = 0.381, p = 0.009; Figure 6B; closed arm: ρ = -0.304, p = 0.040; Figure 6D; distance on open arms: ρ = 0.403, p = 0.005; data not shown). Moreover, Cck expression was negatively correlated to threat behavior in the first RIT session (ρ = -0.330, p = 0.025; Figure 6C).
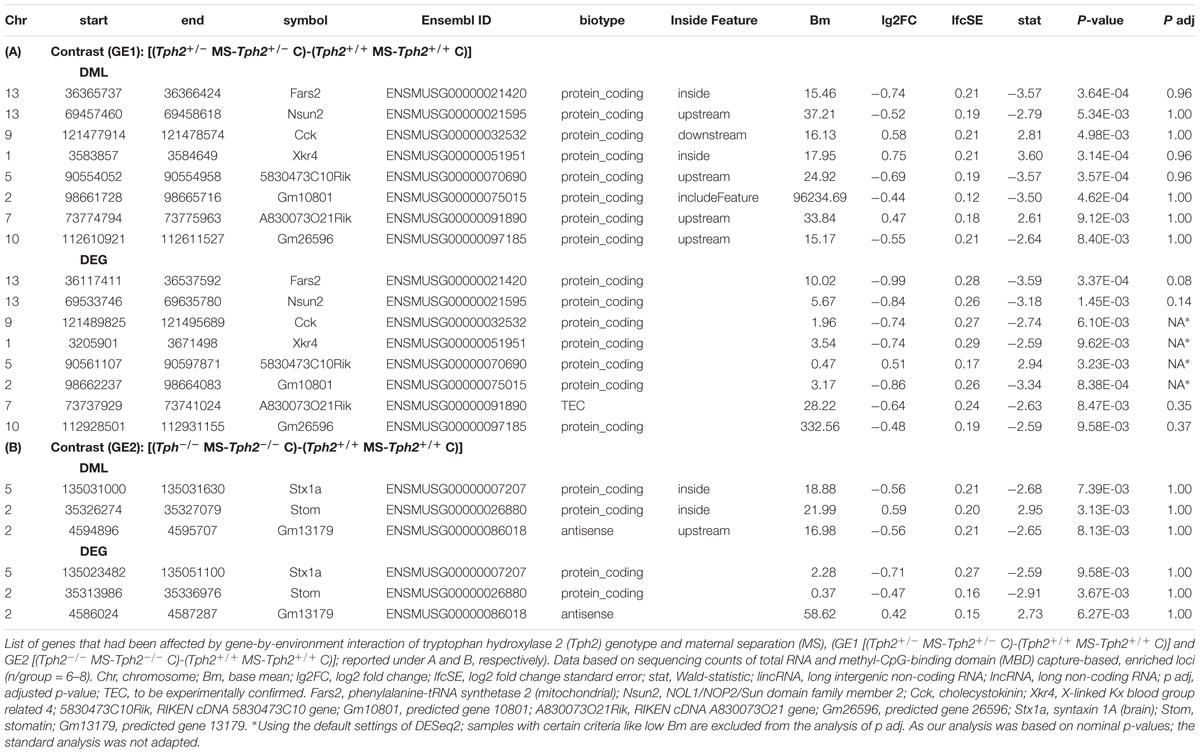
Table 1. Overlapping, differentially methylated loci (DMLs) and differentially expressed genes (DEGs).
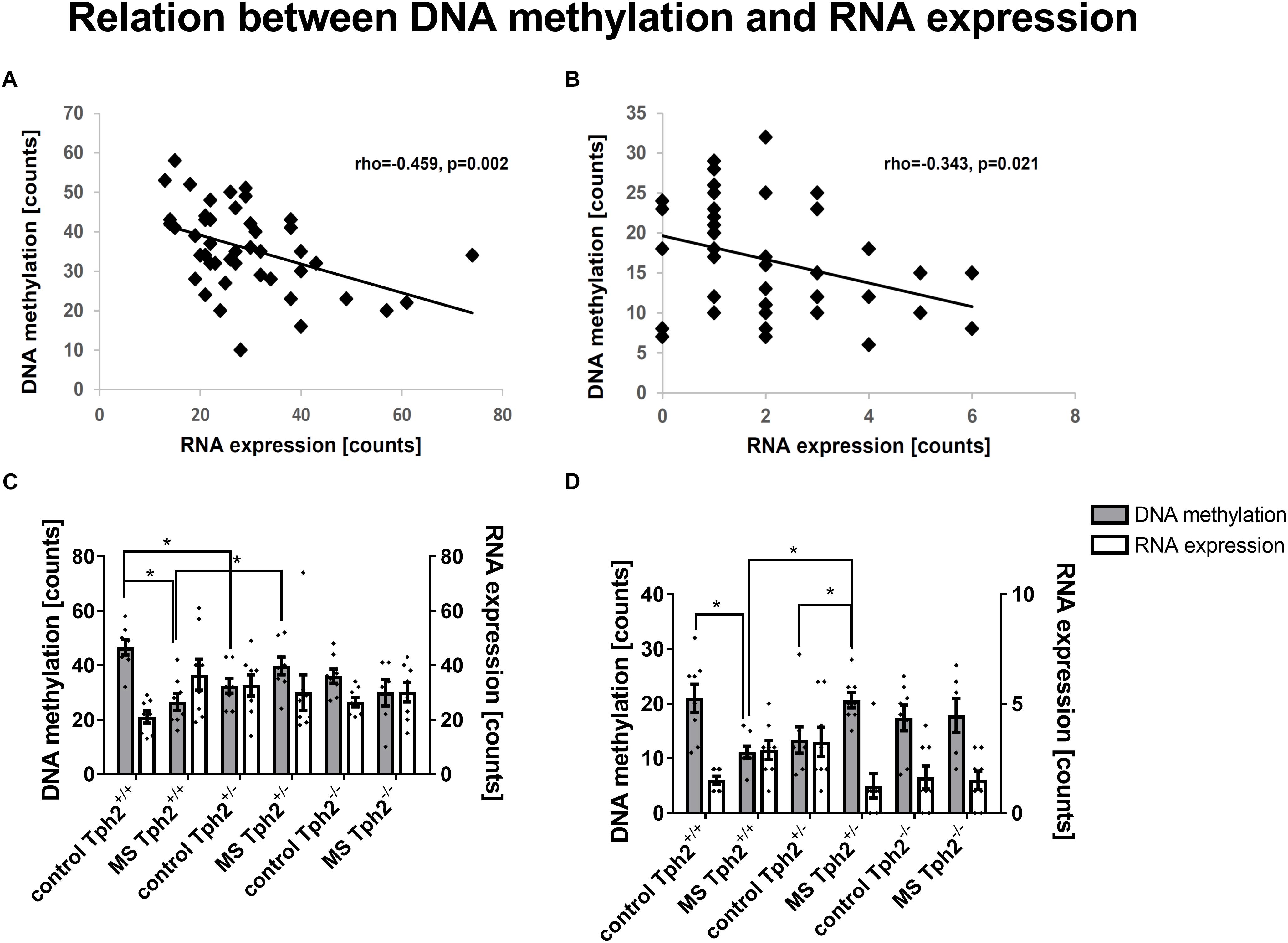
Figure 5. DNA methylation- and RNA expression-related correlations in amygdala. Amongst differentially expressed genes that are furthermore associated with differentially methylated loci, (A) the RIKEN DNA A830073O21Rik and (B) the neuropeptide cholecystokinin (Cck) are correlated amongst each other. Data based on sequencing counts of total RNA and methyl-CpG-binding domain (MBD) capture-based, enriched loci (n/group = 6–8). Correlation analysis was performed using the non-parametrical Spearman correlation (n/group = 5–8). Spearman correlation coefficient rho and p-value are reported. For both genes A830073O21Rik (C) and Cck (D) the comparison GE1 [(Tph2+/- MS-Tph2+/- C)-(Tph2+/+ MS-Tph2+/+ C)] with a significant difference between effect of neonatal, maternal separation (MS) compared to control in tryptophan hydroxylase 2 (Tph2) heterozygous mice and wildtype mice became apparent. Post hoc analysis revealed mainly group differences for A830073O21Rik and Cck methylation (n/group = 6–8). ∗FDR-corrected p < 0.050 (Post hoc: Mann-Whitney U test).
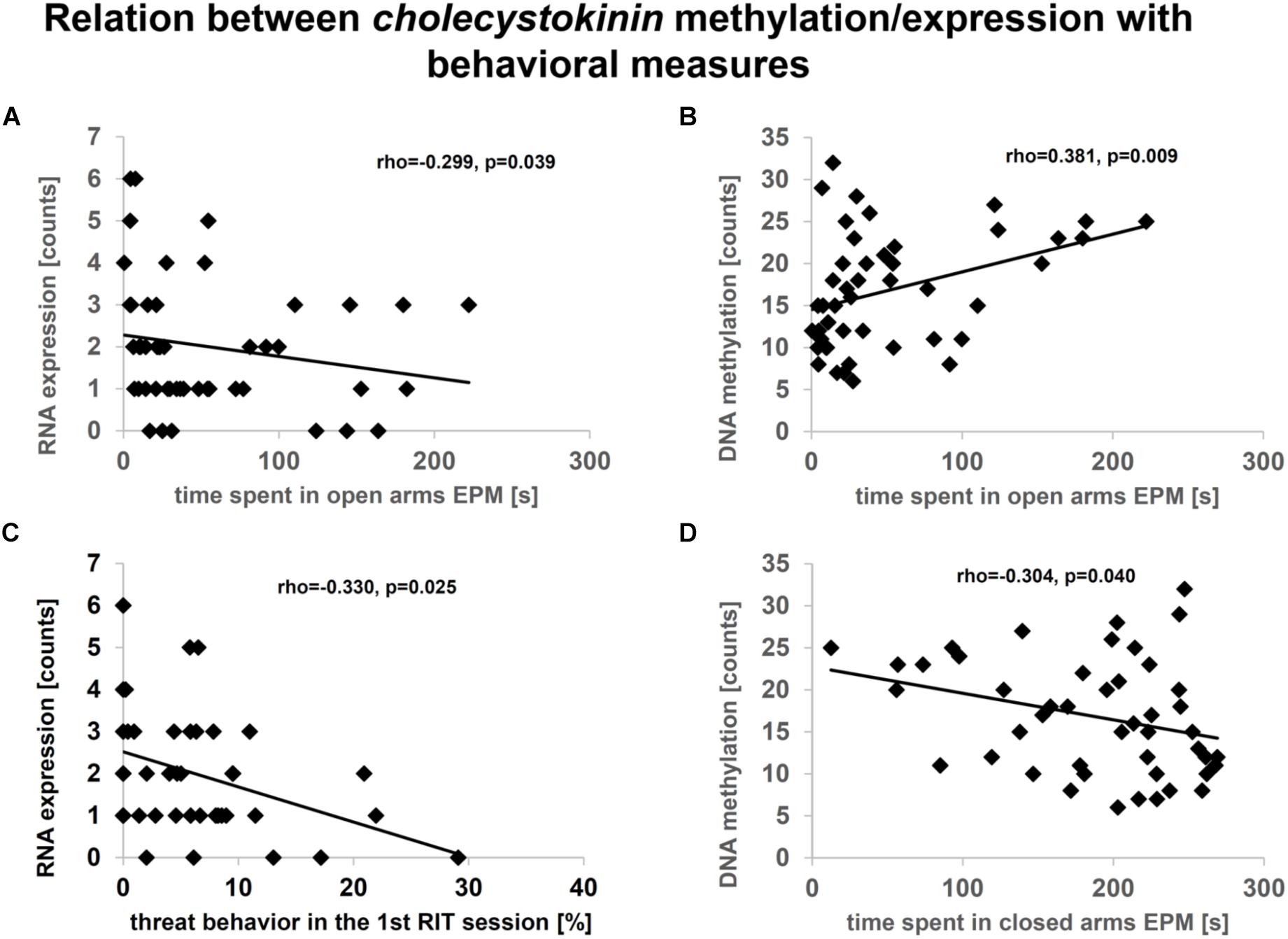
Figure 6. Relationship between cholecystokinin (Cck) expression (A, C) or methylation (B, D) in amygdala and behavior. Data based on sequencing counts of total RNA and methyl-CpG-binding domain (MBD) capture-based, enriched loci (n/group = 6–8). Analysis was performed using the non-parametrical Spearman correlation. Spearman correlation coefficient rho and p-value are reported. Correlation analysis was performed over all conditions.
Discussion
In the present study lifelong TPH2 inactivation and, consequently, depletion of brain 5-HT in Tph2-/- mice induced substantial changes in socio-emotional behaviors, while heterozygous Tph2+/- mice showed a more ambiguous, behavioral profile. Moreover, MS was shown to induce more subtle behavioral effects, which were dependent on the Tph2 genotype. On the molecular level, we were able to relate various observed behavioral changes to expression and methylation of genes in the amygdala, potentially regulated through 5-HT and its interaction with MS.
In accordance with previously reported data (Gutknecht et al., 2015), we observed decreased anxiety in the EPM, as indicated by the time spent on the open arms, in Tph2-/- mice. This was, however, dependent on the experience of MS during early life and Tph2+/- and Tph2+/+ mice of the MS group showed similar levels of anxiety. Interestingly, though the time in closed arms as well as the distance on open arms was affected by Tph2 genotype, independent of MS. Albeit effects in the EPM were very strong, no change was observable in the DLB. The discrepancy between these two approach-avoidance conflict-based anxiety tests might be owed to the nature of challenge and methodology. In the EPM, animals are placed into the center from which they can move into closed arms, which are perceived as safe, or onto open arms, which represent a threatening environment due to their elevated and more exposed nature (Lister, 1990). In the DLB animals are placed into the dark compartment, which is perceived as safe. From there, they are free to decide to move into the light compartment or remain in the dark compartment. Furthermore, in the EPM, appraisal of the situation is more complex due to the set-up of the maze (Casarrubea et al., 2013), while in the DLB, mice know the layout of the adjacent compartment after their first visit. Therefore, the observed deviation of behavior in the EPM seems to represent an altered appraisal in Tph2-/- mice. Furthermore, our data suggests an altered exploratory activity based on MS in Tph2-/- mice, as they spent more time and covered a greater distance on open arms when compared to their Tph2+/+ and Tph2+/- littermates, while Tph2-/- control mice only covered a greater distance, without spending more time on open arms compared to the respective Tph2+/+ and Tph2+/- littermates. In a recent study, we reported a similar interaction of Tph2 genotype, early adversity and acute challenge, in female mice (Auth et al., 2018). The genotype- and stressor-dependent behavioral effects in that study were associated with distinct patterns of brain activity, which furthermore depended on the nature of the acute challenge (i.e., the behavioral test). Findings by other groups, with regard to the effect of 5-HT deficiency on anxiety, were inconsistent (reviewed in Mosienko et al., 2015) and most likely to be accounted for by various factors such as the experimental read-out of the behavioral test used (Arabo et al., 2010) and the genetic background and life histories of investigated animals (Holmes et al., 2003). One hypothesis regarding an alternative read-out describes the time spent on the open arms as probing for a way out, describing increased flight-related behavior, rather than decreased anxiety. Regarding the genetic background, an extensive study, investigating 16 of the most frequently used laboratory mouse lines, discovered a variance of up to 78% between strains. Two of the strains with most opposing results regarding EPM behavior included C57BL6/J mice with an open-arm avoidance index of more than 90% and BALB/cJ mice with an index of 15% (Trullas and Skolnick, 1993). These two strains furthermore, showed opposing profiles when comparing aversive to non-aversive OF. Both strains showed low activity under dim light, with C57BL6 mice increasing their distance travelled under bright light, and BALB/c mice decreasing their distance travelled. Of note, BALB/c J mice carry a functional single nucleotide polymorphism in the Tph2 gene, leading to a decreased TPH2 activity (Zhang et al., 2004; Kulikov et al., 2005; Bach et al., 2011).
Interestingly, monitoring FCM levels throughout the experiment revealed a genotype-dependent profile, which was totally unaffected by MS. We found in particular Tph2-/- males deviated in their FCM profile compared to Tph2+/- and Tph2+/+ mice. In contrast to previously reported, overall lower FCM levels in male Tph2-/- mice, they showed a heightened FCM level at baseline, which might suggest a heightened activity of the HPA axis before behavioral testing in the current study. Following test exposure, Tph2-/- offspring of the current study showed a more profound decrease in FCM levels compared to Tph2+/+ and Tph2+/- mice. Interestingly, this profile seems to correspond with the activation profile of the basolateral amygdala, described before. In that study, Waider et al. (2017) observed a higher neural activation in Tph2-/- males, under home cage conditions. This activation was abolished following exposure to a novel environment. Given the prominent role amygdala activity plays in HPA axis regulation (Herman et al., 2005), these findings might hint at a potential connection between the observed FCM levels and amygdala activity.
The observed, initially higher FCM levels might be explained by post-weaning environment (Hall, 1998). In the present study, males had been single-housed from weaning onward, which has been previously reported to alter the endocrine response in male mice (Bartolomucci et al., 2003). Furthermore, post-weaning social isolation was found to alter exploratory activity (Bartolomucci et al., 2003) as well as increase anxiety-related behaviors, which, only recently, was shown to be dependent on the corticotrophin releasing hormone (CRH) receptor 2 in DR (Bledsoe et al., 2011). The DR CRH receptor 2, has been associated with stress-induced TPH2 activity (Donner et al., 2016), suggesting a potential link with 5-HT deficiency. This might explain, why we observed an altered HPA axis activity in Tph2-deficient mice but not in their Tph2+/+ littermates. Moreover, 5-HT synapses have been identified on CRH-releasing cells in the PVN (Liposits et al., 1987) and 5-HT projections innervate relevant brain structures, such as the central amygdala (Asan et al., 2013) that are involved in regulating complex processes at the PVN (Herman and Cullinan, 1997; Van de Kar, 1997; Herman et al., 2005).
In the current study, in contrast to various other reports on early adversity, like MS, in rodents (Weaver et al., 2004; Weinstock, 2008; Wong et al., 2015), we were not able to find any effects of MS on HPA axis reactivity. One potential explanation for the lack of MS effects on the FCM profile might be that the MS paradigm, used in this study, was rather mild, even though similar paradigms reported strong effects. A moderating factor of MS in this regard might have been the maternal response to MS exposure (Own et al., 2013). The effect of maternal behavior and maternal stress level previously has been associated with the phenotype, observed in adult offspring (Meaney, 2001; de Souza et al., 2013; Murgatroyd et al., 2015). One mediating factor of maternal behavior is the genetic background. Tph2-deficient mice had previously been reported to exert altered maternal behavior (Angoa-Pérez and Kane, 2014) and altered stress reactivity (Gutknecht et al., 2015), both of which might have affected offspring development during MS. This is in line with the investigated behavioral parameters, which showed only subtle effects for MS, while most of the reported changes were induced by Tph2 deficiency.
With regard to territorial, social behavior, Tph2-deficient animals had been reported to be significantly more aggressive in comparison to wildtype mice (Angoa-Pérez et al., 2012; Mosienko et al., 2012; Gutknecht et al., 2015). Although observable on a nominal level, none of the investigated aggression parameters showed a statistically significant increase in Tph2-/- males in the current study. Furthermore, there was no effect of MS, supporting the previously discussed attenuating effect of maternal behavior also with regard to the spectrum of social behaviors. Interestingly though, the absolute time, Tph2-deficient males spent sniffing the intruder was significantly decreased during the first encounter, suggesting altered prosocial behavior in mice with lifelong 5-HT deficiency. This finds support in the fact that several prosocial parameters measured in this study approach significance with an FDR-corrected p < 0.10. The observed anti-social effect was particularly strong in Tph2-/- males. Comparable results were reported earlier (Kane et al., 2012; Waider et al., 2017), where Tph2-/- mice displayed e.g., decreased social interaction as well as less preference for socially relevant odors such as urine and, while showing a comparable habituation, failed dishabituation after the introduction of a novel social target. Testing general olfactory capacities in those animals did not reveal any defects in TPH2-deficient mice (Kane et al., 2012). Of note, social contact was found to activate raphe neurons, in rats, supporting the idea of 5-HT involvement in normal social behaviors (Haller et al., 2005b). A lack of 5-HT response to the initial activation of raphe neurons, thus, might impair the animals’ appraisal and, consequently, affect the behavioral reaction to the intruder. In line with this, we observed deviations from normal behavioral patterns regarding the aggressive interaction in 5-HT-deficient mice. We found that more Tph2-/- mice attacked the belly of intruders during the fourth session of the RIT. The belly is a vulnerable body part and attacks toward it are usually avoided in offensive, aggressive encounters (Litvin et al., 2007). Thus, Tph2-/- mice seem to be less restricted by the social convention of attack placements and display rule-breaking behavior. Interestingly, adrenalectomy induced a similar increase in attacks aimed at vulnerable targets in rats (Haller et al., 2001) and abolished the positive relationship between attack bites at non-vulnerable regions and 5-HT neuron activity in DR (Haller et al., 2005b). This is emphasizing the close interaction between 5-HT transmission and stress response.
Taken together, results of the behavioral screening suggest two major conclusions. Firstly, Tph2-/- mice might perceive a stressful stimulus differently when compared to their Tph2+/+ counterparts. Under aversive conditions 5-HT deficiency altered the approach toward potentially aversive cues. This might be mediated via an altered regulation of the stress response. An alternate explanation for the abnormal behavior in the EPM might be a dysregulation of 5-HT-dependent signaling involved in the regulation of anxiety- and panic-related behaviors (Paul et al., 2014). As described in the Deakin and Graeff hypothesis 5-HT is necessary to suppress the active motor reaction emerging from the PAG. Thus, lack of 5-HT, might be driving behavior toward a more activity-oriented coping strategy (Paul et al., 2014; Auth et al., 2018). However, in the current model it is not possible to determine if the observed behavioral consequences arise from an acute deficit in 5-HT, as would be suggested by the Deakin and Graeff hypothesis or if compensatory, developmental mechanisms are taking effect. Furthermore, based on our observation that exaggerated behavior was mostly observed in the context of aversive stimuli, a combination of altered appraisal and behavioral disinhibition might explain the observed behavioral phenotype. Notably, Tph2+/- mice displayed an inconclusive behavioral profile. This is most likely due to compensatory mechanisms involving decreased degradation of 5-HT (Márquez et al., 2013; Mosienko et al., 2014). Tph2+/- mice had been observed to show reductions of only 10–20% in 5-HT levels (Mosienko et al., 2012). This disproportional reduction in brain 5-HT is suggested to be a consequence of compensatory processes, involving e.g., decreased activity of MAO-A (Mosienko et al., 2014). Moreover, the behavioral distinction between Tph2+/- and Tph2-/- mice, or, dependent on the interrogated task, Tph2+/+ mice might be dependent on the involved brain circuitries, of which each might affect unique features. Therefore, Tph2+/- offspring might show phenotypes, related to Tph2-/- offspring or Tph2+/+ offspring, dependent on the task and experienced aversiveness.
Secondly, MS exerted only indirect effects by reinforcing Tph2 genotype-dependent, behavioral effects. Based on previous work, we did expect an effect of MS on anxiety- and aggression-related behaviors (Veenema et al., 2006, 2007). One potential explanation for the lack of consistent MS effects might be based on the involvement of several modulating factors. As discussed earlier, a moderating factor of MS in this regard might have been the maternal response to MS exposure (Own et al., 2013). The molecular basis of this phenomenon of less effect of adverse factors has been investigated in manifold experiments and a number of behavioral, physiological and molecular factors were identified as relevant mediators of individual susceptibility to stress (Jakob et al., 2014; Anacker et al., 2016; Han and Nestler, 2017). As one important factor of individual stress susceptibility epigenetic modifications such as DNA methylation have been identified (Dudley et al., 2011). As set out in the introduction epigenetic modifications are affected by environmental factors and the animal’s genetic make-up e.g., with regard to functional 5-HT system components.
To investigate the molecular underpinnings of Tph2 function-dependent effects of MS, whole genome RNA expression and DNA methylation profiling was performed in tissue homogenate of amygdala, which comprises a multilayered assembly of subregions and neuronal types that are involved in the regulation of complex behaviors in a highly specific manner (Asan et al., 2013). The amygdala is extensively innervated by serotonergic terminals (Asan et al., 2013) and has been reported as one of the structural entities regulating coping-related behavioral reaction to environmental challenges (Hale et al., 2008a,b, 2010; Tovote et al., 2016). Recently, altered neural activity in the basolateral amygdala of male Tph2-/- mice compared to their Tph2+/- littermates under home cage conditions was reported (Waider et al., 2017), while Tph2+/- and Tph2+/+ mice did not differ in this respect. Electrophysiological investigation of the excitability of amygdala neurons revealed altered amygdala reactivity in Tph2-/- and Tph2+/- males. Priming the amygdala using the stress-related neuropeptide urocortin 1 resulted not only in increased social anxiety, but was also linked to Tph2 expression changes (Donner et al., 2012). Overall, and given the role amygdala activity plays in stress response regulation (Herman et al., 2005), it represents a relevant hub of 5-HT signaling and early-life programming. However, characterization of other regions involved in anxiety and social behaviors is warranted.
Within the amygdala, we found that the number of DEGs for gene-by-environment interactions differed notably, dependent on the Tph2 genotype. DNA methylation changes were found to be relatively equal across comparisons. This discrepancy between methylation and expression changes suggests a Tph2-dependent effect on early-life programming by MS, partially independent of DNA methylation. Notably, only a fraction of the DEGs were found to be associated with DMLs, and of the overlapping genes only two showed a statistically significant correlation between expression and DNA methylation. This relatively low rate of DML-associated genes, overlapping with DEGs and the lack of significant correlation between expression and methylation of genes is in accordance with findings in other studies (Schraut et al., 2014) and most likely explained by the highly complex interaction of a multitude of epigenetic and structural factors, regulating gene expression (Jenuwein and Allis, 2001; Narlikar et al., 2002; Barski et al., 2007; Garske et al., 2010; Kratz et al., 2010; Fuchs et al., 2011). Furthermore, it has to be considered, that the method of MBD capture is not specific for cytosine methylation, but to a certain extent will also capture cytosine hydroxymethylation (Du et al., 2015). However, the binding capacity for this cytosine modification is several magnitudes less compared to their affinity for methylated cytosines at CpG sites. Lastly, it has to be mentioned that the investigated tissue has been taken after the animals experienced a variety of behavioral tests. Therefore, it might be possible, that several effects of Tph2∗MS interaction have been compensated or reinforced by further life experiences associated with behavioral testing, which has previously been shown (van den Hove et al., 2014). Nevertheless, designed to model aversive environment in humans, the inclusion of further life experiences is naturalistic.
Despite these limitations, we uncovered an interesting candidate-gene, Cck, to be involved in mediating the effects of early adversity dependent on 5-HT. As one of two genes showing an associated expression and methylation for the interaction of the Tph2+/- genotype and MS, Cck was furthermore, extensively associated with observed behaviors. For example, lower expression of Cck was related to more relative time displaying threat in the first session of the RIT. Furthermore, lower expression and higher methylation of Cck were associated with lower levels of anxiety. In particular with regard to this association, we observed an interaction across the levels of DNA methylation, RNA expression, and behavior. More specifically, Cck methylation was found to be decreased and its expression increased by MS in Tph2+/+ offspring, while in Tph2+/- offspring MS had an opposing effect, leading to an increase in Cck methylation and a decrease in expression. In Tph2-/- offspring, MS had no effect on either Cck methylation, or expression and showed constantly low expression and high methylation levels. This might indicate gene-by-environment-dependent epigenetic programming and altered susceptibility to MS in Tph2-/- offspring.
CCK is a peptide that was originally identified in the gut. However, it appears in greater amounts in the brain than in the periphery and is one of the most abundant neuropeptides (Innis et al., 1979; Crawley, 1985; Moran and Schwartz, 1994). Effects of CCK are mediated by CCK1 and CCK2 receptors (Moran et al., 1986). The CCK2 receptor is mainly found in the brain and shown to be involved in emotion regulation (Hughes et al., 1990; Chen et al., 2006). A study in rats, investigating CCK system functioning following MS, showed an increase in sensitivity toward CCK-4 injections in animals that were subjected to a separation paradigm (Greisen et al., 2005), supporting the idea of MS-induced epigenetic regulation. Of note, adrenalectomized rats, known to display increased abnormal aggressive behavior, showed a lower expression of CCK and decreased activation of CCK-expressing neurons in the prefrontal cortex (Haller et al., 2005a). Decreased CCK-positive neuron activation was furthermore related to the increased incident of vulnerable attacks, in line with the observed behavior and low Cck expression profile in Tph2-/- mice. In general, CCK has been shown to interact with the 5-HT system. In rats, administration of the selective 5-HT1a receptor antagonists (+)WAY100135 and WAY100635 resulted in an attenuation of aversion-related behaviors that were induced by CCK-8 injection (Bickerdike et al., 1995) while CCKB receptor antagonist reversed reduced exploratory activity induced by selective serotonin reuptake inhibitor (Kõks et al., 1999). Direct 5-HT administration was shown to enhance CCK release via activation of 5-HT3 receptors (Raiteri et al., 1993). Taken together these results suggest a positive association between 5-HT and CCK, which is in line with the observations in the current study, where mice, completely depleted of 5-HT showed low levels of Cck expression independent of MS. In limbic regions, such as the hypothalamus and amygdala, converged effects of 5-HT and CCK occur at a subset of neurons, allowing for direct modulatory interaction of these signaling molecules (Zippel et al., 1999; Asan et al., 2013). Moreover, CCK is particularly associated to anxiety- and panic-related behaviors across species (Harro et al., 1993). This has been further discussed in light of the 5-HT hypothesis of defense (Graeff et al., 2015), where the authors conclude that CCK neurotransmission is positively associated with escape-related behaviors, such as the escape response in the elevated T-maze, while 5-HT neurotransmission on the contrary is negatively associated with these behaviors. This is in contrast to the positive relationship observed between CCK and 5-HT, discussed earlier and also observed in the current study. Potential explanations for this discrepancy are brain region specificity, most likely dependent on the available 5-HT receptors and further modulatory factors. A clear interaction between CCK and 5-HT seems to be likely, whereby 5-HT might serve as modulatory interface between environment and CCK expression and consequently effect by affecting epigenetic regulation. All in all, the involvement of Cck in the observed 5-HT-dependent behavioral aberrations seems to be likely and altered Cck regulation by MS might represent a potential, 5-HT-dependent mechanism of early-life adversity.
Next to Cck, the RIKEN cDNA A830073O21 was found to be modulated by the interaction of 5-HT depletion and MS in a similar pattern as observed for Cck expression and methylation. It is an unclassified gene located on chromosome 7 that was suggested to be a mitochondrial protein in a combined mass spectrometry, GFP tagging, and machine learning approach resulting in 1058 gene assembly associated with mitochondria called MitoCarta (Pagliarini et al., 2008), but no longer appeared in the MitoCarta 2.0 (Calvo et al., 2016). Further work toward elucidating its function is thus warranted. Its comparably high expression (amongst the top 10%) in the amygdala and the equally high susceptibility to gene-by-environment-dependent effects suggest a role in 5-HT-dependent response to environmental cues. This finds further support by a predicted interaction with in total 164 microRNAs2.
Taken together, our results highlight epigenetic Cck regulation in the amygdala by early-life adversity as potential mechanism involved in anxiety-like and aberrant social behaviors of a lifelong deficiency in 5-HT synthesis.
Data Availability
The datasets GSE110330 generated for this study can be found in NCBI GEO https://www.ncbi.nlm.nih.gov/geo/query/acc.cgi?acc=GSE110330. Other relevant data is provided as Supplementary Material.
Ethics Statement
This study was carried out in accordance with the recommendations of the European Parliament and Council Directive (2010/63/EU). The protocol was approved by local authorities (Government of Lower Franconia, Würzburg: 55.2-2531.01- 57/12).
Author Contributions
K-PL, DvdH, and MW conceived and designed the study. MW, FM, LDG, and RP acquired the data. RL, LE, and KF performed bioinformatics processing and analysis of sequencing data. MW wrote the first draft of the manuscript. MW, DvdH, K-PL, JW, JG, RL, LE, FM, AS-B, SP, TS, RP, and HS contributed to the interpretation of the data, preparation of the figures, writing and/or revising of the manuscript.
Funding
This work was funded by the Deutsche Forschungsgemeinschaft (DFG CRC TRR 58/A1 and A5 to K-PL, DvdH, and AS-B, and WA 3446/2-1 to JW), the European Union’s Seventh Framework Programme under grant no. 602805 (Aggressotype) to K-PL, TS, JG, and DvdH, EC: MATRICS FP7/No. 603016 to JG, the Horizon 2020 Research and Innovation Programme under grant no. 728018 (Eat2beNICE) to K-PL and JG, the Innovative Medicines Initiative 2 Joint Undertaking No. 115916 (PRISM) to JG, the 5–100 Russian Academic Excellence Project to K-PL and TS. MW was supported by a grant of the German Excellence Initiative to the Graduate School of Life Sciences (GSLS), University of Würzburg. RL was supported by a fellowship as part of the Netherlands Organization for Scientific Research (NWO) grant 022.005.019. This publication was funded by the German Research Foundation (DFG) and the University of Würzburg in the funding programme Open Access Publishing. The funders had no role in study design, data collection and analysis, decision to publish or preparation of the manuscript.
Conflict of Interest Statement
The authors declare that the research was conducted in the absence of any commercial or financial relationships that could be construed as a potential conflict of interest.
Acknowledgments
Special thanks go to G. Ortega and B. Machiels for excellent technical assistance. This manuscript is based on parts of the dissertation of M. T. Weidner.
Supplementary Material
The Supplementary Material for this article can be found online at: https://www.frontiersin.org/articles/10.3389/fnins.2019.00460/full#supplementary-material
Footnotes
- ^ https://www.sdmproject.com/utilities/?show=FDR
- ^ http://www.informatics.jax.org/interaction/explorer?markerIDs=MGI:2443692, as consulted online on 11.04.2019
References
Alenina, N., Kikic, D., Todiras, M., Mosienko, V., Qadri, F., Plehm, R., et al. (2009). Growth retardation and altered autonomic control in mice lacking brain serotonin. Proc. Natl. Acad. Sci. U.S.A. 106, 10332–10337. doi: 10.1073/pnas.0810793106
Anacker, C., Scholz, J., O’Donnell, K. J., Allemang-Grand, R., Diorio, J., Bagot, R. C., et al. (2016). Neuroanatomic differences associated with stress susceptibility and resilience. Biol. Psychiatry 79, 840–849. doi: 10.1016/j.biopsych.2015.08.009
Angoa-Pérez, M., and Kane, M. (2014). Brain serotonin determines maternal behavior and offspring survival. Genes Brain Behav. 13, 1–37. doi: 10.1111/gbb.12159/full
Angoa-Pérez, M., Kane, M. J., Briggs, D. I., Sykes, C. E., Shah, M. M., Francescutti, D. M., et al. (2012). Genetic depletion of brain 5HT reveals a common molecular pathway mediating compulsivity and impulsivity. J. Neurochem. 121, 974–984. doi: 10.1111/j.1471-4159.2012.07739.x
Arabo, A., Potier, C., and Roy, V. (2010). “Measuring behavior 2010,” in Proceedings of the 7th International Conference on Methods and Techniques in Behavioral Research, eds A. J. Spink, F. Grieco, O. E. Krips, L. W. S. Loijens, L. P. J. J. Noldus, and P. H. Zimmerman (Eindhoven: Noldus).
Asan, E., Steinke, M., and Lesch, K. P. (2013). Serotonergic innervation of the amygdala: targets, receptors, and implications for stress and anxiety. Histochem. Cell Biol. 139, 785–813. doi: 10.1007/s00418-013-1081-1
Auth, C. S., Weidner, M. T., Popp, S., Strekalova, T., Schmitt-Böhrer, A. G., van den Hove, D. L. A., et al. (2018). Differential anxiety-related behaviours and brain activation in Tph2-deficient female mice exposed to adverse early environment. Eur. Neuropsychopharmacol. 28, 1270–1283. doi: 10.1016/j.euroneuro.2018.07.103
Bach, H., Arango, V., Huang, Y. Y., Leong, S., John Mann, J., and Underwood, M. D. (2011). Neuronal tryptophan hydroxylase expression in BALB/cJ and C57Bl/6J mice. J. Neurochem. 118, 1067–1074. doi: 10.1111/j.1471-4159.2011.07379.x
Barski, A., Cuddapah, S., Cui, K., Roh, T. Y., Schones, D. E., Wang, Z., et al. (2007). High-resolution profiling of histone methylations in the human genome. Cell 129, 823–837. doi: 10.1016/j.cell.2007.05.009
Bartolomucci, A., Palanza, P., Sacerdote, P., Ceresini, G., Chirieleison, A., Panerai, A. E., et al. (2003). Individual housing induces altered immuno-endocrine responses to psychological stress in male mice. Psychoneuroendocrinology 28, 540–558. doi: 10.1016/s0306-4530(02)00039-2
Bennett, A. J., Lesch, K. P., Heils, A., Long, J. C., Lorenz, J. G., Shoaf, S. E., et al. (2002). Early experience and serotonin transporter gene variation interact to influence primate CNS function. Mol. Psychiatry 7, 118–122. doi: 10.1038/sj.mp.4000949
Bickerdike, M. J., Fletcher, A., and Marsden, C. A. (1995). Attenuation of CCK-induced aversion in rats on the elevated x-Maze by the selective 5-HT1A receptor antagonists. (+)WAY100135 and WAY100635. Neuropharmacology 34, 805–811. doi: 10.1016/0028-3908(95)00037-7
Blanchard, R. J., Wall, P. M., and Blanchard, D. C. (2003). Problems in the study of rodent aggression. Horm. Behav. 44, 161–170. doi: 10.1016/s0018-506x(03)00127-2
Bledsoe, A. C., Oliver, K. M., Scholl, J. L., and Forster, G. L. (2011). Anxiety states induced by post-weaning social isolation are mediated by CRF receptors in the dorsal raphe nucleus. Brain Res. Bull. 85, 117–122. doi: 10.1016/j.brainresbull.2011.03.003
Boulle, F., Pawluski, J. L., Homberg, J. R., Machiels, B., Kroeze, Y., Kumar, N., et al. (2016). Developmental fluoxetine exposure increases behavioral despair and alters epigenetic regulation of the hippocampal BDNF gene in adult female offspring. Horm. Behav. 80, 47–57. doi: 10.1016/j.yhbeh.2016.01.017
Calvo, S. E., Clauser, K. R., and Mootha, V. K. (2016). MitoCarta2.0: an updated inventory of mammalian mitochondrial proteins. Nucleic Acids Res. 44, D1251–D1257. doi: 10.1093/nar/gkv1003
Caramaschi, D., de Boer, S. F., de Vries, H., and Koolhaas, J. M. (2008). Development of violence in mice through repeated victory along with changes in prefrontal cortex neurochemistry. Behav. Brain Res. 189, 263–272. doi: 10.1016/j.bbr.2008.01.003
Casarrubea, M., Roy, V., Sorbera, F., Magnusson, M. S., Santangelo, A., Arabo, A., et al. (2013). Temporal structure of the rat’s behavior in elevated plus maze test. Behav. Brain Res. 237, 290–299. doi: 10.1016/j.bbr.2012.09.049
Caspi, A., McClay, J., Moffitt, T. E., Mill, J., Martin, J., Craig, I. W., et al. (2002). Role of genotype in the cycle of violence in maltreated children. Science 297, 851–854. doi: 10.1126/science.1072290
Caspi, A., Sugden, K., Moffitt, T. E., Taylor, A., Craig, I. W., Harrington, H., et al. (2003). Influence of life stress on depression: moderation by a polymorphism in the 5-HTT gene. Science 301, 386–389. doi: 10.1126/science.1083968
Champoux, M., Bennett, A., Shannon, C., Higley, J. D., Lesch, K. P., and Suomi, S. J. (2002). Serotonin transporter gene polymorphism, differential early rearing, and behavior in rhesus monkey neonates. Mol. Psychiatry 7, 1058–1063. doi: 10.1038/sj.mp.4001157
Chen, Q., Nakajima, A., Meacham, C., and Tang, Y.-P. (2006). Elevated cholecystokininergic tone constitutes an important molecular/neuronal mechanism for the expression of anxiety in the mouse. Proc. Natl. Acad. Sci. U.S.A. 103, 3881–3886. doi: 10.1073/pnas.0505407103
Crawley, J., and Goodwin, F. K. (1980). Preliminary report of a simple animal behavior model for the anxiolytic effects of benzodiazepines. Pharmacol. Biochem. Behav. 13, 167–170. doi: 10.1016/0091-3057(80)90067-2
Crawley, J. N. (1985). Cormparative distribution of cholecystokinin and other neuropeptides. Ann. N. Y. Acad. Sci. 448, 1–8. doi: 10.1111/j.1749-6632.1985.tb29900.x
de Almeida, R. M. M., Ferrari, P. F., Parmigiani, S., and Miczek, K. (2005). Escalated aggressive behavior: dopamine, serotonin and GABA. Eur. J. Pharmacol. 526, 51–64. doi: 10.1016/j.ejphar.2005.10.004
de Almeida, R. M. M., and Miczek, K. A. (2002). Aggression escalated by social instigation or by discontinuation of reinforcement (“frustration”) in mice: inhibition by anpirtoline: a 5-HT1B receptor agonist. Neuropsychopharmacology 27, 171–181. doi: 10.1016/s0893-133x(02)00291-9
de Souza, M. A., Centenaro, L. A., Menegotto, P. R., Henriques, T. P., Bonini, J., Achaval, M., et al. (2013). Prenatal stress produces social behavior deficits and alters the number of oxytocin and vasopressin neurons in adult rats. Neurochem. Res. 38, 1479–1489. doi: 10.1007/s11064-013-1049-5
Donner, N. C., Johnson, P. L., Fitz, S. D., Kellen, K. E., Shekhar, A., and Lowry, C. A. (2012). Elevated tph2 mRNA expression in a rat model of chronic anxiety. Depress. Anxiety 29, 307–319. doi: 10.1002/da.21925
Donner, N. C., Siebler, P. H., Johnson, D. T., Villarreal, M. D., Mani, S., Matti, A. J., et al. (2016). Serotonergic systems in the balance: CRHR1 and CRHR2 differentially control stress-induced serotonin synthesis. Psychoneuroendocrinology 63, 178–190. doi: 10.1016/j.psyneuen.2015.09.024
Du, Q., Luu, P. L., Stirzaker, C., and Clark, S. J. (2015). Methyl-CpG-binding domain proteins: readers of the epigenome. Epigenomics 7, 1051–1073. doi: 10.2217/epi.15.39
Dudley, K. J., Li, X., Kobor, M. S., Kippin, T. E., and Bredy, T. W. (2011). Epigenetic mechanisms mediating vulnerability and resilience to psychiatric disorders. Neurosci. Biobehav. Rev. 35, 1544–1551. doi: 10.1016/j.neubiorev.2010.12.016
Fuchs, S. M., Krajewski, K., Baker, R. W., Miller, V. L., and Strahl, B. D. (2011). Influence of combinatorial histone modifications on antibody and effector protein recognition. Curr. Biol. 21, 53–58. doi: 10.1016/j.cub.2010.11.058
García-Cáceres, C., Lagunas, N., Calmarza-Font, I., Azcoitia, I., Diz-Chaves, Y., García-Segura, L. M., et al. (2010). Gender differences in the long-term effects of chronic prenatal stress on the HPA axis and hypothalamic structure in rats. Psychoneuroendocrinology 35, 1525–1535. doi: 10.1016/j.psyneuen.2010.05.006
Gardner, K. L., Hale, M. W., Oldfield, S., Lightman, S. L., Plotskyc, P. M., and Lowry, C. A. (2009). Adverse experience during early life and adulthood interact to elevate tph2 mRNA expression in serotonergic neurons within the dorsal raphe nucleus. Neuroscience 163, 991–1001. doi: 10.1016/j.neuroscience.2009.07.055
Garske, A. L., Oliver, S. S., Wagner, E. K., Musselman, C. A., LeRoy, G., Garcia, B. A., et al. (2010). Combinatorial profiling of chromatin binding modules reveals multisite discrimination. Nat. Chem. Biol. 6, 283–290. doi: 10.1038/nchembio.319
Graeff, F., Sant’Ana, A., Vilela-Costa, H., and Zangrossi, H. Jr. (2015). New findings on the neurotransmitter modulation of defense in the dorsal periaqueductal gray. CNS Neurol. Disord. Drug Targets 14, 988–995. doi: 10.2174/1871527314666150909114558
Greisen, M. H., Bolwig, T. G., and Wörtwein, G. (2005). Cholecystokinin tetrapeptide effects on HPA axis function and elevated plus maze behaviour in maternally separated and handled rats. Behav. Brain Res. 161, 204–212. doi: 10.1016/j.bbr.2005.02.006
Gutknecht, L., Araragi, N., Merker, S., Waider, J., Sommerlandt, F. M. J., Mlinar, B., et al. (2012). Impacts of brain serotonin deficiency following Tph2 inactivation on development and raphe neuron serotonergic specification. PLoS One 7:e43157. doi: 10.1371/journal.pone.0043157
Gutknecht, L., Popp, S., Waider, J., Sommerlandt, F. M. J., Göppner, C., Post, A., et al. (2015). Interaction of brain 5-HT synthesis deficiency, chronic stress and sex differentially impact emotional behavior in Tph2 knockout mice. Psychopharmacology 232, 2429–2441. doi: 10.1007/s00213-015-3879-0
Gutknecht, L., Waider, J., Kraft, S., Kriegebaum, C., Holtmann, B., and Reif, A. (2008). Deficiency of brain 5-HT synthesis but serotonergic neuron formation in Tph2 knockout mice. J. Neural Transm. 115, 1127–1132. doi: 10.1007/s00702-008-0096-6
Hale, M. W., Hay-schmidt, A., Mikkelsen, J. D., Poulsen, B., Adriaan, J., Evans, A. K., et al. (2008a). Exposure to an open-field arena increases c-Fos expression in a subpopulation of neurons in the dorsal raphe nucleus, including neurons projecting to the basolateral amygdaloid complex. Neuroscience 157, 733–748. doi: 10.1016/j.neuroscience.2008.09.050
Hale, M. W., Hay-schmidt, A., Mikkelsen, J. D., Poulsen, B., and Lowry, C. A. (2008b). Exposure to an open-field arena increases c-Fos expression in a distributed anxiety-related system projecting to the basolateral amygdaloid complex. Neuoscience 155, 659–672. doi: 10.1016/j.neuroscience.2008.05.054
Hale, M. W., Johnson, P. L., Westerman, A. M., Abrams, J. K., Shekhar, A., and Lowry, C. A. (2010). Multiple anxiogenic drugs recruit a parvalbumin-containing subpopulation of GABAergic interneurons in the basolateral amygdala. Prog. Neuro-Psychopharmacol. Biol. Psychiatry 34, 1285–1293. doi: 10.1016/j.pnpbp.2010.07.012
Hall, F. S. (1998). Social deprivation of neonatal, adolescent, and adult rats has distinct neurochemical and behavioral consequences. Crit. Rev. Neurobiol. 12, 129–162. doi: 10.1615/critrevneurobiol.v12.i1-2.50
Haller, J., Mikics, E., Halász, J., and Tóth, M. (2005a). Mechanisms differentiating normal from abnormal aggression: glucocorticoids and serotonin. Eur. J. Pharmacol. 526, 89–100. doi: 10.1016/j.ejphar.2005.09.064
Haller, J., Tóth, M., and Halász, J. (2005b). The activation of raphe serotonergic neurons in normal and hypoarousal-driven aggression: a double labeling study in rats. Behav. Brain Res. 161, 88–94. doi: 10.1016/j.bbr.2005.01.006
Haller, J., Van De Schraaf, J., and Kruk, M. R. (2001). Deviant forms of aggression in glucocorticoid hyporeactive rats: a model for ‘pathological’ aggression? J. Neuroendocrinol. 13, 102–107. doi: 10.1111/j.1365-2826.2001.00600.x
Han, M.-H., and Nestler, E. J. (2017). Neural substrates of depression and resilience. Neurotherapeutics 14, 677–686. doi: 10.1007/s13311-017-0527-x
Harro, J., Vasar, E., and Bradwejn, J. (1993). CCK in animal and human research on anxiety. Trends Pharmacol. Sci. 14, 244–249. doi: 10.1016/0165-6147(93)90020-k
Hellstrom, I. C., Dhir, S. K., Diorio, J. C., and Meaney, M. J. (2012). Maternal licking regulates hippocampal glucocorticoid receptor transcription through a thyroid hormone-serotonin-NGFI-A signalling cascade. Philos. Trans. R. Soc. Lond. B Biol. Sci. 367, 2495–2510. doi: 10.1098/rstb.2012.0223
Herman, J. P., and Cullinan, W. E. (1997). Neurocircuitry of stress: central control of the hypothalamo-pituitary-adrenocortical axis. Trends Neurosci. 20, 78–84. doi: 10.1016/s0166-2236(96)10069-2
Herman, J. P., Ostrander, M. M., Mueller, N. K., and Figueiredo, H. (2005). Limbic system mechanisms of stress regulation: hypothalamo-pituitary- adrenocortical axis. Prog. Neuro-Psychopharmacol. Biol. Psychiatry 29, 1201–1213. doi: 10.1016/j.pnpbp.2005.08.006
Hiroi, R., Carbone, D. L., Zuloaga, D. G., Bimonte-Nelson, H. A., and Handa, R. J. (2016). Sex-dependent programming effects of prenatal glucocorticoid treatment on the developing serotonin system and stress-related behaviors in adulthood. Neuroscience 320, 43–56. doi: 10.1016/j.neuroscience.2016.01.055
Holmes, A., Li, Q., Murphy, D. L., Gold, E., and Crawley, J. N. (2003). Abnormal anxiety-related behaviour in serotonin transporter null mutant mice: the influence of genetic background. Genes Brain Behav. 2, 365–380. doi: 10.1046/j.1601-1848.2003.00050.x
Hughes, J., Boden, P., Costall, B., Domeney, A., Kelly, E., Horwell, D. C., et al. (1990). Development of a class of selective cholecystokinin type B receptor antagonists having potent anxiolytic activity. Proc. Natl. Acad. Sci. U.S.A. 87, 6728–6732. doi: 10.1073/pnas.87.17.6728
Innis, R. B., Corrêa, F. M., Uhl, G. R., Schneider, B., and Snyder, S. H. (1979). Cholecystokinin octapeptide-like immunoreactivity: histochemical localization in rat brain. Proc. Natl. Acad. Sci. U.S.A. 76, 521–525. doi: 10.1073/pnas.76.1.521
Jakob, S., Schraut, K.-G., Schmitt, A. G., Scholz, C.-J., Ortega, G., Steinbusch, H. W. M., et al. (2014). Differential effects of prenatal stress in female 5-Htt-deficient mice: towards molecular mechanisms of resilience. Dev. Neurosci. 36, 454–464. doi: 10.1159/000363695
Jenuwein, T., and Allis, C. D. (2001). Translating the histone code. Science 293, 1074–1080. doi: 10.1126/science.1063127
Kane, M. J., Angoa-Peréz, M., Briggs, D. I., Sykes, C. E., Francescutti, D. M., Rosenberg, D. R., et al. (2012). Mice genetically depleted of brain serotonin display social impairments, communication deficits and repetitive behaviors: possible relevance to autism. PLoS One 7:e48975. doi: 10.1371/journal.pone.0048975
Kelder, T., Van Iersel, M. P., Hanspers, K., Kutmon, M., Conklin, B. R., Evelo, C. T., et al. (2012). WikiPathways: building research communities on biological pathways. Nucleic Acids Res. 40, 1301–1307. doi: 10.1093/nar/gkr1074
Kõks, S., Bourin, M., Voikar, V., Soosaar, A., and Vasar, E. (1999). Role of CCK in anti-exploratory action of paroxetine, 5-HT reuptake inhibitor. Int. J. Neuropsychopharmacol. 2, 9–16. doi: 10.1017/s1461145799001297
Koolhaas, J. M., Coppens, C. M., de Boer, S. F., Buwalda, B., Meerlo, P., and Timmermans, P. J. A. (2013). The resident-intruder paradigm: a standardized test for aggression, violence and social stress. J. Vis. Exp. e4367. doi: 10.3791/4367
Kratz, A., Arner, E., Saito, R., Kubosaki, A., Kawai, J., Suzuki, H., et al. (2010). Core promoter structure and genomic context reflect histone 3 lysine 9 acetylation patterns. BMC Genom. 11:257. doi: 10.1186/1471-2164-11-257
Kulikov, A. V., Osipova, D. V., Naumenko, V. S., and Popova, N. K. (2005). Association between Tph2 gene polymorphism, brain tryptophan hydroxylase activity and aggressiveness in mouse strains. Genes Brain Behav. 4, 482–485. doi: 10.1111/j.1601-183x.2005.00145.x
Kutmon, M., Riutta, A., Nunes, N., Hanspers, K., Willighagen, E. L., Bohler, A., et al. (2016). WikiPathways: capturing the full diversity of pathway knowledge. Nucleic Acids Res. 44, D488–D494. doi: 10.1093/nar/gkv1024
Kutmon, M., van Iersel, M. P., Bohler, A., Kelder, T., Nunes, N., Pico, A. R., et al. (2015). PathVisio 3: an extendable pathway analysis toolbox. PLoS Comput. Biol. 11:e1004085. doi: 10.1371/journal.pcbi.1004085
Lesch, K.-P., Araragi, N., Waider, J., Van den Hove, D. L. A., and Gutknecht, L. (2012). Targeting brain serotonin synthesis: insights into neurodevelopmental disorders with long-term outcomes related to negative emotionality, aggression and antisocial behaviour. Philos. Trans. R. Soc. Lond. B Biol. Sci. 367, 2426–2443. doi: 10.1098/rstb.2012.0039
Liposits, Z., Phelix, C., and Paull, W. K. (1987). Synaptic interaction of serotonergic axons and corticotropin releasing factor (CRF) synthesizing neurons in the hypothalamic paraventricular nucleus of the rat - A light and electron microscopic immunocytochemical study. Histochemistry 86, 541–549. doi: 10.1007/bf00489545
Lister, R. G. (1987). The use of a plus-maze to measure anxiety in the mouse. Psychopharmacology 92, 180–185.
Lister, R. G. (1990). Ethologically-based animal models of anxiety disorders. Pharmacol. Ther. 46, 321–340. doi: 10.1016/0163-7258(90)90021-s
Litvin, Y., Blanchard, C. D., Pentkowski, N. S., and Blanchard, R. J. (2007). A pinch or a lesion: a reconceptualization of biting consequences in mice. Aggress. Behav. 33, 545–551. doi: 10.1002/ab.20222
Love, M. I., Huber, W., and Anders, S. (2014). Moderated estimation of fold change and dispersion for RNA-seq data with DESeq2. Genome Biol. 15:550.
Lupien, S. J., McEwen, B. S., Gunnar, M. R., and Heim, C. (2009). Effects of stress throughout the lifespan on the brain, behaviour and cognition. Nat. Rev. Neurosci. 10, 434–445. doi: 10.1038/nrn2639
Márquez, C., Poirier, G. L., Cordero, M. I., Larsen, M. H., Groner, A., Marquis, J., et al. (2013). Peripuberty stress leads to abnormal aggression, altered amygdala and orbitofrontal reactivity and increased prefrontal MAOA gene expression. Transl. Psychiatry 3:e216. doi: 10.1038/tp.2012.144
McCormick, C. M., Smythe, J. W., Sharma, S., and Meaney, M. J. (1995). Sex-specific effects of prenatal stress on hypothalamic-pituitary-adrenal responses to stress and brain glucocorticoid receptor density in adult rats. Dev. Brain Res. 84, 55–61. doi: 10.1016/0165-3806(94)00153-q
Meaney, M. J. (2001). Maternal care, gene expression, and the transmission of individual differences in stress reactivity across generations. Annu. Rev. Neurosci. 24, 1161–1192. doi: 10.1146/annurev.neuro.24.1.1161
Miczek, K. A., Maxson, S. C., Fish, E. W., and Faccidomo, S. (2001). Aggressive behavioral phenotypes in mice. Behav. Brain Res. 125, 167–181. doi: 10.1016/s0166-4328(01)00298-4
Migliarini, S., Pacini, G., Pelosi, B., Lunardi, G., and Pasqualetti, M. (2013). Lack of brain serotonin affects postnatal development and serotonergic neuronal circuitry formation. Mol. Psychiatry 18, 1106–1118. doi: 10.1038/mp.2012.128
Moran, T. H., Robinson, P. H., Goldrich, M. S., and McHugh, P. R. (1986). Two brain cholecystokinin receptors:implications for behavioral actions. Brain Res. 362, 175–179. doi: 10.1016/0006-8993(86)91413-7
Moran, T. H., and Schwartz, G. J. (1994). Neurobiology of cholecystokinin. Crit. Rev. Neurobiol. 9, 1–28.
Mosienko, V., Beis, D., Pasqualetti, M., Waider, J., Matthes, S., Qadri, F., et al. (2015). Life without brain serotonin: reevaluation of serotonin function with mice deficient in brain serotonin synthesis. Behav. Brain Res. 277, 78–88. doi: 10.1016/j.bbr.2014.06.005
Mosienko, V., Bert, B., Beis, D., Matthes, S., Fink, H., Bader, M., et al. (2012). Exaggerated aggression and decreased anxiety in mice deficient in brain serotonin. Transl. Psychiatry 2:e122. doi: 10.1038/tp.2012.44
Mosienko, V., Matthes, S., Hirth, N., Beis, D., Flinders, M., Bader, M., et al. (2014). Adaptive changes in serotonin metabolism preserve normal behavior in mice with reduced TPH2 activity. Neuropharmacology 85, 73–80. doi: 10.1016/j.neuropharm.2014.05.015
Murgatroyd, C. A., Peña, C. J., Podda, G., Nestler, E. J., and Nephew, B. C. (2015). Early life social stress induced changes in depression and anxiety associated neural pathways which are correlated with impaired maternal care. Neuropeptides 52, 103–111. doi: 10.1016/j.npep.2015.05.002
Narlikar, G. J., Fan, H. Y., and Kingston, R. E. (2002). Cooperation between complexes that regulate chromatin structure and transcription. Cell 108, 475–487. doi: 10.1016/s0092-8674(02)00654-2
Natarajan, D., and Caramaschi, D. (2010). Animal violence demystified. Front. Behav. Neurosci. 4:9. doi: 10.3389/fnbeh.2010.00009
Onaivi, E. S., and Martin, B. R. (1989). Neuropharmacological and physiological validation of a computer-controlled two-compartment black and white box for the assessment of anxiety. Prog. Neuropsychopharmacol. Biol. Psychiatry 13, 963–976.
Ottenhof, K. W., Sild, M., Lévesque, M. L., Ruhé, H. G., and Booij, L. (2018). TPH2 polymorphisms across the spectrum of psychiatric morbidity: a systematic review and meta-analysis. Neurosci. Biobehav. Rev. 92, 29–42. doi: 10.1016/j.neubiorev.2018.05.018
Own, L. S., Iqbal, R., and Patel, P. D. (2013). Maternal separation alters serotonergic and HPA axis gene expression independent of separation duration in mice. Brain Res. 1515, 29–38. doi: 10.1016/j.brainres.2013.03.032
Pagliarini, D. J., Calvo, S. E., Chang, B., Sheth, S. A., Vafai, S. B., Ong, S. E., et al. (2008). A mitochondrial protein compendium elucidates complex I disease biology. Cell 134, 112–123. doi: 10.1016/j.cell.2008.06.016
Paul, E. D., Johnson, P. L., Shekhar, A., and Lowry, C. A. (2014). The Deakin/Graeff hypothesis: focus on serotonergic inhibition of panic. Neurosci. Biobehav. Rev. 46, 379–396. doi: 10.1016/j.neubiorev.2014.03.010
Paul, E. D., and Lowry, C. A. (2013). Functional topography of serotonergic systems supports the Deakin/Graeff hypothesis of anxiety and affective disorders. J. Psychopharmacol. 27, 1090–1106. doi: 10.1177/0269881113490328
Post, A. M., Weyers, P., Holzer, P., Painsipp, E., Pauli, P., Wultsch, T., et al. (2011). Gene-environment interaction influences anxiety-like behavior in ethologically based mouse models. Behav. Brain Res. 218, 99–105. doi: 10.1016/j.bbr.2010.11.031
Raiteri, M., Paudice, P., and Vallebuona, F. (1993). Release of cholecystokinin in the central nervous system. Neurochem. Int. 22, 519–527. doi: 10.1016/0197-0186(93)90025-z
Sachs, B. D., Rodriguiz, R. M., Tran, H. L., Iyer, A., Wetsel, W. C., and Caron, M. G. (2015). Serotonin deficiency alters susceptibility to the long-term consequences of adverse early life experience. Psychoneuroendocrinology 53, 69–81. doi: 10.1016/j.psyneuen.2014.12.019
Schraut, K. G., Jakob, S. B., Weidner, M. T., Schmitt, A. G., Scholz, C. J., Strekalova, T., et al. (2014). Prenatal stress-induced programming of genome-wide promoter DNA methylation in 5-HTT-deficient mice. Transl. Psychiatry 4:e473. doi: 10.1038/tp.2014.107
Stark, R., and Brown, G. (2013). DiffBind?: differential binding analysis of ChIP-Seq peak data DiffBind works primarily with peaksets, which are sets of genomic intervals representing candidate. University of Cambridge: Cambridge. 1–40
Takahashi, A., and Miczek, K. A. (2014). Neurogenetics of aggressive behavior – studies in rodents. Curr. Top. Behav. Neurosci. 17, 289–320.
Touma, C., Palme, R., and Sachser, N. (2004). Analyzing corticosterone metabolites in fecal samples of mice: a noninvasive technique to monitor stress hormones. Horm. Behav. 45, 10–22. doi: 10.1016/j.yhbeh.2003.07.002
Touma, C., Sachser, N., Möstl, E., and Palme, R. (2003). Effects of sex and time of day on metabolism and excretion of corticosterone in urine and feces of mice. Gen. Comp. Endocrinol. 130, 267–278. doi: 10.1016/s0016-6480(02)00620-2
Tovote, P., Esposito, M. S., Botta, P., Chaudun, F., Fadok, J. P., Markovic, M., et al. (2016). Midbrain circuits for defensive behaviour. Nature 534, 206–212. doi: 10.1038/nature17996
Trullas, R., and Skolnick, P. (1993). Differences in fear motivated behaviors among inbred mouse strains. Psychopharmacology 111, 323–331. doi: 10.1007/bf02244948
Van de Kar, L. D. (1997). “5-HT receptors involved in the regulation of hormone secretion,” in Handbook of Experimental Pharmacology, eds H. G. Baumgarten and M. Goethert (Berlin: Springer), 537–562. doi: 10.1007/978-3-642-60921-3_20
van den Hove, D. L. A., Leibold, N. K., Strackx, E., Martinez-Claros, M., Lesch, K.-P., Steinbusch, H. W. M., et al. (2014). Prenatal stress and subsequent exposure to chronic mild stress in rats; interdependent effects on emotional behavior and the serotonergic system. Eur. Neuropsychopharmacol. 24, 595–607. doi: 10.1016/j.euroneuro.2013.09.006
van den Hove, D. L. A., Van den Hove, D., Jakob, S. B., Schraut, K.-G., Kenis, G., Schmitt, A. G., et al. (2011). Differential effects of prenatal stress in 5-Htt deficient mice: towards molecular mechanisms of gene × environment interactions. PLoS One 6:e22715. doi: 10.1371/journal.pone.0022715
van Iersel, M. P., Kelder, T., Pico, A. R., Hanspers, K., Coort, S., Conklin, B. R., et al. (2008). Presenting and exploring biological pathways with PathVisio. BMC Bioinform. 9:399. doi: 10.1186/1471-2105-9-399
Veenema, A. H., Blume, A., Niederle, D., Buwalda, B., and Neumann, I. D. (2006). Effects of early life stress on adult male aggression and hypothalamic vasopressin and serotonin. Eur. J. Neurosci. 24, 1711–1720. doi: 10.1111/j.1460-9568.2006.05045.x
Veenema, A. H., Bredewold, R., and Neumann, I. D. (2007). Opposite effects of maternal separation on intermale and maternal aggression in C57BL/6 mice: link to hypothalamic vasopressin and oxytocin immunoreactivity. Psychoneuroendocrinology 32, 437–450. doi: 10.1016/j.psyneuen.2007.02.008
Waider, J., Popp, S., Lange, M. D., Kern, R., Kolter, J. F., Kobler, J., et al. (2017). Genetically driven brain serotonin deficiency facilitates panic-like escape behavior in mice. Transl. Psychiatry 7:e1246. doi: 10.1038/tp.2017.209
Wang, F., Kessels, H. W., and Hu, H. (2014). The mouse that roared: neural mechanisms of social hierarchy. Trends Neurosci. 37, 674–682. doi: 10.1016/j.tins.2014.07.005
Weaver, I. C. G., Cervoni, N., Champagne, F. A., D’Alessio, A. C., Sharma, S., Seckl, J. R., et al. (2004). Epigenetic programming by maternal behavior. Nat. Neurosci. 7, 847–854.
Weidner, M. T. (2018). Brain Serotonin Throughout Development-for Better and for Worse. Dissertation, Maastricht University/Julius-Maximilians-Universität Würzburg, Maastricht.
Weinstock, M. (2008). The long-term behavioural consequences of prenatal stress. Neurosci. Biobehav. Rev. 32, 1073–1086. doi: 10.1016/j.neubiorev.2008.03.002
Winslow, J. T., and Miczek, K. A. (1984). Habituation of aggressive behavior in mice: a parametric study. Aggress. Behav. 10, 103–113. doi: 10.1186/1744-9081-7-3
Wong, P., Sze, Y., Gray, L. J., Chang, C. C. R., Cai, S., and Zhang, X. (2015). Early life environmental and pharmacological stressors result in persistent dysregulations of the serotonergic system. Front. Behav. Neurosci. 9:94. doi: 10.3389/fnbeh.2015.00094
Zhang, T. Y., Labonté, B., Wen, X. L., Turecki, G., and Meaney, M. J. (2013). Epigenetic mechanisms for the early environmental regulation of hippocampal glucocorticoid receptor gene expression in rodents and humans. Neuropsychopharmacology 38, 111–123. doi: 10.1038/npp.2012.149
Zhang, X., Beaulieu, J.-M. M., Sotnikova, T. D., Gainetdinov, R. R., and Caron, M. G. (2004). Tryptophan hydroxylase-2 controls brain serotonin synthesis. Science 305:217. doi: 10.1126/science.1097540
Zhang, Y., Liu, T., Meyer, C. A., Eeckhoute, J., Johnson, D. S., Bernstein, B. E., et al. (2008). Model-based analysis of ChIP-Seq. (MACS). Genome Biol. 9:R137. doi: 10.1186/gb-2008-9-9-r137
Zhu, L. J., Gazin, C., Lawson, N. D., Pagès, H., Lin, S. M., Lapointe, D. S., et al. (2010). ChIPpeakAnno: a bioconductor package to annotate ChIP-seq and ChIP-chip data. BMC Bioinform. 11:237. doi: 10.1186/1471-2105-11-237
Keywords: serotonin, maternal separation, mouse, emotional behavior, DNA methylation, RNA expression
Citation: Weidner MT, Lardenoije R, Eijssen L, Mogavero F, De Groodt LPMT, Popp S, Palme R, Förstner KU, Strekalova T, Steinbusch HWM, Schmitt-Böhrer AG, Glennon JC, Waider J, van den Hove DLA and Lesch K-P (2019) Identification of Cholecystokinin by Genome-Wide Profiling as Potential Mediator of Serotonin-Dependent Behavioral Effects of Maternal Separation in the Amygdala. Front. Neurosci. 13:460. doi: 10.3389/fnins.2019.00460
Received: 27 February 2019; Accepted: 24 April 2019;
Published: 10 May 2019.
Edited by:
Benjamin E. Reese, University of California, Santa Barbara, United StatesReviewed by:
Tod Edward Kippin, University of California, Santa Barbara, United StatesSulev Kõks, University of Tartu, Estonia
Copyright © 2019 Weidner, Lardenoije, Eijssen, Mogavero, De Groodt, Popp, Palme, Förstner, Strekalova, Steinbusch, Schmitt-Böhrer, Glennon, Waider, van den Hove and Lesch. This is an open-access article distributed under the terms of the Creative Commons Attribution License (CC BY). The use, distribution or reproduction in other forums is permitted, provided the original author(s) and the copyright owner(s) are credited and that the original publication in this journal is cited, in accordance with accepted academic practice. No use, distribution or reproduction is permitted which does not comply with these terms.
*Correspondence: Klaus-Peter Lesch, a3BsZXNjaEBtYWlsLnVuaS13dWVyemJ1cmcuZGU=
†These authors have contributed equally to this work