- 1School of Life Sciences and Technology, Xidian University, Xi’an, China
- 2The First Affiliated Hospital, Xi’an Jiaotong University, Xi’an, China
- 3Shanghai Mental Health Center, Shanghai Jiao Tong University, Shanghai, China
Objectives: The frontal-striatum-thalamus pathway is important in the glutamic neural circuit. The hypofunction of GRIN1 and GRIA2 subunits from glutamic receptors has been hypothesized as the primary process in the etiology of schizophrenia. Identified gene polymorphism involved in the pathogenesis of schizophrenia may uncover relevant mechanism pathways.
Methods: We selected two loci of rs11146020 and rs3813296 distributed in GRIN1 and GRIA2 genes and tested their main and interaction effects on causality connections and structural characteristics in the frontal-striatum-thalamus pathway in 55 Han Chinese first-episode negative schizophrenia patients.
Results: We found that: (1) rs11146020 has a significant main effect on the causality connections between the bilateral dorsolateral prefrontal cortex, and rs3813296 mainly influences those of the descending pathway from the prefrontal cortex to the striatum; (2) interaction effect of rs11146020 and rs3813296 on causality connections are located in the ascending pathway from the pallidum to the dorsolateral prefrontal cortex; and (3) the two loci have effects on the volumes of several regions of this pathway.
Conclusion: Our results suggested there is modulation on glutamic frontal-striatum-thalamus pathway by rs11146020 and rs3813296 gene polymorphism. Patients with different genotypes have different neuroimaging characteristics, which indirectly reminded clinicians those patients should receive different clinical interventions.
Introduction
Traditionally, abnormal dopamine has been considered the major underlying cause of schizophrenia (Gründer and Cumming, 2016). However, this conventional hypothesis does not readily elucidate the negative symptoms and cognitive deficits that are often observed in schizophrenia (Yang and Tsai, 2017). Glutamate, an important excitatory neurotransmitter in the brain, is necessary for neuronal growth, maturation, and synaptic plasticity (Garthwaite and Balazs, 1978; Choi, 1988; Derkach et al., 2007; Lau and Zukin, 2007). Previous studies have demonstrated that altered glutamate signaling may provide a better explanation for the pathological basis of schizophrenia (Stahl, 2007). One hypothesis declares that aberrant functioning of glutamatergic synapses leads to an imbalance between excitation and inhibition and, ultimately, to generate changes in the neural circuitry, such as in the frontal-striatum-thalamus pathway which is an important glutamic neural circuit, that drives psychosis and the impairment of cognitive functions (Schwartz et al., 2012).
N-methyl-D-aspartate (NMDA) and α-amino-3-hydroxy-5-methylisoxazole-4-propionic acid (AMPA) are two ionotropic receptors of glutamate that have been proposed as mediators of numerous common neuropsychiatric phenotypes such as cognition deficit, psychosis, and degeneration (Nakanishi et al., 1998). NMDA receptor is crucial for neuronal communication and the formation of tetrameric complexes of its was encoded by seven homologous subunits genes (Traynelis et al., 2010; Zhu et al., 2016). Although all are good candidate genes for the pathogenesis of schizophrenia, GRIN1 gene gets special attention, which codes NMDA receptor subunit 1 (NR1). Reducing expression of NR1 in mice gives rise to behavioral anomalies which is similar to those observed in pharmacologically induced animal models of schizophrenia (Mohn et al., 1999). rs11146020 is located in the 5′ untranslated region (UTR) in the GRIN1 gene, which may influence gene expression by affecting transcription, stability of mRNA, and translation efficiency (Meijer and Thomas, 2002; Wilkie et al., 2003). Liu et al. (2019) have illustrated that single nucleotide polymorphism (SNP) of rs11146020 in the promoter region of the GRIN1 gene are associated with schizophrenia in a Chinese Han population.
α-amino-3-hydroxy-5-methylisoxazole-4-propionic acid receptor is mainly located in excitatory synapses, where it mediates the most of fast synaptic transmission and participates in synaptic plasticity (Huganir and Nicoll, 2013). There are four AMPA receptor subunits assembled into functional homo- and hetero-tetrameric receptor complexes (Keinanen et al., 1990). Four genes (GRIA1-4) encode these receptor subunits which are expressed in several brain regions, such as the nucleus accumbens, striatum and prefrontal cortex (Reimers et al., 2011). Among the four receptor subunits, the Ca2+ permeability of AMPA receptors is dependent on the encoding of GRIA2 gene and AMPA receptors not containing the GRIA2 subunit are Ca2+ impermeable, increasing neuronal vulnerability to excitotoxicity, which results in neuropsychiatric symptoms (Isaac et al., 2007). GRIA2 is expressed on pyramidal cells and GABAergic interneurons, the cellular source of the expression difference would have a substantial effect on its physiological consequences (Lake et al., 2016). Moreover, The association of genotype rs3813296 T/T in the GRIA2 gene with a low efficacy of antipsychotics against negative symptoms was demonstrated when studying the association of polymorphisms of GRIA2 encoding a number of subunits of AMPA (Gareeva, 2018).
A previous study concluded that a series of glutamic neurons that begin in the prefrontal lobe connect and project into brainstem, midbrain, and limbic system (Schwartz et al., 2012). By this means, neurons originating in the prefrontal cortex may penetrate into deeper brain areas to control over midbrain neurons that are primarily in charge of creating and projecting neurotransmitter activities that are eventually responsible for drive and affective initiation. These deeper brain areas, such as the striatum and thalamus, play an important role on creating appropriate perceptual balance versus psychosis (Marx et al., 2015).
More importantly, twin and family studies indicated that genetic factors contributed substantially to the possibility of developing schizophrenia (Ripke et al., 2014). The extant data suggested that schizophrenia involved complex interactions between multiple genes, each exerting relatively small effects on vulnerability. Several SNPs have been associated with increased risk for developing schizophrenia, although few of these findings have been replicated (Jagannath et al., 2018). If confirmed in additional studies, these genetic markers would implicate glutamatergic neurotransmitter pathways in the pathogenesis of schizophrenia (Dauvermann et al., 2017; Saini et al., 2017; Han et al., 2018).
Taken together, the modulation relationship between the SNP variants in specific genes and glutamic neural circuits remains a challenge to understand. If we obtained the association relationship between them, it could potentially help clinicians regulate intervention strategies for those patients with some genotypes. Based on this challenge, in the present study, we selected an important glutamic neural circuit, the frontal-striatum-thalamus pathway, and two SNPs in GRIN1 and GRIA2 genes to explore their effects on the causality connections and structural characteristics of this neural pathway and then investigated the correlation between the causality connection strength and clinical cognitive behavioral scores. The hypothesis is that there is a modulation on glutamic pathway of frontal-striatum-thalamus by rs11146020 and rs3813296 gene polymorphism in first-episode negative schizophrenia.
Materials and Methods
Participant Selection
We selected fifty-five first-episode negative schizophrenic patients from the Shanghai Mental Health Center. All patients met the inclusion criteria as follows: (1) they were first-episode and had no medication history; (2) they were diagnosed as schizophrenia by senior clinical psychiatrists using a structural clinical interview from the DSM-IV-TR (patient edition); (3) they did not present severe agitation or aggression; and (4) they were 18–45 years old and right-handed.
Behavioral Measurement Scales
The patients were assessed using scales for the assessment of negative symptoms (SANS), duration of untreated psychosis (DUP), and education years (EDU). Intravenous peripheral blood of each patient was drawn for the extraction genotype. More importantly, the internationally recognized consensus version of cognitive function tests for schizophrenia were measured, including the trail making test (TMT); brief assessment of cognition in schizophrenia-symbol coding (BACS-SC); verbal fluency (VF); continuous performance test-identical pairs (CPT-IP); Wechsler memory scale, third edition: spatial span (WMS-III SS); Hopkins verbal learning test, revised (HVLT-R); brief visuospatial memory test, revised (BVMT-R); neuropsychological assessment battery, mazes (NAB-M); and Mayer-Salovey-Caruso emotional intelligence test, managing emotions (MSCEIT-ME). All tests were confirmed by three experienced psychiatrists who underwent consistency training for approximately 1 week, and all scores were assessed objectively. For more detailed information, please see Table 1.
Genotyping
Peripheral blood was drawn from a participant’s vein into a sterile tube containing EDTA. We stored the plasma samples at −80°C. Genomic DNA was isolated from peripheral blood leukocytes according to the manufacturer’s protocol (Thermo Fisher Scientific, United States). DNA was also stored at −80°C for SNP analysis. Genotyping was performed for all SNPs by SnaPshot using a 3730xl DNA Analyzer (Thermo Fisher Scientific, United States).
Single nucleotide polymorphism rs11146020 from the GRIN1 gene and rs3813296 from the GRIA2 gene were genotyped in all patients by allele-specific polymerase chain reaction primers. The success rate of the genotyping in our study was 100%. Information from the Hardy-Weinberg equilibrium (HWE) and minor allele frequency (MAF) are shown in Table 1. In addition, we have uploaded the SNP data to a publicly available repository1. The link to the SNP data is https://www.synapse.org/#!Synapse:syn21788916/tables/.
There were two genotypes for rs11146020 among the 55 schizophrenia patients: CG (23 patients) and GG (32 patients). Analogously, there were three genotypes for rs3813296: GT (24 patients), TT (29 patients), and GG (2 patients). Because only two participants were the carriers of genotype GG, we did not select these participants. To further investigate the interaction effect of rs11146020 and rs3813296, we divided the patients into four subgroups (14 GG/GT, 17 GG/TT, 10 CG/GT, and 12 CG/TT) for follow-up analysis.
Data Acquisition
All MRI images were scanned using a 3T Siemens Magnetom Verio Syngo MR B17 scanner. Participants were informed to keep their eyes closed, not to focus their thoughts on anything and stay awake.
The parameters of functional MRI data are as follows: echo time [TE] = 30 ms, repetition time [TR] = 3 s, flip angle [FA] = 90°, slice thickness = 3.0 mm, slices = 45, field of view [FOV] = 220 mm × 220 mm, matrix size = 64 × 64, voxel size = 3 mm × 3 mm × 3 mm and 170 slices.
Structural MRI data were obtained with a high-resolution T1-weighted magnetization-prepared rapid gradient echo (MPRAGE) sequence. The parameters used are as follows: TE = 2.56 ms, TR = 2530 ms, FA = 7°, FOV = 256 mm × 256 mm, matrix = 256 × 256, slice thickness = 1 mm, inversion time = 1100 ms, and 192 coronal slices.
Data Preprocessing
T1-Weighted Data Preprocessing
We performed T1-weighted data processing with the FSL-VBM protocol with the FMRIB Software Library 4.1 (FSL2). More detailed processing, please see our recent study (Cai et al., 2017a). There were four steps: brain extraction; the segmentation of white matter, gray matter and cerebrospinal fluid; image registration to the standard template; and image smoothing with a Gaussian kernel with 8 mm.
We segmented white matter and gray matter and divided the images into four subgroups based on different genotypes (GG/GT, GG/TT, CG/GT, and CG/TT) for follow-up statistical analysis.
fMRI Data Preprocessing
fMRI data processing was performed using a MATLAB toolbox called DPABI (Yan et al., 2016), which evolved from REST (Song et al., 2011), and DPARSF (Chao-Gan and Yu-Feng, 2010). For more details on fMRI data processing, please see our previous study (Cai et al., 2015). There were eight steps: discarding the first ten time points, slice timing correction, correcting for head motion (exclusion criteria: exceeding 1.5 mm in any dimension of x, y, and z or 1.5° in any angular motion; two participants were removed), normalizing to individual T1-weighted anatomical images, smoothing images, removing linear trends, filtering (0.01–0.1 Hz) and regressing the covariates (A Friston-24 parameter, the global mean signal, cerebrospinal fluid signal, and white matter signal were the nuisance covariates) (Friston et al., 1996; Yan et al., 2013). We also examined if there are any differences in head motion among groups as described in the study of Power et al. (2012).
Data Processing and Statistical Analysis
Frontal-Striatum-Thalamus Pathway and Core Regions Selection
At a molecular level, glutamate neurotransmitter release and synaptic discharge circuit are mainly from the frontal lobe, and go through the striatum to the basal ganglia (Carlsson et al., 1999; Schwartz et al., 2012; Figures 1A,B). Based on these studies, we concluded and focused on one of the glutamic neurotransmission pathways: the frontal-striatum-thalamus pathway (Figure 1) and selected 10 core brain regions in this pathway: left and right dorsolateral prefrontal cortex (L/R. dLPFC), L/R. caudate, L/R. putamen, L/R. pallidum, and L/R. thalamus (Schwartz et al., 2012). We extracted these brain regions using an anatomical automatic labeling (AAL) template implemented with REST (Song et al., 2011). It should be noted that dLPFC is a functional definition, thus we used dorsolateral superior frontal gyrus to represent this region based on the AAL template. Ten brain regions were resampled to the spatial resolution of fMRI images.
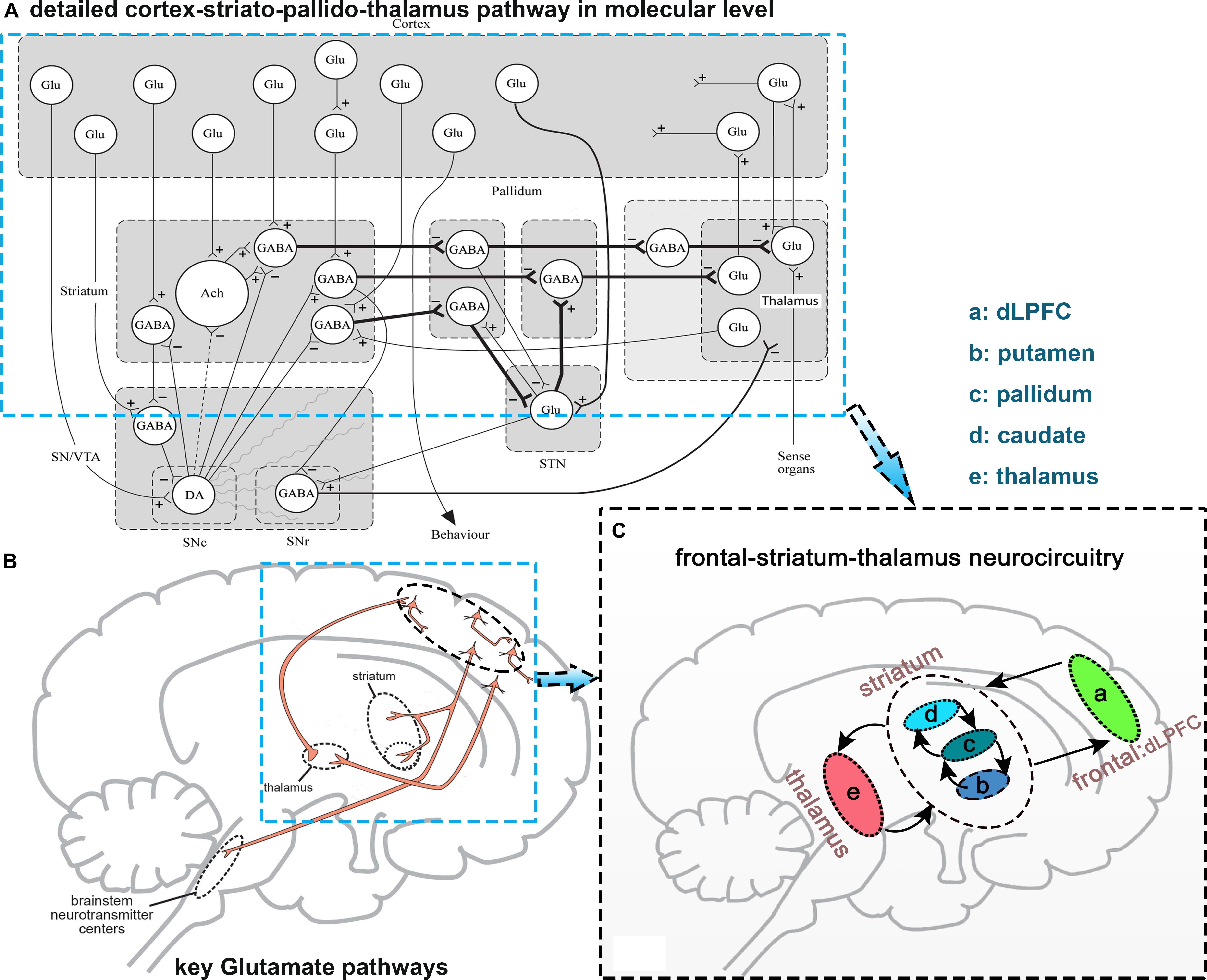
Figure 1. Diagram explaining the selection of the frontal-striatum-thalamus pathway. (A) Detailed cortex-striato-pallido-thalamus pathway at the molecular level, this figure is from Carlsson et al. (1999); (B) several key glutamic pathways in synaptic release level, this figure from Schwartz et al. (2012); (C) selected frontal-striatum-thalamus pathway. Abbreviations: Glu, Glutamate; DA, dopamine; GABA, gamma aminobutyric acid.
Granger Causality Analysis
Granger causality analysis (GCA) is an approach used to explore the dynamic causal relationship between two time series (Granger, 1969). A brief introduction of the Granger procedure is provided here. For two given fMRI time series x(t) and y(t), x(t) is the Granger causing y(t) if the past information of x(t) can improve the prediction of the current value of y(t). The Granger causal relationship between the two series is often estimated by vector autoregressive (VAR) modeling. Granger causality can evaluate the direct linear influence from x(t) to y(t) (FXtoY) and the linear direct influence from y(t) to x(t) (FYtoX). Formula (1) is the mathematic model of GCA:
where zj(t) represents up to q exogenous processes (six orthogonal motion estimates and physiological noise) independent of the path network (j = 1,…., q). Contributions of each lagged variable to the prediction of its respective target are denoted by α; β corresponds to the covariate effect, and prediction errors of individual models are denoted by ε. A similar method was used in one of our previous studies (Cai et al., 2017b).
We applied bivariate coefficient-based GCA to compute the causality of the 10 brain regions for each participant. The average time courses of each region were input to the GCA using REST (Song et al., 2011). Then, we obtained the path coefficients characterized by the direction and the strength of the temporal relation among the 10 brain regions.
Main and Interaction Effect Analysis of rs11146020 and rs3813296
To understand the main and interaction effects of rs11146020 and rs3813296 on the frontal-striatum-thalamus pathway, we performed multivariable general linear model (GLM) analysis with gender, EDU, and age as regressors using IBM SPSS Statistical 23. There are two main effect analyses of rs11146020 and rs3813296 and one interaction effect analysis of rs11146020∗rs3813296 in 90 () causality connections. If there are significant interactions, simple effect analysis was conducted with a script embedded into IBM SPSS Statistical 23. We applied the Mann–Whitney U test to test significance and false discovery rate (FDR) to correct the multiple comparisons (P < 0.01).
Gray and White Matter Structural Analysis
To investigate the differences between four subgroups (GG/GT, GG/TT, CG/GT, and CG/TT) in the gray and white matter structure, we applied two-sample t-tests to assess structural alterations in gray and white matter with gender, ages and EDU as regressors. The statistical images were corrected by FDR for multiple comparisons correction (P < 0.01).
Correlation Analysis Between Connection Strength and Behavioral Scores
The Spearman test for correlation was applied to investigate the correlation between connection strength, which showed significant group difference, and clinical scales, including TMT, BACS-SC, VF, CPT-IP, WMS-III SS, HVLT-R, BVMT-R, NAB-M, and MSCEIT-ME. The significance levels were set at P < 0.01 (two-tailed, FDR correction).
Results
No Significant Subgroup Difference in Demographic Information and Clinical Scores
No significant difference were found in gender, age, gender, head motion, EDU, DUP, SANS, TMT, BACS-SC, VF, CPT-IP, WMS-III SS, HVLT-R, BVMT-R, NAB-M, and MSCEIT-ME among the four subgroups (P > 0.05). There is no difference in head motion among groups. All demographic information and clinical scores are shown in Table 1.
Main and Interaction Effects on Causality Connectivity in the Frontal- Striatum-Thalamus Pathway
Rs11146020 mainly affects the causality connectivity within the dLPFC: L. dLPFC → R. dLPFC and L. dLPFC → R. dLPFC (Figure 2A and Table 2). Rs3813296 mainly affects the causality connectivity of the descending pathway from the dorsolateral prefrontal cortex to thalamus and striatum: L. dLPFC → R. caudate and R. dLPFC → R. thalamus (Figure 2B and Table 2).
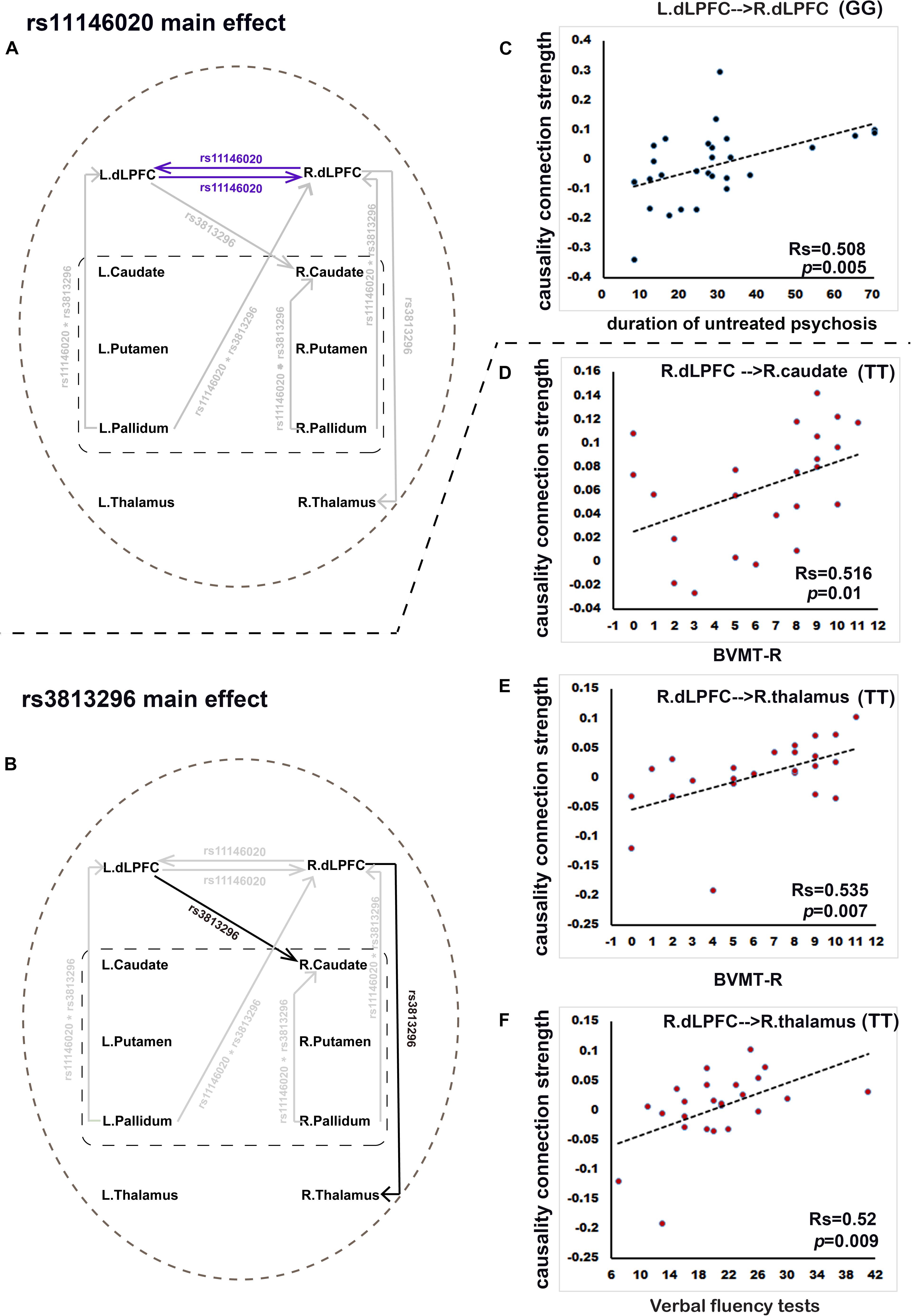
Figure 2. Rs11146020 and rs3813296 main effects on causality connections and relationships with behavioral variables (P < 0.01, FDR correction). (A) rs11146020 and (B) rs3813296 main effects on causality connections; (C) causality connection strength of L. dLPFC → R. dLPFC has significant positive correlation with duration of untreated psychosis in patients with the GG genotype; (D) causality connection strength of R. dLPFC → R. caudate has a significant positive correlation with BVMT scores in patients with the TT genotype; causality connection strength of R. dLPFC → R. thalamus has a significant positive correlation with BVMT-R scores (E) and verbal fluency test scores (F) in patients with the TT genotype. Abbreviations: dLPFC, dorsolateral prefrontal cortex; BVMT-R, brief visuospatial memory test, revised.
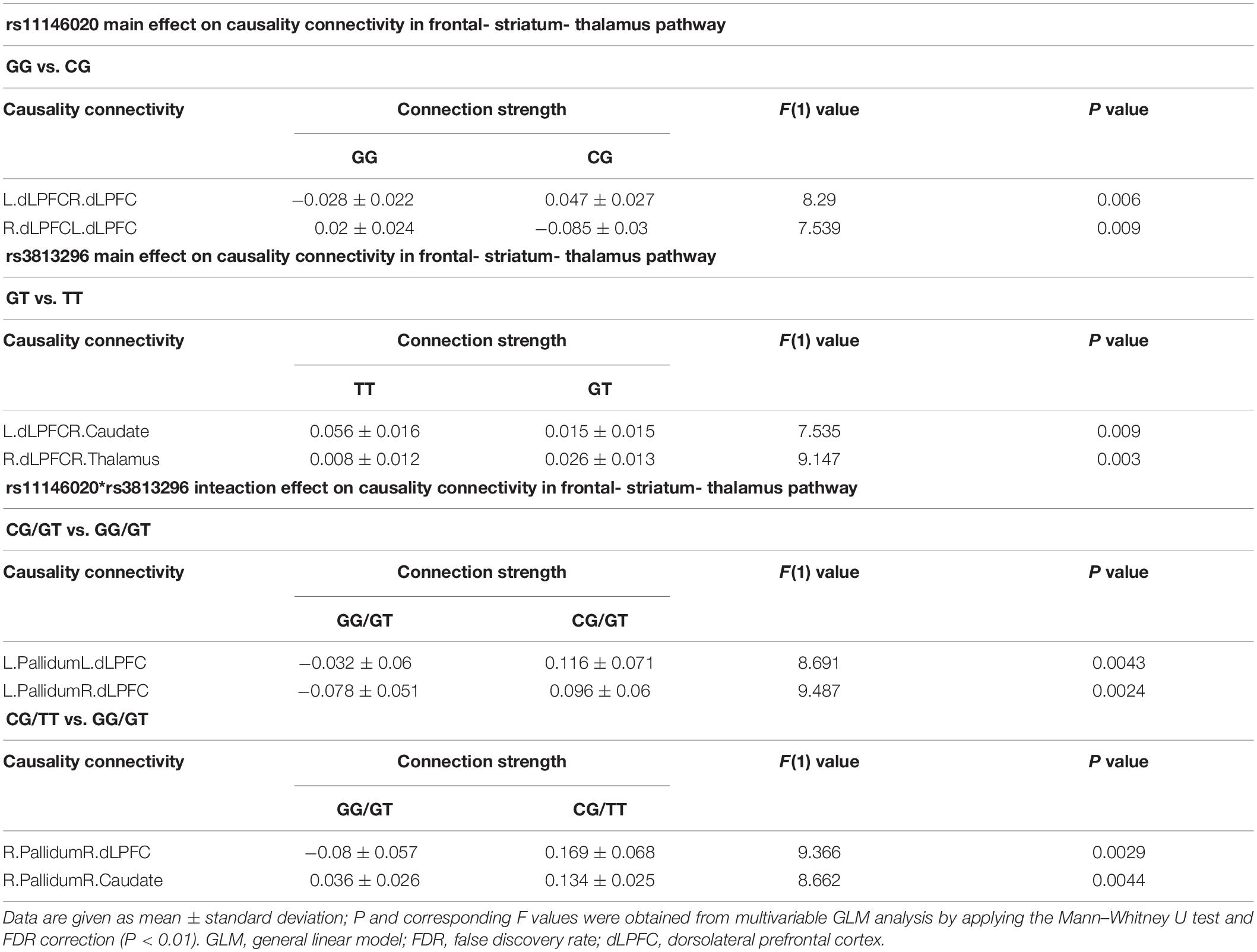
Table 2. Main and interaction effects of rs11146020 and rs3813296 on causality connectivity in frontal-striatum-thalamus pathway.
Rs11146020 and rs3813296 interactively affect the information flow of the upstream pathway from striatum to dLPFC: L. pallidum → L. dLPFC, L. pallidum → R. dLPFC, R. pallidum → R. dLPFC, and R. pallidum → R. caudate (Figure 3A and Table 2).
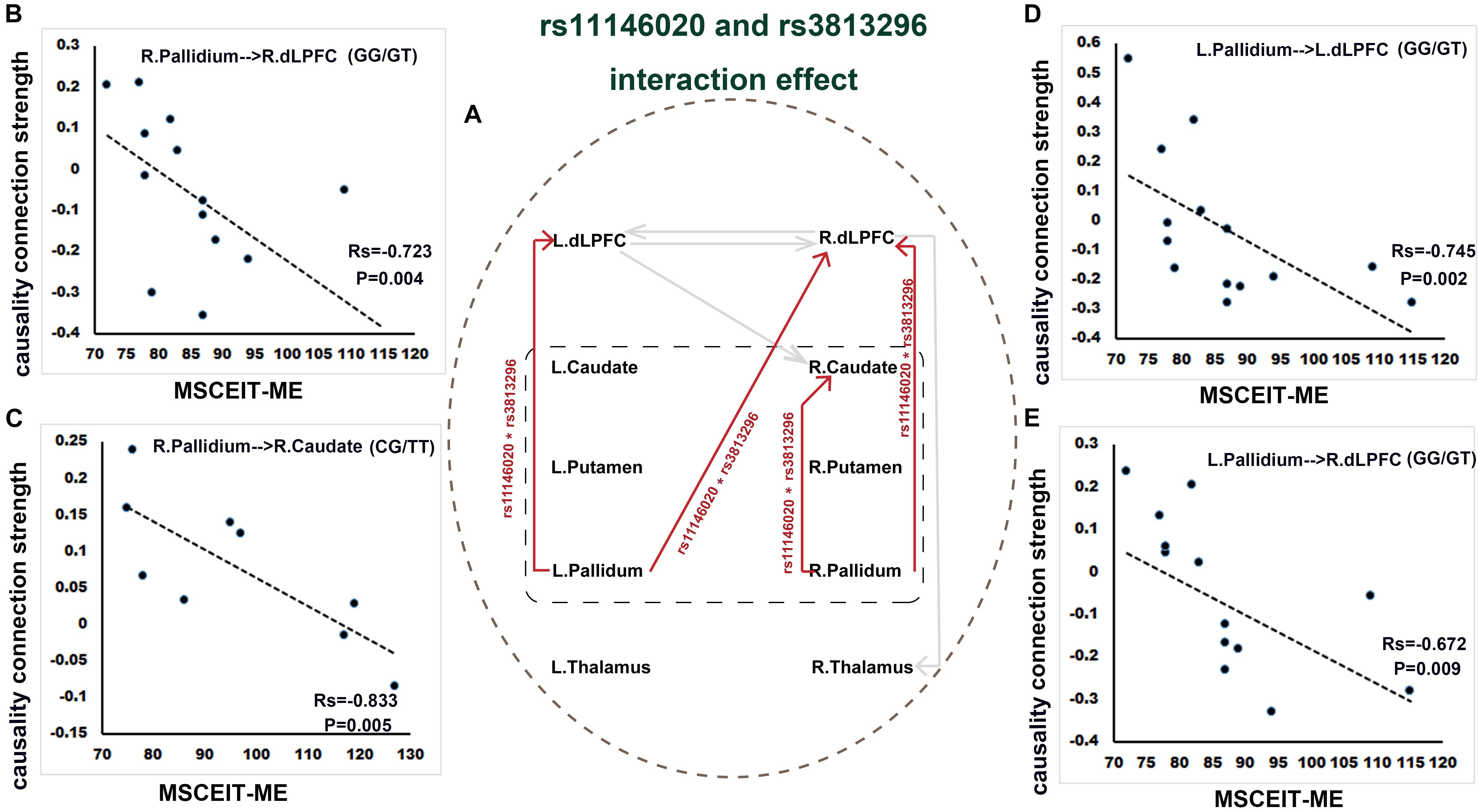
Figure 3. Interaction effect of rs11146020 and rs3813296 on causality connections and relationship with behavioral variables (P < 0.01, FDR correction). (A) interaction effect of rs11146020 and rs3813296 on causality connections; causality connection strength of R. pallidum → R. dLPFC (B), L. pallidum → L. dLPFC (D) and L. pallidum → R. dLPFC (E) has a significant negative correlation with MSCEIT-ME in patients with the GG/GT genotype; causality connection strength of R. pallidum → R. caudate (C) has a significant negative correlation with MSCEIT-ME in patients with the CG/TT genotype; Abbreviations: dLPFC, dorsolateral prefrontal cortex; MSCEIT-ME, Mayer-Salovey-Caruso emotional intelligence test (managing emotions).
Main and Interaction Effects on Gray and White Matter Structures
There is no main effect of rs11146020 on gray and white matter volumes and no main effect of rs3813296 on gray matter volumes. Main effect of rs3813296 on white matter were located in left/right superior corona radiata fiber (Figure 4B and Table 3) (P < 0.01, FDR correction). The interaction effect of rs3813296∗rs11146020 on gray and white matter volumes were located in the left/right putamen, left/right caudate, left/right thalamus (Figure 4A and Table 3) and left/right superior corona radiata fiber (Figure 4C and Table 3), respectively (P < 0.01, FDR correction).
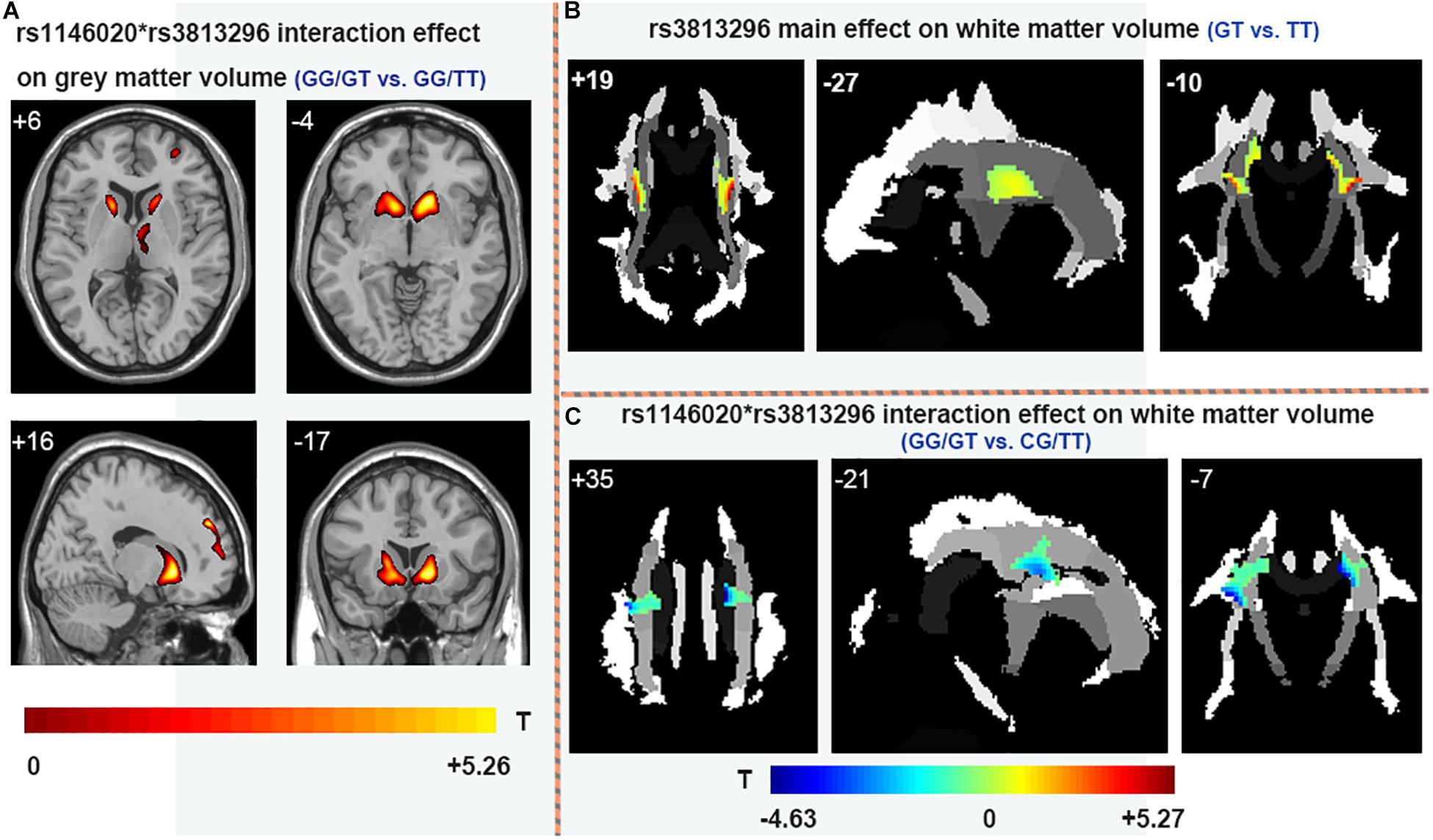
Figure 4. Main and interaction effects of rs11146020 and rs3813296 on gray and white matter (P < 0.01, FDR correction). (A) rs11146020 and rs3813296 interaction effect on gray matter volume (GG/GT vs. GG/TT); (B) rs3813296 main effect on white matter volume (GT vs. TT); (C) rs11146020 and rs3813296 interaction effect on white matter volume (GG/GT vs. CG/TT).
Significant Correlation Between Causality Connection Strength and Behavioral Scales
We found that the strength of causality connection L. dLPFC → R. dLPFC has a significant positive correlation with DUP (Figure 2C and Table 4); the connection strengths of R. dLPFC → R. caudate and R. dLPFC → R. thalamus have significant positive correlations with BVMT-R scores (Figures 2D,E and Table 4); and the connection strength of R. dLPFC → R. thalamus has a significant positive correlation with VF test scores (Figure 2F and Table 4). More interestingly, four ascending causality connections interactively effected by rs11146020 and rs3813296 were all significant negative correlation with MSCEIT-ME scores (Figures 3B–E and Table 4).
Discussion
The hypo-function of GRIN1 and GRIA2 subunits from glutamic receptors has been hypothesized as a primary process in the pathophysiology of schizophrenia. Identified gene polymorphism involved in the etiology of schizophrenia may reveal relevant mechanistic pathways. Whether the polymorphisms in the subunit genes of GRIN1 and GRIA2 receptors contribute to the risk of schizophrenia is still in question. In our study, we selected two SNPs distributed in GRIN1 and GRIA2 genes and tested their effects on the causality connections and structural characteristics of the frontal-striatum-thalamus pathway in Han Chinese schizophrenia patients. There were three major findings: (1) rs11146020 has a significant main effect on the causality connections between the left and right dLPFC and rs3813296 mainly influences the descending pathway from the prefrontal lobe to the striatum; (2) the interaction effect of rs11146020 and rs3813296 is mainly located in the ascending pathway from the bilateral pallidum to the right caudate and the bilateral dLPFC; and (3) the two SNPs have main and interaction effects on the volumes of gray and white matter in several regions of this pathway. Some causality connection strengths affected by the two SNPs have remarkable correlation with clinical cognitive performances in VF, visuospatial memory and emotion management. The detailed explanation is as follows.
Main Effect of rs11146020 on Causality Connectivity in Frontal-Striatum-Thalamus Pathway
Rs11146020 has a significant main effect on the causality connections between the left and right dLPFC. The ancestral and variant alleles of rs11146020 are G and C, respectively. There is a significant difference between homozygous GG and heterozygous GC in schizophrenia patients, indicating that genotype influences functional connections. Zhao et al. (2006) found that the C allele was expressed in a high frequency in schizophrenia patients. rs11146020 is located in the GRIN1 gene, which is a subunit of NMDAR. The gene responsible for its expression is located at 9q34 in the promoter region (Begni et al., 2003). The GRIN1 gene product plays a foundational role in many brain functions, and its involvement in the pathogenesis of schizophrenia has been widely investigated (Hung et al., 2002; Zhao et al., 2006). Moreover, GRIN1 knockout animals showed abnormal behavior characteristics which were commonly similar with patients with schizophrenia, such as impairment of working memory, reduced “self-care” (nest building) and social activity (Tatard-Leitman et al., 2015). Gray et al. (2015) reported higher expression levels of the majority of glutamatergic genes, especially GRIN1, were detected in the dLPFC. The rs11146020 genotype has a significant association with the causality connection between the left and right dLPFC, which is opportunely proved the suggestion by Zhao et al. (2006) that rs11146020 is a potential candidate to alter the risk of schizophrenia and worth further replication and functional investigation.
Interestingly, causality connection between the left and right dLPFC in schizophrenia patients with ancestral genotype GG has a significant positive correlation with DUP but was not found in GC carriers. For patients who carried the GG genotype, Increased connection strength could lead to protracted DUP in patients who carried the GG genotype. Research shows that there is a close relationship between longer DUP and poorer outcomes in first-episode psychosis (Rubio and Correll, 2017). Hence, enhanced connectivity between the left and right dLPFC is not an optimistic phenomenon, especially in patients with the GG genotype.
In terms of brain structure, we did not find a remarkable difference in the volumes of gray and white matter between GG and GC genotypes, suggesting that the association between genotype and function was unrelated to cortex and subcortex sizes. This is possibly because the mutation from allele G to C is not enough to change brain volume.
Main Effect of rs3813296 on Causality Connectivity in the Frontal-Striatum-Thalamus Pathway
Rs3813296 mainly influences the causality connections of the descending pathway from the prefrontal lobe to the striatum, including L. dLPFC → R. caudate and R. dLPFC → R. thalamus. The connection strength of L. dLPFC → R. caudate in schizophrenia patients with the GT genotype is significantly lower than those with the TT genotype. Rs3813296 is located in the GRIA2 gene which is one subunit of the AMPA receptor (Lu et al., 2009). The Ca2+ permeability of AMPA receptors rely on the GRIA2 subunit and AMPA receptors without the GRIA2 subunit are Ca2+ impermeable, which increases the neuronal vulnerability to excitotoxicity and can result in neuropsychiatric symptoms (Isaac et al., 2007). The lower connection strength of L. dLPFC → R. caudate in patients with the GT genotype may be due to a deficit in Ca2+ permeability from cortex to subcortex. Based on the current result regarding to rs3813296, deficit Ca2+ permeability should be associated with the variation of T to G. However, this variation has significant relevance to the enhanced connection strength of R. dLPFC → R. thalamus, which could be interpreted as compensation phenomenon.
A literature study returned two studies containing rs3813296 (Crisafulli et al., 2012; Iamjan et al., 2018). Few studies are similar with our study; thus, we tried to search for supporting results from the current dataset and found that the white matter volume of the superior corona radiata in schizophrenia patients with the GT genotype is significantly larger than those with the TT genotype. Corona radiata are the most prominent projection fibers, and they are afferents that carry information to the cerebral cortex and efferent that carry information away from it (Morecraft et al., 2002). Gray matter of the striatum and white matter of the corona radiata are the main components of the basal ganglia. The difference of the white matter volume of the superior corona radiata between GT and TT genotypes explains the lower connection strength of L. dLPFC → R. caudate in patients with the GT genotype: inflated volume of the superior corona radiata indirectly causes dispersive connection strength between the two regions.
Causality connection strengths of L. dLPFC → R. caudate and R. dLPFC → R. thalamus in patients with the TT genotype have a significant positive correlation with scores in VF and brief visuospatial memory tests. The functional anatomy of VF has been well characterized in normal participants using positron emission tomography (PET) (Spence et al., 1998). The study also demonstrated that generating words beginning with a given letter activates the dLPFC. Another study suggested that the thalamus is involved in the encoding of verbal material and that thalamic damage impairs verbal recall (Ruggeri, 2016). Benson et al. (2008) hypothesized that cognitive impairments could be related to the dysfunction of the physiological metabolic activity between the dLPFC and subcortical regions. In particular, hypoactivation of the dLPFC observed in schizophrenia patients could result in hyperactivation of subcortical structures, such as the striatum and thalamus (Groerewegen, 1991). Thus, the significant correlation suggested that VF and brief visuospatial memory tests scores have important clinical significance. As suggested in previous studies, it is also closely associated with the degeneration of the brain in schizophrenia patients (Zhang et al., 2012, 2014; Cetin-Karayumak et al., 2019). Further, recent research mentioned that words and visuospatial memory information are conveyed across aforesaid regions via the excitatory projections of glutamatergic pyramidal neurons (Hoftman et al., 2017; Nikolova et al., 2017). More importantly, the current results are especially helpful for reminding clinical researchers to pay attention to the first-episode negative schizophrenia patients with the TT genotype. These patients are vulnerable to VF and visual spatial memory. In the long run, patients with the TT genotype have different characteristic and should be treated using different clinical interventions.
Interaction Effect of rs11146020∗rs3813296 on Causality Connectivity in the Frontal-Striatum-Thalamus Pathway
The interaction effects of rs11146020∗rs3813296 on the causality connection are mainly located in the ascending pathway from the bilateral pallidum to the right caudate and bilateral dLPFC. After the interaction effect of the two SNPs, simple effect tests showed that modulation by rs11146020 on the causality connection of L. pallidum → R. dLPFC and L. pallidum → R. dLPFC is influenced by the GT genotype in rs3813296. Similarly, modulation by rs3813296 on the causality connection of R. pallidum → R. caudate is influenced by the GG genotype in rs11146020. Moreover, the interaction effect of the two SNP is also on the gray and white matter volumes of several regions in this pathway, such as parts of the caudate, thalamus, putamen and fiber of the superior corona radiata. Taken together, these results imply that the effect of two SNPs on brain structure and function is greater than that of a single SNP. It is consistent with a comment provided by previous research that suggested that the risk of common diseases is potentially determined by the complex interaction between genetic factors, including SNPs (Lohmueller et al., 2003; Mechanic et al., 2008).
Moreover, four causality connections, among those mentioned, have a significant negative correlation with MSCEIT-ME scores. To understand the negative correlation between them, we investigated the literature and examined gamma aminobutyric acid (GABA) interneurons, which are purported to ultimately inhibit the generation of excessive mesolimbic dopamine activity (Stahl, 2007). Disturbances in GABA neurotransmission could represent a common pathophysiology for different domains of cortical dysfunction in schizophrenia (Hashimoto et al., 2008). For example, if the GABA interneuron was dysfunctional, it would lead to excessive suppression and produce decreasing activity. In the current study, the stronger the connectivity strength of the ascending pathway from the bilateral pallidum to the bilateral dLPFC, the stronger the inhibition projecting onto the dLPFC, leading to restrained glutamate release. Buzsáki and Draguhn (2004) demonstrated that the regulation or stabilization of GABA interneurons is critical for the coordination of cortical-mediated behaviors. The dLPFC is activated in emotion tasks and is a main region in managing emotion control (Aupperle et al., 2012). Thus, decreased release of glutamate excitatory neurotransmitter in dLPFC indirectly results in weak emotion management ability.
In addition, gray matter volumes of parts of bilateral caudate, thalamus and putamen in patients with the GG/GT genotype are larger than those with the GG/TT genotype. It was suggested that the T allele in rs3813296 was indirectly associated with inflated volume in these regions. In white matter, the volume of the superior corona radiata fiber in patients with the GG/GT genotype is lower than those with the CG/TT genotype. As mentioned above, the white matter of the corona radiata is the main joint component of the basal ganglia and striatum. Combining the two results regarding gray and white matter, we explained the opposite phenomenon (larger vs. lower) as increscent gray matter volumes (caudate, thalamus, and putamen) extruded contiguous white matter (superior corona radiata fiber) in patients with the GG/GT genotype. This interpretation is inspired by Alliey-Rodriguez et al. (2017).
Until now, we noticed that dLPFC is a key region in the frontal-striatum-thalamus pathway and has the strongest association with other regions. In particular, the causality connections of dLPFC exhibit a significant relationship with clinical behaviors. The current results verified that dLPFC is an important region in the treatment or research of schizophrenia (Olagunju et al., 2017; Schneider et al., 2017).
Limitations
Two limitations of this study should be considered. First, there was a relatively small sample size in the four subgroups. Currently, gene and neuroimaging data are being collected, and we will replicate and verify these results using a larger sample in the near future. Additionally, the lack of knowledge about the relationships between the region-specific variation in glutamic neurotransmission and temporal patterns of GRIN1 and GRIA2 expression is a common concern to studies of neuroimaging genetics.
Conclusion
We investigated the main and interaction effects of rs11146020 and rs3813296 on causality connections and structural characteristics in the frontal-striatum-thalamus pathway in Han Chinese patients with schizophrenia. Significant association was found between them, and causality connection strengths affected by two SNPs were remarkably correlated with clinical cognitive performance. Our results suggested that patients with different genotypes have different characteristics, and those patients should receive different clinical interventions.
Data Availability Statement
The datasets generated in this study is publicly available on the SNP dataset repository: https://www.synapse.org/#!Synapse:syn21788916/tables/, with the following accession no: syn21788916.
Ethics Statement
The studies involving human participants were reviewed and approved by the Shanghai Mental Health Center Ethics Committee (Serial number: 2012-45). The participants or their legal guardian, provided their written informed consent to participate in this study.
Author Contributions
SC and YL collected the data. KH and WZ analyzed the data and performed the measurements. SC wrote the manuscript. LH and JW had the major responsibility for preparing the manuscript. QW revised the manuscript.
Funding
This work was supported by the National Natural Science Foundation of China (grant nos. 81671778, 81801789, and 81671332); China Postdoctoral Science Foundation (grant no. 2017M623128); National Natural Science Foundation of Shaanxi Province of China (grant no. 2020JM-212); and the Fundamental Research Funds for the Central Universities (grant no. XJS201203).
Conflict of Interest
The authors declare that the research was conducted in the absence of any commercial or financial relationships that could be construed as a potential conflict of interest.
Footnotes
References
Alliey-Rodriguez, N., Grey, T. A., Shafee, R., Padmanabhan, J., Tandon, N., Klinger, M., et al. (2017). Common variants of NRXN1, LRP1B and RORA are associated with increased ventricular volumes in psychosis-GWAS findings from the B-SNIP deep phenotyping study. bioRxiv. [preprint]. doi: 10.1101/175489
Aupperle, R. L., Allard, C. B., Grimes, E. M., Simmons, A. N., Flagan, T., Behrooznia, M., et al. (2012). Dorsolateral prefrontal cortex activation during emotional anticipation and neuropsychological performance in posttraumatic stress disorder. Arch. Gen. Psychiatry 69, 360–371.
Begni, S., Moraschi, S., Bignotti, S., Fumagalli, F., Rillosi, L., Perez, J., et al. (2003). Association between the G1001C polymorphism in the GRIN1 gene promoter region and schizophrenia. Biol. Psychiatry 53, 617–619. doi: 10.1016/s0006-3223(02)01783-3
Benson, B. E., Willis, M. W., Ketter, T. A., Speer, A., Kimbrell, T. A., George, M. S., et al. (2008). Interregional cerebral metabolic associativity during a continuous performance task (Part II): differential alterations in bipolar and unipolar disorders. Psychiatry Res. Neuroimaging 164, 30–47. doi: 10.1016/j.pscychresns.2007.12.016
Buzsáki, G., and Draguhn, A. (2004). Neuronal oscillations in cortical networks. Science 304, 1926–1929. doi: 10.1126/science.1099745
Cai, S., Chong, T., Zhang, Y., Li, J., Von Deneen, K. M., Ren, J., et al. (2015). Altered functional connectivity of fusiform gyrus in subjects with amnestic mild cognitive impairment: a resting-state fMRI study. Front. Hum. Neurosci. 9:471. doi: 10.3389/fnhum.2015.00471
Cai, S., Jiang, Y., Wang, Y., Wu, X., Ren, J., Lee, M. S., et al. (2017a). Modulation on brain gray matter activity and white matter integrity by APOE ε4 risk gene in cognitively intact elderly: a multimodal neuroimaging study. Behav. Brain Res. 322, 100–109. doi: 10.1016/j.bbr.2017.01.027
Cai, S., Peng, Y., Chong, T., Zhang, Y. M., Von Deneen, K., Huang, L., et al. (2017b). Differentiated effective connectivity patterns of the executive control network in progressive MCI: a potential biomarker for predicting AD. Curr. Alzheimer Res. 14, 937–950.
Carlsson, A., Waters, N., and Carlsson, M. L. (1999). Neurotransmitter interactions in schizophrenia—therapeutic implications. Biol. Psychiatry 46, 1388–1395. doi: 10.1016/s0006-3223(99)00117-1
Cetin-Karayumak, S., Di Biase, M. A., Chunga, N., Reid, B., Somes, N., Lyall, A. E., et al. (2019). White matter abnormalities across the lifespan of schizophrenia: a harmonized multi-site diffusion MRI study. Mol. Psychiatry.
Chao-Gan, Y., and Yu-Feng, Z. (2010). DPARSF: a MATLAB toolbox for “pipeline” data analysis of resting-state fMRI. Fron. Syst. Neurosci. 4:13. doi: 10.3389/fnsys.2010.00013
Choi, D. W. (1988). Glutamate neurotoxicity and diseases of the nervous system. Neuron 1, 623–634. doi: 10.1016/0896-6273(88)90162-6
Crisafulli, C., Chiesa, A., De, R. D., Han, C., Lee, S. J., Park, M. H., et al. (2012). Influence of GRIA1, GRIA2 and GRIA4 polymorphisms on diagnosis and response to antipsychotic treatment in patients with schizophrenia. Neurosci. Lett. 506, 170–174. doi: 10.1016/j.neulet.2011.10.074
Dauvermann, M. R., Lee, G., and Dawson, N. (2017). Glutamatergic regulation of cognition and functional brain connectivity: insights from pharmacological, genetic and translational schizophrenia research. Br. J. Pharmacol. 174, 3136–3160. doi: 10.1111/bph.13919
Derkach, V. A., Oh, M. C., Guire, E. S., and Soderling, T. R. (2007). Regulatory mechanisms of AMPA receptors in synaptic plasticity. Nat. Rev. Neurosci. 8, 101. doi: 10.1038/nrn2055
Friston, K. J., Williams, S., Howard, R., Frackowiak, R. S., and Turner, R. (1996). Movement-related effects in fMRI time-series. Magn. Reson. Med. 35, 346–355. doi: 10.1002/mrm.1910350312
Gareeva, A. (2018). Peculiarities of the effect of antipsychotics: pharmacogenetic studies. Hum. Physiol. 44, 706–719. doi: 10.1134/s0362119718050031
Garthwaite, J., and Balazs, R. (1978). Supersensitivity to the cyclic GMP response to glutamate during cerebellar maturation. Nature 275, 328–329. doi: 10.1038/275328a0
Granger, C. W. (1969). Investigating causal relations by econometric models and cross-spectral methods. Econometrica 37, 424–438.
Gray, A., Hyde, T., Deep-Soboslay, A., Kleinman, J., and Sodhi, M. (2015). Sex differences in glutamate receptor gene expression in major depression and suicide. Mol. Psychiatry 20, 1057–1068. doi: 10.1038/mp.2015.91
Groerewegen, H. (1991). “Functional anatomy of the ventral, limbic system-innervated striatum,” in The Mesolimbic Dopaminec System: From Motivation to Action, eds P. Willner and J. Scheel-Krüger (Hoboken, NJ: Wiley), 19–59.
Gründer, G., and Cumming, P. (2016). “The dopamine hypothesis of schizophrenia: Current status,” in The Neurobiology of Schizophrenia, eds T. Abel and T. Nickl-Jockschat (Amsterdam: Elsevier), 109–124.
Han, S., An, Z., Luo, X., Zhang, L., Zhong, X., Du, W., et al. (2018). Association between CMYA5 gene polymorphisms and risk of schizophrenia in U ygur population and a meta-analysis. Early Interv. Psychiatry 12, 15–21. doi: 10.1111/eip.12276
Hashimoto, T., Bazmi, H. H., Mirnics, K., Wu, Q., Sampson, A. R., and Lewis, D. A. (2008). Conserved regional patterns of GABA-related transcript expression in the neocortex of subjects with schizophrenia. Am. J. Psychiatry 165, 479–489. doi: 10.1176/appi.ajp.2007.07081223
Hoftman, G. D., Dienel, S. J., Bazmi, H. H., Zhang, Y., Chen, K., and Lewis, D. A. (2017). Altered gradients of glutamate and gamma-aminobutyric acid transcripts in the cortical visuospatial working memory network in schizophrenia. Biol. Psychiatry 83, 670–679. doi: 10.1016/j.biopsych.2017.11.029
Huganir, R. L., and Nicoll, R. A. (2013). AMPARs and synaptic plasticity: the last 25 years. Neuron 80, 704–717. doi: 10.1016/j.neuron.2013.10.025
Hung, C.-C., Chen, H.-Y., and Chen, C.-H. (2002). Systematic mutation analysis of the human glutamate receptor, ionotropic, N-methyl-D-aspartate 1 gene (GRIN1) in schizophrenic patients. Psychiatr. Genet. 12, 225–230. doi: 10.1097/00041444-200212000-00005
Iamjan, S. A., Thanoi, S., Watiktinkorn, P., Reynolds, G. P., and Nudmamudthanoi, S. (2018). Genetic variation of GRIA3 gene is associated with vulnerability to methamphetamine dependence and its associated psychosis. J. Psychopharmacol. 32, 309–315. doi: 10.1177/0269881117750153
Isaac, J. T., Ashby, M. C., and Mcbain, C. J. (2007). The role of the GluR2 subunit in AMPA receptor function and synaptic plasticity. Neuron 54, 859–871. doi: 10.1016/j.neuron.2007.06.001
Jagannath, V., Gerstenberg, M., Correll, C. U., Walitza, S., and Grünblatt, E. (2018). A systematic meta-analysis of the association of neuregulin 1 (NRG1), D-amino acid oxidase (DAO), and DAO activator (DAOA)/G72 polymorphisms with schizophrenia. J. Neural. Transm. 125, 89–102. doi: 10.1007/s00702-017-1782-z
Keinanen, K., Wisden, W., Sommer, B., Werner, P., Herb, A., Verdoorn, T. A., et al. (1990). A family of AMPA-selective glutamate receptors. Science 249, 556–560. doi: 10.1126/science.2166337
Lake, B. B., Ai, R., Kaeser, G. E., Salathia, N. S., Yung, Y. C., Liu, R., et al. (2016). Neuronal subtypes and diversity revealed by single-nucleus RNA sequencing of the human brain. Science 352, 1586–1590. doi: 10.1126/science.aaf1204
Lau, C. G., and Zukin, R. S. (2007). NMDA receptor trafficking in synaptic plasticity and neuropsychiatric disorders. Nat. Rev. Neurosci. 8, 413–426. doi: 10.1038/nrn2153
Liu, Y.-P., Ding, M., Zhang, X.-C., Liu, Y., Xuan, J.-F., Xing, J.-X., et al. (2019). Association between polymorphisms in the GRIN1 gene 5’ regulatory region and schizophrenia in a northern Han Chinese population and haplotype effects on protein expression in vitro. BMC Med. Genet. 20:26. doi: 10.1186/s12881-019-0757-3
Lohmueller, K. E., Pearce, C. L., Pike, M., Lander, E. S., and Hirschhorn, J. N. (2003). Meta-analysis of genetic association studies supports a contribution of common variants to susceptibility to common disease. Nat. Genet. 33, 177–182. doi: 10.1038/ng1071
Lu, W., Shi, Y., Jackson, A. C., Bjorgan, K., During, M. J., Sprengel, R., et al. (2009). Subunit composition of synaptic AMPA receptors revealed by a single-cell genetic approach. Neuron 62, 254–268. doi: 10.1016/j.neuron.2009.02.027
Marx, M. C., Billups, D., and Billups, B. (2015). Maintaining the presynaptic glutamate supply for excitatory neurotransmission. J. Neurosci. Res. 93, 1031–1044. doi: 10.1002/jnr.23561
Mechanic, L. E., Luke, B. T., Goodman, J. E., Chanock, S. J., and Harris, C. C. (2008). Polymorphism Interaction Analysis (PIA): a method for investigating complex gene-gene interactions. BMC Bioinform. 9:146. doi: 10.1186/1471-2105-9-146
Meijer, H. A., and Thomas, A. A. (2002). Control of eukaryotic protein synthesis by upstream open reading frames in the 5’-untranslated region of an mRNA. Biochem. J. 367, 1–11. doi: 10.1042/bj20011706
Mohn, A. R., Gainetdinov, R. R., Caron, M. G., and Koller, B. H. (1999). Mice with reduced NMDA receptor expression display behaviors related to schizophrenia. Cell 98, 427–436. doi: 10.1016/s0092-8674(00)81972-8
Morecraft, R. J., Herrick, J. L., Stilwell-Morecraft, K. S., Louie, J. L., Schroeder, C. M., Ottenbacher, J. G., et al. (2002). Localization of arm representation in the corona radiata and internal capsule in the non-human primate. Brain 125, 176–198. doi: 10.1093/brain/awf011
Nakanishi, S., Nakajima, Y., Masu, M., Ueda, Y., Nakahara, K., Watanabe, D., et al. (1998). Glutamate receptors: brain function and signal transduction1. Brain Res. Rev. 26, 230–235. doi: 10.1016/s0165-0173(97)00033-7
Nikolova, S., Stark, S. M., and Cel, S. (2017). 3T hippocampal glutamate-glutamine complex reflects verbal memory decline in aging. Neurobiol. Aging 54, 103–111. doi: 10.1016/j.neurobiolaging.2017.01.026
Olagunju, A., Clark, S., and Baune, B. (2017). Transcranial magnetic stimulation of the dorsolateral prefrontal cortex: a review of methods and utility in psychiatry. Biol. Psychiatry 81:S132.
Power, J. D., Barnes, K. A., Snyder, A. Z., Schlaggar, B. L., and Petersen, S. E. (2012). Spurious but systematic correlations in functional connectivity MRI networks arise from subject motion. Neuroimage 59, 2142–2154. doi: 10.1016/j.neuroimage.2011.10.018
Reimers, J. M., Milovanovic, M., and Wolf, M. E. (2011). Quantitative analysis of AMPA receptor subunit composition in addiction-related brain regions. Brain Res. 1367, 223. doi: 10.1016/j.brainres.2010.10.016
Ripke, S., Neale, B. M., Corvin, A., Walters, J. T., Farh, K.-H., Holmans, P. A., et al. (2014). Biological insights from 108 schizophrenia-associated genetic loci. Nature 511, 421–427. doi: 10.1038/nature13595
Rubio, J. M., and Correll, C. U. (2017). Duration and relevance of untreated psychiatric disorders, 2: nonpsychotic psychiatric disorders and substance use disorders. J. Clin. Psychiatry 78, 464–465. doi: 10.4088/jcp.17ac11544
Ruggeri, M. (2016). Left dorsomedial thalamic damage impairs verbal recall more than recognition: a case report. Cogn. Behav. Neurol. 29, 158–165. doi: 10.1097/wnn.0000000000000103
Saini, S. M., Mancuso, S., Mostaid, M. S., Liu, C., Pantelis, C., Everall, I., et al. (2017). Meta-analysis supports GWAS-implicated link between GRM3 and schizophrenia risk. Transl. Psychiatry 7:e1196. doi: 10.1038/tp.2017.172
Schneider, M., Walter, H., Moessnang, C., Schã¤Fer, A., Erk, S., Mohnke, S., et al. (2017). Altered DLPFC-Hippocampus connectivity during working memory: independent replication and disorder specificity of a putative genetic risk phenotype for schizophrenia. Schizophr. Bull. 43, 1114–1122. doi: 10.1093/schbul/sbx001
Schwartz, T. L., Sachdeva, S., and Stahl, S. M. (2012). Glutamate neurocircuitry: theoretical underpinnings in schizophrenia. Front. Pharmacol. 3:195. doi: 10.3389/fphar.2012.00195
Song, X.-W., Dong, Z.-Y., Long, X.-Y., Li, S.-F., Zuo, X.-N., Zhu, C.-Z., et al. (2011). REST: a toolkit for resting-state functional magnetic resonance imaging data processing. PLoS One 6:e25031. doi: 10.1371/journal.pone.0025031
Spence, S. A., Hirsch, S. R., Brooks, D. J., and Grasby, P. M. (1998). Prefrontal cortex activity in people with schizophrenia and control subjects: evidence from positron emission tomography for remission of ‘hypofrontality’with recovery from acute schizophrenia. Br. J. Psychiatry 172, 316–323. doi: 10.1192/bjp.172.4.316
Stahl, S. M. (2007). Beyond the dopamine hypothesis to the NMDA glutamate receptor hypofunction hypothesis of schizophrenia. CNS Spectr. 12, 265–268. doi: 10.1017/s1092852900021015
Tatard-Leitman, V. M., Jutzeler, C. R., Suh, J., Saunders, J. A., Billingslea, E. N., Morita, S., et al. (2015). Pyramidal cell selective ablation of N-methyl-D-aspartate receptor 1 causes increase in cellular and network excitability. Biol. Psychiatry 77, 556–568. doi: 10.1016/j.biopsych.2014.06.026
Traynelis, S. F., Wollmuth, L. P., Mcbain, C. J., Menniti, F. S., Vance, K. M., Ogden, K. K., et al. (2010). Glutamate receptor ion channels: structure, regulation, and function. Pharmacol. Rev. 62, 405–496.
Wilkie, G. S., Dickson, K. S., and Gray, N. K. (2003). Regulation of mRNA translation by 5’-and 3’-UTR-binding factors. Trends Biochem. Sci. 28, 182–188. doi: 10.1016/s0968-0004(03)00051-3
Yan, C. G., Cheung, B., Kelly, C., Colcombe, S., Craddock, R. C., Di Martino, A., et al. (2013). A comprehensive assessment of regional variation in the impact of head micromovements on functional connectomics. Neuroimage 76, 183–201. doi: 10.1016/j.neuroimage.2013.03.004
Yan, C.-G., Wang, X.-D., Zuo, X.-N., and Zang, Y.-F. (2016). DPABI: data processing & analysis for (Resting-State) brain imaging. Neuroinformatics 14, 339–351. doi: 10.1007/s12021-016-9299-4
Yang, A. C., and Tsai, S.-J. (2017). New targets for schizophrenia treatment beyond the dopamine hypothesis. Int. J. Mol. Sci. 18:1689. doi: 10.3390/ijms18081689
Zhang, Y., Lin, L., Lin, C. P., Zhou, Y., Chou, K. H., Lo, C. Y., et al. (2012). Abnormal topological organization of structural brain networks in schizophrenia. Schizophr. Res. 141, 109–118. doi: 10.1016/j.schres.2012.08.021
Zhang, Y., Su, T. P., Liu, B., Zhou, Y., Chou, K. H., Lo, C. Y., et al. (2014). Disrupted thalamo-cortical connectivity in schizophrenia: a morphometric correlation analysis. Schizophr. Res. 153, 129–135. doi: 10.1016/j.schres.2014.01.023
Zhao, X., Li, H., Shi, Y., Tang, R., Chen, W., Liu, J., et al. (2006). Significant association between the genetic variations in the 5’ end of the N-methyl-D-aspartate receptor subunit gene GRIN1 and schizophrenia. Biol. Psychiatry 59, 747–753. doi: 10.1016/j.biopsych.2005.10.023
Keywords: single nucleotide polymorphism, magnetic resonance imaging, glutamic pathway, causality connection, schizophrenia
Citation: Cai S, Lv Y, Huang K, Zhang W, Wang Q, Huang L and Wang J (2020) Modulation on Glutamic Pathway of Frontal-Striatum-Thalamus by rs11146020 and rs3813296 Gene Polymorphism in First-Episode Negative Schizophrenia. Front. Neurosci. 14:351. doi: 10.3389/fnins.2020.00351
Received: 03 September 2019; Accepted: 23 March 2020;
Published: 21 April 2020.
Edited by:
Wei Wu, South China University of Technology, ChinaReviewed by:
Yuanchao Zhang, University of Electronic Science and Technology of China, ChinaDandan Zhang, Shenzhen University, China
Copyright © 2020 Cai, Lv, Huang, Zhang, Wang, Huang and Wang. This is an open-access article distributed under the terms of the Creative Commons Attribution License (CC BY). The use, distribution or reproduction in other forums is permitted, provided the original author(s) and the copyright owner(s) are credited and that the original publication in this journal is cited, in accordance with accepted academic practice. No use, distribution or reproduction is permitted which does not comply with these terms.
*Correspondence: Liyu Huang, huangly@mail.xidian.edu.cn; Jijun Wang, jijunwang27@163.com
†ORCID: Suping Cai orcid.org/0000-0001-8605-0874 Liyu Huang orcid.org/0000-0001-6534-2712